- 1Neonatology, National Maternity Hospital, Dublin, Ireland
- 2National Children's Research Centre, Dublin, Ireland
- 3Paediatrics, Royal College of Surgeons in Ireland, Dublin, Ireland
- 4University College Dublin School of Medicine and Medical Sciences, Conway Institute of Biomolecular and Biomedical Science, University College Dublin, Dublin, Ireland
- 5Haematology, Our Lady's Children's Hospital, Dublin, Ireland
- 6Paediatrics, Trinity College, The University of Dublin, Dublin, Ireland
- 7Critical Care, Our Lady's Children's Hospital, Dublin, Ireland
- 8Neonatology, Our Lady's Children's Hospital, Dublin, Ireland
- 9Paediatrics, Coombe Women's and Infant's University Hospital, Dublin, Ireland
Objective: Sepsis is major cause of morbidity and mortality in the Pediatric Intensive Care Unit (PICU). PICU patients may develop transient immune deficiency during sepsis. Activated Protein C (APC) has significant anti-inflammatory and cytoprotective effects. Clinical trials of APC in adult sepsis initially showed improved outcome but recent trials showed no benefit in adults or children. We aimed to assess the effects of APC treatment on innate immune responses in children.
Design and Subjects: We compared neutrophil and monocyte responses to lipopolysaccharide (LPS) with and without APC treatment in PICU patients at the time of evaluation for sepsis compared with healthy adults and age-matched pediatric controls. We used flow cytometry to examine cell activation (CD11b expression), function [intracellular reactive oxygen intermediate (ROI) release] and LPS recognition [Toll like Receptor 4 (TLR4) expression].
Results: PICU patients had significantly decreased protein c levels and LPS responses compared with adult and pediatric controls for all parameters. APC reduced LPS-induced neutrophil PICU TLR4 and adult ROI (p < 0.05). PICU non-survivors had increased LPS induced neutrophil and monocyte ROI production vs. survivors which was significantly reduced by APC.
Conclusion: PICU patients demonstrate significantly reduced endotoxin reactivity which may predispose them to sepsis and alter effective antibacterial responses. APC reduces LPS-induced ROI production in adults and may have a role in treating severely compromised PICU patients especially given that newer APC forms are associated with decreased bleeding risk and enhanced anti-inflammatory effects.
Introduction
Sepsis is the 2nd leading cause of death in children and the 4th leading cause of death in infants. The incidence of sepsis in USA is 0.56/1,000 children with an overall mortality of 10.6% and an overall cost of almost 2 billion dollars per annum (1). Sepsis is characterized by a systemic inflammatory response to infection and may result in tissue damage, multi-organ dysfunction (MOD), and death. Persistent activation of leukocytes may contribute to this process while excessive leukocyte elimination may contribute to immunoparesis, bacterial overgrowth, and death. Establishing a balance between over-activation of leukocytes and excessive leukocyte elimination reduces tissue damage and mortality (2).
Septic adult patients often demonstrate defects in cell surface antigen expression and reactive oxygen intermediate (ROI) production, dysregulation of cytokine release, and enhanced apoptosis (3). We were interested in studying immune cell LPS recognition, activation and adhesion in Pediatric Intensive Care Unit (PICU) patients.
Toll-Like Receptors (TLRs) are a group of vital transmembrane receptors that initiate innate immune responses to an array of micro-organisms. TLR4 is the primary receptor that recognizes lipopolysaccharide (LPS) and mediates nuclear factor-kappa B (NF-kB) activation and proinflammatory cytokine synthesis (4). Baseline monocyte and neutrophil TLR4 expression is increased in adult sepsis (5).
CD11b is a subunit of the β2 integrin adhesion molecule and is expressed on myelocytes and more mature granulocytes (6). Upon binding bacterial LPS, neutrophil and monocyte expression of CD11b increases (7). Weirich et al. demonstrated increased neutrophil CD11b expression in infants with infection (6). Similarly, adults with sepsis and organ dysfunction show increased monocyte CD11b expression (8).
Reactive Oxygen Intermediate (ROI) production is essential for intracellular killing of invading microorganisms following phagocytosis by neutrophils. Intracellular ROI generation is upregulated both in neutrophils and monocytes in adults with sepsis (9). Neutrophil respiratory burst is increased in neonates showing signs of infection with Escherichia coli (10).
In the era of increasing antibiotic resistance, the development of adjunctive therapies for sepsis is crucial. Protein C is an endogenous vitamin K dependent glycoprotein that circulates in plasma as an inactive zymogen. Activated Protein C (APC) plays a role in coagulation and regulation of inflammation (11). In the PROWESS (Recombinant human Protein C World Evaluation in Severe Sepsis) study, the administration of APC reduced mortality in adult patients with severe sepsis and high risk of death (12). However, subsequent studies showed no benefit and APC (Xigris) was withdrawn from the market by Eli Lilly. A phase 1b open-label study found that the pharmacokinetics and pharmacodynamics of APC are similar in children and adults. However, the RESOLVE (Researching severe Sepsis and Organ dysfunction in children: a global perspective) study investigated the efficiency and safety of APC in children and concluded that it had no effect in children with sepsis (13). Yet recently modified versions of APC have been developed as immunomodulators in sepsis and have demonstrated less haemorrhagic potential and greater anti-inflammatory properties.
