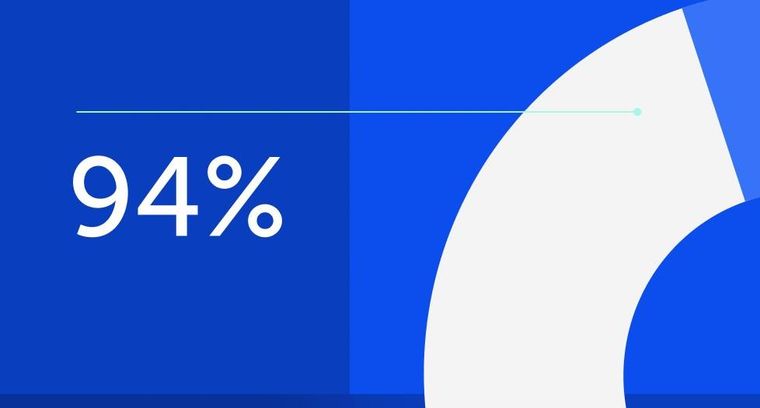
94% of researchers rate our articles as excellent or good
Learn more about the work of our research integrity team to safeguard the quality of each article we publish.
Find out more
ORIGINAL RESEARCH article
Front. Oncol., 25 September 2020
Sec. Cancer Imaging and Image-directed Interventions
Volume 10 - 2020 | https://doi.org/10.3389/fonc.2020.573279
This article is part of the Research TopicBreakthrough in Imaging-Guided Precision Medicine in OncologyView all 35 articles
Background: Reductions in tumor movement allow for more precise and accurate radiotherapy with decreased dose delivery to adjacent normal tissue that is crucial in stereotactic body radiotherapy (SBRT). Deep inspiration breath-hold (DIBH) is an established approach to mitigate respiratory motion during radiotherapy. We assessed the feasibility of combining modern optical surface-guided radiotherapy (SGRT) and image-guided radiotherapy (IGRT) to ensure and monitor reproducibility of DIBH and to ensure accurate tumor localization for SBRT as an imaging-guided precision medicine.
Methods: We defined a new workflow for delivering SBRT in DIBH for lung and liver tumors incorporating SGRT and IGRT with cone beam computed tomography (CBCT) twice per treatment fraction. Daily position corrections were analyzed and for every patient two points retrospectively characterized: an anatomically stable landmark (predominately Schmorl’s nodes or spinal enostosis) and a respiratory-dependent landmark (predominately surgical clips or branching vessel). The spatial distance of these points was compared for each CBCT and used as surrogate for intra- and interfractional variability. Differences between the lung and liver targets were assessed using the Welch t-test. Finally, the planning target volumes were compared to those of free-breathing plans, prepared as a precautionary measure in case of technical or patient-related problems with DIBH.
Results: Ten patients were treated with SBRT according this workflow (7 liver, 3 lung). Planning target volumes could be reduced significantly from an average of 148 ml in free breathing to 110 ml utilizing DIBH (p < 0.001, paired t-test). After SGRT-based patient set-up, subsequent IGRT in DIBH yielded significantly higher mean corrections for liver targets compared to lung targets (9 mm vs. 5 mm, p = 0.017). Analysis of spatial distance between the fixed and moveable landmarks confirmed higher interfractional variability (interquartile range (IQR) 6.8 mm) than intrafractional variability (IQR 2.8 mm). In contrast, lung target variability was low, indicating a better correlation of patients’ surface to lung targets (intrafractional IQR 2.5 mm and interfractional IQR 1.7 mm).
Conclusion: SBRT in DIBH utilizing SGRT and IGRT is feasible and results in significantly lower irradiated volumes. Nevertheless, IGRT is of paramount importance given that interfractional variability was high, particularly for liver tumors.
Focused delivery of high radiation doses to an extracranial tumor in few fractions is defined as stereotactic body radiotherapy (SBRT). It has become a commonly available and recognized treatment option for early stage primary tumors of or oligometastases from liver and lung primaries with a high rate of local control, often comparable to surgical resection (1–3). Due to technological advances in radiotherapy over the last decade, radiation plans with highly conformal dose distributions are widely available. This sculpted delivery of radiation dose is dependent on three-dimensional on-board imaging allowing image-guided radiotherapy (IGRT), which is now standard in modern linear accelerators. These improvements facilitate precise patient positioning and a safe and accurate characterization of dose deposition that are mandatory for SBRT. Nevertheless, moving targets are still challenging and respiratory motion management is the most crucial aspect for safe and effective utilization of SBRT (4).
