- 1Department of Congenital Heart Disease and Pediatric Cardiology, German Heart Center Munich, Technical University Munich, Munich, Germany
- 2Faculty of Sport and Health Sciences, Institute of Preventive Pediatrics, Technical University Munich, Munich, Germany
- 3Department of Pediatric Hematology and Oncology, Dr. von Hauner Children's Hospital, Ludwig-Maximilians-University Munich, Munich, Germany
Background: Survivors of childhood cancer are at risk for anthracycline- and/or radiotherapy-induced cardiotoxicity.
Aims: The aim of this study was to assess clinical, laboratory, and imaging parameters of subclinical cardiovascular disease in childhood cancer survivors.
Methods: Patients underwent cardiopulmonary exercise test (CPET), laboratory testing, transthoracic echocardiography (TTE) with tissue doppler imaging (TDI) and speckle tracking. A subset of patients also underwent cardiovascular magnetic resonance imaging (CMR). Findings were correlated to cumulative anthracycline and exposure to mediastinal irradiation during cancer treatment. In a subgroup analysis, TTE and CMR findings were compared to data from 40 gender- and age-matched patients with childhood onset hypertrophic cardiomyopathy (HCM).
Results: Cardiac evaluation was performed in 79 patients (43 males) at 11.2 ± 4.5 years after cancer treatment. Oncologic diagnosis at a median age of 12.0 years was Hodgkin lymphoma in 20, sarcoma in 17, acute leukemia in 24, relapse leukemia in 10, and others in 8 patients. Cumulative anthracycline dose exceeded 300 mg/m2 in 28 patients. Twenty six patients also received mediastinal irradiation. Decreased peak respiratory oxygen uptake in % predicted on CPET, increased levels of N-terminal pro-brain natriuretic peptide (NTproBNP), increased global longitudinal strain on TTE speckle tracking, and diastolic dysfunction on TDI were the most prominent findings on detailed cardiology follow-up. In contrast to HCM patients, childhood cancer survivors did not show left ventricular hypertrophy (LVPWd z-score median 0.9 vs. 2.8, p < 0.001), hyperdynamic systolic function on TTE (Ejection fraction 62 ± 7 vs. 72 ± 12%, p = 0.001), or fibrotic myocardial changes on CMR (Late gadolinium positive 0/13 vs. 13/36, p = 0.001; extracellular volume fraction 22 ± 2 vs. 28 ± 3, p < 0.001) at time of follow-up. There was no correlation between chest radiation exposure and abnormal cardiac findings. Cumulative anthracycline dose was the only significant independent predictor on multivariate analysis for any cardiovascular abnormality on follow-up (p = 0.036).
Conclusion: Increasing cumulative anthracycline dose during cancer treatment correlates with subclinical cardiac dysfunction in childhood cancer survivors best detected by elevated cardiac serum biomarkers, decreased exercise capacity on CPET, and abnormalities on echocardiographic speckle tracking and TDI.
Introduction
Due to advancements in treatment, survival rates for childhood cancer have substantially improved during the past decades with an overall 5-year survival rate of over 80% today (1–3). More than half of childhood cancer patients receive anthracyclines as part of their cancer therapy (4) and anthracycline-mediated cardiovascular complications contribute to morbidity and mortality in the growing population of childhood cancer survivors [reviewed in Franco et al., (5), Raj et al., (6), Todaro et al., (7), Van Der Pal et al., (8) and (9)]. Anthracyclines express their antiproliferation of cancer cell effects through inhibition of DNA replication, RNA replication, DNA cross-linking, and topoisomerases (10). The pathophysiology of anthracycline mediated cardiotoxicity is multifactorial and involves multiple mechanisms. Oxidative stress (11–14), induction of apoptosis, formation of toxic metabolites, altered cardiomyocyte gene expression, transcription and translation (15–18), as well as impaired oxidative phosphorylation and adenosine triphosphate (ATP) synthesis (19), have been suggested to play a major role in anthracycline-induced cardiotoxicity. Survivors of childhood cancer are especially vulnerable to these risks given their young age at the time of treatment and the exposure of cardiotoxicity of their growing and developing heart. Exposure to mediastinal irradiation might contribute additionally to cardiotoxic effects (8, 20–24). Cardiotoxicity can manifest at an early stage of therapy (during or immediately after treatment) (25) or many years thereafter (9, 26). The clinical presentation of childhood cancer survivors suffering from cancer-treatment related cardiotoxicity varies from asymptomatic cardiac dysfunction to overt congestive heart failure (8, 9, 20). Other cardiovascular sequelae include coronary artery disease, stroke, arrhythmias, and valvular and vascular dysfunction (20). There is now a growing body of evidence that genetic risk factors might contribute to the large variability of cardiotoxic disease in individuals (27–30).
The primary prevention of long-term adverse effects of childhood cancer treatment includes modification of therapeutic exposures (31–33), the concomitant use of cardioprotective therapies (34–37), or the use of alternative chemotherapeutics with maximal efficacy and minimal short- and long-term adverse effects (36).
Secondary prevention after completion of anticancer treatment, such as improved surveillance, appropriate screening strategies, and preventative therapies for cardiovascular complications, require an interdisciplinary approach between oncologists and cardiologist.
Standardized clinical evaluation with regular follow-up and assessment of the entire cardiovascular system is necessary in childhood cancer survivors (38). However, even though consensus-based guidelines for cardiotoxicity monitoring following chemotherapy using echocardiography exist (38), the optimal timing and cost-effectiveness for such monitoring needs further investigation (39, 40). Identification of subclinical cardiotoxicity can be challenging (41).
Data are scarce with regards to the optimal use of serum biomarkers, the mode and sensitivity of distinct imaging modalities, and optimal management strategies once subclinical cardiotoxicity has been detected, specifically in children.
The aim of the study was to assess laboratory, cardiopulmonary exercise testing, echocardiographic, and cardiac magnetic resonance imaging findings in clinically asymptomatic childhood cancer survivors on long-term follow-up. The identification of monitoring parameters shall optimize risk stratification, identify subclinical cardiovascular disease, and enable preventative treatment measures in time before overt clinical disease in childhood cancer survivors becomes evident.
Materials and Methods
Study Design
In this retrospective single tertiary care center study, medical charts were reviewed from all childhood cancer survivors followed at the pediatric cardiology outpatient unit at the German Heart Center Munich between March 2015 and October 2018. Demographic, clinical, laboratory, transthoracic echocardiographic (TTE), cardiopulmonary exercise testing (CPET), cardiovascular magnetic resonance (CMR), and other patient-related data were collected retrospectively by review of patients' charts at last follow-up.
Patient Population
Data from a total of 79 patients with a primary diagnosis of childhood cancer and initial cancer treatment between 0 and 18 years of age were analyzed. For subgroup analysis, TTE and CMR findings were compared with 40 gender- and age-matched patients with childhood onset hypertrophic cardiomyopathy (HCM). The diagnosis of HCM was based on clinical evaluation and cardiac catheterization or TTE detecting myocardial hypertrophy (defined as a z-score of >2) in the absence of another cardiac or systemic disease causing the degree of left ventricular hypertrophy identified (42, 43). This patient cohort was selected for comparison to evaluate similarities and differences between cardiomyopathic changes according to underlying etiology. Data were collected as previously described (44).
Cardiopulmonary Exercise Testing
CPET was performed in sitting position on a bike-ergometer. A standardized testing protocol was performed and physical working capacity, peak oxygen uptake (VO2 max), evidence of myocardial ischemia or arrhythmia and escalation of heart rate or blood pressure during or after exertion were recorded (45). For the peak respiratory oxygen uptake in % predicted [VO2 max (% norm)], a cut-off level of <80 was determined as “abnormal.”
Serum Biomarkers
The serum biomarkers cardiac troponin T (cTNT) and N-terminal pro-brain natriuretic peptide (NTproBNP) levels were determined as marker for early systolic dysfunction or myocardial stress. Values >0.01 ng/dL and >100 ng/dL, respectively, were considered as “elevated.”