We hypothesized that APC diminishes neutrophil and monocyte activation and therefore reduces tissue damage in both adult and neonatal sepsis. In this study, we aimed to investigate neutrophil and monocyte CD11b/TLR4 expression and ROI production following LPS stimulation in pediatric sepsis patients ex vivo and to investigate the effect of APC treatment on these responses.
Materials and Methods
Reagents
Lipopolysaccharide (LPS) E. coli serotype 0111:B4, Phorbol 12-Myristate 13-Acetate (PMA), Dihydrorhodamine 123 (DHR), Fetal Bovine serum (heat inactivated), and phosphate buffered saline (PBS) were purchased from Sigma Aldrich Ltd. (www.sigmaaldrich.com). Dulbecco's modified Eagle's medium (DMEM) was purchased from Bioscience Ltd. (www.biosciences.ie). Penicillin streptomycin solution and L-glutamine serum (FCS) were purchased from GIBCO Life Technologies (www.invitrogen.com). Drotrecoginalfa (Xigris: Activated Protein C [APC]) was purchased from Eli Lilly (www.lilly.ie). CD11b was purchased from eBioscience (www.ebioscience.com). FACS lysing solution and Toll like Receptor (TLR4) were purchased from BD Bioscience (www.bdbiosciences.com) and all remaining chemicals were purchased from Sigma Aldrich company Ltd (Dorset UK) unless otherwise stated.
Patient Population
This study was approved by the Institutional Research Ethics Committee and fully informed written consent was obtained from all participants. The PICU in Our Lady's Children's Hospital Crumlin (OLCH) is the largest in Ireland and has 21 bedded Intensive Care/HDU. The adult control group consisted of healthy adult volunteers from the laboratory and hospital. Pediatric controls included children admitted to the surgical day ward for elective minor surgical procedures with no significant medical history. Samples were taken in theater during cannula insertion by the anesthetic team.
The PICU group included children admitted to the PICU for different medical and surgical conditions who underwent a septic workup for clinical suspicion of sepsis including fever, clinical deterioration, or increased inflammatory markers. Patients with neutropenic sepsis, malignancy, and cardiac bypass surgery in the preceding week, primary immune deficiency or who were older than 12 years were excluded from this study. The septic workup included a full blood count (FBC), C-reactive protein (CRP), Blood, sputum, and urine cultures as well as a coagulation screen (PT, APTT, Fibrinogen), Protein C levels and lumbar puncture if clinically indicated. Detailed information was taken from patient records including multiple organ outcome data to allow scoring of the degree of organ dysfunction (14).
Sample Processing
Whole blood samples were kept in ice and processed within 90 min. Whole blood was incubated for 1 h at 37°C with stimulatory agent LPS 20 ng/mL to mimic an inflammatory response in vitro. In addition APC 200 ng/mL was added ±LPS following a dose response study (results not shown) and in accordance with the in vitro studies of Galley et al. (15).
Protein C Activity (Chromogenic)
The Protein C level in patient plasma is measured in two stages: Incubation of the plasma with Protein C Activator; and quantification of Activated Protein C with a synthetic chromogenic substrate. Paranitroaniline released is monitored kinetically at 405 nm and is directly proportional to the Protein C level in the test sample. The HemosIL Protein C Kit used contains lyophilized substrate, Protein C Activator, and a saline diluent. Blood was collected (vol. 9) in 0.109 M (i.e., 3.2%) trisodium citrate anticoagulant bottles (Vol. 1) and samples were centrifuged at 4,000 rpm (2,500 rcf/g) for 10 min. The testing was completed within 4 h of sample collection. The sample was inverted a number of times or vortexed to ensure adequate mixing. Mixing is critical before testing, as precipitation of certain proteins may occur with freezing. Samples should be tested immediately. Any samples that were clotted or appeared haemolyzed were rejected. To ensure internal quality control, two different controls (Normal Control and HemosIL Low Abnormal) were included at the start of each working day and subsequently every 4 h throughout the day.
Quantification of Cell Surface Antigen Expression
The expression of CD11b and TLR-4 antigens on the surface of neutrophils and monocytes was measured by flow cytometry. Whole blood (50 μL) was treated with 5 μL of PE-CD11b and 2.5 μL of anti-human TLR4 antibodies and was then left at 4°C for 20 min. FACS was added and incubated for 10 min at room temperature. The sample was centrifuged at 3,000 rpm for 5 min at 4°C. The pellet was resuspended twice with DMEM 500 μL and stored on ice before analysis by flow cytometry. The fluorescence intensity is denoted by mean channel fluorescence, which is the average intensity of fluorescence emitted by all cells chosen for measurement and is comparable to the relative number of receptors present on the surface of each cell. The flow cytometer used was a BD Accuri C6 and a minimum of 5,000 events were collected and analyzed with a CFlow Plus software. All measurements were performed under the same instrument settings (16–20). Scatter profiles we used to delineate leukocyte populations using forward scatter and side scatter. Whole blood was used and neutrophil and monocyte populations were gated and confirmed by cell sorting in Flow Cytometer gates. A consultant hematologist and histologist then examined the sorted cells which had been fixed on a slide, stained, and analyzed under a microscope (16).
