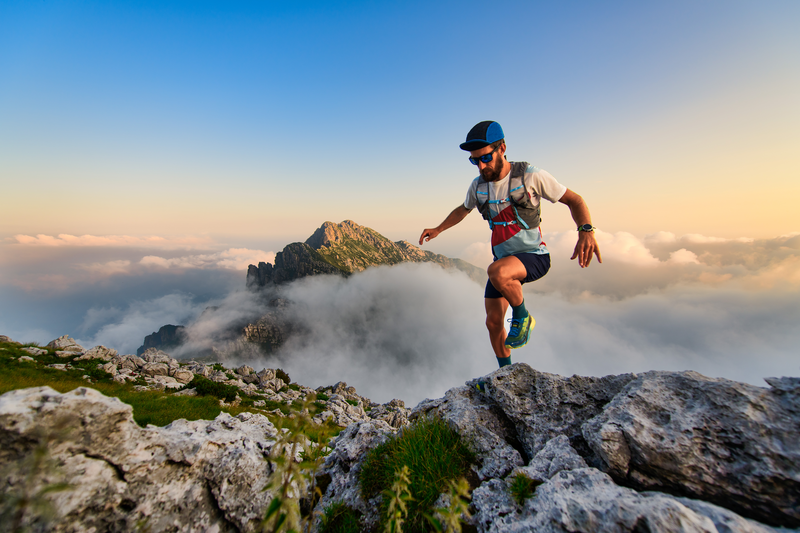
94% of researchers rate our articles as excellent or good
Learn more about the work of our research integrity team to safeguard the quality of each article we publish.
Find out more
ORIGINAL RESEARCH article
Front. Oncol. , 15 September 2020
Sec. Radiation Oncology
Volume 10 - 2020 | https://doi.org/10.3389/fonc.2020.528686
This article is part of the Research Topic Modeling for Prediction of Radiation-Induced Toxicity to Improve Therapeutic Ratio in the Modern Radiation Therapy Era View all 35 articles
Radiation induced lung fibrosis (RILF) is a common late complication after radiotherapy without effective treatment. Thyroid hormone (TH) is known to reverse bleomycin-induced pulmonary fibrosis in recent study. We therefore sought to examine TH effect in RILF. Aerosolized TH delivery prevented pulmonary fibrosis according to either micro-computed tomography scans or histological evaluations, without significant changes in serum THs in a murine model of RILF by attenuating TGF-β1 and phosphorylated Smad2/3 expressions and reducing the accumulation of M2-like macrophages. Furthermore, hypothyroidism was significantly correlated with RILF in a retrospectively analyzed data from nasopharyngeal carcinoma patients treated by intensity-modulated radiation therapy with a median follow-up time of 25.5 months. Together, aerosolized TH may prevent RILF by inhibiting the TGF-β1/SMADs signaling pathway.
Radiotherapy is widely used in tumor treatment. More than 50 percent of malignant cancer patients require radiation therapy for both curative and palliative purposes (1). However, the application of radiotherapy is limited by the radiation-induced lung injury (RILI), which is a common complication after radiotherapy of thoracic malignant tumors. RILI often causes early radiation pneumonitis and late-onset radiation induced lung fibrosis (RILF) usually occurs in 1 year after radiotherapy (2). The incidence of symptomatic RILF in patients receiving thoracic radiotherapy is about 5–24%, and higher in patients with subclinical damage (3). Because effective treatments are lacking, RILF has an adverse impact on the quality of life of suffering patients, causing cough, shortness of breath, fever, progressive respiratory dysfunction, or even death (4–6). Currently, corticosteroids are commonly used in the clinic for radiation induced lung injury. Several prevention strategies have shown certain promising effects in murine models of RILF, including treatments with amifostine, ACE inhibitors, angiotensin II receptor inhibitors, pentoxifylline as well as with inhibitors of PDGF, VEGF, FGF, TGF-β1, and Cox-2, but firm clinical evidence is lacking (7–9).
Thyroid hormone is one of the most important hormones that regulate energy metabolism. The levels of active triiodothyronine and its precursor thyroxine (T4) are mainly regulated by DIO2 in cells and tissues (10). There is growing evidence of the correlation between thyroid function and fibrosis disease in patients. Grazinao (11) found that patients with idiopathic retroperitoneal fibrosis (IRF) had a higher risk of hypothyroidism than controls (OR = 3.56, 95%CI 1.48–8.59, and P = 0.004). Nearly a quarter of IRF patients received L-thyroxine at the end of the follow-up (median, 45 month), but only 3% of controls needed treatment in the same period. The incidence of hypothyroidism in idiopathic pulmonary fibrosis (IPF) was 16.8%, compared with 7.1% in control group; multivariate analysis showed that hypothyroidism was an independent predictor of death risk in IPF patients (12). Clinical data from a large sample showed that hypothyroidism significantly correlated with non-alcoholic fatty liver fibrosis (OR = 2.23, 95%CI 1.18–4.23, and P = 0.014) (13). Hypothyroidism also plays an important role in myocardial fibrosis (14) and diabetic nephropathy (15).
Furthermore, recent studies have shown that TH may have a therapeutic effect in acute lung injury (16, 17). Yu et al. (18) demonstrated that TH significantly attenuated adverse signs in a mouse model of bleomycin-induced pulmonary fibrosis by restoring epithelial mitochondrial function. TH efficacy was higher than that of pirfenidone and nintedanib, which are currently approved for IPF by the US Food and Drug Administration. That study suggested that in contrast to the expensive drugs mentioned above, TH may have broad application prospects in IPF. However, the role of TH in RILF has not been investigated so far.
It is generally accepted that TGF-β1 (19, 20) and the polarization of M2 phenotype macrophages (21, 22) play critical roles in multiple organ fibrosis as well as in RILF. Therefore, inhibition of the TGF-β1 signaling pathway may be a plausible strategy to alleviate RILF. It has been reported that TH significantly reduced liver fibrosis and skin fibrosis in mice by inhibiting transcriptional activation evoked by TGF-β/SMAD (23). Based on this evidence, we explored potential protective effects of TH in RILF in the present study.
