- 1Division of Pediatric Critical Care, University of Tennessee Health Science Center, Memphis, TN, United States
- 2Division of Critical Care, St. Jude Children's Research Center, Memphis, TN, United States
Pulmonary complications are common following hematopoietic cell transplantation (HCT) and contribute significantly to its morbidity and mortality. Diffuse alveolar hemorrhage is a devastating non-infectious complication that occurs in up to 5% of patients post-HCT. Historically, it carries a high mortality burden of 60–100%. The etiology remains ill-defined but is thought to be due to lung injury from conditioning regimens, total body irradiation, occult infections, and other comorbidities such as graft vs. host disease, thrombotic microangiopathy, and subsequent cytokine release and inflammation. Clinically, patients present with hypoxemia, dyspnea, and diffuse opacities consistent with an alveolar disease process on chest radiography. Diagnosis is most commonly confirmed with bronchoscopy findings of progressively bloodier bronchoalveolar lavage or the presence of hemosiderin-laden macrophages on microscopy. Treatment with glucocorticoids is common though dosing and duration of therapy remains variable. Other agents, such as aminocaproic acid, tranexamic acid, and activated recombinant factor VIIa have also been tried with mixed results. We present a review of diffuse alveolar hemorrhage with a focus on its pathogenesis and treatment options.
Introduction
Hematopoietic cell transplant (HCT) is increasingly used as a treatment for various malignant and non-malignant disease processes. Post-transplant, pulmonary complications are common, occurring in up to 40–60% of transplant recipients, and contribute to significant morbidity and mortality (1). Diffuse alveolar hemorrhage (DAH) is a clinical syndrome characterized by dyspnea, pulmonary infiltrates on chest radiography, and progressively bloodier bronchoalveolar lavage on bronchoscopy (2). DAH was first described by Robbins et al. in adults following autologous HCT for various oncologic processes (3, 4). Since then, there have been several case series examining DAH in patients who have received autologous and allogeneic HCT for both malignant and non-malignant diseases (4, 5). The incidence of DAH is typically reported between 2.5 and 5% of patients undergoing allogeneic HCT (4–7); however, incidence has been reported to occur in up to 40% of HCT recipients in some series (1). DAH historically yielded a high overall mortality rate between 64 and 100%, most commonly due to respiratory failure, multiorgan failure, and sepsis (2, 4, 6, 8, 9). While more recent case series describing the use of newer treatment agents, such as activated human recombinant factor VII, have reported improved mortality rates below 50%, (10–13), there remains a great need for improved understanding of this disease process in order to develop precision treatment modalities.
Pathophysiology
Alveolar hemorrhage results from damage of the pulmonary microcirculation, loss of integrity in the alveolar-capillary basement membrane, and accumulation of red blood cells in the alveolar space (14). It presents with a spectrum of histologic findings, including pulmonary capillaritis, bland pulmonary hemorrhage, and diffuse alveolar damage (DAD) (14). Of these, pulmonary capillaritis is the most commonly described histologic subtype overall and is frequently seen in the setting of systemic vasculitis or connective tissue disorders (14). However, there is significant overlap of DAD and DAH on post-mortem exam in HCT patients, suggesting that not only is DAD the most common histologic subtype of DAH in this patient population, but that DAD likely contributes to the development and progression of DAH (14–16).
The exact pathogenesis of DAH in the post-HCT population has not been well-understood but is thought to result from a direct insult to the lungs followed by significant inflammation and cytokine release leading to damage of the alveolar capillaries (2, 8, 14) (Figure 1).
