- 1Department of Pediatric, Hospital Universitario Virgen de la Arrixaca, Murcia, Spain
- 2Biomedical Research Institute of Murcia (IMIB), Palmar, Spain
- 3Department of Surgery, Pediatric, Obstetric and Gynaecology, University of Murcia, Murcia, Spain
Asthmatic adults with lower lung function have been described as having had this worse condition early in life. Lung function is reduced in children with persistent asthma and continues low throughout adult life. The challenge is to know if impaired lung function is a risk factor of asthma, as a consequence of special congenital characteristics of the airways, or whether asthmatic patients suffer a loss in lung function as early as 9 years of age as a consequence of very precocious remodeling of the airways. The loss is so early in life that it is probably a congenital characteristic, however there is not a cut-off point with clinical interest to predict risk of asthma later in life. There are contradictory results regarding whether asthmatic children lose lung function as a consequence of the airway remodeling by the illness itself. This aspect seemed to be shown for children at risk—the offspring of asthmatic mothers. The early BHR seems to be very frequent even in healthy infants, but is probably not a risk factor for asthma years later; except in the offspring of asthmatic mothers in which it has been shown. There are still many uncertainties in this field; so, more research is needed in order to better understand the pathophysiology of asthma, the early risk factors and to design new therapeutic targets and early interventions to change the natural history of the disease.
The Global Initiative for Asthma (GINA) (1) defines asthma as “a heterogeneous disease, generally characterized by chronic inflammation of the respiratory tract. It is defined by the history of symptoms such as wheezing, dyspnoea, chest tightness, and cough that vary with time and intensity, together with a variable limitation of the expiratory air flow.” Therefore, from the point of view of lung function, the essential characteristic in asthma is the variable limitation of the expiratory flow. In children, and especially in infants, the assessment of this limitation is not easy and not only due to a lack of collaboration, but also due to the lack of consensus regarding the best technique for clinical use, as well as the fact that there are no good reference equations available (2). Despite these limitations, just 3 years ago, the official practical guides by the ATS on recurrent and persistent wheezing recommended lung function studies in infants by means of the raised volume rapid thoracoabdominal compression (RVRTC) technique in order to assess its usefulness for better patient management (3).
It has been described that adult asthmatics who have a reduced lung function have had this condition since at least the age of 9 years (4). A recent metanalysis showed that serious infant asthma may be accompanied by a reduction in lung function that persists into adult life (5). The challenge is to know if the altered lung function is a risk factor for asthma, as a consequence of congenital characteristics in the airways, or if asthmatic patients suffer from a loss in lung function as early as 9 years of age, or even before, as a consequence of an early remodeling of the airways.
Cohort studies offer the major advantage of enabling the factors that precede the development of asthma to be studied and, therefore, help us to establish risk criteria. With regard to the development of lung function and asthma throughout life what has been learnt from cohort studies can be approached from different points of view:
(1) Is congenital reduced lung function a risk factor for the future development of asthma?
(2) Does asthma itself cause a loss in lung function in an early stage of life?
(3) Is the development of bronchial hyperresponsiveness (BHR) a risk factor for future asthma?
Is Congenital Reduced Lung Function a Risk Factor for Future Asthma?
Several cohort studies have demonstrated that altered lung function in the first weeks of life and before the first wheezing episode is subsequently associated with asthma (Table 1) (6–12, 14, 16–18).
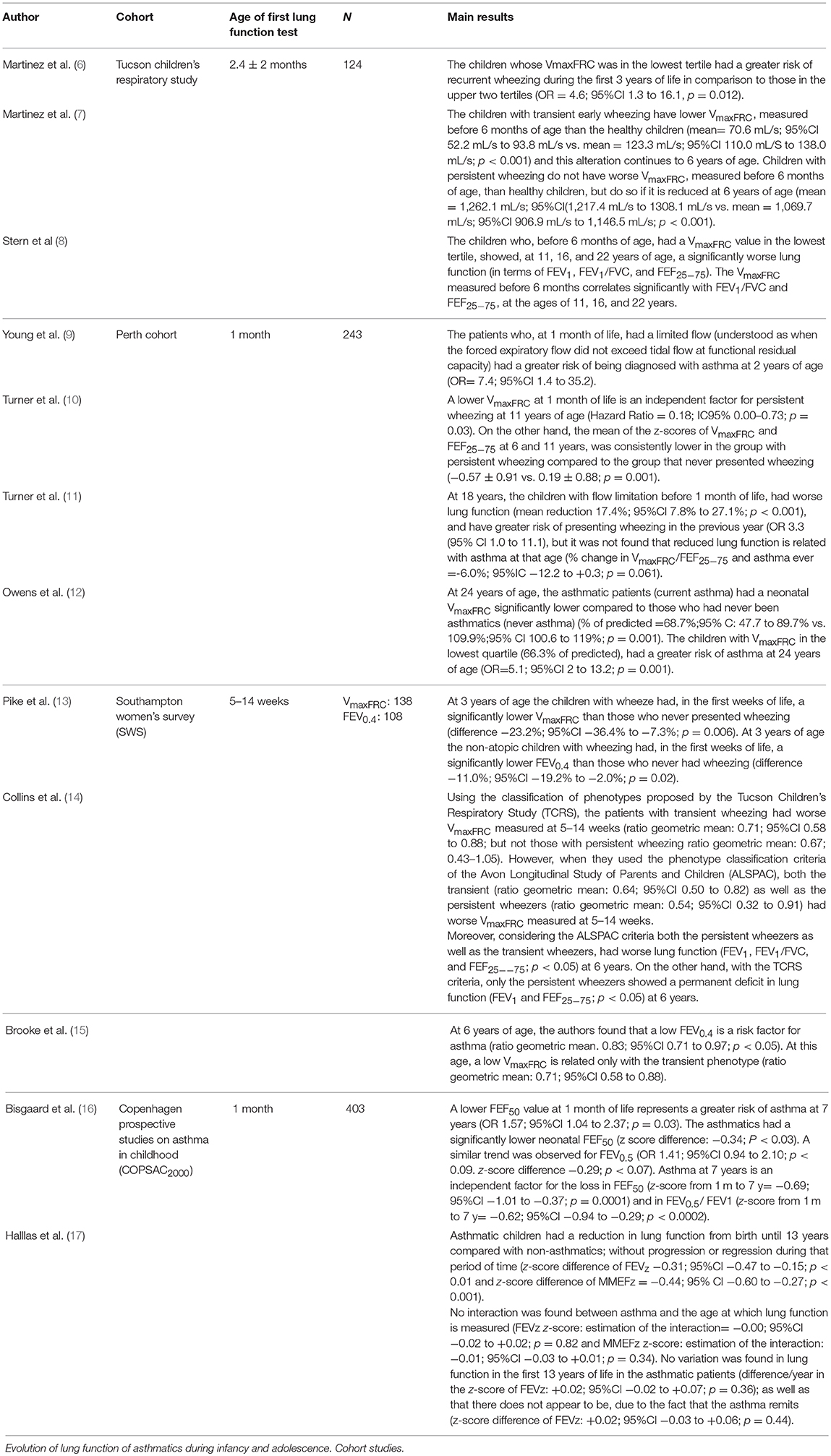
Table 1. Relationship of lung function measured in new-borns and infants and its relationship with asthma.
