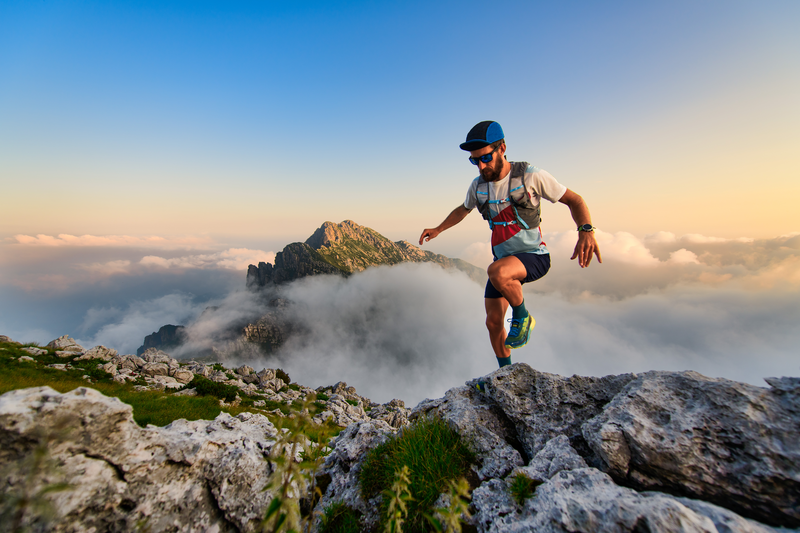
95% of researchers rate our articles as excellent or good
Learn more about the work of our research integrity team to safeguard the quality of each article we publish.
Find out more
REVIEW article
Front. Pediatr. , 19 September 2018
Sec. Pediatric Critical Care
Volume 6 - 2018 | https://doi.org/10.3389/fped.2018.00257
Measurement of energy expenditure is important in order to determine basal metabolic rate and inform energy prescription provided. Indirect calorimetry is the reference standard and clinically recommended means to measure energy expenditure. This article reviews the historical development, technical, and logistic challenges of indirect calorimetry measurement, and provides case examples for practicing clinicians. Formulae to estimate energy expenditure are highly inaccurate and reinforce the role of the indirect calorimetry and the importance of understanding the strength and limitation of the method and its application.
Living organisms are dependent on the constant expenditure of energy-rich adenosine tri-phosphate (ATP) for survival. Energy production is tightly controlled by the organism and is required to sustain cellular homeostasis, organ function, and growth (1–3). The continuous, formation of energy requires a constant supply of substrates: primarily glucose, fatty acids, and oxygen (O2). Energy from their combination is used to produce ATP by oxidative phosphorylation, and results in the by-products carbon dioxide (CO2) and water (H2O) (1, 3).
Understanding of cellular metabolism advanced in the Eighteenth century when Lavoisier and Laplace described the measurement of heat exchange (4). In the late Nineteenth century, the first direct calorimeter, the Atwater-Rosa calorimeter, was made. Using the calorimeter scientists were able to demonstrate the law of conservation of energy: the equivalency between fuel (energy) consumed and heat (energy) produced. This work showed that the expenditure of energy by organisms can be measured directly by direct calorimeters or estimated by measuring oxygen consumption (O2): indirect calorimetry (4) (Figure 1). Indirect calorimetry is now considered the reference standard for measurement of energy expenditure in critically ill children (5). It is particularly recommended in children with nutritional deficits or derangements due to underlying disorders like cancers or acute diseases like sepsis or multiple trauma that are associated with large inaccuracies in estimation of energy needs, and those who fail attempts at liberation from mechanical ventilation (5–8).
Figure 1. Direct calorimetry measures heat production and indirect calorimetry measures gas exchange: oxygen consumption and carbon dioxide production.
In this review, we summarize the historical context for development of indirect calorimeters, the different systems utilized in energy expenditure measurement along with their limitations, and the clinical considerations required to perform and interpret a measurement of energy expenditure done with indirect calorimetry in critically ill children.
Interest in the development of technology to measure energy started in the 1800's when Regnault and Reiset devised a closed-circuit system for measurement of O2 consumption, and demonstrated the ratio of CO2 produced to O2 consumed varied according to the type of food ingested (9, 10) (Figure 2). von Voit and von Pettenkofer determined the proportions of carbon, nitrogen, and O2 involved in metabolism in different dietary conditions, and demonstrated that metabolism could be fully interpreted in terms of the oxidation of three types of food substances: protein, fat, and carbohydrate(4, 10). Rubner, in 1894, built a direct calorimeter that measured the heat given by animals based on the temperature change in a surrounding water medium. The animal chamber was also connected to a Pettenkofer respiration chamber for respiratory analysis. Rubner demonstrated complete agreement between the two methods (10). Five years later, the Atwater and Rosa calorimeter employed the open-circuit Pettenkofer system for indirect estimation of heat production, and they coined the term 'respiration calorimeter'. After it had been established that the estimate of heat production from respiratory analysis agreed with the direct measurement of heat, the term indirect calorimeter came to be applied to respiration chambers.
