- 1Duke-NUS Medical School, Singapore, Singapore
- 2Children's Intensive Care Unit, Department of Pediatrics, KK Women's and Children's Hospital, Singapore, Singapore
- 3Department of Pediatrics, Duke University Medical Center, Durham, NC, United States
- 4Duke Clinical Research Institute, Durham, NC, United States
- 5Duke University Medical Center Library and Archives, Durham, NC, United States
Objectives: This study aimed to identify alterations in pharmacokinetics in children on extracorporeal membrane oxygenation (ECMO), identify knowledge gaps, and inform future pharmacology studies.
Data Sources: We systematically searched the databases MEDLINE, CINAHL, and Embase from earliest publication until November 2018 using a controlled vocabulary and keywords related to “ECMO” and “pharmacokinetics,” “pharmacology,” “drug disposition,” “dosing,” and “pediatrics.”
Study Selection: Inclusion criteria were as follows: study population aged <18 years, supported on ECMO for any indications, received any medications while on ECMO, and reported pharmacokinetic data.
Data Extraction: Clearance and/or volume of distribution values were extracted from included studies.
Data Synthesis: Forty-one studies (total patients = 574) evaluating 23 drugs met the inclusion criteria. The most common drugs studied were antimicrobials (n = 13) and anticonvulsants (n = 3). Twenty-eight studies (68%) were conducted in children <1 year of age. Thirty-three studies (80%) were conducted without intra-study comparisons to non-ECMO controls. Increase in volume of distribution attributable to ECMO was demonstrated for nine (56%) drugs: cefotaxime, gentamicin, piperacillin/tazobactam, fluconazole, micafungin, levetiracetam, clonidine, midazolam, and sildenafil (range: 23–345% increase relative to non-ECMO controls), which may suggest the need for higher initial dosing. Decreased volume of distribution was reported for two drugs: acyclovir and ribavirin (50 and 69%, respectively). Decreased clearance was reported for gentamicin, ticarcillin/clavulanate, bumetanide, and ranitidine (range: 26–95% decrease relative to non-ECMO controls). Increased clearance was reported for caspofungin, micafungin, clonidine, midazolam, morphine, and sildenafil (range: 25–455% increase relative to non-ECMO controls).
Conclusions: There were substantial pharmacokinetic alterations in 70% of drugs studied in children on ECMO. However, studies evaluating pharmacokinetic changes of many drug classes and those that allow direct comparisons between ECMO and non-ECMO patients are still lacking. Systematic evaluations of pharmacokinetic alterations of drugs on ECMO that incorporate multidrug opportunistic trials, physiologically based pharmacokinetic modeling, and other methods are necessary for definitive dose recommendations.
Trial Registration Prospero Identifier: CRD42019114881.
Introduction
Extracorporeal membrane oxygenation (ECMO) is a cardiopulmonary bypass support system used to temporarily sustain cardiac and/or respiratory function in critically ill patients. ECMO has been established as an effective modality in neonates and children who have failed conventional intensive care management. However, the ECMO circuit has been shown to sequester drug molecules in a highly unpredictable manner (1, 2) particularly with highly lipophilic and protein-bound drugs, as shown in a series of ex vivo experiments in which drugs were administered into isolated ECMO circuits (3, 4).
Pharmacokinetic studies conducted in critically ill neonates supported on ECMO have demonstrated marked alterations in drug pharmacokinetics and disposition. Specifically, the use of ECMO has been shown to increase the volume of distribution (Vd) of drugs and alter the clearance of certain drugs (5, 6). Given the high variability in drug pharmacokinetics and disposition in patients on ECMO, a thorough understanding of the pharmacokinetic alterations that occur during ECMO is critical in guiding clinicians in determining dose adjustments.
To date, most studies involving pediatric ECMO clinical pharmacology have been conducted with anti-infective agents, but data have started to emerge for other classes of commonly used drugs in this patient population. However, there is currently no systematic review of the current evidence base of the alterations in drug pharmacokinetics and disposition in children supported on ECMO. We therefore performed this systematic review to assess and summarize the current literature up for alterations in drug pharmacokinetics, specifically clearance and Vd, in critically ill pediatric patients supported on ECMO.
Materials and Methods
This systematic review was registered in PROSPERO with the registration number CRD42019114881.
Search Strategy
A systematic, computerized search of the literature in MEDLINE (via PubMed), CINAHL, and Embase was conducted by a medical research librarian (B.M.) using a controlled vocabulary and keywords related to extracorporeal membrane oxygenation (ECMO) and pharmacokinetics, pharmacology, drug disposition, or dosing in the pediatric population (from birth to 18 years). Our search time frame was from database inception to November 1, 2018. The search strategies are shown in the Supplementary Information 1. The reference lists of all selected publications were checked to retrieve relevant publications that were not identified in the computerized search.
Study Selection and Risk-of-Bias Assessment
The final search results were compiled and imported into Covidence (Veritas Health Innovation, Melbourne, Australia). Two reviewers (N.S. and J.K.) independently screened and reviewed titles and abstracts to assess their eligibility. Full-text articles were retrieved if the abstract provided insufficient information to establish eligibility or if the article passed the first eligibility screening. Disagreements on study eligibility were resolved by consensus or by arbitration from a third independent reviewer (J.H.L).
Studies that fulfilled the following inclusion criteria were included: (1) study population aged 18 years and below, including neonates (0–28 days of age), infants (29 days−1 year of age), children (>1–12 years of age), and adolescents (>12–18 years of age), (2) study population receiving ECMO for any clinical indications and durations, (3) study population receiving any medications or therapeutics while on ECMO, and (4) studies reporting pharmacokinetic parameters, specifically clearance and Vd, in patients supported on ECMO. Case reports, case series, abstracts, and conference proceedings were all included. We only included studies published in the English language. Articles with only pharmacodynamics, response, or safety data were not included. Other exclusion criteria included (1) ex vivo studies, (2) animal studies, and (3) studies with predominantly adult population with no separate description of a pediatric subgroup. Given the nature of pharmacokinetic studies, traditional risk-of-bias assessment with tools, such as the Newcastle–Ottawa scale, was not conducted. Instead, each article was evaluated for completeness of reporting based on the consensus-based ClinPK statement by Kanji et al. (7).