Respiratory Burst Activity
Generation of ROI was evaluated by flow cytometry using the technique of Smith and Wiedemann (17). Whole blood (50 μL) was incubated with DHR 123 (50 μL) and PBS (450 μL) at 37°C for 10 min. Cells were stimulated with 1 μL (16 μM) phorbol 12-myrisate 13-acetate (PMA) for 20 min at 37°C. The reaction was then halted by placing samples on ice. Neutrophil and monocyte fluorescence intensity was assessed by flow cytometry and expressed as Ln mean channel fluorescence (LnMCF). DHR 123 has been shown to detect intracellular H2O2 and OH radical production (21).
Statistical Methods
PASW statistical package version 18 (IBM Corp, Chicago, IL, USA; www.ibm.com/SPSS Statistics) was used to statistically analyse experimental and clinical data. For comparing conditions (Control/LPS/LPS+APC) within each group (Adults/Pediatric/PICU) matched paired t-tests were used. For comparing across groups one -way ANOVA with Tukey post-hoc comparison method was used and a p < 0.05 was considered significant.
Results
Patient Demographics
There were 15 adult controls, 25 pediatric controls and 15 PICU patients enrolled in this study. The adult controls had a median age of 26 years (22–38 years) with nine males and six females. The pediatric controls had a median age 21 months (4 months−12 years) including 14 males and 11 females. The PICU group had a median age 6 months (1 week−11 years) and there were seven males and eight females. The most common diagnosis in the PICU group was congenital heart disease (Table 1). Five children died during their PICU stay and all children enrolled were on antibiotics at the time of sampling. Cultures from blood, sputum, central line, or urine were positive in 12 out of 14 patients (15 samples) and one blood culture was positive (Table 1). Therefore, only one child had culture-positive sepsis although nine children had abnormal CRP levels (range 11–320 mg/l) and were managed as culture-negative sepsis. The Multiple organ dysfunction scores (MODS) were as follows: 4 organs (n = 1); 3 organs (n = 6); 2 organs (n = 5); 1 organ (n = 2); 0 organs (n = 0) (14).
Protein C Levels
Adult controls had Protein C levels within or above the normal range with the median (range) 1.29 (0.94–2.29) mg/L. All pediatric controls had normal Protein C levels: 0.7 (0.47–1.04) mg/L. Protein C levels were available in seven PICU patients: 0.52 (<0.01–0.99) mg/L. Only one PICU patient had a low Protein C level (<0.01) and this patient died.
Reactive Oxygen Intermediates
A significantly increased ROI release was noted in adult control neutrophils in response to LPS. LPS also induced an increased ROI release from pediatric control PMNs although not to the same degree as adults. In contrast, PICU patients were LPS hyporesponsive. There was a significant decrease in LPS stimulated ROI release from adults following APC (p = 0.01). However, there were no changes in pediatric controls or PICU patients' ROI responses to APC (Figure 1A).
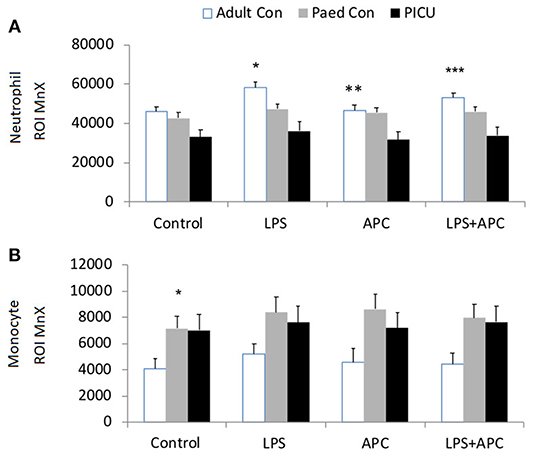
Figure 1. Neutrophil and monocyte LPS responses and APC modulation: Whole blood from healthy adult controls (n = 15), pediatric controls (p = 15), and PICU patients (n = 15) was incubated with LPS + APC for 1 h. Then stimulated with DHR and PMA. Results were expressed as the Ln mean channel fluorescence + SEM. (A) Neutrophil ROI: *p < 0.05 vs. pediatric controls and PICU patients; **p < 0.05 vs. adult controls; ***p < 0.05 vs. adult LPS response. (B) Monocyte ROI: *p < 0.05 vs. adults controls.
Monocytes produce ~80% less ROIs than neutrophils. Monocytes showed the same trends as seen in neutrophils with increased monocyte ROI production in adult and pediatric controls in response to LPS compared to PICU patients (p = 0.015 and p = 0.049, respectively; Figure 1B).
Neutrophil and Monocyte Cell Surface Receptor Expression
There was a significant increase in neutrophil CD11b in all groups in response to LPS. There was increased LPS responsiveness in adult and pediatric controls compared to PICU patients. APC had no effect in reducing LPS stimulated neutrophil CD11b in any group (Figure 2A). Similar CD11b responses were seen in monocytes and there was increased LPS stimulated monocyte CD11b in all groups. However, monocytes from PICU patients were significantly LPS hyporesponsive compared with adults and pediatric controls (p = 0.012 and p = 0.001, respectively; Figure 2B).
