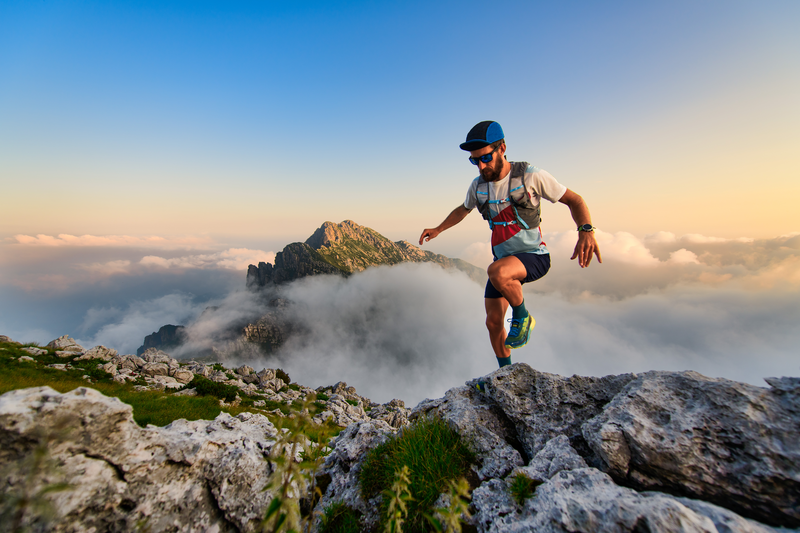
94% of researchers rate our articles as excellent or good
Learn more about the work of our research integrity team to safeguard the quality of each article we publish.
Find out more
REVIEW article
Front. Pediatr. , 29 July 2020
Sec. Pediatric Gastroenterology, Hepatology and Nutrition
Volume 8 - 2020 | https://doi.org/10.3389/fped.2020.00408
Humans and their gut microbiota have co-evolved over thousands of years, resulting in the establishment of a complex host–microbiota ecosystem. Early life environmental factors, such as delivery mode, nutrition, and medication use, have been shown to substantially affect both host–microbiota interactions and health outcomes. However, the effects of urbanization (characterized by the spectrum of rural and urban populations) on these early life events have been overlooked. A deeper understanding of the relationship between urbanization and microbiota development will allow for the identification of novel biological and social approaches that can be implemented to prevent and treat disease and promote maternal and infant/child health. The aim of this narrative review is to summarize how factors associated with urbanization differentially impact delivery mode, nutrition, and medication use, and how these changes subsequently affect the gut microbiota and health outcomes of infants. This narrative review also describes the important evidence gaps associated with these relationships and recommends actions that can be taken to improve the health of mothers and infants worldwide.
Of the many relationships that humans experience in their lifetime, the relationship between human host and gut microbiota is possibly one of the most important. This relationship has developed over thousands of years, allowing for the establishment of longstanding and essential bi-directional host–microbiota interactions. Host–microbiota interactions and functions influence nutrient metabolism, vitamin synthesis, mucosal maturation, immune-system development, and biochemical signaling between the gut and the brain (1–4). Accordingly, various human diseases, including immune-related diseases, chronic metabolic diseases, and neurodevelopmental diseases, have been associated with disruptions to the gut microbiota composition and function. Combined, these interactions and associations provide evidence for the important influence of the gut microbiota on human health outcomes.
One important player in this complex host–microbiota relationship is the external environment. Multiple, often interrelated, environmental factors influence both the human gut microbiota and health outcomes. Humans are most susceptible to environmental factors during early life, and their effects on human health can be evident even in later-life. The first 1,000 days of life is a crucial period for the rapid overall growth and development of a child (5), and it is estimated that over 1 million new neural connections are formed every second in the first few years of life (6). In parallel with infant growth and development, the infant gut microbiota is also being established. During the first few months of life the infant gut microbiota is highly dynamic, which is essential for its maturation and for the stimulation of the immune system. Delivery mode, nutrition, and medication use are well-studied environmental factors known to influence the infant gut microbiota and to contribute to early and later health outcomes (7–10). Other factors, such as stress and home environment (e.g., number of siblings, pets), can also play a role (11–13). However, how urbanization may influence these early life relationships has been overlooked [Box 1; (16, 17)].
Box 1. Urbanization: definition and its association with income group.
According to the United Nations (UN) Population Division, urbanization can be characterized by the transition from a rural to an urban population. This transition is associated with “explosive growth of cities, and shifts from an agriculture-based economy to mass industry, technology and services” (14). Based on the 2018 Revision of World Urbanization Prospects (produced by the UN), 55% of the world's population lives in urban areas. In 2018, regions exhibiting high percentages of urbanization included Northern America (82%), Latin America and the Caribbean (81%), Europe (74%), and Oceania (68%). Africa, on the other hand, was considered rural, with only 43% of the population living in urban areas (14). These findings demonstrate the general relationship between urbanization and geographical location. Urbanization gradients are also closely linked with income-group (as determined by the World Bank) (14, 15). Most high-income countries, such as Australia, Canada, Japan, the United States of America, and most European countries, exhibit relatively high levels of urbanization (14).
Urban and rural populations can be defined by the different social structures and associated environmental factors that they are exposed to. For example urban and rural populations can differ by demographics, governance, medical interventions, nutrition, hygiene and sanitation, medication use, and cultural beliefs (Box 2). Accordingly, these differences could differentially impact the factors known to shape the infant gut microbiota and related health outcomes. In fact, research in adult populations has already demonstrated that urban diets, increased sanitation and antibiotic exposure contribute to what has been termed “microbiota insufficiency syndrome” (“the loss of microbial taxa and associated [microbial] functions that were part of our evolutionary past”) (4, 18). Adult microbiota composition can even differ across urbanization gradients within the same country (19). Combined, these factors have also been associated with an increase in immune and metabolic diseases in urban adult populations (4).
Box 2. Differences between urban and rural settings.
The aim of this narrative review is to summarize the factors associated with urbanization that differentially impact the well-studied factors of delivery mode, nutrition and medication use, which in turn impact the infant gut microbiota and health outcomes in early and later life (Figure 1; Supplementary Table 1). Next, this narrative review highlights the most important evidence gaps associated with these well-studied factors, such as the relationships with malnutrition in all its forms. This understanding provides insights for the potential use of biological applications (e.g., modification of the gut microbiota by diet) and the need for standardization of certain mother–child health policies across urbanization gradients, which will contribute to efforts to improve the health outcomes for mothers and their infants/children worldwide.
Figure 1. The aim of this narrative review is to describe the relationships between factors associated with urbanization and three well-studied factors—delivery mode, nutrition, and medication use—known to influence the infant gut microbiota and short- and long-term health outcomes. This figure was created using images from Servier Medical Art Commons Attribution 3.0 Unported License (http://smart.servier.com). Servier Medical Art by Servier is licensed under a Creative Commons Attribution 3.0 Unported License.
An infant's first encounter with microbes is currently still debated. While some studies provide evidence for in utero colonization due to the presence of low abundant microbes in the placenta and amniotic fluid (20–22), others have argued that contamination from sampling and DNA extraction kits cannot be confidently dismissed (23, 24). Regardless, differences between the gut microbiota of infants born by vaginal delivery or cesarean section (C-section) are already evident very early in life. Initially, infants born by vaginal delivery were thought to be exposed to and colonized by the resident microbes of the lower genital tract of the mother, with Lactobacillus species being the most dominant (25, 26). However, two recent studies of the neonate gut microbiota showed that the prevalence and abundance of Lactobacillus species were similar in both vaginally delivered and C-section groups (27, 28). Instead, Bifidobacterium, Escherichia, Bacteroides, and Parabacteroides species (Table 1) were enriched in the gut microbiota of vaginally delivered infants, and were possibly inherited from maternal fecal microbes (27, 28). These findings complement those from other studies that demonstrated that maternal gut microbes can be transmitted to infants via vaginal delivery (35, 36). Transmission and colonization of the maternal gut microbiota in infants delivered vaginally is thought to have protective and advantageous functions, as these microbiota are suited to the environment of the gut and have the capacity to contribute to nutrient acquisition in early life (28).
Table 1. The influence of delivery mode, nutrition, and medication use on the infant gut microbiota.
In contrast to vaginally delivered infants, infants delivered by C-section are initially colonized by microbes present in the delivery environment, in the maternal mouth and on the maternal skin [Table 1; (3, 7, 8, 25)]. Infants delivered by C-section have a relatively low initial abundance of Bacteroidetes (due to inefficient colonization of the gut) and a higher relative abundance of opportunistic pathogens in comparison to vaginally delivered infants (27–29). The effects of C-section delivery on the gut microbiota have been shown to impact the immune and metabolic development of an infant (7, 37, 38). Indeed, increased childhood immune and metabolic diseases have been associated with C-section delivery (38). C-section delivery has also been shown to influence breast milk composition, as mothers who deliver by elective C-section do not release hormones usually associated with labor (39, 40). This suggests a possible role for delivery mode in early life nutrition. Lastly, some infants delivered by C-section are exposed to antibiotics at birth, depending on placental clamping procedures used during prophylactic antibiotic administration (41, 42); the effects of antibiotics on the gut microbiota and health outcomes of infants are covered in the “Medication Use, the Gut Microbiota, and Health Outcomes” section of this review.
Although C-section deliveries are an important medical intervention for high-risk pregnancies globally, they are generally over-utilized in low risk pregnancies, particularly in urban and concurrent middle– and high–income countries [Boxes 1, 2; (43)]. According to a WHO report, in 2008, an estimated 6.2 million of the C-section deliveries performed worldwide were unnecessary, with some of the highest C-section rates belonging to China, Brazil and the USA (44).
Maternal socioeconomic status, healthcare infrastructure (i.e., the accessibility, availability, and affordability of high quality healthcare), type of healthcare facility (i.e., public or private) and physician practices are all factors associated with urbanization that contribute to C-section overuse [Figure 2; (45–47)]. In Brazil, for example the incidence of C-section deliveries was shown to be 1.6 times higher in private facilities compared to public facilities, even though maternal preference was for vaginal delivery (45). Moreover, the effects of physician's practices on C-section overuse can be related to several concerns. This can include lower risks of accusations of malpractice in the event of C-section delivery complications, or time and infrastructure concerns, as C-section deliveries can be “scheduled,” thereby allowing for the time and infrastructure to perform more deliveries (48).