Prednisone (PDN, HY-B0214) and 3,3′,5-triiodo-L-thyronine (T3, HY-A0070A) were purchased from MedChemExpress (Monmouth Junction, NJ, United States) and formulated in dimethyl sulfoxide solution. RILF was modeled in female C57BL/6 mice because they were likely to develop lung fibrosis (24). A total of 60 female C57BL/6J mice (Experimental Animal Center of Hubei province, China) aged 6–8 weeks were randomly assigned to five groups: control group (n = 12), aerosolized T3 group (n = 12), radiation treatment (RT) group (n = 12), RT + T3 group (n = 12), and RT + PDN (n = 12). Drug delivery of T3 or PDN was started on the first day of RT and continued daily for 1 month after RT. Each time, six mice were simultaneously exposed to a nebulizer chamber where T3 suspension was aerosolized at a dose of 40 μg/kg in 6 mL of phosphate buffered saline by an ordinary aerosol nebulizer (Omron) until atomization stopped (18). PDN was given intraperitoneally at 5 mg/kg. Mice developed pulmonary fibrosis after RT at a single dose of 16 Gy, as previously described (25). Mice were maintained in the specific pathogen-free animal facility of the Huazhong University of Science and Technology.
At weeks 6, 16, and 25 after radiation, three mice per group were randomly selected and sacrificed for thorax CT with a micro-CT scanner (Skyscan 1176, Bruker Inc, Billerica, MA, United States). The obtained CT images were imported into RadiAnt DICOM Viewer 3.4.11. Lung slice image analysis was performed in the most typical slice that exhibited pulmonary fibrosis features. Four regions per slice were selected as measurement points: anterior and posterior in right and left lungs. Quantitative lung density of one mouse was represented as the mean Hounsfield unit (HU) value ± standard error of the mean (SEM).
Lung histological analysis was conducted as previously described (25). Briefly, three mice per group were euthanized for histology on the days of micro-CT chest scans. 25 weeks post RT, all remaining mice were killed by cervical dislocation. The left lungs were fixed in 4% paraformaldehyde for 24 h, embedded in paraffin, then cut into 5 μm thick slices and stained with hematoxylin-eosin stain and Masson’s trichrome stain for collagen deposition.
The degree of pulmonary fibrosis was assessed by the modified Ashcroft score (26) on a range from 0 to 8 by examining five random microscopic fields at 200 × magnification. The final fibrosis degree was determined by an average score of all fields.
Expression of proteins was examined by immunohistochemistry (IHC) using staining with antibodies against αSMA (1:100, 14395-1-AP, Proteintech, Wuhan, China), F4/80 (1:400, ab111101, Abcam, United Kingdom), CD163 (1:200, ab182422, Abcam), iNOS (1:400, ab15323, Abcam), CD206 (1:200, ab64693, Abcam), and DIO2 (1:200, 26513-1-AP, Proteintech) according to previously described standard procedures (25). IHC results were semi-quantitatively analyzed. In brief, each sample was scored by combining immunoreactive signal intensity (negative = 0, weak = 1, moderate = 2, and strong = 3) and the percentage of positively stained cells (<10% positive cells = 0, 10%–30% positive cells = 1, 30%–50% positive cells = 2, and >50% positive cells = 3). All slides were individually analyzed by two pathologists, using a light microscope. All histological samples were randomly numbered in a blinded fashion to avoid observer bias.
Human lung samples were collected from the Department of Pathology of the Tongji Hospital, guided by Ethics Board at the hospital without the need for specific consent. Lung samples were obtained from pulmonary malignancy patients who underwent surgery more than 6 months after radiotherapy. Surgical lung specimens from patients that did not receive radiotherapy at the same period were included as control. All patients were operated at the Department of Thoracic Surgery in our hospital.
In order to explore the role of hypothyroidism in RILF, we retrospectively analyzed data from nasopharyngeal carcinoma patients (NPC) treated by intensity-modulated radiation therapy (IMRT) at the Tongji Hospital of the Huazhong University of Science and Technology between January 2010 and December 2018, for thyroid dysfunction is prevalent after radiotherapy in NPC. The bilateral upper lungs were delineated on computed tomography (CT) scans, and lung doses were calculated. Eligible cases had to meet the following inclusion criteria: confirmed NPC without lung metastasis was present before radiotherapy; IMRT was conducted in our hospital, thus complete information was available; thyroid function was examined before and after IMRT; patients with hypothyroidism before IMRT were also included in the analysis; patients had a series of chest CT scans in the follow-up every 2–3 months within 2 years after treatment, and every 3–6 months later, finally annually after 5 years. We excluded subjects who had no irradiation dose in lungs, were diagnosed with connective tissue diseases, or were followed for less than 3 months. We restaged all patients using the 7th American Joint Committee on Cancer staging system. RILI was confirmed according to Common Terminology Criteria for Adverse Events (version 4.0).
Total serum TH in NPC patients, including thyroid-stimulating hormone (TSH), free triiodothyronine (fT3), and free thyroxine (fT4) were measured in the Laboratory Department of our hospital by using the electrochemiluminescence immunoassay. The normal values for TSH, fT3, and fT4 were considered to be 0.35–4.94 μIU/mL, 1.71–3.71 pg/mL, and 0.7–1.48 ng/dL, respectively. The presence of abnormally elevated TSH in the serum was defined as hypothyroidism, with or without decreases in fT4.
Serum samples were prepared from mouse blood collected from eyelids using BD vacutainers on the days of micro-CT chest scans. In the remaining mice, serum was obtained at week 25 after RT using the same method. Total serum T3, T4, and TSH levels were measured using a T3 (ELK1339, ELK Biotechnology, Wuhan, China), T4 (ELK1204, ELK Biotechnology), and TSH (ELK2284, ELK Biotechnology) ELISA kits, following the manufacturers’ protocols.
Right lung hydroxyproline level was analyzed with a hydroxyproline colorimetric assay kit (A030-2, Nanjing Jiancheng Bioengineering Institute, Nanjing, China) to assess collagen content as previously described (25).