Data Extraction and Synthesis
A standardized data collection form was used to extract the relevant data from each eligible study. The following data were collected: key characteristics of the study (e.g., study year, study design, and type of publication), characteristics of the study population (e.g., age, clinical indications of ECMO, and modality of ECMO), interventions received by the study population (e.g., medications received while on ECMO, including their dosage form and dose regimen), and pharmacokinetic parameters measured or estimated. For the purpose of this review, we specifically focused on two pharmacokinetic parameters—clearance and Vd.
Comparison of Clearance and Volume of Distribution of Drugs Between the ECMO and Non-ECMO Groups
We extracted clearance and/or Vd values from the included studies and compared these pharmacokinetic parameters between children supported with ECMO and children not on ECMO. For studies with intra-study comparators, the pharmacokinetic parameters were compared between the ECMO and non-ECMO groups within each study. For studies that did not include non-ECMO control groups, we compared these pharmacokinetic parameters against historical controls in other pharmacokinetic studies conducted in pediatric patients not supported with ECMO. For each drug, a computerized search was conducted in MEDLINE (via PubMed) using keywords related to the drug and pharmacokinetics, pharmacology, or dosing in the pediatric population (from birth to 18 years). The titles and abstracts of the articles were screened, and full-text articles were subsequently reviewed for pharmacokinetic parameters of the drug and its metabolites (where applicable) in non-ECMO pediatric patients. Wherever possible, the non-ECMO historical controls were age-matched against the ECMO group. In addition, to evaluate the association between changes in Vd or drug clearance and the drug's physicochemical properties, we compared the log P-values between drugs with increased, decreased, or no change in Vd or drug clearance.
Assessment of Quality of Reporting of Pharmacokinetic Studies
We evaluated each study included in this systematic review for their compliance rates with each item in the ClinPK checklist, a 24-item checklist for transparent and consistent reporting of clinical pharmacokinetic studies developed by Kanji et al. (7). The checklist included criteria such as study rationale, eligibility criteria of study participants, coadministration of study drugs with potentially interacting drugs, validation of quantitative bioanalytical methods, pharmacokinetic modeling methods, and reporting of results with appropriate measures.
Results
Characteristics of Included Studies and Study Populations
Out of the 3,428 records retrieved by the systematic search and hand search of reference lists, 41 studies met the inclusion criteria and included a total of 574 pediatric patients (Figure 1). These 41 publications reported clearance and Vd data for 23 drugs: six antibiotics (cefotaxime, gentamicin, meropenem, piperacillin/tazobactam, ticarcillin/clavulanate, and vancomycin), three antiviral agents (acyclovir, oseltamivir, and ribavirin), four antifungal agents (caspofungin, fluconazole, micafungin, and voriconazole), three anticonvulsants (fosphenytoin, levetiracetam, and phenobarbital), and others (bumetanide, clonidine, heparin, midazolam, morphine, ranitidine, and sildenafil). Twenty-eight studies (68%) were conducted in children <1 year of age. The clinical and methodological characteristics of included studies are summarized in Table 1 and Supplementary Table 1. The studies were published between 1989 and 2018.
There was substantial heterogeneity in the pharmacokinetic sampling and treatment modalities used across studies. These variations included differences in route of administration (intravenous or oral), dosages of medications, administration method (bolus, intermittent dosing, or continuous infusion), and usage of co-medications. Twelve of the 41 studies (29.3%) included patients on continuous renal replacement therapy (CRRT) (15, 16, 19, 20, 26–28, 30, 31, 33, 35, 42). Renal and liver functions were not consistently reported in majority of the studies. Specifically, baseline renal functions were reported only in 19 out of 41 studies (46.3%) (8–11, 20–24, 28, 30–33, 37, 39, 40, 43, 46) while only 12 out of 41 studies (29.3%) reported baseline liver functions (8, 10, 21, 24, 28, 30–32, 40, 42, 43, 46). In addition, 17 studies (41%) did not report the type of ECMO used (11–13, 16–19, 22, 25–27, 30, 31, 38, 41, 44, 47). Of those that reported the type of ECMO used, 10 studies (24%) employed both veno-venous (VV) and venous–arterial (VA) ECMO (8, 14, 20, 24, 32, 35, 40, 42) 10 studies (24%) used VA ECMO only (9, 15, 21, 23, 28, 37, 39, 43, 45, 46), and the remaining four studies (10%) used VV ECMO only (29, 33, 34, 36). Of 41 studies, 21 studies (51.2%) specified the type of oxygenator used, with Avecor 0800 oxygenator (Avecor Cardiovascular Inc.) being the most commonly used (8, 10, 15, 17, 21–24, 28, 31, 34, 36–38, 40, 42–46, 48). However, only 14 studies (34.1%) reported the prime volume (range: 200–750 ml) (8, 11, 14, 15, 21, 24, 31, 32, 37, 40, 43, 44, 48, 49). Only seven studies (17%) were conducted with intra-study comparisons to non-ECMO controls (9, 12, 22, 30, 34, 35, 40).
Alterations in Volume of Distribution of Drugs in ECMO-Supported Patients
Of the 23 drugs studied, Vd values were reported for 16 drugs (70%) (8–14, 18, 20–24, 26, 28, 30–40, 42, 43, 47, 50) with the remaining seven other drugs having only clearance values reported without Vd values (15–17, 19, 27, 29, 44–46). Relative to non-ECMO controls, significant alterations in Vd were observed in pediatric patients supported on ECMO for 11 of 16 studied drugs (Table 2). Increased Vd attributable to ECMO was demonstrated for nine drugs: cefotaxime, gentamicin, piperacillin/tazobactam, fluconazole, micafungin, levetiracetam, clonidine, midazolam, and sildenafil (range: 23.8–345% increase relative to non-ECMO controls), suggesting the need for higher initial dosing (11, 16, 17, 22, 32, 39, 41, 46, 47). Decreased Vd was reported for two drugs: acyclovir and ribavirin (50 and 69%, respectively) (26, 28). Vd of the following drugs were not substantially altered in patients supported on ECMO relative to non-ECMO controls: vancomycin, voriconazole, fosphenytoin, phenobarbital, and bumetanide (2, 22, 35, 39).