Transthoracic Echocardiography
Echocardiograms were obtained at the time of outpatient visits and were remeasured by blinded study staff (AK). TTE was performed using standard equipment in routine clinical practice and using standard views according to the American Society of Echocardiography guidelines (46–48). Echopac Software (General Electric, Vingmed, Horten, Norway) was used for offline analysis. Measurements of left ventricular wall thickness and enddiastolic ventricular diameters were standardized to body surface area and given as z-scores [http://parameterz.blogspot.com/2008/09/z-scores-of-cardiac-structures.html, (49)]. Z-scores of <-2 or > +2 are considered as “abnormal,” myocardial hypertrophy is defined as a z-score of >2 (42). Global longitudinal strain (GLS) was assessed by speckle tracking and diastolic function by tissue and pulse wave Doppler echocardiography on apical four-chamber views at the lateral and septal mitral valve annulus as previously described (48, 50). Reference values for normal and abnormal measurements were extracted from literature of large gender- and age-matched cohorts with similar ethnicity (49, 51–53). An ejection fraction (EF) of <50 (51) and GLS >-20 (53, 54) were considered as abnormal. Left atrial (LA) volume standardized to body surface area (BSA) of >34 ml/m2 was considered as enlarged (51). Mitral valve E/A ratio of >2 and a septal E/E' ratio of >8 was considered as abnormal (52, 55).
Cardiovascular Magnetic Resonance Tomography
CMR was performed on a 1.5 Tesla MR scanner (Magnetom Avanto, Siemens Healthcare, Software Version VD13, Erlangen, Germany). Cine images (balanced steady-state free precession) were acquired in short axis and four chamber orientations in breath hold to evaluate the ventricular volume, ventricular mass, ejection fraction, and regional wall anomalies. Late Gadolinium Enhancement (LGE) was acquired using a T1-weighted phase-sensitive inversion recovery sequence 10 min after intravenous administration of an extracellular MR contrast agent (Gadopentetat) to detect focal fibrosis (56).
Native and post contrast T1 mapping, using a modified look-locker inversion recovery sequence (MOLLI) (57), with non-rigid motion correction reconstruction, were assessed in short axis and four chamber orientation. Image quality was assessed by revising T1 maps and error maps of the region of interest. Extracellular volume (ECV) was calculated as previously described (58). An ECV >25 was considered as abnormal.
Ethics
The study was conducted in accordance with the Declaration of Helsinki (revision 2008) and the Good Clinical Practice guidelines. The study protocol was approved by the local ethical board of the Technical University Munich (ethical approval number 243/17S, 10/16/2017).
Statistics
Data are presented as mean and standard deviation or as median and minimum / maximum according to distribution. Kolmogorov-Smirnoff Test was used to assess distribution of variances. Independent t-Test and Mann-Whitney-Test were used according to data distribution to compare parameters between the two groups. ANOVA (analysis of variances) or Kruskall-Wallis-test were used to compare differences between multiple groups (oncologic diagnosis) according to data distribution. Fisher's Test or Pearson chi-square was used for comparison of categorical variables. Pearson correlation was used to assess the correlation between anthracycline dose, radiation exposure, age at cancer treatment, age at follow-up, follow-up time, and cardiovascular findings. Binary outcome variables (abnormal or normal) were created using cut-off for the respective variables. Backward multivariate binary logistic regression analysis was done for the categorical variable “Any cardiovascular abnormality” defined as decreased exercise capacity, NTproBNP level, or increased septal E/E' ratio on TDI as dependent variable and cumulative anthracycline dose, radiation exposure, gender, age at cancer treatment, age at follow-up, and follow-up time as covariates. Cut-off levels of cumulative anthracycline dose predictive for any cardiovascular abnormality on follow-up were derived from the Receiver operating characteristic (ROC) curve. The significance level of p-value was set at <0.05. Data were analyzed on IBM SPSS Version 25.
Results
Patient Characteristics
Demographic and clinical patient characteristics are summarized in Table 1. A detailed cardiac evaluation was performed in 79 patients (43 males) at 11.2 ± 4.5 years after cancer treatment. Underlying oncologic diagnosis was acute (N = 24) or relapse leukemia (N = 10), Hodgkin lymphoma (N = 20), sarcoma (N = 17), nephroblastoma (N = 4), neuroblastoma (N = 3), and hepatoblastoma (N = 1). Median age at primary oncologic diagnosis was 12.0 years. Mean cumulative anthracycline dose was 261±104 mg/m2 and exceeded 300 mg/m2 in 36% of patients. Patients with sarcomas received significantly higher doses of anthracycline compared to the remaining patients. Twenty-six patients, most of them with Hodgkin lymphomas and relapse ALL, also received mediastinal irradiation with heart exposure. All patients with relapse ALL and one patient with ALL received mediastinal irradiation in the setting of total body irradiation for preparation to bone marrow transplant (Table 1). Cumulative ionizing radiation dose was <30 Gray in all these patients.
Cardiovascular Findings in Childhood Cancer Survivors
All patients were clinically asymptomatic and in NYHA functional class I at the time of follow-up. Abnormal findings on detailed cardiovascular examination in childhood cancer survivors included decreased exercise capacity on CPET, increased NTproBNP levels on laboratory testing, impaired global longitudinal strain on TTE speckle tracking, and evidence of diastolic dysfunction on tissue Doppler echocardiography (Figure 1). No patient had a medical history or clinical evidence of significant pulmonary disease. Decreased exercise capacity as shown by a peak respiratory oxygen uptake in % predicted (VO2 max) of <80 on CPET was evident in a third of patients. Cardiac troponin T levels were normal in all patients. NTproBNP levels >100 ng/dL were measured in 29% of childhood cancer survivors. Whereas, left ventricular systolic function (EF) and left ventricular dimensions were normal on TTE in most patients, there were abnormal GLS measurements in off-line echocardiographic speckle tracking analysis in about 28% of patients. No left atrial enlargement was documented on TTE, but pulse wave and tissue Doppler examination showed signs of diastolic dysfunction in 21, 24, and 11%, respectively (abnormal MV E/A ratio, E deceleration time and septal E/E'. There was no evidence of focal (LGE positive) or interstitial (increased ECV on T1 map) myocardial fibrosis on CMR imaging (Figure 1).
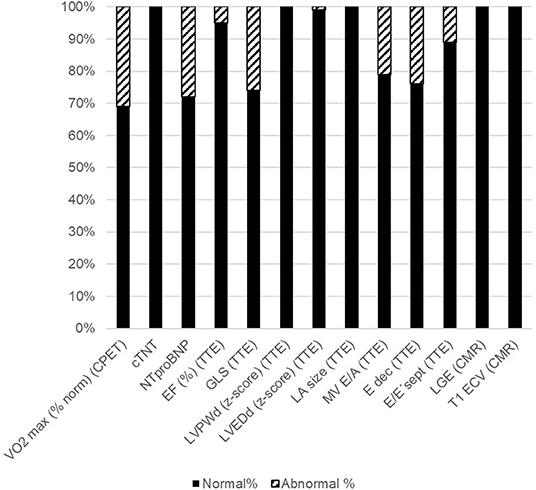
Figure 1. Abnormal cardiovascular parameters. Shown are the percentages of childhood cancer survivors with normal cardiovascular parameters (black bars) and those with abnormal cardiovascular parameters (striped bars). Please see methods section for cut-off values. CPET: cardiopulmonary exercise testing; VO2 max (% norm): peak respiratory oxygen uptake in % predicted; EF, Ejection Fraction; GLS, Global longitudinal strain; LVPWd, Enddiastolic left ventricular posterior wall dimension; LVEDd, Left ventricular enddiastolic diameter; LA, left atrium; MV, Mitral valve; CMR, cardiovascular magnetic resonance imaging; LGE, Late gadolinium enhancement; ECV, extracellular volume fraction.
TTE and CMR data of childhood cancer survivors were compared to age- and gender-matched patients with an underlying diagnosis of childhood hypertrophic cardiomyopathy (HCM) secondary to mutations in sarcomere genes. The majority of HCM patients carried a pathogenic variant in either the MYH7 (14/40, 35%) or the MYBPC3 (12/40, 30%) gene. Other genes affected included TNNT2, TNNI3, ML2, TPM1 in 7 of 40 HCM patients (17.5%) and multiple mutations were detected in 4 of 40 HCM patients (10 %). Molecular diagnostic testing was negative in 3 of 40 HCM patients (7.5 %). Left ventricular outflow tract obstruction was present in 14 of 40 HCM patients (35 %). In contrast to HCM patients, childhood cancer survivors did not show left ventricular hypertrophy or hyperdynamic systolic function on TTE (Table 2). Fibrotic myocardial changes, such as focal fibrosis assessed by the presence of LGE on CMR and interstitial fibrosis assessed by increased ECV on CMR T1 map, were observed in a subset of HCM patients but not in childhood cancer survivors at the time of follow-up (Table 2). Evidence of diastolic dysfunction on echocardiographic pulse wave and tissue Doppler was observed in both groups, but only HCM and not childhood cancer survivor patients presented with enlarged standardized left atrial volumes (Table 2). Enlarged left atria documented in HCM patients occurred independently from moderate mitral regurgitation noted in only 3 of the 10 HCM patients with enlarged left atria. No higher degree valve stenosis was noted in HCM patients.
