- 1Department of Medical Genetics, Shahid Beheshti University of Medical Sciences, Tehran, Iran
- 2Department of Anatomical Sciences, Faculty of Medicine, Birjand University of Medical Sciences, Birjand, Iran
- 3Urogenital Stem Cell Research Center, Shahid Beheshti University of Medical Sciences, Tehran, Iran
Dysregulation of metabolic pathways in cancer cells is regarded as a hallmark of cancer. Identification of these abnormalities in cancer cells dates back to more than six decades, far before discovery of oncogenes and tumor suppressor genes. Based on the importance of these pathways, several researchers have aimed at modulation of these functions to intervene with the pathogenic course of cancer. Numerous genes have been shown to participate in the regulation of metabolic pathways, thus aberrant expression of these genes can be involved in the pathogenesis of cancer. The recent decade has experienced a significant attention toward the role of long non-coding RNAs (lncRNAs) in the biological functions. These transcripts regulate expression of genes at several levels, therefore influencing the activity of cancer-related pathways. Among the most affected pathways are those modulating glucose homeostasis, as well as amino acid and lipid metabolism. Moreover, critical roles of lncRNAs in regulation of mitochondrial function potentiate these transcripts as novel targets for cancer treatment. In the current review, we summarize the most recent literature regarding the role of lncRNAs in the cancer metabolism and their significance in the design of therapeutic modalities.
Introduction
Altered metabolic pathways in cancer has been attracting researchers for more than six decades when Warburg hypothesized that the tumorigenesis process is initiated by a deficient cellular respiration due to the mitochondrial function impairment (1). This research area remarkably precedes the identification of the role of oncogenes and tumor suppressors in the carcinogenesis (2). While normal cells obtain energy principally via mitochondrial oxidative phosphorylation (3), cancer cells can fulfill the requirements of their fast and uncontrolled proliferation by excessive glycolysis and the subsequent lactic acid fermentation even in the existence of plentiful oxygen supply. This kind of aerobic glycolysis has been characterized as the Warburg effect (4). Carcinogenesis process is accompanied by the extensive synchronized activation of metabolic pathways that maintain this process by dysregulation of PI3K-AKT-mTOR signaling pathways, deficiency of tumor suppressors, and induction of oncogenes (2). The altered metabolic functions in the cancer cells have been shown to facilitate the attainment and preservation of malignant features. Since some of these characteristics have been detected rather commonly across many kinds of cancer cells, altered metabolic function is regarded as a hallmark of cancer (5). This aberrant metabolic function facilitates anabolic growth in the course of nutrient-depleted situations, catabolism to sustain cell survival for the period of nutrient insufficiency, and protection of redox homeostasis to neutralize the metabolic influences of oncogene activation, tumor suppressor deficiency or other cellular stresses (6). Such metabolic reprogramming involves several genes and molecular pathways among them are long non-coding RNAs (lncRNAs) (7). These transcripts comprise a large proportion of human transcriptome, have sizes larger than 200 nucleotides and share several features with mRNA coding genes; yet, they lack considerable open reading frames (8). Not only can they regulate cell proliferation, cell death, migration, invasion and stemness properties (9), but also they have critical roles in the regulation of cancer metabolism (7). The latter has been supported by a bunch of evidence which reports aberrant expression of metabolism-related lncRNAs in cancer cells. Moreover, functional studies have verified their roles in the context of cancer in some cases. The current review has focused on the role of lncRNAs in cancer metabolism and provides key examples in this regard. Based on the ever growing literature on this topic, this review cannot provide the exhaustive list of all related researches.
Mechanisms of lncRNAs Functions in Regulation of Gene Expression
LncRNAs can exert their regulatory functions through different mechanisms such as modulation of chromatin structure and DNA methylation status and interacting with transcription factors and DNA motifs, thus regulating transcription of target genes. They also influence mRNA processing to affect gene expression at post-transcriptional level. Besides, their interactions with certain proteins enable them to regulate protein translation and post-translational alterations such as phosphorylation and ubiquitination (10). These transcripts can function as miRNA sponges to modulate expression of miRNA target genes or serve as precursors for miRNA or small interfering RNAs (10). LncRNAs can also modulate alternative splicing processes and consequently modulate spatial and temporal expression of genes (10).
Oncogenic lncRNAs That Regulate Cancer Metabolism
Oncogenic lncRNAs regulate different aspects of cancer metabolism such as glutaminolysis and lipid metabolism. For instance, UCA1 has an established role in the regulation of glutamine metabolism (11). Expression of this lncRNA in bladder cancer tissues and cell lines is significantly correlated with GLS2 expression. Moreover, up-regulation of UCA1 enhances GLS2 expression and increases glutaminolysis in these cells. This function has been mediated through sponging miR-16, a miRNA that targets GLS2 (11). Besides, the oncogenic lncRNA CCAT2 has been shown to alter glutamine metabolism in colon cancer (12). Functional studies revealed the interaction between CCAT2 and CFIm complex, a protein complex that modulates the alternative splicing and the poly(A) site choosing in GLS transcript, leading to the privileged expression of the more aggressive variant GAC (12). The lncRNA PCGEM1 has been shown to enhance glucose entry in the cells to increase aerobic glycolysis, coupling with the pentose phosphate shunt to enhance lipogenesis in prostate cancer cells (12).