Figure 2. The effects of urbanization on the three well-studied factors, delivery mode, nutrition, and medication use known to influence the infant gut microbiota and short- and long-term health outcomes. TCM, traditional and complementary medication. This figure was created using images from Servier Medical Art Commons Attribution 3.0 Unported License (http://smart.servier.com). Servier Medical Art by Servier is licensed under a Creative Commons Attribution 3.0 Unported License.
Early life nutrition is a well-studied factor that profoundly impacts the infant gut microbiota and is vital for the overall growth and development of a child (5, 49, 50). Feeding modes, such as breastfeeding, formula feeding, mixed feeding, and later weaning (~6 months) have different effects on the gut microbiota and on child health outcomes.
Breastfed infants are exposed to various microbial communities and biological components present on the mother's skin and in breast milk [Table 1; (7, 31, 51)]. These factors are essential for the development of the infant gut microbiota and immune system. This is mainly because the biological components present in breast milk include immune factors (such as antibodies, cytokines, and leukocytes), growth factors and human milk oligosaccharides (HMOs) (52–54). HMOs, in particular, are exclusively found in breast milk and have several beneficial properties. HMOs can: (1) act as metabolic substrates for the growth of beneficial commensal gut bacteria (e.g., Bifidobacterium), as HMOs are indigestible by infants, (2) protect against pathogens and toxins by directly binding to pathogens or inhibiting binding to host cell receptors, and (3) contribute to the development of the infant brain (52, 54, 55). Other breast milk components, such as the hormone leptin, are involved in infant appetite regulation, and long-chain polyunsaturated fatty acids facilitate brain development and are protective against type-2 diabetes (T2D) (56).
In comparison to breast milk, formula milk is generally higher in protein content, does not contain leptin, and often does not contain long-chain polyunsaturated fatty acids (56, 57). Some new generation formulas are supplemented with microbiota such as Bifidobacterium and Lactobacillus species (58). However, due to a lack of data, these supplementations show varying effects (58). Regardless, in general, formula-fed infants show increased gut permeability and bacterial loads, especially of pro-inflammatory taxa of the gut microbiota [Table 1; (57)]. In addition, formula-fed infants exhibit adult-like gut microbiota patterns earlier in life than breastfed infants (7).
Accordingly, breastfeeding is positively associated with child health outcomes. In early life, breastfed infants are less susceptible to infections (such as necrotizing enterocolitis) and diarrhea (56, 59). Later in life, breastfed infants have lower adolescent obesity and T2D risks and better cognitive skills (7, 56, 57, 59–61). In addition, mother–infant bonding during breastfeeding is considered to positively impact infant cognition (56). However, early cessation of breastfeeding, either via the initiation of formula feeding or the premature introduction of solid foods, has been associated with childhood obesity, and facilities the transition of the infant gut microbiota toward an adult-like pattern (5, 7, 62).
Although the implications of breastfeeding and formula feeding have been extensively studied, little information exists on mixed-feeding practices. While this is surprising considering that the majority of infants from middle– and high–income countries are mixed-fed by 3 months of age (57), it is also expected given that the term “mixed-feeding” is not well-defined. Mixed feeding could refer to infants who receive a combination of breast milk and formula milk, or to infants who are initially exclusively breastfed and then switched to formula milk. Only by strictly defining the term “mixed-feeding” can we begin to establish the impacts of early “combined” or “switched” feeding methods on both the gut microbiota and the health outcomes of infants (57).
Following breast-, formula-, or mixed-feeding, infants and children should have adequate access to high quality and diverse foods for optimal growth and development (59). However, when maternal and child nutrition is poor, mothers and their infants can be burdened with malnutrition. Historically, malnutrition was defined by overweight (including obesity) and undernutrition (including stunting and wasting), but a recent series by UNICEF added a third factor termed “hidden hunger” to this definition (59). Hidden hunger refers to micronutrient deficiencies (i.e., lack of vitamins and other essential nutrients) in the diet, which can also contribute to negative health outcomes (59). The effects of malnutrition on the gut microbiota and health outcomes of infants, together with its association to urbanization is described in the “The Effects of Urbanization on Maternal and Child Malnutrition” section of this review.
Nutrition education, cultural beliefs, workforce obligations, and breastfeeding stigmatization are factors associated with urbanization that contribute to the early cessation of breastfeeding, and these factors are differentially observed across urbanization gradients (Figure 2).
Lack of nutrition education differentially affects mothers residing in (and raised in) urban and rural communities. In urban settings, mothers can be inappropriately advised by maternity hospitals to give supplemental infant formula to their infants (57). A survey by the CDC, for example concluded that most hospitals in the USA “do not conform to international recommendations for best practices in maternity care and interfere with mothers' abilities to breastfeed” (63). Across urbanization gradients, early cessation of breastfeeding can be associated with lack of nutrition education and cultural beliefs. In some lessurban Sub-Saharan African countries, for example, breast milk is believed to be lacking in water. Water, which is often contaminated in these settings, and solid foods native to the region are then substituted for breast milk (59, 64). A similar trend can be observed in countries considered to be more urban. Chinese immigrant mothers living in Australia, for example, tend to feed their babies formula milk in the evening, given the recommendation from their elders that doing so facilitates breast milk production because of maternal rest (65). In addition, prior to the recommended weaning age, these mothers also tend to feed their babies water, honey, and rice porridge under the assumption that doing so provides the infant with additional nutrients (65, 66).
Workforce obligations across urbanization gradients significantly contribute to breastfeeding cessation. While mothers (and in some cases fathers) from most countries in the world are entitled to paid parental leave, there are still some countries, such as the United States, that do not guarantee paid parental leave (OECD Family Database). Therefore, along with the effects of an, on average, 18-week period of paid parental leave (in OECD countries), unpaid parental leave places an additional pressure on mothers to return to work early and possibly stop breastfeeding. Indeed, short maternity leave (<6 weeks) has been shown to negatively affect both initiation and continuation of breastfeeding (62). Moreover, regardless of these policies, in rural settings factors such as poverty, income dependency on work and long working hours cause mothers to return to work soon after infant delivery, making it challenging for them to continue breastfeeding exclusively (59).
Breastfeeding stigmatization also plays a role in breastfeeding cessation (67). Mothers have expressed the challenge and stigmatization they experience related to pumping milk in the work place and in relation to public breastfeeding, although the latter is less evident in rural communities (62, 67, 68).
Lastly, it is important to note that numerous other factors contribute to breastfeeding cessation. These can include infant fussiness, low infant weight, pre-term birth and maternal stress and depression (which has also been shown to affect the gut microbiota and health outcomes of infants); all of which, to some degree, could also be associated with urbanization (12, 62).
Factors associated with urbanization that affect maternal and child nutrition include food environments (i.e., the accessibility, availability, and affordability of healthy foods), food practices (i.e., food preparation and dietary/lifestyle habits), and nutrition education [Figure 2; (59)]. Maternal and child nutrition further impacts maternal breast milk, and maternal and infant gut microbiota, and the health outcomes of infants.
Geographical location is closely linked with urbanization and the factors associated with it (Boxes 1, 2). Geographical location has been shown to differentially impact breast milk lipids and microbiota composition in mothers from Spain, Finland, South Africa, and China (69). Similarly the breast milk metabolites of mothers from Australia, Japan, the USA, Norway, and South Africa have also been shown to differ (70). Breast milk microbiota composition can also differ across urbanization gradients within countries. For example the breast milk microbiota of mothers residing in a rural village in India showed higher microbial alpha diversity and richness compared to that of Indian mothers residing in an urban metropolitan city (71). The breast milk of the rural mothers also had a moderate abundance of bacteria belonging to the Firmicutes phyla, while the breast milk of the urban mothers exhibited a higher abundance of Proteobacteria (which generally comprise of a variety of pathogenic bacteria) (71). Accordingly, infants from different geographical areas across differing urbanization gradients are exposed to different components in breast milk, which, in turn, could affect the gut microbiota and health outcomes of these infants.
Maternal diet during pregnancy, which differs across urbanization gradient, can also impact the gut microbiota and health outcomes of infants. Though studies of these relationships are limited, a recent in vivo mice study has shown that the maternal gut microbiota, in response to different diets during pregnancy, can influence the metabolic outcomes of the offspring (72). This relationship is thought to be mediated by differences in short chain fatty acid production, which bind to receptors in the sympathetic nervous system, adipose tissues, pancreas, and intestine of the embryo (72). Similarly, studies in humans have also demonstrated a relationship between the maternal diet during pregnancy and the infant gut microbiota. First, a study by Lundgren et al. demonstrated that the maternal diet during pregnancy influenced the infant gut microbiota in a delivery mode-dependent manner (73). A second study showed that the maternal diet during pregnancy modulated the maternal gut microbiota, where maternal carbohydrate and protein intake was associated with clusters enriched by Prevotella, and maternal fiber, vegetable, protein, and polyphenol intake was associated with clusters enriched by Ruminococcus. These differences were further associated with the initial establishment of the infant gut microbiota potentially affecting later life BMI (74).
Urbanization, and the factors associated with it, also differentially impacts the diets of children, thereby differentially affecting the gut microbiota and health outcomes of children. For example the diets of children from a rural village in Burkina Faso were found to be low in fat and animal protein, but rich in starch, fiber, and plant polysaccharides, while the diets of urban Italian children were high in animal protein, sugar, starch, and fat, but low in fiber (75). When comparing the gut microbiota of these two groups, the children in Burkina Faso had a significantly higher abundance of Bacteroidetes, but a low abundance of Firmicutes, whereas the opposite was observed for Italian children. Notably, higher ratios of Firmicutes to Bacteroidetes have been associated with a predisposition to obesity (76). It has been shown that an urban diet, especially diets high in fructose and sugar substitutes, can alter the metabolic capacity of the gut microbiota, thereby increasing the risk of obesity and obesity-related diseases [e.g., non-alcoholic fatty liver disease and its more severe form, non-alcoholic steatohepatitis; reviewed in (77, 78)]. In contrast, children in Burkina Faso had significantly more short chain fatty acids than Italian children, and their gut contained a distinct abundance of bacteria (Prevotella and Xylanibacter genus) associated with maximizing energy intake from fibers in response to a polysaccharide-rich diet, thereby possibly protecting them from inflammation and non-infectious colonic diseases.
