- 1Division of Pediatric Hematology/Oncology, Department of Pediatrics and Adolescent Medicine, Medical University of Graz, Graz, Austria
- 2Center for Medical Research (ZMF), Core Facility Flow Cytometry, Medical University of Graz, Graz, Austria
- 3Department of Pediatric and Adolescent Surgery, Medical University of Graz, Graz, Austria
Objective: Primary infection with human herpes virus 6 (mainly HHV-6B) commonly occurs in the first 2 years of life leading to persistence and the possibility of virus reactivation later in life. Consequently, a specific cellular immune response is essential for effective control of virus reactivation. We have studied cell-mediated immune response to HHV-6 (U54) in healthy children and adolescents.
Materials and Methods: By flow cytometry, the amount of cytokine (interferon gamma—IFN- γ, interleukin 2—IL-2, tumor necrosis factor alpha—TNF-α) secreting T-cells were measured after 10 days of pre-sensitization and 6 h of re-stimulation with mixtures of pooled overlapping peptides from U54, staphylococcal enterotoxin B (SEB, positive control), or Actin (negative control) in healthy children and adolescents without any underlying immune disorder or infectious disease.
Results: All individuals showed a virus-specific response for at least one cytokine in either CD4+ or CD8+ cells. Percentages of individuals with HHV-6-specific TNF-α response in CD4+ (48% of individuals) as well as CD8+ (56% of individuals) were always the highest. Our data show significantly higher frequencies of HHV-6-specific TNF-α producing CD8+ T-cells in individuals older than 10 years of life (p = 0.033). Additionally, the frequency of HHV-6 specific TNF-α producing CD8+ T-cells positively correlated with the age of the individuals. Linear regression analysis showed a positive relation between age and frequency of HHV-6-specific TNF-α producing CD8+ T-cells.
Conclusion: Results indicate that T-cell immune response against HHV-6 is commonly detectable in healthy children and adolescents with higher frequencies of antigen-specific T-cells in older children and adolescents possibly reflecting repeated stimulation by viral persistence and subclinical reactivation.
Introduction
Human herpesvirus-6 (type HHV-6A) was isolated for the first time in 1986 from patients with lymphoproliferative disorders (1). The clinically more relevant species HHV-6B is known as a causative agent of exanthema subitum (2).
Primary infection with HHV-6B occurs within the first 2 years of life and is often associated with exanthema subitum (roseola) (3, 4). In most regions of the world, seroprevalence of HHV-6 ranges up to 100% (5). Like other herpes viruses, the virus persists in the host after primary infection (latent infection) and may reactivate under immunosuppression (6). In healthy individuals, HHV-6 reactivation usually occurs without significant symptoms, but in immunocompromised subjects such as solid organ or hematopoietic stem cell transplant recipients, reactivation can lead to CNS morbidity, pneumonitis, transplant rejection, or delayed engraftment (6, 7). In addition, an inherited chromosomal integration of HHV-6 DNA (ciHHV-6) into the human genome has been described leading to high DNA copy numbers by using quantitative PCR. Despite an estimated prevalence of 0.2–5%, the clinical impact of this phenomenon still remains unclear (8, 9).
Like other herpesviruses, HHV-6 has developed immunomodulatory strategies for its life-long persistence in the host's organism (10). HHV-6 replicates in various immune cell types, mainly in CD4 + T-cells and causes a suppression of the immune system (11). Despite various immune-invasive strategies, the healthy immune system is capable to control the virus reactivation during its life-long persistence.
For the closely related human cytomegalovirus (HCMV), the cellular response of CD4+ and CD8+ T-cells has been shown to be fundamental for the control of primary infection and reactivation (12, 13). The aim of this study was to describe HHV-6 specific T-cell immunity in healthy children and adolescents.
Materials and Methods
Clinical Samples
For this prospective, cross-sectional study, peripheral venous blood was drawn from children and adolescents without any inflammatory, immunological or infectious disease before elective surgery for orthopedic pathologies, implant removals, herniotomies, circumcisions, and others. This study was approved by the Institutional Review Board of the Medical University Graz and patients, parents or legal guardians of patients gave written informed consent in accordance with the Declaration of Helsinki.
Peripheral Blood Mononuclear Cells Isolation
Peripheral blood mononuclear cells (PBMCs) were isolated by Ficoll (Ficoll® Paque Plus, GE Healthcare, UK) density gradient centrifugation and were washed twice with DPBS (DPBS, Thermo Fisher Scientific, Carlsbad, California). Trypan Blue (STEMCELL Technologies, 0.4% (m/v), Cologne, Germany) staining was used for the discrimination between viable and non-viable cells and cell numbers were determined via Neubauer chamber.