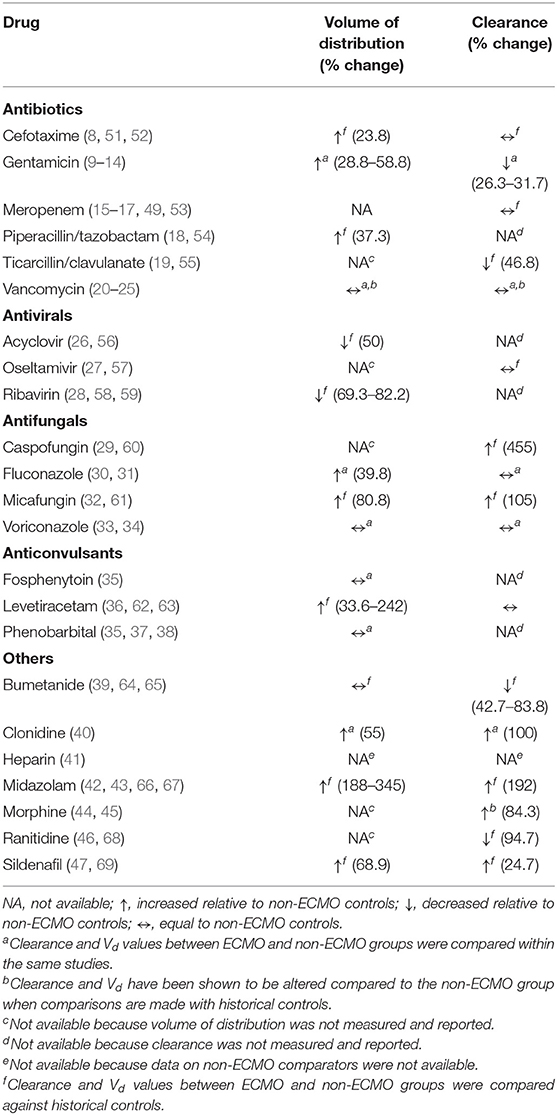
Table 2. Summary of pharmacokinetic changes (Vd and clearance) published in critically ill pediatric patients on ECMO.
Of note, vancomycin was studied in six separate studies, only one of which included a non-ECMO comparator group (22) while the remaining five did not (20, 21, 23, 24, 50) Although a trend was observed toward smaller Vd of vancomycin relative to non-ECMO controls, the difference did not reach statistical significance in the study by Buck (22) However, when the Vd values were compared against historical controls, increased Vd was observed compared to pediatric patients not on ECMO (20, 21, 23, 24, 50). In addition, we did not identify any significant correlation between lipophilicity (as measured by log P) of drugs and changes in Vd attributable to ECMO (Supplementary Table 1).
Alterations in Drug Clearance in ECMO-Supported Patients
Out of the 23 drugs studied, drug clearance was reported for 17 drugs (74%) (8–17, 19–24, 27, 29–32, 36, 39, 40, 42–47, 50) with the remaining five other drugs having only Vd values reported (18, 26, 28, 35, 37, 38). Compared to non-ECMO controls, significant changes in clearance were observed in pediatric patients supported on ECMO for 10 of 17 studied drugs (Table 2). Decreased clearance was reported for gentamicin, ticarcillin/clavulanate, bumetanide, and ranitidine (range: 26–95% decrease relative to non-ECMO controls) (9, 19, 39, 46). Increased clearance was reported for caspofungin, micafungin, clonidine, midazolam, morphine, and sildenafil (range: 25–455% increase relative to non-ECMO controls) (32, 34, 40, 43, 45, 47). Changes in clearance were not observed for cefotaxime, meropenem, vancomycin, oseltamivir, fluconazole, voriconazole, and levetiracetam (8, 15, 22, 27, 30, 34, 36).
Similarly, for vancomycin, the study by Buck (22), which included a non-ECMO comparator group within the same study, did not report a statistically significant difference in clearance. However, comparison of vancomycin clearance values in pediatric patients supported on ECMO against historical controls showed decreased clearance (20, 24, 25, 50). No significant association was found between log P-values of drugs and changes in clearance attributable to ECMO (Supplementary Table 1).
Quality of Reporting of Published Pharmacokinetic Studies of Drugs in Pediatric Patients Supported on ECMO
Of the 24 items in the ClinPK checklist, all included studies reported the drugs and patient population studied. Majority of studies (>50%) also provided an explanation of the study rationale, objectives and hypotheses, eligibility criteria of study participants, information on ethics approval, drug preparation and administration techniques, details on blood sampling including quantification of drugs and their metabolites using validated approaches, pharmacokinetic modeling methods, and formulas for calculation of variables. The detailed percentages and lists of studies are tabulated in Supplementary Table 2. Majority of studies (>50%) also provided information on relevant variables that may account for inter- and intra-patient variability in pharmacokinetics, and pharmacokinetic parameters were reported appropriately for most studies (Supplementary Table 2). However, only 14 of the 41 included studies (34.1%) provided background information on the pharmacokinetic data that are known and relevant to the drugs being evaluated. In addition, only 16 of the 41 studies (39.0%) reported on the concurrent administration (or lack thereof) of study drugs with other drugs or food substances that may potentially interact with the study drugs. Study withdrawals or subjects lost to follow-up (or lack thereof), as well as quantification of missing or excluded data, were also rarely reported in only 10 and 2 studies (24.4 and 4.9%), respectively.