Similar to maternal and child nutrition, food environments (i.e., the accessibility, availability, and affordability of healthy foods), food practices (i.e., food preparation and dietary/lifestyle habits) and nutrition education [Figure 2; (59)] are factors associated with urbanization that collectively influence maternal and child malnutrition, and the gut microbiota, and health outcomes of infants and children.
Unsurprisingly, malnutrition in all its forms varies across urbanization gradients and associated income groups (Box 1). For example, overweight is more prevalent in urban communities, yet it is also on the rise in low– and middle–income countries. As a result, individuals from low– and middle–income countries, which struggle with undernutrition, experience a double burden of both overweight and undernutrition (59, 79). Hidden hunger, on the other hand, is a global issue that has been associated with different factors. For example hidden hunger in urban areas is associated with easy access to unhealthy food options (e.g., nutrient poor, ultra-processed, high fat/sugar foods), while in rural areas hidden hunger is associated with the community's dependence on food security (characterized by sufficient access to high quality, nutritious, and diverse foods required to meet recommended dietary needs). Food security in rural areas can be further threatened by climate and seasonal changes (59).
Maternal pre-pregnancy overweight increases the risk of childhood overweight, and has been shown to be mediated by the combined relationship of C-section delivery and Firmicutes richness of the infant gut microbiota (80). Maternal overweight can also differentially impact the gut microbiota and health outcomes of infants via breast milk composition. For example breast milk of overweight mothers can differ in comparison to that of normal weight mothers in two ways. First, breast milk of overweight mothers can contain lower levels of transforming growth factor and secretory CD14, which are components that contribute to infant immune system maturation and are initially obtained in low amounts during breastfeeding (81). Second, breast milk of overweight mothers have higher levels of Staphylococci (skin bacteria) and lower levels of Bifidobacteria, which are important primary colonizers of the infant gut (81). These differences have been generally associated with allergies, inflammatory diseases, and metabolic diseases (81–83). Moreover, maternal overweight during pregnancy is also a predictor of childhood obesity and chronic disease, and can be a risk factor for mothers themselves through increased risks of gestational diabetes, pre-eclampsia and obstetric complications (59, 84).
Along with the effects of maternal overweight, childhood overweight can also impact the gut microbiota and health outcomes of children. Similar to the effects of maternal overweight on the breast milk microbiota, the gut microbiota of overweight children show higher numbers of Staphylococcus aureus and lower numbers of Bifidobacteria in comparison to normal weight children (82). Moreover, a study by Payne et al. (85) showed that the metabolic activity of the gut microbiota of obese children was increased in comparison to that of normal weight children. Several negative short- and long-term health outcomes have been associated with childhood obesity, including cardiovascular problems, poor self-esteem, and obesity and diabetes in later life (59).
Of the few studies that have been performed on the relationship between maternal undernutrition and the maternal and infant microbiota, most have been performed in vivo. For example a mouse study by Connor et al. (86) showed no change in bacterial composition and richness in the gut microbiota of caloric-restricted pregnant mice in comparison to healthy controls. This study, however, was limited by its small sample size and because dams were killed prior to the delivery of the pups, which meant that potential changes to breast milk composition (including, microbiota and biological constituents) and the transmission of maternal gut microbiota to offspring could not be studied (86). The importance of this is demonstrated in a study by Charbonneau et al. (87), which showed that sialylated HMOs were significantly less abundant in the breast milk of Malawian mothers of undernourished infants than they were in mothers of healthy infants. Using two in vivo models (in mice and piglets), the authors further demonstrated that offspring's growth outcomes were improved by adding sialylated HMOs to their diet (87). These findings are complemented by studies looking at the effects of seasonality on breast milk composition and child health outcomes in rural communities. In the Gambia, for example, an increase in maternal energy intake during dry seasons (when food is plentiful and disease burden is low) resulted in significantly increased breast milk HMO production and was associated with decreased infant morbidity and improved infant weight- and height-for-age (88). Along with these outcomes, maternal malnutrition has also been associated with prenatal complications, preterm birth, low birth weight and chronic diseases in the later life of the infant (59, 89).
In contrast to maternal undernutrition, the effects of child undernutrition on the infant gut microbiota and health outcomes have been well-studied. In general, Proteobacteria (particularly E. coli) and Streptococcus are increased in the gut microbiota of undernourished children, while Bacteroidetes and anaerobic Firmicutes are decreased [reviewed in (90)]. These changes are associated with the possible inefficient transmission of health-related maternal gut microbiota. Accordingly, childhood undernutrition is quite possibly associated with the combined insult of the presence of highly pathogenic microbes and microbial deficiency in the guts of undernourished children (90). The plausibility of this is suggested by studies performed on children with severe acute malnutrition (SAM) caused by deficient breastfeeding and food and water insecurity (90). For example a longitudinal comparative study of the fecal samples of Malawian twin pairs discordant for SAM demonstrated that the gut microbiota of the twin with SAM was immature in comparison with the gut microbiota of their respective healthy twin control (91). Using gnotobiotic mice, the authors showed that the gastrointestinal tracts of mice containing the SAM microbiota were comprised of higher proportions of Bilophila wadsworthia and Clostridium innocuum in comparison to healthy controls; species that have been associated with inflammatory bowel disease and immune-compromised individuals, respectively (91–95). These findings suggest that these microbes are possibly associated with malnourishment on a more global scale.
In another study, children with SAM were provided with ready-to-use therapeutic food (RUTF; made from peanut paste, sugar, vegetable oil, and milk fortified with vitamins and minerals) and administered broad-spectrum oral antimicrobial agents (96). With this combination, children with SAM exhibited reduced mortality and improved recovery (94–96). These findings suggest that the antibiotic treatment may have targeted potential pathobionts in the gut, thereby facilitating the colonization of beneficial bacteria in accordance with the specific diet of the individual. This is further validated by a study that showed that chronic malnourishment in children (aged 2–5 years) was associated with an overgrowth of oral bacteria in the gut (97).
Aside from the effects of child malnutrition on the infant gut microbiota, child malnutrition in general can also impact the health outcomes of children, and has been associated with infection, death, and poor outcomes regarding growth, cognition, school-readiness, school performance, and earning potential in later life (59).
Given that hidden hunger was only recently described, and is complex to analyze, the specific effects of maternal and child hidden hunger on the microbiota and child health outcomes have not yet been well-studied. It has been noted, however, that maternal hidden hunger during pregnancy is associated with maternal morbidity and mortality, neural tube defects and impaired cognitive development in newborns, preterm births, and low birth weight (59). Child hidden hunger, on the other hand, is associated with poor outcomes regarding growth, development, immunity, tissue development, and health (59).
The effects of medication use on the gut microbiota have been extensively described in adults. It is known that antibiotics and some non-antibiotic drugs (such as proton pump inhibitors) can target the native bacteria of the gut and impair the microbial ecology of the gut, thereby leaving the gut susceptible to colonization by extrinsic commensal bacteria (such as microbes from the mouth) and enteric pathogens (3, 25, 98–100). Changes to gut microbiota composition due to antibiotic drugs can persist well after antibiotic administration, though in some cases the gut microbiota can recover within 1 month (101–103). Medication use also causes intestinal dysbiosis, which renders the gut susceptible to infections, dysregulates metabolism, and immune system homeostasis, and can lead to long-term metabolic effects, such as obesity (104–107). Moreover, continued exposure to both antibiotics and some non-antibiotic drugs can increase the presence of antibiotic-resistant genes in the gut microbiota (3, 25, 99, 105, 108).
Of the studies that have been performed during prenatal and early life, most concern the effects of antibiotic use. Maternal antibiotic use during the prenatal period, for example, has been shown to impact both the maternal gut microbiota and the breast milk microbiota, and is associated with an increased risk of childhood obesity (109, 110). Similarly, studies performed on the effects of antibiotics on children demonstrated that antibiotic use was associated with a less diverse gut microbiota at both the species- and strain-level, with a reduction in Clostridiales and an increase in antibiotic-resistant bacteria [Table 1; (9, 34)]. The negative effects of early life antibiotic use are so evident that even the positive effects of long breastfeeding duration on the infant gut microbiota and childhood BMI is reduced by early life exposure to antibiotics (111).
Medical practices and behaviors, such as antibiotic overuse or misuse and traditional and complementary medication use, have been shown to differ across urbanization gradients and can result in early life medication exposure. Distal factors associated with urbanization that impact medical practices and behaviors include policies and regulatory systems, the medication environment (i.e., accessibility, availability, and affordability of medications) and cultural beliefs (Figure 2).
Globally, and across urbanization gradients, only half of antibiotics are used correctly (112). This, as well as the misuse of antibiotics, can be attributed to unstandardized, and often poor, policies, and regulatory systems (41). An example of the latter is the easy acquisition of antibiotics from local shops or over the internet without prescription in some geographical locations (113, 114). Moreover, this relationship can also be observed in animal husbandry and agriculture (41). In the USA (an urban and high-income country, Box 1), for example, 80% of the total annual antimicrobial consumption is attributed to the livestock sector (112). Given that humans consume livestock, overuse or misuse of antimicrobials in livestock is also a concern and could be an additional route of early life exposure to antibiotics (115).
Contrary to popular belief, the use of traditional and complementary medication (TCM) is also widely common globally (116). Different factors can contribute to TCM use, but it often complements conventional medication use in more urban countries, whereas less urban countries are more dependent on TCM as a form of primary healthcare (116). The latter is due to the easier access of TCM and the cultural beliefs/acceptance of TCM in these regions (116, 117).
While commonly used to treat chronic medications, there is increasing evidence for the use of maternal TCM (particularly herbal medicine) during pregnancy, childbirth and postpartum, including during breastfeeding (117–121). Studies regarding the effects of TCM use on breast milk components, the breast milk microbiota, and the gut microbiota are lacking. However, an article by An et al. (122) reasoned that herbal medications can affect the gut microbiota in one of two ways. The first is via metabolism of herbal medication by the gut microbiota. The second is by regulating the composition and metabolites of the gut microbiota (122). An example of the latter is that certain herbal medications can act as probiotics and promote the growth of beneficial gut microbiota (122). The benefits and limitations of probiotic use, and its effects on the gut microbiota, have already been extensively [reviewed in (123)]. Another study, also investigating the effects of herbal medication on the gut microbiota, showed that some herbal medication supplementation can promote gut bacteria associated with positive health outcomes and reduce the relative abundance of potential pathogens (124). Though the reported outcomes of some TCM use on the gut microbiota seem promising, it is important to acknowledge that more research on TCM needs to be performed, particularly relating to drug–drug interactions and possible adverse side-effects (116). Given the increased statistics of TCM use worldwide, and its use during pregnancy, childbirth, and postpartum (especially during breastfeeding), more knowledge needs to be obtained about the effects of using various kinds of TCM on the gut microbiota and health outcomes of infants.