The Tucson Children's Respiratory Study (TCRS) was the first study designed to clarify if respiratory infections in the lower airways led to lesser lung function, or whether an early deteriorated lung function—prior to the first airway infection—increased the risk of wheezing. In that prospective study, the pre-morbid total respiratory conductance was measured in 124 neonates and the authors informed that the risk of having a wheezing episode was 3.7 times greater (95%CI 0.9 to 15.5; p = 0.06) among infants whose measurements were in the lowest tertile, in comparison with those infants with values in the highest two tertiles (18). This led the authors to suggest that the initial diameter of the airway and other specific characteristics of the lung parenchyma could predispose to wheezing during infancy with airway respiratory viruses. Moreover, the same study showed, by evaluating lung function through Rapid Thoracoabdominal Compression (RTC) to obtain the maximum flow to the functional residual capacity (VmaxFRC), that those children whose VmaxFRC was in the lowest tertile had a greater risk of recurrent wheezing during the first 3 years of life in comparison with those in the upper two tertiles (OR = 4.6; 95%CI 1.3 to 16.1, p = 0.012) (6).
Several years later, in 1995 and when the cohort had reached 6 years of age, the study published three phenotypes of children with wheeze: (1) transient wheezing; (2) late-onset wheezing; and (3) persistent wheezing. It showed a low pre-morbid VmaxFRC measured before the age of 6 months, only in the phenotype of transient wheezing. However, at 6 years of age it was reduced both in those children with the transient wheezing phenotype, as well as in those that presented the persistent phenotype (7) (Table 1). This suggests that some children have a congenital deteriorated lung function (phenotype of transient wheezing), but that, in other children, the lung function is deteriorated very early on; before the age of 6 years of life.
In 2007, the result of the follow-up of lung function to the age of 22 years of the Tucson cohort was published (8): the authors found that infant lung function correlated with the measurements of the expiratory volume and flows (FEV1, FEF25−75, and FEV1/FVC) between 11 and 22 years and that the patients in the lowest quartile of infant lung function had a ratio of FEV1, FEF25−75, and FEV1/FVC lower at 22 years of age than those in the upper three quartiles together. In the words of the authors: this finding suggests that those persons with obstruction in the airflow at birth will have greater probabilities of remaining in the lowest end of the distribution until adult life (8). However, they did not find that patients with the lowest VmaxFRC had a greater risk of asthma at 11, 16, or 22 years. The authors even speculated about the possibility that these infants with low lung function at birth with transient wheezing may be in danger of developing chronic obstructive pulmonary disease and that the prevention of this disease could begin intrauterine.
In summary, we could state that the principal findings of this cohort are that: (1) a group of infants has a congenitally diminished lung function; (2) said alteration in lung function persists until adult life; and (3) said functional alteration is a risk factor for wheezing in the first 3 years of life, but is not a risk factor for asthma, at least at 11, 16, and 22 years of age.
The Perth cohort (9–12) also showed a relationship between low pre-morbid lung function and the subsequent diagnosis of asthma (Table 1): they found that infants with flow limitation (which they defined as when the value of VmaxFRC is not greater than the current volume at functional residual capacity) have a greater risk of asthma at 2 years of age (9). On the other hand, at 11 years of age, the VmaxFRC at 1 month of life is an independent risk factor for persistent asthma (Hazard Ratio: 0.18; 95%IC 0.00 to 0.73; p = 0.03) and the lung function z-score (i.e., VmaxFRC at 1 month and FEF25−75 at 6 and 11 years of age) is significantly lower in the group with persistent asthma compared to the non-asthmatic group (p = 0.001) (10). These results contradict those described in the Tucson study, since the Australian study suggests that the development of persistent asthma until school age is, at least partially, predetermined by a congenitally affected lung function.
That same study found that, at 18 years of age (11), those children with flow limitation before 1 month of life, had worse lung function and, additionally, greater risk of presenting wheezing in the previous year (Table 1). However, they did not find reduced lung function to be related with persistent asthma; although the trend seems clear and was found to be very near to statistical significance (% change in VmaxFRC/FEF25−75–asthma ever = −6.0%; 95%IC −12.2 to +0.3; p = 0.061). In fact, some years later in this same cohort it was found, at the age of 24 years, that the asthmatic patients had a neonatal VmaxFRC significantly lower compared to those who had never been asthmatic (% of predicted = 68.7%; 95% C: 47.7 to 89.7% vs. 109.9%; 95% CI 100.6 to 119%; p = 0.001) and also that those neonates with VmaxFRC in the lowest quartile (66.3% of predicted), had a greater risk of asthma at 24 years (OR = 5.1; 95%CI 2 to 13.2; p = 0.001) (12). Another interesting aspect of this evaluation at 24 years of age is that the patients whose asthma remitted had a neonatal lung function significantly better that those who suffered from persistent asthma (mean VmaxFRC = 105%; 95% CI: 88–123% vs. 69%; 95% CI: 53–84%; P = 0.003) (12).