Several other up-regulated lncRNAs in the cancer cells have been shown to alter cancer metabolism. The metabolism-induced tumor activator 1 (MITA1) is an lncRNA which has been shown to be over-expressed in hepatocellular carcinoma (HCC) and participates in the metastatic potential of these cells. This lncRNA is remarkably activated by energy stress. This process is controlled by the LKB1-AMPK pathway and DNA methylation (13). Another experiment in the HCC cells has shown correlation between expressions of the lncRNA RAET1K and both HIF1A and miR-100-5p. LncRNA RAET1K has been shown to act as a molecular sponge for miR-100-5p, thus inhibiting its expression. On the other hand, HIF1A binds with the promoter region of lncRNA RAET1K to enhance its transcription. LncRNA RAET1K knock down has inhibited proliferation and invasion of HCC cells and also overturned hypoxia-induced upsurge in lactate levels and glucose uptake. Functional studies have verified the role of the HIF1A/lncRNA RAET1K/miR-100-5p axis in regulation of hypoxia-induced glycolysis in HCC cells (14). PVT1, as an up-regulated lncRNA in HCC tissues and cell lines, can directly interact with miR-150 to suppress its expression and subsequently up-regulating expression hypoxia-inducible protein 2 (HIG2) which is targeted by the miR-150. The PVT1/miR-150/HIG2 axis has been shown to regulate iron metabolism in HCC cells (15). In gastric cancer cells, LINC00152 has been shown to modulate aerobic glycolysis through modulation of miR-139-5p and PRKAA1 expressions (16). LINK-A, the upregulated lncRNA in the glioma cells, has been shown to regulate expression of lactate dehydrogenase A (LDH-A), thus enhancing glycolysis and proliferation in these cells (17). Expression of HOTAIR has been increased in hepatocellular carcinoma samples. Its expression has been enhanced under hypoxia condition. Its silencing suppressed glycolysis in these cells. Functional studies verified the role of HOTAIR as a molecular sponge for miR-130a-3p. This miRNA has been shown to inhibit expression of HIF1A. HOTAIR silencing inhibited glycolysis through modulating miR-130a-3p and HIF1A in HCC cells under hypoxic conditions (18). This lncRNA regulates cancer metabolism in pancreatic adenocarcinoma cells as well. Up-regulation of HOTAIR enhances lactate synthesis, glucose uptake and ATP synthesis. Besides, it increases HK2 expression, while HK2 up-regulation had no remarkable influence on HOTAIR expression amounts. HOTAIR has been shown to enhance cancer cell energy metabolism in this kind of cancer through increasing HK2 expression (19). UCA1 has been shown to increase mitochondrial function in bladder cancer cells. This lncRNA acts as a molecular sponge for miR-195 to control mitochondrial function through enhancing expression of ARL2. The role of UCA1 through miR-195/ARL2 axis in promotion of bladder tumor growth has been verified in animal models (20). Figure 1 shows a summary of role of UCA1 in the regulation of cancer metabolism.
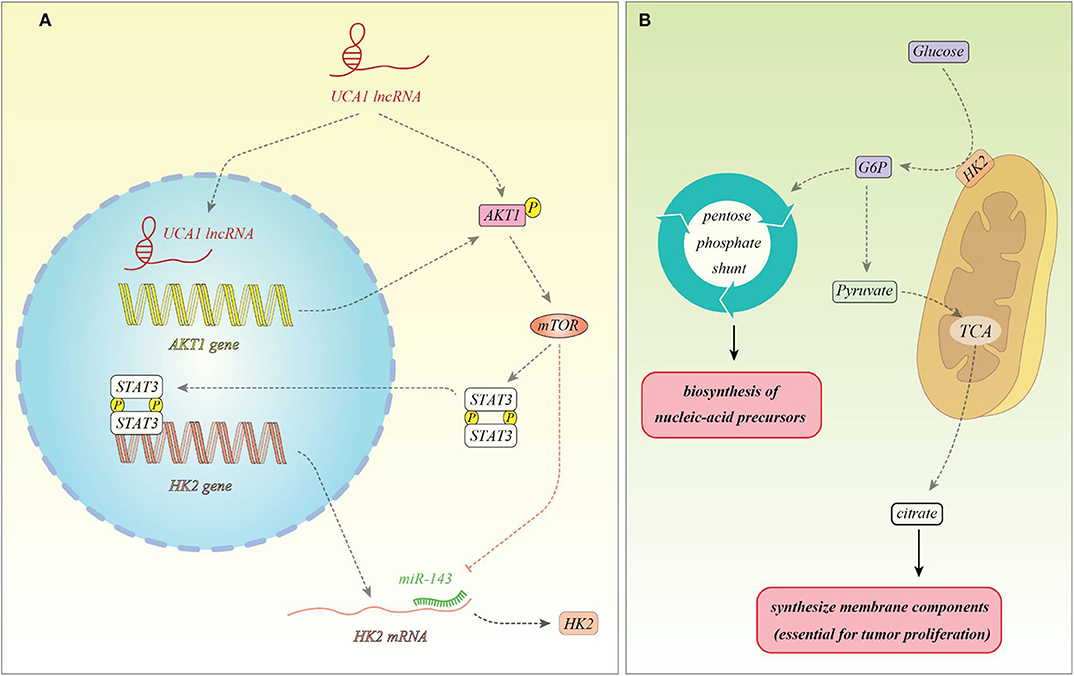
Figure 1. (A) UCA1 can enhance expression of AKT1 and activate it through phosphorylation. AKT1 activates mTOR, thus facilitating STAT3 phosphorylation and nuclear translocation. These events lead to over-expression of HK2. In addition, mTOR inhibits miR-143 which targets HK2. Therefore, mTOR has a prominent effect in enhancing HK2 expression (21). (B) HK2 is located in the mitochondrial membrane and facilitates conversion of glucose to glucose-6-phosphte. Glucose-6-phosphate enters the pentose phosphate pathway to produce pentose phosphate for nucleic acid synthesis which is needed for proliferation of cancer cells. In addition, glucose-6-phosphate can be converted to pyruvate to enter tri-carboxylic acid (TCA) cycle and produce citrate which is needed for the synthesis of membrane components (phospholipids and cholesterol), thus being necessary for tumor proliferation (22).
Table 1 summarizes the role of oncogenic lncRNAs in the cancer metabolism in all kinds of human malignancies.