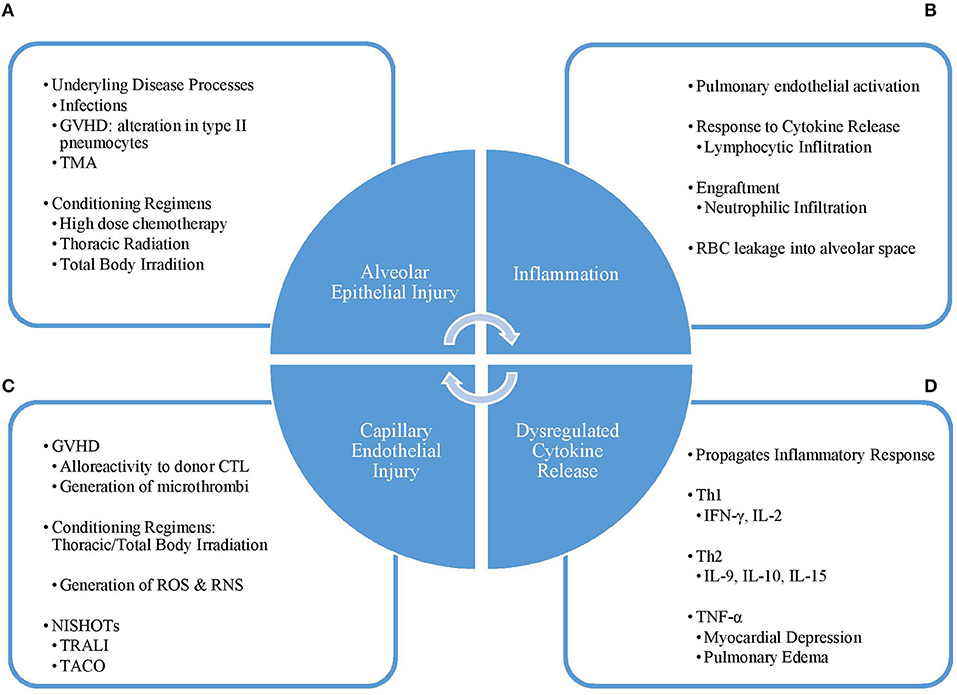
Figure 1. Pathogenesis cycle of DAH. (A) The initial injury to the lung alveoli is caused by condition regimens or other disease processes such as infection, TMA, or GVHD leading to (B) influx of inflammatory cells and molecules into the alveoli and (C) a dysregulated pattern of cytokine release. This response leads to (D) damage of the capillary endothelium, which can be worsened by supportive therapies, such as TRALI and TACO. Disruption of the capillary endothelium, and thus the alveolar-capillary complex, furthers lung injury and leads back into a vicious cycle. GVHD, graft vs. host disease; TMA, thrombotic microangiopathy; Th1, type 1 helper T-cell; Th2, type 2 helper T-cell; IFN-γ, interferon-gamma; IL, interleukin; TNF-α, tumor necrosis factor-alpha; CTL, cytotoxic T-lymphocyte; ROS, reactive oxygen species; RNS, reactive nitrogen species; NISHOT, non-infectious serious hazards of transfusions; TRALI, transfusion-related acute lung injury; TACO, transfusion-associated circulatory overload.
Direct injury to the lung results from underlying systemic diseases such as occult infections, graft vs. host disease (GVHD), thrombotic microangiopathy, and the use of conditioning regimens including high-dose chemotherapy agents, thoracic irradiation, and total body irradiation (2, 7, 8, 17). The alveolar-capillary complex is especially sensitive to damage resulting from thoracic and total body irradiation, which causes injury through generation of reactive oxygen and nitrogen species that results in damage to the alveolar epithelium, loss of endothelial integrity of the pulmonary capillaries leading to loss of barrier function, and reduction of lung perfusion promoting the development of hypoxia (18, 19).
Diffuse alveolar damage is a common finding in both DAH and idiopathic pneumonia syndrome (IPS), suggesting that DAH could possibly be a distinct subset of IPS which involves alveolar injury resulting from exposure to intensive conditioning regimens, radiation therapy, and occult infections (2, 20). While IPS and DAH share many similar clinical features, DAH is distinguished from IPS in that it maintains a pro-inflammatory cytokine environment with very little fibrotic effect (2). On the other hand in IPS, as leukocyte activation progresses, there is dysregulated wound healing and a shift in the cytokine environment to one that promotes pulmonary fibrosis (21). This was demonstrated by a small study conducted by Vusse et al. in which the authors showed that patients with DAH in the context of IPS had different cytokine concentrations on BAL when compared to that of patients with IPS but no DAH. However, there was no significant differences in serum cytokine concentrations between the two groups suggesting that there is a local pathobiological process (22). While the evidence is limited, this study suggests that intrapulmonary cytokine levels may be a helpful biomarker in diagnosis and development of precise immunomodulatory therapies for DAH.