Total protein was extracted from mouse left lung tissue, and its concentration was determined using the bicinchoninic acid assay. Then the constituent proteins were separated by electrophoresis in a 10% sodium dodecyl sulfate polyacrylamide gel, transferred to polyvinylidene fluoride membranes (Millipore, Billerica, MA, United States), covered with 5% milk at room temperature for 1 h, and incubated overnight with appropriate primary antibodies diluted in 0.1% Tween 20 (TBST) at 4°C. Primary antibodies against the following proteins were used: SMAD2/3 (8685T, Cell Signaling Technology, Beverly, MA, United States), p-SMAD2/3 (8828S, Cell Signaling Technology), TGF-β1 (3711S, Cell Signaling Technology); GAPDH (AC002, Abclonal, Wuhan, China); αSMA (14395-1-AP, Proteintech), collagen I (14695-1-AP, Proteintech), and PAI-1 (TA504056S, Origene, Maryland). After washing with TBST, the membranes were incubated with anti-rabbit or anti-mouse IgG horseradish peroxidase conjugated antibody (Cell Signaling Technology) for 1 h at room temperature. The protein bands were visualized using SuperSignale West Pico plus Chemiluminescent Substrate (Thermo Fisher Scientifice, Waltham, MA, United States).
For the analysis of patient data, univariate analysis was performed by the chi-square test, Fisher’s exact test, or Student’s t-test to find possible risk factors associated with RILF. One-way analysis of variance (ANOVA) was used to reveal whether the grouping factor (treatment) differentially affected the results in more than three groups. Significant variables in univariate analysis were included into multivariate analysis with binary logistic regression model. Comparisons between the experimental groups for lung density, hydroxyproline content, fibrosis score, and protein expression levels were performed by ANOVA or Student’s t-test using Prism 6.01 (GraphPad Software, San Diego, CA, United States) or SPSS 19.0 (IBM Corp, Armonk, NY, United States). All statistical analyses were conducted with a significance level of α = 0.05 (P < 0.05).
Micro-CT scans performed at three time points after RT revealed increased number of diffuse and patchy shadows in the RT group. A trend of increase in lung density was observed after RT, which turned to be significantly consolidated at week 16 and gradually stabilized over 6 months, suggesting the development of RILF (Figures 1A,B). The irradiated field of mouse hair slowly turned gray during the post-irradiation period (Figure 1C). This indicated that our irradiated area coincided with the lungs of the mice. The western blot assay indicated that α-SMA, a myofibroblast activation marker that indicates fibrosis severity, and PAI-1 expressions increased significantly in lung tissue at week 16 after RT (Figures 1D,E). All these results suggested obvious fibrotic changes in lung tissues by week 16 post RT.
Figure 1. Establishment and confirmation of radiation-induced lung fibrosis in a murine model. (A) Representative images of micro-CT scan for lung density at weeks (W) 6, 16, and 25 after irradiation in control or RT group. (B) Lung density evaluated by Hounsfield units (HU) presented as the mean ± SEM in radiation-treated and control C57BL/6J mice over time (n = 3); *P < 0.05. (C) Photograph of a representative mouse at week 16 after radiation treatment. (D,E) The expression levels of α-SMA and PAI-1 in treated or control groups at week 16 was determined by western blotting. **P < 0.01.
To test the potential radioprotective effect of TH in RILF, micro-CT and histological analyses of lung samples were performed under the light microscope at 6 months post RT in each experimental group. Manifestations such as the presence of alveolar wall thickening, fibrotic nodules, and destruction of alveolar structures after irradiation were less pronounced in the RT + T3 group but not in the RT + PDN group, as compared to the parameters in RT group according to hematoxylin and eosin staining (Figure 2A) and modified Ashcroft scale (P < 0.01, Figure 2E). No specific reduction of blue collagen deposition was observed by using Masson’s trichrome stain (Figure 2B) at the end point of the experiment in RT + PDN group compared to the level of blue collagen in RT group. In contrast, we found that lung hydroxyproline content was dramatically decreased in RT + T3 group (Figure 2B; P < 0.05, Figure 2H) and α-SMA expression was markedly reduced (Figure 2D; P < 0.01, Figure 2G) compared to the values in RT group. Additionally, we observed appearance of abnormal morphological changes of patchy shadows, ground-glass opacity, and consolidation of large areas of lung tissues (Figure 2C) in RT and RT + PDN groups. However, the treatment of PDN could not inhibit the formation of pulmonary fibrosis caused by RT. We further quantitatively evaluated pulmonary fibrosis by estimating lung density using HU values. A reduction of approximately 324 HU (Figure 2F) was observed in RT + T3 group compared to the value in RT group at 25 weeks post RT.
Figure 2. Aerosolized T3 prevents pulmonary fibrosis post radiation treatment in mice. (A,B) Representative images of hematoxylin/eosin (200×; scale bar = 100 μm) and Masson’s trichrome staining (100×; scale bar = 250 μm) from each group at 25 weeks after radiation treatment. (C) Images of representative micro-CT scans obtained at week 25 post radiation treatment in each group. (D) Representative immunohistochemistry staining images with α-SMA protein expression (200×; scale bar = 100 μm) in lung tissues in each group at the end of the experiment. (E) Grading of pulmonary fibrosis evaluated blindly by the modified previously described Ashcroft Scale at week 25 post radiation treatment. (F) Lung density values evaluated quantitatively by Hounsfield units derived from micro-CT scans at week 25 post irradiation (n = 3 for each individual group). (G) Immunohistochemistry scores for lung histopathological changes calculated using a semi-quantitative scoring system. (H) Collagen deposition in mouse right lung tissue assessed by measuring hydroxyproline content in each group at the end of the experiment. Data are expressed as the mean ± SEM, *P < 0.05, **P < 0.01. There were six mice in each group, except where indicated otherwise.
In order to improve drug absorption in the lungs, aerosolized T3 (40 μg/kg) was administered to treated mice. We sought to verify whether this method of administration affected serum thyroid function levels and/or had possible side effects. Serum thyroid function tests were performed at 6, 16, and 25 weeks after radiation scheduled on micro-CT chest scans. There were no significant differences in serum T3, T4, and TSH levels among these groups at those three experimental points (Figures 3A–C). These results suggested that there was no obvious correlation between the improvement of RILF by aerosolized T3 and possible changes in serum TH levels.
Figure 3. Serum levels of thyroid hormones in mice at different time points. (A–C) Serum T3 (pmol/mL), T4 (pmol/mL), and TSH (pg/mL) levels in each experimental group at weeks 6, 16, and 25 after radiation treatment. No significant effect of time on concentrations was observed. Data are presented as the mean ± SEM. Weeks 6 and 16: n = 3; week 25: n = 6.