Thus, the Perth cohort shows that neonatal reduction in lung function is a risk factor for persistent asthma throughout life and that this functional respiratory limitation is also maintained, at least, until youth. The authors themselves (12) suggest that “subjects with more significant asthma symptoms had a defect in lung development or growth in utero or very early in life that persisted as a reduction in lung function into adulthood.”
It is not easy to analyse the reason for such contradictory results between the Tucson and Perth cohorts. The limited number of patients recruited is probably responsible. It should be noted that the Tucson cohort, which did not find such differences, in the measurements taken around 2 months of life the group of those that will have persistent asthma is only represented by 16 patients compared to the 67 children without wheezing. In the Perth cohort, the number of patients in the persistent wheezing group was 17 vs. the 67 who had never had wheezing. On the other hand, the phenotype classification criteria may not coincide exactly between the two studies. In this sense, Collins et al. (14) performed, with the data from the Southampton Women's Survey (SWS) cohort, the comparative analysis among the six phenotypes proposed in the Avon Longitudinal Study of Parents and Children (ALSPAC) (19) with the four phenotypes proposed by the TCRS and showed that using the ALSPAC criteria both the children with transient wheezing as well as those who suffered from persistent wheezing, had a low VmaxFRC in the first weeks of life, but that using the TCRS criteria, only the transient ones showed that deficit. The persistent group in the TCRS study included two ALSPAC sub-groups: those with very early-onset wheezing, around 6 months of age, and those who began shortly after, around 18 months. These latter ones, named as the intermediate-onset group by ALSPAC, did not have lung function alterations in the first weeks of life [according to the study by Collins et al. (19)], whilst the persistent wheezing group did. An overrepresentation of “intermediate-onset” patients in the “persistent” group of the TCRS study could explain the absence of differences in the VmaxFRC, in the first weeks of life, when the persistent wheezing group is compared with the non-wheezers. Another argument in favor of this possibility is that, from 6 years of age, the TCRS persistent wheezers (7) and both the intermediate-onset and the persistent wheezers in the ALSPAC (19), had a limited lung function compared to the group that never had wheeze. Thus, are these incongruences merely a problem of classification criteria and of sample size? The answer is probably yes.
The Southampton Women's Survey cohort (13) was the first to measure the forced expiratory volume in 0.4 s (FEV0.4) by means of the RVRTC technique, as well as the VmaxFRC. The authors found, once again, a statistically significant association between low VmaxFRC with suffering wheezing until 3 years of age (Table 1). On the other hand, it was the non-atopic children who still had wheezing into their third year of life who had the lowest FEV0.4, measured between 5 and 14 weeks of life; the values of FEV0.4 in the atopic children were not significantly different among those who had had wheezing or had not (p = 0.4). This suggests that the caliber of the lower airway, in some children, may be a risk factor for asthma, independently of the atopic status. That cohort also showed that, at 6 years of age, a low neonatal FEV0.4 is a risk factor for asthma. Although, at that age, a low neonatal VmaxFRC is associated only with the transient phenotype (15).
The Copenhagen Prospective Studies on Asthma in Childhood (COPSAC) cohort (16) found that those patients diagnosed as asthmatic at 7 years of age had a lower lung function when they were neonates, specifically the forced expiratory flow at 50% of the forced vital capacity (FEF50); moreover, the drop in the neonatal FEF50 is a risk factor for asthma at that age (Table 1). Similar trends were observed with the FEV0.5. The Danish cohort did not classify the patients by phenotype. Another important finding was that at 7 years, the asthmatic patients showed a reduced lung function in terms of FVC (p = 0.008), FEV1 (p < 0.0001), FEV1/FVC (p = 0.0001), and FEF50 (p < 0.0001). The recent publication of the results obtained with the follow-up until 13 years (17) showed similar results: the children that developed asthma already had a reduced lung function as neonates and this remains altered to the age of 13 years compared to the non-asthmatics (Table 1). The COPSAC study (16, 17) was designed to research into the development of asthma in children at risk; it is a prospective clinical study of a cohort recruited at birth because their mothers had a history of asthma diagnosed by a physician after the age of 7 years; that may affect the external validity of the conclusions. Its principal strength, however, lies in the fact that the number of neonates whose lung function was measured was 403.
Thus, with the exception of the Tucson Children's Respiratory Study, the rest of the cohorts coincide in that the lung function was worse, measured in the first months of life, in those children who developed asthma, and that said lung function is determined very early on, probably congenitally, and remains altered throughout life; at least into their youth. It is important that it has not been described whether a cut-off point with clinical interest exists to predict the future asthma risk.
What Factors Are Related With Early Loss in Lung Function?
Several factors related with the early loss in lung function have been described:
1. Prematurity. It has been described that lung function in infants born pre-term is diminished (20, 21) and that this reduction probably remains throughout their life, at least between the ages of 4 and 19 years (22).
2. Pre-natal exposure to Tobacco. The Perth cohort found that the VmaxFRC of those infants whose mothers smoked during the pregnancy was significantly lower than that of the children of non-smoker mothers (p = 0.05) (23). The COPSAC cohort studied the factors that determine neonatal lung function and found a 7% loss in the FEV0.5 in the children of smoker mothers (ß = 0.930; 95%CI 0.878 to 0.985; p = 0.013) (24).