Exposure to these factors leads to activation of the endothelium in the pulmonary microvasculature and release of various inflammatory mediators. Dysregulated production of cytokines including both Th1 (IFN-γ, IL-2) and Th2 (IL-9, IL-10, IL-15) cytokines as well as an increase in IL-6, IFN-γ-inducible protein 10 and TNF-α (23). Lymphocytic and neutrophilic infiltration into the lungs from cytokine action and engraftment/bone marrow recovery, respectively, further potentiates pulmonary tissue damage (3, 21, 24). Neutrophils and neutrophilic products can be found in the lower respiratory tract even in the setting of peripheral neutropenia (24). Damage to the alveolar-capillary complex allows for leakages of red blood cells into the alveolar space and further potentiates the inflammatory response. (2, 8, 25). In the setting of acute GVHD, alloreactivity of the donor cytotoxic T-cells contribute to endothelial damage in the lungs (17, 25). In human and murine models, there is an increase in neutrophils and microthrombi in the alveolar capillaries, and alterations in the type 2 pneumocytes are also seen (26).
Aside from the direct pulmonary effects, the release of cytokines such as TNF-α can lead to myocardial depression (27). The inevitable transfusion of blood products to correct coagulopathy/thrombocytopenia in the peri-HCT period can result in transfusion-related circulatory overload (TACO) (28). The combination of fluid overload and depressed myocardial function worsen leakage of blood and fluid through the already damaged alveolar capillary endothelium (29).
Risk Factors
Prior to its description as a complication following HSCT, alveolar hemorrhage was described in various systemic disease processes associated with direct injury or secondary to indirect inflammatory damage to the pulmonary vasculature. Among these were systemic vasculitides, collagen vascular diseases, mitral valve disease, infections, and medications such as penicillamine, abciximab, nitrofurantoin, propylthiouracil, and amiodarone (2, 8, 30). Since then, several factors have been implicated in the development of DAH post-HCT. Initial reports of DAH were described in patients >40 years old who underwent autologous HCT for an underlying solid malignancy. The onset of symptoms was at time the time of engraftment and associated with fever and severe mucositis (3). Intensive pre-transplant conditioning with total body irradiation or thoracic radiation, severe graft-vs.-host disease (GVHD), second transplant, dimethyl sulfoxide used as a cryopreservative, and acquisition of neutrophil, T cell, and B cell immunologic deficits secondary to the underlying disease process or primary treatment have subsequently been identified as risk factors for the development of alveolar hemorrhage (7, 16, 17, 20, 31–33). Additionally, the use of certain medications such as sirolimus and defibrotide to treat GVHD and sinusoid obstruction syndrome, respectively, have also been associated with the development of pulmonary/diffuse alveolar hemorrhage post-HCT (34, 35). The overall incidence of DAH is similar between autologous and allogeneic HCT (2) with reported incidence of 0.9–21% and 2.3–12.2% following autologous (3, 6, 36) and allogeneic (4–6, 32, 33, 37) transplantation, respectively. Use of umbilical cord blood graft was associated with a higher incidence of DAH compared to use of peripheral blood or bone marrow grafts (32, 37). In patients transplanted with peripheral blood or bone marrow grafts, delayed neutrophil engraftment and graft failure were identified as risk factors for development of DAH (32).
Renal insufficiency is associated with the development of DAH. The association was thought to be secondary to uremia-induced platelet dysfunction or fluid overload (3). However, as more is understood about transplant associated thrombotic microangiopathy (TMA), it is thought TMA is more likely the link between DAH and renal failure.
In pediatrics, there is a trend toward increased DAH in patients who received allogeneic HCT for non-malignant diseases rather than malignancies (4). In these patients, age <1 year, early WBC recovery and fever are associated with the development of DAH (4, 37). The role of thrombocytopenia in the development of DAH is unclear. Most patients are thrombocytopenic at the onset of DAH and prolonged thrombocytopenia may be associated with development of DAH (4, 5, 32). However, neither the platelet nadir nor platelet transfusions appears to affect disease outcome (3, 4, 17, 38). As thrombocytopenia is a component of thrombotic microangiopathy (TMA), which can lead to DAH, it could be the link in certain cases. Coagulopathy in uncommon and does not appear to play a significant role in DAH (32, 33, 39).