Figure 2. The apparatus of Henri Victor Regnault and Jules Reiset (1849). Adapted from Regnault and Reiset. In this closed loop device, oxygen was supplied to the dog by a tube on the left and carbon dioxide was removed by the tubes on the right. Oxygen was delivered as required to replace that used up by the animal, therefore oxygen consumption was measured by the amount required to maintain constant system pressure. Carbon dioxide was removed by an absorbent and then returned to the respiration chamber to be used over again. Weighing of the absorption vessels allowed measurement of carbon dioxide produced.
The major development in the indirect measurement of heat production was the development of portable systems. The Tissot spirometer (1904) and the Douglas bag (1911) collected all expired air for analysis and these were limited by the collection vessel size. Therefore, mask methods were developed by (11, 12), and (13) that involved connecting the subject to a static device for measuring change in gas volume. In 1952, Müller and Franz developed an open circuit mask system that could be carried in a bag (10). Since then, the development of indirect calorimetry has allowed its application in the sports and medicine fields.
Indirect calorimetry methods for energy expenditure measurements are based on the following assumptions (10, 14):
1- Any fuel consumed has an intrinsic energy content that upon metabolic modifications in the living system will result in heat or energy production.
2- The combustion or synthesis of carbohydrate, fat, or protein is the end result of all the biochemical reactions occurring in the body.
3- The oxidation of glucose, fat, or protein results in a substance-specific fixed ratio between the quantities of O2 consumed and CO2 produced.
4- Loss of substrates is negligible in feces and urine.
The second assumption overlooks the metabolism of minerals that account for 7% of total bodyweight, and the third assumption entails that fat and protein have uniform properties. Notwithstanding the limitations of those assumptions, indirect calorimetry has been found to be consistent and in close agreement with direct calorimetry (10). The methods for estimating the calorific factors and for calculating heat production were developed during the late eighteenth and early Nineteenth centuries and are now mainly of historical and educational interest (15). Newer methods based on algebraic analysis of the calculation procedures are shorter, simpler, and more versatile (10, 16). In open-circuit systems, the following is measured: ventilation rate and the composition of inlet and outlet air, then O2 consumption (O2), respiratory quotient (RQ), and metabolic rate () are computed.
Gas flowing into the system has a total air flow of VI that has a fraction of inspired O2 (FiO2) and a fraction of inspired CO2 (FiCO2), whereas gas flowing out has a total air flow of VE with an FeO2 and FeCO2. Additionally, inspired air has nitrogen therefore
FiN2 = 1 – FiO2 – FiCO2. In the presence of steady state conditions, defined as when sufficient time has elapsed for the outlet gas concentrations to equilibrate with the levels of gas exchange by the subject, the quantity of O2 consumed is then VO2 = VI x FiO2 – VE x FeO2. Since it is technically difficult to measure the small difference in volumes of inspiratory and expiratory air, VI is usually calculated using the Haldane transformation that assumes nitrogen is equal in inspired and expired gas (17, 18), therefore VI x FiN2 = VE x FeN2
As for heat production and the formula used by current indirect calorimeters for energy expenditure calculation, Weir showed that (19):
(kcal/min) = [3.941 x O2 (liters/ min)] + [1.106 x CO2 (liters/min)] – [2.17 x urinary nitrogen (g/day)]
(kcal/min) * 1440 = (kcal/day).
Indirect calorimetry systems estimate respiratory gas exchange as a surrogate for substrates consumed and produced during metabolism. This is done by one of 4 methods: confinement, closed-circuit, total collection, and open-circuit approaches.
1- Confinement systems: The rates of change of gas concentrations in a fixed volume is measured for a subject held in a sealed chamber. The limitation is that the measurement has to be restricted to a short period before O2 depletion occurs (10).
2- Closed-circuit systems: The subject is placed in a closed space with CO2 and moisture absorbers, and the quantity of O2 used up by the subject is measured. In most versions, only O2 consumption is measured (10). The major advantage of this system is that it can be used in patients with high FiO2 needs since Haldane transformation is not used (20). The major limitations are the equipment size and poor portability. Closed-circuits could lead to reduction of alveolar ventilation due to increased compressibility of the breathing circuit and may result in increased work of breathing (21, 22).
3- Total collection systems: All expired gas by a subject is collected and its volume and chemical composition are measured. Examples of this system is the Douglas bag, which is considered a reference standard in measurement of gas exchange. Limitations are the size of collection bag required and the potential of gas leak from the collection system(23).
4- Open circuit systems: The open-circuit chamber method was one of the earliest types of calorimeters and the one used now (10). The subject breathes from the atmosphere and expires into a separate outlet or the subject inspires and expires to a stream of passing air. In both, the flow of air is measured either on the inlet or outlet side of the subject and is either collected periodically or sampled continuously for analysis of gases.
The most commonly used method for gas analysis are the paramagnetic or fuel cell O2 sensor and the infrared CO2 analyzer (10). This system should not be used in patients requiring FiO2 > 0.8 since they utilize the Haldane transformation for VO2 calculation, patients with unstable FiO2 within a breath or between breaths, patients with non-reversible endotracheal tube leaks, air leak, or extracorporeal CO2 removal (ECMO or dialysis) due to CO2 loss that can't be measured, and small patient size (machine variable, lower limit ranges from 5 to 10 kg). All the above affect the applicability of different devices in the pediatric critically ill population (20, 21, 24) (Table 1).