In order to compensate for target motion, the International Commission on Radiation Units and Measurements (ICRU) introduced the concept of an internal margin to account for respiratory-induced changes in size, shape and position of a clinical target volume (CTV) (5). The addition of these internal margins to the CTV results in an internal target volume (ITV) to which further external margins for planning uncertainties are added to obtain a final planning target volume (PTV). This PTV is the volume that in the end receives the prescribed radiation dose. Yet, when this motion-encompassing approach is used for SBRT, the final PTV may become large or close to organs at risk (OAR), impeding the delivery of the high radiation doses needed for effective treatment. As a consequence, motion mitigation techniques were developed, which include: abdominal compression, beam-gating and breath-hold (6). Abdominal compression significantly reduces movement of the diaphragm and enables a good set-up accuracy for SBRT of liver and lower lung lobe targets in free-breathing (7, 8). For gated treatments an individual part of the respiratory cycle, usually the end-exhale phase, is chosen as a treatment window and further mitigates respiratory motion (4, 9). Tracking of targets with the beam is another method to manage respiratory motion, but requires real-time imaging during the treatment delivery (4, 10). The most reduction of respiratory movement, however, is achieved by breath-hold techniques that primarily target deep inspiration, which is tolerated longer than the end-exhale breath-hold phase and also carries less residual motion than gating of free breathing (4, 11).
For precise and accurate SBRT delivery, the reproducibility of deep inspiration breath-hold (DIBH) is crucial and has to be confirmed for several breath-hold cycles that are needed for a SBRT session. Depending on the PTV size, the beam-on time for SBRT typically varies between 2 and 5 min, despite already higher dose rates obtained by omission of the commonly used flattening filter (12). Reproducibility of DIBH can be assessed without additional radiation dose by either optical surface imaging solutions, such as AlignRTTM (Vision RT, London, United Kingdom), or active breathing control devices, such as ABCTM (Elekta, Stockholm, Sweden), which both reduce residual spatial uncertainties to 1–2 mm in standard radiotherapy of breast and lung cancer (13, 14).
Nevertheless, only limited data on SGRT and DIBH for SBRT is available. Here, we present our initial experience in utilizing a combination of SGRT and IGRT for patient positioning and treatment monitoring during SBRT of lung and liver targets.
Ten patients transferred for lung or liver SBRT to our department in 2018/19 were included in this pilot study. Only patients who could hold their breath for at least 30 s were eligible for study inclusion. All patients gave informed consent for an individualized SBRT approach using DIBH instead of treatment in free-breathing and abdominal compression which is standard of care. For monitoring of correct DIBH reproducibility the hard- and software tools of Vision RT Ltd. (London, United Kingdom) were used. These commercial solutions are officially approved and licensed for this purpose. The analysis was approved by the local ethics committee (S-063/2019).
Planning simulation was performed for all patients in vacuum cushion immobilization (BlueBAG BodyFIXTM, Innovative Technologie Völp (IT-V), Innsbruck, Austria). The edges of the cushion near to the target area were folded and smoothed to prevent shadowing and concealment of parts of the body surface for appropriate optical surface-guidance. Abdominal compression was not used to mitigate free breathing motion as it would prevent DIBH, recognition of the body surface by shadowing and could potentially modify the patient’s surface in an unreproducible manner. The AZ-733V Respiratory Gating System (Anzai Medical Co., Ltd., Japan) was utilized for registration of breathing motion and recording of time resolved, four-dimensional (4D) CTs. After 4D-CT acquisition, the respiratory belt was unstrapped and additional CT series in free-breathing as well as DIBH were recorded.
All CT data were transferred to our institutional treatment planning system (RayStation 6B, RaySearch Laboratories, Stockholm, Sweden). Target volumes were delineated using available contrast-enhanced imaging in both free-breathing and DIBH sequences. Planning target volumes (PTV) were generated in DIBH by adding a 3–5 mm safety margin to the clinical target volume (CTV). The CTV was created from the gross tumor volume (GTV) with an isotropic margin of 5–7 mm to account for microscopic spread. In contrast, the free-breathing PTV was created by a 2–3 mm expansion of an internal target volume (ITV) that integrated all motion information of the CTV extracted from the 4D-CT data. Treatment plans were calculated for both DIBH and free-breathing PTV, with the latter prepared as a precautionary measure in case of technical issues or patient-related problems with breath-hold.
The SBRT workflow of this study incorporates positioning and monitoring of patients in DIBH with surface-guidance (Figure 1A). A screenshot of the software (AlignRTTM version 5.1.1, Vision RT, London, United Kingdom) used for DIBH positioning and monitoring is shown in Figure 1B.
Figure 1. (A) Work flow of patient set-up and localization until treatment delivery together with (B) an example screenshot of one of our SBRT patients showing a typical region of interest (ROI) placed to the lower thorax for DIBH monitoring of a liver target.
In brief, the software allows for accurate patient set-up in DIBH and a radiation-free real-time feedback of DIBH positioning during the treatment session. Reproducibility of DIBH position was validated in-room by one to two repetitions. To verify the surface-guided position, a fast cone-beam computed tomography (CBCT), which lasts a breath-hold of 30 s, was acquired in DIBH. After registration to the planning CT, the couch was moved in DIBH accordingly and this new image guided position directly captured as a new surface reference in the SGRT software. To validate the reproducibility of tumor position in different breath-hold sequences, an additional fast CBCT was acquired. If a new registration resulted in shifts >2 mm, the couch was moved in DIBH as needed and the reference surface updated. Finally, orthogonal 2D-MV portal imaging was acquired in DIBH to confirm correct isocenter positioning.
