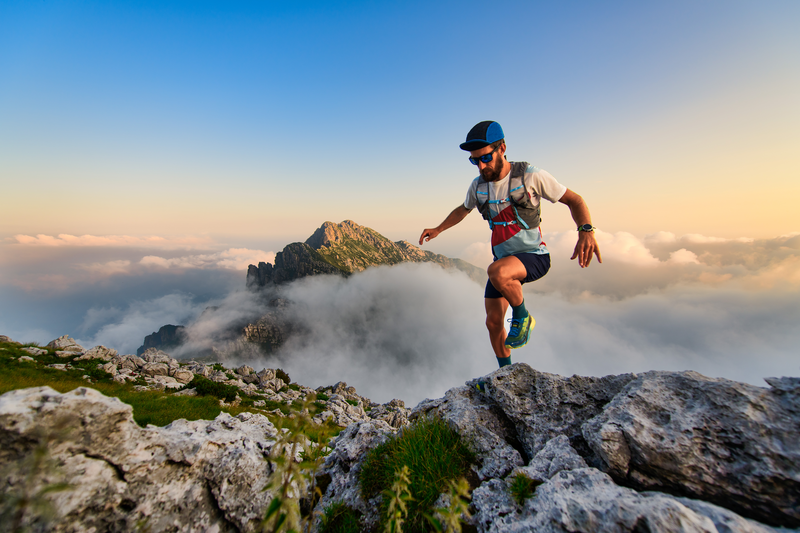
94% of researchers rate our articles as excellent or good
Learn more about the work of our research integrity team to safeguard the quality of each article we publish.
Find out more
MINI REVIEW article
Front. Pediatr. , 18 June 2019
Sec. Pediatric Immunology
Volume 7 - 2019 | https://doi.org/10.3389/fped.2019.00242
This article is part of the Research Topic Infection-Related Immune-Mediated Diseases and Microbiota View all 10 articles
Kawasaki disease (KD) has replaced acute rheumatic fever as the most common cause of acquired heart disease in children in the developed world and is increasingly being recognized from several developing countries. It is a systemic vasculitis with a predilection for coronary arteries. The diagnosis is based on a constellation of clinical findings that appear in a temporal sequence. Quite understandably, this can become a problem in situations wherein the clinical features are not typical. In such situations, it can be very difficult, if not impossible, to arrive at a diagnosis. Several biomarkers have been recognized in children with acute KD but none of these has reasonably high sensitivity and specificity in predicting the course of the illness. A line up of inflammatory, proteomic, gene expression and micro-RNA based biomarkers has been studied in association with KD. The commonly used inflammatory markers e.g. erythrocyte sedimentation rate (ESR), C-reactive protein (CRP), and total leucocyte counts (TLC) lack specificity for KD. Proteomic studies are based on the identification of specific proteins in serum, plasma and urine by gel electrophoresis. A host of genetic studies have identified genes associated with KD and some of these genes can predict the course and coronary outcomes in the affected individuals. Most of these tests are in the early stages of their development and some of these can predict the course, propensity to develop coronary artery sequelae, intravenous immunoglobulin (IVIg) resistance and the severity of the illness in a patient. Development of clinical criteria based on these tests will improve our diagnostic acumen and aid in early identification and prevention of cardiovascular complications.
Kawasaki disease (KD) is a common childhood vasculitis. The disease was first described in Japanese children in 1967 by a Japanese pediatrician, Dr. Tomisaku Kawasaki (1). The highest incidence is seen in Japan, Korea and Taiwan (2). However, the disease is now being reported world over including several developing countries like India (3–7).
The diagnosis of KD is essentially clinical (8). The clinical features mimic many self-resolving exanthematous febrile illnesses of childhood (e.g., measles, adenoviral infection, scarlet fever, dengue fever). These illnesses share some common clinical features with KD like fever, rash, mucocutaneous manifestations, lymphadenopathy, and elevated inflammatory parameters. The diagnosis of KD can be easily missed if the cascade of clinical findings goes unrecognized especially in cases of incomplete KD. Things can get even more complicated when KD occurs in association with an infection. The associated coronary artery abnormalities (CAAs) may go undetected and can have significant long-term implications if treatment is not initiated at the right time. Unlike other vasculitides and other rheumatological disorders, there are no pathognomonic laboratory tests for diagnosis of this condition. For the treating clinician, it is often difficult to confirm a diagnosis of KD on the bedside. It is, therefore, important to establish a set of laboratory markers that are sensitive, specific, reproducible and help the treating pediatrician in arriving at a diagnosis. As intravenous immunoglobulin (IVIg) is an expensive product, it is imperative that it be used only in situations where it is definitely indicated. However, in a setting of incomplete KD, the pediatrician often faces the dilemma of under-treatment with its attendant risks of CAAs vs. using IVIg in circumstances where it may not really be indicated.
Several biomarkers have been studied in association with KD and some of these have been shown to be predictive of resistance to IVIg while others may be indicative of an increased risk of development of CAAs. This manuscript overviews some of the important biomarkers that have been studied in KD and highlights their role in the diagnosis and assessment of disease severity.
These are the conventional biomarkers that mirror inflammatory activity and are not specific to KD. Erythrocyte sedimentation rate (ESR) is consistently elevated during the acute phase of KD but may be unreliable as a marker of disease activity after the administration of IVIG (9–11). There is neutrophilic leukocytosis during the acute phase of the disease and the degree of leukocytosis has been correlated with myocardial dysfunction (10, 12). Thrombocytosis, usually seen after the completion of the first week of illness, is a marker of ongoing inflammation. Persistent thrombocytosis has been linked to the development of CAAs but the association is tenuous (9, 18). C-reactive protein (CRP) is known to have a significant association with disease severity and the development of CAAs (9, 10, 12). Procalcitonin levels have been shown to be elevated during the acute phase and this rise is especially marked in children with resistance to IVIg (13, 16). Peripheral blood eosinophilia (PBE) (17) and low albumin has been associated with increased risk of IVIG resistance and coronary complications (14). Low mean platelet volume (MPV) (15), platelet distribution width (PDW) (19), and platelet-derived microparticles (PDMP) (20) have been shown to be markers of platelet activation and inflammation in acute stages of KD. However, their use as biomarkers for the disease requires replication of results across different populations.