Discussion
To our best knowledge, this is the first systematic review that encompasses drugs across all therapeutic classes and focuses on the available data in critically ill pediatric patients. Raffaeli et al. (70) recently provided an overview of the available evidence pertaining to determinants of altered drug disposition and evidence-based pharmacotherapy recommendations during ECMO specifically in neonates. The present study includes the available data in critically ill pediatric patients across different age groups. Using a comprehensive systematic search strategy, we identified a total of 41 studies evaluating 23 drugs. In line with the results of ex vivo studies that demonstrated drug extraction by the ECMO circuit, particularly for highly lipophilic and protein-bound drugs (2, 3) we found substantial pharmacokinetic alterations, in either drug clearance or Vd or both, reported in 70% of drugs studied in pediatric patients supported on ECMO.
Most studies demonstrated increased Vd and decreased clearance of drugs between ECMO and non-ECMO patients. The differences in pharmacokinetics for which we have the most confidence are those generated from studies that included the non-ECMO comparator groups. However, majority of the studies did not include the non-ECMO comparator groups, and the comparisons were made based on pharmacokinetic parameters reported in different studies. The differences in Vd and clearance of some of the studied drugs, such as vancomycin, between ECMO and non-ECMO controls showed marked intra-study variability, with some studies demonstrating increased values for the pharmacokinetic parameters (20, 21, 23, 24, 50) while others demonstrated decreased values or no change (22).
Despite our best efforts to compare these values across studies with similar patient demographics, it is unclear whether the differences, or lack thereof, are confounded by differences in patient profiles, study designs, and methodology. Notably, the lack of controlled data and inclusion of non-ECMO controls within these studies may have contributed to such heterogeneity. For this reason, it is difficult to draw definite conclusions regarding the pharmacokinetic differences between the ECMO and non-ECMO groups and further highlights the need for future studies evaluating the impact of ECMO on drug pharmacokinetics and disposition to include the non-ECMO comparator groups. Additionally, in contrast to the findings of ex vivo studies which showed the sequestration of drugs by the ECMO circuit to be particularly profound for more lipophilic drugs, we did not observe any correlation between log P-values of drugs and changes in both clearance and Vd attributable to ECMO. However, this may be due in part to the limited number of drugs studied to date, which makes it difficult to draw conclusive results.
There are several limitations to our systematic review. Firstly, most of the included studies had small sample sizes and were performed mainly in neonates and infants, with some studies including a mixed pediatric population. Given that the effect of ECMO on drug pharmacokinetics and disposition can vary by patient age, the extrapolation of these results to older children supported on ECMO cannot be robustly justified and requires dedicated pharmacokinetic trials to address. Importantly, the ECMO setup has evolved considerably over time. Considering that most of the studies were conducted in the 1990s, the differences in drug extraction between the older ECMO components and contemporary ECMO setup remain unclear and require further investigation.
The heterogeneity across studies, in terms of not only drugs studied but also clinical indications for which those drugs were administered and other patient factors, contributes substantially to large pharmacokinetic variability and may limit the conclusions that can be drawn. Such heterogeneity is further compounded by physiological derangements associated with critical illnesses, renal replacement therapy, drug–drug interactions, genetic polymorphisms, and the use of ECMO (71–74). Clearances of drugs in patients supported on ECMO are also determined by renal and hepatic flow and function. Unfortunately, these pieces of information were not included in most publications included in the review, and we were unable to address this in our analysis. A much deeper understanding of the interplay between these factors is critical in improving our ability to provide personalized dosing to pediatric patients on ECMO.
Pediatric pharmacokinetic research presents specific challenges, some of which can be circumvented with the use of model-based approaches to study design and analysis, such as population pharmacokinetic modeling and physiologically based pharmacokinetic modeling. As such, identification of the most optimal study design and pharmacokinetic protocol would be crucial for future pharmacology studies in pediatric ECMO patients. Furthermore, the transparent and complete reporting of study data in clinical pharmacokinetic studies is essential for better assessment and evaluation of study information and its clinical translation. Although validated tools for assessment of the quality and validity of pharmacokinetic studies have not yet been developed, the use of the ClinPK consensus in this systematic review has highlighted the poor compliance of most studies to the 24-item checklist considered to be necessary for the reporting of pharmacokinetic studies. We recommend more complete reporting of future pharmacokinetic studies that meet at least the minimum reporting criteria in this patient population. This may improve the utility and comparability of study findings and further circumvent the unique challenges associated with pediatric pharmacology studies.
In addition, the majority of pharmacokinetic data in ECMO-supported pediatric populations to date have been from studies conducted in neonates and infants, with antimicrobial agents and anticonvulsants being the most commonly studied drugs. Notably, studies evaluating pharmacokinetic changes of many drugs such as dexmedetomidine as well as other drug classes, including analgesics and cardiovascular, sedative, and anesthetic agents, which are commonly used in critically ill pediatric patients on ECMO, are still lacking and represent important areas of future studies. This highlights the urgent need for pharmacokinetic studies in these children for specific and clinically important drug classes, using a contemporary ECMO setup and with appropriate study designs, including the inclusion of appropriate controls.
Conclusions
While the total number of drugs studied to date remains limited, we found substantial pharmacokinetic alterations in terms of Vd and/or clearance in 69.5% of drugs studied in children on ECMO. We also identified major limitations of the existing evidence base, which explains at least partially our current inability to readily predict pharmacokinetic changes and thus dose adjustments of drugs in critically ill children on ECMO. Systematic evaluations of pharmacokinetic alterations of drugs on ECMO that incorporate multidrug opportunistic trials, physiologically based pharmacokinetic modeling, ex vivo studies, and other methods are necessary for definitive dose recommendations.
Data Availability Statement
All datasets generated for this study are included in the article/Supplementary Materials.
Author's Note
This manuscript has been released as a preprint at Research Square, Sutiman et al. (75).