The prevalence of communicable diseases (CDs) and non-communicable diseases (NCDs) differ across urbanization gradients and associated income groups (Boxes 1, 2). In 2016, for example, 50% of all deaths in low-income countries were due to CDs, nutritional deficiencies and maternal complications during pregnancy and childbirth, while only 7% of deaths in high-income countries were due to these causes (125). In contrast, of the 71% of deaths caused by NCDs, 88% occurred in high-income countries (125). Along with these statistics, low– and middle–income countries are experiencing a rise in NCDs, resulting in a double burden of CDs and NCDs in these countries (5, 59, 125). Accordingly, the gut microbiota and health outcomes of infants can be differentially influenced by medication use associated with maternal and child disease across urbanization gradients.
The effects of NCDs on the gut microbiota (and vice versa) and their health outcomes have been well-studied (4), while the effects of CDs have been understudied. This narrative review will highlight some of the few studies that have been performed on the effects of maternal and child CDs and associated medication use on the gut microbiota and health outcomes of infants. As medication use is closely linked with disease, it can be difficult to distinguish the effects of medication use and disease on the gut microbiota.
One example of a maternal medication that is used as a result of disease is antiretroviral therapy (ART), which is used to combat HIV. Although HIV has been relatively well-managed globally, it is still a major health concern in sub-Saharan Africa [which is considered relatively less urban, Box 1; (126)]. While there have been studies on the effects of HIV on breast milk composition [(127); reviewed in (110)], the combined effects of HIV and ART on the maternal gut microbiota during pregnancy have been little studied. There is some evidence, however, of the effects of HIV and ART exposure during pregnancy on the gut microbiota and health outcomes of infants.
Due to preventative treatment administered to HIV-positive mothers during pregnancy, per year more than 1 million infants are born HIV exposed but uninfected with HIV (HEU) to HIV-positive mothers worldwide. The mortality rates of these infants are twice that of infants not exposed to HIV from the same environment (128–130). In addition to high mortality rates, HEU infants also experience decreased growth, differences in metabolism and immune system development, and increased susceptibility to infections (130–135). Moreover, the gut microbiota composition of HEU infants differs from that of infants unexposed to HIV, and the gut bacterial communities of HEU infants are also less mature—a characteristic generally observed in HIV-positive individuals (136). As maternal gut microbes can be transmitted to the infant during delivery, it may be the impact of HIV and ART on the maternal gut microbiota, along with changes to the breast milk composition (as mothers with HIV are still advised to breast feed), that contributes to the differences observed in HEU babies. Moreover, HEU babies are placed on prophylactic ART treatment for 4–6 weeks following birth, and this too could have a major impact on the gut microbiota and health outcomes of these infants (137). Studies focused on the effects of ART in general have demonstrated that ART prevents restoration of gut symbiosis, thereby potentially facilitating gut dysbiosis (138–143).
Diarrhea is the second leading cause of death in children under 5 years of age, leading to an estimated 1.5 million deaths each year (144, 145). The combined insult of diarrhea and medication use (and often also severe malnutrition) can have enormous negative effects on the gut microbiota and health outcome of infants and children (50, 59, 90). For example the combined treatment of antibiotics and iron fortification supplements that is generally provided to sub-Saharan African infants to treat or prevent iron-deficiency anemia can contribute to childhood diarrheal prevalence (146, 147). Moreover, a small study by Paganini et al. (147) demonstrated that this combined treatment resulted in a decreased abundance of Bifidobacterium (bacteria associated with a healthy gut microbiota) and no decrease in pathogenic E. coli (one of the bacterial species known to cause diarrhea) in the infant gut. These findings highlight the importance of exhibiting caution when providing infants with antibiotic and iron fortification supplements when they are suffering from and being treated for diarrheal infections. These results further indicate the possibility of modulating the gut microbiota to potentially facilitate positive health outcomes in the event that there is no other option than to provide combined treatment. In this case, it would be interesting to determine whether the addition of Bifidobacterium probiotics (at the strain level) could improve outcomes with the combined antibiotic and iron fortification supplementation treatment.
Interestingly, the relationship between the childhood gut microbiota and infections can also determine the efficacy of certain childhood medications, and thereby the health outcomes of infected children. An example of this is the rotavirus vaccine (RVV), which is used to treat rotavirus infection, one of the key causes of diarrhea-related deaths. A pilot study by Harris et al. (148) hypothesized and demonstrated that low RVV efficacy was correlated with microbiota composition in a cohort of Pakistani infants. The gut microbiota of infants who responded to the RVV had an increased relative abundance of broadly categorized pathogenic bacteria (e.g., Clostridium cluster XI and Proteobacteria) in comparison to non-responders. Moreover, higher RVV efficacy was generally observed in a Dutch infant cohort of RVV-responders, demonstrating higher RVV efficacy in infants from high-income countries (148). This could, in part, be explained by differences in breast milk HMO composition across geographical regions and urbanization gradients. Indeed, a study by Ramani et al. (149) demonstrated that a particular geographically distinct neonatal rotavirus strain in India was increased in an in vitro model in response to particular HMO constituents. These findings highlight the importance of understanding how the effects of urbanization across geographical locations can impact HMO composition and, as such, influence medication response in children with disease.
This narrative review highlights some of the many factors associated with urbanization that differentially affect delivery mode, nutrition, and medication use, which in turn differentially affect the gut microbiota and health outcomes of infants across urbanization gradients. We have shown that urbanization contributes to: C-section overuse in urban communities, poor infant feeding practices and behaviors across urbanization gradients, different degrees and forms of malnutrition across urbanization gradients, and early life medication exposure due to both medical practices and behaviors, and distinct disease burdens across urbanization gradients. All of these relationships have varying effects on the gut microbiota and health outcomes of infants.
Taking these relationships and their effects into consideration, we conclude and propose the following:
• The overuse of C-section deliveries, particularly in urban areas, highlights the need for global, standardized, and ethical C-section delivery recommendations/guidelines, and maternal education on delivery rights. Interventions to optimize C-section use have been well-reviewed by the “Optimizing Cesarean Section Use” Lancet series (48).
• Exclusive breastfeeding is highly recommended during early life due to its clear benefits (150). However, for these recommendations to be realized, factors associated with urbanization that contribute to early breastfeeding cessation or non-exclusive breastfeeding need to be taken into account. Our narrative review highlights the important need for:
◦ Governmental support for breastfeeding practices (particularly in public and workplace environments) to normalize breastfeeding in our societies, to prevent the negative effects of unpaid and/or little parental leave, and to encourage mothers, particularly working mothers, to exclusively breastfeed.
◦ Societal education and awareness about proper exclusive breastfeeding practices and their benefits (from public to workforce and healthcare systems).
• The various examples of maternal and child nutrition and malnutrition in this review highlight the important need to improve food environments, food practices, and nutrition education across urbanization gradients. This has already been well and extensively reviewed in a recent UNICEF report (59). Moreover, we showed in this review the important interaction between diet, the gut microbiota and health outcomes, and how essential it is to monitor the gut microbiota, for example to determine the effects of ready-to-use therapeutic foods (and in some cases antibiotic use) on the gut microbiota and health outcomes of children with SAM.
• Medical practices and behaviors across urbanization gradients contribute to early life medication exposure as a result of poor policies and regulatory systems, medication environments, and cultural beliefs. This highlights the need to standardize policies and regulatory systems (for both human and agricultural medication use), to improve medication environments (by improving accessibility, availability and affordability of high-quality, regulated medications) and to improve our understanding of how cultural beliefs can contribute to these practices and behaviors. It also highlights the importance of educating the public of the effects of medication use and to only use medication if absolutely necessary.
• Medication use associated with maternal and child disease differs across urbanization gradients, with a lack of studies performed on the effects of CDs on the gut microbiota and health outcomes of infants. CDs and associated medication use not only differentially impact the gut microbiota and health outcomes of infants and children, but they can also contribute to reducing medication efficacy. Accordingly, knowledge of these interactions can facilitate targeted modulation of the gut microbiota to facilitate positive health outcomes for infected mothers and children because, more often than not, medication use associated with disease is required to fight the disease. Moreover, these findings highlight the importance of taking these factors into consideration when implementing applications and policies to improve the health outcomes of mothers and children with disease.
The factors covered in this narrative review are numerous, but not exhaustive. Other factors to be considered in future studies include various genetic, epigenetic, and environmental factors such as infant sex, parental race/ethnicity, parental diseases, smoking, pet exposure, stress/depression, pollution, climate change, marginalized communities (such as refugees), and cultural practices (such as the Chinese “sitting in period”) (12, 59, 151). Irrespective, based on the examples provided in this narrative review, the differential effects of urbanization on the gut microbiota and health outcomes of infants are clear.
To further expand our understanding of how the microbiota impacts health and can be modified to improve health in a global context, microbiota studies should be continued in both urban and rural populations, and factors associated with urbanization that can contribute to maternal and infant health outcomes should be taken into consideration. By deepening our understanding of these relationships, and by increasing studies across urbanization gradients, we can identify and implement new biological and social approaches to prevent and treat disease and promote positive maternal and infant/childhood health world-wide.
SB and TS performed the literature search and wrote the manuscript. SR, MK, and AZ contributed to writing and critical editing of the paper. All authors contributed to manuscript revision, read, and approved the submitted version.
SB was supported by EUCAN-connect, a federated FAIR platform enabling large-scale analysis of high-value cohort data connecting Europe and Canada in personalized health. This project received funding from the European Union's Horizon 2020 research and innovation program under Grant Agreement No. 824989. TS holds an MD/Ph.D. scholarship from the Junior Scientific Masterclass, University of Groningen. MK was support by ZonMw Grant No. 736300015. AZ was supported by ERC Starting Grant No. 715772, NWO-VIDI Grant No. 016.178.056, the Netherlands Heart Foundation CVON Grant No. 2018-27, and NWO Gravitation grant ExposomeNL.
The authors declare that the research was conducted in the absence of any commercial or financial relationships that could be construed as a potential conflict of interest.