3. Increase in weight in the first months of life. The COPSAC cohort found that the neonatal FEV0.5 was 14% lower in children with a body mass index in the uppermost quartile (24). On the other hand, the lung function at 6 weeks of life was not significantly different in babies born small for their gestational age compared to new-borns with appropriate weight for their gestational age (25). Perhaps the weight at a specific moment is not as important as its overly rapid increase, since the Perth cohort found that during the first year of life, the VmaxFRC was 10.39 mL lower per Kg of weight of the child (23) and they described that the gain in VmaxFRC in the first year of life was inversely related to the weight gain in that same period of time (26). One recent publication (27) has studied the relationship between fetal weight gain (estimated by means of ultrasound) and weight gain during the first year of life, with the lung function measured at 10 years of age. That study found a close relationship between the patterns of fetal growth and during the first year of life with lung function; thus, those children with restricted fetal weight growth had worse FEV1 independently of whether they caught-up or not compared to children with normal fetal and infant growth patterns. However, the authors appreciated that the growth pattern of accelerated fetal weight if it is followed by an accelerated growth pattern in infancy is accompanied by a significant increase in FVC and a decrease in the FEV1/FVC ratio without affecting the FEV1; therefore, disynaptic development with the accelerated weight gain is found. Unfortunately, this cohort does not report lung function measurements in the first months of life.
A metanalysis of lung function in 24 European cohorts compiling the data of 24,938 children checked that those with a greater monthly weight increase, in the first year of life, had a lower FEV1/FVC when their lung function was measured between the ages of 4 and 19 years, suggesting a disynaptic development of lung function in children with a greater weight gain during infancy (22).
4. Genetics. Some information regarding the prenatal determination of lung function has been contributed by genetic studies in recent years. Different single nucleotide polymorphisms (SNP) have been studied in different genes. Thus, in a cohort of 73 children recruited if one of their parents were atopic (28), a reduction in neonatal VmaxFRC was demonstrated in the children with the polymorphism Gln27 in some allele in the beta-adrenergic receiver gene when compared with the polymorphism Glu27Glu (95%CI of difference, −68 to −10; p = 0.011) and also in children with the SNP as Arg16 in some allele compared with those that did not have Arg16 (95% CI of difference −1.06 to −0.10; p = 0.02). Additionally, the 19 children of that cohort with the SNP Arg16 and Gln27 in some allele, had a clearly marked reduction in neonatal VmaxFRC compared to those that had no allele with such SNP (81 ± 36 mL/s vs. 145 ± 66; p < 0.001, respectively).
The influence of genetical variants was studied in the COPSAC cohort (29), which prior studies had demonstrated to be associated with adult lung function; to do so, 26 SNP were selected related with the FEV1/FVC ratio and eight SNP related with FEV1. The authors found no relationship with neonatal lung function but did find a relationship with the lung function measured at 7 years of age, thus suggesting that this group of genes is important in lung development, and opens a window of opportunity in these first years for interventions in the expression of these genes. They additionally did not find a relationship between different SNP of the Vascular Endothelial Growth Factor-A (VEGF-A) gene and neonatal lung function, although a relationship was found at school age (30), so it would seem that these genes also play a more important role in the development of post-natal lung function. However, some years before it had been described that the homozygotes CC of the rs3025028 SNP in the VEGF-A gene had significantly higher V'maxFRC and higher z-scores of FEF50 and FEF25−75 compared with other genotypes (31). The results cannot be considered contradictory since the SNP of the VEGF-A gene studied in both studies are different.
In the SWS cohort (32) the association of SNP was studied in four different genes and it was found that the rs1529672 SNP of the retinoic acid receptor b (RARB) gene and the rs12477314 of the histone deacetylase 4 (HDAC4) gene were associated with a greater VmaxFRC.
The Perth cohort (33) studied the significant association of the A38G secretoglobin 1A1 (SCGB1A1) gene and, although they found no significant relationship with the VmaxFRC, they did find it with bronchial hyperresponsiveness.
The finding of the interaction between intrauterine tobacco exposure and the expression of the Transferase genes (GSTT1, GSTP1) that are associated with a reduction in the VmaxFRC and to hyperresponsiveness in the exposed infants is particularly interesting (34).
Does Asthma Itself Cause a Loss in Lung Function at an Early Stage of Life?
The data of cohorts that study the evolution of lung function from infancy to adulthood (4, 5, 35) show that patients with persistent asthma have a worse lung function both in adulthood as well as in infancy, but the differences with non-asthmatics do not change over time; this suggests that the loss occurs before the age at which the lung function was first evaluated in the cohorts (9 and 7 years, respectively).
The Tucson Children's Respiratory Study found a lower lung function in patients with persistent asthma at 6, 11, and 16 years(36), but not at 2 months of life; suggesting a structural change in the airways (airway remodeling) as a consequence of the asthma itself, before the age of 6 years. However, there does not seem to be any further drop with age with respect to the rest of the phenotypes nor with respect to those who never wheezed (36); therefore, the airway remodeling, if it indeed exists, must occur very early on in life (that is to say, before 6 years of age) and not progress later on in life.
However, the Perth cohort (10) showed that lung function was permanently low in persistent asthmatics in comparison with the non-asthmatic group, which suggests a congenitally-affected lung function. The data at 24 years of this cohort confirm this suggestion since the adult asthmatic patients showed a persistently worse lung function than the non-asthmatics (mean reduction: 16.2%; 95%CI 8.1 to 24.3%; p < 0.0001 considering VmaxFRC until 12 months and FEF25−75 from 6 years) (11). In this same cohort, the authors studied some risk factors for loss in lung function (12) and did not find that persistent asthma, in itself, reduced the lung function between 1 month and 18 years, although there seems to be a trend that is very near to statistical significance, since the percentage of change in the VmaxFRC-FEF25−75 related with the history of asthma was −6.0%; 95%IC −12.2 to +0.3; p = 0.061.