Inflammatory markers are largely nonspecific as these are also elevated in many other inflammatory and infective conditions. In a clinical setting of KD, while these biomarkers can reflect ongoing inflammation, they are of limited use in arriving at a definitive diagnosis.
Myeloid dendritic cells (mDCs), which serve as a bridge between adaptive and innate immunity, have been shown to be decreased in acute KD. Takahashi et al. (21) have suggested that decreased levels are due to either recirculation into affected tissues or increased peripheral destruction in cases of acute KD. Furukawa et al. (22) studied CD14+ macrophages in KD patients and showed that they were higher in those with CAAs, thereby suggesting that absolute counts of CD14+ monocytes can be a marker of the severity of KD. These studies point toward a possible dysfunction in the innate immunity axis which could contribute to the inflammatory upregulation seen in acute stages of KD.
Studies have shown a decreased number of CD8 T cells during acute stages of KD (23). Immunohistochemistry studies in coronary arteries of KD patients at autopsy have shown that CD8 T cells preferentially sequester in the coronary arteries and are responsible for the inflammatory vasculitis (24, 26). Ehara et al. (27) showed that markers of early T cell activation [CD69(+)CD8T cells] increased in acute stages and can be used as a marker of disease progression and response to IVIg. Helper T cells (Th1 and Th2) have been shown to be upregulated during acute stages (22, 25). There is an apparent imbalance of T helper 17 cells (Th17) and regulatory T cells (Tregs) in acute KD. Th17 proportions have been shown to be upregulated while Treg proportions were found to be downregulated during the acute phase of KD (33).
Soluble markers of inflammation have been studied at great length in association with KD. Plasma levels of Th1 (IFN-Y, IL-12) and Th2 (IL-4, IL-13) cytokines have been shown to be elevated during acute stages of KD (34). Multiple pro-inflammatory and anti-inflammatory cytokines have been studied in acute stages of KD, but none of these has been standardized as a biomarker of KD (29). Zhou et al. (28) showed a close relation between levels of vascular endothelial growth factor (VEGF), IL-6, and development of CAAs. TGF-β signaling has been implicated in the development of coronary artery aneurysms (29). Th1-associated (CXCL10) and Th2-associated chemokines (CCL2) are elevated in acute stages of KD and have been shown to decrease with IVIG (35, 36). Tumor Necrosis Factor α (TNFα) has a role in the recruitment of inflammatory cells to coronary endothelium and has been shown to have a role in the development of CAAs. TNFα blocking agents have been extensively studied as a therapy for KD (37).
Data on immunological markers in KD are derived from studies on relatively small groups of patients. The significance of these biomarkers in predicting the disease course, response to therapy and complications is not clear and needs confirmation across a different population.
Several proteins have been studied in KD.
NT-proBNP has been used as a marker of myocardial damage in KD. It is a marker of cardiomyocyte stress imposed by pressure or volume overload and is increased in response to cardiac dilatation and neuro-humoral factors. According to Dahdah et al. (31), myocardial involvement in acute KD is universal from the histological and functional perspectives and hence the role of NT-proBNP as a potential biomarker has been extensively studied. The interpretation of serum NT-proBNP levels in children is difficult because these are age-dependent, being highest in infancy and decreasing thereafter. The sensitivity and specificity of cut-off values as a biomarker for KD are derived from receiver operating characteristics (ROC) analysis, the upper normal limit for age, and Z-scores for age. Shiraishi et al. (32) showed that the sensitivity and specificity of diagnosing acute KD were 97.8 and 47%, respectively, at a z score > 2. A meta-analysis on NT-proBNP in KD has substantiated its use as a diagnostic marker and cut-off values between 96 and 260 pg/ml have been shown to have sensitivity between 66 and 98% in identifying cases of KD (30). NT-proBNP levels are higher in patients with CAAs (values 515–1,300 pg/ml have a sensitivity and specificity of 73–95 and 61–85%, respectively) (38) and can predict IVIG resistance. A value between 629 and 1,300 pg/ml has a high sensitivity (70–79%) as well as specificity (58–77%) for diagnosis of KD (39, 40). From our center, we have reported a cut-off at 1,025 pg/mL for NT-proBNP levels which has a sensitivity of 88% and specificity of 96% (41) in the acute phase of KD.
Suppression of tumorigenicity-2 (sST2) is a member of the interleukin 1 (IL-1) receptor family and reflects cardiovascular stress and fibrosis. It is elevated in acute stages of KD and the levels correlate with impaired myocardial relaxation. However, the prognostic significance in acute KD is unclear (42).
Kim et al. (43) have described cardiac troponin I (cTnI) in relation with KD and showed a significant increase in the level of cTnI in the acute stage of KD. Periostin is a matricellular protein that mediates responses associated with cardiovascular injury. Reindel et al. (44) have shown that periostin gets upregulated in coronary arteries during the acute and chronic phases of KD when compared to other febrile controls. Gamma-glutamyl transferase (GGT) and alanine transferase (ALT) have also been studied as biomarkers of cardiac inflammation. However, these are rather nonspecific and are not a reliable marker for KD per se (42).
TSP-1 and TSP-2 are proteins involved in cardiovascular inflammation and maintaining the integrity and function of cardiac structures. These have been shown to be elevated in the acute phase of KD in comparison with other febrile controls and higher values are seen in those with IVIg resistance. With a cut-off value of 31.50 ng/mL, the sensitivity of TSP-2 has been shown to be 82.35% and specificity 64.81% in predicting IVIG resistance in acute KD (45).
Clusterin is a part of the high-density lipoproteins (HDL) and has a role in many physiologic processes including maintaining the integrity of coronary artery walls. Plasma clusterin levels have been studied in KD and values lower than 12 mg/L have been associated with the occurrence of CAAs in KD patients (46). Yu et al. (46, 55) have studied several proteins of fibrinogen cascade in KD. It was found that these were increased in patients with KD and may serve as a good biomarker of KD. The expression of nitric oxide synthases (iNOS) has been shown to correlate with the extent of coronary damage and progression of CAAs in patients with acute KD (47). Tenascin- C (TN-C) is a marker of tissue injury and inflammation and has a role in the maintenance of the extracellular matrix of cardiac tissue. Okuma et al. (48) have studied serum TN-C level in association with the risk of developing CAAs and resistance to IVIg therapy in the acute phase of KD.
