- 1Associazione Italiana delle Banche del Latte Umano Donato, Milan, Italy
- 2Neonatology Nutrition, Lactarium Bordeaux-Marmande, CIC Pédiatrique 1401 Children's Hospital, Bordeaux, France
- 3Lactarium Auvergne Rhone Alpes, Hospices Civils de Lyon, Lyon, France
- 4Fundació Banc Sang i Teixits de les Illes Balears, Palma de Mallorca, Spain
- 5Institute of Sciences of Food Production, National Research Council, Turin, Italy
- 6CARAG AG, Baar, Switzerland
- 7Banco Regional de Leche Materna, Hospital Universitario 12 de Octubre, Madrid, Spain
- 8Laboratory of Molecular Virology, Department of Clinical and Biological Sciences, University of Turin, Turin, Italy
- 9Department of Neonatology, Faculty of Health Science, Medical University of Warsaw, Warsaw, Poland
- 10Division of Neonatology, Department of Pediatrics, Istanbul Medeniyet University, Istanbul, Turkey
- 11Greater Glasgow and Cycle Donor Milk Bank, Royal Hospital for Sick Children, Glasgow, United Kingdom
- 12Neonatal Unit of Turin University, City of Health and Science, Turin, Italy
- 13PhAN, Institut National de la Recherche Agronomique, Université de Nantes, CRNH-Ouest, Nantes, France
- 14Abteilung Neonatologie Klinik und Poliklinik für Kinder und Jugendliche, Leipzig, Germany
- 15Breast Milk Bank, Oslo University Hospital, Oslo, Norway
- 16The Milk Bank, Imperial College Healthcare NHS Trust, London, United Kingdom
- 17CarMeN Unit, INSERM U1397, Claude Bernard University Lyon 1, Lyon, France
Background: A mother's own milk (MOM) is the gold standard for the feeding and nutrition of preterm and full term infants. When MOM is not available or there is not enough, donor human milk (DHM) should be used. Milk delivered to Human Milk Banks (HMBs) should be pasteurized to inactivate viral and bacterial agents. Currently, a pasteurization process at 62.5°C for 30 min (Holder pasteurization, HoP) is recommended in all international HMBs guidelines.
State of the art: It is known that HoP affects some of the nutritional and biological components of human milk. Studies have demonstrated that temperature cycle in HoP is not always controlled or calibrated. A better check of these parameters in the pasteurizers on the market today may contribute to an improvement of the quality of HM, still maintaining some of the negative effects of the heat treatment of human milk. So, food industry, and dairy industry in particular, are evaluating innovative methodologies alternative to HoP to better preserve the nutritional and biological properties of fresh human milk, while assuring at least the same microbiological safety of HoP. The most studied processing techniques include High-Temperature-Short-Time (HTST) pasteurization, High Pressure Processing (HPP), and Ultraviolet-C (UV-C) irradiation. HTST is a thermal process in which milk is forced between plates or pipes that are heated on the outside by hot water at a temperature of 72°C for 5–15 s. HPP is a non-thermal processing method that can be applied to solid and liquid foods. This technology inactivates pathogenic microorganisms by applying a high hydrostatic pressure (usually 300–800 MPa) during short-term treatments (<5–10 min). UV irradiation utilizes short-wavelength ultraviolet radiation in the UV-C region (200–280 nm), which is harmful to microorganisms. It is effective in destroying the nucleic acids in these organisms, so that their DNA is disrupted by UV radiation.
Aim: The aim of this paper is to present the EMBA recommendations on processing of HM, based on the most recent results obtained with these new technologies.
Conclusions: Although research on the most promising technologies that will represent an alternative to HoP (HTST, HPP, UV-C) in the future is progressing, it is now important to recognize that the consistency and quality assurance of the pasteurizers on the market today represent a fundamental component that was previously lacking in the Holder approach.
Background
The increasing number of infants who are born extremely preterm and survive at birth and beyond, with a gestational age as low as 22 weeks, represents a new challenge for neonatal nutrition. In the last few decades, human milk (HM) has been identified as the normative standard for premature infant feeding and nutrition by health organizations and scientific societies (1–3). HM confers to these infants protection against necrotizing enterocolitis, sepsis and other infections, and severe retinopathy, decreases the risk of death, and improves their long-term neurocognitive development and cardiovascular health. In addition, the benefits of breast-feeding to promote psychological health and mother-infant bonding are well-known. A mother's own milk (MOM) is the first choice for premature infant feeding. When there is not sufficient MOM (a common occurrence in Neonatal Intensive care Units), donor human milk (DHM) obtained from well-established human milk banks (HMBs) is the best alternative. The WHO/UNICEF Joint Statement clearly indicates: “HMBs should be made available in appropriate situations” (4).
DHM delivered to HMBs should be pasteurized to inactivate viral and bacteriological agents (5). The ideal pasteurization process should consist of a rapid heating phase, followed by a phase in which the temperature is maintained constant, and a final rapid cooling phase. Currently, a pasteurization process performed at a temperature of 62.5°C for 30 min, which is known as the Holder pasteurization (HoP) method, is recommended in all international guidelines for the establishment and management of HMBs (5, 6). Pasteurized HM is known to retain many beneficial and protective components of fresh HM (5). However, it also affects some of the nutritional and biological properties of HM and eliminates the beneficial microbiota of fresh HM, thus resulting in the reduction of some bacteriostatic mechanisms that render milk more susceptible to postheating bacterial contamination, and decreases in its nutritional value (5).
Due to the present limitations of HoP in processing of HM, there is the need to evaluate alternative processing methods able to preserve better the bioactivity of a higher number of HM components in order to improve the nutritional and immunological quality of DHM.
New technologies are under study and the purpose of this manuscript is to evaluate the results obtained from the most promising ones.
State of the Art and Future Trends
HM is a functional and dynamic biologic system: it provides nutrients, bioactive components and immune factors, and it promotes an adequate and healthy growth of newborn infants. Milk delivered to HMBs should be pasteurized to inactivate viral and bacterial agents, as well as a mother's own milk in specific clinical situations.