Cell Culture and Stimulation Assays
Two different attempts for cell stimulation (overnight and 10 days stimulation) were performed: For the overnight stimulation, PBMCs were suspended in TexMACS medium (Miltenyi Biotec GmbH, Bergisch-Gladbach, Germany). Per well, 1.25*106 cells were suspended in 250 μl TexMACS medium in a 48-well plate and were stimulated with mixtures of overlapping 15-mer peptides that encompass the whole protein from U54 (transactivator protein) (PepMix U54, JPT, Berlin, Germany), pp65 (PepMix HCMVA pp65, JPT, Berlin, Germany), and Actin (PepMix Human Actin, JPT; negative control) at a final concentration of 10 μg/ml. On the next day, staphylococcal enterotoxin B (Sigma, Taufkirchen, Germany; positive control, SEB) was added at a final concentration of 2.5 μg/ml to an unstimulated well. Brefeldin A (Sigma, Taufkirchen, Germany) was added as a Golgi stop with a final concentration of 2.5 μg/ml to every well for the last 4 h of incubation before staining.
For 10 days stimulation, PBMCs were suspended in TexMACS medium (Miltenyi Biotec GmbH, Bergisch-Gladbach, Germany). 1.25*106 cells were suspended in wells of a 48-well plate and were stimulated with the same antigens as for the overnight stimulation except SEB and pp65 as described above. Cells then were expanded for 10 days in cell culture with interleukin-2 (IL-2; 10 U/mL; Cellgenix, Freiburg, Germany) and interleukin-7 (IL-7; 10 ng/mL; Cellgenix, Freiburg, Germany). Medium was changed every or every second day. On day 9 cytokines were washed out and on day 10 cells were restimulated for 6 h with the same antigens as on day 1 (U54 and Actin). In addition, a previously unstimulated well was stimulated with staphylococcal enterotoxin B (Sigma, Taufkirchen, Germany; positive control). Before staining, Brefeldin A (Sigma, Taufkirchen, Germany) was added to all wells as a Golgi stop for the last 4 h of incubation.
Flow Cytometry Staining
PBMCs were then surface-stained with the following monoclonal antibodies to: CD3 (PerCP, Bio Legend, San Diego, USA), CD4 (Alexa Flour 700, Becton Dickinson, San Diego, CA, USA), CD8 (APC-H7, Becton Dickinson, San Diego, CA, USA), CD56 (FITC Becton Dickinson, San Diego, CA, USA). Next, monoclonal antibodies were washed out and stained for live/dead discrimination with an aminereactive dye (Alexa Fluor 350 carboxylic acid, succinimidyl ester, Invitrogen, Carlsbad, CA, USA). After 10 min of incubation at room temperature, cells were fixed and permeabilized using a fix and perm kit® (An Der Grub Bio Research GmbH, Vienna, Austria). Afterwards, intracellular staining was conducted with antibodies against interferon-γ (IFN-γ, BV605 Becton Dickinson, San Diego, CA, USA), interleukin-2 (IL-2, BV510 Becton Dickinson, San Diego, CA, USA) and tumor necrosis factor-α (TNF-α, BV421 Becton Dickinson, San Diego, CA, USA). Measurements were carried out on a flow cytometer LSR II (Becton Dickinson, San Diego, CA, USA) and FACSDIVA acquisition/analysis software (Becton Dickinson, San Diego, CA, USA) was used for data analysis.
Statistical Analysis
The Kruskal-Wallis test and the Fisher's exact test were used to describe the differences in the antigen-specific responses between the two age groups (< 10 years and 10–18 years). Spearman rank correlation was used for describing the degree of association between age and frequency of HHV-6 specific T-cells. P-values < 0.05 were considered statistically significant. All statistical evaluations, linear regression, and correlation analyses were performed by SPSS version 23 for Windows (SPSS, Inc., Chicago, IL, USA).
To define the HHV-6 (U54) specific T- cell response, the following 2 criteria had to be fulfilled:
(I) The frequency of cytokine-secreting cells after U54 stimulation in the total population (CD4+ or CD8+ T-cells) was at least 0.1 percentage points higher than in unstimulated cells of the negative control.
(II) The frequency of cytokine-secreting cells after U54 stimulation was at least two times higher than in the negative control (see Figure 1).