Clinical Presentation and Diagnosis
While the onset of DAH is typically within the first 30 days post-HCT, it has been reported after the first month of transplant (2, 4, 8, 40). There are no standardized clinical, radiographic, or laboratory markers to make the diagnosis. Due to the lack of standardization, there is significant heterogeneity in diagnostic criteria. Historically, various clinical criteria have been used to diagnose alveolar hemorrhage, including a high percentage (≥ 20%) of hemosiderin-laden alveolar macrophages, Golde score >100, indicating a high hemosiderin content within macrophages, and progressively bloodier BAL fluid on bronchoscopy (8, 41). It is now classically characterized as a constellation of findings including dyspnea, hypoxemia, diffuse pulmonary infiltrates on chest radiography, and progressively bloodier bronchoalveolar lavage return on bronchoscopy (2, 4, 5, 8, 14, 31). There should be evidence of widespread alveolar injury and abnormal pulmonary physiology associated with an increased alveolar to arterial oxygen gradient and restrictive ventilatory defect (2). While patients often present with fever, cough, and dyspnea, hemoptysis is surprisingly uncommon in adults (5, 8, 20) but is more commonly reported in children (4). Abnormalities in chest radiography can be seen prior to the clinical symptoms of DAH (36). Radiographic findings are initially non-specific and often characterized by diffuse pulmonary infiltrates, most pronounced in the bilateral perihilar areas and lower lobes (4, 20, 36, 42). As the disease progresses, worsening of the chest radiograph to a diffuse, severe reticular alveolar pattern is appreciated (36). Findings on computed tomography are similar to those seen on plain radiography, with predominately ground glass opacities and/or a reticular pattern of injury, consistent with acute alveolar/interstitial disease (42). Oftentimes, respiratory compromise or a new infiltrate on chest radiography leads to bronchoscopy (6), which is used in these patients to establish the diagnosis and exclude other causes for these symptoms such as infection or recurrence of malignancy. During bronchoscopy, there should be progressively bloodier fluid returned or hemosiderin-laden macrophages on bronchoalveolar lavage samples with sequential instillation of saline (4, 14, 43). However, an early study called into question the utility of BAL in the diagnosis of DAH (15), demonstrating that 7/13 patients with hemorrhagic BAL fluid did not have histologic evidence of DAH on post-mortem exam while 4/8 with DAH did not have hemorrhagic BAL fluid. Agusti et al. hypothesized that these findings may be explained by processes that cause hemorrhagic exudates in the alveolar spaces such as CMV pneumonitis or pulmonary aspergillosis and that bronchoscopy may not have explored the area of DAH, respectively (15). While pulmonary infections can lead to alveolar hemorrhage, the diagnosis of diffuse alveolar hemorrhage in the post-HCT period is reserved for alveolar hemorrhage from a non-infectious etiology (2, 8). As such, many studies exclude those with signs of infection such as with a recent positive bronchoalveolar lavage culture or localized pulmonary hemorrhage from other causes such as chronic bronchitis, bronchiectasis, or tumors (2, 5, 8, 31).
Outcomes
Outcomes of DAH following HCT have historically been dismal, with most with in-hospital mortality commonly cited between 64 and 100% (3, 4, 9, 36, 44, 45). Most, if not all, patients require intensive care and mechanical ventilatory support (4–8, 31, 32, 43, 45, 46). Poor prognostic factors include allogeneic transplant, umbilical cord blood as the graft source, infection-related alveolar hemorrhage, and need for mechanical ventilation (1, 8, 32). Factors associated with improved outcomes include early onset (<30 days after HCT) of DAH, which is also associated with an improved response to systemic glucocorticoids, and DAH occurring during the periengraftment period (+/– 5 days from neutrophil engraftment) (8, 31). The improvement in survival periengraftment is hypothesized to be secondary to the transient nature of engraftment and potential for improvement following the resolution of the periengraftment cytokine storm (31).