Several protein biomarkers have been studied in relation to KD but most of these studies are based in small cohorts of patients at a single center. NT-proBNP is widely believed to be a useful marker for confirmation of the diagnosis of KD at the bedside. The levels of NT-proBNP in KD, however, may overlap those in other febrile illnesses with cardiac dysfunction. Other biomarkers are still in their early stages of discovery and will require validation in larger populations before the results can be used in clinical settings.
Kentsis et al. (49) studied urine protein biomarkers in relation with KD and showed an abundance of markers of tissue injury (filamin and talin), complement regulator (CSMD3), cytokine protease (meprin A), and immune pattern recognition receptor (muclin) in the urine of affected patients. However, these results need replication in larger studies before these can be used as noninvasive biomarkers in KD.
A genetic basis of KD has been strongly considered taking into account the geographical differences in the incidence and high risk of occurrence in family members. Incidence of KD in Japan, Korea and Taiwan is more than 10 times the incidence in Western countries (50–53). This difference is a pointer toward a possible genetic susceptibility or may reflect environmental or lifestyle differences amongst these populations. A higher incidence is also known amongst Japanese Americans settled in Hawaii and this is comparable with the incidence in Japan, further pointing toward a possible genetic association of the disease (75). Studies have shown that the risk of developing KD in siblings of a KD patient is 10–30-fold higher as compared to the general population (76). Two types of approaches have been utilized for studying the genetic basis of KD:
1. Candidate gene approach
2. Genome-wide approach
Candidate gene studies are the well-known approach to study genes associated with KD based on their function in the pathophysiology of the disease.
Early genetic studies on KD were focused on HLA alleles. HLA class 1 antigens have been studied in great detail in various populations (54, 77). Significant predominant association of HLA-Bw54 has been found in Japanese KD population while single nucleotide polymorphisms (SNP) located in the HLA-E gene was suggested to be associated with KD in the Han Chinese population (77). A positive association was found between HLA-Bw51 and KD in the White and Jewish population while HLA-Bw51 was reported to be negatively associated in the Korean population. In a recent GWAS, association with HLA class II antigens peaked at the intergenic region between HLA-DQB2 and HLA-DOB (78). SNPs in HLA class III region have also been described in association with KD (79). TNF∞ expression has been shown to be elevated in association with CAAs in KD in Korean, Taiwanese, Chinese and Caucasian populations, but these results could not be replicated in studies from Japan (80).
Burns et al. (78) found an SNP in the promoter region of the IL-4 gene to be asymmetrically transmitted in children with KD. MHC-class-I-chain-related gene A (MICA) alleles were reported as biomarkers for evaluation of coronary aneurysms in KD. Lower frequency of MICA allele 276 A4 was reported in KD patients with aneurysms (81).
GWLS were used to map the genetic loci of diseases by analyses of related individuals through the logarithm of odds (LOD) score. The first GWLS on KD was done by Onouchi et al. (82) who studied 79 families of children with KD and analyzed 75 sibling pairs. They identified 10 chromosomal loci with positive linkage, among which the 12q24 region showed the most significant evidence of linkage. GWLS studies identified Inositol 1,4,5-trisphosphate 3-kinase C (ITPKC) gene in association with risk of KD (83).
GWAS are based on the analysis of a genome-wide set of genetic variants typically SNP in affected individuals and healthy controls to see if a variant is repeatedly associated with a disease. GWAS studies on KD have identified several genes in association with the disease. The significant susceptibility genes identified include caspase-3 (CASP3) (69), calcium release-activated calcium modulator 1 (ORAI1) (59), ATP-binding cassette sub-family C member 4 gene(ABCC4) (84), Fc Fragment of IgG Receptor IIa (FCGR2A) (66), Transforming growth factor β pathway (TGFB2, TGFBR2, and SMAD3) (85), B lymphocyte kinase (BLK) (63), matrix metalloproteinase 11 (MMP-11) (74), and CD40 (86).
CD40L–CD40 interaction is known in relation to triggering and progression of acute coronary syndromes (87). Higher CD40 ligand expression on CD4 T-cells of KD patients as compared to febrile controls has been reported by a previous study. This over-expression decreased after IVIg administration (88). SNPs around CD40 showed association with KD susceptibility in Japanese (rs4813003, located 4.9 Kb downstream) and Taiwanese (rs1569723, located 4.8 Kb upstream) population. GWAS from Taiwan and Japan have also shown the association of SNPs of BLK and CD40 in the pathogenesis of KD (66).
BLK has a role in signal transduction of B cells (64). GWAS from Taiwan and Japan have shown the association of SNPs of BLK and CD40 in the pathogenesis of KD (68). Kawasaki Disease Genetics Consortium (2013) confirmed the association of BLK with KD susceptibility in Korean and European population (89).
FCGR2A is present on Fc region of IgG and encodes for cell surface receptor protein on phagocytic cells. GWAS done in five independent centers have confirmed the association of FCGR2A with KD susceptibility (66). Associations of SNPs rs2736340, rs4813003, rs3818298, and rs1801274 with KD were reported in a Han Chinese population (60, 66).
ITPKC is involved in Ca2+/NFAT pathway and acts as a potential candidate for immune activation and T-cell receptor signaling (68, 83). Association of functional SNP (rs28493229) in ITPKC with development of KD and CAA risk was first reported in Japanese and American children (83). However, no significant association was found between rs28493229 and KD risk in Taiwanese patients (90). Significant associations of C-allele of rs28493229 with KD and BCG scar reactivation in the acute stage were reported by Lin et al. (56).
ORAI1 is a Ca2+ channel protein involved in T cell regulation and inflammatory responses. Onouchi et al. (59) showed a significant non-synonymous association of SNP (rs3741596) in exon 2 of the ORAI1 gene with a high risk of KD in Japan. Another study reported a rare variant (rs141919534) in association with KD (91).
CASP3 gene is an execution-phase caspase involved in apoptosis of immune cells. Various SNPs including rs2720378, rs72689236, and rs113420705 have been reported in association with KD (69). Kuo et al. found that SNP rs28493229 (ITPKC) and rs113420705 (CASP3) showed an increased risk of IVIG resistance and development of CAAs in Japanese but not in Taiwanese population (92).
The TGF-β pathway is an essential component of inflammation, T-cell activation and tissue remodeling. Genetic variations in TGF-β2, TGF-βR2, and SMAD3 are associated with CAA formation and was confirmed in replication studies (85). SNPrs6550004 in TGF-βR2 and rs1495592 in SMAD5 showed significant associations with KD in the Korean population (93).