Gas and flowrate measurements are essential components for accurate indirect calorimetry measurements. The most commonly used gas analyzers are paramagnetic O2 analyzers, galvanic O2 sensors, and infra-red CO2 analyzers (10, 25). While measurement of flow rate can be achieved either by the measurement of the volume of gas expired over a period of time or by the integration of a continuous measurement of rate of flow (Pneumotachometers) by 4 different forms: pressure-differential, turbines, pitot tubes, and hot-wire anemometers (26).
Accurate calibration of gas analyzers is one of the most essential requirements in calorimetry. If an evaluation of the full energy balance of an individual subject, or the metabolic response to some applied experimental treatment is required, then energy production must be measured with the greatest possible accuracy and precision. Additionally, it is important both in calibration and in subsequent measurements that gases entering the analyzers are always conditioned to the same fixed levels of humidity, pressure, flow rate and temperature (25, 26).
Accepted standard for levels of precision and accuracy for indirect calorimetry systems are not available. Some authors have suggested that the overall system is dependent on the levels of accuracy and precision of the volume and gas sensors, and to the precision and accuracy of the VO2 measurement(27–31). An acceptable level of accuracy for VO2 measurement is considered ±4–10% (32, 33).
There is inconsistency in the criteria deciding comparability between a reference technique and new devices for energy expenditure measurements. Other fields in medicine have developed acceptable limits of agreement between devices (34, 35). The most commonly utilized systems in pediatric critical care are summarized below.
1- Deltatrac II: This open-circuit calorimeter has two chambers. The first collects the expiratory gas, that is then sampled and the FeO2 and FeCO2 analyzed using paramagnetic and infrared analyzers, respectively (36). Next, the expired gas is passed at a constant flow rate (Q) through an air dilution chamber, sampled, and the fraction of CO2 is analyzed allowing calculation of the volume of CO2 expired: VCO2 = FeCO2 x Q and VO2 is calculated through the Haldane transformation14. Thus, the minute ventilation of the patient is not directly measured and all gases released at the expiratory port are sampled. The Deltatrac is not affected by the ventilator's bias flow since it lacks a flow measurement technique (17, 34).
2- Ultima CCM Express: The CCM Express measures gas exchange through a breath-by-breath technique. This is achieved by the utilizing a pneumotach flowmeter directly connected to the endotracheal tube and gas is collected through a sampling line in the flowmeter. A galvanic fuel cell and an infrared analyzer are utilized for the O2 and CO2 measurement, respectively. The bias flow provided by the ventilator does not affect measurements since ventilation is measured at the endotracheal tube (37).
3- Vmax Series: The Vmax measures mixed expired gas on a breath by breath basis. The O2 sensor is an electrochemical fuel cell, and CO2 sensor is an infrared one. It utilizes a mass flow sensor connected to exhaust port of the ventilator.
Deltatrac is considered the reference standard after validation studies demonstrated low bias and good precision in comparison to Douglas bag (38). This is the first device that allowed the minute to minute data analysis but has now been discontinued from commercial sale. Takala, Levinson, and colleagues showed that VO2 obtained from Deltatrac was consistently slightly higher than those obtained by pulmonary artery catheter or mass spectrometry, however accurate for energy expenditure measurements (18, 39). The VO2 discrepancy could be partially related to the lung O2 consumption that is not measured by thermodilution (34). Sundström and colleagues compared the Ultima CCM Express to Deltatrac II in mechanically ventilated adult patients and found CCM Express produced 64% higher mean REE values than Deltatrac(37). The VCO2 was in particular higher leading to errors in the RQ. No validity studies utilizing the Vmax system were identified in mechanically ventilated patient, however in healthy adults, the Vmax has been shown to have acceptable validity in comparison to the Deltatrac, with limits of agreement of 5 – 10%(40).
Total energy expenditure is a composite of resting energy expenditure (REE) that forms most of the total energy expenditure in critically ill children, thermic effect of feeding (TEF) and activity related energy expenditure (AEE) (41). By convention, indirect calorimetry is used to assess REE while certain conditions are met to mitigate the effect of TEF and AEE. TEF is greater with bolus feeds and less with continuous feeding (2, 3). AEE is lessened by ensuring a resting state for 2 h prior to the measurement (42, 43).
Some reports suggest that energy expenditure measurements should be performed in quiet rooms and mild lighting, but the effect of noise has not been studied (44). Room temperature has been demonstrated to affect energy expenditure in healthy adults (45–47). For accurate resting energy expenditure measurement, attention must be given to ensure steady-state conditions. Steady state is defined by the degree of variation in VO2 and VCO2 over a set time period. In mechanically ventilated patients, 5-min measurements with 5% coefficient of variation can be equivalent to 30- min measurements with 10% coefficient of variation and both are considered acceptable representations of a steady state (48–50). As for the variability of the energy expenditure measurement during a 24-h period, studies have demonstrated that in critically ill adults, short durations of monitoring are representative of the 24-h and the lack of significant variability between night or daytime measurements (51, 52).
Prior to the start of the measurement, the indirect calorimeter should be calibrated, minimal ventilator circuit or endotracheal tube leaks ensured, FiO2 < 80% and inspired tidal volumes larger than the lower limit set by the manufacturer should be confirmed (Table 1) (14). The patient should be at rest, with last endotracheal tube suction done at least 20 min before the measurement, no ventilatory changes, and minimal change in medications administered for the hour prior. Continuous enteral and parenteral nutrition should be continued. If clinician is interested in TEF in addition to REE, then bolus fed patients should be measured within 1-h of last bolus, otherwise the measurement should occur > 5-h after a feed. The operator should review the results as they are being obtained in order to address measurement issues prior to completion of the study (14, 42, 53, 54).