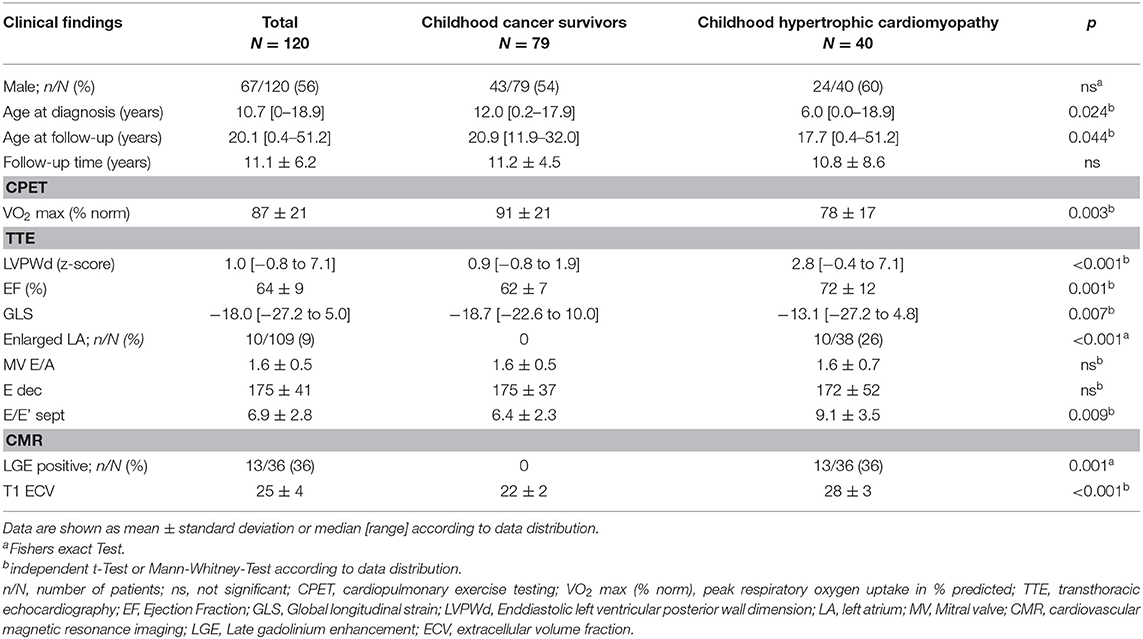
Table 2. Cardiovascular findings of childhood cancer survivors compared to childhood hypertrophic cardiomyopathy patients.
Univariate Analysis of Risk Factors for Abnormal Clinical Features
Patients were grouped into five categories according to oncologic diagnosis and variance analysis was carried out to assess if there were differences of abnormalities in cardiovascular findings between groups. There were significant differences between oncologic groups concerning decreased exercise capacity, NTproBNP levels and evidence of diastolic dysfunction on echocardiographic tissue Doppler imaging (mitral valve septal E/E'). Elevated NTproBNP levels and evidence for diastolic dysfunction were mostly observed in patients treated for sarcoma (Table 3).
There was no influence of age of diagnosis, age at follow-up and follow-up time on cardiac features on Pearson's correlation in univariate analysis.
There was a positive correlation between increasing cumulative anthracycline doses and decreased exercise capacity on CPET (Figure 2A). Septal E/E' ratio on pulse wave and tissue Doppler echocardiography as a correlate of diastolic function increased significantly with increasing cumulative anthracycline doses (Figure 2B). There was a trend toward increased NTproBNP levels on Pearson correlation (Figure 2C), and patients with increased NTproBNP levels had received a significantly higher cumulative anthracycline dose compared to patients with normal NTproBNP levels Figure 3A). Increasing NTproBNP values correlated with decreasing exercise capacity, decreasing echocardiographic systolic left ventricular function, and increasing echocardiographic diastolic dysfunction parameters (Figures 3B–D). Patients with abnormal cardiovascular findings were exposed to a significantly higher amount of cumulative anthracycline compared to patients with normal evaluations (cumulative anthracycline dose 294 ± 105 mg/m2 vs. 231 ± 95 mg/m2, p = 0.007, independent t-Test).
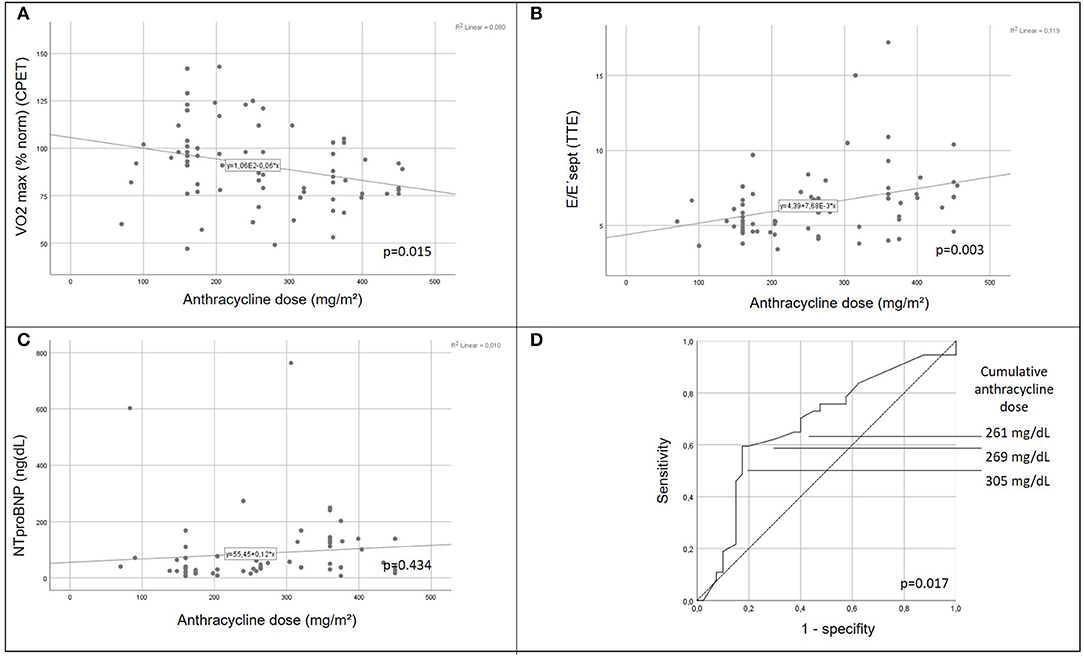
Figure 2. Correlation of cardiomyopathic changes with anthracycline dose. Peak respirator oxygen uptake on cardiopulmonary exercise testing (A) decreases and septal mitral valve E/E' ratio (B) increases significantly with increasing cumulative anthracycline dose (p = 0.015 and p = 0.003, respectively, pearson chi square). Trend to increased NTproBNP values with increasing cumulative anthracycline dose (C). Receiver operating characteristic (ROC) curve illustrating the ability of cumulative anthracycline dose predicting any cardiovascular abnormality (decreased exercise capacity, increased NTproBNP level, or abnormal tissue Doppler septal E/E'ratio) on follow-up (D). CPET, cardiopulmonary exercise testing; VO2 max (% norm), peak respiratory oxygen uptake in % predicted; EF, Ejection Fraction; TTE, transthoracic echocardiography.