Author Contributions
NS conceptualized and designed the study, obtained funding, designed the data collection instruments, collected data, carried out the analysis and interpretation of the data, and drafted and revised the manuscript. JL conceptualized and designed the study, obtained funding, collected data, carried out the analysis and interpretation of the data, and reviewed and revised the manuscript. JK collected data, carried out analysis, and interpretation of data and reviewed the manuscript. KW and CH collected data, carried out the analysis and interpretation of the data, and drafted, reviewed, and revised the manuscript. YC collected data, carried out the analysis and interpretation of the data, and reviewed and revised the manuscript. BM provided technical support, collected data, and carried out the analysis and interpretation of the data. All authors approved the final manuscript as submitted and agree to be accountable for all aspects of the work.
Funding
This work was supported by the SingHealth Medical Student Talent Development Award (SMSTDA) (FY2019 Cycle 1).
Conflict of Interest
The authors declare that the research was conducted in the absence of any commercial or financial relationships that could be construed as a potential conflict of interest.
Supplementary Material
The Supplementary Material for this article can be found online at: https://www.frontiersin.org/articles/10.3389/fped.2020.00260/full#supplementary-material
References
1. Shekar K, Roberts JA, Mcdonald CI, Fisquet S, Barnett AG, Mullany DV, et al. Sequestration of drugs in the circuit may lead to therapeutic failure during extracorporeal membrane oxygenation. Crit Care Lond Engl. (2012) 16:R194. doi: 10.1186/cc11679
2. Harthan AA, Buckley KW, Heger ML, Fortuna RS, Mays K. Medication adsorption into contemporary extracorporeal membrane oxygenator circuits. J Pediatr Pharmacol Ther. (2014) 19:288–95. doi: 10.5863/1551-6776-19.4.288
3. Shekar K, Roberts JA, Mcdonald CI, Ghassabian S, Anstey C, Wallis SC, et al. Protein-bound drugs are prone to sequestration in the extracorporeal membrane oxygenation circuit: results from an ex vivo study. Crit Care Lond Engl. (2015) 19:164. doi: 10.1186/s13054-015-0891-z
4. Mehta NM, Halwick DR, Dodson BL, Thompson JE, Arnold JH. Potential drug sequestration during extracorporeal membrane oxygenation: results from an ex vivo experiment. Intensive Care Med. (2007) 33:1018–24. doi: 10.1007/s00134-007-0606-2
5. Anderson HL, Coran AG, Drongowski RA, Ha HJ, Bartlett RH. Extracellular fluid and total body water changes in neonates undergoing extracorporeal membrane oxygenation. J Pediatr Surg. (1992) 27:1003–7; discussion 1007–8. doi: 10.1016/0022-3468(92)90547-K
7. Kanji S, Hayes M, Ling A, Shamseer L, Chant C, Edwards DJ, et al. Reporting guidelines for clinical pharmacokinetic studies: The ClinPK statement. Clin Pharmacokinet. (2015) 54:783–95. doi: 10.1007/s40262-015-0236-8
8. Ahsman MJ, Wildschut ED, Tibboel D, Mathot RA. Pharmacokinetics of cefotaxime and desacetylcefotaxime in infants during extracorporeal membrane oxygenation. Antimicrob Agents Chemother. (2010) 54:1734–41. doi: 10.1128/AAC.01696-09
9. Cohen P, Collart L, Prober CG, Fischer AF, Blaschke TF. Gentamicin pharmacokinetics in neonates undergoing extracorporal membrane oxygenation. Pediatr Infect Dis J. (1990) 9:562–6. doi: 10.1097/00006454-199008000-00007
10. Moffett BS, Morris J, Galati M, Munoz FM, Arikan AA. Population pharmacokinetic analysis of gentamicin in pediatric extracorporeal membrane oxygenation. Ther Drug Monit. (2018) 40:581–8. doi: 10.1097/FTD.0000000000000547
11. Southgate WM, DiPiro JT, Robertson AF. Pharmacokinetics of gentamicin in neonates on extracorporeal membrane oxygenation. Antimicrob Agents Chemother. (1989) 33:817–9. doi: 10.1128/AAC.33.6.817
12. Dodge WF, Jelliffe RW, Zwischenberger JB, Bellanger RA, Hokanson JA, Snodgrass WR. Population pharmacokinetic models: effect of explicit versus assumed constant serum concentration assay error patterns upon parameter values of gentamicin in infants on and off extracorporeal membrane oxygenation. Ther Drug Monit. (1994) 16:552–9. doi: 10.1097/00007691-199412000-00004
13. Munzenberger PJ, Massoud N. Pharmacokinetics of gentamicin in neonatal patients supported with extracorporeal membrane oxygenation. ASAIO J. (1991) 37:16–8. doi: 10.1097/00002216-199101000-00006
14. Bhatt-Mehta V, Johnson CE, Schumacher RE. Gentamicin pharmacokinetics in term neonates receiving extracorporeal membrane oxygenation. Pharmacotherapy. (1992) 12:28–32.
15. Cies JJ, Moore WS, Conley SB, Dickerman MJ, Small C, Carella D, et al. Pharmacokinetics of continuous infusion meropenem with concurrent extracorporeal life support and continuous renal replacement therapy: a case report. J Pediatr Pharmacol Ther. (2016) 21:92–7. doi: 10.5863/1551-6776-21.1.92
16. Alqaqaa Y, Witcher R, Ramirez M, Fisher J, Malaga-Dieguez L, Chopra A. 1851: Continuous infusion of meropenem in a patient on ECMO and CRRT. Crit Care Med. (2016) 44:538. doi: 10.1097/01.ccm.0000510524.47813.84
17. Cies JJ, Moore WS, Dickerman MJ, Small C, Carella D, Chopra A, et al. Pharmacokinetics of continuous-infusion meropenem in a pediatric patient receiving extracorporeal life support. Pharmacother J Hum Pharmacol Drug Ther. (2014) 34:e175–9. doi: 10.1002/phar.1476
18. Cies J, Chopra A. 702: piperacillin/tazobactam (PTZ) pharmacokinetics (PK) in critically ill children on extracorporeal membrane oxygenation (ECMO). Crit Care Med. (2011) 39:196. doi: 10.1097/01.ccm.0000408627.24229.88
19. Lindsay CA, Bawdon R, Quigley R. Clearance of ticarcillin-clavulanic acid by continuous venovenous hemofiltration in three critically ill children, two with and one without concomitant extracorporeal membrane oxygenation. Pharmacother J Hum Pharmacol Drug Ther. (1996) 16:458–62.