After correct patient positioning the treatment was delivered in DIBH by a linear accelerator (Versa HD, Elekta, Stockholm, Sweden) using a flattening filter free (FFF) technique to reduce beam-on time whenever possible. We decided to set the individual defined region of interest (ROI), for which the SGRT software calculates differences in patient’s actual surface and the reference surface, to the lower thorax to measure thoracic motion during DIBH and to avoid large distances between the treatment isocenter and the ROIs’ centroid in liver SBRT. During treatment delivery the maximum allowed position error was 3 mm and 2° for translational and rotational differences, respectively. For patients’ comfort the treatment delivery was also stopped every 20–40 s to allow for breaks and prevent patients from becoming out of breath. The overall treatment time including positioning and IGRT varied between 20 and 60 min depending on various patient specific factors concerning breath-hold (maximum tolerated breath-hold duration, time until normalization of the respiratory rate, reproducibility of breath-hold) resulting in different numbers of breath-holds needed.
Differences in couch coordinates were assessed during the course of daily CBCT imaging (intrafractional) and compared to the derived shifts from subsequent treatment days (interfractional). For each patient and CBCT, a static, breathing-independent anatomic landmark (mostly Schmorl’s nodes or enostosis of the spine at the level of the gross tumor) and a moving, breathing-dependent landmark (such as clips near the liver target lesion or a pulmonary vessel branching adjacent to the lung target lesion) were defined and their intra- and interfractional positions compared.
Prism 7.04 software (GraphPad, San Diego, CA, United States) was used to perform statistical analysis and graphical plotting of results. Results were considered significant when the two-tailed p-value was less than 0.05. D’Agostino and Pearson omnibus K2 normality tests suggested a Gaussian distribution for the continuous variables of the vector length of position correction and spatial distance of fix and moving points, gross tumor volumes (GTV) and planning target volumes as well as the differences in planning target volumes in free-breathing and in DIBH for the same patient. Statistical differences between volumes of lung and liver targets were assessed using unpaired t-tests with Welch correction (Welch t-tests), which are more reliable for populations with unequal sample sizes and different variances than Student’s t-test. Paired t-tests were conducted for comparison of PTVs in DIBH and in free-breathing of the same patients. Frequencies of needs for a second position correction were grouped by target location and analyzed in a 2 × 2 contingency table and differences analyzed using Fisher’s exact test.
As a proof of concept analysis, we performed SBRT in DIBH in a total of 10 patients and for 41 treatment fractions using a combination of CBCT image- and surface-guidance for position verification and monitoring of DIBH. Details of our workflow are depicted in Figure 1 and explained in the methods section. Targets were either a primary tumor or single metastasis of the lungs and liver in 3 and 7 patients, respectively. Details on patients’ demographics and treatment delivery are shown in Table 1. Lung targets were mostly small peripheral lesions and could be treated in 3 fractions. In contrast, the liver tumors differed in size and some lesions were adjacent to organs at risk such as the gastrointestinal tract requiring individual dose fractionations between 3 and 8 fractions.
Despite correct alignment of patients’ surface to the reference surface in DIBH, a subsequent CBCT-based image correction was required for most treatment fractions. These couch shifts ranged from −7 to 9 mm, −25 to 12 mm, and −13 to 12 mm in lateral, longitudinal and vertical direction, respectively. Nevertheless, median values of all shift directions of first CBCT were close to zero. Grouping patients by target location revealed higher shifts for tumors in the liver compared to lung targets. Highest ranges were observed for shifts in cranial-caudal direction along the y-axis (Figure 2A).
Figure 2. Box plots with boxes extending from 25th to 75th percentiles. Whiskers are drawn down to 5th and up to 95th percentiles, respectively. (A) Couch shifts are shown in left (+) to right (–), cranial (+) to caudal (–) and anterior (+) to posterior (–) directions along the x-, y-, and z-axis, respectively. Values were grouped by target location and derived from first CBCTs for position verification after alignment of current DIBH surface to that of the reference surface. (B) Length of correction vectors of first CBCTs for image-guidance in DIBH and grouped by target location. Statistical difference was calculated by Welch’s t-test.
The mean lengths of applied position correction vectors were 5.4 mm (2.0–10.2) and 8.8 mm (1.7–26.9) for lung and liver targets, respectively. Compared to liver targets, the correction vectors of lung targets were significantly smaller (p = 0.0172, Welch’s t-test, Figure 2B).
A second position verification by CBCT in DIBH utilizing the newly captured reference surface could confirm a correct set-up in most cases. A new couch shift was required in 1 of 9 (11%) and 7 of 34 (21%) treatment sessions for lung and liver targets, respectively. Despite higher rates for liver SBRT, there was no significant difference in the frequency of the need for a second position correction for lung and liver targets (p = 1.0, Fisher’s exact test).