MicroRNAs (miRNAs) are 20–26 nucleotides long non-coding single-stranded RNA segments that modify post-transcriptional mRNA gene expression. Shimizu et al. (94) showed 6 miRNAs (miR-143, miR-199b-5p, miR-618, miR-223, miR-145, and miR-145) that were differentially expressed in acute KD. In another study, miRNA-200c and miRNA-371-5p have also been studied as diagnostic biomarkers of KD (54). miR-27b suppresses endothelial cell proliferation and has been studied as a therapeutic target in patients of acute KD (95).
• In a recent multicenter study by Wright et al. (96), the use of whole-blood gene expression patterns was explored and a 13-transcript blood gene expression signature has been developed to distinguish KD from other infectious and inflammatory febrile illnesses in the first week of illness.
• Whole genome sequencing in a family with KD has also shown genetic variations in the toll-like receptor-6 (TLR6) gene in their two affected children (97).
Summary: Most of the genetic studies have been carried out in small cohorts of patients with KD and the results are not reproducible across different populations and ethnicities. These studies require validations in larger and multinational cohorts with additional case-control sets for a better understanding of the genetic profiles.
The need for a robust set of biochemical biomarkers to validate the diagnosis of KD in the clinical setting has become the need of the hour. Clinical application of these biomarkers is limited. Inflammatory parameters can, at best, facilitate confirmation of a clinical diagnosis of KD but none of these is pathognomonic of KD. Further, these markers have very low specificity for the diagnosis of KD. The newer proteomic studies have identified some biomarkers in association with KD but these also need validation across different populations before these can be used for confirming a diagnosis of KD. Genome-wide studies, linkage studies and miRNA-based biomarkers are still in their early stage of development and fall short of being a diagnostic test for this enigmatic condition. These genetic markers are pointers toward a diagnostic panel for KD but clearly, these are early days and much more work needs to be done before a robust laboratory diagnostic test can be established.
HC, JN, RK: Literature review, interpretation of data and draft of the manuscript. VP: critical review and editing of the manuscript. DS: critical review of studies cited in the manuscript, editing of manuscript. AR: concept and design of the manuscript, critical review of studies cited in the manuscript, editing of manuscript. SS: concept and design of the manuscript, critical review, and editing of the manuscript.
The authors declare that the research was conducted in the absence of any commercial or financial relationships that could be construed as a potential conflict of interest.
1. Kawasaki T, Kosaki F, Okawa S, Shigematsu I, Yanagawa H. A new infantile acute febrile mucocutaneous lymph node syndrome (MLNS) prevailing in Japan. Pediatrics. (1974) 54:271–6.
2. Lin M-T, Wu M-H. The global epidemiology of Kawasaki disease: review and future perspectives. Glob Cardiol Sci Pract. (2017) 2017:e201720. doi: 10.21542/gcsp.2017.20
3. Singh S, Bansal A, Gupta A, Kumar RM, Mittal BR. Kawasaki disease: a decade of experience from North India. Int Heart J. (2005) 46:679–89. doi: 10.1536/ihj.46.679
4. Khubchandani RP, Khemani C. Kawasaki disease registries reap results experience in Mumbai. Indian J Pediatr. (2006) 73:545–6. doi: 10.1007/BF02759910
6. Narayanan SN, Krishnaveni null, Sabarinathan K. Kawasaki disease. Indian Pediatr. (1997) 34:139–43.
7. Suresh N, Varadarajan VV, Ranjith MS. Kawasaki disease in south India: a prospective, case-control study. Ann Trop Paediatr. (2007) 27:277–83. doi: 10.1179/146532807X245661
8. Singh S, Jindal AK, Pilania RK. Diagnosis of Kawasaki disease. Int J Rheum Dis. (2018) 21:36–44. doi: 10.1111/1756-185X.13224
9. Rahbari-Manesh AA, Salamati P, Ghaforian S, Zekavat M. Relationship between ESR, CRP, platelet count and coronary artery disease in Kawasaki disease. Iran J Pediatr. (2005) 15:139–44. Available online at: https://www.sid.ir/en/journal/ViewPaper.aspx?id=38243
10. Huang M-Y, Gupta-Malhotra M, Huang J-J, Syu F-K, Huang T-Y. Acute-phase reactants and a supplemental diagnostic aid for Kawasaki disease. Pediatr Cardiol. (2010) 31:1209–13. doi: 10.1007/s00246-010-9801-y
11. Tremoulet AH, Jain S, Chandrasekar D, Sun X, Sato Y, Burns JC. Evolution of laboratory values in patients with Kawasaki disease. Pediatr Infect Dis J. (2011) 30:1022–6. doi: 10.1097/INF.0b013e31822d4f56
12. Koyanagi H, Yanagawa H, Nakamura Y, Yashiro M. Leukocyte counts in patients with Kawasaki disease: from the results of nationwide surveys of Kawasaki disease in Japan. Acta Paediatr. (1997) 86:1328–32. doi: 10.1111/j.1651-2227.1997.tb14907.x
13. Dominguez SR, Martin B, Heizer H, Jone P-N, Tong S, Davidson J, et al. Procalcitonin (PCT) and Kawasaki disease: does PCT correlate with IVIG-resistant disease, admission to the intensive care unit, or development of coronary artery lesions? J Pediatr Infect Dis Soc. (2016) 5:297–302. doi: 10.1093/jpids/piv019