20. Zylbersztajn BL, Izquierdo G, Santana RC, Fajardo C, Torres JP, Cordero J, et al. Therapeutic drug monitoring of vancomycin in pediatric patients with extracorporeal membrane oxygenation support. J Pediatr Pharmacol Ther. (2018) 23:305–10. doi: 10.5863/1551-6776-23.4.305
21. Amaker RD, DiPiro JT, Bhatia J. Pharmacokinetics of vancomycin in critically ill infants undergoing extracorporeal membrane oxygenation. Antimicrob Agents Chemother. (1996) 40:1139–42. doi: 10.1128/AAC.40.5.1139
22. Buck ML. Vancomycin pharmacokinetics in neonates receiving extracorporeal membrane oxygenation. Pharmacother J Hum Pharmacol Drug Ther. (1998) 18:1082–6.
23. Cies JJ, Moore WS, Nichols K, Knoderer CA, Carella DM, Chopra A. Population pharmacokinetics and pharmacodynamic target attainment of vancomycin in neonates on extracorporeal life support. Pediatr Crit Care Med. (2017) 18:977–85. doi: 10.1097/PCC.0000000000001250
24. Moffett BS, Morris J, Galati M, Munoz F, Arikan AA. Population pharmacokinetics of vancomycin in pediatric extracorporeal membrane oxygenation*. Pediatr Crit Care Med. (2018) 19:973–80. doi: 10.1097/PCC.0000000000001682
25. Cies JJ. Neonatal vancomycin dosing on extracorporeal membrane oxygenation. Crit Care Med. (2010) A215. doi: 10.1097/01.ccm.0000390903.16849.8c
26. Chopra A, Miller K, Shea P, Conley S, Cies J. 1130: Continuous acyclovir for neonatal disseminated HSV with concurrent ECMO and CVVH circuits. Crit Care Med. (2013) 41:A286. doi: 10.1097/01.ccm.0000440364.04664.75
27. Eyler RF, Klein KC, Mueller BA. The pharmacokinetics of oseltamivir and oseltamivir carboxylate in a critically ill pediatric patient receiving extracorporeal membrane oxygenation and continuous venovenous hemodialysis. J Pediatr Pharmacol Ther. (2012) 17:173–6. doi: 10.5863/1551-6776-17.2.173
28. Aebi C, Headrick CL, McCracken GH, Lindsay CA. Intravenous ribavirin therapy in a neonate with disseminated adenovirus infection undergoing extracorporeal membrane oxygenation: pharmacokinetics and clearance by hemofiltration. J Pediatr. (1997) 130:612–5. doi: 10.1016/S0022-3476(97)70246-4
29. Koch BCP, Wildschut ED, Goede AL de, Hoog M de, Brüggemann RJM. Insufficient serum caspofungin levels in a paediatric patient on ECMO. Med Mycol Case Rep. (2012) 2:23–4. doi: 10.1016/j.mmcr.2012.12.006
30. Watt KM, Gonzalez D, Benjamin DK, Brouwer KLR, Wade KC, Capparelli E, et al. Fluconazole population pharmacokinetics and dosing for prevention and treatment of invasive candidiasis in children supported with extracorporeal membrane oxygenation. Antimicrob Agents Chemother. (2015) 59:3935–43. doi: 10.1128/AAC.00102-15
31. Watt KM, Benjamin DK, Cheifetz IM, Moorthy G, Wade KC, Smith PB, et al. Pharmacokinetics and safety of fluconazole in young infants supported with extracorporeal membrane oxygenation. Pediatr Infect Dis J. (2012) 31:1042–7. doi: 10.1097/INF.0b013e31825d3091
32. Autmizguine J, Hornik CP, Benjamin DK, Brouwer KLR, Hupp SR, Cohen-Wolkowiez M, et al. Pharmacokinetics and safety of micafungin in infants supported with extracorporeal membrane oxygenation. Pediatr Infect Dis J. (2016) 35:1204–10. doi: 10.1097/INF.0000000000001268
33. Brüggemann RJM, Antonius T, Heijst A van, Hoogerbrugge PM, Burger DM, Warris A. Therapeutic drug monitoring of voriconazole in a child with invasive aspergillosis requiring extracorporeal membrane oxygenation. Ther Drug Monit. (2008) 30:643. doi: 10.1097/FTD.0b013e3181898b0c
34. Spriet I, Annaert P, Meersseman P, Hermans G, Meersseman W, Verbesselt R, et al. Pharmacokinetics of caspofungin and voriconazole in critically ill patients during extracorporeal membrane oxygenation. J Antimicrob Chemother. (2009) 63:767–70. doi: 10.1093/jac/dkp026
35. Dillman NO, Messinger MM, Dinh KN, Placencia JL, Moffett BS, Guaman MC, et al. Evaluation of the effects of extracorporeal membrane oxygenation on antiepileptic drug serum concentrations in pediatric patients. J Pediatr Pharmacol Ther. (2017) 22:352–7. doi: 10.5863/1551-6776-22.5.352
36. Larochelle JM, Murvant MD, Creel AM, Tilton A. Levetiracetam use during extracorporeal membrane oxygenation in an adolescent patient. Crit Care Shock. (2016) 19:41–3.