The planning CT and the two CBCTs acquired for every treatment session and each patient were retrospectively analyzed for intra- and interfractional difference. Figure 3 shows the calculated spatial distances from an individually selected, breathing-independent point (mostly bony structures of the spine at the level of the target) to another individually chosen, breathing-dependent point near the gross tumor (mostly surgical clips or a vessel branching) for each patient and CBCT.
Figure 3. Assessment of (A) intra-and (B) interfractional variations by comparison of absolute distances between a breathing independent point to a breathing dependent movable point of first compared to second CBCT and of planning CT compared to first CBCT for every fraction, respectively. Values are grouped by target location. The dotted line indicates theoretical equality of distances in imaging.
In case of a perfect match, the distances between the breathing-independent point and the breathing-dependent point were equal for every imaging series (planning CT and CBCT). Equality of distances of first and second CBCT as well as of planning CT and first CBCT would correspond to no intra- and no interfractional difference, respectively.
Intra-fraction distances of both lung and liver targets were close to the line of equality (Figure 3A). On average, the intrafractional differences were 1.6 mm (-3.9 to 0.5) and 1.2 mm (-27.8 to 28.3) for targets in the lungs and liver, respectively. Despite higher ranges for liver targets compared to lung targets the interquartile ranges (IQR) for both lung and liver targets were similar (2.5 mm vs. 2.8 mm). There were no significant differences of intrafractional spatial distances between lung and liver target location (p = 0.12, Welch’s t-test).
In contrast, mean interfractional differences for lung targets were 0.9 mm (0.7–2.1; IQR 1.7 mm), whereas in liver targets the average difference was 3.8 mm (−32.7 to 13.8) with a higher IQR of 6.8 mm (Figure 3B). These interfractional differences were statistically significant (p = 0.01, Welch’s t-test), indicating higher variability between treatment sessions for liver SBRT compared to lung SBRT.
The mean gross tumor volumes (GTV) of lung targets, liver targets and all lesions were 1.9, 67, and 48 ml, respectively, with significantly smaller GTVs for lung targets (p = 0.018, Welch’s t-test). The mean internal target volumes (ITV) which comprise the clinical target volumes (CTV) with additional margins obtained from 4D-CT data in free-breathing was 108 ml for all lesions. By using DIBH these margins could be omitted and the CTV in DIBH was 28% smaller (77 ml, p < 0.01, paired t-test, Figure 4A). Consequently, planning target volumes of all targets could be reduced significantly by 26% from an average of 148 ml in free-breathing (FB) to 110 ml utilizing DIBH (p < 0.01, paired t-test). This reduction of target volumes by using DIBH was seen for both lung and liver targets. However, statistical significance was not reached for the smaller sub-group of lung targets (Figure 4B).
Figure 4. Volumes of gross tumor (GTV), clinical target volume (CTV), internal target volume (ITV) and planning target in DIBH (CTV DIBH and PTV DIBH) as well as in free-breathing (ITV FB and PTV FB) for (A) all patients and (B) grouped by target location. Statistical differences were calculated by paired t-test.
We share a new work-flow for SBRT in DIBH by combining SGRT and IGRT in patients with targets in the lungs and liver and demonstrate feasibility. Utilizing DIBH for SBRT significantly reduced the irradiated volumes compared to free-breathing treatment plans. SGRT enabled initial couch shifts based on first CBCT in DIBH to be close to zero, but the ranges and absolute correction vectors of liver targets were significantly higher when compared to lung targets. This interfractional variability resulted in a daily need for position correction and for an updated reference surface for most patients for every treatment session. Intrafractional movements on the other hand, were quite low with second CBCTs in DIBH rarely requiring correction of patient positioning in both liver and lung SBRT. Most liver SBRT patients exhibited reproducible intrafractional positioning in DIBH. In contrast to targets in the liver, both intra- and interfractional variability of lung targets was small indicating a better correlation of patients’ surface to lung targets rather than to liver targets.
Deep inspiration breath-hold was introduced many years ago for radiotherapy of left sided breast cancer patients to reduce the irradiated heart volume by enlarging the distance of the heart to the chest well (15). Reproducibility was ensured by the active breathing coordinatorTM (ABCTM, Elekta, Stockholm, Sweden), a commercial device consisting of a nose clamp and a mouthpiece that is connected to a breathing tube containing a valve which closes once a pre-defined target air volume is inhaled by the patient. The prospective United Kingdom HeartSpare Study compared this ABCTM-assisted breath-hold with a voluntary breath-hold technique and found that the latter was non-inferior in terms of reproducibility and normal tissue sparing. Moreover, voluntary breath-hold was faster and preferred by both patients and therapists (16).