Next, we conducted a single-center retrospective clinical case study. NPC patients that were regularly examined for serum TH and had chest CT scans before and after IMRT were included in this investigation. Dosimetric parameters of patients’ lungs were calculated by a physicist. A total of 82 patients from January 2010 to August 2018 met the inclusion criteria. Two patients had already suffered from decreased thyroid function at the beginning of radiotherapy. Hypothyroidism is mainly caused by significantly elevated TSH. Of the 82 subjects, 61 males and 21 females, 38.75% of the patients developed hypothyroidism (Figure 4A) and 22.0% (18 patients) had mild RILF (Figure 4B) during the median follow-up time of 25.5 months (2–79 months).
Figure 4. Changes of serum thyroid function and bilateral pulmonary apex in patients with nasopharyngeal carcinoma after radiotherapy. (A) The cumulative incidence curve of radiation-induced hypothyroidism (two patients had already suffered from hypothyroidism before radiotherapy). The cumulative incidence was 49.58% at 3 years after radiotherapy. (B) CT images of patients before and after radiotherapy. Increased density of apex or superior lobe of lungs is seen on the follow-up CT examination after radiotherapy. Such changes without serious clinical symptoms could be classified as grade 1 radiation-induced lung injury.
In the univariate analysis, V50 Gy was 16.11 ± 13.59 cm3 in the RILI group and 4.29 ± 5.77 cm3 in the non-RILI group (P = 0.002). At the same time, hypothyroidism [odds ratio (OR) 4.095, 95% confidence interval (CI) 1.349–12.429, P = 0.01] and N stage (P = 0.018) were also significantly correlated with RILI, respectively, (Table 1). For the N stage, chi-square test showed that N3 stage was significantly associated with RILI (N3/N1: OR 6.60, 95%CI 1.515–28.747, P = 0.014; N3/N2: OR 5.20, 95%CI 1.181–22.891, P = 0.047), while N2 had no statistical significance compared with N1 (OR 1.269, 95%CI 0.366–4.398, P = 0.707). Finally, multivariate logistic regression analysis model found that V50 Gy (OR 1.173, 95% CI 1.077–1.278, P < 0.001) and hypothyroidism (OR 6.137, 95%CI 1.448–26.002, P = 0.014) were significantly associated with RILI (Table 2).
Irradiated lung tissue was acquired from the surgical samples of five patients treated between 2011 and 2018 in our hospital. They were four squamous lung cancers and one lung metastases from colon carcinoma, who had relapsed in situ after radiation therapy. They all had received preoperative radiation treatment with a total dose ranging from 50 to 66 Gy between year 2008 and 2017. Untreated control lung samples were obtained from another five patients that did not receive any radiotherapy. In contrast to control samples, samples from patients with RILF displayed thickening of the alveolar septum or loss of alveolar structure and deposition of collagen fibers (Figure 5A). Considering the established role of iodothyronine deiodinase in T4 to active T3 conversion in tissues, we investigated the expression of DIO2 gene in order to verify the relationship between TH and RILF. Interestingly, DIO2 was highly expressed in epithelial cells within fibrotic regions of irradiated human lung tissue compared to its low-level control group (Figures 5B,C), suggesting that locally increased T3 concentration may improve energy metabolism of alveolar epithelial cells in the stressed fibrotic lung. Given the very limited number of human RILF tissue samples, this result implies a potentially protective role of TH on RILF.
Figure 5. Increased DIO2 expression in lung tissue after radiation treatment and inhibition of the TGF-β1 signaling pathway by thyroid hormone. (A) Representative images of hematoxylin/eosin and Masson’s trichrome staining (100×; scale bar = 250 μm) from treated (n = 5) and control (n = 5) patients. Absence of alveolar structures and apparent collagen deposition can be seen in the irradiated lung tissue. Representative immunohistochemistry staining (B) and immunohistochemistry scores (C) for DIO2 (200×; scale bar = 100 μm) in human lung tissue samples (n = 5) showing DIO2 expression in the lung affected by radiation-induced lung fibrosis (left) and in control lung (right). (D,E) Western blots of profibrotic growth proteins (collagen I, TGF-β1) and p-Smad2/3, a downstream protein in the TGF-β1 signaling pathway, in mouse lung tissues of each group at 25 weeks post radiation treatment are shown. *P < 0.05; **P < 0.01.
In order to investigate the mechanisms whereby T3 exerts a protective effect on RILF, we evaluated the expression of pro-fibrotic growth factors in the lung tissues of mice using western blotting. Collagen I and TGF-β1 were found to be highly expressed in the lungs of RT and RT + PDN groups. In contrast, the expression of the principal pro-fibrotic factor TGF-β1 was decreased in sham RT and RT + T3 groups. In addition, western blotting also demonstrated that phosphorylated Smad2/3 levels were reduced in RT + T3 group (Figures 5D,E). These results suggested that T3 likely mitigated RILF by inhibiting expression of TGF-β1 and its downstream signaling molecules.
We explored the role of macrophages in clinical and preclinical animal levels. Compared with the control group, scattered macrophages could be found in the pulmonary fibrosis area of mice at 25 weeks after irradiation, which had the morphological characteristics of nuclear deviation and relatively large cell volume (Figure 6A). F4/80 (macrophage marker) positive cells were observed in pulmonary fibrosis tissues induced by irradiation than that of sham irradiation controls (P < 0.01, Figures 6B,E). Notably, in the lung tissues of RT + T3 group, but not RT + PDN group, the decrease of F4/80 positive macrophage proportion was detected along with the remission of pulmonary fibrosis (P < 0.05, Figures 6B,E). An obvious increase of M2-like macrophages (CD206 positive cells) were predominantly located in lung fibrosis tissues. Moreover, M2-like macrophages were significantly reduced in RT + T3 group (P < 0.05, Figure 6E). In contrast, there was no significant change in the proportion of M1-like macrophages (iNOS positive cells) in each group (Figure 6E). We then also assessed the accumulation of M2-like macrophages in irradiated human lung tissues. Compared to non-irradiated control lung tissues, M2-like macrophages (CD163 positive cells) were significantly increased in human pulmonary fibrotic area (P < 0.05, Figures 6C,D). These results suggest that M2 rather than M1-like macrophages are associated with RILF, and that aerosolized T3 attenuates RILF in mice accompanied by a decrease in M2-like macrophages in the fibrosis tissue.