The authors of the COPSAC study (16) found that at 7 years the asthmatic patients, in comparison with the non-asthmatics, not only had had a lower lung function when they were neonates, but also suffered a progressive decrease in those 7 years in both FEF50 and in FEV0.5 (neonatal)/FEV1 (at 7 years). However, in the most recent publication on this cohort (17) when the children reached 13 years, the authors, with the aim of demonstrating whether there was a relationship between lung function loss and asthma development, did not find an interaction between asthma and the age that the measurement was performed, which suggests to them that the lung function in asthmatics is predetermined at a very early age, probably congenitally, and remains stable throughout infancy, even though the symptoms may cease. It is interesting that they also analyzed the change in lung function for each year of having asthma and found no significant variations; there do not seem to be any variations due to asthma remission either (Table 1). Using the authors own words (17): The lung function deficit was present before the children developed asthma, did not progress with symptoms, and remained even if symptoms ceased.
Therefore, with the exception of the TRCS cohort (36), the rest of the cohorts did not find the evolution of asthma to be a risk factor for losses in lung function and there seems to be a lower, but parallel, trajectory in lung function of asthmatics compared to healthy subjects, at least, until youth.
One interesting study assessed the pathological characteristics associated to airway remodeling in biopsies of bronchial mucosa, in children under the age of 2 years with recurrent wheezing compared with healthy children. No difference was found with regard to the thickness of the basal membrane, or in the number of inflammatory cells, and they concluded that said alterations are not present in symptomatic infants with reversible obstruction to the flow, even when they are atopic (37). Some years later, they reassessed the same biopsies when the patients had already reached 8 years and once again found no relationship between the thickness of the basal membrane nor the smooth bronchial muscle area with the diagnosis of asthma, lung function, nor atopic status, at that age (38). The results suggest that the pathological process initiated by asthma seems to be different from the eosinophilic inflammation and the airway remodeling characteristic of asthma in adults.
Is the Development of Bronchial Hyperresponsiveness at an Early age a Risk Factor for Asthma?
Bronchial hyperresponsiveness (BHR) was assessed in the first months of life in some studies (17, 28, 39–43) with the aim of analyzing its relationship with the subsequent development of asthma (Table 2). The first article of this type was published in 1992 by Clark et al. (39), who evaluated BHR by means of increasing doses of inhaled histamine to determine the concentration that caused a 30% decrease in VmaxFRC (PC30) in 45 infants; 23 of whom had previously suffered lower respiratory tract infections (LRTI); and 22 had not. They did not find differences in the PC30 between the infants with LTRI and those without, which suggests that, at the age of 6 months, BHR does not discriminate between those with or without LTRI and, for those authors, the lower respiratory tract symptoms are not associated with the capacity of the bronchial response. That same cohort was studied at 10 years of age and the authors found no correlation between PC30 measured around 6 months of age with PC20 evaluated at 10 years of age; on the other hand, they found that a lower PC30 in infancy is a risk factor for suffering wheezing until 4 years, but that it is not so at 10 years of age (28) (Table 2). That is to say, the authors proposed that the early transient wheeze phenotype is related with BHR in the first months of life, but that early BHR is not associated with BHR nor wheezing at 10 years of age.
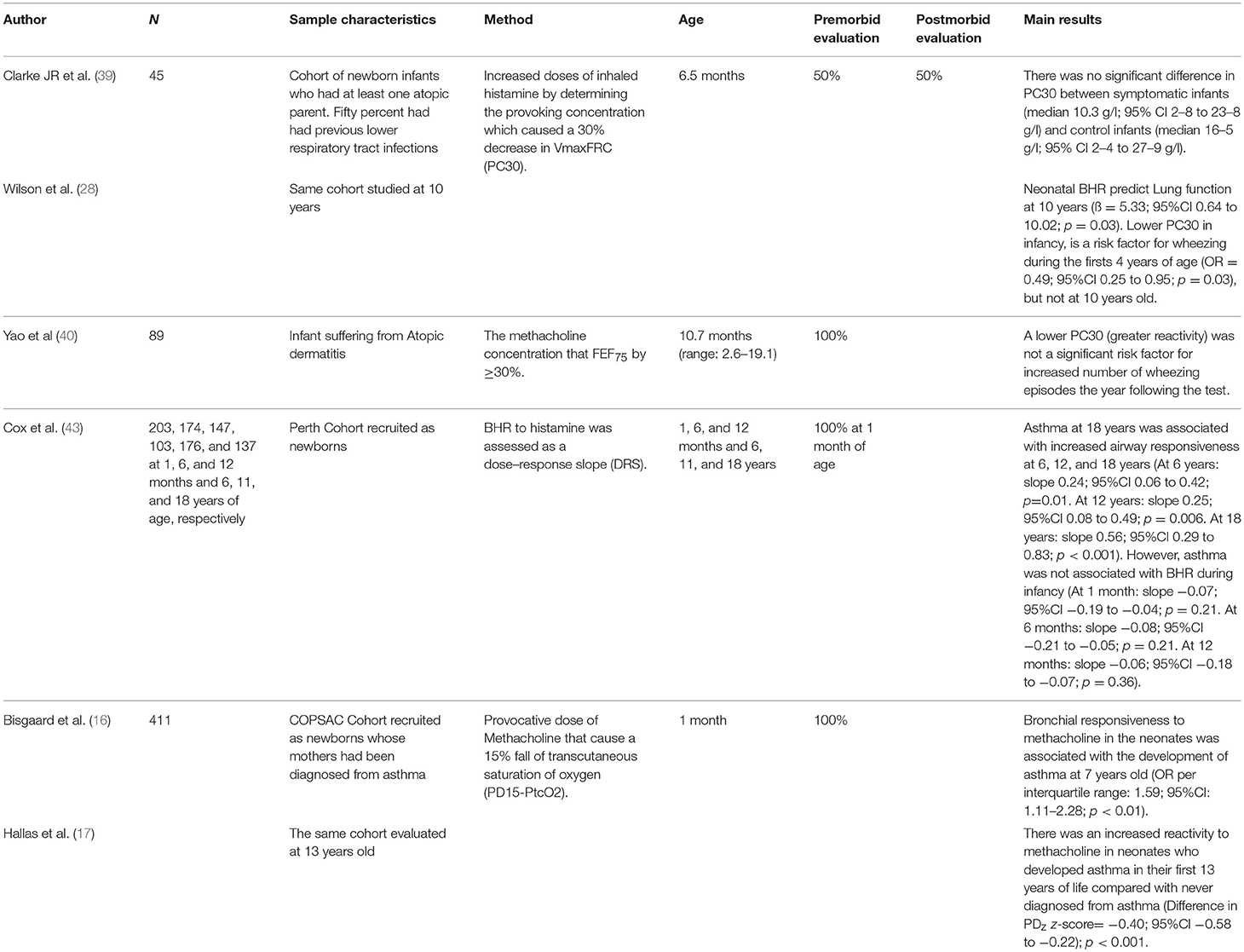
Table 2. Relationship between bronchial hyperresponsiveness (BHR) assessed in infants and asthma later in life.