14. Kuo H-C, Liang C-D, Wang C-L, Yu H-R, Hwang K-P, Yang KD. Serum albumin level predicts initial intravenous immunoglobulin treatment failure in Kawasaki disease. Acta Paediatr. (2010) 99:1578–83. doi: 10.1111/j.1651-2227.2010.01875.x
15. Roy S, Majumdar SD, Chakrabartty S, Chakravarti S. Mean platelet volume as a marker of Kawasaki disease in children. Indian J Child Health. (2017) 318–21. Available online at: https://www.researchgate.net/profile/Soumya_Roy11/publication/318392865_Mean_platelet_volume_as_a_marker_of_Kawasaki_disease_in_children/links/5966fb9da6fdcc18ea60a96e/Mean-platelet-volume-as-a-marker-of-Kawasaki-disease-in-children.pdf
16. Lee NH, Choi HJ, Kim YH. Clinical usefulness of serum procalcitonin level in distinguishing between Kawasaki disease and other infections in febrile children. Korean J Pediatr. (2017) 60:112–7. doi: 10.3345/kjp.2017.60.4.112
17. Öner T, Yilmazer MM, Güven B, Devrim I, Cilengiroglu ÖV, Demirpençe S, et al. An observational study on peripheral blood eosinophilia in incomplete Kawasaki disease. Anatol J Cardiol. (2012) 12:160–4. doi: 10.5152/akd.2012.042
18. Xiu-Yu S, Jia-Yu H, Qiang H, Shu-Hui D. Platelet count and erythrocyte sedimentation rate are good predictors of Kawasaki disease: ROC analysis. J Clin Lab Anal. (2010) 24:385–8. doi: 10.1002/jcla.20414
19. Liu R, Gao F, Huo J, Yi Q. Study on the relationship between mean platelet volume and platelet distribution width with coronary artery lesion in children with Kawasaki disease. Platelets. (2012) 23:11–6. doi: 10.3109/09537104.2011.586073
20. Jin J, Wang J, Lu Y, Fan Z, Huang N, Ma L, et al. Platelet-derived microparticles: a new index of monitoring platelet activation and inflammation in Kawasaki disease. Indian J Pediatr. (2018) 86:250–55. doi: 10.1007/s12098-018-2765-2
21. Tomoyuki Takahashi SK. Circulating myeloid dendritic cells is decreased in the acute phase of kawasaki disease. J Clin Exp Cardiol. (2013) 4:272. doi: 10.4172/2155-9880.1000272
22. Furukawa S, Matsubara T, Yabuta K. Mononuclear cell subsets and coronary artery lesions in Kawasaki disease. Arch Dis Child. (1992) 67:706–8. doi: 10.1136/adc.67.6.706
23. Brogan PA, Shah V, Clarke LA, Dillon MJ, Klein N. T cell activation profiles in Kawasaki syndrome. Clin Exp Immunol. (2008) 151:267–74. doi: 10.1111/j.1365-2249.2007.03567.x
24. Rivas MN, Lee Y, Wakita D, Chiba N, Dagvadorj J, Shimada K, et al. CD8+ T cells contribute to the development of coronary arteritis in the Lactobacillus casei extract-induced murine model of Kawasaki disease. Arthritis Rheumatol. (2017) 69:410–21. doi: 10.1002/art.39939
25. Wang Y, Wang W, Gong F, Fu S, Zhang Q, Hu J, et al. Evaluation of intravenous immunoglobulin resistance and coronary artery lesions in relation to Th1/Th2 cytokine profiles in patients with Kawasaki disease. Arthritis Rheum. (2013) 65:805–14. doi: 10.1002/art.37815
26. Brown TJ, Crawford SE, Cornwall ML, Garcia F, Shulman ST, Rowley AH. CD8 T lymphocytes and macrophages infiltrate coronary artery aneurysms in acute Kawasaki disease. J Infect Dis. (2001) 184:940–3. doi: 10.1086/323155
27. Ehara H, Kiyohara K, Izumisawa Y, Ito T. Early activation does not translate into effector differentiation of peripheral CD8T cells during the acute phase of Kawasaki disease. Cell Immunol. (2010) 265:57–64. doi: 10.1016/j.cellimm.2010.07.003
28. Zhou Y, Wang S, Zhao J, Fang P. Correlations of complication with coronary arterial lesion with VEGF, PLT, D-dimer and inflammatory factor in child patients with Kawasaki disease. Eur Rev Med Pharmacol Sci. (2018) 22:5121–6. doi: 10.26355/eurrev_201808_15706
29. Sohn MH, Noh SY, Chang W, Shin KM, Kim DS. Circulating interleukin 17 is increased in the acute stage of Kawasaki disease. Scand J Rheumatol. (2003) 32:364–6. doi: 10.1080/03009740410005034
30. Lin K-H, Chang S-S, Yu C-W, Lin S-C, Liu S-C, Chao H, et al. Usefulness of natriuretic peptide for the diagnosis of Kawasaki disease: a systematic review and meta-analysis. BMJ Open. (2015) 5:6703. doi: 10.1136/bmjopen-2014-006703
31. Dionne A, Dahdah N. A decade of NT-proBNP in acute Kawasaki disease, from physiological response to clinical relevance. Children. (2018) 5:141. doi: 10.3390/children5100141
32. Shiraishi M, Fuse S, Mori T, Doyama A, Honjyo S, Hoshino Y, et al. N-terminal pro-brain natriuretic Peptide as a useful diagnostic marker of acute Kawasaki disease in children. Circ J. (2013) 77:2097–101. doi: 10.1253/circj.CJ-12-1281
33. Jia S, Li C, Wang G, Yang J, Zu Y. The T helper type 17/regulatory T cell imbalance in patients with acute Kawasaki disease. Clin Exp Immunol. (2010) 162:131–7. doi: 10.1111/j.1365-2249.2010.04236.x
34. Kimura J, Takada H, Nomura A, Ohno T, Mizuno Y, Saito M, et al. Th1 and Th2 cytokine production is suppressed at the level of transcriptional regulation in Kawasaki disease. Clin Exp Immunol. (2004) 137:444–9. doi: 10.1111/j.1365-2249.2004.02506.x
35. Ko T-M, Kuo H-C, Chang J-S, Chen S-P, Liu Y-M, Chen H-W, et al. CXCL10/IP-10 is a biomarker and mediator for Kawasaki disease. Circ Res. (2015) 116:876–83. doi: 10.1161/CIRCRESAHA.116.305834
36. Shikishima Y, Saeki T, Matsuura N. Chemokines in Kawasaki disease: measurement of CCL2, CCL22 and CXCL10. Asian Pac J Allergy Immunol. (2003) 21:139–43.