Management
Supportive Measures
The approach to management of DAH should reflect this entity's diverse and complex pathogenesis. Most patients with DAH require care in an intensive care unit and invasive mechanical ventilation (8). Mechanical ventilation strategies include maintaining a high positive end-expiratory pressure (PEEP) in effort to tamponade capillary bleeding and on occasion escalation to high frequency oscillatory ventilation (HFOV) (13, 39). Supportive measures such as optimization of fluid and electrolyte balance, correction of coagulopathies and prophylactic antibiotics are a mainstay of treatment for DAH (6).
Transfusion of Blood Products
Most patients are thrombocytopenic at time of DAH onset. However, outcomes have not been shown to be affected by either the nadir platelet count or its correction by platelet transfusions (3, 4, 38). Coagulopathy associated with abnormalities in prothrombin time (PT), international normalized ratio (INR), and activated partial thromboplastin time (aPTT) is uncommon in patients with DAH (32, 33, 39). While transfusion of blood products, particularly platelets, is common in the management of DAH, the unrestrained use of platelet transfusions is not without risks. Platelet and red blood cell (RBC) transfusions are independently associated with a higher risk of venous thromboembolisms, arterial thromboembolisms, and in-hospital mortality in hospitalized oncology patients (47). Platelet transfusions have been implicated in transfusion reactions such as allergic reactions, febrile non-hemolytic reactions, transfusion-associated sepsis, and transfusion-related acute lung injury (TRALI) (48). The potential for injury is further elevated in these patients as HCT has been identified as risk factor for developing TRALI, and; during periods of inflammation, such as is seen in DAH, platelet transfusions may increase vascular permeability and thereby worsening capillary leak (48–50).
As traditional transfusion practices have not shown to impact outcomes in DAH, thromboelastography (TEG) and rotational thromboelastometry (ROTEM) TEG/ROTEM-guided transfusion may be considered and is an area for future research. They have been used to assess global clotting function in various bleeding disorders, during cardiac surgery, liver transplantation, and traumas (51). In patients with hemophilia, TEG/ROTEM assay results correlated well with phenotypic disease severity. They are useful for monitoring response to bypassing agents, such as recombinant activated FVII (51). Furthermore, a Cochrane Review of TEG/ROTEM use in cardiac surgery showed a trend toward improved mortality and an overall significant reduction in pooled red blood cell, fresh frozen plasma, and platelet requirements using TEG/ROTEM-guided transfusions (52).
Anemia is also a common finding in the critically-ill pediatric population, occurring in 74% of these patients with 49% receiving one or more RBC transfusions during their PICU course (53). While RBC transfusions can correct severe anemia, thus increasing the oxygen content of blood, it does not necessarily increase tissue oxygen delivery and oxygen consumption (54). Furthermore, they have been associated with non-infectious serious hazards of transfusions (NISHOTs) such as TRALI/TACO, and mortality (53–55). In 2007, Lacroix et al. showed that in hemodynamically stable patients, a restrictive strategy of RBC transfusions, defined as a hemoglobin threshold of 7 gram/deciliter, did not result in an increase in new or progressive multiple-organ dysfunction syndrome and that patients received 44% fewer transfusions than those in the liberal-transfusion, defined as a hemoglobin threshold of 9.5 gram/deciliter, group (56). In 2018, the Pediatric Critical Care Transfusion and Anemia expertise Initiative (TAXI) published good practice guidelines and recommendations for the use of RBC transfusions. The guidelines supported Lacroix's restrictive transfusion threshold of 7 g/dl in hemodynamically stable patients and urged clinicians to regard the need for transfusion in the context of the patient's broader clinical picture rather than simply looking at a threshold number (54, 56, 57).
Glucocorticoids
Glucocorticoids (GC) have been used for many years in various inflammatory and autoimmune disorders. Cases of DAH which coincide with marrow recovery are thought to be due to an inflammatory diffuse alveolar damage potentially responsive to glucocorticoids. This has made glucocorticoids the mainstay of treatment (2, 4, 8, 24). First demonstrated by Metcalf et al. patients treated with high dose steroids, defined as a daily dose of methylprednisolone 30 mg or equivalent, had an increase in overall survival and decreased need for invasive mechanical ventilation without an increase in rates of systemic bacterial or fungal infections when compared to patients treated with low-dose steroids or supportive care alone (24).