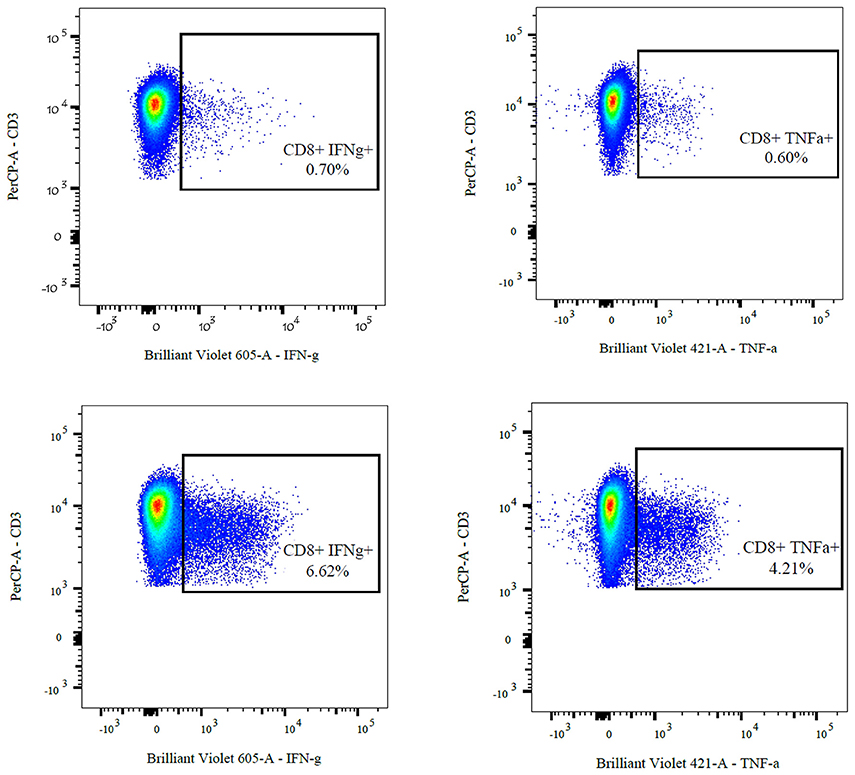
Figure 1. FACS analysis of cytokine-producing CD8 + T-cells of a 17-year-old adolescent after stimulation and 10 days of expansion in cell culture. The upper two images show the negative controls (ACTS) compared to the U54 stimulated cells (left: IFN-γ, right: TNF-α).
Results
Short Term Stimulation
The short overnight stimulation in three individuals (5.7, 8.3, and 9.8 years) with tegument phosphoprotein pp65 showed a CMV-specific immune response in all 3 patients (IFN- γ positive in 1.55, 1.59, and 3.21% of CD4+ T-cells and 1.91, 2.03, and 3.44% of CD8+ T-cells, IL-2 positive in 1.22, 1.71, and 3.41% of CD4+ T-cells and 1.0, 1.54, and 1.93 of CD8+ T-cells, TNF-α positive in 3.11, 3.61, and 3.75% of CD4+ T-cells and 2.6, 3.44, and 3.69 of CD8+ T-cells). In contrast, no HHV-6-specific immune response was detected after stimulation with U54 in none of the tested individuals. For this reason, the stimulation and expansion of the cells was carried out for 10 days in culture for further analyses.
Long Term Stimulation (10 Days)
In these, 25 healthy children and adolescents were included: 72% male, 28% female, median age 8.2 (range 3.1–18.3) years. All individuals showed an U54-specific response for at least one cytokine in either CD4+ or CD8+ T-cells. Twenty-four percentages of all individuals had a positive response in both cell types. Sixty-eight percentages of individuals had a CD8+ T-cell response for every cytokine. In Table 1, number (percentages) of individuals with U54-specific T-cell responses are shown for different cytokines and cell types.