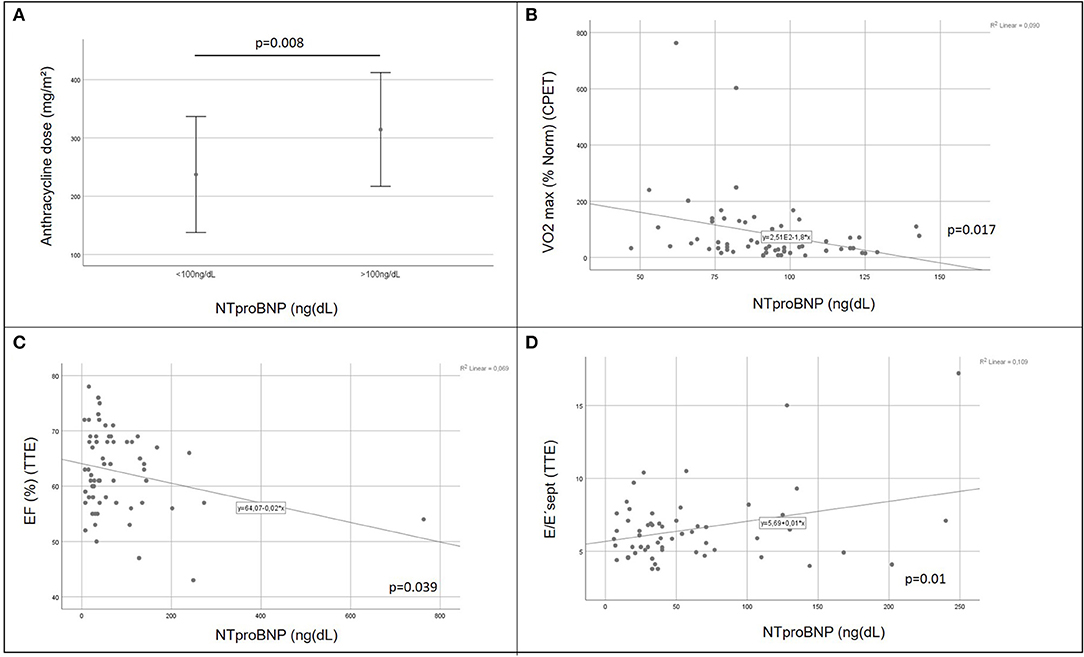
Figure 3. Role of the laboratory biomarker NTproBNP in cardiovascular impairment of childhood cancer survivors. Patients with elevated NTproBNP values were exposed to significantly higher doses of cumulative anthracycline compared to patients with NTproBNP values within the normal range [(A) independent t-Test 0.008]; increasing NTproBNP values correlated with decreasing exercise capacity [VO2 max (% norm), (B) Pearson correlation p = 0.017], decreasing echocardiographic systolic left ventricular function [EF, (C) Pearson correlation p = 0.039], and increasing echocardiographic diastolic dysfunction parameters [tissue Doppler septal E/E'ratio, (D) Pearson correlation p = 0.01]; CPET, cardiopulmonary exercise testing; VO2 max (% norm), peak respiratory oxygen uptake in % predicted; EF, Ejection Fraction; TTE, transthoracic echocardiography.
There was no significant correlation between cumulative anthracycline dose and decreased systolic echocardiographic function as shown by TTE ejection fraction (EF) on follow-up.
There was no correlation between exposure to mediastinal irradiation or exposure to total body irradiation and abnormal cardiac findings in univariate analysis. Patients with relapse ALL who were all exposed to mediastinal radiation in the setting of TBI for bone marrow transplantation conditioning had also received higher cumulative anthracycline doses, so that mediastinal radiation exposure was eliminated as independent predictor on multivariate analysis (see below).
Multivariate Analysis and Identification of Predictors for Risk Stratification
Increased cumulative anthracycline dose during cancer treatment was the only independent predictor for any cardiovascular abnormality (decreased exercise capacity or elevated NTproBNP or evidence of diastolic dysfunction) after elimination of influencing factors, such as oncologic diagnosis, gender, age at diagnosis, age at follow-up, follow-up time or exposure to irradiation, on multivariate logistic regression analysis (p = 0.036). Cut-off levels of the cumulative anthracycline dose associated with any cardiac abnormality on follow-up examination on the ROC-curve were 261, 269, and 305 mg/dL for a true positive prediction rate (sensitivity) of 62.2, 59.5, and 54.1%, and a false-positive prediction rate (1- specificity) of 30, 20, and 17.5%, respectively (Figure 2D).
Discussion
This study reports the results of a detailed cardiovascular examination in a large group of clinically asymptomatic childhood cancer survivors on long-term follow-up. All patients were in NYHA functional class I and had a normal echocardiographic ejection fraction. Subclinical cardiovascular disease was evident by increased circulating NTproBNP levels, decreased exercise capacity, increased global longitudinal strain on echocardiographic speckle tracking, and evidence of diastolic dysfunction on echocardiographic tissue Doppler. The only independent predictor for any abnormal cardiovascular finding identified on multivariate analysis was the amount of cumulative anthracycline dose administered during cancer treatment. Major findings include the absence of overt clinical signs but the presence of subtle impairment of cardiovascular function most prevalent in patients late after successful treatment of sarcoma, as well as a correlation of these subclinical findings to the exposure of higher amounts of anthracycline during cancer treatment. When comparing patients with abnormal cardiovascular findings to those without, the cumulative anthracycline dose was close to 300 mg/m2 in those who showed cardiovascular impairment. The data support a threshold of >300 mg/m2 as critical cumulative anthracycline dose that puts patients at a higher risk for later myocardial remodeling (59–62).
No correlation was found between mediastinal irradiation exposure and abnormal cardiovascular findings in the current study, although this has previously been suggested by others (8, 9, 63). Two thirds of patients with Hodgkin disease were exposed to mediastinal irradiation, but their cumulative anthracycline dose was lower compared to the other groups and they displayed the lowest amount of any cardiovascular abnormalities. Cardiovascular abnormalities occurred more frequently in patients with relapse ALL who were all exposed to mediastinal irradiation. However, those patients had also received higher doses of cumulative anthracycline because of recurrence of disease. Since the current analysis did not reveal any correlation with abnormal cardiovascular findings and exposure to mediastinal irradiation after correction for cumulative anthracycline dose, gender, age, height and weight differences, the patients were evaluated as one group to assess correlation with anthracycline dose. According to others (63) there is a high risk of developing cardiomyopathy if >15 Gray of mediastinal irradiation is given in addition to >100 mg/m2 of cumulative anthracycline doses. Not detecting an aggravating effect from radiotherapy in the current study may be because the time of 11.2 ± 4.5 years from cancer diagnosis to the follow-up cardiac examination was rather short, since deteriorating effects from mediastinal irradiation are expected to occur at a later point in time (8).
The reported positive correlation of decreased exercise capacity on CPET with increasing cumulative anthracycline dose and increasing NTproBNP levels contributes to the large body of evidence about impaired exercise capacity in childhood cancer survivors (64–66) and supports the value of CPET in identifying subclinical changes (67).
The role of circulating biomarkers as predictors for subclinical cardiovascular disease in childhood cancer survivors has been suggested by others (68). Serum cardiac troponins are widely used in assessing myocardial damage in adults and children (69). No patient had elevated cardiac troponin T (cTNT) levels in the current study providing evidence for the absence of any acute ischemic or clinically overt myocardial disease. Thus, cardiac troponin T does not seem suitable to detect cardiac sequelae late after childhood cancer treatment. In contrast, NTproBNP levels were increased in patients exposed to high cumulative anthracycline doses even in the absence of clinical signs of heart failure or decreased ejection fraction on echocardiography. NTproBNP is elevated in response to ventricular wall stress (70) and increased levels have been shown as marker for diastolic dysfunction or myocardial stress (71, 72). The results of the current study together with other data (68) support the role of circulating serum NTproBNP levels as biomarkers for subclinical cardiovascular impairment in asymptomatic childhood cancer survivors.
On transthoracic echocardiography, there were no abnormalities in morphologic parameters, such as myocardial wall thickness, left ventricular enddiastolic dimensions or left atrial volumes in the patients studied, supporting the evidence of subclinical rather than clinical cardiovascular impairment. However, patients after treatment for sarcoma and most exposure to anthracyclines had lower enddiastolic left ventricular dimension and thinner posterior walls compared to the other groups. This was observed by others (73, 74) and supports the theory of myocardial wall thinning secondary to progressive cardiomyocyte loss after cardiotoxic cancer treatment (75, 76). The observations of the current study are supported by the comparison with a cohort of childhood hypertrophic cardiomyopathy patients which shows fundamental differences between the two types of cardiomyopathy.
Because myocardial and interstitial fibrosis occurs in childhood hypertrophic cardiomyopathy patients (77) and has been described in histopathological specimens obtained at autopsy of childhood cancer survivors (78) and in adult patients with anthracycline-induced decreased left ventricular function poor LV function (79, 80), CMR was performed in a subset of the studied cohort. There was no evidence of myocardial fibrosis in the studied cohort, suggesting that myocardial fibrosis is rather a sign of late disease and not evident in subclinical disease status. However, the prevalence of myocardial fibrosis and its impact on rhythm disorders or ventricular dysfunction in adult childhood cancer survivors >30 years of age cannot be assessed based on the present data because of the follow-up time reaching to young adulthood only. Based on those findings it can be concluded that CMR studies can be preserved to those patients with overt cardiac dysfunction on echocardiography or to specific questions.
As shown in other studies (81), global longitudinal strain on echocardiographic speckle tracking was impaired in about a third of childhood cancer survivors in the current study in the absence of a decreased ejection fraction, supporting the use of speckle-tracking echocardiography to detect subtle changes in cardiac dysfunction. However, speckle tracking echocardiography needs to be performed offline and is time-consuming, thus elevating costs during regular patient follow-up.