Tumor Suppressor lncRNAs That Regulate Cancer Metabolism
A number of studies have assessed the association between tumor suppressor lncRNAs and metabolic pathways. NEF as a down-regulated lncRNA in NSCLC tissues has been shown to regulate cell proliferation and glucose uptake in these cells through modulation of GLUT1 expression. Thus, this lncRNA can target glucose transportation to suppress lung tumorigenesis (76). LINC01537 as another tumor suppressor lncRNA has been demonstrated to enhance cellular sensitivity to nilotinib. This lncRNA also targets phosphodiesterase 2A (PDE2A) and enhance it expression through RNA–RNA interaction. Based on the role of PDE2A in energy metabolism, Warburg effect and mitochondrial respiration, LINC01537 has been identified as a regulator of cancer metabolism (77). The lncRNA GASL1 has been shown to enhance Bcl-2 expression, while down-regulating GLUT-1 expression. Thus, the role of this lncRNA in suppression of proliferation of prostate cancer cells has been exerted through modulation of metabolism (78). LINC01554, the down-regulated lncRNA in hepatocellular carcinoma has been shown to be suppressed by miR-365a. This lncRNA enhances the ubiquitin-mediated destruction of PKM2 and suppresses Akt/mTOR signaling pathway to stop aerobic glycolysis in hepatocellular cancer cells (79). FILNC1 has been identified as an energy stress-induced lncRNA. FILNC1 silencing in renal cancer cells lessens energy stress-induced apoptosis and considerably induces progression of this type of cancer. Notably, FILNC1 silencing increases glucose uptake and lactate synthesis via induction of c-Myc. In energy stress conditions, this lncRNA binds with AUF1, a c-Myc interacting protein. Thus, it prevents AUF1 from binding with c-Myc transcript, resulting in under-expression of c-Myc protein (80). Expression of the lncRNA HAND2-AS1 has been decreased in osteosarcoma tissues and serum samples of the affected patients compared with control samples. There was a significant association between serum levels of this lncRNA and tumor size. Notably, in vitro studies revealed that HAND2-AS1-silencing enhances osteosarcoma cell proliferation, upsurges glucose uptake and increases GLUT1 levels. Thus, HAND2-AS1 has a tumor suppressor role in osteosarcoma through modulating glucose metabolism (81). GATA6-AS is another tumor suppressor lncRNA that regulates expression of GLUT1. Up-regulation of this lncRNA has reduced glucose uptake and GLUT1 expression in the mantle cell lymphoma. Thus, the lncRNA GATA6-AS might suppress cancer cell proliferation through decreasing GLUT1 expression (82). The lncRNA MORT has a similar role in suppression of glucose uptake and GLUT1 expression in prostate cancer cell lines (83). In prostate cancer cells, up-regulation of GASL1 has enhanced Bcl-2 expression and decreased GLUT-1 levels (78). CASC8 is also involved in the regulation of the glycolysis in bladder cancer cells through modulating expression of the fibroblast growth factor receptor 1 (FGFR1). The interaction between this lncRNA and FGFR1 has been shown to suppress FGFR1-associated lactate dehydrogenase A phosphorylation, which decreases the lactate synthesis from pyruvate (84). Table 2 summarizes the role of tumor suppressor lncRNAs in the cancer metabolism.
Significance of Metabolism-Related lncRNAs in Cancer Diagnosis and Prognosis
Consistent with the crucial roles of metabolism-related lncRNAs in the evolution of human cancers, dysregulation of these lncRNAs have been associated with patients' outcome. Moreover, transcript levels of them have been exploited as diagnostic markers in diverse cancers. For instance, serum levels of the lncRNA AWPPH have been elevated in patients with colon cancer compared with normal subjects. Receiver operating characteristic (ROC) curve analysis has shown the appropriateness of serum levels of this lncRNA for diagnosis of colon cancer with diagnostic power of 0.84 (37). Serum levels of the lncRNA NEF have been shown to have diagnostic power of 0.94 for NSCLC. Moreover, the overall survival rate of patients with elevated serum levels of this lncRNA was remarkably better compared with those having low level of this lncRNA. Taken together, serum concentrations of NEF might be considered as diagnostic and prognostic markers for this kind of cancer (76). In patients with cholangiocarcinoma, Kaplan-Meier survival analysis has demonstrated decreased overall survival (OS) and disease-free survival (DFS) in patients with high levels of TUG1 expression. Univariate analysis has also verified the effect of TUG1 expression levels in determination of OS and DFS (69). Several other lncRNAs that regulate cancer metabolism have been identified as diagnostic/prognostic markers in cancer. Table 3 summarizes the results of studies which reported diagnostic/prognostic significance of these lncRNAs.
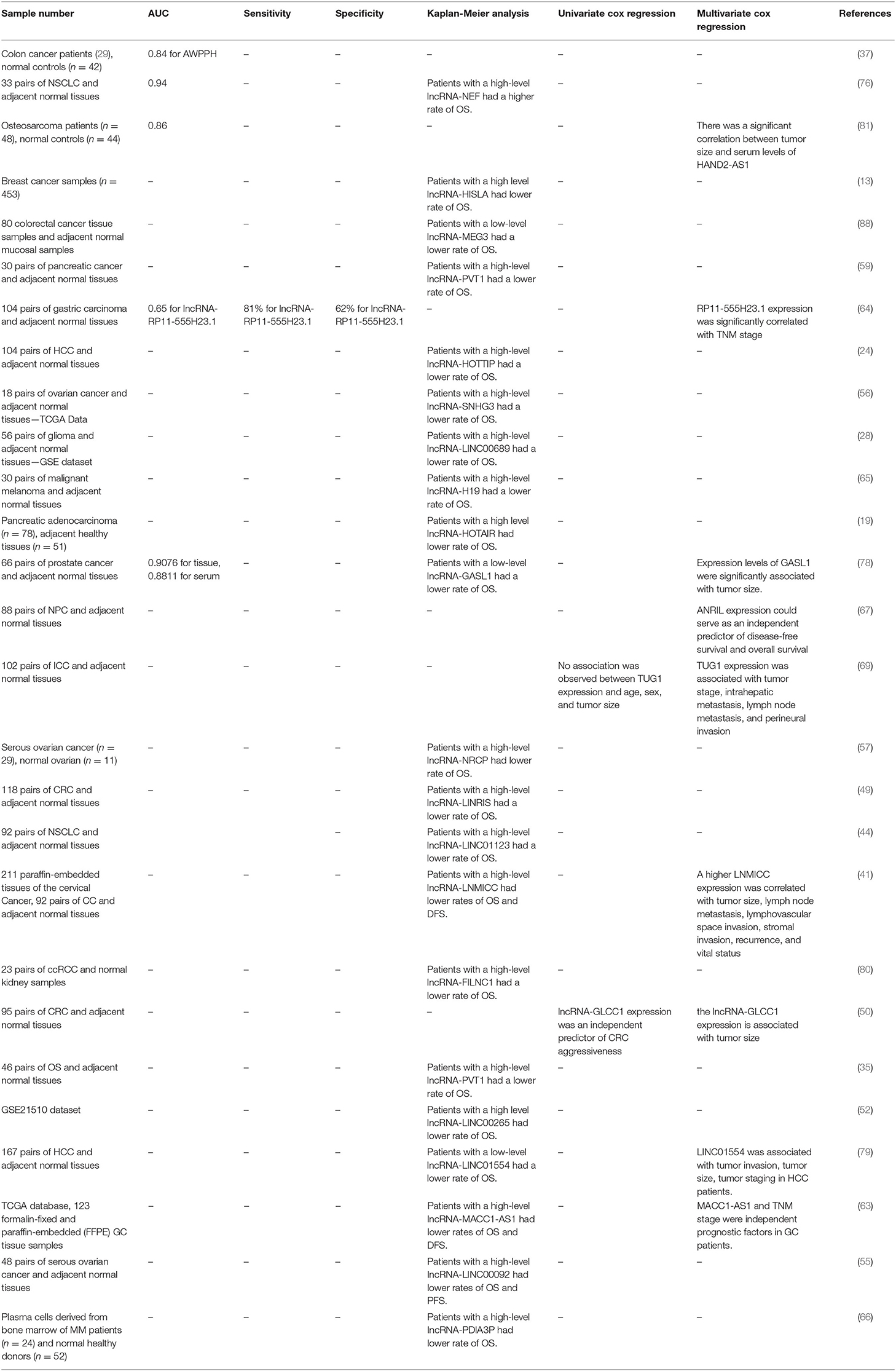
Table 3. The role of metabolism-related lncRNAs in cancer diagnosis and prognosis (DFS, disease free survival; OS, overall survival; PFS, progression free survival).