Surface-guided radiotherapy describes the use of commercially available surface imaging solutions that were primarily developed to assist with patient set-up before radiotherapy delivery. These tools precisely indicate the spatial difference of a region of interest (ROI) on the patients’ body surface and a corresponding reference surface generated from the external contour of the planning simulation CT, facilitating a fast and accurate patient set-up with six degrees of freedom (17). SGRT is non-invasive, patients are not exposed to additional radiation dose, it basically does not rely on skin marks and allows monitoring of DIBH with most data available for SGRT with DIBH for adjuvant radiotherapy in left sided breast-cancer (18). Since SGRT allows not only monitoring of the patient-setup and breathing before, but also during treatment it is of particular interest for SBRT to ensure a safe, accurate and precise dose delivery during the entire treatment session.
For SBRT in targets that move with respiration, an abdominal compression is traditionally employed to mitigate breathing motion and to decrease the residual range of motion, as assessed during time resolved CT acquisition. These 4D-CT data are used to create a PTV that encompasses the tumor during the entire breathing cycle or to define a specific gating window which results in reduced irradiation volumes but, on the other hand, extend treatment delivery time (4). Several reports, however, have commented that the 4D-CT information from planning is not necessarily representative of the motion amplitude during treatment (19, 20). Moreover, breathing patterns may change both within and between treatments (intra- and interactionally) (21, 22). Therefore, we tested the feasibility of combining SGRT with IGRT to enable SBRT in DIBH.
Earlier data on SGRT and SBRT in free-breathing suggests that the pre-imaging treatment set-up for SBRT can be improved by SGRT compared to in-room laser localization of skin tattoos or skin marks (23). Moreover, SGRT reliably detects intrafractional shifts during treatment delivery when deviations extend 2 mm, as confirmed by CBCT (24). In addition, DIBH improves image quality and reduces craniocaudal registration uncertainties compared to free-breathing in lung cancer radiotherapy (25). Furthermore, using breath-hold for SBRT delivery reduces motion artifacts that is especially important for small lung tumors that are poorly visualized on imaging even with modern linear accelerators (26). Yet to perform IGRT in combination with DIBH, imaging within a breath-hold of approximately 30 s was required. We achieved this through our technique by not performing a complete gantry rotation and instead using a higher than standard gantry rotation speed without appreciable loss of image information. As previously suggested, faster CBCTs show no significant registration differences compared to standard CBCTs and a higher gantry velocity with fewer projections produces fewer reconstruction artifacts (27).
We observed highest interfractional variability in cranio-caudal direction and lowest in left-right direction, underpinning the idea that respiratory motion, which is mostly performed by the diaphragm, impacts most on target localization. Indeed, using SGRT for positioning of breast cancer patients in free-breathing showed least errors for lateral set-up compared to imaging (28). Our data further suggest a higher correlation of thoracic DIBH surface to intrathoracic targets than to abdominal targets, despite the fact that we chose to set the ROI for DIBH monitoring to the lower thorax for both thoracic and abdominal targets. Indeed, the correlation of skin to tumor is not necessarily constant, especially for liver and pancreas as previously reported (29, 30). In addition, lung volumes in DIBH may not necessarily always be the same for every breath-hold, although SGRT may confirm a match within a ROI on the patient’s body surface to the corresponding reference surface. Such variations in lung volumes during breath-hold were recently reported to have an impact on target localization (31).
Previous work on employing active breathing coordinator (ABCTM)-controlled breath-hold for lung and liver SBRT reported good intrafractional reproducibility of liver position in the majority of patients. However, interfractional reproducibility was worse, suggesting a need for daily image guidance (31–33). Another study, conducted by Lu et al., also found higher interfractional than intrafractional motion but observed clinically significant intrafractional motion >3 mm in 26 and 47% of patients with liver and lung cancer, respectively (34). This intrafractional differences could be explained by an intra-breath-hold residual motion of the diaphragm that was recently estimated by an ultrasound-based monitoring of the diaphragm dome during ABCTM-controlled breath-holds to be <2 mm and <5 mm in 59 and 95% of 385 DIBHs in 13 patients (35).
The most elegant solution for precise and accurate SBRT in DIBH is probably possible with MR-guidance since on-board magnetic resonance (MR) imaging in linear accelerators (MR-LINAC) for set-up and treatment delivery is non-invasive, exposes the patient to no additional radiation and allows for direct target localization and real-time visualization (36). In contrast, SGRT remains an indirect visualization of the patient’s surface although it gives feedback of localization in real-time, too. Nevertheless, MR-LINACs are currently rarely available and there are still some issues concerning reliability of gating and tracking procedures, the additional time needed for dose-optimization and the dose delivery time, that is yet mostly slower than in conventional linear accelerators (37). Thus, a SBRT session in DIBH at a MR-LINAC would require a patient to perform more breath-holds. In addition, a MR LINAC treatment is not an option for every patient depending on body size, claustrophobia and metal implants or implanted electronic devices.