Results obtained will include measures of O2, CO2, RQ, REE, and a coefficient of variation of O2. Normative values for O2 and CO2 are reported to be 120 ml/min/m2 and 100 ml/min/m2 respectively and REE of 25–40 kcal/kg/day (55). An acceptable level of coefficient of variation is < 10% for a 30-min measurement. RQ is the ratio of CO2 to O2 and used as a marker for substrate utilization. Under standard metabolic conditions with stable respiratory function, the range of RQ in humans is ~0.7–1, with 0.7 representing predominantly fat utilization, 0.8 for mixed diet, and 1 for carbohydrates (20, 53, 56, 57). A summary of causes of altered results are summarized in Table 2.
All critically ill patients are susceptible to under and over nutrition, hence measurement of energy expenditure and the titration of intake based on the results is recommended (5). However, indirect calorimetry has suffered from limited spread that could be related to the high monetary cost to purchase and maintain the equipment, and the time cost to perform the measurement (58). This has led nutritional societies to develop recommendations for certain patient populations where measurement of energy expenditure should be performed: (a) clinical conditions that significantly alter REE; (b) when patients fail to respond to presumed adequate nutrition support; and (c) in order to individualize the nutrition support in the ICU. More particular examples include patients with altered body composition, continued weight loss in face of “adequate” nutrition, persistent inflammatory state (for example severe burns, trauma, prolonged septic states), and difficulty in mechanical ventilation weaning (5, 58, 58–60). This recommendation aims to assist clinicians in prioritizing a scarce resource, however is inconsistent with the statements of both ASPEN and ESPEN that indirect calorimetry is the reference standard for energy expenditure measurement (5, 59). Energy expenditure measurement has been shown to affect patient management, particularly resulting in a change in the nutritional prescription in 75–80% of patients in two recent studies, but how this impacts patients' outcomes remains unclear (61, 62). Moreover, no prospective pediatric trials have been conducted to compare the effect of titrated nutritional delivery to energy expenditure and its effects on outcome. In the latest and largest pediatric randomized trial in nutrition (PePANIC), formulas were used to estimate caloric needs of patients, therefore the effects of their intervention is difficult to interpret since the patient population could have been under- or over-fed (63).
Predictive equations that estimate energy expenditure have been developed and are used when access to indirect calorimetry is not available. Those developed to estimate energy expenditure in healthy children and extrapolated to use in the critically ill have been reported to be inaccurate in different populations, and their detailed review is out of the scope of this manuscript (6, 64–67). However, two equations have been derived for use in critically ill children: White and Meyer (68, 69). A recent validation study compared energy expenditure predicted by those two equations to measured energy expenditure by indirect calorimetry in a patient population similar to the one the equations were derived from and found errors of −20 to +50% in both (70). This suggests that clinical use is associated with significant errors in estimation of energy requirements in critically ill children. This inaccuracy could be related to: limitations of equation development methods/techniques, narrow range of children studied (disease/conditions/treatments), evaluation of modest numbers of children in datasets used for initial development and validation, and the numbers studied of the above with reduction in power to exclude important effects.
A 16-year-old male weighing 90 kg, admitted after a subarachnoid hemorrhage. He was mechanically ventilated, pain controlled with a morphine infusion, sedated with intermittent diazepam, and muscle relaxed with a cisatracurium infusion. Patient had an external ventricular drain for intracranial pressure monitoring and cerebrospinal fluid drainage. An indirect calorimetry measurement was performed on day 4 after admission. The patient was normothermic, with no seizures on electroencephalogram. He was on continuous feeds with a nasogastric tube with total caloric intake of 1,500 Kcal/day and protein of 1.5 g/kg/day.
The patient had an endotracheal tube (ETT) suction done 1 h ago, and he had no ETT leak. The coefficient of variation in the first 5 min was 4%. The measurement was made over a period of 30 min. At the conclusion of the test, the results were: VO2 = 0.49 L/min, VCO2 = 0.35 L/min, RQ = 0.71, measured REE = 3336 Kcal/day.
1- Was the measurement performed at an appropriate time based on the data provided? Yes, the patient was continuously fed, on stable doses of medication, afebrile, had no seizures, and no ETT leak.
2- Can this measurement be accepted as a reliable one? Yes, the coefficient of variation is within accepted limits.
3- What does an RQ of 0.71 represent? This is an RQ within the acceptable normal range for substrate utilization and suggests predominantly fat utilization. This RQ indicates that the patient might require additional caloric intake in the form of carbohydrates to mitigate the lipid metabolism. Causes for abnormally low or high RQ are in Table 2.
4- What does an REE of 3336 Kcal/day mean? There are no reported normal REE ranges according to age or body size in critically ill children (5). Adult guidelines suggest that 25–30 Kcal/kg/day is an acceptable energy target (71). Our patient's REE is 37 Kcal/kg/day and the clinician should adjust the nutritional prescription to provide the current REE due to the significant morbidities associated with both under- and over-feeding (63, 72–80). REE does not provide the clinician with insight in regards to protein turnover and needs, a 24 h urinary nitrogen would be helpful in that case. An acceptable rule of thumb is a protein intake of 1.5–2 g/kg/day based on adult and pediatric nutrition guidelines (5, 71).