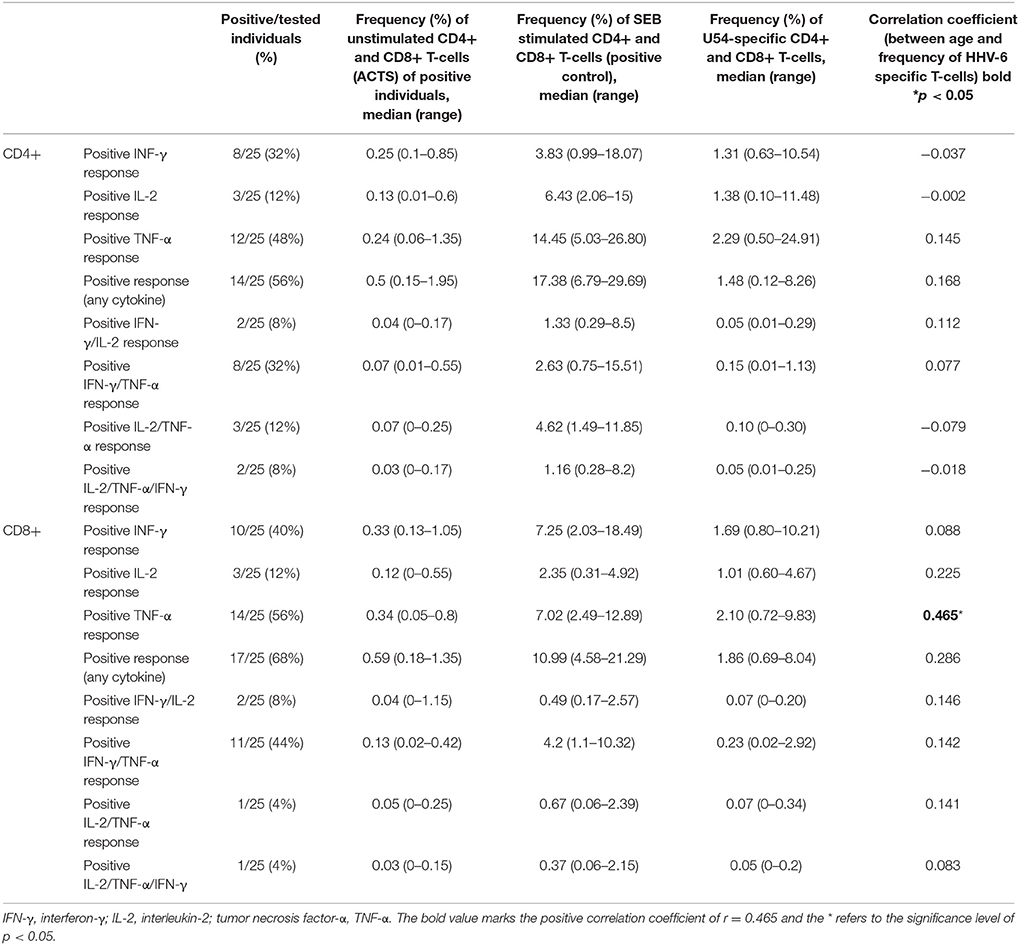
Table 1. Number (percentages) of individuals with U54-specific T-cell response, frequencies of CD4+ and CD8+ T-cells after stimulation with ACTS (negative control), frequencies of CD4+, and CD8+ T-cells after stimulation with SEB (positive control), frequencies of U54-specific CD4+, and CD8+ T-cells and correlation coefficients between age and frequency of HHV-6 specific T-cells (Spearman Rho).
Percentages of individuals with HHV-6-specific TNF-α response were the highest for CD4+ (48% of individuals) as well as for CD8+ (56% of individuals) and TNF-α secreting CD4+ cells were the most prominent HHV-6-specific cells. Noteworthy, only 12% of individuals showed a positive IL-2 response in both CD4+ and CD8+ cells, while IL-2 response was clearly positive after stimulation with SEB (positive control) proofing reliable intracellular staining with the used antibody (see Table 1).
Comparing the age groups (<10 years, n = 13 and 10–18 years, n = 12), significantly higher frequencies of HHV-6-specific TNF-α producing CD8+ T-cells in the older age group (p = 0.033) were detected [<10 years, median age 6.6 (range 3.1–9.2) and 10–18 years, median age 17.2 (range 10.5–18.3)] (see Figure 2).
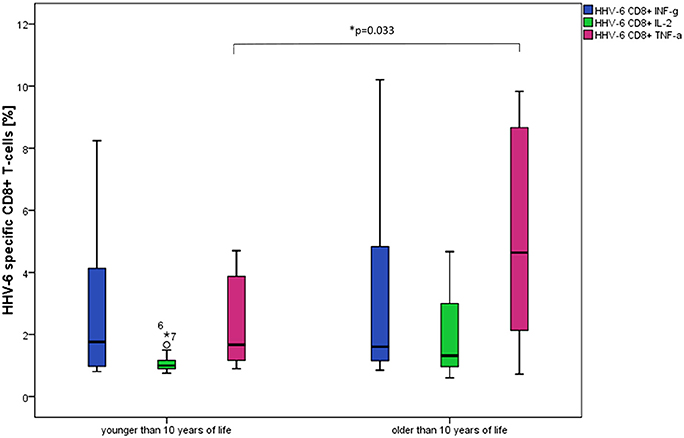
Figure 2. Box plots show significantly higher frequencies of TNF-α producing CD8+ T-cells in individuals older than 10 years after stimulation with HHV-6 specific antigen U54. *p = 0.033. Differences between the age groups were not statistically significant for the other cytokine patterns and CD4+ T-cells.