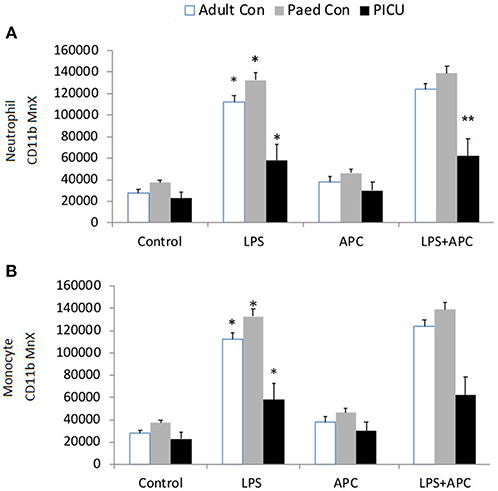
Figure 2. Neutrophil and monocyte CD11b expression: Whole blood from healthy adult controls (n = 15), pediatric controls (p = 15) and PICU patients (n = 15) was incubated with LPS + APC for 1 h. Neutrophils and monocytes were assessed for CD11b expression using a PE-labeled mAb and mean channel fluorescence analyzed using flow cytometry. The neutrophil and monocyte populations were selected based on their scatter profile: forward scatter and side scatter (A) Neutrophil CD11b expression: *p < 0.05 vs. respective controls; **p < 0.05 vs. PICU patients. (B) Monocyte CD11b expression: *p < 0.05 vs. respective controls.
Adults and pediatric controls did not show increased neutrophil TLR4 expression in response to LPS. Increased neutrophil expression of TLR4 was noted in response to LPS in PICU patients (p = 0.02). This was significantly reduced by APC (p = 0.005; Figure 3A). There was no increase in monocyte TLR4 expression in response to LPS in any group (Figure 3B).
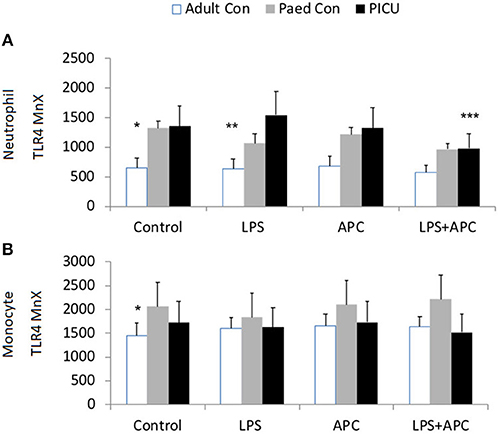
Figure 3. Neutrophil and monocyte TLR4 responses and APC modulation: Whole blood from healthy adult controls (n = 15), pediatric controls (p = 15), and PICU patients (n = 15) was incubated with LPS + APC for 1 h. Neutrophils and monocytes were then labeled with Alex Fluor 647 TLR4 mAb and mean channel fluorescence was analyzed using flow cytometry. The neutrophil and monocyte populations were selected via flow cytometry based on their scatter profile: forward scatter and side scatter. (A) Neutrophil TLR4 expression: *p < 0.05 vs. PICU control; **p < 0.05 vs. PICU LPS response; ***p < 0.05 vs. PICU LPS response. (B) Monocyte TLR4 expression: *p < 0.05 vs. PICU controls.
Comparison of PICU Survivors vs. Non-survivors
Baseline neutrophil and monocyte ROI expression was higher in PICU non-survivors compared to survivors. Non-survivors had significant increased neutrophil and monocyte ROI production in response to LPS in contrast to survivors (p = 0.001). Non-survivors LPS-induced ROI production was significantly reduced by APC (p = 0.034; Figures 4A,B). There was no significant difference in CD11b expression at baseline in survivors and non-survivors. Neutrophil and monocyte CD11b was significantly increased in both groups with LPS and was not altered by APC. There was no significant difference at baseline in neutrophil and monocyte TLR4 expression in non-survivors vs. survivors. Similarly, there was no significant difference between neutrophil and monocyte TLR4 expression in response to LPS in these groups (Figure 4C).