We thank Kate McIntyre for editing the text.
The Supplementary Material for this article can be found online at: https://www.frontiersin.org/articles/10.3389/fped.2020.00408/full#supplementary-material
1. Bailey M, Haverson K, Inman C, Harris C, Jones P, Corfield G, et al. The development of the mucosal immune system pre- and post-weaning: balancing regulatory and effector function. Proc Nutr Soc. (2005) 64:451–7. doi: 10.1079/PNS2005452
2. Carabotti M, Scirocco A, Maselli MA, Severi C. The gut-brain axis: interactions between enteric microbiota, central and enteric nervous systems. Ann Gastroenterol. (2015) 28:203–9.
3. Clemente JC, Ursell LK, Parfrey LW, Knight R. The impact of the gut microbiota on human healh: an integrative view. Cell. (2016) 148:1258–70. doi: 10.1016/j.cell.2012.01.035
4. Sonnenburg ED, Sonnenburg JL. The ancestral and industrialized gut microbiota and implications for human health. Nat Rev Microbiol. (2019) 17:383–90. doi: 10.1038/s41579-019-0191-8
5. Fewtrell M, Michaelsen KF, van der Beek E, van Elburg R. Growth in Early Life: Growth Trajectory and Assessment, Influencing Factors and Impact of Early Nutrition. Milton, QLD: John Wiley & Sons (2016).
6. Swanson LW. Brain Architecture: Understanding the Basic Plan. 2 ed. New York, NY: Oxford University Press (2012).
7. Bäckhed F, Roswall J, Peng Y, Feng Q, Jia H, Kovatcheva-Datchary P, et al. Dynamics and stabilization of the human gut microbiome during the first year of life. Cell Host Microbe. (2015) 17:690–703. doi: 10.1016/j.chom.2015.04.004
8. Nuriel-Ohayon M, Neuman H, Koren O. Microbial changes during pregnancy, birth, and infancy. Front Microbiol. (2016) 7:1031. doi: 10.3389/fmicb.2016.01031
9. Yassour M, Vatanen T, Siljander H, Hämäläinen AM, Härkönen T, Ryhänen SJ, et al. Natural history of the infant gut microbiome and impact of antibiotic treatment on bacterial strain diversity and stability. Sci Transl Med. (2016) 8:343ra81. doi: 10.1126/scitranslmed.aad0917
10. Chong CYL, Bloomfield FH, O'Sullivan JM. Factors affecting gastrointestinal microbiome development in neonates. Nutrients. (2018) 10:274. doi: 10.3390/nu10030274
11. Laursen MF, Zachariassen G, Bahl MI, Bergström A, Høst A, Michaelsen KF, et al. Having older siblings is associated with gut microbiota development during early childhood. BMC Microbiol. (2015) 15:154. doi: 10.1186/s12866-015-0477-6
12. Zijlmans MAC, Korpela K, Riksen-Walraven JM, De Vos WM, De Weerth C. Maternal prenatal stress is associated with the infant intestinal microbiota. Psychoneuroendocrinology. (2015) 53:233–45. doi: 10.1016/j.psyneuen.2015.01.006
13. Tun HM, Konya T, Takaro TK, Brook JR, Chari R, Field CJ, et al. Exposure to household furry pets influences the gut microbiota of infants at 3-4 months following various birth scenarios. Microbiome. (2017) 5:40. doi: 10.1186/s40168-017-0254-x
14. United Nations Department of Economic and Social Affairs Population Division. World Urbanization Prospects 2018: Highlights (ST/ESA/SER.A/421). (2019).
15. World Bank. World Bank Country and Lending Groups – World Bank Data Help Desk. World Bank. (2017). p. 1–8. Available online at: https://datahelpdesk.worldbank.org/knowledgebase/articles/906519-world-bank-country-and-lending-groups (accessed February 28, 2020).
16. Almeida A, Mitchell AL, Boland M, Forster SC, Gloor GB, Tarkowska A, et al. A new genomic blueprint of the human gut microbiota. Nature. (2019) 568:499–504. doi: 10.1038/s41586-019-0965-1
17. Pasolli E, Asnicar F, Manara S, Zolfo M, Karcher N, Armanini F, et al. Extensive unexplored human microbiome diversity revealed by over 150,000 genomes from metagenomes spanning age, geography, and lifestyle. Cell. (2019) 176:649–62.e20. doi: 10.1016/j.cell.2019.01.001
18. Fragiadakis GK, Smits SA, Sonnenburg ED, Van Treuren W, Reid G, Knight R, et al. Links between environment, diet, and the hunter-gatherer microbiome. Gut Microbes. (2019) 10:216–27. doi: 10.1080/19490976.2018.1494103
19. McCall LI, Callewaert C, Zhu Q, Song SJ, Bouslimani A, Minich JJ, et al. Home chemical and microbial transitions across urbanization. Nat Microbiol. (2019) 5:108–15. doi: 10.1038/s41564-019-0593-4
20. Stout MJ, Conlon B, Landeau M, Lee I, Bower C, Zhao Q, et al. Identification of intracellular bacteria in the basal plate of the human placenta in term and preterm gestations. Am J Obstet Gynecol. (2013) 208:226.e1–226.e7. doi: 10.1016/j.ajog.2013.01.018
21. Aagaard K, Ma J, Anthony KM, Petrosino J, Versalovic J. The placenta harbors a unique microbiome. Sci T ransl Med. (2016) 6:237ra65. doi: 10.1126/scitranslmed.3008599
22. Collado MC, Rautava S, Aakko J, Isolauri E, Salminen S. Human gut colonisation may be initiated in utero by distinct microbial communities in the placenta and amniotic fluid. Sci Rep. (2016) 6:1–13. doi: 10.1038/srep23129
23. Lauder AP, Roche AM, Sherrill-Mix S, Bailey A, Laughlin AL, Bittinger K, et al. Comparison of placenta samples with contamination controls does not provide evidence for a distinct placenta microbiota. Microbiome. (2016) 4:29. doi: 10.1186/s40168-016-0172-3
24. de Goffau MC, Lager S, Sovio U, Gaccioli F, Cook E, Peacock SJ, et al. Human placenta has no microbiome but can contain potential pathogens. Nature. (2019) 572:329–34. doi: 10.1038/s41586-019-1451-5
25. Gaskins HR. Developmental microbial ecology of the neonata GIT. Am J Clin Nutr. (1999) 69:1035S−45. doi: 10.1093/ajcn/69.5.1035s
26. Aagaard K, Riehle K, Ma J, Segata N, Mistretta TA, Coarfa C, et al. A metagenomic approach to characterization of the vaginal microbiome signature in pregnancy. PLoS ONE. (2012) 7:e36466. doi: 10.1371/journal.pone.0036466
27. Shao Y, Forster SC, Tsaliki E, Vervier K, Strang A, Simpson N, et al. Stunted microbiota and opportunistic pathogen colonization in caesarean-section birth. Nature. (2019) 574:117–21. doi: 10.1038/s41586-019-1560-1
28. Mitchell C, Hogstrom L, Bryant AM, Bergerat A, Cher A, Pochan S, et al. Delivery mode impacts newborn gut colonization efficiency. bioRxiv. (2020). doi: 10.1101/2020.01.29.919993
29. Jakobsson HE, Abrahamsson TR, Jenmalm MC, Harris K, Quince C, Jernberg C, et al. Decreased gut microbiota diversity, delayed Bacteroidetes colonisation and reduced Th1 responses in infants delivered by caesarean section. Gut. (2014) 63:559–66. doi: 10.1136/gutjnl-2012-303249
30. Penders J, Thijs C, Vink C, Stelma FF, Snijders B, Kummeling I, et al. Factors influencing the composition of the intestinal microbiota in early infancy. Pediatrics. (2006) 118:511–21. doi: 10.1542/peds.2005-2824
31. Fallani M, Young D, Scott J, Norin E, Amarri S, Aguilera M, et al. Intestinal microbiota of 6-week-old infants across Europe: geographic influence beyond delivery mode, breast-feeding, and antibiotics. J Pediatr Gastroenterol Nutr. (2010) 51:77–84. doi: 10.1097/MPG.0b013e3181d1b11e
32. Palmer C, Bik EM, DiGiulio DB, Relman DA, Brown PO. Development of the human infant intestinal microbiota. PLoS Biol. (2007) 5:1556–73. doi: 10.1371/journal.pbio.0050177
33. Garcia-Mantrana I, Collado MC. Obesity and overweight: Impact on maternal and milk microbiome and their role for infant health and nutrition. Mol Nutr Food Res. (2016) 60:1865–75. doi: 10.1002/mnfr.201501018
34. Bokulich NA, Chung J, Battaglia T, Henderson N, Jay M, Li H, et al. Antibiotics, birth mode, and diet shape microbiome maturation during early life. Sci Transl Med. (2016) 8:343ra82. doi: 10.1126/scitranslmed.aad7121
35. Korpela K, Costea P, Coelho LP, Kandels-Lewis S, Willemsen G, Boomsma DI, et al. Selective maternal seeding and environment shape the human gut microbiome. Genome Res. (2018) 28:561–8. doi: 10.1101/gr.233940.117
36. Yassour M, Jason E, Hogstrom LJ, Arthur TD, Tripathi S, Siljander H, et al. Strain-level analysis of mother-to-child bacterial transmission during the first few months of life. Cell Host Microbe. (2018) 24:146–54.e4. doi: 10.1016/j.chom.2018.06.007
37. Sevelsted A, Stokholm J, Bønnelykke K, Bisgaard H. Cesarean section chronic immune disorders. Pediatrics. (2015) 135:e92–8. doi: 10.1542/peds.2014-0596
38. Sandall J, Tribe RM, Avery L, Mola G, Visser GH, Homer CS, et al. Short-term and long-term effects of caesarean section on the health of women and children. Lancet. (2018) 392:1349–57. doi: 10.1016/S0140-6736(18)31930-5
39. Cabrera-Rubio R, Collado MC, Laitinen K, Salminen S, Isolauri E, Mira A. The human milk microbiome changes over lactation and is shaped by maternal weight and mode of delivery. Am J Clin Nutr. (2012) 96:544–51. doi: 10.3945/ajcn.112.037382
40. Cabrera-Rubio R, Mira-Pascual L, Mira A, Collado MC. Impact of mode of delivery on the milk microbiota composition of healthy women. J Dev Orig Health Dis. (2016) 7:54–60. doi: 10.1017/S2040174415001397
41. WHO. Worldwide Country Situation Analysis: Response to Antimicrobial Resistance. WHO. (2015). p. 1–50. Available online at: http://www.who.int/drugresistance/documents/situationanalysis/en/
42. Bollig C, Nothacker M, Lehane C, Motschall E, Lang B, Meerpohl JJ, et al. Prophylactic antibiotics before cord clamping in cesarean delivery: a systematic review. Acta Obstet Gynecol Scand. (2018) 97:521–35. doi: 10.1111/aogs.13276
43. Adewuyi EO, Auta A, Khanal V, Tapshak SJ, Zhao Y. Cesarean delivery in Nigeria: prevalence and associated factors—a population-based cross-sectional study. BMJ Open. (2019) 9:e027273. doi: 10.1136/bmjopen-2018-027273
44. Gibbons L, Belizán J, Lauer J, Betrán A, Merialdi M, Althabe F. The Global Numbers and Costs of Additionally Needed and Unnecessary Caesarean Sections Performed Per Year : Overuse as a Barrier to Universal Coverage. (2010). Available online at: https://www.who.int/healthsystems/topics/financing/healthreport/30C-sectioncosts.pdf (accessed March 24, 2020).