37. Elliott ES, Buck ML. Phenobarbital dosing and pharmacokinetics in a neonate receiving extracorporeal membrane oxygenation. Ann Pharmacother. (1999) 33:419–22. doi: 10.1345/aph.18248
38. Pokorná P, Šíma M, Vobruba V, Tibboel D, Slanar O. Phenobarbital pharmacokinetics in neonates and infants during extracorporeal membrane oxygenation. Perfusion. (2018) 33:80–6. doi: 10.1177/0267659118766444
39. Wells TG, Fasules JW, Taylor BJ, Kearns GL. Pharmacokinetics and pharmacodynamics of bumetanide in neonates treated with extracorporeal membrane oxygenation. J Pediatr. (1992) 121:974–80. doi: 10.1016/S0022-3476(05)80355-5
40. Kleiber N, Mathôt RAA, Ahsman MJ, Wildschut ED, Tibboel D, de Wildt SN. Population pharmacokinetics of intravenous clonidine for sedation during paediatric extracorporeal membrane oxygenation and continuous venovenous hemofiltration. Br J Clin Pharmacol. (2017) 83:1227–39. doi: 10.1111/bcp.13235
41. Grimaud M, Urien S, Borgel D, Amar G, Gaudin R, Burgos P, et al. Abstract P-114: pharmacokinetic analysis of unfractionated heparin in critically ill children during extracorporeal membrane oxygenation do we achieve the target? Pediatr Crit Care Med. (2018) 19:83–4. doi: 10.1097/01.pcc.0000537571.01928.c4
42. Mulla H, Lawson G, Peek GJ, Firmin RK, Upton DR. Plasma concentrations of midazolam in neonates receiving extracorporeal membrane oxygenation. ASAIO J. (2003) 49:41–7. doi: 10.1097/00002480-200301000-00007
43. Ahsman MJ, Hanekamp M, Wildschut ED, Tibboel D, Mathot RAA. Population pharmacokinetics of midazolam and its metabolites during venoarterial extracorporeal membrane oxygenation in neonates. Clin Pharmacokinet. (2010) 49:407–19. doi: 10.2165/11319970-000000000-00000
44. Peters JWB, Anderson BJ, Simons SHP, Uges DRA, Tibboel D. Morphine metabolite pharmacokinetics during venoarterial extra corporeal membrane oxygenation in neonates. Clin Pharmacokinet. (2006) 45:705–14. doi: 10.2165/00003088-200645070-00005
45. Geiduschek JM, Lynn AM, Bratton SL, Sanders JC, Levy FH, Haberkern CM, et al. Morphine pharmacokinetics during continuous infusion of morphine sulfate for infants receiving extracorporeal membrane oxygenation. Crit Care Med. (1997) 25:360. doi: 10.1097/00003246-199702000-00027
46. Wells TG, Heulitt MJ, Taylor BJ, Fasules JW, Kearns GL. Pharmacokinetics and pharmacodynamics of ranitidine in neonates treated with extracorporeal membrane oxygenation. J Clin Pharmacol. (1998) 38:402–7. doi: 10.1002/j.1552-4604.1998.tb04443.x
47. Ahsman MJ, Witjes BC, Wildschut ED, Sluiter I, Vulto AG, Tibboel D, et al. Sildenafil exposure in neonates with pulmonary hypertension after administration via a nasogastric tube. Arch Dis Child Fetal Neonatal Ed. (2010) 95:F109–14. doi: 10.1136/adc.2009.168336
48. Mulla H, McCormack P, Lawson G, Firmin RK, Upton DR. Pharmacokinetics of midazolam in neonates undergoing extracorporeal membrane oxygenation. Anesthesiol J Am Soc Anesthesiol. (2003) 99:275–82. doi: 10.1097/00000542-200308000-00008
49. Cies JJ, Moore WS, Enache A, Chopra A. Population pharmacokinetics and pharmacodynamic target attainment of meropenem in critically ill young children. J Pediatr Pharmacol Ther. (2017) 22:276–85. doi: 10.5863/1551-6776-22.4.276
50. Donadello K, Roberts JA, Cristallini S, Beumier M, Shekar K, Jacobs F, et al. Vancomycin population pharmacokinetics during extracorporeal membrane oxygenation therapy: a matched cohort study. Crit Care Lond Engl. (2014) 18:632. doi: 10.1186/s13054-014-0632-8
51. Maksoud E, Koehl B, Facchin A, Ha P, Zhao W, Kaguelidou F, et al. Population pharmacokinetics of cefotaxime and dosage recommendations in children with sickle cell disease. Antimicrob Agents Chemother. (2018) 62:e00637–17. doi: 10.1128/AAC.00637-17
52. Gouyon JB, Pechinot A, Safran C, Chretien P, Sandre D, Kazmierczak A. Pharmacokinetics of cefotaxime in preterm infants. Dev Pharmacol Ther. (1990) 14:29–34. doi: 10.1159/000480936
53. Pettit RS, Neu N, Cies JJ, Lapin C, Muhlebach MS, Novak KJ, et al. Population pharmacokinetics of meropenem administered as a prolonged infusion in children with cystic fibrosis. J Antimicrob Chemother. (2016) 71:189–95. doi: 10.1093/jac/dkv289
54. Cies JJ, Shankar V, Schlichting C, Kuti JL. Population pharmacokinetics of piperacillin/tazobactam in critically ill young children. Pediatr Infect Dis J. (2014) 33:168–73. doi: 10.1097/INF.0b013e3182a743c7
55. Watt KM, Hornik CP, Balevic SJ, Mundakel G, Cotten CM, Harper B, et al. Pharmacokinetics of ticarcillin-clavulanate in premature infants. Br J Clin Pharmacol. (2019) 85:1021–7. doi: 10.1111/bcp.13882
56. Sampson MR, Bloom BT, Lenfestey RW, Harper B, Kashuba AD, Anand R, et al. Population pharmacokinetics of intravenous acyclovir in preterm and term infants. Pediatr Infect Dis J. (2014) 33:42–9. doi: 10.1097/01.inf.0000435509.75114.3d
57. Kimberlin DW, Acosta EP, Prichard MN, Sánchez PJ, Ampofo K, Lang D, et al. Oseltamivir pharmacokinetics, dosing, and resistance among children aged <2 years with influenza. J Infect Dis. (2013) 207:709–20. doi: 10.1093/infdis/jis765