Currently, HoP is the most frequently studied and recommended method for the heat treatment of donor HM (5–8). A recent review has shown a significant variability in the data reported in scientific literature concerning the effects of HoP on the biological components of HM (9). Possible explanations for this variability could be the heterogeneity of the test protocols applied in the studies, the fact that HoP is often simulated in laboratories on small aliquots of milk rather than being performed with commercial instruments on the larger volumes of milk utilized inside the HMBs, and, last but not least, modern pasteurizers require significantly less time for heating and cooling than older ones, thus changing the kinetics of the thermal response for heat-sensitive compounds (9–12).
The loss of some biologically active components as a result of HoP, including immunological components, is the main limit to the spread of donor HM utilization in the feeding of preterm infants (10, 11).
The optimization of the biological and nutritional quality of DHM is considered, by the European Association of Human Milk Banks (EMBA), as a scientific and social priority. In order to investigate this aspect, the EMBA Board of Directors set up a Working Group (WG), that is, a dynamic network of scientists who perform research in the field of HMBs and HM treatment, and who operate in different European countries. This WG is aimed to evaluate old and new methodologies in order to determine their effects on the final quality of DHM delivered from HMBs. The objective is to obtain optimum levels of quality and safety of DHM from milk banks in Europe and to decrease the variability of HM, at least as far as the aspects related to the effects of heat treatment are concerned. Quality has been discerned as a powerful tool for the improvement of the well-being of premature infants. A better management of DHM in HMBs will improve the services for donor women (those who donate milk) and for the recipients (the newborn infants who receive it).
The main technologies taken into considerations by this WG are the following: low-temperature long-time pasteurization (LTLT), which has been evaluated by a French group in Lyon (RB and JCP); high-temperature short-time pasteurization (HTST), evaluated by two Italian groups located in Turin and Milan (EB, LC, DL, and GEM); high pressure processing (HPP), evaluated by a French group located in Bordeaux (CB and GD) and a Polish group located in Warsaw (AW); and ultraviolet (UV) irradiation, performed by the Spanish group located in Palma de Mallorca (JC and AG).
Low-Temperature Long Time Pasteurization
At present, the most common practice utilized for the treatment of DHM is a low-temperature (62.5°C) long-time (30 min) pasteurization (LTLT), which is known as Holder pasteurization (HoP). HoP is recommended in all the international guidelines. Milk pasteurization with HoP is known to retain many of the beneficial and protective effects of HM, such as a reduction in NEC and sepsis, protection against bronchopulmonary dysplasia, and a lower rate of long-term complications, such as cardiovascular diseases and neurodevelopmental disabilities (3).
However, some significant concerns have arisen, related to the possible alterations of the nutritional and biological properties of DHM, as a result of the heat treatment. HoP produces a loss in the quantity and/or activity of some biologically functional milk components to varying degrees (9). Other nutritional and biological components, such as oligosaccharides, lactose, glucose, LCPUFAs, gangliosides, vitamins A, D, E, and B12, folic acid, some cytokines, and some growth factors are instead preserved (9).
Different devices have been produced to perform LTLT pasteurization. The most common heat source for pasteurization is hot water, but moving hot air has also been used in some other devices. In 2017, Buffin et al. showed that air pasteurizers have a very different pasteurization pattern from water pasteurizers (12). When the temperature recorded in the different bottles inside at pasteurizer's bath was measured, it was not homogenous, with a difference of 21.7 min between the first probe and the last probe reaching 62.5°C. Moreover, the plateau duration was on average 10 min longer in air pasteurizers than in water pasteurizers. Therefore, the exposure to temperature seems to be more prolonged in the former devices (12). In fact, air is a less effective thermal conductor than water. Its propulsion is uneven and leads to temperature inhomogeneity in pasteurizer. This phenomenon leads to the bottles undergoing a different treatment from each other, and it is difficult to provide adjustments to improve the process.
Water is the most homogeneous environment heat conducting source, and it is therefore the most widely used medium for HoP. Different devices exist on the market today, but not all of them are provided with a temperature control system. The duration of the heat treatment and the maximum temperature of milk exposure have been shown to be essential for the preservation of human milk. Evans et al. already showed, back in 1978, that the alteration of the immunological components of human milk began at a temperature of 60°C and became more significant at 65°C (13). This was later confirmed by Czank et al. who demonstrated a significant impact of the temperature and an early alteration starting at 58°C (14). The study also demonstrated the influence of the duration of pasteurization, with a loss of 1.6, 1.7, and 2.4%, respectively, for IgA, lysozyme and lactoferrin per each minute spent at a temperature of 62.5°C (14).
The recording of the temperature of milk in several bottles, by means of external probes, during the pasteurization cycle showed significant differences, in terms of temperature or the duration of exposure of HM, depending on the device. The increase in the temperature of milk is in fact fast up to 58°C, but the inertia of heating is then responsible for a slowing down of the rise in temperature up to 62.5°C. It has been demonstrated that HM immune components start to be damaged significantly from 58°C (14). The regulation of each pasteurizer is therefore crucial to minimize the exposure time responsible for the damage to HM. Buffin et al. reported that the difference in exposure above 58°C could be as much as 10 min longer, depending on the device. In addition, the average temperature of the plateau can vary by nearly 0.8°C. These effects are only visible when several calibrated probes are used (12, 15). It is important to note that the milk was shaken during the heat treatment in both types of tested pasteurizers (12).
A single recording probe in just one of the bottles does not allow either the whole pasteurizing process to be understood or deviances in the system to be detected. Since HoP is currently conducted at a relatively high temperature (62.5°C), it is important to control this temperature and the duration of exposure (12, 15). Furthermore, most of the studies that have evaluated or compared HoP with other techniques have not described the pasteurizer cycle precisely. The differences that exist between pasteurizers can be important and can have an important impact on the assessed components. This could explain the discrepancies that have been found between the different results in literature. These inconsistencies make it difficult to make a definitive statement on the effects of HoP. For these reasons, any future study on HoP should adopt a standardized approach to ensure consistency. However, it is important to recognize that where research on HoP is being carried out, consistency, and quality assurance adds a necessary component that was previously lacking in the approach.