The BHR was assessed in a cohort of children diagnosed with atopic dermatitis, by means of the concentration of methacholine that could decrease the forced expiratory flow at 75% of the expired volume (FEF75) by ≥30%, at an age range of between 2.6 and 16.9 months (40). The methacholine test was carried out before any episode of wheezing in all the infants. The authors' conclusion was that a higher BHR is not a risk factor for a greater number of wheezing episodes in the year following the test (p > 0.12).
The Perth cohort also evaluated the relationship between BHR, by means of the dose-response slope, and the subsequent development of asthma (41–43). They performed the BHR test at 1 month of life, at 6 and 12 months and also at 6, 11, and 18 years of age. The authors concluded that the asthma at 18 years was associated with BHR measured at 6 years (p = 0.01), 12 years (p = 0.006), and 18 years (p < 0.001) but not with that determined during infancy; i.e., at 1 month (p = 0.21), 6 months (p = 0.21), and 12 months (0.36) (43). The authors proposed that the BHR during infancy may reflect the initial geometry of the airways in the first years of life, but that from school age BHR could be a consequence of the effect of the immunological and environmental factors on the airways and, therefore, the response capacity of the airways measured later on in infancy becomes a better predictor of future asthma (43).
All these studies concur in considering that, although BHR may be present very early on in infancy, it bears no association on future asthma. In fact, they concur with the study by Montgomery and Tepper (44) in healthy infants, in which the authors described the decrease in sensitivity to methacholine as age increases.
The Copenhagen cohort, on the other hand, shows that BHR evaluated at 1 month of life is a risk factor for asthma at 7 years of age (Table 2) (16). These results were corroborated shortly after, when the participants of the cohort had reached 13 years (17), in such a way that the asthmatics had presented greater BHR at 1 month of life compared to the non-asthmatics. For the authors, this suggests that there are some inherent and stable characteristics, not caused by inflammation during symptomatic periods, that predispose to developing asthma, hyperresponsiveness, and intermittent bronchial obstruction (17).
Conclusions
In children with persistent asthma the lung function seems to be reduced at a very early age and remains low throughout their whole adult life. This loss so early on is probably a congenital characteristic, although factors such as prematurity, fetal, and immediate postnatal growth as well as exposure to tobacco smoke may also be important deleterious factors.
There are contradictory results as to whether asthmatic children lose lung function as a consequence of airway remodeling due to the disease itself; but the majority of the cohort studies find a lower but parallel path of lung function in asthmatics compared to non-asthmatics.
Bronchial hyperresponsiveness measured in the first months of life seems to be very frequent even in healthy infants, but is probably not a risk factor for asthma years later, and is only a risk factor for wheezing when it appears at pre-school age.
Great uncertainty still abounds in this field; therefore, further research is needed to better understand the physiopathology of asthma, its early risk factors and to design new therapeutic goals and early interventions to change the natural history of the disease.
Author Contributions
The author confirms being the sole contributor of this work and has approved it for publication.
Conflict of Interest Statement
The author declares that the research was conducted in the absence of any commercial or financial relationships that could be construed as a potential conflict of interest.
References
1. Global Initiative for Asthma. Global Strategy for Asthma Management and Prevention. (2018). Available online at: www.ginasthma.org (accessed November 5, 2018).