58. Connor E, Morrison S, Lane J, Oleske J, Sonke RL, Connor J. Safety, tolerance, and pharmacokinetics of systemic ribavirin in children with human immunodeficiency virus infection. Antimicrob Agents Chemother. (1993) 37:532–9. doi: 10.1128/AAC.37.3.532
59. McJunkin JE, Nahata MC, De Los Reyes EC, Hunt WG, Caceres M, Khan RR, et al. Safety and pharmacokinetics of ribavirin for the treatment of la crosse encephalitis. Pediatr Infect Dis J. (2011) 30:860–5. doi: 10.1097/INF.0b013e31821c922c
60. Neely M, Jafri HS, Seibel N, Knapp K, Adamson PC, Bradshaw SK, et al. Pharmacokinetics and safety of caspofungin in older infants and toddlers. Antimicrob Agents Chemother. (2009) 53:1450–6. doi: 10.1128/AAC.01027-08
61. Leroux S, Jacqz-Aigrain E, Elie V, Legrand F, Barin-Le Guellec C, Aurich B, et al. Pharmacokinetics and safety of fluconazole and micafungin in neonates with systemic candidiasis: a randomized, open-label clinical trial. Br J Clin Pharmacol. (2018) 84:1989–99. doi: 10.1111/bcp.13628
62. Shin JW, Jung YS, Park K, Lee SM, Eun HS, Park MS, et al. Experience and pharmacokinetics of Levetiracetam in Korean neonates with neonatal seizures. Korean J Pediatr. (2017) 60:50–4. doi: 10.3345/kjp.2017.60.2.50
63. Chhun S, Jullien V, Rey E, Dulac O, Chiron C, Pons G. Population pharmacokinetics of levetiracetam and dosing recommendation in children with epilepsy. Epilepsia. (2009) 50:1150–7. doi: 10.1111/j.1528-1167.2008.01974.x
64. Sullivan JE, Witte MK, Yamashita TS, Myers CM, Blumer JL. Pharmacokinetics of bumetanide in critically ill infants. Clin Pharmacol Ther. (1996) 60:405–13. doi: 10.1016/S0009-9236(96)90197-6
65. Marshall JD, Wells TG, Letzig L, Kearns GL. Pharmacokinetics and pharmacodynamics of bumetanide in critically ill pediatric patients. J Clin Pharmacol. (1998) 38:994–1002. doi: 10.1177/009127009803801102
66. de Wildt SN, de Hoog M, Vinks AA, van der Giesen E, van den Anker JN. Population pharmacokinetics and metabolism of midazolam in pediatric intensive care patients. Crit Care Med. (2003) 31:1952–8. doi: 10.1097/01.ccm.0000084806.15352.da
67. Wildt SN de, Kearns GL, Hop WCJ, Murry DJ, Abdel-Rahman SM, van den Anker JN. Pharmacokinetics and metabolism of intravenous midazolam in preterm infants. Clin Pharmacol Ther. (2001) 70:525–31. doi: 10.1067/mcp.2001.120683
68. Asseff IL, Gaucin GB, Olguín HJ, Nájera JAG, López AT, Guillé GP, et al. Pharmacokinetics of ranitidine in preterm and term neonates with gastroesophageal reflux. BMC Pediatr. (2016) 16:90. doi: 10.1186/s12887-016-0630-x
69. Olguín HJ, Martínez HO, Pérez CF, Mendiola BR, Espinosa LR, Pacheco JLC, et al. Pharmacokinetics of sildenafil in children with pulmonary arterial hypertension. World J Pediatr. (2017) 13:588–92. doi: 10.1007/s12519-017-0043-4
70. Raffaeli G, Pokorna P, Allegaert K, Mosca F, Cavallaro G, Wildschut ED, et al. Drug disposition and pharmacotherapy in neonatal ECMO: from fragmented data to integrated knowledge. Front Pediatr. (2019) 7:360. doi: 10.3389/fped.2019.00360 [cited: Apr 19, 2020].
71. Smith BS, Yogaratnam D, Levasseur-Franklin KE, Forni A, Fong J. Introduction to drug pharmacokinetics in the critically III patient. Chest. (2012) 141:1327–36. doi: 10.1378/chest.11-1396
73. Pistolesi V, Morabito S, Di Mario F, Regolisti G, Cantarelli C, Fiaccadori E. A guide to understanding antimicrobial drug dosing in critically ill patients on renal replacement therapy. Antimicrob Agents Chemother. (2019) 63:e00583-19. doi: 10.1128/AAC.00583-19
74. Churchwell MD, Mueller BA. The clinical application of CRRT-current status: drug dosing during continuous renal replacement therapy. Semin Dial. (2009) 22:185–8. doi: 10.1111/j.1525-139X.2008.00541.x
Keywords: ECMO, extracorporeal, pharmacokinetics, disposition, pediatrics, critically ill
Citation: Sutiman N, Koh JC, Watt K, Hornik C, Murphy B, Chan YH and Lee JH (2020) Pharmacokinetics Alterations in Critically Ill Pediatric Patients on Extracorporeal Membrane Oxygenation: A Systematic Review. Front. Pediatr. 8:260. doi: 10.3389/fped.2020.00260
Received: 29 March 2020; Accepted: 27 April 2020;
Published: 26 June 2020.
Edited by:
Graeme MacLaren, National University Hospital, SingaporeReviewed by:
Ravi R. Thiagarajan, Harvard Medical School and Boston Children's Hospital, United StatesKarel Allegaert, University Hospitals Leuven, Belgium
Copyright © 2020 Sutiman, Koh, Watt, Hornik, Murphy, Chan and Lee. This is an open-access article distributed under the terms of the Creative Commons Attribution License (CC BY). The use, distribution or reproduction in other forums is permitted, provided the original author(s) and the copyright owner(s) are credited and that the original publication in this journal is cited, in accordance with accepted academic practice. No use, distribution or reproduction is permitted which does not comply with these terms.
*Correspondence: Jan Hau Lee, lee.jan.hau@singhealth.com.sg