Therefore, a routine recording of the milk temperature in one bottle located in the middle of a bath is important to control each pasteurization cycle. This probe is not present in all the pasteurizers available on the market today, and is present even less in a simple water bath. Each pasteurizer should be made to undergo regular quality controls, performed by each HMB using several external probes. Some criteria have recently been proposed (Table 1) (12, 15). Since 2016, such quality controls have been performed regularly, at least once a year, in the 36 French HMBs. Manufacturers should provide these criteria when they propose qualified pasteurizers to HMBs.
This qualification has two purposes:
• The first is to highlight a dysregulation of the pasteurizer;
• The second, which is based on the results, is to require the manufacturer to adjust and optimize the regulation of the pasteurizer in order to minimize the temperature plateau range, the duration of the pasteurization plateau, and to ensure cycle accuracy and repeatability.
Moreover, since HoP is the most frequently used technique, it should be considered, as part of the optimization, whether a value of 62.5°C is the best temperature for the treatment of human milk. Czank et al. have shown the effectiveness of pasteurization on bacteria at temperatures above 57°C. However, it is known that Cytomegalovirus persists at this temperature, but it could be useful to test intermediate temperatures, such as 60°C and / or shorter exposure times (14).
Finally, it should be kept in mind that the heating phase should be followed immediately by a rapid chilling phase to 4°C to minimize the additional time during which the milk is exposed to the high water bath temperatures and to reduce the further destruction of heat labile components. This thermic shock could also prevent aerobic spore-forming bacteria from multiplying.
As long as HoP remains the main technique utilized in HMBs, it should be made as optimal as possible, with quality assurance being obtained through checks and calibration. When comparing HoP with new techniques, it should be ascertained that HoP is performed correctly, and the comparison should be made in conditions as close as possible to the routine daily practices in the HMBs.
High-Temperature Short-Time (HTST) Pasteurization
HTST was the first non-HoP technique tested to improve the nutritional and immunological quality of milk, and it was first established in the dairy industry in the 1930s (16). HTST is usually performed by heating thin layers of milk in continuous flow systems at 72°C for 15 s. This technology has been applied to the treatment of DHM with promising results (9). Immunological components, and in particular immunoglobulins (Igs), are known to be affected by HoP (17–19), and have often been targeted as qualitative/functional parameters in studies on alternative HM pasteurization technologies. Goldsmith et al. were the first to test HTST pasteurization on HM using a stainless steel laboratory capillary heat exchanger (20). They reported comparable degradation after HTST and HoP for Igs and lactoferrin. The retention of HM Igs was found to decrease as the temperature and holding time increased. Therefore, the search for an optimal compromise between microbiological safety and biological quality should be made considering the pasteurization equipment and the working conditions. Data regarding the effect of HTST pasteurization on lactoferrin and lysozyme concentrations and activities are sometimes divergent, due to the fact that different methods were used to apply the HTST technology in the different studies.
Overall, it has emerged that HTST performed at a laboratory scale or pilot scale is at least equivalent to HoP in ensuring HM microbiological safety, but is better at preserving the HM antioxidant potential, lactoferrin content and structure, B and C vitamins, and some cytokines.
Two HTST pasteurizers have recently been specifically designed and validated for human milk processing (21, 22).
In the first study, a new small-scale, continuous-flow, HTST pasteurizer was designed to treat HM. The efficacy of the new HTST device was assessed on inoculated Listeria monocytogenes, Staphylococcus aurous, and Chronobacter sakazakii, as well as on raw human milk bacteria. The biological quality of the milk was assessed after HTST pasteurization and compared with a standard HoP, by determining the secretory IgA (sIgA) content, the protein profile, lysozyme and the Bile Salt Stimulated Lipase (BSSL) activities. No pathogen or bacterial growth was detected after HTST pasteurization with the new instrument. Changes in the protein profile were observed in the milk pasteurized with both processes. The sIgA content and BSSL activity were significantly higher in the milk pasteurized with the new device than in the same milk treated with the standard HoP. In conclusion, the new HTST apparatus was able to effectively pasteurize HM and showed a better retention of the sIgA content and a better BSSL activity (21). However, these results still have to be confirmed in HMB conditions.
Escuder-Vieco et al. described HTST equipment designed specifically for the continuous and adaptable (time-temperature combination) processing of DHM, considering the specific needs of a human milk bank (22). Microbiological quality, the activity of the indicator enzymes and indices for thermal damage to HM were evaluated before and after HTST treatment using different temperature and time combinations and the results were compared with the results obtained after HoP (22). The HTST system had an accurate and simple operation mechanism, which allowed the pasteurization of variable amounts of DHM and reduced both the processing time and the labor force. HTST processing at 72°, for at least 10 s, effectively destroyed all the vegetative forms of the microorganisms that were initially present in the raw DHM. Alkaline phosphatase was completely destroyed after HTST processing at 72 and 75°, but γ-glutamil transpeptidase showed higher thermoresistance, thus indicating that this could be used as a quick, simple, and inexpensive test. The furosine concentrations in HTST-treated donor HM were lower than those obtained after HoP, and the lactulose content for HTST-treated DHM was below the detection limit of the analytical method (10 mg/L). The absence of lactulose and the small amount of furosine found in HTST-treated DHM indicated that a heat treatment with this new HTST equipment did not induce any significant heat damage to DHM. In addition, a higher retention of immunoglobulins, some hormones, BSSL activity and antioxidant capacity were found in HTST-treated DHM samples than in the samples treated by means of HoP (22).
High Pressure Processing (HPP)
HPP is a well-known technique in the food industry, and it is considered a promising alternative to the thermal pasteurization of HM. HPP is a non-thermal processing method that can be applied to solid and liquid foods to provide microbiologically safe, nutritionally intact, and sensory high-quality products (23). This technique inactivates pathogenic microorganisms by applying high hydrostatic pressure (usually 400–800 MPa) through short-term treatments (<5–10 min) (24).