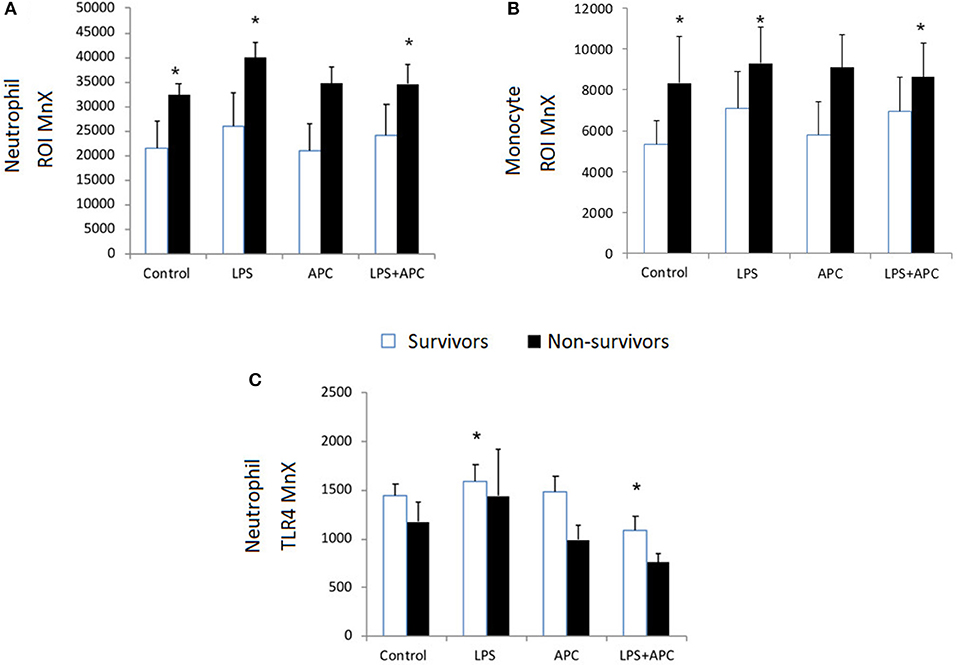
Figure 4. Neutrophil and Monocyte ROI and Neutrophil TLR4 expression in survivors and non-survivors in PICU: Whole blood was incubated with LPS ± APC for 1 h and then stimulated with DHR and PMA and evaluated by flow cytometry. Results were expressed as the Ln mean channel fluorescence + SEM. (A) Neutrophil ROI generation: *p < 0.05 vs. survivor controls. (B) Monocyte ROI generation: *p < 0.05 vs. survivor controls. (C) Neutrophil TLR4 expression: Whole blood was incubated with LPS ± APC for 1 h. Neutrophils were labeled with Alex Fluor 647 TLR4mAb and mean channel fluorescence (MCF) analyzed using flow cytometry. *p < 0.05 vs. non-survivor controls.
Discussion
PICU patients were hyporesponsive to LPS with decreased neutrophil and monocyte CD11b and ROI expression. Repetitive stimulation of monocytes with LPS leads to decreased responsiveness termed “endotoxin tolerance” (22). Relative endotoxin hyporesponsiveness in PICU patients may relate to endogenous endotoxin stimulation and exhaustion of neutrophils and monocytes in vivo. Neutrophils and monocytes from adults with sepsis have reduced activation and production of pro inflammatory cytokines (IL-1α, IL-6, TNF-α) in response to LPS (23, 24). Overwhelming sepsis is associated with a significant reduction of neutrophil chemotaxis (25) and adherence (26). This immune hyporeactivity state (immmunoparesis) during sepsis may explain the persistence of infection, late nosocomial infection, and death (27). In the PICU, immunoparalysis is associated with a higher risk of nosocomial infection and mortality (28).
Neutrophil CD11b expression in response to LPS increases in human whole blood in a dose-dependent manner (29). Neutrophil and monocyte CD11b expression is significantly increased in infected compared to non-infected and healthy neonates (30, 31). We have shown significantly increased neutrophil and monocyte CD11b expression in pediatric and adults controls vs. PICU patients. Recently APC has been shown to inhibit NETosis in neutrophils via a Mac-1 (CD11b) dependent mechanism (15, 32).
We showed increased neutrophil ROI release in response to LPS in both adult and pediatric controls compared to PICU patients. Increased ROI generation is often linked with tissue damage and is increased in multiorgan dysfunction (MOD) associated with the systemic inflammatory response syndrome (SIRS) in adult sepsis (33, 34). In addition, increased monocyte and neutrophil ROIs are associated with severe sepsis in adults (9). However, in this study LPS-induced ROI responses were suppressed in PICU patients, which may be associated with immunoparesis.
Neutrophil TLR4 expression in PICU patients was increased vs. pediatric and adult controls in response to LPS. APC significantly reduced neutrophil LPS-induced TLR4 expression in PICU patients only. Previous studies have concentrated only on baseline unstimulated innate immune cell function with APC. Viemann et al. found that baseline unstimulated neutrophil TLR4 expression was significantly higher in septic children vs. healthy controls (35). Previous studies have shown that baseline monocyte expression of TLR2 and TLR4 in patients with sepsis in intensive care is significantly up-regulated compared with healthy controls (5). Additionally, it has been demonstrated that baseline monocyte TLR4 expression is increased in septic compared to healthy neonates (36) and that isolated neutrophils and monocytes from adults with sepsis have higher mean fluorescence for TLR4 expression than cells from controls (37).
In this study, APC decreased the LPS-induced neutrophil ROI response in adults. However, this response was not seen in PICU patients as they were deemed LPS hyporesponsive and already demonstrated minimal ROI production. We found that despite using isolated neutrophils and not whole blood, there was no significant reduction in LPS-induced ROI with APC. These findings were supported by other groups and this response may be attributable to a plasma factor (38). APC decreased adult ROI LPS responses but this was not found in pediatric controls or PICU patients. The differences in adult and pediatric immune responses to APC may partially explain the differences in outcome of the adult APC sepsis trial vs. the pediatric groups in the PROWESS and RESOLVE trials. PICU non-survivors had increased neutrophil LPS responses which decreased with APC suggesting a possible role for APC or its variants in a subgroup of children with sepsis. However, in view of the potential for hemorrhage associated with APC treatment, the study of newer forms of APC with decreased haemorrhagic potential and enhanced anti-inflammatory effect is essential. A mutant APC generated with three alanine mutations (3K3A-APC) has been created and demonstrates potent neuroprotective properties and reduced anticoagulant activity. The creation of mutant APC forms with less anticoagulant activity may increase the utility of APC in sepsis especially in populations vulnerable to hemorrhage (39). Further studies (including protein engineering studies) aiming to minimize side-effects while preserving therapeutic and cytoprotective effects of APC are ongoing (40).