Surface-guided radiotherapy in DIBH for lung and liver tumors using a combination of SGRT and IGRT is feasible. This approach is easy to incorporate in contemporary practice and does not require any breathing tubes connected to the patient. Nevertheless, daily 3D imaging is of paramount importance given that interfractional variability is high, particularly in DIBH for liver SBRT.
The datasets presented in this article are not readily available because legal regulations disallow and as a requirement of local ethics committee clinical raw data must not be made available to others than those involved in the study. Requests to access the datasets should be directed tocGF0cmljay5uYXVtYW5uQG1lZC51bmktaGVpZGVsYmVyZy5kZQ==.
The studies involving human participants were reviewed and approved by Ethikkommission Heidelberg. The patients/participants provided their written informed consent to participate in this study.
PN and VB were responsible for conceptualization, data curation and formal analysis. BR and JD supervised the project. PN drafted the manuscript. PN, VB, JF, BR, and ET-M participated in patient treatment. BF and JL critically reviewed the manuscript. All authors read and approved the final manuscript.
This research received no external funding, but we acknowledge financial support for parts of publication costs by Deutsche Forschungsgemeinschaft within the funding program Open Access Publishing, by the Baden-Württemberg Ministry of Science, Research and the Arts and by Ruprecht-Karls-Universität Heidelberg.
In the past 5 years JD attended advisory board meetings of MERCK KGaA (Darmstadt), for which the University hospital Heidelberg received travel grants and honoraria. His department further received funding for research projects and for educational grants to the University hospital of Heidelberg by Accuray (2016), Merck KGaA (2015-open), Siemens GmbH (2015-open), Viewray (2018-open), Vision RT (2017-open). He is CEO of the HIT GmbH and also member of the kuratorium of the Physikalisch Technische Bundesanstalt (PTB). As chair of HIRO (Heidelberg Institute of Radiation Oncology) and director of the NCT (National Center for Tumor Diseases) he is responsible for collaborations with a multitude of companies and institutions. JD confirms that to the best of his knowledge none of the above funding sources was involved in the data analysis and preparation of this work.
The remaining authors declare that the research was conducted in the absence of any commercial or financial relationships that could be construed as a potential conflict of interest.
We thank all involved patients as well as all therapists for their patience. Without their support the study would not have succeeded.
1. Salama JK, Kirkpatrick JP, Yin, FF. Stereotactic body radiotherapy treatment of extracranial metastases. Nature Rev Clin Oncol. (2012) 9:654–65. doi: 10.1038/nrclinonc.2012.166
2. Tree AC, Khoo VS, Eeles RA, Ahmed M, Dearnaley DP, Hawkins MA, et al. Stereotactic body radiotherapy for oligometastases. Lancet Oncol. (2013) 14:e28–37.
3. Guckenberger M, Baus WW, Blanck O, Combs SE, Debus J, Engenhart-Cabillic R, et al. Definition and quality requirements for stereotactic radiotherapy: consensus statement from the DEGRO/DGMP working group stereotactic radiotherapy and radiosurgery. Strahlenther Onkol. (2020) 196:417–20. doi: 10.1007/s00066-020-01603-1
4. Dieterich S, Green O, Booth, J. SBRT targets that move with respiration. Phys Med. (2018) 56:19–24. doi: 10.1016/j.ejmp.2018.10.021
5. Landberg T, Chavaudra J, Dobbs J, Gerard J, Hanks G, Horiot J, et al. Report 62. J ICRU. (1999) os32.
6. Keall PJ, Mageras GS, Balter JM, Emery RS, Forster KM, Jiang SB, et al. The management of respiratory motion in radiation oncology report of AAPM task group 76. Medical Phys. (2006) 33:3874–900. doi: 10.1118/1.2349696
7. Herfarth KK, Debus J, Lohr F, Bahner ML, Fritz P, Hoss A, et al. Extracranial stereotactic radiation therapy: set-up accuracy of patients treated for liver metastases. Int J Radiation Oncol Biol Phys. (2000) 46:329–35. doi: 10.1016/s0360-3016(99)00413-7
8. Bouilhol G, Ayadi M, Rit S, Thengumpallil S, Schaerer J, Vandemeulebroucke J, et al. Is abdominal compression useful in lung stereotactic body radiation therapy? A 4DCT and dosimetric lobe-dependent study. Phys Med. (2013) 29:333–40. doi: 10.1016/j.ejmp.2012.04.006