45. Potter JE, Berquó E, Perpétuo IHO, Leal OF, Hopkins K, Souza MR, et al. Unwanted caesarean sections among public and private patients in Brazil: prospective study. Br Med J. (2001) 323:1155–8. doi: 10.1136/bmj.323.7322.1155
46. Kyei-Nimakoh M, Carolan-Olah M, McCann TV. Access barriers to obstetric care at health facilities in sub-Saharan Africa-a systematic review. Syst Rev. (2017) 6:110. doi: 10.1186/s13643-017-0503-x
47. Boatin AA, Schlotheuber A, Betran AP, Moller A-B, Barros AJD, Boerma T, et al. Within country inequalities in caesarean section rates: observational study of 72 low and middle income countries. BMJ. (2018) 360:k55. doi: 10.1136/bmj.k55
48. Betrán AP, Temmerman M, Kingdon C, Mohiddin A, Opiyo N, Torloni MR, et al. Interventions to reduce unnecessary caesarean sections in healthy women and babies. Lancet. (2018) 392:1358–68. doi: 10.1016/S0140-6736(18)31927-5
49. Hanson MA, Bardsley A, De-Regil LM, Moore SE, Oken E, Poston L, et al. The international federation of gynecology and obstetrics (FIGO) recommendations on adolescent, preconception, and maternal nutrition: “think Nutrition First”. Int J Gynecol Obstet. (2015) 131:S213. doi: 10.1016/S0020-7292(15)30023-0
50. Subramanian S, Blanton LV, Frese SA, Charbonneau M, Mills DA, Gordon JI. Cultivating healthy growth and nutrition through the gut microbiota. Cell. (2015) 161:36–48. doi: 10.1016/j.cell.2015.03.013
51. Favier CF, Vaughan EE, Vos WM, De Akkermans ADL. Molecular monitoring of succession of bacterial communities in human neonates molecular monitoring of succession of bacterial communities in human neonates. Appl Environ Microbiol. (2002) 68:219–26. doi: 10.1128/AEM.68.1.219-226.2002
52. Bode L. Human milk oligosaccharides: every baby needs a sugar mama. Glycobiology. (2012) 22:1147–62. doi: 10.1093/glycob/cws074
53. Renz H, Brandtzaeg P, Hornef M. The impact of perinatal immune development on mucosal homeostasis and chronic inflammation. Nat Rev Immunol. (2012) 12:9–23. doi: 10.1038/nri3112
54. Victora CG, Bahl R, Barros AJD, França GVA, Horton S, Krasevec J, et al. Breastfeeding in the 21st century : epidemiology, mechanisms, and lifelong eff ect. Lancet. (2016) 387:475–90. doi: 10.1016/S0140-6736(15)01024-7
55. Musilova S, Rada V, Vlkova E, Bunesova V. Beneficial effects of human milk oligosaccharides on gut microbiota. Benef Microbes. (2014) 5:273–83. doi: 10.3920/BM2013.0080
56. Horta BL, Victora CG. Long-term Effects of Breastfeeding: A Systematic Review. WHO. (2013) Available Online at: www.who.int (accessed February 14, 2020).
57. O'Sullivan A, Farver M, Smilowitz JT. The Influence of early infant-feeding practices on the intestinal microbiome and body composition in infants. Nutr Metab Insights. (2015) 8:1. doi: 10.4137/NMI.S41125
58. Skórka A, Piescik-Lech M, Kolodziej M, Szajewska H. To add or not to add probiotics to infant formulae? An updated systematic review. Benef Microbes. (2017) 8:717–25. doi: 10.3920/BM2016.0233
59. UNICEF. The State of the World's Children 2019. Children, Food and Nutrition: Growing well in a changing world. New York, NY: UNICEF (2019).
60. Gillman MW, Rifas-Shiman SL, Camargo CA, Berkey CS, Frazier AL, Rockett HRH, et al. Risk of overweight among adolescents who were breastfed as infants. J Am Med Assoc. (2001) 285:2461–7. doi: 10.1001/jama.285.19.2461
61. Bar S, Milanaik R, Adesman A. Long-term neurodevelopmental benefits of breastfeeding. Curr Opin Pediatr. (2016) 28:559–66doi: 10.1097/MOP.0000000000000389
62. Rollins NC, Bhandari N, Hajeebhoy N, Horton S, Lutter CK, Martines JC, et al. Why invest, and what it will take to improve breastfeeding practices? Lancet. (2016) 387:491–504. doi: 10.1016/S0140-6736(15)01044-2
63. Perrine CG, Shealy KR, Scanlon KS, Grummer-Strawn LM, Galuska DA, Dee DL, et al. Vital signs: hospital practices to support breastfeeding - United States, 2007 and 2009. Morb Mortal Wkly Rep. (2011) 60:1020–5.
64. Reid G, Nduti N, Sybesma W, Kort R, Kollmann TR, Adam R, et al. Harnessing microbiome and probiotic research in sub-Saharan Africa: recommendations from an African workshop. Microbiome. (2014) 2:12. doi: 10.1186/2049-2618-2-12
65. Kuswara K, Laws R, Kremer P, Hesketh KD, Campbell KJ. The infant feeding practices of Chinese immigrant mothers in Australia: a qualitative exploration. Appetite. (2016) 105:375–84. doi: 10.1016/j.appet.2016.06.008
66. Bolton KA, Kremer P, Hesketh KD, Laws R, Kuswara K, Campbell KJ. Differences in infant feeding practices between Chinese-born and Australian-born mothers living in Australia: a cross-sectional study. BMC Pediatr. (2018) 18:209. doi: 10.1186/s12887-018-1157-0
67. MacKean G, Spragins W. The Challenges of Breastfeeding in a Complex World: a Critical Review of the Qualitative Literature on Women and Their Partners'/Supporters' Perceptions About Breastfeeding. Alberta Health Services. (2012). p. 65. Available online at: http://www.albertahealthservices.ca/ps-1029951-pregnancy-2012-breastfeeding-lit-review.pdf (accessed February 15, 2020).