Technologic advancement has allowed for the portable measurement of energy expenditure at the bedside via indirect calorimeters. Recently, the incorporation of energy expenditure measurement modules into ventilators has expanded the reach and utility of this tool, but clinicians need to be aware that these new generation ventilators have not been validated against existing reference standards for energy expenditure measurement in critically ill children. The nutritional community must perform validation studies prior to incorporating those measurements into daily practice. Future prospects could include the return of Douglas bag measurements in critically ill patients since these were considered “simple” to use with a lower monetary and time cost. Additional opportunities that have not been explored in this field include utilization of the electronic medical record data and inclusion of artificial intelligence algorithms that would generate energy expenditure as a continuous vital sign. Prior to the development of algorithms though, well designed studies that attempt to determine the effect of different clinical factors and interventions on energy expenditure are required.
Indirect calorimetry is the reference standard for measurement of energy expenditure in the critically ill. The technology has limitations that should be understood by the clinician performing and interpreting the measurement. Current equations derived for use in critically ill children are not valid and their use should be avoided. The large discrepancies between the estimations and measurement of energy expenditure influences the nutritional management and could impact the outcomes of critically ill children.
All authors listed have made a substantial, direct, and intellectual contribution to the work and approved it for publication.
This paper received no specific grant from any funding agency in the public, commercial, or not-for-profit sectors. The corresponding author declares an award from Canadian Institute of Health Research, Frederick Banting and Charles Best Canada Graduate Scholarship-Master's (CGS-M) to pursue graduate studies (Restracomp award, Research Institute, Hospital for Sick Children, Toronto).
The authors declare that the research was conducted in the absence of any commercial or financial relationships that could be construed as a potential conflict of interest.
1. Wilson DF. Regulation of cellular metabolism: programming and maintaining metabolic homeostasis. J Appl Physiol. (2013):15:1583–8. doi: 10.1152/japplphysiol.00894.2013
2. Weekes CE. Controversies in the determination of energy requirements. Proc Nutr Soc. (2007) 66:367–77. doi: 10.1017/S0029665107005630
3. Lowell BB, Spiegelman BM. Towards a molecular understanding of adaptive thermogenesis. Nature (2000) 404:652–60. doi: 10.1038/35007527
4. Kenny GP, Notley SR, Gagnon D. Direct calorimetry: a brief historical review of its use in the study of human metabolism and thermoregulation. Eur J Appl Physiol. (2017) 117:1765–85. doi: 10.1007/s00421-017-3670-5
5. Mehta NM, Skillman HE, Irving SY, Coss-Bu JA, Vermilyea S, Farrington EA, et al. Guidelines for the provision and assessment of nutrition support therapy in the pediatric critically ill patient: society of critical care medicine and american society for parenteral and enteral nutrition. Pediatr Crit Care Med. (2017) 18:675–715. doi: 10.1177/0148607117711387
6. Mtaweh H, Smith R, Kochanek PM, Wisniewski SR, Fabio A, Vavilala MS et al. Energy expenditure in children after severe traumatic brain injury. Pediatr Crit Care Med. (2014) 15:242–9. doi: 10.1097/PCC.0000000000000041
7. Turi RA, Petros AJ, Eaton S, Fasoli L, Powis M, Basu R, et al. Energy metabolism of infants and children with systemic inflammatory response syndrome and sepsis. Annal Surgery (2001) 233:581–7.
8. Goran MI, Broemeling L, Herndon DN, Peters EJ, Wolfe RR. Estimating energy requirements in burned children: a new approach derived from measurements of resting energy expenditure. Am J Clin Nutr. (1991) 54:35–40.
9. Poncet S, Dahlberg L. The legacy of henri victor regnault in the arts and sciences. Intl J Arts Sci. (2011) 4:377–400. Available online at: https://hal.archives-ouvertes.fr/hal-00678894/document
10. McLean JA, Tobin G. Animal and Human Calorimetry. New York, NY: Cambridge University Press. (1987).
11. Krogh A. Methods for measuring the respiratory exchange. In: The Respiratory Exchange of Animals and Man. London: Longmans Green & Co. (1916). p. 14–51.
12. Benedict FG, Collins WE. A Clinical apparatus for measuring basal metabolism. Boston Med Surg J. (1920) 183:449–58.
13. Roth P. Modifications of apparatus and improved technic adaptable type of respiration apparatus. III. Graphic method for the estimation of the metabolic rate. Boston Med Surg J. (1922) 186:491–8.
14. Porter C, Cohen NH. Indirect calorimetry in critically ill patients: role of the clinical dietitian in interpreting results. J Am Diet Assoc. (1996) 96:49–57.
15. Lusk G. The Elements of the Science of Nutrition. 4th ed. Philadelphia, PA; Saunders company. (1928).
16. Abramson E. Computation of Results from Experiments with Indirect Calorimetry. Acta Physiol Scand. (1943) 6:1–19. doi: 10.1111/j.1748-1716.1943.tb02822.x
17. Haugen AH, Chan LN, Li F. Indirect calorimetry: a practical guide for clinicians. Nutr Clin Pract. (2007) 22:377–88. doi: 10.1177/0115426507022004377
18. Takala J, Keinanen O, Vaisanen P, Kari A. Measurement of gas exchange in intensive care: laboratory and clinical validation of a new device. Crit Care Med. (1989) 17:1041–7.