2. Rosenfeld M, Allen J, Arets BH, Aurora P, Beydon N, Calogero C, et al. An official American Thoracic Society workshop report: optimal lung function tests for monitoring cystic fibrosis, bronchopulmonary dysplasia, and recurrent wheezing in children less than 6 years of age. Ann Am Thorac Soc. (2013) 10:S1–11. doi: 10.1513/AnnalsATS.201301-017ST
3. Ren CL, Esther CR Jr, Debley JS, Sockrider M, Yilmaz O, Amin N, et al. Official american thoracic society clinical practice guidelines: diagnostic evaluation of infants with recurrent or persistent wheezing. Am J Respir Crit Care Med. (2016) 194:356–73. doi: 10.1164/rccm.201604-0694ST
4. Sears MR, Greene JM, Willan AR, Wiecek EM, Taylor DR, Flannery EM, et al. A longitudinal, population-based, cohort study of childhood asthma followed to adulthood. N Engl J Med. (2003) 349:1414–22. doi: 10.1056/NEJMoa022363
5. Ma H, Li Y, Tang L, Peng X, Jiang L, Wan J, et al. Impact of childhood wheezing on lung function in adulthood: a meta-analysis. PLoS ONE. (2018) 13:e0192390. doi: 10.1371/journal.pone.0192390
6. Martinez FD, Morgan WJ, Wright AI, Holberg CJ, Taussig LM, the Group Health Medical Associates. Initial airway function is a risk factor for recurrent wheezing respiratory illnesses during the first three years of life. Am Rev Respir Dis. (1991) 143:312–6. doi: 10.1164/ajrccm/143.2.312
7. Martinez FD, Wright AL, Taussig LM, Holberg CJ, Halonen M, Morgan WJ. Asthma and wheezing in the first six years of life. N Engl J Med. (1995) 332:133–8. doi: 10.1056/NEJM199501193320301
8. Stern DA, Morgan WJ, Wright AL, Guerra S, Martinez FD. Poor airway function in early infancy and lung function by age 22 years: a non-selective longitudinal cohort study. Lancet. (2007) 370:758–64. doi: 10.1016/S0140-6736(07)61379-8
9. Young S1, Arnott J, Le Souef PN, Landau LI. Flow limitation during tidal expiration in symptom-free infants and the subsequent development of asthma. J Pediatr. (1994) 124(5 Pt 1):681–8. doi: 10.1016/S0022-3476(05)81355-1
10. Turner SW, Palmer LJ, Rye PJ, Gibson NA, Judge PK, Cox M, et al. The relationship between infant airway function, childhood airway responsiveness, and asthma. Am J Respir Crit Care Med. (2004) 169:921–7. doi: 10.1164/rccm.200307-891OC
11. Turner S, Fielding S, Mullane D, Cox DW, Goldblatt J, Landau L, et al. A longitudinal study of lung function from 1 month to 18 years of age. Thorax. (2014) 69:1015–20. doi: 10.1136/thoraxjnl-2013-204931
12. Owens L, Laing IA, Zhang G, Le Souëf PN. Infant lung function predicts asthma persistence and remission in young adults. Respirology. (2017) 22:289–94. doi: 10.1111/resp.12901
13. Pike KC, Rose-Zerilli MJ, Osvald EC, Inskip HM, Godfrey KM, Crozier SR, et al. The relationship between infant lung function and the risk of wheeze in the preschool years. Pediatr Pulmonol. (2011) 46:75–82. doi: 10.1002/ppul.21327
14. Collins SA, Pike KC, Inskip HM, Godfrey KM, Roberts G, Holloway JW, et al. Validation of novel wheeze phenotypes using longitudinal airway function and atopic sensitization data in the first 6 years of life: evidence from the Southampton Women's survey. Pediatr Pulmonol. (2013) 48:683–92. doi: 10.1002/ppul.22766
15. Brooke E, Lucas JS, Collins SA, Holloway JW, Roberts G, Inskip H, et al. Infant lung function and wheeze in later childhood in the Southampton Women's Survey. Eur Respir J. (2014) 43: 919–21. doi: 10.1183/09031936.00131613
16. Bisgaard H, Jensen SM, Bønnelykke K. Interaction between asthma and lung function growth in early life. Am J Respir Crit Care Med. (2012) 185:1183–9. doi: 10.1164/rccm.201110-1922OC
17. Hallas HW, Chawes BL, Rasmussen MA, Arianto L, Stokholm J, Bønnelykke K, et al. Airway obstruction and bronchial reactivity from age 1 month until 13 years in children with asthma: a prospective birth cohort study. PLoS Med. (2019) 16:e1002722. doi: 10.1371/journal.pmed.1002722
18. Martinez FD, Morgan WJ, Wright AI, Holberg CJ, Taussig LM. Diminished lung function as a predisposing factor for wheezing respiratory illness in infants. N Engl J Med. (1988) 319:1112–7. doi: 10.1056/NEJM198810273191702
19. Henderson J, Granell R, Heron J, Sherriff A, Simpson A, Woodcock A, et al. Associations of wheezing phenotypes in the first 6 years of life with atopy, lung function and airway responsiveness in mid-childhood. Thorax. (2008) 63:974–80. doi: 10.1136/thx.2007.093187
20. Friedrich L, Stein RT, Pitrez PM, Corso AL, Jones MH. Reduced lung function in healthy preterm infants in the first months of life. Am J Respir Crit Care Med. (2006) 173:442–7. doi: 10.1164/rccm.200503-444OC
21. Hoo AF, Gupta A, Lum S, Costeloe KL, Huertas-Ceballos A, Marlow N, et al. Impact of ethnicity and extreme prematurity on infant pulmonary function. Pediatr Pulmonol. (2014) 49:679–87. doi: 10.1002/ppul.22882
22. den Dekker HT, Sonnenschein-van der Voort AMM, de Jongste JC, Anessi-Maesano I, Arshad SH, Barros H, et al. Early growth characteristics and the risk of reduced lung function and asthma: A meta-analysis of 25,000 children. J Allergy Clin Immunol. (2016) 137:1026–35. doi: 10.1016/j.jaci.2015.08.050
23. Young S, Sherrill DL, Arnott J, Diepeveen D, LeSouëf PN, Landau LI. Parental factors affecting respiratory function during the first year of life. Pediatr Pulmonol. (2000) 29:331–40. doi: 10.1002/(SICI)1099-0496(200005)29:5<331::AID-PPUL1>3.0.CO;2-A
24. Bisgaard H, Loland L, Holst KK, Pipper CB. Prenatal determinants of neonatal lung function in high-risk newborns. J Allergy Clin Immunol. (2009) 123:651–7, 657.e1–4. doi: 10.1016/j.jaci.2008.11.036