Viazis et al. were the first to point out the retention of nutrients and the bioactivity and microbial safety of pascalized HM (25, 26).
Other researchers have shown that HM activity after processed over a 300 to 650 MPa HPP range is similar to heat-treated milk. IgA, IgM, IgG, lysozyme, lactoferrin, cytokines (EGF, TGF-β1 and TGF-β2, IL-6, IL-8, TNF-α, IL-12, IL-17, and IFN-γ) α- and δ-tocopherol, and free nucleotide monophosphates are partly preserved (27–32).
The destruction of Listeria monocytogenes, Eschericha coli, Staphylococcus aurous, and Salmonella spp, within the 300–400 MPa pressure range, is comparable with the microbiological purity obtained after thermal pasteurization (25, 28, 33).
Moreover, recently obtained results suggest that anHPP treatment, at pressures below 600 MPa for 15 min, allows the antirotaviral activity to be retained (34).
This technique respects the sensorial and nutritional properties of food better than HoP, because of the absence of a heat treatment (35, 36). As far as the safety and taste satisfaction of donor milk recipients are concerned, the profile of the volatile milk components has been examined after processing. Generally, the change in the sensory quality of human milk after a high-pressure treatment has been found to be less than that caused by HoP (35, 36).
It should be taken into consideration that a change in the lipid fraction may take place as a result of HPP. Milk fat is distributed as globules in colloidal fluid produced by the mammary gland. Any physical factor that is able to influence the stabilization of this component, either pressurization or a warm temperature, causes a decreased fat globule size, which is defined as a homogenization (37).
Exposure to pressures below 600 MPa has not been found to influence the content or composition of the lipid fraction of HM. However, increasing pressure above this limit might result in undesirable changes in the content of selected fatty acids in human milk. A risk of lipid oxidation products in HM after processing has been reported (38, 39).
The HPP technique seems to offer clear advantages over HoP: it results in an improved nutritional quality product; it is faster and perhaps more convenient than HoP; it can be applied to frozen milk samples and it can be used on samples of variable size.
A French team from Bordeaux and a Polish team from Warsaw, with representatives from the EMBA Working Group (CB and AW), have evaluated this technology with positive results. HPP seems to be able to better maintain some milk proteins (HGF, lactoferrin, IgG), and to preserve active hormones (leptine, adiponectine, insulin, erythropoietin) and enzymes (lipase) (EMBA International Conference on Donor Human Milk, Glasgow, October 5–6th, 2017). Until recently, it was considered that vegetative cells are more effectively destroyed by HPP than endosporic forms. Billaud and Demazeau have recently optimized the operational parameters (pressure, rate, decompression, and application mode) and this has allowed the inactivation of B. cereus spores. Under these conditions, the activity of certain important human milk biological components, such as lipase activity and immune proteins, is maintained. These results were obtained with a pressure of 350 MPa (40).
The main obstacle to the use of HPP in human milk treatment, is the scaling down of the equipment and the investment and operating costs. It has been calculated on the basis of a cost consequence analysis conducted with a regional model of human milk banking operating in Poland, that the cost of pascalized donor milk will be 130% higher than milk treated by means of Holder (unpublished data). However, there are some small and medium-size enterprises in Poland that are interested in investing in the human milk bank market. The prototype equipment for human milk pascalization has already been described, and the next step will be to obtain the money to construct and validate the device (Figure 1).
At present, only prototypes of these HPP devices exist, and this technique still has to be tested under HMB conditions.
Ultraviolet-C Irradiation
Ultraviolet (UV) irradiation utilizes short-wavelength ultraviolet radiation in the UV-C region (200–280 nm), which is harmful to microorganisms. It is effective in destroying the nucleic acids in these organisms, so that their DNA is disrupted by the UV radiation, leaving them unable to perform vital cellular functions. The greater the exposure to UV rays, the better the result, and this ensures a complete destruction of all the microorganisms (1, 3). UV light only penetrates food materials by several millimeters, depending on the optical properties of the product. Ultraviolet light penetrates the cells, but does not alter the food that is being treated. The color and/or turbidity of the liquid influences its optical absorption coefficient. UV light cannot penetrate milk or other cloudy foods, like other opaque foods. As a consequence, these substances must be presented to the system as a thin layer, and this constitutes a concern when large volumes of donor HM in HMBs are being treated daily (41, 42).
Some preliminary reports have shown that UV irradiation is able to produce a reduction of 5 log 10 in the exogenously-added bacteria in HM, without affecting the lipase activity (43). The concentrations of lactoferrin, lysozyme and immunoglobulin A (IgA) have been described as basically being unaltered (44), and it has also recently been reported that ultraviolet -C radiation is able to inactivate cytomegalovirus in HM under the correct conditions (45).
The main challenge to testing this methodology is the lack of appropriate equipment in the human milk bank context. In order to further analyze the potential application of UV-C irradiation in this context, a Spanish group from Palma de Mallorca (JC and AG), has designed an instrument in which milk is kept in motion, through the use of a magnetic stirrer bar, which creates a low velocity, laminar flow vortex, thus transporting and overcoming the low penetrance of UV irradiation. Figure 2 demonstrates the instrument, which allows 500 ml of milk to be processed: it consists of a graduated cylinder glass tube in which a 26 cm long UV-C lamp with 8 w power has been introduced, so that 10 min of treatment equals 9,600 Joules/Liter (LIT-06; Instrumentación Científico Técnica S.L., La Rioja, Spain). In this treatment, the milk is kept at room temperature and agitated with a magnetic bar and a stirrer at 200 rpm.