In the absence of enlarged left atria, the ratio of mitral valve septal annular E to the early velocity as measured by tissue Doppler imaging (E') (E/E') was increased in patients with subclinical cardiovascular impairment in the current study, correlating with the amount of cumulative anthracycline exposure as a sign of subclinical diastolic dysfunction. This was also shown by others (67, 82–84) and supports the hypothesis of a progressive, restrictive-like cardiomyopathy pattern previously described in serial echocardiographic studies of long-term childhood cancer survivors treated with anthracyclines (59, 74, 85–88).
In summary the presented data provide evidence of subclinical cardiovascular disease in childhood cancer survivors exposed to high doses of anthracyclines. Subtle changes may precede clinical manifestation and can be evaluated by laboratory testing (biomarkers) and focused echocardiographic imaging techniques including speckle tracking and tissue Doppler.
Of course, focus should always be on the primary prevention of cardiotoxicity (89) by limiting cumulative dosage, using liposomal anthracyclines (31–33), and providing adjunctive cardioprotective therapies (34–37) [specifically the iron-chelating agent dexrazoxane (90–92)]. On the other hand, patients at risk may benefit from early detection of subclinical heart failure in order to start preventative cardiovascular measures in time (74, 93–96).
Conclusion
Long-term childhood cancer survivors exposed to cardiotoxic therapy show subtle impairment of cardiovascular function best detected by decreased exercise capacity, elevated NTproBNP levels, increased global longitudinal strain on speckle tracking, and abnormal mitral valve annular septal tissue doppler velocities in the absence of gross morphological changes, such as myocardial fibrosis on CMR, or echocardiographic decreased ejection fraction, enlarged left ventricular chamber sizes or left atria. Increased cumulative anthracycline dose for cancer treatment is the only independent predictor for the presence of those abnormal cardiovascular findings. Regular application of blood biomarkers and new imaging technologies can optimize risk stratification of childhood cancer survivors and can facilitate identification of subclinical cardiovascular disease and enable preventative treatment measures in time before overt clinical disease in high risk childhood cancer patients.
Data Availability Statement
The raw data supporting the conclusions of this article will be made available by the authors, without undue reservation, to any qualified researcher.
Ethics Statement
The studies involving human participants were reviewed and approved by the Technical University of Munich Institutional Review Board (ethical approval number 243/17S, 10/16/2017). Written informed consent from the participants' legal guardian/next of kin was not required to participate in this study in accordance with the national legislation and the institutional requirements.
Author's Note
Data were in part presented at the Annual Meeting of the Association for European Pediatric and Congenital Cardiology in Athens, 9 May – 12 May 2018.
Author Contributions
CW, RO, PE, IS, and JW designed the study and data collection instruments, coordinated and supervised data collection, statistical analysis and interpretation of data, drafted, reviewed and critically revised the manuscript. BR, AK, AH, JM, and CM collected cardiovascular data. BR and AH analyzed cardiopulmonary exercise testing data. AK and AH performed measurements on transthoracic echocardiography, specifically of diastolic dysfunction on tissue Doppler and speckle tracking for global longitudinal strain analysis. CM performed and analyzed cardiovascular magnetic resonance imaging and contributed to interstitial fibrosis quantification by determination of extracellular volume fraction on T1 map as well as quantification of late gadolinium enhancement and critically revised the article for important intellectual content regarding cardiovascular magnetic resonance imaging. IS collected oncologic data. CW carried out statistical analyses and drafted the manuscript. All authors listed have made substantive intellectual contributions to this study. Each author has provided final approval of the version to be published and has given agreement to be accountable for all aspects of the work in ensuring that questions related to the accuracy or integrity of any part of the work are appropriately investigated and resolved.
Conflict of Interest
The authors declare that the research was conducted in the absence of any commercial or financial relationships that could be construed as a potential conflict of interest.
Acknowledgments
We thank the German Heart Center Munich and the Institute of Preventive Pediatrics, Faculty of Sport and Health Sciences, Technical University Munich, Germany, for supporting this study.
Abbreviations
HCM, Hypertrophic cardiomyopathy; TTE, Transthoracic echocardiography; ECG, Electrocardiogram; CPET, Cardiopulmonary exercise testing; CMR, Cardiovascular magnetic resonance; LVPWd, Enddiastolic left ventricular posterior wall dimension; LVEDd, Left ventricular enddiastolic diameter; EF, Ejection Fraction; GLS, Global longitudinal strain; LA, Left atrium; MV, Mitral valve; LGE, Late gadolinium enhancement; MOLLI, Modified look-locker inversion recovery sequence; ECV, Extracellular volume fraction; NTproBNP, N-terminal pro-brain natriuretic peptide.
References
1. Siegel RL, Miller KD, Jemal A. Cancer statistics, 2015. CA Cancer J Clin. (2015) 65:5–29. doi: 10.3322/caac.21254
2. Gatta G, Capocaccia R, Coleman MP, Ries LA, Berrino F. Childhood cancer survival in Europe and the United States. Cancer. (2002) 95:1767–72. doi: 10.1002/cncr.10833
3. Jemal A, Siegel R, Ward E, Murray T, Xu J, Smigal C, et al. Cancer statistics, 2006. CA Cancer J Clin. (2006) 56:106–30. doi: 10.3322/canjclin.56.2.106
4. Krischer JP, Epstein S, Cuthbertson DD, Goorin AM, Epstein ML, Lipshultz SE. Clinical cardiotoxicity following anthracycline treatment for childhood cancer: the pediatric oncology group experience. J Clin Oncol. (1997) 15:1544–52. doi: 10.1200/JCO.1997.15.4.1544
5. Franco VI, Henkel JM, Miller TL, Lipshultz SE. Cardiovascular effects in childhood cancer survivors treated with anthracyclines. Cardiol Res Pract. (2011) 2011:134679. doi: 10.4061/2011/134679
6. Raj S, Franco VI, Lipshultz SE. Anthracycline-induced cardiotoxicity: a review of pathophysiology, diagnosis, and treatment. Curr Treat Options Cardiovasc Med. (2014) 16:315. doi: 10.1007/s11936-014-0315-4
7. Todaro MC, Oreto L, Qamar R, Paterick TE, Carerj S, Khandheria BK. Cardioncology: state of the heart. Int J Cardiol. (2013) 168:680–7. doi: 10.1016/j.ijcard.2013.03.133
8. Van Der Pal HJ, Van Dalen EC, Van Delden E, Van Dijk IW, Kok WE, Geskus RB, et al. High risk of symptomatic cardiac events in childhood cancer survivors. J Clin Oncol. (2012) 30:1429–37. doi: 10.1200/JCO.2010.33.4730
9. Van Der Pal HJ, Van Dalen EC, Hauptmann M, Kok WE, Caron HN, Van Den Bos C, et al. Cardiac function in 5-year survivors of childhood cancer: a long-term follow-up study. Arch Intern Med. (2010) 170:1247–55. doi: 10.1001/archinternmed.2010.233
10. Ewer MS, Ewer SM. Cardiotoxicity of anticancer treatments. Nat Rev Cardiol. (2015) 12:620. doi: 10.1038/nrcardio.2015.65
11. Lebrecht D, Setzer B, Ketelsen UP, Haberstroh J, Walker UA. Time-dependent and tissue-specific accumulation of mtDNA and respiratory chain defects in chronic doxorubicin cardiomyopathy. Circulation. (2003) 108:2423–9. doi: 10.1161/01.CIR.0000093196.59829.DF
12. Minotti G, Menna P, Salvatorelli E, Cairo G, Gianni L. Anthracyclines: molecular advances and pharmacologic developments in antitumor activity and cardiotoxicity. Pharmacol Rev. (2004) 56:185–229. doi: 10.1124/pr.56.2.6
13. Davies KJ, Doroshow JH. Redox cycling of anthracyclines by cardiac mitochondria. I Anthracycline radical formation by NADH dehydrogenase. J Biol Chem. (1986) 261:3060–7.
14. Doroshow JH, Davies KJ. Redox cycling of anthracyclines by cardiac mitochondria. II formation of superoxide anion, hydrogen peroxide, and hydroxyl radical. J Biol Chem. (1986) 261:3068–74.