25. Lum S, Hoo AF, Dezateux C, Goetz I, Wade A, DeRooy L, et al. The association between birthweight, sex, and airway function in infants of nonsmokers mothers. Am J Respir Crit Care Med. (2001) 164:2078–84. doi: 10.1164/ajrccm.164.11.2104053
26. Turner S, Zhang G, Young S, Cox M, Goldblatt J, Landau L, et al. Associations between postnatal weight gain, change in postnatal pulmonary function, formula feeding and early asthma. Thorax. (2008) 63:234–9. doi: 10.1136/thx.2006.064642
27. den Dekker HT, Jaddoe VWV, Reiss IK, de Jongste JC, Duijts L. Fetal and infant growth patterns and risk of lower lung function and asthma. The generation R study. Am J Respir Crit Care Med. (2018) 197:183–192. doi: 10.1164/rccm.201703-0631OC
28. Wilson NM, Lamprill JR, Mak JC, Clarke JR, Bush A, Silverman M. Symptoms, lung function, and beta2-adrenoceptor polymorphisms in a birth cohort followed for 10 years. Pediatr Pulmonol. (2004) 38:75–81. doi: 10.1002/ppul.20049
29. Kreiner-Møller E, Bisgaard H, Bønnelykke K, Prenatal and postnatal genetic influence on lung function development. J Allergy Clin Immunol. (2014) 134:1036–42.e15. doi: 10.1016/j.jaci.2014.04.003
30. Kreiner-Møller E, Chawes BL, Vissing NH, Koppelman GH, Postma DS, Madsen JS, et al. VEGFA variants are associated with pre-school lung function, but not neonatal lung function. Clin Exp Allergy. (2013) 43:1236–45. doi: 10.1111/cea.12188
31. Simpson A, Custovic A, Tepper R, Graves P, Stern DA, Jones M, et al. Genetic variation in vascular endothelial growth factor-a and lung function. Am J Respir Crit Care Med. (2012) 185:1197–204. doi: 10.1164/rccm.201112-2191OC
32. Collins SA, Lucas JS, Inskip HM, Godfrey KM, Roberts G, Holloway JW, et al. HHIP, HDAC4, NCR3 and RARB polymorphisms affect fetal, childhood and adult lung function. Eur Respir J. (2013) 41:756–7. doi: 10.1183/09031936.00171712
33. Laing IA, de Klerkz NH, Turner SW, Judge PK, Hayden CM, Landau LI, et al. Cross-sectional and longitudinal association of the secretoglobin 1A1 gene A38G polymorphism with asthma phenotype in the Perth Infant Asthma Follow-up cohort. Clin Exp Allergy. (2009) 39:62–71. doi: 10.1111/j.1365-2222.2008.03102.x
34. Murdzoska J, Devadason SG, Khoo SK, Landau LI, Young S, Goldblatt J, et al. In utero smoke exposure and role of maternal and infant glutathione S-transferase genes on airway responsiveness and lung function in infancy. Am J Respir Crit Care Med. (2010) 181:64–71. doi: 10.1164/rccm.200812-1887OC
35. Phelan PD, Robertson CF, Olinsky A. The Melbourne asthma study: 1964–1999. J Allergy Clin Immunol. (2002) 109:189–94. doi: 10.1067/mai.2002.120951
36. Morgan WJ, Stern DA, Sherrill DL, Guerra S, Holberg CJ, Guilbert TW, et al. Outcome of asthma and wheezing in the first 6 years of life: follow-up through adolescence. Am J Respir Crit Care Med. (2005) 172:1253–8. doi: 10.1164/rccm.200504-525OC
37. Saglani S, Malmström K, Pelkonen AS, Malmberg LP, Lindahl H, Kajosaari M, et al. Airway remodeling and inflammation in symptomatic infants with reversible airflow obstruction. Am J Respir Crit Care Med. (2005) 171:722–7. doi: 10.1164/rccm.200410-1404OC
38. Malmström K, Malmberg LP, O'Reilly R, Lindahl H, Kajosaari M, Saarinen KM, et al. Lung function, airway remodeling, and inflammation in infants: outcome at 8 years. Ann Allergy Asthma Immunol. (2015) 114:90–6. doi: 10.1016/j.anai.2014.09.019
39. Clarke JR, Reese A, Silverman M. Bronchial responsiveness and lung function in infants with lower respiratory tract illness over the first six months of life. Arch Dis Child. (1992) 67:1454–8. doi: 10.1136/adc.67.12.1454
40. Yao W, Barbé-Tuana FM, Llapur CJ, Jones MH, Tiller C, Kimmel R, et al. Evaluation of airway reactivity and immune characteristics as risk factors for wheezing early in life. J Allergy Clin Immunol. (2010) 126:483–8.e1. doi: 10.1016/j.jaci.2010.06.028
41. Palmer LJ, Rye PJ, Gibson NA, Burton PR, Landau LI, Lesouëf PN. Airway responsiveness in early infancy predicts asthma, lung function, and respiratory symptoms by school age. Am J Respir Crit Care Med. (2001) 163:37–42. doi: 10.1164/ajrccm.163.1.2005013
42. Turner SW, Young S, Goldblatt J, Landau LI, Le Souëf PN. Childhood asthma and increased airway responsiveness: a relationship that begins in infancy. Am J Respir Crit Care Med. (2009) 179:98–104. doi: 10.1164/rccm.200805-804OC
43. Cox DW, Mullane D, Zhang GC, Turner SW, Hayden CM, Goldblatt J, et al. Longitudinal assessment of airway responsiveness from 1 month to 18 years in the PIAF birth cohort. Eur Respir J. (2015) 46:1654–61. doi: 10.1183/13993003.00397-2015
Keywords: wheeze, asthma, lung function, bronchial (airway) hyperresponsiveness, asthma risk factors
Citation: Sánchez-Solís M (2019) Early Lung Function and Future Asthma. Front. Pediatr. 7:253. doi: 10.3389/fped.2019.00253
Received: 09 January 2019; Accepted: 03 June 2019;
Published: 19 June 2019.
Edited by:
Jose Antonio Castro-Rodriguez, Pontifical Catholic University of Chile, ChileReviewed by:
Iulia Ioan, Hôpital d'Enfants, FranceIgnacio Tapia, Children's Hospital of Philadelphia, United States
Copyright © 2019 Sánchez-Solís. This is an open-access article distributed under the terms of the Creative Commons Attribution License (CC BY). The use, distribution or reproduction in other forums is permitted, provided the original author(s) and the copyright owner(s) are credited and that the original publication in this journal is cited, in accordance with accepted academic practice. No use, distribution or reproduction is permitted which does not comply with these terms.
*Correspondence: Manuel Sánchez-Solís, msolis@um.es