19. Weir JB. New methods for calculating metabolic rate with special reference to protein metabolism. J Physiol. (1949) 109:1–9.
21. Branson RD, Johannigman JA. The measurement of energy expenditure. Nutr Clin Pract. (2004) 19:622–36. doi: 10.1093/jn/121.11.1897
22. Keppler T, Dechert RE, Arnoldi DK, Filius R, Bartlett RH. Evaluations of the Waters MRM-6000 and Biergy VVR closed-circuit indirect calorimeters. Resp Care (1989) 34:28–35.
23. Douglas CG. A method for determining the total respiratory exchange in man. J Physiol. (1911) 42:17–23.
24. Browning JA, Linberg SE, Turney SZ, Chodoff P. The effects of a fluctuating Fio2 on metabolic measurements in mechanically ventilated patients. Crit Care Med. (1982) 10:82–5.
25. Gilder H, Cornell GN, Thorbjarnarson B. Human energy expenditure in starvation estimated by expired-air analysis. J Appl Physiol. (1967) 23:297–303.
26. Macfarlane DJ. Automated metabolic gas analysis systems: a review. Sports Med. (2001) 31:841–61. doi: 10.2165/00007256-200131120-00002
27. Davis JA. Direct determination of aerobic power. In: Maud PJ, Foster C, editors. Physiological Assessment of Human Fitness. Champaign, IL: Human Kinetics (1995). p.9–17.
28. Withers RT, Gore CJ, Gass G. Determination of maximal oxygen consumption (VO2max) or maximal aerobic power. In: Gore CJ, editor. Physiological Testing for Elite Athletes (Australian Sports Commission). Champaign, IL: Human Kinetics (2000). p. 114–27.
29. Society AT. Standardization of Spirometry, 1994 Update. Am J Resp Crit Care Med. (1995) 152:1107–36.
31. Thoden JS. Testing aerobic power. In: MacDougall JD, Wenger HA, Green HJ, editors. Canadian Association of Sport Sciences. Physiological Testing of the High-Performance Athlete. 2nd ed. Champaign, IL: Human Kinetics Books (1991). p. 107–73.
32. Wagner JA, Horvath SM, Dahms TE, Reed S. Validation of open-circuit method for the determination of oxygen consumption. J Appl Physiol. (1973) 34:859–63.
33. Davies EE, Hahn HL, Spiro SG, Edwards RH. A new technique for recording respiratory transients at the start of exercise. Respir Physiol. (1974) 20:69–79.
34. Black C, Grocott MP, Singer M. Metabolic monitoring in the intensive care unit: a comparison of the Medgraphics Ultima, Deltatrac II, and Douglas bag collection methods. Br J Anaesth. (2015) 114:261–8. doi: 10.1093/bja/aeu365
35. Critchley LA, Critchley JA. A meta-analysis of studies using bias and precision statistics to compare cardiac output measurement techniques. J Clin Monit Comput. (1999) 15:85–91.
37. Sundstrom M, Tjader I, Rooyackers O, Wernerman J. Indirect calorimetry in mechanically ventilated patients. A systematic comparison of three instruments. Clin Nutr. (2013) 32:118–21. doi: 10.1016/j.clnu.2012.06.004
38. Tissot S, Delafosse B, Bertrand O, Bouffard Y, Viale JP, Annat G. Clinical validation of the Deltatrac monitoring system in mechanically ventilated patients. Intensive Care Med. (1995) 21:149–53.
39. Levinson MR, Groeger JS, Miodownik S, Ray C, Brennan MF. Indirect calorimetry in the mechanically ventilated patient. Crit Care Med. (1987) 15:144–7.