The mechanism by which glucocorticoids modulate inflammation occurs through genomic and non-genomic pathways (Table 1). Within the cytoplasm, glucocorticoid receptors (GCR) bind to free glucocorticoids with high affinity (58). The glucocorticoid-glucocorticoid receptor complex enters the nucleus and binds to specific DNA sequences known as either positive or negative glucocorticoid-responsive elements (GREs). When bound to positive GREs, the glucocorticoid-GCR complexes exerts a direct genomic effect through activating the transcription of anti-inflammatory proteins such as IL-10, Annexin-1, mitogen-activated protein kinase (MAPK) phosphatase-1, and inhibitors of nuclear factor NF-kB (59, 60). Annexin-1 blocks the release of arachidonic acid and its conversion to various eicosanoids such as prostaglandins, thromboxanes, prostacyclins, and leukotrienes (58). The glucocorticoid-GCR complex induces indirect genomic effects by competitive binding with (NF)-kB and activator protein 1 (AP-1) thereby suppressing the expression of pro-inflammatory molecules (COX-2, cytokines such as IL-2, TNF-α, IFN-γ, chemokines, cell adhesion molecules, complement factors, and their receptors) (58–62). It is thought that most of the desired anti-inflammatory effects are secondary to the suppressive effects on gene transcription (60). Another genomic effect by which glucocorticoids inhibit inflammation is through decreasing mRNA stability for inflammatory proteins such as vascular endothelial growth factor and cyclooxygenase 2 (63). The genomic effects of glucocorticoids can be appreciated at lower doses but require a longer onset of action, at least 30 min but sometimes taking up to hours or days, than the non-genomic effects (58, 60, 63, 64). The non-genomic effects occur quickly, within seconds to minutes following administration of a glucocorticoid (58, 63, 65).
Glucocorticoids inhibit the cycling of sodium and calcium across the plasma membrane, reducing the availability of intracellular free calcium, which inhibits neutrophil degranulation (58, 60, 66). Within the mitochondria, they increase proton leak and inhibit oxidative phosphorylation, decreasing production of ATP, an essential energy source of cytokine synthesis and antigen processing/presentation by macrophages (60). Additionally, glucocorticoids contribute to the integrity of the alveolar-capillary complex through activation of the phosphatidylinositol-3-hydroxykinase (PI3K/Akt) signaling pathway which leads to the production of nitric oxide (NO), a mediator of vascular integrity with anti-inflammatory properties (58, 67, 68). Glucocorticoids promote vasoconstriction of the airway vasculature via alpha adrenergic effects and by potentiating angiotensin II (58) and inhibits platelet activating factor and in turn decreases exudative leakage in the airways (69, 70).
Glucocorticoid dosing varies widely and can range up to a 200-fold difference depending on the indication for use (64, 71, 72). The genomic effects of glucocorticoids can be elicited with usage of relatively lower doses, as low as 7.5–100 mg prednisone equivalent a day used in maintenance therapy for rheumatologic conditions saturating <50% of the glucocorticoid receptors (64). However, in order for them to have maximal genomic and non-genomic anti-inflammatory effects, very high doses, defined as over 250 mg prednisone equivalent a day, are required (64). Even higher doses of up to 1,000–2,000 mg of methylprednisolone equivalent per day, referred to as pulse dosing, are used in treatment of acute rheumatologic disease exacerbations in adults (73). This dosing in adults translates to ~15–30 mg/kg/day methylprednisolone equivalents in children (73). While methylprednisolone is most often used for pulse-dosing and has the advantage of faster penetration into the cellular membrane and thus onset of action, dexamethasone, another corticosteroid with high glucocorticoid effects, has been used in a pulse-dose manner (73, 74). When administered at 4–5 mg/kg up to 20 mg/dose, dexamethasone is a more potent anti-inflammatory agent due to its increased affinity for glucocorticoid receptors and non-genomic effects when compared to methylprednisolone (73–75). At these doses, not only is there 100% saturation of the cytosolic glucocorticoid receptors, thus exerting full genomic effects, there is also full elicitation of the more immediate, non-genomic effects (64). It is likely that the addition of the non-genomic effects of glucocorticoids contribute significantly to the termination of acute exacerbations in these inflammatory processes (64).