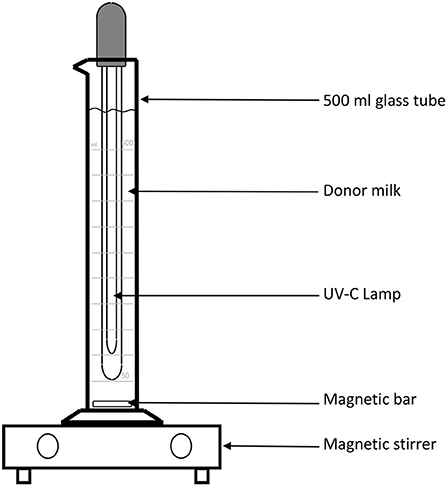
Figure 2. Schematic representation of the device used to treat donor human milk with UV-irradiation.
With this device, Calvo and Gaya tested five different 500 ml batches of DHM that had been discarded during routine processing in their HMB due to the presence of high levels of contamination. Of the five analyzed samples, two presented >105 CFU/ml and three >106 CFU/ml. In all cases, the contamination was due to a mixed flora, including gram-negative bacteria. A sample was taken at different times after the treatment was started (0, 15, 30, 45, and 60 min) and the number of CFU was quantified by means of conventional microbiological techniques. These experiments showed that, after 30 min of treatment, the amount of CFU/ml was reduced by five orders of magnitude (log10) in all cases. The fact that the used samples were representative of the DHM samples usually treated in a HMB is of particular relevance.
Bacillus is a bacteria genus that is frequently found in DHM, and it represents a special concern for HMBs, as these bacteria are capable of producing heat-resistant toxins and forming spores that are resistant to pasteurization (46, 47). In the HMB of Palma de Mallorca, about 10% of the milk is generally discarded due to the presence of Bacillus sp. In order to test the susceptibility of Bacillus sp to the UV treatment, the researchers used two different batches of donor milk that had been discarded as a result of contamination with Bacillus sp., 3,000 CFU/ml in both cases. The results showed that after 45 min of treatment, the Bacillus sp. were eliminated.
The Spanish group then evaluated the effect on the biological components of HM. One of the main biological components is IgA, which constitutes 90% of all the immunoglobulins present in colostrum and HM. Its importance lies not only in its concentration, but also in its biological activity (48). It has been pointed out that pasteurization affects IgA levels to different extents, depending on the pasteurization temperature (14). In the case of HoP, a clear decrease in IgA concentration was observed, although there were large discrepancies in the range of reduction, from 20 to 60% (9). The results of this group have shown that, after testing seven different batches of DHM, the IgA levels measured by conventional nephelometry techniques, were 96% of the pre-treatment levels, and in five samples, a 100% activity was preserved.
From these results, it can be concluded that the treatment with UV-C light has a number of features that make it a good candidate as an alternative to HoP. In addition to providing a better protection of the biological components than other methods, it is also capable of producing an at least 5-log10 decrease in the number of bacteria (including Bacillus sp) present in DHM. Furthermore, the ability of UV-C radiation to eliminate active forms of Cytomegalovirus in HM has also been demonstrated (45). Unfortunately, until now, there is neither a device nor even a prototype that would enable the use of this technology in an HMB setting.
EMBA Working Group Recommendations
One important aspect that should be considered when evaluating the processing of human milk is the viral inactivation effect of the new methodologies.
The ability of LTLT pasteurization to inactivate viral pathogens is well-known. The list of human viruses inactivated by HoP includes pathogens for which transmission through breastfeeding has been conclusively demonstrated (i.e., the human immunodeficiency virus, human T-cell lymphotrofic virus, cytomegalovirus), and viruses that can be transmitted via breast milk, such as human papillomavirus, Zikavirus, Ebola and the Marbourg virus (49–55).
On the other hand, virus inactivation still has to be carefully evaluated for each alternative technique and device designed to treat breast milk. This is an important issue for future research.
We can state that fundamental knowledge of new technologies of HM processing is still lacking. Their effects on safety and bioactive components of HM need further evaluation. Table 2 presents the “state of the art” at the moment, explaining advantages, and disadvantages of the processing techniques described in this paper.
On the basis of evidence taken from the literature and on the personal experience of its members, the Working Group on the Processing of Human Milk makes the following recommendations:
When testing new technologies, the following requirements should be fulfilled:
- The equipment should be described precisely
- The control of the equipment and repeatability of the process should be demonstrated
- The process parameters should be recorded
- Tests should not be performed only at a lab scale, but also in an HMB environment
The final aim of HM processing performed with new technologies should be:
- To improve the preservation of the nutritional and bioactive components of raw HM (in order to at least ensure the same microbiological safety as HoP)
- To improve the microbiological safety of treated DHM, taking into account the inactivation of spores, even though this aspect is not at present considered in all the guidelines that regulate the activity of HMBs
- To inactivate the viral effect on human viruses for which transmission through breastfeeding has been demonstrated
- Easy placement of the new plant in HMBs
- Low cost, in order to overcome the problem of the limited financial resources of the majority of HMBs.
A workflow that can be considered suitable to assess the basic performance of new pasteurization technologies for HM is shown in Figure 3 and Table 3. Since Holder pasteurization is not efficient in eradicating spore-forming bacteria, this parameter has not been included in the validation targets. However, any new pasteurization system that could prove to be efficient against spore-forming bacteria (while maintaining all the other aforementioned characteristics) would represent a great advantage for the improvement of HM safety.
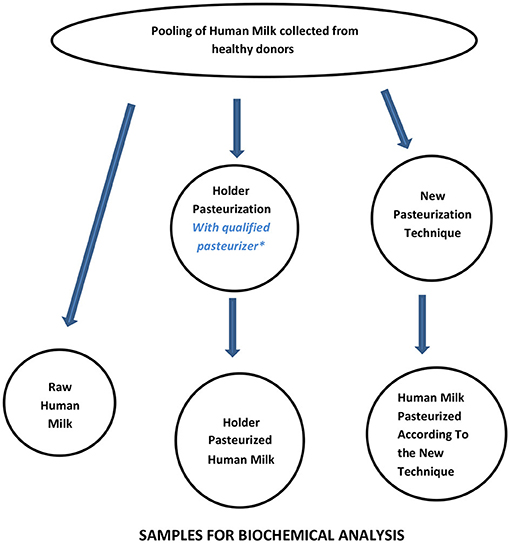
Figure 3. Workflow for assessing the performance of new pasteurization technologies for human milk. *For the qualification of the Holder pasteurizer, see Buffin et al. (12).