40. Cooper JA, Watras AC, O'Brien MJ, Luke A, Dobratz JR, Earthman CP, et al. Assessing validity and reliability of resting metabolic rate in six gas analysis systems. J Am Diet Assoc. (2009) 109:128–32. doi: 10.1016/j.jada.2008.10.004
41. Wiskin AE, Davies JH, Wootton SA, Beattie RM. Energy expenditure, nutrition and growth. Arch Dis Child. (2011) 96:567–72. doi: 10.1136/adc.2009.158303
42. Compher C, Frankenfield D, Keim N, Roth-Yousey L. Best practice methods to apply to measurement of resting metabolic rate in adults: a systematic review. J Am Diet Assoc. (2006) 106:881–903. doi: 10.1016/j.jada.2006.02.009
43. Hickmann CE, Roeseler J, Castanares-Zapatero D, Herrera EI, Mongodin A, Laterre PF. Energy expenditure in the critically ill performing early physical therapy. Intensive Care Med. (2014) 40:548–55. doi: 10.1007/s00134-014-3218-7
44. McClave SA, Martindale RG, Kiraly L. The use of indirect calorimetry in the intensive care unit. Curr Opin Clin Nutr Metab Care (2013) 16:202–8. doi: 10.1097/MCO.0b013e32835dbc54
45. Ueno S, Ikeda K, Tai T. Metabolic rate prediction in young and old men by heart rate, ambient temperature, weight and body fat percentage. J Occup Health (2014) 56:519–25. doi: 10.1539/joh.14-0063-BR
46. Chen KY, Brychta RJ, Linderman JD, Smith S, Courville A, Dieckmann W, et al. Brown fat activation mediates cold-induced thermogenesis in adult humans in response to a mild decrease in ambient temperature. J Clin Endocrinol Metab. (2013) 98:E1218–23. doi: 10.1210/jc.2012-4213
47. van Marken Lichtenbelt WD, Frijns AJ, van Ooijen MJ, Fiala D, Kester AM, van Steenhoven AA. Validation of an individualised model of human thermoregulation for predicting responses to cold air. Int J Biometeorol. (2007) 51:169–79. doi: 10.1007/s00484-006-0060-9
48. McClave SA, Spain DA, Skolnick JL, Lowen CC, Kieber MJ, Wickerham PS, et al. Achievement of steady state optimizes results when performing indirect calorimetry. J Parent Enteral Nutr. (2003) 27:16–20. doi: 10.1177/014860710302700116
49. Cunningham KF, Aeberhardt LE, Wiggs BR, Phang PT. Appropriate interpretation of indirect calorimetry for determining energy expenditure of patients in intensive care units. Am J Surg. (1994) 167:547–9. doi: 10.1016/0002-9610(94)90255-0
51. Frankenfield DC, Wiles CE III, Bagley S, Siegel JH. Relationships between resting and total energy expenditure in injured and septic patients. Crit Care Med. (1994) 22:1796–804.
52. van Lanschot JJ, Feenstra BW, Vermeij CG, Bruining HA. Accuracy of intermittent metabolic gas exchange recordings extrapolated for diurnal variation. Crit Care Med. (1988) 16:737–42.
53. da Rocha EE, Alves VG, da Fonseca RB. Indirect calorimetry: methodology, instruments and clinical application. Curr Opin Clin Nutr Metab Care (2006) 9:247–56. doi: 10.1097/01.mco.0000222107.15548.f5
54. Wooley JA. Indirect calorimetry: applications in practice. Respir Care Clin N Am. (2006) 12:619–33. doi: 10.1016/j.rcc.2006.09.005
55. Bartlett RH. Physiology of extracorporeal llife support. In: Annich GM, Lynch WR, MacLaren G, Wilson JM, Bartlett RH, editors. Extracorporeal Cardiopulmonary Support in Critical Care. 4th ed. Ann Arbor, MI: ELSO (2012). p. 11–31.
56. Jequier E, Acheson K, Schutz Y. Assessment of energy expenditure and fuel utilization in man. Annu Rev Nutr. (1987) 7:187–208. doi: 10.1146/annurev.nu.07.070187.001155
57. McClave SA, Lowen CC, Kleber MJ, McConnell JW, Jung LY, Goldsmith LJ. Clinical use of the respiratory quotient obtained from indirect calorimetry. J Parent Enter Nutr. (2003) 27:21–6. doi: 10.1177/014860710302700121
58. van der Kuip M, Oosterveld MJ, van Bokhorst-de van der Schueren MA, de Meer K, Lafeber HN, Gemke RJ. Nutritional support in 111 pediatric intensive care units: a European survey. Intensive Care Med. (2004) 30:1807–13. doi: 10.1007/s00134-004-2356-8
59. Kreymann KG, Berger MM, Deutz NE, Hiesmayr M, Jolliet P, Kazandjiev G, et al. ESPEN Guidelines on enteral nutrition: intensive care. Clin Nutr. (2006) 25:210–23. doi: 10.1016/j.clnu.2006.01.021
60. Mehta NM, Compher C, Directors ASPENBo. A.S.P.E.N. Clinical guidelines: nutrition support of the critically ill child. J Parent Enter Nutr. (2009) 33:260–76. doi: 10.1177/0148607109333114
61. Ladd AK, Skillman HE, Haemer MA, Mourani PM. Preventing underfeeding and overfeeding: a clinician's guide to the acquisition and implementation of indirect calorimetry. Nutr Clin Pract. (2017) 198–205. doi: 10.1177/0884533617710214
62. Larsen BMK, Beggs MR, Leong AY, Kang SH, Persad R, Garcia Guerra G. Can energy intake alter clinical and hospital outcomes in PICU? Clin Nutr ESPEN (2018):41–6. doi: 10.1016/j.clnesp.2018.02.002
63. Fivez T, Kerklaan D, Verbruggen S, Vanhorebeek I, Verstraete S, Tibboel D, et al. Impact of withholding early parenteral nutrition completing enteral nutrition in pediatric critically ill patients (PEPaNIC trial): study protocol for a randomized controlled trial. Trials (2015) 16:202. doi: 10.1186%2Fs13063-015-0728-8
64. Briassoulis G, Venkataraman S, Thompson AE. Energy expenditure in critically ill children. Critical Care Med. (2000) 28:1166–72.