Discussion
The carcinogenesis process is associated with high glucose uptake, lactate over-production, aerobic glycolysis as well as glutamine and lipid metabolism (89). The above-mentioned data support the role of lncRNAs in these metabolic pathways in the context of cancer. Notably, HCC has been the most investigated cancer type regarding the role of lncRNAs in the metabolic pathways. Apart from the function of lncRNAs in this regard, metabolic changes have been previously recognized to evidently distinguish HCC tumors. Several clinical parameters that are presently utilized to evaluate liver functions reveal alterations in both enzyme activity and metabolites. Actually, alterations in glucose and acetate consumption are regarded as effective clinical means for classification of patients with HCC. Besides, elevated serum lactate can differentiate HCC from healthy individuals, and serum lactate dehydrogenase is applied as a determinant of prognosis of HCC patients under therapeutic regimens (90). Thus, it is not surprising that the role of lncRNAs has been vastly assessed in this context. The underlying mechanism of participation of lncRNAs in the regulation of metabolic pathways has been clarified in several cases. Glucose transporters (GLUTs) as important modulators of glucose utilization which are commonly dysregulated in cancer (91), have been shown to be targeted by several lncRNAs such as LINC01638, Ftx, XIST, YIYA (LINC00538), HISLA, AWPPH, and UCA1. Most notably, several lncRNA/ miRNA/mRNA comprising axes have been shown to modulate cancer metabolism. Therefore, the complex interactions between these trios should be considered in the design of any therapeutic option. Moreover, numerous lncRNAs have direct or indirect interactions with the well-known oncogene c-Myc. This oncogene is an important modulator of pathways that regulate metabolism of glucose, glutamine and lipid in cancer (92). Thus, all of these lncRNAs are putative regulators of different aspects of cancer metabolism.
Several oncogenic lncRNAs mainly exert their effects through modulation of these pathways. Thus, modulation of expression of these lncRNAs through application of antisense oligonucleotides or CRISPR/Cas9-based modalities can be regarded as a therapeutic option in cancer. Yet, the main obstacles in this regard are their off-target effects or unstable efficiency resulting from the space-time related features of lncRNAs (93). Small interfering (si)RNA-mediated silencing of oncogenic lncRNAs has been hampered by unavailability of efficient delivering systems. Yet, this such hurdle has been rather solved by the advent of biocompatible nanoparticle delivery systems (86).
The relevance of metabolism-associated lncRNAs in the treatment of cancer has been highlighted by a number of studies. For instance, the lncRNA-UCA1 has been shown to modulate radioresistance in cervical cancer cell through the HK2/glycolytic pathway (53). The same lncRNA has been shown to be upregulated in AML patients after Adriamycin (ADR)-based chemotherapy. UCA1 silencing has enhanced the cytotoxic effect of this chemotherapeutic agent and suppressed the HIF-1α-associated glycolysis in ADR-resistant AML cells. Based on these results, UCA1 has been shown to exert a positive role in conquering the chemoresistance in pediatric AML patients (72).
Several lncRNAs such as NEF, HISLA, MEG3, PVT1, HOTTIP, SNHG3, LINC00689, H19, HOTAIR, GASL1, NRCP, LINRIS, and FILNC1 have been identified as predictive markers for OS or DFS of cancer patients. The potential of a number of lncRNAs including AWPPH, NEF, HAND2-AS1, lncRNA- RP11-555H23.1, and GASL1 as diagnostic markers in cancer patients has also been verified. These data suggest the importance of these lncRNAs in diverse aspects of cancer biology.
Taken together, regulation of cancer metabolism is a critical role of lncRNAs which has been shown by several in vitro investigations and a number of clinical studies. Thus, these transcripts are putative therapeutic targets in cancer. The importance of this function of lncRNAs is further highlighted by the eminent role of tumor microenvironment in the evolution of cancer and the ubiquitous dysregulation of metabolism in different cancer types. Thus, therapeutic targeting of these lncRNAs can be applied in diverse cancer types.
Author Contributions
SG-F and MT wrote the draft and revised it. HS collected the required information and data. All authors contributed equally and fully aware of submission.
Funding
This study was financially supported by Shahid Beheshti University of Medical Sciences.
Conflict of Interest
The authors declare that the research was conducted in the absence of any commercial or financial relationships that could be construed as a potential conflict of interest.