The frequency of TNF-α producing CD8+ T-cells positively correlated with the age of the individuals r = 0.465 (Spearman-Rho) on a two-sided significance level of p = 0.019 and linear regression analysis showed a positive relation between age and frequency of HHV-6-specific TNF-α producing CD8+ T-cells with a regression coefficient of r = 0.490 (see Figure 3). No positive correlations were found for the other cytokines.
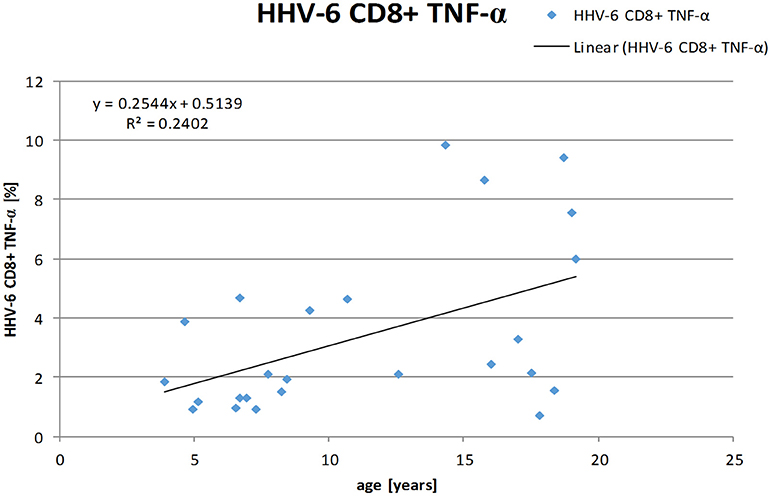
Figure 3. Linear regression analysis shows a positive correlation between age and frequencies of HHV-6-specific TNF-α producing CD8+ T-cells. (Spearman-Rho r = 0.490).
Discussion
After primary infection, human herpes viruses establish a lifelong latent infection in the host and persist in certain host cells. Latently infected cells may express virus-specific antigens on their cell surface (15, 17). However, to protect themselves from immuno-recognition, human herpes viruses have a limited viral gene expression during latency (13). Subsets of T-cells that have specific cytotoxic activity against virus-infected or transformed cells are crucial for the suppression of reactivation (16, 17).
We, therefore, analyzed secretion of IL-2, IFN-γ as well as TNF-α in both CD4+ and CD8+ T-cells after stimulation with the HHV-6 specific antigen U54 and demonstrated the presence of HHV-6-reactive CD4+ and CD8+ T-cells in the peripheral blood of healthy children and adolescents. We have chosen a peptide mix derived from U54, which is a tegument protein of HHV-6 and the analog of the CMV lower matrix phosphoprotein 65 (pp65). The pp65 protein is one of the most dominant CD4+ and CD8+ T-cell antigens of CMV and is therefore widely used for the evaluation of CMV-specific immunity (14).
In a first set of experiments, we tested a short overnight stimulation of PBMCs with U54 antigen, but we were not able to reliably detect an antigen-specific response. A short-term stimulation reflects the in vivo situation more accurately but the in vivo frequencies of U54 specific T-cells were too low to be detected by flow cytometry. Consequently, we have decided to culture the cells in medium and interleukins for 10 days to obtain an adequate antigen-specific immune response against U54 (12).
For expansion of antigen-specific T-cells we used the cytokines IL-2 and IL-7. IL-7 promotes the proliferation of immature naïve human T-cells that have recently exited from the thymus (11, 12). IL-7 together with IL-2 primes naïve peripheral blood T-cells as well as recently exited T-cells for Fas (First apoptotic signal)-mediated cell death. However, IL-2/IL-7-stimulated memory T-cells are significantly more resistant to Fas-induced cell death (13). It must be considered that IL-2 and IL-7 promote homeostatic expansion of memory T-cells while limiting the size of the overall T-cell pool.
After stimulation, 10 days of cultivation and re-stimulation of PBMCs, an U54-specific response was observed in all individuals proofing the in vivo presence of HHV-6 specific T-cells capable of expansion after adequate in vitro stimulation. It might be assumed that these cells would also expand in vivo in clinical conditions necessitating increased immunological response. Individuals older than 10 years had significantly higher frequencies of HHV-6 specific CD8 + TNF-α T-cells than those younger than 10 years of age and data analysis showed a positive correlation between age of the individuals and frequency of these virus specific T-cells. In further studies, cytotoxicity assays should analyze the functional impact of these observed numeric differences.