Figure 6. Macrophage accumulation in the lungs of mice and humans after thoracic radiotherapy. (A) Representative images of macrophage in mice lung 25 weeks post irradiation compared to controls in HE staining. arrows indicated macrophages (200×; scale bar = 100 μm). (B) Representative images of total, M1 and M2 macrophages in lung tissues of each group identified by F4/80, iNOS, and CD206, respectively, using immunohistochemistry (400×; scale bar = 50 μm). (C) Representative immunohistochemistry images of CD163 positive macrophage in lung tissues (200×; scale bar = 100 μm). (D) Imimmunohistochemistry assessment for macrophage quantification in lungs of control and irradiation patients (n = 3 per group). (E) The numbers of F4/80, iNOS, and CD206 positive macrophages were counted at high magnification field and take the average of three times (n = 3 per group), data are expressed as the mean ± SEM, *P < 0.05.
Radiation induced lung fibrosis is a common complication in the management of radiotherapy and it seriously affects patients’ quality of life. To date, however, there is still no viable therapeutic strategy for RILF, and its mechanism remains unclear. With the growing incidence and mortality rate of lung cancer worldwide (27), therapies that prevent RILF represent an unmet clinical need. In the current study, we have demonstrated a therapeutic effect of aerosolized T3 treatment in a rodent experiment model during the post RT phase. Secondly, we showed that the antifibrotic effect of T3 does not require increased serum levels of T3, T4, or TSH, suggesting that aerosolized delivery may be effective without side effects of iatrogenically elevated thyroid hormones. Thirdly, we found that hypothyroidism increases the risk of RILF in NPC patients; moreover, M2-like macrophages were associated with RILF. Considering these results, we suggest the delivery of aerosolized T3 as a new potential treatment strategy for RILF attenuation.
Thyroid hormone regulates diverse biological processes, from growth to metabolism, and is critically important for nearly all tissues (28–30). Both T4 and T3 can be deiodinated either into the active form by DIO1 and DIO2 or into the inactive form — by DIO3 (31). DIO2 plays a major role in the synthesis of biologically active TH. Increased expression of DIO2 in tissues may reflect either a lack of TH or the need for increased metabolism. Previous studies have shown that hypothyroidism is associated with poor prognosis in many critical diseases, including heart failure (32), non-alcoholic fatty liver disease (33), chronic kidney disease (34), and lung disease (28). Hypothyroid mice suffered more severe lung injury than those with normal serum TH levels in a mouse model of ventilator-induced lung injury, and administration of T3 reduced chemokine and cytokine levels in Dio2 knockout mice (16).
Our results showed that the relationship between both the expression of DIO2 protein as well as TH levels and RILF was consistent with the above results, indicating that there is a correlation between RILF and TH. It is common to find pulmonary shadows in follow-up CT image examinations in some NPC patients due to upper lungs being exposed to the radiation field, which could also be classified as RILI. According to two population-based studies, the incidence of radiation-induced hypothyroidism varied from 27 to 70% in patients that received a dose of 7.5–40 Gy (35, 36). The incidence of hypothyroidism induced by radiation was 38.75% during the follow-up in our study. All patients observed in this cohort had subclinical hypothyroidism. The reasons for this finding likely include insufficient follow-up duration in a small sample and wide use of IMRT in our hospital (37, 38). Bhandare’s study (39) reported that the median latency of clinical hypothyroidism was 4.8 years. RILF occurred in 18 (22.0%) of 82 patients from our cohort during a median follow-up of 25.5 months (range 2–79 months). The proportion of RILF in subjects with hypothyroidism was obviously higher than that in subjects with normal thyroid function, and hypothyroidism was significantly associated with RILF in multivariate logistic regression analysis model. This finding may help understanding the mechanisms of RILF occurrence.
The pathological mechanism of hypothyroidism leading to RILF remains unclear. One possible link may lie in thyroid transcription factor-1 (TTF-1). It plays an important role in the differentiation and formation of both thyroid and lung. Increased expression of TTF-1 was found in some thyroiditis patients (40). In the lung, TTF-1 regulates the differentiation of alveolar epithelial cells and the expression of alveolar surfactant protein, which is very important to maintain alveolar ventilation function and repair lung injury (41). Another possible link maybe related to the biological functions of thyroid hormone, which is not only an important in regulating human endocrine metabolism, but also affects mitochondrial function and transformation. Mitochondrial damage contributes to the development of RILF.
Treatment with TH, an old but probably underused drug, may be utilized in cases with pathologies other than thyroid dysfunction. Some synthetic TH mimetics have shown encouraging results in the experimental treatment of obesity, dyslipidemia, and liver cancer (42). T3 also could alleviate the pulmonary fibrosis in TGF transgenic mice, but the mechanism is not fully explained (18). Recent studies showed that TH attenuated skin and pulmonary fibrosis induced by bleomycin and liver fibrosis caused by carbon tetrachloride in mouse models. These actions may be explained by TH effects on mitochondrial biogenesis and inhibition of TGF-β1-dependent transcription (18, 23). TGF-β1 plays a critical role in profibrotic signals: about 80% of the proteins encoded by genes dysregulated in pulmonary tissues from IPF patients have been reported to be associated with TGF-β1 signaling pathway (43). RILF is similar to other forms of lung fibrosis, especially IPF. Aerosolized TH treatment significantly reduced expression of profibrotic growth proteins, including collagen I, PAI-1 and TGF-β1, whereas no such down-regulation was observed in the RT + PDN group in our study. We concluded that PDN did not inhibit the elevation of TGF-β1 and thus had no anti-fibrotic effect previously suggested by Arata et al. (44). Furthermore, we also consistently found that expression level of phosphorylated Smad2/3, an important transcription factor downstream of the TGF-β1 pathway, was significantly decreased in TH treatment group.