15. Chen B, Peng X, Pentassuglia L, Lim CC, Sawyer DB. Molecular and cellular mechanisms of anthracycline cardiotoxicity. Cardiovasc Toxicol. (2007) 7:114–21. doi: 10.1007/s12012-007-0005-5
16. Ito H, Miller SC, Billingham ME, Akimoto H, Torti SV, Wade R, et al. Doxorubicin selectively inhibits muscle gene expression in cardiac muscle cells in vivo and in vitro. Proc Natl Acad Sci USA. (1990) 87:4275–9. doi: 10.1073/pnas.87.11.4275
17. Zhang S, Liu X, Bawa-Khalfe T, Lu LS, Lyu YL, Liu LF, et al. Identification of the molecular basis of doxorubicin-induced cardiotoxicity. Nat Med. (2012) 18:1639–42. doi: 10.1038/nm.2919
18. Thompson KL, Rosenzweig BA, Zhang J, Knapton AD, Honchel R, Lipshultz SE, et al. Early alterations in heart gene expression profiles associated with doxorubicin cardiotoxicity in rats. Cancer Chemother Pharmacol. (2010) 66:303–14. doi: 10.1007/s00280-009-1164-9
19. Gianni L, Herman EH, Lipshultz SE, Minotti G, Sarvazyan N, Sawyer DB. Anthracycline cardiotoxicity: from bench to bedside. J Clin Oncol. (2008) 26:3777–84. doi: 10.1200/JCO.2007.14.9401
20. Armenian SH, Armstrong GT, Aune G, Chow EJ, Ehrhardt MJ, Ky B, et al. Cardiovascular disease in survivors of childhood cancer: insights into epidemiology, pathophysiology, and prevention. J Clin Oncol. (2018) 36:2135–44. doi: 10.1200/JCO.2017.76.3920
21. Bansal N, Amdani SM, Hutchins KK, Lipshultz SE. Cardiovascular disease in survivors of childhood cancer. Curr Opin Pediatr. (2018) 30:628–38. doi: 10.1097/MOP.0000000000000675
22. Franco VI, Lipshultz SE. Cardiac complications in childhood cancer survivors treated with anthracyclines. Cardiol Young. (2015) 25 (Suppl. 2):107–16. doi: 10.1017/S1047951115000906
23. Trachtenberg BH, Landy DC, Franco VI, Henkel JM, Pearson EJ, Miller TL, et al. Anthracycline-associated cardiotoxicity in survivors of childhood cancer. Pediatr Cardiol. (2011) 32:342–53. doi: 10.1007/s00246-010-9878-3
24. Scully RE, Lipshultz SE. Anthracycline cardiotoxicity in long-term survivors of childhood cancer. Cardiovasc Toxicol. (2007) 7:122–8. doi: 10.1007/s12012-007-0006-4
25. Lipshultz SE, Alvarez JA, Scully RE. Anthracycline associated cardiotoxicity in survivors of childhood cancer. Heart. (2008) 94:525–33. doi: 10.1136/hrt.2007.136093
26. Elbl L, Hrstkova H, Chaloupka V. The late consequences of anthracycline treatment on left ventricular function after treatment for childhood cancer. Eur J Pediatr. (2003) 162:690–6. doi: 10.1007/s00431-003-1275-y
27. Krajinovic M, Elbared J, Drouin S, Bertout L, Rezgui A, Ansari M, et al. Polymorphisms of ABCC5 and NOS3 genes influence doxorubicin cardiotoxicity in survivors of childhood acute lymphoblastic leukemia. Pharmacogenomics J. (2016) 16:530–5. doi: 10.1038/tpj.2015.63
28. Visscher H, Ross CJ, Rassekh SR, Barhdadi A, Dube MP, Al-Saloos H, et al. Pharmacogenomic prediction of anthracycline-induced cardiotoxicity in children. J Clin Oncol. (2012) 30:1422–8. doi: 10.1200/JCO.2010.34.3467
29. Aminkeng F, Ross CJ, Rassekh SR, Hwang S, Rieder MJ, Bhavsar AP, et al. Recommendations for genetic testing to reduce the incidence of anthracycline-induced cardiotoxicity. Br J Clin Pharmacol. (2016) 82:683–95. doi: 10.1111/bcp.13008
30. Lee JW, Aminkeng F, Bhavsar AP, Shaw K, Carleton BC, Hayden MR, et al. The emerging era of pharmacogenomics: current successes, future potential, and challenges. Clin Genet. (2014) 86:21–8. doi: 10.1111/cge.12392
31. Smith LA, Cornelius VR, Plummer CJ, Levitt G, Verrill M, Canney P, et al. Cardiotoxicity of anthracycline agents for the treatment of cancer: systematic review and meta-analysis of randomised controlled trials. BMC Cancer. (2010) 10:337. doi: 10.1186/1471-2407-10-337
32. Creutzig U, Ritter J, Zimmermann M, Hermann J, Gadner H, Sawatzki DB, et al. Idarubicin improves blast cell clearance during induction therapy in children with AML: results of study AML-BFM 93. AML-BFM Study Group. Leukemia. (2001) 15:348–54. doi: 10.1038/sj.leu.2402046
33. Feijen EA, Leisenring WM, Stratton KL, Ness KK, Van Der Pal HJ, Caron HN, et al. Equivalence ratio for daunorubicin to doxorubicin in relation to late heart failure in survivors of childhood cancer. J Clin Oncol. (2015) 33:3774–80. doi: 10.1200/JCO.2015.61.5187
34. Lipshultz SE, Cochran TR, Franco VI, Miller TL. Treatment-related cardiotoxicity in survivors of childhood cancer. Nat Rev Clin Oncol. (2013) 10:697–710. doi: 10.1038/nrclinonc.2013.195
35. Lipshultz SE, Sambatakos P, Maguire M, Karnik R, Ross SW, Franco VI, et al. Cardiotoxicity and cardioprotection in childhood cancer. Acta Haematol. (2014) 132:391–9. doi: 10.1159/000360238
36. Lipshultz SE, Diamond MB, Franco VI, Aggarwal S, Leger K, Santos MV, et al. Managing chemotherapy-related cardiotoxicity in survivors of childhood cancers. Paediatr Drugs. (2014) 16:373–89. doi: 10.1007/s40272-014-0085-1
37. Harake D, Franco VI, Henkel JM, Miller TL, Lipshultz SE. Cardiotoxicity in childhood cancer survivors: strategies for prevention and management. Future Cardiol. (2012) 8:647–70. doi: 10.2217/fca.12.44
38. Shankar SM, Marina N, Hudson MM, Hodgson DC, Adams MJ, Landier W, et al. Monitoring for cardiovascular disease in survivors of childhood cancer: report from the cardiovascular disease task force of the children's oncology group. Pediatrics. (2008) 121:e387–96. doi: 10.1542/peds.2007-0575
39. Landier W, Skinner R, Wallace WH, Hjorth L, Mulder RL, Wong FL, et al. Surveillance for late effects in childhood cancer survivors. J Clin Oncol. (2018) 36:2216–22. doi: 10.1200/JCO.2017.77.0180
40. Wong FL, Bhatia S, Landier W, Francisco L, Leisenring W, Hudson MM, et al. Cost-effectiveness of the children's oncology group long-term follow-up screening guidelines for childhood cancer survivors at risk for treatment-related heart failure. Ann Intern Med. (2014) 160:672–83. doi: 10.7326/M13-2498
41. Kremer LC, Van Der Pal HJ, Offringa M, Van Dalen EC, Voute PA. Frequency and risk factors of subclinical cardiotoxicity after anthracycline therapy in children: a systematic review. Ann Oncol. (2002) 13:819–29. doi: 10.1093/annonc/mdf167
42. Rakowski H, Sasson Z, Wigle ED. Echocardiographic and doppler assessment of hypertrophic cardiomyopathy. J Am Soc Echocardiogr. (1988) 1:31–47. doi: 10.1016/S0894-7317(88)80062-2
43. Elliott PM, Anastasakis A, Borger MA, Borggrefe M, Cecchi F, Charron P, et al. 2014 ESC guidelines on diagnosis and management of hypertrophic cardiomyopathy: the task force for the diagnosis and management of hypertrophic cardiomyopathy of the european society of cardiology (ESC). Eur Heart J. (2014) 35:2733–79. doi: 10.1093/eurheartj/ehu284
44. Schleihauf J, Cleuziou J, Pabst Von Ohain J, Meierhofer C, Stern H, Shehu N, et al. Clinical long-term outcome of septal myectomy for obstructive hypertrophic cardiomyopathy in infants. Eur J Cardiothorac Surg. (2018) 53:538–44. doi: 10.1093/ejcts/ezx369
45. American Thoracic S, American College of Chest P. ATS/ACCP statement on cardiopulmonary exercise testing. Am J Respir Crit Care Med. (2003) 167:211–77. doi: 10.1164/rccm.167.2.211
46. Zoghbi WA, Enriquez-Sarano M, Foster E, Grayburn PA, Kraft CD, Levine RA, et al. Recommendations for evaluation of the severity of native valvular regurgitation with two-dimensional and Doppler echocardiography. J Am Soc Echocardiogr. (2003) 16:777–802. doi: 10.1016/S0894-7317(03)00335-3