References
1. Warburg O. On the origin of cancer cells. Science. (1956) 123:309–14. doi: 10.1126/science.123.3191.309
2. Deberardinis RJ, Chandel NS. Fundamentals of cancer metabolism. Sci Adv. (2016) 2:e1600200. doi: 10.1126/sciadv.1600200
3. Vander Heiden MG, Cantley LC, Thompson CB. Understanding the warburg effect: the metabolic requirements of cell proliferation. Science. (2009) 324:1029–33. doi: 10.1126/science.1160809
4. Koppenol WH, Bounds PL, Dang CV. Otto Warburg's contributions to current concepts of cancer metabolism. Nat Rev Cancer. (2011) 11:325–37. doi: 10.1038/nrc3038
5. Hanahan D, Weinberg RA. Hallmarks of cancer: the next generation. Cell. (2011) 144:646–74. doi: 10.1016/j.cell.2011.02.013
6. Boroughs LK, Deberardinis RJ. Metabolic pathways promoting cancer cell survival and growth. Nat Cell Biol. (2015) 17:351–9. doi: 10.1038/ncb3124
7. Lin W, Zhou Q, Wang C-Q, Zhu L, Bi C, Zhang S, et al. LncRNAs regulate metabolism in cancer. Int J Biol Sci. (2020) 16:1194–206. doi: 10.7150/ijbs.40769
8. Iyer MK, Niknafs YS, Malik R, Singhal U, Sahu A, Hosono Y, et al. The landscape of long noncoding RNAs in the human transcriptome. Nat Genet. (2015) 47:199. doi: 10.1038/ng.3192
9. Batista PJ, Chang HY. Long noncoding RNAs: cellular address codes in development and disease. Cell. (2013) 152:1298–307. doi: 10.1016/j.cell.2013.02.012
10. Zhang X, Wang W, Zhu W, Dong J, Cheng Y, Yin Z, et al. Mechanisms and functions of long non-coding RNAs at multiple regulatory levels. Int J Mol Sci. (2019) 20:5573. doi: 10.3390/ijms20225573
11. Li H-J, Li X, Pang H, Pan J-J, Xie X-J, Chen W. Long non-coding RNA UCA1 promotes glutamine metabolism by targeting miR-16 in human bladder cancer. Jpn J Clin Oncol. (2015) 45:1055–63. doi: 10.1093/jjco/hyv132
12. Redis RS, Vela LE, Lu W, De Oliveira JF, Ivan C, Rodriguez-Aguayo C, et al. Allele-specific reprogramming of cancer metabolism by the long non-coding RNA CCAT2. Mol Cell. (2016) 61:520–34. doi: 10.1016/j.molcel.2016.01.015
13. Ma M, Xu H, Liu G, Wu J, Li C, Wang X, et al. Metabolism-induced tumor activator 1 (MITA1), an energy stress–inducible long noncoding RNA, promotes hepatocellular carcinoma metastasis. Hepatology. (2019) 70:215–30. doi: 10.1002/hep.30602
14. Zhou Y, Huang Y, Hu K, Zhang Z, Yang J, Wang Z. HIF1A activates the transcription of lncRNA RAET1K to modulate hypoxia-induced glycolysis in hepatocellular carcinoma cells via miR-100-5p. Cell Death Dis. (2020) 11:1–14. doi: 10.1038/s41419-020-2366-7
15. Xu Y, Luo X, He W, Chen G, Li Y, Li W, et al. Long non-coding RNA PVT1/miR-150/HIG2 axis regulates the proliferation, invasion and the balance of iron metabolism of hepatocellular carcinoma. Cell Physiol Biochem. (2018) 49:1403–19. doi: 10.1159/000493445
16. Sun K, Hu P, Xu F. LINC00152/miR-139-5p regulates gastric cancer cell aerobic glycolysis by targeting PRKAA1. Biomed Pharmacother. (2018) 97:1296–302. doi: 10.1016/j.biopha.2017.11.015
17. Wu D, Zhao B, Cao X, Wan J. Long non-coding RNA LINK-A promotes glioma cell growth and invasion via lactate dehydrogenase A. Oncol Rep. (2017) 38:1525–32. doi: 10.3892/or.2017.5806
18. Hu M, Fu Q, Jing C, Zhang X, Qin T, Pan Y. LncRNA HOTAIR knockdown inhibits glycolysis by regulating miR-130a-3p/HIF1A in hepatocellular carcinoma under hypoxia. Biomed Pharmacother. (2020) 125:109703. doi: 10.1016/j.biopha.2019.109703
19. Ma Y, Hu M, Zhou L, Ling S, Li Y, Kong B, et al. Long non-coding RNA HOTAIR promotes cancer cell energy metabolism in pancreatic adenocarcinoma by upregulating hexokinase-2. Oncol Lett. (2019) 18:2212–9. doi: 10.3892/ol.2019.10551
20. Li H-J, Sun X-M, Li Z-K, Yin Q-W, Pang H, Pan J-J, et al. LncRNA UCA1 promotes mitochondrial function of bladder cancer via the MiR-195/ARL2 signaling pathway. Cell Physio Biochem. (2017) 43:2548–61. doi: 10.1159/000484507
21. Li Z, Li X, Wu S, Xue M, Chen W. Long non-coding RNA UCA1 promotes glycolysis by upregulating hexokinase 2 through the mTOR–STAT3/microRNA143 pathway. Cancer Sci. (2014) 105:951–5. doi: 10.1111/cas.12461
22. Mathupala S, Ko YA, Pedersen PL. Hexokinase II: cancer's double-edged sword acting as both facilitator and gatekeeper of malignancy when bound to mitochondria. Oncogene. (2006) 25:4777–86. doi: 10.1038/sj.onc.1209603
23. Chen X, Wang L, Wang H. LINC01638 lncRNA promotes cancer cell proliferation in hepatocellular carcinoma by increasing cancer cell glucose uptake. Oncol Lett. (2019) 18:3811–6. doi: 10.3892/ol.2019.10682
24. Staff TPG. Correction: fMiRNA-192 and miRNA-204 directly suppress lncRNA HOTTIP and interrupt GLS1-mediated glutaminolysis in hepatocellular carcinoma. PLoS Genet. (2016) 12:e1005825. doi: 10.1371/journal.pgen.1005825
25. Li X, Zhao Q, Qi J, Wang W, Zhang D, Li Z, et al. lncRNA Ftx promotes aerobic glycolysis and tumor progression through the PPARγ pathway in hepatocellular carcinoma. Int J Oncol. (2018) 53:551–66. doi: 10.3892/ijo.2018.4418
26. Cheng Z, Luo C, Guo Z. LncRNA-XIST/microRNA-126 sponge mediates cell proliferation and glucose metabolism through the IRS1/PI3K/Akt pathway in glioma. J Cell Biochem. (2019) 121:2170–83. doi: 10.1002/jcb.29440
27. He Z, You C, Zhao D. Long non-coding RNA UCA1/miR-182/PFKFB2 axis modulates glioblastoma-associated stromal cells-mediated glycolysis and invasion of glioma cells. Biochem Biophys Res Commun. (2018) 500:569–76. doi: 10.1016/j.bbrc.2018.04.091
28. Liu X, Zhu Q, Guo Y, Xiao Z, Hu L, Xu Q. LncRNA LINC00689 promotes the growth, metastasis and glycolysis of glioma cells by targeting miR-338-3p/PKM2 axis. Biomed Pharmacother. (2019) 117:109069. doi: 10.1016/j.biopha.2019.109069
29. Xing Z, Zhang Y, Liang K, Yan L, Xiang Y, Li C, et al. Expression of long noncoding RNA YIYA promotes glycolysis in breast cancer. Cancer Res. (2018) 78:4524–32. doi: 10.1158/0008-5472.CAN-17-0385
30. Zheng X, Han H, Liu GP, Ma YX, Pan RL, Sang LJ, et al. LncRNA wires up hippo and hedgehog signaling to reprogramme glucose metabolism. EMBO J. (2017) 36:3325–35. doi: 10.15252/embj.201797609
31. Zhang L, Fu Y, Guo H. c-Myc-induced long non-coding RNA small nucleolar RNA host gene 7 regulates glycolysis in breast cancer. J Breast Cancer. (2019) 22:533–47. doi: 10.4048/jbc.2019.22.e54
32. Ma F, Liu X, Zhou S, Li W, Liu C, Chadwick M, et al. Long non-coding RNA FGF13-AS1 inhibits glycolysis and stemness properties of breast cancer cells through FGF13-AS1/IGF2BPs/Myc feedback loop. Cancer Lett. (2019) 450:63–75. doi: 10.1016/j.canlet.2019.02.008
33. Han X, Yang Y, Sun Y, Qin L, Yang Y. LncRNA TUG1 affects cell viability by regulating glycolysis in osteosarcoma cells. Gene. (2018) 674:87–92. doi: 10.1016/j.gene.2018.06.085
34. Kang Y, Zhu X, Xu Y, Tang Q, Huang Z, Zhao Z, et al. Energy stress-induced lncRNA HAND2-AS1 represses HIF1α-mediated energy metabolism and inhibits osteosarcoma progression. Am J Cancer Res. (2018) 8:526.