A recent study showed that M2-like tissue-infiltrating macrophages played an important role in RILF (21), but the relationship between local or recruited alveolar macrophages and RILF is still worthy of further study (45). Alveolar macrophages are highly heterogeneous. M1 like macrophages mediate resistance to pathogens, while M2 like macrophages have anti-inflammatory and repair functions (46). T3 can promote the polarization of mouse bone marrow-derived monocytes to M1 macrophages phenotype and inhibit activated M2 macrophage phenotype (47). Tumor infiltrating myeloid-derived cells secrete high levels of TGFβ, and up-regulate CD206 expression (48). In the pulmonary fibrosis model induced by TGF-β1, reduced pulmonary M2 macrophages had a significant anti-fibrosis effect (49). A study suggested that the development of RILF may depend on TGFβ to promote the transformation of macrophages into M2 phenotype (50). However, glucocorticoids can reduce the number of M1 phenotype differentiation of macrophages (51) and have the ability to promote the activation of M2 phenotype macrophages (52). In our study, we also found that the M2 macrophages in lung tissues did not decrease, and even showed a trend of increasing in RT + PDN group. This may partly explain the role of thyroid hormone in alleviating RILF, which may be associated with TGFβ1 and macrophages.
Our study had some limitations. First, our clinical data were obtained from a retrospective analysis of a small size cohort that did not have very long follow-up. Secondly, we included into the analysis the patients that did not receive thoracic radiotherapy and had no advanced RILF. Thirdly, several questions related to the mechanism of TH involvement were not studied. For example, we mainly focused on the DIO2, whereas other deiodinases were not detected, and the mechanism of the role of DIO2 in the development of fibrosis after irradiation need to be further explored. Furthermore, the mechanism underlying inhibitory effects of TH on TGF-β1/SMAD signaling pathway and M2-like macrophage has to be elucidated.
In conclusion, despite recent advances in radiation treatment planning and image-guide radiation therapy, RILF still remains a limiting factor for local tumor control by radiotherapy. To the best of our knowledge, our study for the first time demonstrated that hypothyroidism maybe associated with an increased risk of RILF in patients and provided the first evidence that T3 may be a safe therapeutic option to prevent RILF.
All datasets generated for this study are included in the article/supplementary material.
The studies involving human participants were reviewed and approved by Ethics committee of Tongji hospital, Huazhong University of Science and Technology. The patients/participants provided their written informed consent to participate in this study. The animal study was reviewed and approved by Institutional Animal Care and Use Committee of the Tongji Medical College at the Huazhong University of Science and Technology. Written informed consent was obtained from the individual(s) for the publication of any potentially identifiable images or data included in this manuscript.
LL, XN, and MY carried out experiments. LL and XN analyzed data. WQ and XY designed the experiments. LL, FL, and BW wrote the manuscript. All authors had final approval of the submitted and published versions.
This work was supported by the National Natural Science Foundation of China (Grant No. 81472921).
The authors declare that the research was conducted in the absence of any commercial or financial relationships that could be construed as a potential conflict of interest.
We thank the members of our laboratory. We would also like to thank Editage (www.editage.cn) for English language editing.
1. De Ruysscher D, Niedermann G, Burnet NG, Siva S, Lee A, Hegi-Johnson F. Radiotherapy toxicity. Nat Rev Dis Primers. (2019) 5:13. doi: 10.1038/s41572-019-0064-5
2. Giuranno L, Ient J, De Ruysscher D, Vooijs MA. Radiation-induced lung injury (RILI). Front Oncol. (2019) 9:877. doi: 10.3389/fonc.2019.00877
3. Xiong S, Pan X, Xu L, Yang Z, Guo R, Gu Y, et al. Regulatory T cells promote beta-catenin–mediated epithelium-to-mesenchyme transition during radiation-induced pulmonary fibrosis. Int J Radiat Oncol Biol Phys. (2015) 93:425–35. doi: 10.1016/j.ijrobp.2015.05.043
4. Rajan RR, Chandrasekharan G. Pulmonary injury associated with radiation therapy – assessment, complications and therapeutic targets. Biomed Pharmacother. (2017) 89:1092–104. doi: 10.1016/j.biopha.2017.02.106
5. Simone CN. Thoracic radiation normal tissue injury. Semin Radiat Oncol. (2017) 27:370–7. doi: 10.1016/j.semradonc.2017.04.009
6. Beach TA, Groves AM, Williams JP, Finkelstein JN. Modeling radiation-induced lung injury: lessons learned from whole thorax irradiation. Int J Radiat Biol. (2018) 96:129–44. doi: 10.1080/09553002.2018.1532619
7. Bickelhaupt S, Erbel C, Timke C, Wirkner U, Dadrich M, Flechsig P, et al. Effects of CTGF blockade on attenuation and reversal of radiation-induced pulmonary fibrosis. J Natl Cancer Inst. (2017) 109:djw339. doi: 10.1093/jnci/djw339
8. Choi SH, Hong ZY, Nam JK, Lee HJ, Jang J, Yoo RJ, et al. A hypoxia-induced vascular endothelial-to-mesenchymal transition in development of radiation-induced pulmonary fibrosis. Clin Cancer Res. (2015) 21:3716–26. doi: 10.1158/1078-0432.CCR-14-3193
9. Flechsig P, Dadrich M, Bickelhaupt S, Jenne J, Hauser K, Timke C, et al. LY2109761 attenuates radiation-induced pulmonary murine fibrosis via reversal of TGF-beta and BMP-associated proinflammatory and proangiogenic signals. Clin Cancer Res. (2012) 18:3616–27. doi: 10.1158/1078-0432.CCR-11-2855