65. Hardy CM, Dwyer J, Snelling LK, Dallal GE, Adelson JW. Pitfalls in predicting resting energy requirements in critically ill children: a comparison of predictive methods to indirect calorimetry. Nutr Clin Prac. (2002) 17:182–9. doi: 10.1177/0115426502017003182
66. Vazquez Martinez JL, Martinez-Romillo PD, Diez Sebastian J, Ruza Tarrio F. Predicted versus measured energy expenditure by continuous, online indirect calorimetry in ventilated, critically ill children during the early postinjury period. Pediatr Crit Care Med. (2004) 5:19–27. doi: 10.1097/01.PCC.0000102224.98095.0A
67. Framson CM, LeLeiko NS, Dallal GE, Roubenoff R, Snelling LK, Dwyer JT. Energy expenditure in critically ill children. Pediatr Crit Care Med. (2007) 8:264–7. doi: 10.1097/01.PCC.0000262802.81164.03
68. White MS, Shepherd RW, McEniery JA. Energy expenditure in 100 ventilated, critically ill children: improving the accuracy of predictive equations. Crit Care Med. (2000) 28:2307–12.
69. Meyer R, Kulinskaya E, Briassoulis G, Taylor RM, Cooper M, Pathan N, et al. The challenge of developing a new predictive formula to estimate energy requirements in ventilated critically ill children. Nutr Clin Pract. (2012) 27:669–76. doi: 10.1177/0884533612448479
70. Jotterand Chaparro C, Taffe P, Moullet C, Laure Depeyre J, Longchamp D, Perez MH, et al. Performance of predictive equations specifically developed to estimate resting energy expenditure in ventilated critically Ill children. J Pediatr. (2017) 184:220–6.e5. doi: 10.1016/j.jpeds.2016.12.063
71. McClave SA, Taylor BE, Martindale RG, McCarthy M, Roberts P, Taylor B, et al. Guidelines for the provision and assessment of nutrition support therapy in the adult critically Ill patient: Society of Critical Care Medicine (SCCM) and American Society for Parenteral and Enteral Nutrition (A.S.P.E.N.). JPEN J Parent Enter Nutr. (2016) 40:159–211. doi: 10.1177/0148607109335234
72. Arabi YM, Aldawood AS, Haddad SH, Al-Dorzi HM, Tamim HM, Samir HH, et al. Permissive Underfeeding or Standard Enteral Feeding in Critically Ill Adults. N Engl J Med. (2015) 372:2398–408. doi: 10.1164/rccm.201605-1012OC
73. Singer P, Anbar R, Cohen J, Shapiro H, Shalita-Chesner M, Lev S, et al. The tight calorie control study (TICACOS): a prospective, randomized, controlled pilot study of nutritional support in critically ill patients. Intensive Care Med. (2011) 37:601–9. doi: 10.1007/s00134-011-2146-z
74. Casaer MP, Hermans G, Wilmer A, Van den Berghe G. Impact of early parenteral nutrition completing enteral nutrition in adult critically ill patients (EPaNIC trial): a study protocol and statistical analysis plan for a randomized controlled trial. Trials (2011) 12:21. doi: 10.1186/1745-6215-12-21
75. Mehta NM, Duggan CP. Nutritional deficiencies during critical illness. Pediatr Clin North Am. (2009) 56:1143–60. doi: 10.1016%2Fj.pcl.2009.06.007
76. Joffe A, Anton N, Lequier L, Vandermeer B, Tjosvold L, Larsen B, et al. Nutritional support for critically ill children. Cochrane database Syst Rev. (2009) 27:CD005144. doi: 10.1002/14651858.CD005144.pub3
77. Yeh DD, Fuentes E, Quraishi SA, Cropano C, Kaafarani H, Lee J, et al. Adequate nutrition may get you home: effect of caloric/protein deficits on the discharge destination of critically Ill surgical patients. J Parent Enter Nutr. (2015) 40:37–44. doi: 10.1177/0148607115585142
78. McClave S, Codner P, Patel J, Hurt R, Allen K, Martindale R. Should we aim for full enteral feeding in the first week of critical illness? Nutr Clin Pract. (2016) 31:425–31. doi: 10.1177/0884533616653809
Keywords: calorimetry, indirect calorimetry, energy expenditure, closed-circuit calorimetry, open-circuit calorimetry, critically ill children, energy metabolism
Citation: Mtaweh H, Tuira L, Floh AA and Parshuram CS (2018) Indirect Calorimetry: History, Technology, and Application. Front. Pediatr. 6:257. doi: 10.3389/fped.2018.00257
Received: 20 November 2017; Accepted: 28 August 2018;
Published: 19 September 2018.
Edited by:
Hitesh Singh Sandhu, University of Tennessee Health Science Center, United StatesReviewed by:
Ryan J. Stark, Vanderbilt University Medical Center, United StatesCopyright © 2018 Mtaweh, Tuira, Floh and Parshuram. This is an open-access article distributed under the terms of the Creative Commons Attribution License (CC BY). The use, distribution or reproduction in other forums is permitted, provided the original author(s) and the copyright owner(s) are credited and that the original publication in this journal is cited, in accordance with accepted academic practice. No use, distribution or reproduction is permitted which does not comply with these terms.
*Correspondence: Haifa Mtaweh, aGFpZmEubXRhd2VoQHNpY2traWRzLmNh
Disclaimer: All claims expressed in this article are solely those of the authors and do not necessarily represent those of their affiliated organizations, or those of the publisher, the editors and the reviewers. Any product that may be evaluated in this article or claim that may be made by its manufacturer is not guaranteed or endorsed by the publisher.
Research integrity at Frontiers
Learn more about the work of our research integrity team to safeguard the quality of each article we publish.