47. Weidemann F, Eyskens B, Jamal F, Mertens L, Kowalski M, D'hooge J, et al. Quantification of regional left and right ventricular radial and longitudinal function in healthy children using ultrasound-based strain rate and strain imaging. J Am Soc Echocardiogr. (2002) 15:20–8. doi: 10.1067/mje.2002.116532
48. Kuhn A, De Pasquale Meyer G, Muller J, Petzuch K, Fratz S, Rohlig C, et al. Tricuspid valve surgery improves cardiac output and exercise performance in patients with Ebstein's anomaly. Int J Cardiol. (2013) 166:494–8. doi: 10.1016/j.ijcard.2011.11.033
49. Pettersen MD, Du W, Skeens ME, Humes RA. Regression equations for calculation of z scores of cardiac structures in a large cohort of healthy infants, children, and adolescents: an echocardiographic study. J Am Soc Echocardiogr. (2008) 21:922–34. doi: 10.1016/j.echo.2008.02.006
50. Klues HG, Schiffers A, Maron BJ. Phenotypic spectrum and patterns of left ventricular hypertrophy in hypertrophic cardiomyopathy: morphologic observations and significance as assessed by two-dimensional echocardiography in 600 patients. J Am Coll Cardiol. (1995) 26:1699–708. doi: 10.1016/0735-1097(95)00390-8
51. Echocardiographic Normal Ranges Meta-Analysis of the Left Heart C. Ethnic-specific normative reference values for echocardiographic LA and LV Size, LV mass, and systolic function: the EchoNoRMAL study. JACC Cardiovasc Imaging. (2015) 8:656–65. doi: 10.1016/j.jcmg.2015.02.014
52. Dalen H, Thorstensen A, Vatten LJ, Aase SA, Stoylen A. Reference values and distribution of conventional echocardiographic Doppler measures and longitudinal tissue Doppler velocities in a population free from cardiovascular disease. Circ Cardiovasc Imaging. (2010) 3:614–22. doi: 10.1161/CIRCIMAGING.109.926022
53. Yingchoncharoen T, Agarwal S, Popovic ZB, Marwick TH. Normal ranges of left ventricular strain: a meta-analysis. J Am Soc Echocardiogr. (2013) 26:185–91. doi: 10.1016/j.echo.2012.10.008
54. Negishi K, Negishi T, Kurosawa K, Hristova K, Popescu BA, Vinereanu D, et al. Practical guidance in echocardiographic assessment of global longitudinal strain. JACC Cardiovasc Imaging. (2015) 8:489–92. doi: 10.1016/j.jcmg.2014.06.013
55. Kadappu KK, Thomas L. Tissue Doppler imaging in echocardiography: value and limitations. Heart Lung Circ. (2015) 24:224–33. doi: 10.1016/j.hlc.2014.10.003
56. Kellman P, Arai AE, Mcveigh ER, Aletras AH. Phase-sensitive inversion recovery for detecting myocardial infarction using gadolinium-delayed hyperenhancement. Magn Reson Med. (2002) 47:372–83. doi: 10.1002/mrm.10051
57. Messroghli DR, Radjenovic A, Kozerke S, Higgins DM, Sivananthan MU, Ridgway JP. Modified look-locker inversion recovery (MOLLI) for high-resolution T1 mapping of the heart. Magn Reson Med. (2004) 52:141–6. doi: 10.1002/mrm.20110
58. Kellman P, Wilson JR, Xue H, Ugander M, Arai AE. Extracellular volume fraction mapping in the myocardium, part 1: evaluation of an automated method. J Cardiovasc Magn Reson. (2012) 14:63. doi: 10.1186/1532-429X-14-63
59. Sorensen K, Levitt GA, Bull C, Dorup I, Sullivan ID. Late anthracycline cardiotoxicity after childhood cancer: a prospective longitudinal study. Cancer. (2003) 97:1991–8. doi: 10.1002/cncr.11274
60. Kremer LC, Van Dalen EC, Offringa M, Ottenkamp J, Voute PA. Anthracycline-induced clinical heart failure in a cohort of 607 children: long-term follow-up study. J Clin Oncol. (2001) 19:191–6. doi: 10.1200/JCO.2001.19.1.191
61. Nysom K, Holm K, Lipsitz SR, Mone SM, Colan SD, Orav EJ, et al. Relationship between cumulative anthracycline dose and late cardiotoxicity in childhood acute lymphoblastic leukemia. J Clin Oncol. (1998) 16:545–50. doi: 10.1200/JCO.1998.16.2.545
62. Yeh ET, Bickford CL. Cardiovascular complications of cancer therapy: incidence, pathogenesis, diagnosis, and management. J Am Coll Cardiol. (2009) 53:2231–47. doi: 10.1016/j.jacc.2009.02.050
63. Armenian SH, Hudson MM, Mulder RL, Chen MH, Constine LS, Dwyer M, et al. Recommendations for cardiomyopathy surveillance for survivors of childhood cancer: a report from the international late effects of childhood cancer guideline harmonization group. Lancet Oncol. (2015) 16:e123–136. doi: 10.1016/S1470-2045(14)70409-7
64. Miller AM, Lopez-Mitnik G, Somarriba G, Lipsitz SR, Hinkle AS, Constine LS, et al. Exercise capacity in long-term survivors of pediatric cancer: an analysis from the cardiac risk factors in childhood cancer survivors study. Pediatr Blood Cancer. (2013) 60:663–8. doi: 10.1002/pbc.24410
65. De Caro E, Smeraldi A, Trocchio G, Calevo M, Hanau G, Pongiglione G. Subclinical cardiac dysfunction and exercise performance in childhood cancer survivors. Pediatr Blood Cancer. (2011) 56:122–6. doi: 10.1002/pbc.22606
66. Jarfelt M, Kujacic V, Holmgren D, Bjarnason R, Lannering B. Exercise echocardiography reveals subclinical cardiac dysfunction in young adult survivors of childhood acute lymphoblastic leukemia. Pediatr Blood Cancer. (2007) 49:835–40. doi: 10.1002/pbc.21289
67. Christiansen JR, Kanellopoulos A, Lund MB, Massey R, Dalen H, Kiserud CE, et al. Impaired exercise capacity and left ventricular function in long-term adult survivors of childhood acute lymphoblastic leukemia. Pediatr Blood Cancer. (2015) 62:1437–43. doi: 10.1002/pbc.25492
68. Lipshultz SE, Miller TL, Scully RE, Lipsitz SR, Rifai N, Silverman LB, et al. Changes in cardiac biomarkers during doxorubicin treatment of pediatric patients with high-risk acute lymphoblastic leukemia: associations with long-term echocardiographic outcomes. J Clin Oncol. (2012) 30:1042–9. doi: 10.1200/JCO.2010.30.3404
69. Jaffe AS, Ravkilde J, Roberts R, Naslund U, Apple FS, Galvani M, et al. It's time for a change to a troponin standard. Circulation. (2000) 102:1216–20. doi: 10.1161/01.CIR.102.11.1216
70. Martinez-Rumayor A, Richards AM, Burnett JC, Januzzi JL Jr. Biology of the natriuretic peptides. Am J Cardiol. (2008) 101:3–8. doi: 10.1016/j.amjcard.2007.11.012
71. Wang TJ, Larson MG, Levy D, Benjamin EJ, Leip EP, Omland T, et al. Plasma natriuretic peptide levels and the risk of cardiovascular events and death. N Engl J Med. (2004) 350:655–63. doi: 10.1056/NEJMoa031994
72. Grewal J, Mckelvie RS, Persson H, Tait P, Carlsson J, Swedberg K, et al. Usefulness of N-terminal pro-brain natriuretic Peptide and brain natriuretic peptide to predict cardiovascular outcomes in patients with heart failure and preserved left ventricular ejection fraction. Am J Cardiol. (2008) 102:733–7. doi: 10.1016/j.amjcard.2008.04.048
73. Lipshultz SE, Scully RE, Lipsitz SR, Sallan SE, Silverman LB, Miller TL, et al. Assessment of dexrazoxane as a cardioprotectant in doxorubicin-treated children with high-risk acute lymphoblastic leukaemia: long-term follow-up of a prospective, randomised, multicentre trial. Lancet Oncol. (2010) 11:950–61. doi: 10.1016/S1470-2045(10)70204-7
74. Lipshultz SE, Lipsitz SR, Sallan SE, Dalton VM, Mone SM, Gelber RD, et al. Chronic progressive cardiac dysfunction years after doxorubicin therapy for childhood acute lymphoblastic leukemia. J Clin Oncol. (2005) 23:2629–36. doi: 10.1200/JCO.2005.12.121
75. Arola OJ, Saraste A, Pulkki K, Kallajoki M, Parvinen M, Voipio-Pulkki LM. Acute doxorubicin cardiotoxicity involves cardiomyocyte apoptosis. Cancer Res. (2000) 60:1789–92.