10. Biondi B, Wartofsky L. Treatment with thyroid hormone. Endocr Rev. (2014) 35:433–512. doi: 10.1210/er.2013-1083
11. Ceresini G, Urban ML, Corradi D, Lauretani F, Marina M, Usberti E, et al. Association between idiopathic retroperitoneal fibrosis and autoimmune thyroiditis: a case-control study. Autoimmun Rev. (2015) 14:16–22. doi: 10.1016/j.autrev.2014.08.006
12. Oldham JM, Kumar D, Lee C, Patel SB, Takahashi-Manns S, Demchuk C, et al. Thyroid disease is prevalent and predicts survival in patients with idiopathic pulmonary fibrosis. Chest. (2015) 148:692–700. doi: 10.1378/chest.14-2714
13. Kim D, Kim W, Joo SK, Bae JM, Kim JH, Ahmed A. Subclinical hypothyroidism and low-normal thyroid function are associated with nonalcoholic steatohepatitis and fibrosis. Clin Gastroenterol Hepatol. (2018) 16:123–31.e1. doi: 10.1016/j.cgh.2017.08.014
14. Razvi S, Jabbar A, Pingitore A, Danzi S, Biondi B, Klein I, et al. Thyroid hormones and cardiovascular function and diseases. J Am Coll Cardiol. (2018) 71:1781–96. doi: 10.1016/j.jacc.2018.02.045
15. Hataya Y, Igarashi S, Yamashita T, Komatsu Y. Thyroid hormone replacement therapy for primary hypothyroidism leads to significant improvement of renal function in chronic kidney disease patients. Clin Exp Nephrol. (2013) 17:525–31. doi: 10.1007/s10157-012-0727-y
16. Barca-Mayo O, Liao XH, DiCosmo C, Dumitrescu A, Moreno-Vinasco L, Wade MS, et al. Role of type 2 deiodinase in response to acute lung injury (ALI) in mice. Proc Natl Acad Sci USA. (2011) 108:E1321–9. doi: 10.1073/pnas.1109926108
17. Ma SF, Xie L, Pino-Yanes M, Sammani S, Wade MS, Letsiou E, et al. Type 2 deiodinase and host responses of sepsis and acute lung injury. Am J Respir Cell Mol Biol. (2011) 45:1203–11. doi: 10.1165/rcmb.2011-0179OC
18. Yu G, Tzouvelekis A, Wang R, Herazo-Maya JD, Ibarra GH, Srivastava A, et al. Thyroid hormone inhibits lung fibrosis in mice by improving epithelial mitochondrial function. Nat Med. (2018) 24:39–49. doi: 10.1038/nm.4447
19. Stewart AG, Thomas B, Koff J. TGF-beta: master regulator of inflammation and fibrosis. Respirology. (2018) 23:1096–7. doi: 10.1111/resp.13415
20. Yuan X, Liao Z, Liu Z, Wang LE, Tucker SL, Mao L, et al. Single nucleotide polymorphism at rs1982073:T869C of the TGFbeta 1 gene is associated with the risk of radiation pneumonitis in patients with non-small-cell lung cancer treated with definitive radiotherapy. J Clin Oncol. (2009) 27:3370–8. doi: 10.1200/JCO.2008.20.6763
21. Meziani L, Mondini M, Petit B, Boissonnas A, Thomas DMV, Mercier O, et al. CSF1R inhibition prevents radiation pulmonary fibrosis by depletion of interstitial macrophages. Eur Respir J. (2018) 51:1702120. doi: 10.1183/13993003.02120-2017
22. Wynn TA, Vannella KM. Macrophages in tissue repair, regeneration, and fibrosis. Immunity. (2016) 44:450–62. doi: 10.1016/j.immuni.2016.02.015
23. Alonso-Merino E, Martin OR, Ruiz-Llorente L, Martinez-Iglesias OA, Velasco-Martin JP, Montero-Pedrazuela A, et al. Thyroid hormones inhibit TGF-beta signaling and attenuate fibrotic responses. Proc Natl Acad Sci USA. (2016) 113:E3451–60. doi: 10.1073/pnas.1506113113
24. Dabjan MB, Buck CM, Jackson IL, Vujaskovic Z, Marples B, Down JD. A survey of changing trends in modelling radiation lung injury in mice: bringing out the good, the bad, and the uncertain. Lab Invest. (2016) 96:936–49. doi: 10.1038/labinvest.2016.76
25. Qin W, Liu B, Yi M, Li L, Tang Y, Wu B, et al. Antifibrotic agent pirfenidone protects against development of radiation-induced pulmonary fibrosis in a murine model. Radiat Res. (2018) 190:396–403. doi: 10.1667/RR15017.1
26. Hubner RH, Gitter W, El MN, Mathiak M, Both M, Bolte H, et al. Standardized quantification of pulmonary fibrosis in histological samples. Biotechniques. (2008) 44:507–11, 514–7. doi: 10.2144/000112729
27. Bray F, Ferlay J, Soerjomataram I, Siegel RL, Torre LA, Jemal A. Global cancer statistics 2018: GLOBOCAN estimates of incidence and mortality worldwide for 36 cancers in 185 countries. CA Cancer J Clin. (2018) 68:394–424. doi: 10.3322/caac.21492
28. Breitzig MT, Alleyn MD, Lockey RF, Kolliputi N. Thyroid hormone: a resurgent treatment for an emergent concern. Am J Physiol Lung Cell Mol Physiol. (2018) 315:L945–50. doi: 10.1152/ajplung.00336.2018
29. Mullur R, Liu YY, Brent GA. Thyroid hormone regulation of metabolism. Physiol Rev. (2014) 94:355–82. doi: 10.1152/physrev.00030.2013
30. Yen PM. Physiological and molecular basis of thyroid hormone action. Physiol Rev. (2001) 81:1097–142. doi: 10.1152/physrev.2001.81.3.1097
31. Gereben B, McAninch EA, Ribeiro MO, Bianco AC. Scope and limitations of iodothyronine deiodinases in hypothyroidism. Nat Rev Endocrinol. (2015) 11:642–52. doi: 10.1038/nrendo.2015.155
32. Mitchell JE, Hellkamp AS, Mark DB, Anderson J, Johnson GW, Poole JE, et al. Thyroid function in heart failure and impact on mortality. Jacc Heart Fail. (2013) 1:48–55. doi: 10.1016/j.jchf.2012.10.004
33. Ferrandino G, Kaspari RR, Spadaro O, Reyna-Neyra A, Perry RJ, Cardone R, et al. Pathogenesis of hypothyroidism-induced NAFLD is driven by intra- and extrahepatic mechanisms. Proc Natl Acad Sci USA. (2017) 114:E9172–80. doi: 10.1073/pnas.1707797114
34. Zhang Y, Chang Y, Ryu S, Cho J, Lee WY, Rhee EJ, et al. Thyroid hormone levels and incident chronic kidney disease in euthyroid individuals: the Kangbuk Samsung Health Study. Int J Epidemiol. (2014) 43:1624–32. doi: 10.1093/ije/dyu126