Eli Lilly and Company announced a worldwide voluntary market withdrawal of Xigris [Drotrecogin alfa (activated)] in 2011 following the PROWESS-SHOCK study which failed to show a survival benefit for patients with severe sepsis and septic shock (41). We have demonstrated that patients who undergo a sepsis evaluation in PICU have homogenous immune responses to endotoxin despite their heterogeneous diagnoses. Understanding endotoxin tolerance in PICU patients may assist in creating future therapies for pediatric sepsis. In addition, further understanding the sepsis-protective activities of variants of APC will be essential to explore how to administer APC in a clinical setting.
Data Availability Statement
All datasets generated for this study are included in the manuscript/supplementary files.
Ethics Statement
This prospective study was carried out in accordance with the recommendations of the National Children's Research Centre guidelines. Fully informed written parental consent was obtained from all pediatric participants and fully informed written consent was likewise obtained from all adult controls in compliance with the Declaration of Helsinki. The protocol was approved by the Institutional Research Ethics Committee in Our Lady's Children's Hospital Crumlin (OLCHC) Dublin.
Author Contributions
HE, TS, and EM composed and edited the manuscript. HE and TS analyzed data and put together the included data figures and tables. WW, FO'H, AO'N, and AB assisted in sample preparation, laboratory technique troubleshooting, data analysis, and advised on and edited the manuscript. IR, BP, MO'R, MH, BN, and OS assisted HE in patient recruitment, data collection, sample collection, and advised on and edited the manuscript.
Funding
This work was supported by National Children's Research Centre (NCRC), Crumlin, Dublin 12 and Royal College of Surgeons in Ireland.
Conflict of Interest
The authors declare that the research was conducted in the absence of any commercial or financial relationships that could be construed as a potential conflict of interest.
Acknowledgments
We want to thank the patients in PICU, children from the surgical day ward, their parents and the adult volunteers for their contribution in this study. A special thanks to all staff in pediatric intensive care unit, and to the all staff in hematology laboratory in OLCH Crumlin.
References
1. Watson RS, Carcillo JA, Linde-Zwirble WT, Clermont G, Lidicker J, Angus DC. The epidemiology of severe sepsis in children in the United States. Am J Resp Crit Care Med. (2003) 167:695–701. doi: 10.1164/rccm.200207-682OC
2. Huston JM, Rosas-Ballina M, Xue X, Dowling O, Ochani K, Ochani M, et al. Cholinergic neural signals to the spleen down-regulate leukocyte trafficking via CD11b. J Immunol. (2009) 183:552–9. doi: 10.4049/jimmunol.0802684
3. Reddy RC, Chen GH, Tekchandani PK, Standiford TJ. Sepsis-induced immunosuppression. Immunol Res. (2001) 24:273–87. doi: 10.1385/IR:24:3:273
4. Medzhitov R, Preston-Hurlburt P, Janeway CA Jr. A human homologue of the drosophila toll protein signals activation of adaptive immunity. Nature. (1997) 388:394. doi: 10.1038/41131
5. Härter L, Mica L, Stocker R, Trentz O, Keel M. Increased expression of toll-like receptor-2 and-4 on leukocytes from patients with sepsis. Shock. (2004) 22:403–9. doi: 10.1097/01.shk.0000142256.23382.5d
6. Weirich E, Rabin RL, Maldonado Y, Benitz W, Modler S, Herzenberg LA, et al. Neutrophil CD11b expression as a diagnostic marker for early-onset neonatal infection. J Pediatr. (1998) 132:445–51. doi: 10.1016/S0022-3476(98)70018-6
7. Simms HH, D'amico R. Lipopolysaccharide induces intracytoplasmic migration of the polymorphonuclear leukocyte CD11b/CD18 receptor. Shock. (1995) 3:196–203. doi: 10.1097/00024382-199503000-00007
8. Russwurm S, Vickers J, Meier-Hellmann A, Spangenberg P, Bredle D, Reinhart K, et al. Platelet and leukocyte activation correlate with the severity of septic organ dysfunction. Shock. (2002) 17:263–8. doi: 10.1097/00024382-200204000-00004
9. Martins PS, Brunialti MK, Martos LS, Machado FR, Assunçao MS, Blecher S, et al. Expression of cell surface receptors and oxidative metabolism modulation in the clinical continuum of sepsis. Crit Care. (2008) 12:R25. doi: 10.1186/cc6801
10. Gessler P, Nebe T, Birle A, Haas N, Kachel W. Neutrophil respiratory burst in term and preterm neonates without signs of infection and in those with increased levels of C-reactive protein. Pediatr Res. (1996) 39:843–8. doi: 10.1203/00006450-199605000-00017
11. Esmon CT. The protein C anticoagulant pathway. Arterioscler Thromb Vasc Biol. (1992) 12:135–45. doi: 10.1161/01.ATV.12.2.135