35. Song J, Wu X, Liu F, Li M, Sun Y, Wang Y, et al. Long non-coding RNA PVT1 promotes glycolysis and tumor progression by regulating miR-497/HK2 axis in osteosarcoma. Biochem Biophys Res Commun. (2017) 490:217–24. doi: 10.1016/j.bbrc.2017.06.024
36. Zhang G, Ma A, Jin Y, Pan G, Wang C. LncRNA SNHG16 induced by TFAP2A modulates glycolysis and proliferation of endometrial carcinoma through miR-490-3p/HK2 axis. Am J Transl Res. (2019) 11:7137.
37. Bai J, Xu J, Zhao J, Zhang R. Downregulation of lncRNA AWPPH inhibits colon cancer cell proliferation by downregulating GLUT-1. Oncol Lett. (2019) 18:2007–12. doi: 10.3892/ol.2019.10515
38. Wang L, Cao L, Wen C, Li J, Yu G, Liu C. LncRNA LINC00857 regulates lung adenocarcinoma progression, apoptosis and glycolysis by targeting miR-1179/SPAG5 axis. Human Cell. (2020) 33:195–204. doi: 10.1007/s13577-019-00296-8
39. Yang B, Zhang L, Cao Y, Chen S, Cao J, Wu D, et al. Overexpression of lncRNA IGFBP4–1 reprograms energy metabolism to promote lung cancer progression. Mol Cancer. (2017) 16:154. doi: 10.1186/s12943-017-0722-8
40. Wang X, Fa X-E. Knockdown of UCA1 inhibits viability and glycolysis by suppressing PKM2 expression through the mTOR pathway in non-small cell lung cancer cells. RSCAdv. (2018) 8:10610–9. doi: 10.1039/C8RA00860D
41. Shang C, Wang W, Liao Y, Chen Y, Liu T, Du Q, et al. LNMICC promotes nodal metastasis of cervical cancer by reprogramming fatty acid metabolism. Cancer Res. (2018) 78:877–90. doi: 10.1158/0008-5472.CAN-17-2356
42. Gao W, Weng T, Wang L, Shi B, Meng W, Wang X, et al. Long non-coding RNA NORAD promotes cell proliferation and glycolysis in non-small cell lung cancer by acting as a sponge for miR-136-5p. Mol Med Rep. (2019) 19:5397–405. doi: 10.3892/mmr.2019.10210
43. Lang N, Wang C, Zhao J, Shi F, Wu T, Cao H. Long non-coding RNA BCYRN1 promotes glycolysis and tumor progression by regulating the miR-149/PKM2 axis in non-small-cell lung cancer. Mol Med Rep. (2020) 21:1509–16. doi: 10.3892/mmr.2020.10944
44. Hua Q, Jin M, Mi B, Xu F, Li T, Zhao L, et al. LINC01123, a c-Myc-activated long non-coding RNA, promotes proliferation and aerobic glycolysis of non-small cell lung cancer through miR-199a-5p/c-Myc axis. J Hematol Oncol. (2019) 12:1–18. doi: 10.1186/s13045-019-0773-y
45. Gu S, Niu X, Mao F, Xu Z. Long noncoding RNA PCA3 regulates glycolysis, viability and apoptosis by mediating the miR-1/CDK4 axis in prostate cancer. RSC Adv. (2018) 8:37564–72. doi: 10.1039/C8RA08083F
46. Shao M, Yu Z, Zou J. LncRNA-SNHG16 silencing inhibits prostate carcinoma cell growth, downregulate glut1 expression and reduce glucose uptake. Cancer Manag Res. (2020) 12:1751. doi: 10.2147/CMAR.S231370
47. Hung C-L, Wang L-Y, Yu Y-L, Chen H-W, Srivastava S, Petrovics G, et al. A long noncoding RNA connects c-Myc to tumor metabolism. Proc Natl Acad Sci USA. (2014) 111:18697–702. doi: 10.1073/pnas.1415669112
48. Christensen LL, True K, Hamilton MP, Nielsen MM, Damas ND, Damgaard CK, et al. SNHG16 is regulated by the Wnt pathway in colorectal cancer and affects genes involved in lipid metabolism. Mol Oncol. (2016) 10:1266–82. doi: 10.1016/j.molonc.2016.06.003
49. Wang Y, Lu J-H, Wu Q-N, Jin Y, Wang D-S, Chen Y-X, et al. LncRNA LINRIS stabilizes IGF2BP2 and promotes the aerobic glycolysis in colorectal cancer. Mol Cancer. (2019) 18:1–18. doi: 10.1186/s12943-019-1105-0
50. Tang J, Yan T, Bao Y, Shen C, Yu C, Zhu X, et al. LncRNA GLCC1 promotes colorectal carcinogenesis and glucose metabolism by stabilizing c-Myc. Nat Commun. (2019) 10:1–15. doi: 10.1038/s41467-019-11447-8
51. Cui S, Yang X, Zhang L, Zhao Y, Yan W. LncRNA MAFG-AS1 promotes the progression of colorectal cancer by sponging miR-147b and activation of NDUFA4. Biochem Biophys Res Commun. (2018) 506:251–8. doi: 10.1016/j.bbrc.2018.10.112
52. Sun S, Li W, Ma X, Luan H. Long noncoding RNA LINC00265 promotes glycolysis and lactate production of colorectal cancer through regulating of miR-216b-5p/TRIM44 axis. Digestion. (2019) 101:3911–400. doi: 10.1159/000500195
53. Fan L, Huang C, Li J, Gao T, Lin Z, Yao T. Long non-coding RNA urothelial cancer associated 1 regulates radioresistance via the hexokinase 2/glycolytic pathway in cervical cancer. Int J Mol Med. (2018) 42:2247–59. doi: 10.3892/ijmm.2018.3778
54. Wu F, Zhou D, Cui Y, Shen G, Li Y, Wei F. Long non-coding RNA UCA1 modulates the glycolysis of cervical cancer cells by miR-493-5p/HK2. Int J Clin Exp Pathol. (2018) 11:3943.