Conclusions
This paper presents the recommendations of the EMBA Working Group on the “Processing of HM.” Although research on the most promising technologies, which will represent a reasonable alternative to HoP in the future (HTST, HPP, UV-C) is progressing, at the moment it is important to recognize that the consistency and quality assurance of the pasteurizers currently available on the market today represent a fundamental approach that was previously lacking in HoP practice.
EMBA recognizes that HoP is at present the safest compromise for the treatment of DHM; however, further studies are needed to improve this technology in order to minimize its effects on the biological components of HM. The new technologies evaluated and studied by the Working Group are being developed rapidly, and EMBA recommends that the final aim of these technologies should be an improved preservation of the nutritional and bioactive components of raw human milk, while assuring microbiological safety of the product, at least at the same level as optimized HoP.
Author Contributions
GEM wrote the manuscript. CB, BR, JC, LaC, LuC, DE-V, AGa, DL, and AW are components of the EMBA Working Group on Processing of Donor Human Milk and contributed to the content of this manuscript. SA, EB, AGr, DB, C-YB, CG, GW, and J-CP are components of the Board of Directors of EMBA and made comments and gave suggestions for the final preparation of the manuscript.
Conflict of Interest Statement
LuC is employed by Carag AG, Switzerland. However, Carag AG did not offer any financial support for this paper.
The remaining authors declare that the research was conducted in the absence of any commercial or financial relationships that could be construed as a potential conflict of interest.
The reviewer MG declared a past co-authorship with the authors JC and AGa to the handling editor.
Acknowledgments
The authors are grateful to the Italian Association of Human Milk Banks (Associazione Italiana Banche del Latte Umano Donato = AIBLUD) for its continuous efforts to promote research in the field of donor human milk and Human Milk Banks, and for the financial support for the publication of this manuscript.
References
1. World Health Organization (WHO). Guidelines on Optimal Feeding of Low Birth-Weight Infants in Low- and Middle-Income Countries. Geneva: WHO. (2011).
2. American Academy of Pediatrics (AAP). Breastfeeding and use of human milk. Pediatrics. (2012) 129:e827–41. doi: 10.1542/peds.2011-3552
3. Arslanoglu S, Corpeleijn W, Moro G, Braegger C, Campoy C, Colomb V, et al. Donor human milk for preterm infants: current evidence and research directions. J Pediatr Gastroenterol Nutr. (2013) 57:535–42 doi: 10.1097/MPG.0b013e3182a3af0a
4. World Health Organization (WHO)/United Nations Children's Fund (UNICEF). Meeting on infant and young child feeding. J Nurs Midw. (1980) 25:31–8.
5. Arslanoglu S, Bertino E, Tonetto P, e Nisi G, Ambruzzi AM, Biasini A, et al. Guidelines for the establishment and operation of a donor human milk bank. J Matern Fetal Neonatal Med. (2010) 23:1–20 doi: 10.3109/14767058.2010.512414
6. Human Milk Bank Association of North America (HMBANA). Guidelines for the Establishment and Operation of a Donor Human Milk Bank. 10th ed. Raleigh, NC: Human Milk Bank Association of North America (2018).
7. Hartmann BT, Pang WW, Keil AD, Hartmann PE, Simmer K. Best practice guidelines for the operation of a donor human milk bank in an Australian NICU. Early Hum Dev. (2007) 83:667–73 doi: 10.1016/j.earlhumdev.2007.07.012
8. Hartmann BT, Pang WW, Keil AD, Hartmann PE, Simmer K. National Guidelines on Lactation Management Centres in Public Health Facilities. Child Health Division, Ministry of Health and family Welfare, Government of India. (2017).
9. Peila C, Moro GE, Bertino E, Cavallarin L, Giribaldi M, Giuliani F, et al. The effect of holder pasteurization on nutrients and biologically-active components in donor human milk: a review. Nutrients. (2016) 8:477–95. doi: 10.3390/nu8080477
10. Moro GE, Arslanoglu S. Heat treatment of human milk. J Pediatr Gastroenterol Nutr. (2012) 54:165–6 doi: 10.1097/MPG.0b013e318235d629
11. Tully DB, Jones F, Tully MR. Donor milk: what's in it and what's not. J Hum Lact. (2001) 17:152–5 doi: 10.1177/089033440101700212
12. Buffin R, Pradat P, Trompette J, Ndiaye I, Basson E, Jordan I, et al. Air and water processes do not produce the same high-quality pasteurization of donor human milk. J Hum Lact. (2017) 33:717–24. doi: 10.1177/0890334417707962
13. Evans TJ, Ryley HC, Neale LM, Dodge JA, Lewarne VM. Effect of storage and heat on antimicrobial proteins in human milk. Arch Dis Childhood. (1978) 53:239–41. doi: 10.1136/adc.53.3.239
14. Czank C, Prime DK, Hartmann B, Simmer K, Hartmann PE. Retention of the immunological proteins of pasteurized human milk in relation to pasteurizer design and practice. Pediatr R Search. (2009) 66:374–79. doi: 10.1203/PDR.0b013e3181b4554a
15. Picaud JC, Buffin R. Human milk-treatment and quality of banked human milk. Clin Perinatol. (2017) 44:95–119 doi: 10.1016/j.clp.2016.11.003