68. Coomson JB, Aryeetey R. Perception and practice of breastfeeding in public in an urban community in Accra, Ghana. Int Breastfeed J. (2018) 13:18. doi: 10.1186/s13006-018-0161-1
69. Kumar H, du Toit E, Kulkarni A, Aakko J, Linderborg KM, Zhang Y, et al. Distinct patterns in human milk microbiota and fatty acid profiles across specific geographic locations. Front Microbiol. (2016) 7:1619. doi: 10.3389/fmicb.2016.01619
70. Gay MCL, Koleva PT, Slupsky CM, Toit E, du Eggesbo M, Johnson CC, et al. Worldwide variation in human milk metabolome: indicators of breast physiology and maternal lifestyle? Nutrients. (2018) 10:1151. doi: 10.3390/nu10091151
71. Vaidya YH, Patel SH, Patel RJ, Pandit RJ, Joshi CG, Kunjadia AP. Human milk microbiome in urban and rural populations of India. Meta Gene. (2017) 13:13–22. doi: 10.1016/j.mgene.2017.04.001
72. Kimura I, Miyamoto J, Ohue-Kitano R, Watanabe K, Yamada T, Onuki M, et al. Maternal gut microbiota in pregnancy influences offspring metabolic phenotype in mice. Science. (2020) 367:eaaw8429. doi: 10.1126/science.aaw8429
73. Lundgren SN, Madan JC, Emond JA, Morrison HG, Christensen BC, Karagas MR, et al. Maternal diet during pregnancy is related with the infant stool microbiome in a delivery mode-dependent manner. Microbiome. (2018) 6:109. doi: 10.1186/s40168-018-0490-8
74. García-Mantrana I, Selma-Royo M, González S, Parra-Llorca A, Martínez-Costa C, Collado MC. Distinct maternal microbiota clusters are associated with diet during pregnancy: impact on neonatal microbiota and infant growth during the first 18 months of life. Gut Microbes. (2020) 11:962–78. doi: 10.1080/19490976.2020.1730294
75. De Filippo C, Cavalieri D, Di Paola M, Ramazzotti M, Poullet JB, Massart S, et al. Impact of diet in shaping gut microbiota revealed by a comparative study in children from Europe and rural Africa. Proc Natl Acad Sci USA. (2010) 107:14691–6. doi: 10.1073/pnas.1005963107
76. Ley RE, Turnbaugh PJ, Klein S, Gordon JI. Microbial ecology: human gut microbes associated with obesity. Nature. (2006) 444:1022–3. doi: 10.1038/4441022a
77. Payne AN, Chassard C, Lacroix C. Gut microbial adaptation to dietary consumption of fructose, artificial sweeteners and sugar alcohols: Implications for host-microbe interactions contributing to obesity. Obes Rev. (2012) 13:799–809. doi: 10.1111/j.1467-789X.2012.01009.x
78. Vajro P, Paolella G, Fasano A. Microbiota and gut-liver axis: their influences on obesity and obesity-related liver disease. J Pediatr Gastroenterol Nutr. (2013) 56:461–8. doi: 10.1097/MPG.0b013e318284abb5
79. Black RE, Victora CG, Walker SP, Bhutta ZA, Christian P, De Onis M, et al. Maternal and child undernutrition and overweight in low-income and middle-income countries. Lancet. (2013) 382:427–51. doi: 10.1016/S0140-6736(13)60937-X
80. Tun HM, Bridgman SL, Chari R, Field CJ, Guttman DS, Becker AB, et al. Roles of birth mode and infant gut microbiota in intergenerational transmission of overweight and obesity from mother to offspring. JAMA Pediatr. (2018) 172:368–77. doi: 10.1001/jamapediatrics.2017.5535
81. Collado MC, Laitinen K, Salminen S, Isolauri E. Maternal weight and excessive weight gain during pregnancy modify the immunomodulatory potential of breast milk. Pediatr Res. (2012) 72:77–85. doi: 10.1038/pr.2012.42
82. Kalliomäki M, Collado MC, Salminen S, Isolauri E. Early differences in fecal microbiota composition in children may predict overweight. Am J Clin Nutr. (2008) 87:534–8. doi: 10.1093/ajcn/87.3.534
83. Collado MC, Isolauri E, Laitinen K, Salminen S. Effect of mother's weight on infant's microbiota acquisition, composition, and activity during early infancy: a prospective follow-up study initiated in early pregnancy. Am J Clin Nutr. (2010) 92:1023–30. doi: 10.3945/ajcn.2010.29877
84. Gohir W, Ratcliffe EM, Sloboda DM. Of the bugs that shape us: Maternal obesity, the gut microbiome, and long-term disease risk. Pediatr Res. (2015) 77:196–204. doi: 10.1038/pr.2014.169
85. Payne AN, Chassard C, Zimmermann M, Müller P, Stinca S, Lacroix C. The metabolic activity of gut microbiota in obese children is increased compared with normal-weight children and exhibits more exhaustive substrate utilization. Nutr Diabetes. (2011) 1:e12. doi: 10.1038/nutd.2011.8
86. Connor KL, Chehoud C, Altrichter A, Chan L, DeSantis TZ, Lye SJ. Maternal metabolic, immune, and microbial systems in late pregnancy vary with malnutrition in mice. Biol Reprod. (2018) 98:579–92. doi: 10.1093/biolre/ioy002
87. Charbonneau MR, O'Donnell D, Blanton LV, Totten SM, Davis JCC, Barratt MJ, et al. Sialylated milk oligosaccharides promote microbiota-dependent growth in models of infant undernutrition. Cell. (2016) 164:859–71. doi: 10.1016/j.cell.2016.01.024
88. Davis JCC, Lewis ZT, Krishnan S, Bernstein RM, Moore SE, Prentice AM, et al. Growth and morbidity of gambian infants are influenced by maternal milk oligosaccharides and infant gut microbiota. Sci Rep. (2017) 7:40466. doi: 10.1038/srep40466
89. Ramakrishnan U, Imhoff-Kunsch B, Martorell R. Maternal nutrition interventions to improve maternal, newborn, and child health outcomes. Nestle Nutr Inst Workshop Ser. (2014) 78:71–80. doi: 10.1159/000354942
90. Million M, Diallo A, Raoult D. Gut microbiota and malnutrition. Microb Pathog. (2017) 106:127–38. doi: 10.1016/j.micpath.2016.02.003
91. Smith MI, Yatsunenko T, Manary MJ, Trehan I, Mkakosya R, Cheng J, et al. Gut microbiomes of Malawian twin pairs discordant for kwashiorkor. Science. (2013) 339:548–54. doi: 10.1126/science.1229000
92. Crum-Cianflone N. Clostridium innocuum bacteremia in a patient with acquired immunodeficiency syndrome. Am J Med Sci. (2009) 337:480–2. doi: 10.1097/MAJ.0b013e31819f1e95
93. Devkota S, Wang Y, Musch MW, Leone V, Fehlner-Peach H, Nadimpalli A, et al. Dietary-fat-induced taurocholic acid promotes pathobiont expansion and colitis in Il10-/-mice. Nature. (2012) 487:104–8. doi: 10.1038/nature11225
94. Garrett WS. Kwashiorkor and the Gut Microbiota. N Engl J Med. (2013) 368:1746–7. doi: 10.1056/NEJMcibr1301297
95. Tilg H, Moschen AR. Malnutrition and microbiota–a new relationship? Nat Rev Gastroenterol Hepatol. (2013) 10:261–2. doi: 10.1038/nrgastro.2013.48
96. Trehan I, Goldbach HS, LaGrone LN, Meuli GJ, Wang RJ, Maleta KM, et al. Antibiotics as part of the management of severe acute malnutrition. N Engl J Med. (2013) 368:435–35. doi: 10.1056/NEJMoa1202851
97. Vonaesch P, Morien E, Andrianonimiadana L, Sanke H, Mbecko JR, Huus KE, et al. Stunted childhood growth is associated with decompartmentalization of the gastrointestinal tract and overgrowth of oropharyngeal taxa. Proc Natl Acad Sci USA. (2018) 115:E8489–98. doi: 10.1073/pnas.1806573115
98. Imhann F, Bonder MJ, Vila AV, Fu J, Mujagic Z, Vork L, et al. Proton pump inhibitors affect the gut microbiome. Gut. (2016) 65:740–8. doi: 10.1136/gutjnl-2015-310376
99. Maier L, Pruteanu M, Kuhn M, Zeller G, Telzerow A, Anderson EE, et al. Extensive impact of non-antibiotic drugs on human gut bacteria. Nature. (2018) 555:623–8. doi: 10.1038/nature25979
100. Vich Vila A, Collij V, Sanna S, Sinha T, Imhann F, Bourgonje AR, et al. Impact of commonly used drugs on the composition and metabolic function of the gut microbiota. Nat Commun. (2020) 11:362. doi: 10.1038/s41467-019-14177-z
101. Sullivan A, Edlund C, Nord CE. Effect of antimicrobial agents on human microflora. Lancet Infect Dis. (2001) 1:101–14. doi: 10.1016/S1473-3099(01)00066-4
102. Jernberg C, Löfmark S, Edlund C, Jansson JK. Long-term ecological impacts of antibiotic administration on the human intestinal microbiota. ISME J. (2007) 1:56–66. doi: 10.1038/ismej.2007.3
103. Dethlefsen L, Huse S, Sogin ML, Relman DA. The pervasive effects of an antibiotic on the human gut microbiota, as revealed by deep 16S rRNA sequencing. PLoS Biol. (2008) 6:e280. doi: 10.1371/journal.pbio.0060280
104. Cox LM, Yamanishi S, Sohn J, Alekseyenko AV, Leung JM, Cho I, et al. Altering the intestinal microbiota during a critical developmental window has lasting metabolic consequences. Cell. (2014) 158:705–21. doi: 10.1016/j.cell.2014.05.052
105. Falony G, Joossens M, Vieira-Silva S, Wang J, Darzi Y, Faust K, et al. Population-level analysis of gut microbiome variation. Science. (2016) 352:560–4. doi: 10.1126/science.aad3503
106. Francino MP. Antibiotics and the human gut microbiome: dysbioses and accumulation of resistances. Front Microbiol. (2016) 6:1543. doi: 10.3389/fmicb.2015.01543
107. Zhernakova A, Kurilshikov A, Bonder MJ, Tigchelaar EF, Schirmer M, Vatanen T, et al. Population-based metagenomics analysis reveals markers for gut microbiome composition and diversity. Science. (2016) 352:565–9. doi: 10.1126/science.aad3369
108. Sinha T, Vich Vila A, Garmaeva S, Jankipersadsing SA, Imhann F, Collij V, et al. Analysis of 1135 gut metagenomes identifies sex-specific resistome profiles. Gut Microbes. (2019) 10:358–66. doi: 10.1080/19490976.2018.1528822
109. Mor A, Antonsen S, Kahlert J, Holsteen V, Jørgensen S, Holm-Pedersen J, et al. Prenatal exposure to systemic antibacterials and overweight and obesity in danish schoolchildren: a prevalence study. Int J Obes. (2015) 39:1450–5. doi: 10.1038/ijo.2015.129
110. Gomez-Gallego C, Garcia-Mantrana I, Salminen S, Collado MC. The human milk microbiome and factors influencing its composition and activity. Semin Fetal Neonatal Med. (2016) 21:400–5. doi: 10.1016/j.siny.2016.05.003
111. Korpela K, Salonen A, Virta LJ, Kekkonen RA, De Vos WM. Association of early-life antibiotic use and protective effects of breastfeeding: Role of the intestinal microbiota. JAMA Pediatr. (2016) 170:750–7. doi: 10.1001/jamapediatrics.2016.0585
112. European Commission. AMR: A Major European and Global challenge. Health and Food Safety (2017). p. 1–2. Available at: https://ec.europa.eu/health/amr/sites/health/files/antimicrobial_resistance/docs/amr_2017_factsheet.pdf (accessed February 25, 2020).
113. Rogawski ET, Platts-Mills JA, Seidman JC, John S, Mahfuz M, Ulak M, et al. Use of antibiotics in children younger than two years in eight countries: a prospective cohort study. Bull World Health Organ. (2017) 95:49–61. doi: 10.2471/BLT.16.176123
114. Auta A, Hadi MA, Oga E, Adewuyi EO, Abdu-Aguye SN, Adeloye D, et al. Global access to antibiotics without prescription in community pharmacies: a systematic review and meta-analysis. J Infect. (2019) 78:8–18. doi: 10.1016/j.jinf.2018.07.001
115. Woolhouse M, Ward M, Van Bunnik B, Farrar J. Antimicrobial resistance in humans, livestock and the wider environment. Philos Trans R Soc B Biol Sci. (2015) 370:20140083. doi: 10.1098/rstb.2014.0083
116. WHO. WHO Traditional Medicine Strategy: 2014 - 2023. (2013). Available online at: www.who.int (accessed February 26, 2020).