55. Zhao L, Ji G, Le X, Wang C, Xu L, Feng M, et al. Long noncoding RNA LINC00092 acts in cancer-associated fibroblasts to drive glycolysis and progression of ovarian cancer. Cancer Res. (2017) 77:1369–82. doi: 10.1158/0008-5472.CAN-16-1615
56. Li N, Zhan X, Zhan X. The lncRNA SNHG3 regulates energy metabolism of ovarian cancer by an analysis of mitochondrial proteomes. Gynecol Oncol. (2018) 150:343–54. doi: 10.1016/j.ygyno.2018.06.013
57. Rupaimoole R, Lee J, Haemmerle M, Ling H, Previs RA, Pradeep S, et al. Long noncoding RNA ceruloplasmin promotes cancer growth by altering glycolysis. Cell Rep. (2015) 13:2395–402. doi: 10.1016/j.celrep.2015.11.047
58. He J, Li F, Zhou Y, Hou X, Liu S, Li X, et al. LncRNA XLOC_006390 promotes pancreatic carcinogenesis and glutamate metabolism by stabilizing c-Myc. Cancer Lett. (2020) 469:419–28. doi: 10.1016/j.canlet.2019.11.021
59. Sun J, Zhang P, Yin T, Zhang F, Wang W. Upregulation of LncRNA PVT1 facilitates pancreatic ductal adenocarcinoma cell progression and glycolysis by regulating MiR-519d-3p and HIF-1A. J Cancer. (2020) 11:2572. doi: 10.7150/jca.37959
60. Liu J, Liu Z-X, Wu Q-N, Lu Y-X, Wong C-W, Ju H-Q, et al. IDDF2019-ABS-0200 Long non-coding RNA LOC148709 regulates PFKFB3-mediated glycolytic reprogramming in esophageal squamous cell carcinoma. BMJ. (2019) 68:1354–66. doi: 10.1136/gutjnl-2019-IDDFAbstracts.37
61. Li D, Cheng M, Niu Y, Chi X, Liu X, Fan J, et al. Identification of a novel human long non-coding RNA that regulates hepatic lipid metabolism by inhibiting SREBP-1c. Int J Biol Sci. (2017) 13:349. doi: 10.7150/ijbs.16635
62. Liu G, Zheng X, Xu Y, Lu J, Chen J, Huang X. Long non-coding RNAs expression profile in HepG2 cells reveals the potential role of long non-coding RNAs in the cholesterol metabolism. Chinese Med J. (2015) 128:91. doi: 10.4103/0366-6999.147824
63. Zhao Y, Liu Y, Lin L, Huang Q, He W, Zhang S, et al. The lncRNA MACC1-AS1 promotes gastric cancer cell metabolic plasticity via AMPK/Lin28 mediated mRNA stability of MACC1. Mol Cancer. (2018) 17:69. doi: 10.1186/s12943-018-0820-2
64. Mo X, Wu Y, Chen L, Zhai M, Gao Z, Hu K, et al. Global expression profiling of metabolic pathway-related lncRNAs in human gastric cancer and the identification of RP11-555H23. 1 as a new diagnostic biomarker. J Clin Lab Anal. (2019) 33:e22692. doi: 10.1002/jcla.22692
65. Luan W, Zhou Z, Ni X, Xia Y, Wang J, Yan Y, et al. Long non-coding RNA H19 promotes glucose metabolism and cell growth in malignant melanoma via miR-106a-5p/E2F3 axis. J Cancer Res Clin Oncol. (2018) 144:531–42. doi: 10.1007/s00432-018-2582-z
66. Yang X, Ye H, He M, Zhou X, Sun N, Guo W, et al. LncRNA PDIA3P interacts with c-Myc to regulate cell proliferation via induction of pentose phosphate pathway in multiple myeloma. Biochem Biophys Res Commun. (2018) 498:207–13. doi: 10.1016/j.bbrc.2018.02.211
67. Zou ZW, Ma C, Medoro L, Chen L, Wang B, Gupta R, et al. LncRNA ANRIL is up-regulated in nasopharyngeal carcinoma and promotes the cancer progression via increasing proliferation, reprograming cell glucose metabolism and inducing side-population stem-like cancer cells. Oncotarget. (2016) 7:61741. doi: 10.18632/oncotarget.11437
68. Zhao C, Bai X, Hu X. Knockdown of lncRNA XIST inhibits hypoxia-induced glycolysis, migration and invasion through regulating miR-381-3p/NEK5 axis in nasopharyngeal carcinoma. Eur Rev Med Pharmacol Sci. (2020) 24:2505–17. doi: 10.26355/eurrev_202003_20518
69. Zeng B, Ye H, Chen J, Cheng D, Cai C, Chen G, et al. LncRNA TUG1 sponges miR-145 to promote cancer progression and regulate glutamine metabolism via Sirt3/GDH axis. Oncotarget. (2017) 8:113650. doi: 10.18632/oncotarget.21922
70. Chu H, Li Z, Gan Z, Yang Z, Wu Z, Rong M. LncRNA ELF3-AS1 is involved in the regulation of oral squamous cell carcinoma cell proliferation by reprogramming glucose metabolism. OncoTargets Ther. (2019) 12:6857. doi: 10.2147/OTT.S217473
71. Wang Y, Zhang X, Wang Z, Hu Q, Wu J, Li Y, et al. LncRNA-p23154 promotes the invasion-metastasis potential of oral squamous cell carcinoma by regulating Glut1-mediated glycolysis. Cancer Lett. (2018) 434:172–83. doi: 10.1016/j.canlet.2018.07.016
72. Zhang Y, Liu Y, Xu X. Knockdown of LncRNA-UCA1 suppresses chemoresistance of pediatric AML by inhibiting glycolysis through the microRNA-125a/hexokinase 2 pathway. J Cell Biochem. (2018) 119:6296–308. doi: 10.1002/jcb.26899
73. Sun L-Y, Li X-J, Sun Y-M, Huang W, Fang K, Han C, et al. LncRNA ANRIL regulates AML development through modulating the glucose metabolism pathway of AdipoR1/AMPK/SIRT1. Mol Cancer. (2018) 17:127. doi: 10.1186/s12943-018-0879-9
74. Wang Y. Upregulated lncRNA-HNGA1, a target of miR-375, contributes to aerobic glycolysis of head and neck squamous cell carcinoma through increasing levels of the glucose transporter protein SCL2A1. Eur J Cancer. (2016) 61:S14–S5. doi: 10.1016/S0959-8049(16)61039-0
75. Liu X, Gan B. lncRNA NBR2 modulates cancer cell sensitivity to phenformin through GLUT1. Cell Cycle. (2016) 15:3471–81. doi: 10.1080/15384101.2016.1249545