16. Holsinger VH, Rajkowski KT, Stabel JR. Milk pasteurisation and safety: a brief history and update. Rev Sci Tech. (1997) 16:441–51 doi: 10.20506/rst.16.2.1037
17. Silvestre D, Ferrer E, Gayá J, Jareño E, Miranda M, Muriach M, et al. Available lysine content in human milk: stability during manipulation prior to ingestion. Biofactors. (2006) 26:71–9. doi: 10.1002/biof.5520260107
18. Góes HC, Torres AG, Donangelo CM, Trugo NM. Nutrient composition of banked human milk in Brazil and influence of processing on zinc distribution in milk fractions. Nutrition. (2002) 18:590–4. doi: 10.1016/S0899-9007(02)00813-4
19. Ford JE, Law BA, Marshall VME, Reiter B. Influence of the heat treatment of human milk on some of its protective constituents. J Pediatric. (1977) 90:29–35. doi: 10.1016/S0022-3476(77)80759-2
20. Goldsmith SJ, Dickson JS, Barnhart HM, Toledo TR, Eiten-Miller RR. IgA, IgG, IgM and lactoferrin contents of human milk during early lactation and the effect of processing and storage. J Food Protec. (1983) 1:4–7 doi: 10.4315/0362-028X-46.1.4
21. Giribaldi M, Coscia A, Peila C, Antoniazzi S, Lamberti C, Ortoffi M, et al. Pasteurization of human milk by a benchtop High-temperature Short-Time device. Innov Food Sci Emerg Tech. (2016) 36:228–33. doi: 10.1016/j.ifset.2016.07.004
22. Escuder-Vieco D, Espinosa-Martos I, Rodríguez JM, Corzo N, Montilla A, Siegfried P, et al. High-temperature short-time pasteurization system for donor milk in a human milk bank setting. Front Microbiol. (2018) 9:1–16. doi: 10.3389/fmicb.2018.00926
23. Considine KM, Kelly AL, Fitzgerald GF, Hill C, Sleator RD. High-pressure processing-effects on microbial food safety and food quality. FEMS Microbiol Lett. (2008) 281:1–9. doi: 10.1111/j.1574-6968.2008.01084.x
24. Huppertz T, Fox PF, de Kruif KG, Kelly AL. High-pressure-induced changes in bovine milk: a review. Int J Dairy Tech. (2006) 59:58–66. doi: 10.1111/j.1471-0307.2006.00246.x
25. Viazis S, Farkas BE, Jaykus LA. Inactivation of bacterial pathogens in human milk by high-pressure processing. J Food Protec. (2008) 71:109–18. doi: 10.4315/0362-028X-71.1.109
26. Sousa SG, Delgadillo I, Saraiva JA. Effects of high-pressure processing on immunoglobulin and lysozyme activity in human milk. J Hum Lact. (2007) 23:253–61. doi: 10.1177/0890334407303945
27. Sousa SG, Delgadillo I, Saraiva JA. Effect of thermal pasteurisation and high-pressure processing on immunoglobulin content and lysozyme and lactoperoxidase activity in human colostrum. Food Chem. (2014)151:79–85. doi: 10.1016/j.foodchem.2013.11.024
28. Permanyer M, Castellote C, Ramírez-Santana C, Audí C, Pérez-Cano FJ, Castell M, et al. Maintenance of breast milk immunoglobulin A after high-pressure processing. J Dairy Sci. (2010) 93:877–83. doi: 10.3168/jds.2009-2643
29. Mayayo C, Montserrat M, Ramos SJ, Martínez-Lor nzo MJ, Calvo M, Sánchez L, et al. Effect of high pressure and at treatments on IgA immunoreactivity and lysozyme activity in human milk. Eur Food Res Technol. (2016) 242:891–8. doi: 10.1007/s00217-015-2595-7
30. Franch A, Audi C, Ramírez-Santana C, Permanyer M, Pérez-Cano FJ, Moltó-Puigmartí C, et al. Banked human milk treatment and immunoactive factors content. Eur Food Res Tech. (2009) 242:891–8. doi: 10.1017/S0029665110000777
31. Mayayo C, Montserrat M, Ramos SJ, Martínez-Lorenzo MJ, Pérez MD. Kinetic parameters for high-pressure-induced denaturation of lactoferrin in human milk. Int Dairy J. (2014) 39:246–52. doi: 10.1016/j.idairyj.2014.07.001
32. Delgado FJ, Cava R, Delgado J, Ramirez R. Tocopherols, fatty acids and cytokines content of holder pasteurized and high pressure processed human milk. Dairy Sci Technol. (2014) 94:145–56. doi: 10.1007/s13594-013-0149-y
33. Windyga B, Rutkowska M, Sokołowska B, Skapska S, Wesołowska A, Wilinska M, et al. Inactivation of Staphylococcus aureus and native microflora in human milk by high pressure processing. High Pressure Res. (2015) 35:181–8. doi: 10.1080/08957959.2015.1007972
34. Parrón JA, Ripollés D, Ramos SJ, Pérez MD, Semen Z, Rubio P, et al. Effect of thermal and high-pressure treatments on the antirotaviral activity of human milk fractions. Innov Food Sci Emerg Tech. (2018) 47:262–70. doi: 10.1016/j.ifset.2018.03.008
35. Garrido M, Contador R, García-Parra J, Delgado FJ, Delgado-Adámez J, Ramírez R. Volatile profile of human milk subjected to high-pressure thermal processing. Food Res Int. (2015) 78:186–94. doi: 10.1016/j.foodres.2015.10.016
36. Contador R, Delgado FJ, García-Parra J, Garrido M, Ramírez R. Volatile profile of breast milk subjected to high-pressure processing or thermal treatment. Food Chem. (2015) 180:17–24. doi: 10.1016/j.foodchem.2015.02.019
37. Huppertz T, Zobrist MR, Uniacke T, Upadhyay V, Fox PF, Kelly AL. Effects of high pressure on constituents and properties of milk. Int Dairy J. (2002) 12:561–72. doi: 10.1016/S0958-6946(02)00045-6
38. Huppertz T, Zobrist MR, Uniacke T, Upadhyay V, Fox PF, Kelly AL. Effect of high pressure and sub-zero temperature on total antioxidant capacity and the content of Vitamin C, fatty acids and secondary products of lipid oxidation in human milk. Pol J Food Nutr Sci. (2017) 67:117–22. doi: 10.1515/pjfns-2016-0011