37. Hui-Yuen JS, Duong TT, Yeung RSM. TNF-α is necessary for induction of coronary artery inflammation and aneurysm formation in an animal model of Kawasaki disease. J Immunol. (2006) 176:6294–301. doi: 10.4049/jimmunol.176.10.6294
38. Kaneko K, Yoshimura K, Tsuji S. Brain natriuretic peptide as a novel diagnostic biomarker in Kawasaki disease. J Compr Pediatr. (2014) 5:19505. doi: 10.17795/compreped-19505
39. Kim SY, Han MY, Cha S-H, Jeon YB. N-terminal pro-brain natriuretic peptide (NT proBNP) as a predictive indicator of initial intravenous immunoglobulin treatment failure in children with Kawasaki disease: a retrospective study. Pediatr Cardiol. (2013) 34:1837–43. doi: 10.1007/s00246-013-0724-2
40. Kim MK, Song MS, Kim GB. Factors predicting resistance to intravenous immunoglobulin treatment and coronary artery lesion in patients with Kawasaki disease: analysis of the Korean nationwide multicenter survey from 2012 to 2014. Korean Circ J. (2018) 48:71–9. doi: 10.4070/kcj.2017.0136
41. Reddy M, Singh S, Rawat A, Sharma A, Suri D, Rohit MK. Pro-brain natriuretic peptide (ProBNP) levels in North Indian children with Kawasaki disease. Rheumatol Int. (2016) 36:551–9. doi: 10.1007/s00296-016-3430-6
42. Sato YZ, Molkara DP, Daniels LB, Tremoulet AH, Shimizu C, Kanegaye JT, et al. Cardiovascular biomarkers in acute Kawasaki disease. Int J Cardiol. (2013) 164:58–63. doi: 10.1016/j.ijcard.2011.06.065
43. Kim M, Kim K. Elevation of cardiac troponin I in the acute stage of Kawasaki disease. Pediatr Cardiol. (1999) 20:184–8. doi: 10.1007/s002469900437
44. Reindel R, Kim K-YA, Baker SC, Shulman ST, Perlman EJ, Lingen MW, et al. Periostin is upregulated in coronary arteriopathy in Kawasaki disease and is a potential diagnostic biomarker. Pediatr Infect Dis J. (2014) 33:659–61. doi: 10.1097/INF.0000000000000233
45. Yang S, Song R, Li X, Zhang T, Fu J, Cui X. Thrombospondin-2 predicts response to treatment with intravenous immunoglobulin in children with Kawasaki disease. BMJ Paediatr Open. (2018) 2:e000190. doi: 10.1136/bmjpo-2017-000190
46. Yu H-R, Kuo H-C, Huang E-Y, Liang C-D, Hwang K-P, Lin I-C, et al. Plasma clusterin levels in predicting the occurrence of coronary artery lesions in patients with Kawasaki disease. Pediatr Cardiol. (2010) 31:1151–6. doi: 10.1007/s00246-010-9769-7
47. Yu X, Hirono K-I, Ichida F, Uese K-I, Rui C, Watanabe S, et al. Enhanced iNOS expression in leukocytes and circulating endothelial cells is associated with the progression of coronary artery lesions in acute Kawasaki disease. Pediatr Res. (2004) 55:688–94. doi: 10.1203/01.PDR.0000113464.93042.A4
48. Yokouchi Y, Oharaseki T, Enomoto Y, Sato W, Imanaka-Yoshida K, Takahashi K. Expression of tenascin C in cardiovascular lesions of Kawasaki disease. Cardiovasc Pathol. (2018) 38:25–30. doi: 10.1016/j.carpath.2018.10.005
49. Kentsis A, Shulman A, Ahmed S, Brennan E, Monuteaux MC, Lee Y-H, et al. Urine proteomics for discovery of improved diagnostic markers of Kawasaki disease. EMBO Mol Med. (2013) 5:210–20. doi: 10.1002/emmm.201201494
50. Nakamura Y, Yashiro M, Uehara R, Sadakane A, Tsuboi S, Aoyama Y, et al. Epidemiologic features of Kawasaki disease in Japan: results of the 2009–2010 nationwide survey. J Epidemiol. (2012) 22:216–21. doi: 10.2188/jea.JE20110126
51. Park YW, Han JW, Hong YM, Ma JS, Cha SH, Kwon TC, et al. Epidemiological features of Kawasaki disease in Korea, 2006-2008. Pediatr Int. (2011) 53:36–9. doi: 10.1111/j.1442-200X.2010.03178.x
52. Holman RC, Belay ED, Christensen KY, Folkema AM, Steiner CA, Schonberger LB. Hospitalizations for Kawasaki syndrome among children in the United States, 1997–2007. Pediatr Infect Dis J. (2010) 29:483–8. doi: 10.1097/INF.0b013e3181cf8705
53. Harnden A, Mayon-White R, Perera R, Yeates D, Goldacre M, Burgner D. Kawasaki disease in England: ethnicity, deprivation, and respiratory pathogens. Pediatr Infect Dis J. (2009) 28:21–4. doi: 10.1097/INF.0b013e3181812ca4
54. Fildes N, Burns JC, Newburger JW, Klitz W, Begovich AB. The HLA class II region and susceptibility to Kawasaki disease. Tissue Antigens. (1992) 39:99–101. doi: 10.1111/j.1399-0039.1992.tb01915.x
55. Yu H-R, Kuo H-C, Sheen J-M, Wang L, Lin I-C, Wang C-L, et al. A unique plasma proteomic profiling with imbalanced fibrinogen cascade in patients with Kawasaki disease. Pediatr Allergy Immunol. (2009) 20:699–707. doi: 10.1111/j.1399-3038.2008.00844.x
56. Lin M-T, Wang J-K, Yeh J-I, Sun L-C, Chen P-L, Wu J-F, et al. Clinical implication of the C allele of the ITPKC gene SNP rs28493229 in Kawasaki disease: association with disease susceptibility and BCG scar reactivation. Pediatr Infect Dis J. (2011) 30:148–52. doi: 10.1097/INF.0b013e3181f43a4e
57. Peng Q, Chen C, Zhang Y, He H, Wu Q, Liao J, et al. Single-nucleotide polymorphism rs2290692 in the 3'UTR of ITPKC associated with susceptibility to Kawasaki disease in a Han Chinese population. Pediatr Cardiol. (2012) 33:1046–53. doi: 10.1007/s00246-012-0223-x
58. Kuo H-C, Yang KD, Juo S-HH, Liang C-D, Chen W-C, Wang Y-S, et al. ITPKC single nucleotide polymorphism associated with the Kawasaki disease in a Taiwanese population. PLoS ONE. (2011) 6:e17370. doi: 10.1371/journal.pone.0017370
59. Onouchi Y, Fukazawa R, Yamamura K, Suzuki H, Kakimoto N, Suenaga T, et al. Variations in ORAI1 gene associated with Kawasaki disease. PLoS ONE. (2016) 11:e0145486. doi: 10.1371/journal.pone.0145486
60. Lou J, Zhong R, Shen N, Lu X, Ke J, Duan J, et al. Systematic confirmation study of GWAS-identified genetic variants for Kawasaki disease in a Chinese population. Sci Rep. (2015) 5:8194. doi: 10.1038/srep08194
61. Cheng S-C, Cheng Y-Y, Wu J-L. [Association between gene polymorphism of CD40 gene and coronary artery lesion in Kawasaki disease]. Chin J Contemp Pediatr. (2014) 16:1025–8.