Our results are in line with the fact that immunity to HHV-6 could evolve over time. Commonly, neonates are protected from HHV-6 infection by maternal-derived antibodies up to 3–9 months postnatally. A primary infection generally occurs before the second year of life leading to the persistence of viruses and latent infection with HHV-6, and in most cases antibodies persist throughout life (17). In parallel, the development of the HHV-6-specific T-cell response after primary infection must be assumed with an evolution of this response throughout life, driven by the interplay between latent infection and immunological response. This might be reflected by the observed higher CD8+ TNF-α T-cell frequencies in older children. In the current study, we did not perform serial testing of antigen-specific immunity to HHV-6 longitudinally but it would be interesting in future studies to test how cellular immunity against HHV-6 develops within single individuals.
HHV-6 specific T-cells play a key role in controlling the virus infection and overcoming immunomodulatory effects of HHV-6. The majority of expanded effector CD4+ and CD8+ populations secrete IFN-γ and TNF-α and have various cytolytic functions, mediated by the secretion of perforin (CD4+) or granzyme B (CD8+). These T-cells can rapidly lyse infected cells and reacquire cytotoxic activity when they are exposed to the antigen (20).
Interestingly, we could only detect few antigen-specific IL-2 producing T-cells. This might be explained by the fact that after restimulation with the U54 antigen both central memory T-cells as well as effector memory T-cells produce high amounts of TNF-α and IFN-γ but IL-2 is mainly produced by central memory cells (21, 22). HHV-6 serology was not tested due to the fact that nearly 100% of individuals older than 2 years of life are seropositive (7). For the same reason, we were not able to include HHV-6 seronegative individuals as negative controls.
There have been a limited number of studies on the specificity of the immune response to HHV-6, although cellular immune responses are fundamental in controlling primary infection and reactivation of the virus. Some earlier studies investigated T-cell proliferative responses to HHV-6 in healthy adults (15, 16). Yakushijin et al. generated CD4+ T-cell clones from HHV-6-stimulated PBMC that were found to proliferate upon stimulation with HHV-6 in the presence of autologous antigen-presenting cells and Yasukawa et al. investigated on the antigenic specifity of CD4+ T-cell clones against HHV-6, HHV-7, and HCMV. However, there are no detailed studies on the cellular immune response in healthy children and adolescents. Interestingly, Koide et al. described an immature lymphocytic response to HHV-6 antigen in children below 2 years (17). This is in line with our results and indicates that the virus-specific immune response after primary infection develops over time.
In individuals with chromosomal integrated HHV-6, antigen-specific T-cells have also been measured by Enzyme Linked Immuno Spot Assay (18).
Virus specific T-cells are also important for patients during immune reconstitution after hematopoietic stem cell transplantation (HSCT). Studies have shown that donor-derived virus-specific T-cells against different viruses were administered with impressive clinical results (19–24). These donor-derived virus- specific T-cells may constitute a cost-effective and more effective alternative to common antiviral agents for allogeneic HSCT recipients but requires a detailed understanding of virus-specific T-cells. Our results are intended to contribute to this knowledge. Furthermore, our results derived from healthy individuals will help to assess and interpret HHV-6 specific T-cell response in selected patient groups at higher risk for complicated HHV-6 infection or reactivation like recipients of HSCT.
To conclude, in our study we tested antigen-specific T-cell immunity to HHV-6 in healthy individuals. HHV-6-specific T-cells can be expanded in 9-day culture with addition of the proliferative cytokines IL-2 and IL-7 and in response to the viral antigen (U54) for analyses. Further studies on HHV-6-specific T-cell immunity in healthy individuals and in patients with impaired immune system should shed more light on the mentioned interplay and the immunopathology of HHV-6 infection and control of the virus.
Data Availability Statement
The raw data supporting the conclusions of this manuscript will be made available by the authors, without undue reservation, to any qualified researcher.
Author Contributions
CS, VS, HS, WS, and CU designed the research study. CS and AR conducted the experiments and acquired data. CS and VS analyzed data. GS and MK provided samples. CS, VS, HS, and GS wrote the manuscript.
Funding
This research project was a part of a project funded by the Styrian Childhood Cancer Organization and the work was carried out within the Doctoral School for Sustainable Health Research (Doctoral Program of Medical Science) at the Medical University of Graz. The funders had no role in study design, data collection, and analyses, decision to publish, or preparation of the manuscript.