9. Brandner ED, Chetty IJ, Giaddui TG, Xiao Y, Huq, MS. Motion management strategies and technical issues associated with stereotactic body radiotherapy of thoracic and upper abdominal tumors: a review from NRG oncology. Medical Phys. (2017) 44:2595–612. doi: 10.1002/mp.12227
10. Schmitt D, Blanck O, Gauer T, Fix MK, Brunner TB, Fleckenstein J, et al. Technological quality requirements for stereotactic radiotherapy : expert review group consensus from the DGMP working group for physics and technology in stereotactic radiotherapy. Strahlenther Onkol. (2020) 196:421–43. doi: 10.1007/s00066-020-01583-2
11. Boda-Heggemann J, Knopf AC, Simeonova-Chergou A, Wertz H, Stieler F, Jahnke A, et al. Deep inspiration breath hold-based radiation therapy: a clinical review. Int J Radiation Oncol Biol Phys. (2016) 94:478–92. doi: 10.1016/j.ijrobp.2015.11.049
12. Navarria P, Ascolese AM, Mancosu P, Alongi F, Clerici E, Tozzi A, et al. Volumetric modulated arc therapy with flattening filter free (FFF) beams for stereotactic body radiation therapy (SBRT) in patients with medically inoperable early stage non small cell lung cancer (NSCLC). Radiother Oncol. (2013) 107:414–8. doi: 10.1016/j.radonc.2013.04.016
13. Alderliesten T, Sonke JJ, Betgen A, Honnef J, van Vliet-Vroegindeweij C, Remeijer, P. Accuracy evaluation of a 3-dimensional surface imaging system for guidance in deep-inspiration breath-hold radiation therapy. Int J Radiation Oncol Biol Phys. (2013) 85:536–42. doi: 10.1016/j.ijrobp.2012.04.004
14. Wong VY, Tung SY, Ng AW, Li FA, Leung, JO. Real-time monitoring and control on deep inspiration breath-hold for lung cancer radiotherapy–combination of ABC and external marker tracking. Medical Phys. (2010) 37:4673–83. doi: 10.1118/1.3476463
15. Sixel KE, Aznar MC, Ung, YC. Deep inspiration breath hold to reduce irradiated heart volume in breast cancer patients. Int J Radiation Oncol Biol Phys. (2001) 49:199–204. doi: 10.1016/s0360-3016(00)01455-3
16. Bartlett FR, Colgan RM, Carr K, Donovan EM, McNair HA, Locke I, et al. The UK HeartSpare study: randomised evaluation of voluntary deep-inspiratory breath-hold in women undergoing breast radiotherapy. Radiother Oncol. (2013) 108:242–7. doi: 10.1016/j.radonc.2013.04.021
17. Schoffel PJ, Harms W, Sroka-Perez G, Schlegel W, Karger, CP. Accuracy of a commercial optical 3D surface imaging system for realignment of patients for radiotherapy of the thorax. Phys Med Biol. (2007) 52:3949–63. doi: 10.1088/0031-9155/52/13/019
18. Hoisak JDP, Pawlicki, T. The role of optical surface imaging systems in radiation therapy. Semin Radiat Oncol. (2018) 28:185–93. doi: 10.1016/j.semradonc.2018.02.003
19. Worm ES, Hoyer M, Fledelius W, Hansen AT, Poulsen, PR. Variations in magnitude and directionality of respiratory target motion throughout full treatment courses of stereotactic body radiotherapy for tumors in the liver. Acta Oncol. (2013) 52:1437–44. doi: 10.3109/0284186x.2013.813638
20. Park JC, Park SH, Kim JH, Yoon SM, Song SY, Liu Z, et al. Liver motion during cone beam computed tomography guided stereotactic body radiation therapy. Medical Phys. (2012) 39:6431–42. doi: 10.1118/1.4754658
21. Ge J, Santanam L, Noel C, Parikh, PJ. Planning 4-dimensional computed tomography (4DCT) cannot adequately represent daily intrafractional motion of abdominal tumors. Int J Radiation Oncol Biol Phys. (2013) 85:999–1005. doi: 10.1016/j.ijrobp.2012.09.014
22. Dhont J, Vandemeulebroucke J, Burghelea M, Poels K, Depuydt T, Van Den Begin R, et al. The long- and short-term variability of breathing induced tumor motion in lung and liver over the course of a radiotherapy treatment. Radiother Oncol. (2018) 126:339–46. doi: 10.1016/j.radonc.2017.09.001
23. Leong B, Padilla, L. Impact of use of optical surface imaging on initial patient setup for stereotactic body radiotherapy treatments. J Appl Clin Med Phys. (2019) 20:149–58. doi: 10.1002/acm2.12779
24. Heinzerling JH, Hampton CJ, Robinson M, Bright M, Moeller BJ, Ruiz J, et al. Use of surface-guided radiation therapy in combination with IGRT for setup and intrafraction motion monitoring during stereotactic body radiation therapy treatments of the lung and abdomen. J Appl Clin Med Phys. (2020) 21:48–55. doi: 10.1002/acm2.12852
25. Josipovic M, Persson GF, Bangsgaard JP, Specht L, Aznar, MC. Deep inspiration breath-hold radiotherapy for lung cancer: impact on image quality and registration uncertainty in cone beam CT image guidance. Br J Radiol. (2016) 89:20160544. doi: 10.1259/bjr.20160544