62. Onouchi Y, Ozaki K, Burns JC, Shimizu C, Terai M, Hamada H, et al. A genome-wide association study identifies three new risk loci for Kawasaki disease. Nat Genet. (2012) 44:517–21. doi: 10.1038/ng.2220
63. Chang C-J, Kuo H-C, Chang J-S, Lee J-K, Tsai F-J, Khor CC, et al. Replication and meta-analysis of GWAS identified susceptibility loci in Kawasaki disease confirm the importance of B lymphoid tyrosine kinase (BLK) in disease susceptibility. PLoS ONE. (2013) 8:e72037. doi: 10.1371/journal.pone.0072037
64. Lee Y-C, Kuo H-C, Chang J-S, Chang L-Y, Huang L-M, Chen M-R, et al. Two new susceptibility loci for Kawasaki disease identified through genome-wide association analysis. Nat Genet. (2012) 44:522–5. doi: 10.1038/ng.2227
65. Duan J, Lou J, Zhang Q, Ke J, Qi Y, Shen N, et al. A genetic variant rs1801274 in FCGR2A as a potential risk marker for Kawasaki disease: a case-control study and meta-analysis. PLoS ONE. (2014) 9:e103329. doi: 10.1371/journal.pone.0103329
66. Khor CC, Davila S, Breunis WB, Lee Y-C, Shimizu C, Wright VJ, et al. Genome-wide association study identifies FCGR2A as a susceptibility locus for Kawasaki disease. Nat Genet. (2011) 43:1241–6. doi: 10.1038/ng.981
67. Taniuchi S, Masuda M, Teraguchi M, Ikemoto Y, Komiyama Y, Takahashi H, et al. Polymorphism of Fc gamma RIIa may affect the efficacy of gamma-globulin therapy in Kawasaki disease. J Clin Immunol. (2005) 25:309–13. doi: 10.1007/s10875-005-4697-7
68. Wang W, Lou J, Zhong R, Qi Y, Shen N, Lu X, et al. The roles of Ca2+/NFAT signaling genes in Kawasaki disease: single- and multiple-risk genetic variants. Sci Rep. (2014) 4:5208. doi: 10.1038/srep05208
69. Onouchi Y, Ozaki K, Buns JC, Shimizu C, Hamada H, Honda T, et al. Common variants in CASP3 confer susceptibility to Kawasaki disease. Hum Mol Genet. (2010) 19:2898–906. doi: 10.1093/hmg/ddq176
70. Kuo H-C, Yu H-R, Juo S-HH, Yang KD, Wang Y-S, Liang C-D, et al. CASP3 gene single-nucleotide polymorphism (rs72689236) and Kawasaki disease in Taiwanese children. J Hum Genet. (2011) 56:161–5. doi: 10.1038/jhg.2010.154
71. Choi YM, Shim KS, Yoon KL, Han MY, Cha SH, Kim SK, et al. Transforming growth factor beta receptor II polymorphisms are associated with Kawasaki disease. Korean J Pediatr. (2012) 55:18–23. doi: 10.3345/kjp.2012.55.1.18
72. Kuo H-C, Onouchi Y, Hsu Y-W, Chen W-C, Huang J-D, Huang Y-H, et al. Polymorphisms of transforming growth factor-β signaling pathway and Kawasaki disease in the Taiwanese population. J Hum Genet. (2011) 56:840–5. doi: 10.1038/jhg.2011.113
73. Peng Q, Deng Y, Yang X, Leng X, Yang Y, Liu H. Genetic variants of ADAM17 are implicated in the pathological process of Kawasaki disease and secondary coronary artery lesions via the TGF-β/SMAD3 signaling pathway. Eur J Pediatr. (2016) 175:705–13. doi: 10.1007/s00431-016-2696-8
74. Ban JY, Kim SK, Kang SW, Yoon KL, Chung J-H. Association between polymorphisms of matrix metalloproteinase 11 (MMP-11) and Kawasaki disease in the Korean population. Life Sci. (2010) 86:756–9. doi: 10.1016/j.lfs.2010.03.012
75. Holman RC, Christensen KY, Belay ED, Steiner CA, Effler PV, Miyamura J, et al. Racial/ethnic differences in the incidence of Kawasaki syndrome among children in Hawai‘i. Hawaii Med J. (2010) 69:194–7.
76. Fujita Y, Nakamura Y, Sakata K, Hara N, Kobayashi M, Nagai M, et al. Kawasaki disease in families. Pediatrics. (1989) 84:666–9.
77. Kato S, Kimura M, Tsuji K, Kusakawa S, Asai T, Juji T, et al. HLA antigens in Kawasaki disease. Pediatrics. (1978) 61:252–5.