12. Bernard GR, Vincent J-L, Laterre P-F, LaRosa SP, Dhainaut J-F, Lopez-Rodriguez A, et al. Efficacy and safety of recombinant human activated protein C for severe sepsis. N Engl J Med. (2001) 344:699–709. doi: 10.1056/NEJM200103083441001
13. Nadel S, Goldstein B, Williams MD, Dalton H, Peters M, Macias WL, et al. Drotrecogin alfa (activated) in children with severe sepsis: a multicentre phase III randomised controlled trial. Lancet. (2007) 369:836–43. doi: 10.1016/S0140-6736(07)60411-5
14. Goldstein B, Giroir B, Randolph A. International pediatric sepsis consensus conference: definitions for sepsis and organ dysfunction in pediatrics. Pediatr Crit Care Med. (2005) 6:2–8. doi: 10.1097/00130478-200501000-00049
15. Galley H, El Sakka N, Webster N, Lowes D, Cuthbertson B. Activated protein C inhibits chemotaxis and interleukin-6 release by human neutrophils without affecting other neutrophil functions. Br J Anaesth. (2008) 100:815–9. doi: 10.1093/bja/aen079
16. O'Hare FM, Watson W, O'Neill A, Grant T, Onwuneme C, Donoghue V, et al. Neutrophil and monocyte toll-like receptor 4, CD11b and reactive oxygen intermediates, and neuroimaging outcomes in preterm infants. Pediatr Res. (2015) 78:82–90. doi: 10.1038/pr.2015.66
17. O'Hare FM, Watson RW, O'Neill A, Blanco A, Donoghue V, Molloy EJ. Persistent systemic monocyte and neutrophil activation in neonatal encephalopathy. J Matern Fetal Neonatal Med. (2016) 29:582–9. doi: 10.3109/14767058.2015.1012060
18. Molloy EJ, O'Neill AJ, Doyle BT, Grantham JJ, Taylor CT, Sheridan-Pereira M, et al. Effects of heat shock and hypoxia on neonatal neutrophil lipopolysaccharide responses: altered apoptosis, Toll-like receptor-4 and CD11b expression compared with adults. Biol Neonate. (2006) 90:34–9. doi: 10.1159/000091743
19. Molloy EJ, O'Neill AJ, Grantham JJ, Sheridan-Pereira M, Fitzpatrick JM, Webb DW, et al. Labor promotes neonatal neutrophil survival and lipopolysaccharide responsiveness. Pediatr Res. (2004) 56:99–103. doi: 10.1203/01.PDR.0000130473.30874.B6
20. Molloy EJ, O'Neill AJ, Grantham JJ, Sheridan-Pereira M, Fitzpatrick JM, Webb DW, et al. Sex-specific alterations in neutrophil apoptosis: the role of estradiol and progesterone. Blood. (2003) 102:2653–9. doi: 10.1182/blood-2003-02-0649
21. Smith JA, Weidemann MJ. Further characterization of the neutrophil oxidative burst by flow cytometry. J Immunol Method. (1993) 162:261–8. doi: 10.1016/0022-1759(93)90391-J
22. Randow F, Syrbe U, Meisel C, Krausch D, Zuckermann H, Platzer C, et al. Mechanism of endotoxin desensitization: involvement of interleukin 10 and transforming growth factor beta. J Exp Med. (1995) 181:1887–92. doi: 10.1084/jem.181.5.1887
23. Munoz C, Carlet J, Fitting C, Misset B, Bleriot J, Cavaillon J. Dysregulation of in vitro cytokine production by monocytes during sepsis. J Clin Invest. (1991) 88:1747–54. doi: 10.1172/JCI115493
24. McCall CE, Grosso-Wilmoth LM, LaRue K, Guzman RN, Cousart SL. Tolerance to endotoxin-induced expression of the interleukin-1 beta gene in blood neutrophils of humans with the sepsis syndrome. J Clin Invest. (1993) 91:853–61. doi: 10.1172/JCI116306
25. Alves-Filho J, Tavares-Murta B, Barja-Fidalgo C, Benjamim C, Basile-Filho A, Arraes S, et al. Neutrophil function in severe sepsis. Endocr Metab Immune Disord. (2006) 6:151–8. doi: 10.2174/187153006777442404
26. Terregino CA, Lubkin CL, Thom SR. Impaired neutrophil adherence as an early marker of systemic inflammatory response syndrome and severe sepsis. Ann Emerg Med. (1997) 29:400–3. doi: 10.1016/S0196-0644(97)70353-6
27. Le Tulzo Y, Pangault C, Amiot L, Guilloux V, Tribut O, Arvieux C, et al. Monocyte human leukocyte antigen–DR transcriptional downregulation by cortisol during septic shock. Am J Resp Crit Care Med. (2004) 169:1144–51. doi: 10.1164/rccm.200309-1329OC
28. Hall MW, Knatz NL, Vetterly C, Tomarello S, Wewers MD, Volk HD, et al. Immunoparalysis and nosocomial infection in children with multiple organ dysfunction syndrome. Intens Care Med. (2011) 37:525–32. doi: 10.1007/s00134-010-2088-x
29. Nandate K, Ogata M, Tamura H, Kawasaki T, Sata T, Shigematsu A. N-acetyl-cysteine attenuates endotoxin-induced adhesion molecule expression in human whole blood. Anesth Analg. (2005) 100:1453–7. doi: 10.1213/01.ANE.0000148616.24996.E7
30. Genel F, Atlihan F, Gulez N, Kazanci E, Vergin C, Terek DT, et al. Evaluation of adhesion molecules CD64, CD11b and CD62L in neutrophils and monocytes of peripheral blood for early diagnosis of neonatal infection. World J Pediatr. (2012) 8:72–5. doi: 10.1007/s12519-011-0304-6
31. Adib M, Ostadi V, Navaei F, Fosoul FS, Oreizi F, Shokouhi R, et al. Evaluation of CD11b expression on peripheral blood neutrophils for early detection of neonatal sepsis. Iran J Allergy Asthma Immunol. (2007) 6:93-6.