26. Peng Y, Vedam S, Chang JY, Gao S, Sadagopan R, Bues M, et al. Implementation of feedback-guided voluntary breath-hold gating for cone beam CT-based stereotactic body radiotherapy. Int J Radiation Oncol Biol Phys. (2011) 80:909–17. doi: 10.1016/j.ijrobp.2010.08.011
27. Arns A, Wertz H, Boda-Heggemann J, Schneider F, Blessing M, Abo-Madyan Y, et al. Ultrafast single breath-hold cone-beam CT lung cancer imaging with faster linac gantry rotation. Radiother Oncol. (2019) 135:78–85. doi: 10.1016/j.radonc.2019.02.004
28. Hattel SH, Andersen PA, Wahlstedt IH, Damkjaer S, Saini A, Thomsen, JB. Evaluation of setup and intrafraction motion for surface guided whole-breast cancer radiotherapy. J Appl Clin Med Phys. (2019) 20:39–44. doi: 10.1002/acm2.12599
29. Velec M, Moseley JL, Craig T, Dawson LA, Brock, KK. Accumulated dose in liver stereotactic body radiotherapy: positioning breathing, deformation effects. Int J Radiation Oncol Biol Phys. (2012) 83:1132–40. doi: 10.1016/j.ijrobp.2011.09.045
30. Minn AY, Schellenberg D, Maxim P, Suh Y, McKenna S, Cox B, et al. Pancreatic tumor motion on a single planning 4D-CT does not correlate with intrafraction tumor motion during treatment. Am J Clin Oncol. (2009) 32:364–8. doi: 10.1097/coc.0b013e31818da9e0
31. Lee S, Zheng Y, Podder T, Biswas T, Verma V, Goss M, et al. Tumor localization accuracy for high-precision radiotherapy during active breath-hold. Radiother Oncol. (2019) 137:145–52. doi: 10.1016/j.radonc.2019.04.036
32. Eccles C, Brock KK, Bissonnette JP, Hawkins M, Dawson, LA. Reproducibility of liver position using active breathing coordinator for liver cancer radiotherapy. Int J Radiation Oncol Biol Phys. (2006) 64:751–9. doi: 10.1016/j.ijrobp.2005.05.066
33. Brock J, McNair HA, Panakis N, Symonds-Tayler R, Evans PM, Brada, M. The use of the active breathing coordinator throughout radical non-small-cell lung cancer (NSCLC) radiotherapy. Int J Radiation Oncol Biol Phys. (2011) 81:369–75.
34. Lu L, Diaconu C, Djemil T, Videtic GM, Abdel-Wahab M, Yu N, et al. Intra- and inter-fractional liver and lung tumor motions treated with SBRT under active breathing control. J Appl Clin Med Phys. (2018) 19:39–45.
35. Vogel L, Sihono DSK, Weiss C, Lohr F, Stieler F, Wertz H, et al. Intra-breath-hold residual motion of image-guided DIBH liver-SBRT: an estimation by ultrasound-based monitoring correlated with diaphragm position in CBCT. Radiother Oncol. (2018) 129:441–8.
36. Klüter, S. Technical design and concept of a 0.35 T MR-Linac. Clin Transl Radiat Oncol. (2019) 18:98–101.
Keywords: stereotactic body radiation, Deep-inspiration breath-hold, surface-guided radiation therapy, Image-guided radiation therapy, precision radiation oncology, lung tumor, liver metastasis
Citation: Naumann P, Batista V, Farnia B, Fischer J, Liermann J, Tonndorf-Martini E, Rhein B and Debus J (2020) Feasibility of Optical Surface-Guidance for Position Verification and Monitoring of Stereotactic Body Radiotherapy in Deep-Inspiration Breath-Hold. Front. Oncol. 10:573279. doi: 10.3389/fonc.2020.573279
Received: 16 June 2020; Accepted: 08 September 2020;
Published: 25 September 2020.
Edited by:
Laurent Dercle, Columbia University Irving Medical Center, United StatesReviewed by:
Cyril Jaudet, Centre François Baclesse, FranceCopyright © 2020 Naumann, Batista, Farnia, Fischer, Liermann, Tonndorf-Martini, Rhein and Debus. This is an open-access article distributed under the terms of the Creative Commons Attribution License (CC BY). The use, distribution or reproduction in other forums is permitted, provided the original author(s) and the copyright owner(s) are credited and that the original publication in this journal is cited, in accordance with accepted academic practice. No use, distribution or reproduction is permitted which does not comply with these terms.
*Correspondence: Patrick Naumann, cGF0cmljay5uYXVtYW5uQG1lZC51bmktaGVpZGVsYmVyZy5kZQ==
Disclaimer: All claims expressed in this article are solely those of the authors and do not necessarily represent those of their affiliated organizations, or those of the publisher, the editors and the reviewers. Any product that may be evaluated in this article or claim that may be made by its manufacturer is not guaranteed or endorsed by the publisher.
Research integrity at Frontiers
Learn more about the work of our research integrity team to safeguard the quality of each article we publish.