Many adverse effects of glucocorticoid therapy have been described including hemodynamic changes (hypertension, bradycardia) associated with intravenous infusions, loss of bone mass, suppression of the HPA axis, weight gain, hyperglycemia/diabetes mellitus, cardiovascular disease, myopathy, cataracts, psychiatric disturbances, growth suppression in children, and increased infection risk (73, 76). The dosing over which these adverse effects develop have not been defined but appear to be related to both average and cumulative dose (76, 77). The rationale for pulse dosing is to maximize the immediate non-genomic effects leading to faster recovery of clinical symptoms, minimize the inflammatory damage from disease, and limit the adverse effects associated with long term glucocorticoid use (73). Treatment at these doses are limited in duration of therapy and require either discontinuation or rapid decreases after a maximum of 5 days (64, 73, 76).
Glucocorticoid dosing in the treatment of adult DAH patients has not been standardized (Table 2). While the initial report by Metcalf et al. supported the use of over 30 mg of MP equivalent per day, there is a paucity of studies comparing different treatment doses. Rathi et al. compared low, medium, and high dose, defined as <250, 250–1,000, and >1,000 mg/day of MP, respectively, and found a significantly lower ICU and hospital mortality in patients treated with lower dose steroids compared to those treated with medium or high doses (45). However, this study was limited by its retrospective nature. Furthermore, while there was no significant difference in mortality predictive indices between the treatment groups at time of admission, the sicker patients at time of DAH diagnosis may have been placed on higher doses of steroids, thereby confounding the results (45). In other case series and reports, high doses of glucocorticoids have been used- methylprednisolone 30 mg/kg/day to a max of 2 gram day is used for 3–5 days which is followed by a slow taper over 2–4 weeks (2, 6, 8).
Reports of glucocorticoid use of DAH following HCT in the pediatric population are limited to small, retrospective reports (Table 3). Ben-Abraham et al. reported a series of 6 children who were treated with moderate glucocorticoid doses (6 mg/kg methylprednisolone) in which only 1 survived the initial injury (4). Heggen et al. reported better overall survival (4/7) but no significant difference between patients treated with 1,000 mg/day (3/4) and <500 mg/day (1/3) of methylprednisolone (5). While one cannot draw definite conclusions between these two studies, it appears that there is a trend toward improved survival with use of higher glucocorticoid doses. Prospective studies are greatly needed to develop treatment regimens as mortality for DAH remains high. At this time, in cases of DAH thought to be caused by inflammation-induced alveolar damage, we advocate for the use of high dose glucocorticoid therapy to achieve maximal genomic and non-genomic effects and minimize side effects. In circumstances where underlying infection is suspected, we recommend weaning over 4–8 weeks.
Aminocaproic Acid
Aminocaproic acid (ACA) is an antifibrinolytic agent that has been used with mixed results in the treatment of DAH following HSCT (43, 45). In the original study conducted by Wanko et al. in 2006, there was a significant decrease in 100 day DAH mortality from 83 to 44% in those patients who were treated with a combination of methylprednisolone (250 mg q6h followed by taper of 50% every 3 days) and aminocaproic acid (1,000 mg IV q6h) compared to those who were treated with methylprednisolone alone. There was no major clinically apparent side effect from the addition of aminocaproic acid. Notably, 6/14 patients in this series had an infectious organism identified with bronchoscopy. The authors acknowledge that classically, DAH occurs in the absence of infectious agents, though question the clinical significance of detection of these agents on the development and progression of DAH (43). However, a follow-up study performed by Rathi et al. in 2015, found no significant difference in mortality (30, 60, 100 day, ICU, hospital), number of ventilator days, ICU length of stay, or hospital length of stay between groups who received a combination of steroid and aminocaproic acid (4 gram IV bolus followed by 1 gram/h infusion) or steroids alone. Again, there was no increase in side effects attributed to aminocaproic acid in the cohort of patients who received combination therapy. The authors argue that the discrepancy in efficacy of ACA may be due to a higher severity of illness in this study as compared to the previous one (69% requiring ICU care as opposed to 28% classified as critically ill) (45).