76. Bernuzzi F, Recalcati S, Alberghini A, Cairo G. Reactive oxygen species-independent apoptosis in doxorubicin-treated H9c2 cardiomyocytes: role for heme oxygenase-1 down-modulation. Chem Biol Interact. (2009) 177:12–20. doi: 10.1016/j.cbi.2008.09.012
77. Maron BJ, Towbin JA, Thiene G, Antzelevitch C, Corrado D, Arnett D, et al. Contemporary definitions and classification of the cardiomyopathies: an American Heart Association Scientific Statement from the Council on Clinical Cardiology, Heart Failure and Transplantation Committee; Quality of Care and Outcomes Research and Functional Genomics and Translational Biology Interdisciplinary Working Groups; and Council on Epidemiology and Prevention. Circulation. (2006) 113:1807–16. doi: 10.1161/CIRCULATIONAHA.106.174287
78. Bernaba BN, Chan JB, Lai CK, Fishbein MC. Pathology of late-onset anthracycline cardiomyopathy. Cardiovasc Pathol. (2010) 19:308–11. doi: 10.1016/j.carpath.2009.07.004
79. Fallah-Rad N, Lytwyn M, Fang T, Kirkpatrick I, Jassal DS. Delayed contrast enhancement cardiac magnetic resonance imaging in trastuzumab induced cardiomyopathy. J Cardiovasc Magn Reson. (2008) 10:5. doi: 10.1186/1532-429X-10-5
80. Fallah-Rad N, Walker JR, Wassef A, Lytwyn M, Bohonis S, Fang T, et al. The utility of cardiac biomarkers, tissue velocity and strain imaging, and cardiac magnetic resonance imaging in predicting early left ventricular dysfunction in patients with human epidermal growth factor receptor II-positive breast cancer treated with adjuvant trastuzumab therapy. J Am Coll Cardiol. (2011) 57:2263–70. doi: 10.1016/j.jacc.2010.11.063
81. Tsai HR, Gjesdal O, Wethal T, Haugaa KH, Fossa A, Fossa SD, et al. Left ventricular function assessed by two-dimensional speckle tracking echocardiography in long-term survivors of Hodgkin's lymphoma treated by mediastinal radiotherapy with or without anthracycline therapy. Am J Cardiol. (2011) 107:472–7. doi: 10.1016/j.amjcard.2010.09.048
82. Karakurt C, Kocak G, Ozgen U. Evaluation of the left ventricular function with tissue tracking and tissue Doppler echocardiography in pediatric malignancy survivors after anthracycline therapy. Echocardiography. (2008) 25:880–7. doi: 10.1111/j.1540-8175.2008.00695.x
83. Rajapreyar P, Lorenzana A, Prabhu A, Szpunar S, Anne P. Tissue Doppler imaging and focal, late-onset anthracycline-induced cardiovascular disease in long term survivors of childhood cancer: a research article. J Clin Diagn Res. (2016) 10:SC01–4. doi: 10.7860/JCDR/2016/19652.8249
84. Hudson MM, Rai SN, Nunez C, Merchant TE, Marina NM, Zalamea N, et al. Noninvasive evaluation of late anthracycline cardiac toxicity in childhood cancer survivors. J Clin Oncol. (2007) 25:3635–43. doi: 10.1200/JCO.2006.09.7451
85. Ganame J, Claus P, Uyttebroeck A, Renard M, D'hooge J, Bijnens B, et al. Myocardial dysfunction late after low-dose anthracycline treatment in asymptomatic pediatric patients. J Am Soc Echocardiogr. (2007) 20:1351–8. doi: 10.1016/j.echo.2007.04.007
86. Iarussi D, Indolfi P, Casale F, Martino V, Di Tullio MT, Calabro R. Anthracycline-induced cardiotoxicity in children with cancer: strategies for prevention and management. Paediatr Drugs. (2005) 7:67–76. doi: 10.2165/00148581-200507020-00001
87. Kapusta L, Thijssen JM, Groot-Loonen J, Antonius T, Mulder J, Daniels O. Tissue Doppler imaging in detection of myocardial dysfunction in survivors of childhood cancer treated with anthracyclines. Ultrasound Med Biol. (2000) 26:1099–108. doi: 10.1016/S0301-5629(00)00252-0
88. Lipshultz SE, Colan SD, Gelber RD, Perez-Atayde AR, Sallan SE, Sanders SP. Late cardiac effects of doxorubicin therapy for acute lymphoblastic leukemia in childhood. N Engl J Med. (1991) 324:808–15. doi: 10.1056/NEJM199103213241205
89. Hutchins KK, Siddeek H, Franco VI, Lipshultz SE. Prevention of cardiotoxicity among survivors of childhood cancer. Br J Clin Pharmacol. (2017) 83:455–65. doi: 10.1111/bcp.13120
90. Anderson B. Dexrazoxane for the prevention of cardiomyopathy in anthracycline treated pediatric cancer patients. Pediatr Blood Cancer. (2005) 44:584–8. doi: 10.1002/pbc.20358
91. Rao VA. Iron chelators with topoisomerase-inhibitory activity and their anticancer applications. Antioxid Redox Signal. (2013) 18:930–55. doi: 10.1089/ars.2012.4877
92. Sterba M, Popelova O, Vavrova A, Jirkovsky E, Kovarikova P, Gersl V, et al. Oxidative stress, redox signaling, and metal chelation in anthracycline cardiotoxicity and pharmacological cardioprotection. Antioxid Redox Signal. (2013) 18:899–929. doi: 10.1089/ars.2012.4795
93. Kalay N, Basar E, Ozdogru I, Er O, Cetinkaya Y, Dogan A, et al. Protective effects of carvedilol against anthracycline-induced cardiomyopathy. J Am Coll Cardiol. (2006) 48:2258–62. doi: 10.1016/j.jacc.2006.07.052
94. Armenian SH, Hudson MM, Chen MH, Colan SD, Lindenfeld L, Mills G, et al. Rationale and design of the children's oncology group (COG) study ALTE1621: a randomized, placebo-controlled trial to determine if low-dose carvedilol can prevent anthracycline-related left ventricular remodeling in childhood cancer survivors at high risk for developing heart failure. BMC Cardiovasc Disord. (2016) 16:187. doi: 10.1186/s12872-016-0364-6
95. Lipshultz SE, Lipsitz, SR, Sallan SE, Simbre VC II, Shaikh SL, Mone SM, Gelber RD, et al. Long-term enalapril therapy for left ventricular dysfunction in doxorubicin-treated survivors of childhood cancer. J Clin Oncol. (2002) 20:4517–22. doi: 10.1200/JCO.2002.12.102
Keywords: cardiotoxicity, childhood cancer, biomarker, speckle tracking, tissue doppler, anthracycline, cardiovascular, subclinical
Citation: Wolf CM, Reiner B, Kühn A, Hager A, Müller J, Meierhofer C, Oberhoffer R, Ewert P, Schmid I and Weil J (2020) Subclinical Cardiac Dysfunction in Childhood Cancer Survivors on 10-Years Follow-Up Correlates With Cumulative Anthracycline Dose and Is Best Detected by Cardiopulmonary Exercise Testing, Circulating Serum Biomarker, Speckle Tracking Echocardiography, and Tissue Doppler Imaging. Front. Pediatr. 8:123. doi: 10.3389/fped.2020.00123
Received: 27 September 2019; Accepted: 06 March 2020;
Published: 31 March 2020.
Edited by:
Andrew Landstrom, Duke University School of Medicine, United StatesReviewed by:
Kai Hu, University Hospital Würzburg, GermanyFederico Quaini, University of Parma, Italy
Copyright © 2020 Wolf, Reiner, Kühn, Hager, Müller, Meierhofer, Oberhoffer, Ewert, Schmid and Weil. This is an open-access article distributed under the terms of the Creative Commons Attribution License (CC BY). The use, distribution or reproduction in other forums is permitted, provided the original author(s) and the copyright owner(s) are credited and that the original publication in this journal is cited, in accordance with accepted academic practice. No use, distribution or reproduction is permitted which does not comply with these terms.
*Correspondence: Jochen Weil, d2VpbCYjeDAwMDQwO2RobS5taG4uZGU=