39. Moltó-Puigmartí C, Permanyer M, Castellote AI, López-Sabate MC. Effects of pasteurization and high-pressure processing on vitamin C, tocopherols and fatty acids in mature human milk. Food Chem. (2011) 124:697–702. doi: 10.1016/j.foodchem.2010.05.079
40. Demazeau G, Plumecocq A, Lehours P, Martin P, Couëdelo L, Billeaud C. A new high hydrostatic pressure process to assure the microbial safety of human milk while preserving the biological activity of its main components. Front Public Health. (2018) 6:306. doi: 10.3389/fpubh.2018.00306
41. Tran MTT, Farid M. Ultraviolet treatment of orange juice. Innov Food Sci Emerg Technol. (2004) 5:495–502. doi: 10.1016/j.ifset.2004.08.002
42. Bintsis T, Litopoulou-Tzanetaki E, Robinson RK. Existing and potential applications of ultraviolet light in the food industry – a critical revision. J Sci Food Agric. (2000) 80:637–45. doi: 10.1002/(SICI)1097-0010(20000501)80:6%3C637::AID-JSFA603%3E3.0.CO;2-1
43. Christen L, Lai CT, Hartmann B, Hartmann PE, Geddes DT. The effect of UV-C pasteurization on bacte iostatic properties and immunological proteins of donor human milk. PLoS ONE. (2013) 8:e85867. doi: 10.1371/journal.pone.0085867
44. Christen L, Lai CT, Hartmann B, Hartmann PE, Geddes DT. Ultraviolet-C irradiatio: a novel pasteurization method for donor human milk. PLoS ONE. (2013) 8:e68120. doi: 10.1371/journal.pone.0068120
45. Lloyd ML, Hod N, Jayaraman J, Marchant EA, Christen L, Chiang P, et al. Inactivation of cytomegalovirus in breast milk using ultraviolet-C irradiation: opportunities for a new treatment option in breast milk banking. PLoS ONE. (2016) 11:1–12. doi: 10.1371/journal.pone.0161116
46. Walker-York-Moore L, Moore SC, Fox EM. Characterization of enterotoxigenic bacillus cereus sensu lato and Staphylococcus aureus isolates and associated enterotoxin production dynamics in milk or meat-based broth. Toxins. (2017) 9:1–15. doi: 10.3390/toxins9070225
47. Rigourd V, Barnier JP, Ferroni A, Nicloux M, Hachem T, Magny JF, et al. Recent actuality about Bacillus cereus and human milk bank: a new sensitive method for microbiological analysis of pasteurized milk. Eur J Clin Microbiol Infect Dis. (2018) 37:1297–303. doi: 10.1007/s10096-018-3249-z
48. Palmeira P, Carneiro-Sampaio M. Immunology of breast milk. Rev Assoc Med Bras. (2016) 62:584–93. doi: 10.1590/1806-9282.62.06.584
49. Eglin RP, Wilkinson AR. HIV infection and pasteurization of breast milk. Lancet. (1987) 1:1093. doi: 10.1016/S0140-6736(87)90521-6
50. Orloff SL, Wallingford JC, McDougal JS. Inactivation of human immunodeficiency virus type I in human milk: effects of intrinsic factors in human milk and of pasteurization. J Hum Lact. (1993) 9:13–7. doi: 10.1177/089033449300900125
51. Hamprecht K, Maschamann J, Muller D, Dietz K, Besenthal I, Goelz R, et al. Cytomegalovirus (CMV) inactivation in breastmilk: reassessment of pasteurization and freeze-thawing. Pediatr Res. (2004) 56:529–35. doi: 10.1203/01.PDR.0000139483.35087.BE
52. Friis H, Andersen HK. Rate of inactivation of cytomegalovirus in raw banked milk during storage at−20°C and pasteurisation. Br Med J. (1982) 285:1604–5. doi: 10.1136/bmj.285.6355.1604
53. Donalisio M, Cagno V, Vallino M, Moro GE, Arslanoglu S, Tonetto P, et al. Inactivation of high-risk human papillomaviruses by Holder pasteurization: implications for donor human milk banking. J Perinat Med. (2014) 42:1–8. doi: 10.1515/jpm-2013-0200
54. Pfaender S, Vielle NJ, Ebert N, Steinmann E, Alves MP, Thiel V. Inactivation of Zika virus in human breast milk by prolonged storage or pasteurization. Virus Res. (2017) 228:58–60. doi: 10.1016/j.virusres.2016.11.025
Keywords: processing of human milk, donor human milk, human milk, human milk bank, preterm infants, infant nutrition
Citation: Moro GE, Billeaud C, Rachel B, Calvo J, Cavallarin L, Christen L, Escuder-Vieco D, Gaya A, Lembo D, Wesolowska A, Arslanoglu S, Barnett D, Bertino E, Boquien C-Y, Gebauer C, Grovslien A, Weaver GA and Picaud J-C (2019) Processing of Donor Human Milk: Update and Recommendations From the European Milk Bank Association (EMBA). Front. Pediatr. 7:49. doi: 10.3389/fped.2019.00049
Received: 15 October 2018; Accepted: 06 February 2019;
Published: 28 February 2019.
Edited by:
Po-Yin Cheung, University of Alberta, CanadaReviewed by:
María Gormaz, Agencia Valenciana de Salud, SpainBritt Nakstad, University of Oslo, Norway
Copyright © 2019 Moro, Billeaud, Rachel, Calvo, Cavallarin, Christen, Escuder-Vieco, Gaya, Lembo, Wesolowska, Arslanoglu, Barnett, Bertino, Boquien, Gebauer, Grovslien, Weaver and Picaud. This is an open-access article distributed under the terms of the Creative Commons Attribution License (CC BY). The use, distribution or reproduction in other forums is permitted, provided the original author(s) and the copyright owner(s) are credited and that the original publication in this journal is cited, in accordance with accepted academic practice. No use, distribution or reproduction is permitted which does not comply with these terms.
*Correspondence: Guido E. Moro, Z3VpZG9lbW9yb0B0aXNjYWxpLml0