Conflict of Interest Statement
The authors declare that the research was conducted in the absence of any commercial or financial relationships that could be construed as a potential conflict of interest.
Acknowledgments
Preliminary data of this study have been selected for poster presentation at the 55th Annual Meeting of the Austrian Society for Pediatrics and Adolescent Medicine (2017, Austria, Graz). The entire data of the manuscript have neither been presented nor submitted or accepted for publication elsewhere. The authors thank Magdalena Holter, BSc MSc (Institute for Medical Informatics, Statistics and Documentation, Medical University of Graz, Austria) for help with statistical issues and Prof. Dr. Tobias Feuchtinger (Ludwig-Maximilians-University Munich) for essential support in developing the used methods.
References
1. Salahuddin SZ, Ablashi D V, Markham PD, Josephs SF, Sturzenegger S, Kaplan M, et al. Isolation of a new virus, HBLV, in patients with lymphoproliferative disorders. Science (1986) 234:596–601. doi: 10.1126/science.2876520
2. Yamanishi K, Shiraki K, Kondo T, Okuno T, Takahashi M, Asano Y, et al. Identification of human herpesvirus-6 as a causal agent for exanthem subitum. Lancet (1988) 331:1065–7. doi: 10.1016/S0140-6736(88)91893-4
3. Okuno T, Takahashi K, Balachandra K, Shiraki K, Yamanishi K, Takahashi M, et al. Seroepidemiology of human herpesvirus 6 infection in normal children and adults. J Clin Microbiol. (1989) 27:651–3.
4. Zerr DM, Meier AS, Selke SS, Frenkel LM, Huang M-L, Wald A, et al. A population-based study of primary human herpesvirus 6 infection. N Engl J Med. (2005) 352:768–76. doi: 10.1056/NEJMoa042207
5. Ward KN, Gray JJ, Fotheringham MW, Sheldon MJ. IgG antibodies to human herpesvirus-6 in young children: changes in avidity of antibody correlate with time after infection. J Med Virol. (1993) 39:131–8. doi: 10.1002/jmv.1890390209
6. Ljungman P, Wang FZ, Clark DA, Emery VC, Remberger M, Ringden O, et al. High levels of human herpesvirus 6 DNA in peripheral blood leucocytes are correlated to platelet engraftment and disease in allogeneic stem cell transplant patients. Br J Haematol. (2000) 111:774–81. doi: 10.1046/j.1365-2141.2000.02422.x
7. De Bolle L, Van Loon J, De Clercq E, Naesens L. Quantitative analysis of human herpesvirus 6 cell tropism. J Med Virol. (2005) 75:76–85. doi: 10.1002/jmv.20240
8. Shiley K, Blumberg E. Herpes viruses in transplant recipients: HSV, VZV, human herpes viruses, and EBV. Hematol Oncol Clin North Am. (2011) 25:171–91. doi: 10.1016/j.hoc.2010.11.012
9. Strenger V, Pfurtscheller K, Wendelin G, Aberle SW, Nacheva EP, Zöhrer B, et al. Differentiating inherited human herpesvirus type 6 genome from primary human herpesvirus type 6 infection by means of dried blood spot from the newborn screening card. J Pediatr. (2011) 159:859–61. doi: 10.1016/j.jpeds.2011.06.032
10. Gerdemann U, Keukens L, Keirnan JM, Katari UL, Nguyen CTQ, De Pagter AP, et al. Immunotherapeutic strategies to prevent and treat human herpesvirus 6 reactivation after allogeneic stem cell transplantation. Blood (2013) 121:207–18. doi: 10.1182/blood-2012-05-430413
11. Hassan J, Reen DJ. Human recent thymic emigrants–identification, expansion, and survival characteristics. J Immunol. (2001) 167:1970–6. doi: 10.4049/jimmunol.167.4.1970
12. Dardalhon V, Jaleco S, Kinet S, Herpers B, Steinberg M, Ferrand C, et al. IL-7 differentially regulates cell cycle progression and HIV-1-based vector infection in neonatal and adult CD4+ T cells. Proc Natl Acad Sci USA. (2001) 98:9277–82. doi: 10.1073/pnas.161272698
13. Jaleco S, Swainson L, Dardalhon V, Burjanadze M, Kinet S, Taylor N. Homeostasis of naive and memory CD4+ T cells: IL-2 and IL-7 differentially regulate the balance between proliferation and Fas-mediated apoptosis. J Immunol. (2003) 171:61–8. doi: 10.4049/jimmunol.171.1.61
14. Sylwester AW, Mitchell BL, Edgar JB, Taormina C, Pelte C, Ruchti F, et al. Broadly targeted human cytomegalovirus-specific CD4 + and CD8 + T cells dominate the memory compartments of exposed subjects. J Exp Med. (2005) 202:673–85. doi: 10.1084/jem.20050882
15. Yakushijin Y, Yasukawa M, Kobayashi Y. T-cell immune response to human herpesvirus-6 in healthy adults. Microbiol Immunol. (1991) 35:655–60. doi: 10.1111/j.1348-0421.1991.tb01597.x
16. Yasukawa M, Yakushijin Y, Furukawa M, Fujita S. Specificity analysis of human CD4+ T-cell clones directed against human herpesvirus 6 (HHV-6), HHV-7, and human cytomegalovirus. J Virol. (1993) 67:6259–64.