117. James PB, Kaikai AI, Bah AJ, Steel A, Wardle J. Herbal medicine use during breastfeeding: a cross-sectional study among mothers visiting public health facilities in the Western area of Sierra Leone. BMC Complement Altern Med. (2019) 19:66. doi: 10.1186/s12906-019-2479-7
118. Chuang CH, Chang PJ, Hsieh WS, Tsai YJ, Lin SJ, Chen PC. Chinese herbal medicine use in Taiwan during pregnancy and the postpartum period: a population-based cohort study. Int J Nurs Stud. (2009) 46:787–95. doi: 10.1016/j.ijnurstu.2008.12.015
119. Sim TF, Sherriff J, Hattingh HL, Parsons R, Tee LBG. The use of herbal medicines during breastfeeding: a population-based survey in western australia. BMC Complement Altern Med. (2013) 13:317. doi: 10.1186/1472-6882-13-317
120. Aleandri V, Bertazzoni G, Romanzi D, Vetrano G, Durazzi F, Mazzanti G, et al. The use of herbal products during breastfeeding: a study from a public Italian Hospital. J Food Process Technol. (2014) 5:1. doi: 10.4172/2157-7110.1000354
121. Laelago T, Yohannes T, Lemango F. Prevalence of herbal medicine use and associated factors among pregnant women attending antenatal care at public health facilities in Hossana Town, Southern Ethiopia: facility based cross sectional study. Arch Public Health. (2016) 74:7. doi: 10.1186/s13690-016-0118-z
122. An X, Bao Q, Di S, Zhao Y, Zhao S, Zhang H, et al. The interaction between the gut microbiota and herbal medicines. Biomed Pharmacother. (2019) 118:109252. doi: 10.1016/j.biopha.2019.109252
123. Suez J, Zmora N, Segal E, Elinav E. The pros, cons, and many unknowns of probiotics. Nat Med. (2019) 25:716. doi: 10.1038/s41591-019-0439-x
124. Peterson CT, Sharma V, Uchitel S, Denniston K, Chopra D, Mills PJ, et al. Prebiotic potential of herbal medicines used in digestive health and disease. J Altern Complement Med. (2018) 24:656–65. doi: 10.1089/acm.2017.0422
125. WHO. The Top 10 Causes of Death. (2014). Available online at: https://www.who.int/news-room/fact-sheets/detail/the-top-10-causes-of-death (accessed February 25, 2020).
126. Fettig J, Swaminathan M, Murrill CS, Kaplan JE. Global epidemiology of HIV. Infect Dis Clin North Am. (2014) 28:323–37. doi: 10.1016/j.idc.2014.05.001
127. González R, Mandomando I, Fumadó V, Sacoor C, Macete E, Alonso PL, et al. Breast milk and gut microbiota in African mothers and infants from an area of high HIV prevalence. PLoS ONE. (2013) 8:e80299. doi: 10.1371/journal.pone.0080299
128. Tobin NH, Aldrovandi GM. Immunology of Pediatric HIV Infection. Immunol Rev. (2013) 254:143–69. doi: 10.1111/imr.12074
129. Bender JM, Li F, Martelly S, Byrt E, Rouzier V, Tobin N, et al. Maternal HIV infection influences the microbiome of HIV uninfected infants. Sci Transl Med. (2016) 8:349ra100. doi: 10.1126/scitranslmed.aaf5103
130. Powis KM, Slogrove AL, Mofenson L. Protecting the health of our AIDS-free generation: beyond prevention of mother-to-child HIV transmission. AIDS. (2017) 31:315–6. doi: 10.1097/QAD.0000000000001315
131. Powis KM, Smeaton L, Ogwu A, Lockman S, Dryden-Peterson S, van Widenfelt E, et al. Effects of in utero antiretroviral exposure on longitudinal growth of HIV-exposed uninfected infants in botswana. JAIDS J Acquir Immune Defic Syndr. (2011) 56:131–8. doi: 10.1097/QAI.0b013e3181ffa4f5
132. Powis KM, Smeaton L, Hughes MD, Tumbare EA, Souda S, Jao J, et al. In-utero triple antiretroviral exposure associated with decreased growth among HIV-exposed uninfected infants in Botswana. AIDS. (2016) 30:211–20. doi: 10.1097/QAD.0000000000000895
133. Slogrove A, Reikie B, Naidoo S, De Beer C, Ho K, Cotton M, et al. HIV-exposed uninfected infants are at increased risk for severe infections in the first year of life. J Trop Pediatr. (2012) 58:505–8. doi: 10.1093/tropej/fms019
134. Reikie BA, Adams RCM, Leligdowicz A, Ho K, Naidoo S, Ruck CE, et al. Altered innate immune development in HIV-exposed uninfected infants. JAIDS J Acquir Immune Defic Syndr. (2014) 66:245–55. doi: 10.1097/QAI.0000000000000161
135. Jao J, Kirmse B, Yu C, Qiu Y, Powis K, Nshom E, et al. Lower preprandial insulin and altered fuel use in HIV/antiretroviral-exposed infants in cameroon. J Clin Endocrinol Metab. (2015) 100:3260–9. doi: 10.1210/JC.2015-2198
136. Bandera A, De Benedetto I, Bozzi G, Gori A. Altered gut microbiome composition in HIV infection: causes, effects and potential intervention. Curr Opin HIV AIDS. (2018) 11:182–90. doi: 10.1097/COH.0000000000000429
137. WHO. Antiretroviral Drugs for Treating Pregnant Women and Preventing HIV Infection in Infants. Geneva: WHO (2010). p. 1–117.
138. Lozupone CA, Li M, Campbell TB, Flores SC, Linderman D, Gebert MJ, et al. Alterations in the gut microbiota associated with HIV-1 infection. Cell Host Microbe. (2013) 14:329–39. doi: 10.1016/j.chom.2013.08.006
139. Lozupone CA, Rhodes ME, Neff CP, Fontenot AP, Campbell TB, Palmer BE. HIV-induced alteration in gut microbiota: driving factors, consequences, and effects of antiretroviral therapy. Gut Microbes. (2014) 5:562–70. doi: 10.4161/gmic.32132
140. Li SX, Armstrong AJS, Neff CP, Shaffer M, Lozupone CA, Palmer BE. Complexities of gut microbiome dysbiosis in the context of HIV infection and antiretroviral therapy. Clin Pharmacol Ther. (2016) 99:600–11. doi: 10.1002/cpt.363
141. Pinto-Cardoso S, Lozupone C, Bricenõ O, Alva-Hernández S, Téllez N, Adriana A, et al. Fecal bacterial communities in treated HIV infected individuals on two antiretroviral regimens. Sci Rep. (2017) 7:43741. doi: 10.1038/srep43741
142. Pinto-Cardoso S, Klatt NR, Reyes-Terán G. Impact of antiretroviral drugs on the microbiome: Unknown answers to important questions. Curr Opin HIV AIDS. (2018) 13:53–60. doi: 10.1097/COH.0000000000000428
143. Villanueva-Millán MJ, Pérez-Matute P, Recio-Fernández E, Lezana Rosales JM, Oteo JA. Differential effects of antiretrovirals on microbial translocation and gut microbiota composition of HIV-infected patients. J Int AIDS Soc. (2017) 20:21526. doi: 10.7448/IAS.20.1.21526
144. UNICEF and WHO. Diarrhoea: Why Children are Still Dying and What can be Done. (2009). Available online at: http://apps.who.int/iris/bitstream/handle/10665/44174/9789241598415_eng.pdf;jsessionid=945E199DB9ED41ECC46006AB3F01FCE6?sequence=1 (accessed November 18, 2018).
145. WHO. Fact Sheet: Diarrhoeal Disease. (2017). Available online at: http://www.who.int/news-room/fact-sheets/detail/diarrhoeal-disease (accessed November 18, 2018).
146. Vist GE, Suchdev PS, De-Regil LM, Walleser S, Peña-Rosas JP. Home fortification of foods with multiple micronutrient powders for health and nutrition in children under 2 years of age. Cochrane Database Syst Rev. (2011) 9:CD008959. doi: 10.1002/14651858.CD008959
147. Paganini D, Uyoga MA, Kortman GAM, Cercamondi CI, Winkler HC, Boekhorst J, et al. Iron-containing micronutrient powders modify the effect of oral antibiotics on the infant gut microbiome and increase post-antibiotic diarrhoea risk: a controlled study in Kenya. Gut. (2019) 68:645–53. doi: 10.1136/gutjnl-2018-317399
148. Harris V, Ali A, Fuentes S, Korpela K, Kazi M, Tate J, et al. Rotavirus vaccine response correlates with the infant gut microbiota composition in Pakistan. Gut Microbes. (2018) 9:93–101. doi: 10.1080/19490976.2017.1376162
149. Ramani S, Stewart CJ, Laucirica DR, Ajami NJ, Robertson B, Autran CA, et al. Human milk oligosaccharides, milk microbiome and infant gut microbiome modulate neonatal rotavirus infection. Nat Commun. (2018) 9:5010. doi: 10.1038/s41467-018-07476-4
150. WHO. Exclusive Breastfeeding for Six Months Best for Babies Everywhere, 5th January Statement. (2011). Available online at: https://www.who.int/mediacentre/news/statements/2011/breastfeeding_20110115/en/ (accessed February 14, 2020).
Keywords: urbanization, gut microbiota, infant, mother, health outcomes, delivery mode, nutrition, medication
Citation: Brushett S, Sinha T, Reijneveld SA, de Kroon MLA and Zhernakova A (2020) The Effects of Urbanization on the Infant Gut Microbiota and Health Outcomes. Front. Pediatr. 8:408. doi: 10.3389/fped.2020.00408
Received: 23 April 2020; Accepted: 12 June 2020;
Published: 29 July 2020.
Edited by:
Eytan Wine, University of Alberta, CanadaReviewed by:
Giulia Paolella, IRCCS Ca' Granda Foundation Maggiore Policlinico Hospital, ItalyCopyright © 2020 Brushett, Sinha, Reijneveld, de Kroon and Zhernakova. This is an open-access article distributed under the terms of the Creative Commons Attribution License (CC BY). The use, distribution or reproduction in other forums is permitted, provided the original author(s) and the copyright owner(s) are credited and that the original publication in this journal is cited, in accordance with accepted academic practice. No use, distribution or reproduction is permitted which does not comply with these terms.
*Correspondence: Alexandra Zhernakova, YS56aGVybmFrb3ZhQHVtY2cubmw=
Disclaimer: All claims expressed in this article are solely those of the authors and do not necessarily represent those of their affiliated organizations, or those of the publisher, the editors and the reviewers. Any product that may be evaluated in this article or claim that may be made by its manufacturer is not guaranteed or endorsed by the publisher.
Research integrity at Frontiers
Learn more about the work of our research integrity team to safeguard the quality of each article we publish.