32. Jimenez MF, Watson RWG, Parodo J, Evans D, Foster D, Steinberg M, et al. Dysregulated expression of neutrophil apoptosis in the systemic inflammatory response syndrome. Arch Surg. (1997) 132:1263–70. doi: 10.1001/archsurg.1997.01430360009002
33. Chen D, Li Y, Lan S, Pan X, Zhou L, Liang M, et al. The level and clinical significance of Toll-like receptor 4 in children with severe sepsis. Chin Crit Care Med. (2011) 23:475–7.
34. Armstrong L, Medford A, Hunter K, Uppington K, Millar A. Differential expression of Toll-like receptor (TLR)-2 and TLR-4 on monocytes in human sepsis. Clin Exp Immunol. (2004) 136:312–9. doi: 10.1111/j.1365-2249.2004.02433.x
35. Viemann D, Dubbel G, Schleifenbaum S, Harms E, Sorg C, Roth J. Expression of toll-like receptors in neonatal sepsis. Pediatr Res. (2005) 58:654. doi: 10.1203/01.PDR.0000180544.02537.FD
36. Sturn DH, Kaneider NC, Feistritzer C, Djanani A, Fukudome K, Wiedermann CJ. Expression and function of the endothelial protein C receptor in human neutrophils. Blood. (2003) 102:1499–505. doi: 10.1182/blood-2002-12-3880
37. Mosnier LO, Yang XV, Griffin JH. Activated protein C mutant with minimal anticoagulant activity, normal cytoprotective activity, and preservation of thrombin activable fibrinolysis inhibitor-dependent cytoprotective functions. J Biol Chem. (2007) 282:33022–33. doi: 10.1074/jbc.M705824200
38. Ranieri VM, Thompson BT, Barie PS, Dhainaut J-F, Douglas IS, Finfer S, et al. Drotrecogin alfa (activated) in adults with septic shock. N Engl J Med. (2012) 366:2055–64. doi: 10.1056/NEJMoa1202290
39. Healy LD, Puy C, Fernández JA, Mitrugno A, Keshari RS, Taku NA, et al. Activated protein C inhibits neutrophil extracellular trap formation in vitro and activation in vivo. J Biol Chem. (2017) 292:8616–29. doi: 10.1074/jbc.M116.768309
40. Healy LD, Rigg RA, Griffin JH, McCarty OJ. Regulation of immune cell signaling by activated protein C. J Leukocyte Biol. (2018) 103:1197–203. doi: 10.1002/JLB.3MIR0817-338R
Keywords: PICU, Sepsis, APC, CD11b, TLR4, ROI, LPS
Citation: Eliwan HO, Watson WRG, Regan I, Philbin B, O'Hare FM, Strickland T, O'Neill A, O'Rourke M, Blanco A, Healy M, Nolan B, Smith O and Molloy EJ (2019) Pediatric Intensive Care: Immunomodulation With Activated Protein C ex vivo. Front. Pediatr. 7:386. doi: 10.3389/fped.2019.00386
Received: 10 March 2019; Accepted: 06 September 2019;
Published: 27 September 2019.
Edited by:
Raffaele Badolato, University of Brescia, ItalyReviewed by:
Yuying Liu, University of Texas Health Science Center at Houston, United StatesBeatriz Elena Marciano, National Institutes of Health (NIH), United States
Copyright © 2019 Eliwan, Watson, Regan, Philbin, O'Hare, Strickland, O'Neill, O'Rourke, Blanco, Healy, Nolan, Smith and Molloy. This is an open-access article distributed under the terms of the Creative Commons Attribution License (CC BY). The use, distribution or reproduction in other forums is permitted, provided the original author(s) and the copyright owner(s) are credited and that the original publication in this journal is cited, in accordance with accepted academic practice. No use, distribution or reproduction is permitted which does not comply with these terms.
*Correspondence: Eleanor J. Molloy, ZWxlYW5vci5tb2xsb3kmI3gwMDA0MDt0Y2QuaWU=