17. Koide W, Ito M, Torigoe S, Ihara T, Kamiya H, Sakurai M. Activation of lymphocytes by HHV-6 antigen in normal children and adults. Viral Immunol. (1998) 11:19–25. doi: 10.1089/vim.1998.11.19
18. Barozzi P, Riva G, Vallerini D, Quadrelli C, Lagreca I, Eccheli R, et al. Circulating functional T cells specific to human herpes virus 6 (HHV6) antigens in individuals with chromosomally integrated HHV6. Clin Microbiol Infect. (2016) 22:893–5. doi: 10.1016/j.cmi.2016.07.006
19. Leen AM, Christin A, Myers GD, Liu H, Cruz CR, Hanley PJ, et al. Cytotoxic T lymphocyte therapy with donor T cells prevents and treats adenovirus and Epstein-Barr virus infections after haploidentical and matched unrelated stem cell transplantation. Blood (2009) 114:4283–92. doi: 10.1182/blood-2009-07-232454
20. Feuchtinger T, Richard C, Joachim S, Scheible MH, Schumm M, Hamprecht K, et al. Clinical grade generation of hexon-specific T cells for adoptive T-cell transfer as a treatment of adenovirus infection after allogeneic stem cell transplantation. J Immunother. (2008) 31:199–206. doi: 10.1097/CJI.0b013e31815ef862
21. Feuchtinger T, Opherk K, Bethge WA, Topp MS, Schuster FR, Weissinger EM, et al. Adoptive transfer of pp65-specific T cells for the treatment of chemorefractory cytomegalovirus disease or reactivation after haploidentical and matched unrelated stem cell transplantation. Blood (2010) 116:4360–7. doi: 10.1182/blood-2010-01-262089
22. Cobbold M, Khan N, Pourgheysari B, Tauro S, McDonald D, Osman H, et al. Adoptive transfer of cytomegalovirus-specific CTL to stem cell transplant patients after selection by HLA–peptide tetramers. J Exp Med. (2005) 202:379–86. doi: 10.1084/jem.20040613
23. Schmitt A, Tonn T, Busch DH, Grigoleit GU, Einsele H, Odendahl M, et al. Adoptive transfer and selective reconstitution of streptamer-selected cytomegalovirus-specific CD8+ T cells leads to virus clearance in patients after allogeneic peripheral blood stem cell transplantation. Transfusion (2011) 51:591–9. doi: 10.1111/j.1537-2995.2010.02940.x
Keywords: HHV-6, T-cell response, flow cytometry, U54, antigen-specific T-cells
Citation: Schwarz CM, Strenger V, Strohmaier H, Singer G, Kaiser M, Raicht A, Schwinger W and Urban C (2018) HHV-6 Specific T-Cell Immunity in Healthy Children and Adolescents. Front. Pediatr. 6:191. doi: 10.3389/fped.2018.00191
Received: 09 March 2018; Accepted: 08 June 2018;
Published: 02 July 2018.
Edited by:
Manuela Zlamy, Innsbruck Medical University, AustriaReviewed by:
Aleksandra Barac, Faculty of Medicine, University of Belgrade, SerbiaLouis Flamand, Laval University, Canada
Copyright © 2018 Schwarz, Strenger, Strohmaier, Singer, Kaiser, Raicht, Schwinger and Urban. This is an open-access article distributed under the terms of the Creative Commons Attribution License (CC BY). The use, distribution or reproduction in other forums is permitted, provided the original author(s) and the copyright owner(s) are credited and that the original publication in this journal is cited, in accordance with accepted academic practice. No use, distribution or reproduction is permitted which does not comply with these terms.
*Correspondence: Volker Strenger, dm9sa2VyLnN0cmVuZ2VyQG1lZHVuaWdyYXouYXQ=