76. Chang L, Xu W, Zhang Y, Gong F. Long non-coding RNA-NEF targets glucose transportation to inhibit the proliferation of non-small-cell lung cancer cells. Oncol Letters. (2019) 17:2795–801. doi: 10.3892/ol.2019.9919
77. Gong W, Yang L, Wang Y, Xian J, Qiu F, Liu L, et al. Analysis of survival-related lncRNA landscape identifies a role for LINC01537 in energy metabolism and lung cancer progression. Int J Mol Sci. (2019) 20:3713. doi: 10.3390/ijms20153713
78. Li Z, Liu H, Ju W, Xing Y, Zhang X, Yang J. LncRNA GASL1 inhibits growth and promotes expression of apoptosis-associated proteins in prostate carcinoma cells through GLUT-1. Oncol Lett. (2019) 17:5327–34. doi: 10.3892/ol.2019.10244
79. Zheng Y-L, Li L, Jia Y-X, Zhang B-Z, Li J-C, Zhu Y-H, et al. LINC01554-mediated glucose metabolism reprogramming suppresses tumorigenicity in hepatocellular carcinoma via downregulating PKM2 expression and inhibiting Akt/mTOR signaling pathway. Theranostics. (2019) 9:796. doi: 10.7150/thno.28992
80. Xiao Z-D, Han L, Lee H, Zhuang L, Zhang Y, Baddour J, et al. Energy stress-induced lncRNA FILNC1 represses c-Myc-mediated energy metabolism and inhibits renal tumor development. Nat Commun. (2017) 8:1–13. doi: 10.1038/s41467-017-00902-z
81. Chen S, Xu X, Lu S, Hu B. Long non-coding RNA HAND2-AS1 targets glucose metabolism and inhibits cancer cell proliferation in osteosarcoma. Oncol Lett. (2019) 18:1323–9. doi: 10.3892/ol.2019.10445
82. Fan Z, Wang X, Li P, Mei C, Zhang M, Zhao C. Overexpression of lncRNA GATA6-AS inhibits cancer cell proliferation in mantle cell lymphoma by downregulating GLUT1. Oncol Lett. (2019) 18:2443–7. doi: 10.3892/ol.2019.10540
83. Shi Z, Guo F, Jia D, Huang J, Chen J, Sun M, et al. Long non-coding RNA mortal obligate RNA transcript suppresses tumor cell proliferation in prostate carcinoma by inhibiting glucose uptake. Oncol Lett. (2019) 18:3787–91. doi: 10.3892/ol.2019.10711
84. Hu R, Zhong P, Xiong L, Duan L. Long noncoding RNA cancer susceptibility candidate 8 suppresses the proliferation of bladder cancer cells via regulating glycolysis. DNA Cell Biol. (2017) 36:767–74. doi: 10.1089/dna.2017.3785
85. Mo X, Li T, Xie Y, Zhu L, Xiao B, Liao Q, et al. Identification and functional annotation of metabolism-associated lnc RNA s and their related protein-coding genes in gastric cancer. Mol Genet Genom Med. (2018) 6:728–38. doi: 10.1002/mgg3.427
86. Liu X, Xiao Z-D, Han L, Zhang J, Lee S-W, Wang W, et al. LncRNA NBR2 engages a metabolic checkpoint by regulating AMPK under energy stress. Nat Cell Biol. (2016) 18:431–42. doi: 10.1038/ncb3328
87. Liao M, Liao W, Xu N, Li B, Liu F, Zhang S, et al. LncRNA EPB41L4A-AS1 regulates glycolysis and glutaminolysis by mediating nucleolar translocation of HDAC2. EBioMedicine. (2019) 41:200–13. doi: 10.1016/j.ebiom.2019.01.035
88. Zuo S, Wu L, Wang Y, Yuan X. Long non-coding RNA MEG3 activated by vitamin d suppresses glycolysis in colorectal cancer via promoting c-myc degradation. Front Oncol. (2020) 10:274. doi: 10.3389/fonc.2020.00274
89. Jones RG, Thompson CB. Tumor suppressors and cell metabolism: a recipe for cancer growth. Genes Dev. (2009) 23:537–48. doi: 10.1101/gad.1756509
90. De Matteis S, Ragusa A, Marisi G, De Domenico S, Casadei Gardini A, Bonafè M, et al. Aberrant metabolism in hepatocellular carcinoma provides diagnostic and therapeutic opportunities. Oxidat Med Cell Longev. (2018) 2018:7512159. doi: 10.1155/2018/7512159
91. Adekola K, Rosen ST, Shanmugam M. Glucose transporters in cancer metabolism. Curr Opin Oncol. (2012) 24:650–4. doi: 10.1097/CCO.0b013e328356da72
92. Liu H, Luo J, Luan S, He C, Li Z. Long non-coding RNAs involved in cancer metabolic reprogramming. Cell Mol Life Sci. (2019) 76:495–504. doi: 10.1007/s00018-018-2946-1
Keywords: lncRNA, cancer metabolism, expression, biomarker, oncogene
Citation: Ghafouri-Fard S, Shoorei H and Taheri M (2020) The Role of Long Non-coding RNAs in Cancer Metabolism: A Concise Review. Front. Oncol. 10:555825. doi: 10.3389/fonc.2020.555825
Received: 26 April 2020; Accepted: 24 August 2020;
Published: 06 October 2020.
Edited by:
Xiao-Bin Lv, Third Affiliated Hospital of Nanchang University, ChinaReviewed by:
Krishna Beer Singh, University of Pittsburgh, United StatesQiyin Zhou, Zhejiang University, China
Kamla Kant Shukla, All India Institute of Medical Sciences Jodhpur, India
Copyright © 2020 Ghafouri-Fard, Shoorei and Taheri. This is an open-access article distributed under the terms of the Creative Commons Attribution License (CC BY). The use, distribution or reproduction in other forums is permitted, provided the original author(s) and the copyright owner(s) are credited and that the original publication in this journal is cited, in accordance with accepted academic practice. No use, distribution or reproduction is permitted which does not comply with these terms.
*Correspondence: Mohammad Taheri, bW9oYW1tYWRfODIzQHlhaG9vLmNvbQ==