35. Colevas AD, Read R, Thornhill J, Adak S, Tishler R, Busse P, et al. Hypothyroidism incidence after multimodality treatment for stage III and IV squamous cell carcinomas of the head and neck. Int J Radiat Oncol Biol Phys. (2001) 51:599–604. doi: 10.1016/s0360-3016(01)01688-1
36. Kumpulainen EJ, Hirvikoski PP, Virtaniemi JA, Johansson RT, Simonen PM, Terava MT, et al. Hypothyroidism after radiotherapy for laryngeal cancer. Radiother Oncol. (2000) 57:97–101. doi: 10.1016/s0167-8140(00)00276-0
37. Lin Z, Chen L, Fang Y, Cai A, Zhang T, Wu VW. Longitudinal study on the correlations of thyroid antibody and thyroid hormone levels after radiotherapy in patients with nasopharyngeal carcinoma with radiation-induced hypothyroidism. Head Neck. (2014) 36:171–5. doi: 10.1002/hed.23285
38. Wu YH, Wang HM, Chen HH, Lin CY, Chen EY, Fan KH, et al. Hypothyroidism after radiotherapy for nasopharyngeal cancer patients. Int J Radiat Oncol Biol Phys. (2010) 76:1133–9. doi: 10.1016/j.ijrobp.2009.03.011
39. Bhandare N, Kennedy L, Malyapa RS, Morris CG, Mendenhall WM. Primary and central hypothyroidism after radiotherapy for head-and-neck tumors. Int J Radiat Oncol Biol Phys. (2007) 68:1131–9. doi: 10.1016/j.ijrobp.2007.01.029
40. Huang H, Li X, Lin L, Shi Y, Lin X, Li L, et al. Upregulation of thyroid transcription factor-1 and human leukocyte antigen class I in Hashimoto’s disease providing a clinical evidence for possible triggering autoimmune reaction. Eur J Endocrinol. (2011) 164:795–800. doi: 10.1530/EJE-10-0960
41. Boggaram V. Thyroid transcription factor-1 (TTF-1/Nkx2.1/TITF1) gene regulation in the lung. Clin Sci. (2009) 116:27–35. doi: 10.1042/CS20080068
42. Kowalik MA, Columbano A, Perra A. Thyroid Hormones, Thyromimetics and their metabolites in the treatment of liver disease. Front Endocrinol. (2018) 9:382. doi: 10.3389/fendo.2018.00382
43. Kaminski N, Allard JD, Pittet JF, Zuo F, Griffiths MJ, Morris D, et al. Global analysis of gene expression in pulmonary fibrosis reveals distinct programs regulating lung inflammation and fibrosis. Proc Natl Acad Sci USA. (2000) 97:1778–83. doi: 10.1073/pnas.97.4.1778
44. Azuma A. Pirfenidone treatment of idiopathic pulmonary fibrosis. Ther Adv Respir Dis. (2012) 6:107–14. doi: 10.1177/1753465812436663
45. Zhou X, Moore BB. Location or origin? What is critical for macrophage propagation of lung fibrosis? Eur Respir J. (2018) 51:1800103. doi: 10.1183/13993003.00103-2018
46. Byrne AJ, Mathie SA, Gregory LG, Lloyd CM. Pulmonary macrophages: key players in the innate defence of the airways. Thorax. (2015) 70:1189–96. doi: 10.1136/thoraxjnl-2015-207020
47. Perrotta C, Buldorini M, Assi E, Cazzato D, De Palma C, Clementi E, et al. The thyroid hormone triiodothyronine controls macrophage maturation and functions: protective role during inflammation. Am J Pathol. (2014) 184:230–47. doi: 10.1016/j.ajpath.2013.10.006
48. Flavell RA, Sanjabi S, Wrzesinski SH, Licona-Limón P. The polarization of immune cells in the tumour environment by TGFbeta. Nat Rev Immunol. (2010) 10:554–67. doi: 10.1038/nri2808
49. Murray LA, Chen Q, Kramer MS, Hesson DP, Argentieri RL, Peng X, et al. TGF-beta driven lung fibrosis is macrophage dependent and blocked by serum amyloid P. Int J Biochem Cell Biol. (2011) 43:154–62. doi: 10.1016/j.biocel.2010.10.013
50. Park HR, Jo SK, Jung U. Ionizing radiation promotes epithelial-to-mesenchymal transition in lung epithelial cells by TGF-β-producing M2 macrophages. In Vivo. (2019) 33:1773–84. doi: 10.21873/invivo.11668
51. Xie Y, Tolmeijer S, Oskam JM, Tonkens T, Meijer AH, Schaaf MJM. Glucocorticoids inhibit macrophage differentiation towards a pro-inflammatory phenotype upon wounding without affecting their migration. Dis Model Mech. (2019) 12:dmm037887. doi: 10.1242/dmm.037887
52. Heideveld E, Hampton-O’Neil LA, Cross SJ, van Alphen FPJ, van den Biggelaar M, Toye AM, et al. Glucocorticoids induce differentiation of monocytes towards macrophages that share functional and phenotypical aspects with erythroblastic island macrophages. Haematologica. (2018) 103:395–405. doi: 10.3324/haematol.2017.179341
Keywords: radiation induced lung fibrosis, aerosolized, thyroid hormone, TGF-β1, macrophage, hypothyroidism
Citation: Li L, Nie X, Yi M, Qin W, Li F, Wu B and Yuan X (2020) Aerosolized Thyroid Hormone Prevents Radiation Induced Lung Fibrosis. Front. Oncol. 10:528686. doi: 10.3389/fonc.2020.528686
Received: 21 January 2020; Accepted: 20 August 2020;
Published: 15 September 2020.
Edited by:
Timothy James Kinsella, Warren Alpert Medical School of Brown University, United StatesReviewed by:
Rihan El Bezawy, Istituto Nazionale dei Tumori (IRCCS), ItalyCopyright © 2020 Li, Nie, Yi, Qin, Li, Wu and Yuan. This is an open-access article distributed under the terms of the Creative Commons Attribution License (CC BY). The use, distribution or reproduction in other forums is permitted, provided the original author(s) and the copyright owner(s) are credited and that the original publication in this journal is cited, in accordance with accepted academic practice. No use, distribution or reproduction is permitted which does not comply with these terms.
*Correspondence: Xianglin Yuan, eXVhbnhpYW5nbGluQGh1c3QuZWR1LmNu
Disclaimer: All claims expressed in this article are solely those of the authors and do not necessarily represent those of their affiliated organizations, or those of the publisher, the editors and the reviewers. Any product that may be evaluated in this article or claim that may be made by its manufacturer is not guaranteed or endorsed by the publisher.
Research integrity at Frontiers
Learn more about the work of our research integrity team to safeguard the quality of each article we publish.