78. Burns JC, Shimizu C, Shike H, Newburger JW, Sundel RP, Baker AL, et al. Family-based association analysis implicates IL-4 in susceptibility to Kawasaki disease. Genes Immun. (2005) 6:438–44. doi: 10.1038/sj.gene.6364225
79. Maggioli E, Boiocchi C, Zorzetto M, Mannarino S, Bossi G, Cuccia M. HLA class III genes involvement in Kawasaki disease: a case–control study in Caucasian population. Int J Immunogenet. (2014) 41:44–53. doi: 10.1111/iji.12077
80. Ichida KHF. Utility of TNF-α as a biomarker and the possibility of anti-TNF-α therapy for Kawasaki disease. Pediatr Ther. (2014) 5:1–6. doi: 10.4172/2161-0665.1000257
81. Huang F-Y, Lee Y-J, Chen M-R, Hsu C-H, Lin S-P, Sung T-C. Polymorphism of transmembrane region of MICA gene and Kawasaki disease. Exp Clin Immunogenet. (2000) 17:130–7. doi: 10.1159/000019132
82. Onouchi Y, Tamari M, Takahashi A, Tsunoda T, Yashiro M, Nakamura Y, et al. A genomewide linkage analysis of Kawasaki disease: evidence for linkage to chromosome 12. J Hum Genet. (2007) 52:179–90. doi: 10.1007/s10038-006-0092-3
83. Onouchi Y, Gunji T, Burns JC, Shimizu C, Newburger JW, Yashiro M, et al. ITPKC functional polymorphism associated with Kawasaki disease susceptibility and formation of coronary artery aneurysms. Nat Genet. (2008) 40:35–42. doi: 10.1038/ng.2007.59
84. Che D, Pi L, Fang Z, Xu Y, Cai M, Fu L, et al. abcc4 variants modify susceptibility to Kawasaki Disease in a Southern Chinese population. Dis Markers. (2018) 2018:1–7. doi: 10.1155/2018/8638096
85. Shimizu C, Oharaseki T, Takahashi K, Kottek A, Franco A, Burns JC. The role of TGF-β and myofibroblasts in the arteritis of Kawasaki disease. Hum Pathol. (2013) 44:189–98. doi: 10.1016/j.humpath.2012.05.004
86. Kuo H-C, Chao M-C, Hsu Y-W, Lin Y-C, Huang Y-H, Yu H-R, et al. CD40 gene polymorphisms associated with susceptibility and coronary artery lesions of Kawasaki disease in the Taiwanese population. Sci World J. (2012) 2012:1–5. doi: 10.1100/2012/520865
87. Aukrust P, Müller F, Ueland T, Berget T, Aaser E, Brunsvig A, et al. Enhanced levels of soluble and membrane-bound CD40 ligand in patients with unstable angina. Possible reflection of T lymphocyte and platelet involvement in the pathogenesis of acute coronary syndromes. Circulation. (1999) 100:614–20. doi: 10.1161/01.CIR.100.6.614
88. Wang C-L, Wu Y-T, Liu C-A, Lin M-W, Lee C-J, Huang L-T, et al. Expression of CD40 ligand on CD4+ T-cells and platelets correlated to the coronary artery lesion and disease progress in Kawasaki disease. Pediatrics. (2003) 111:E140-147. doi: 10.1542/peds.111.2.e140
89. Lee J-K, Hong YM, Jang GY, Yun SW, Yu JJ, Yoon KL, et al. Consortium-based genetic studies of Kawasaki disease in Korea: Korean Kawasaki disease genetics consortium. Korean Circ J. (2015) 45:443. doi: 10.4070/kcj.2015.45.6.443
90. Chi H, Huang F-Y, Chen M-R, Chiu N-C, Lee H-C, Lin S-P, et al. ITPKC gene SNP rs28493229 and Kawasaki disease in Taiwanese children. Hum Mol Genet. (2010) 19:1147–51. doi: 10.1093/hmg/ddp586
91. Kuo HC, Lin YJ, Juo SHH, Hsu YW, Chen WC, Yang KD, et al. Lack of association between ORAI1/CRACM1 Gene Polymorphisms and Kawasaki disease in the Taiwanese children. J Clin Immunol. (2011) 31:650–5. doi: 10.1007/s10875-011-9524-8
92. Zhang W. Gene-Gene Associations with the susceptibility of Kawasaki disease and coronary artery lesions. PLoS ONE. (2015) 10:e0143056. doi: 10.1371/journal.pone.0143056
93. Li Z, Han D, Jiang J, Chen J, Tian L, Yang Z. Association of PECAM-1 gene polymorphisms with Kawasaki disease in Chinese children. Dis Markers. (2017) 2017:1–6. doi: 10.1155/2017/2960502
94. Yun KW, Lee JY, Yun SW, Lim IS, Choi ES. Elevated serum level of microRNA (miRNA)-200c and miRNA-371-5p in children with Kawasaki disease. Pediatr Cardiol. (2014) 35:745–52. doi: 10.1007/s00246-013-0846-6
95. Rong X, Ge D, Shen D, Chen X, Wang X, Zhang L, et al. miR-27b suppresses endothelial cell proliferation and migration by targeting Smad7 in Kawasaki disease. Cell Physiol Biochem Int J Exp Cell Physiol Biochem Pharmacol. (2018) 48:1804–14. doi: 10.1159/000492354
96. Wright VJ, Herberg JA, Kaforou M, Shimizu C, Eleftherohorinou H, Shailes H, et al. Diagnosis of Kawasaki disease using a minimal whole-blood gene expression signature. JAMA Pediatr. (2018) 172:e182293–e182293. doi: 10.1001/jamapediatrics.2018.2293
Keywords: immunology, vasculitis, Kawasaki, biomarkers, childhood vasculitis
Citation: Chaudhary H, Nameirakpam J, Kumrah R, Pandiarajan V, Suri D, Rawat A and Singh S (2019) Biomarkers for Kawasaki Disease: Clinical Utility and the Challenges Ahead. Front. Pediatr. 7:242. doi: 10.3389/fped.2019.00242
Received: 16 January 2019; Accepted: 28 May 2019;
Published: 18 June 2019.
Edited by:
Kyung-Yil Lee, The Catholic University of Korea, South KoreaReviewed by:
Diego F. Coutinho, New York University, United StatesCopyright © 2019 Chaudhary, Nameirakpam, Kumrah, Pandiarajan, Suri, Rawat and Singh. This is an open-access article distributed under the terms of the Creative Commons Attribution License (CC BY). The use, distribution or reproduction in other forums is permitted, provided the original author(s) and the copyright owner(s) are credited and that the original publication in this journal is cited, in accordance with accepted academic practice. No use, distribution or reproduction is permitted which does not comply with these terms.
*Correspondence: Surjit Singh, c3Vyaml0c2luZ2hwZ2lAcmVkaWZmbWFpbC5jb20=
Disclaimer: All claims expressed in this article are solely those of the authors and do not necessarily represent those of their affiliated organizations, or those of the publisher, the editors and the reviewers. Any product that may be evaluated in this article or claim that may be made by its manufacturer is not guaranteed or endorsed by the publisher.
Research integrity at Frontiers
Learn more about the work of our research integrity team to safeguard the quality of each article we publish.