Nebulized Tranexamic Acid
Tranexamic acid (TXA), also a antifibrinolytic agent acts by binding to plasminogen and inhibits its binding to fibrin and activation to plasmin (78). It has been used in the treatment or prevention of bleeding in hemophilia, ITP, and in operative procedures (79). In patients requiring systemic anticoagulation undergoing dental procedures, the local application of TXA through use of a mouthwash resulted in significantly less post-procedural bleeding without elevated levels of TXA in the serum (80). In a case series reported by Solomonov et al. of six patients with pulmonary hemorrhage of varying etiology treated with direct delivery of TXA either through direct instillation during bronchoscopy or nebulized showed cessation of bleeding in all patients (79). In a cohort of pediatric patients who were diagnosed with DAH, Nebulized TXA alone led to complete or near cessation of bleeding in 10/18 and the addition of nebulized rFVIIa led to hemostasis in an additional 6 patients (12). In this series, a documented respiratory infection was negatively associated with response to nebulized TXA, suggesting that nebulized TXA may not target pulmonary hemorrhage secondary infectious processes (12). O'Neil et al. recently published a case series of 19 pediatric patients with pulmonary hemorrhage who were treated with inhaled or endotracheally instilled TXA in which 18/19 patients had cessation of bleeding (81). No adverse effects related to nebulized TXAs were reported in these series (12, 79, 81).
Recombinant Activated Factor VII
Intravenous (IV) recombinant activated factor VII (rFVIIa) was initially developed for use in patients with hemophilia A/B with presence of an inhibitor. Under these conditions, an increase in concentration of activated factor VII (FVIIa) produced an increase in the rate of thrombin generation, suggesting that FVIIa is able to bypass the intrinsic coagulation pathway to directly generate thrombin (82, 83). It has since been used in various situations in the pediatric population outside of hemophilia, such as congenital factor VII deficiency, hepatic dysfunction, post-operative bleeding following cardiac surgery, qualitative platelet disorders, and traumatic hemorrhage (84). In recent years, rFVIIa has been used in the treatment of DAH, both in adults (Table 4) and pediatrics (Table 5). It is hypothesized that there are inhibitors of tissue factor, tissue factor pathway inhibitors (TFPI) in inflamed alveoli. These inhibitors prevent FVIIa-tissue factor formation and factor X activation, making the inflamed lungs more susceptible to bleeding (10). Local administration of rFVIIa overcomes the TFPI and restores thrombin generation (10). Few case reports on the use of intravenous rFVIIa have demonstrated cessation of hemorrhage in DAH; however, it often requires higher and more frequent dosing (86, 87).
In effort to decrease systemic effects of rFVIIa, most notably thromboembolic complications, rFVIIa has been delivered directly to the lungs during bronchoscopy or through nebulization (10, 12, 13, 38, 46, 88, 89). Treatment regimens including rFVIIa and dosing are very variable. It has been used as initial therapy in combination with systemic corticosteroids +/− aminocaproic acid or as rescue therapy in cases that are refractory to treatment with other therapies such as corticosteroids, tranexamic acid, aprotinin, aminocaproic acid, plasmapheresis, or desmopressin (10, 38, 85, 86). Heslet et al. first reported the successful intrapulmonary use of rFVIIa in a case series of six patients who developed DAH and failed to respond to other therapies. They received one to three doses of either intrapulmonary or nebulized rFVIIa with all achieving hemostasis and improvement in hypoxia (38). Baker et al. subsequently reported a series of 6 patients treated with intrapulmonary rFVIIa in which 5 patients achieved hemostasis (10). In a trial using rFVIIa for DAH refractory to treatment with nebulized TXA, 6/8 patients showed clinical response to nebulized rFVIIa (12). Park et al. reported a series of 6 pediatric patients with DAH treated with rFVIIa in conjunction with glucocorticoids in which all patients had cessation of bleeding with no adverse events due to medication administration (13). Our institution has used intrapulmonary rFVIIa to treated 13 patients diagnosed with DAH, of which 6 survived the acute event (90). In these cohorts of patients who had cessation of DAH, there were no reported treatment related complications such as thromboembolic events or recurrence of DAH following treatment (10, 12, 38). While use of intrapulmonary rFVIIa to achieve hemostasis in DAH is encouraging, further prospective studies to evaluate its use and standardize dosing are needed.
Extracorporeal Membrane Oxygenation (ECMO)
The presence of severe bleeding is often considered a contraindication for use of extracorporeal membrane oxygenation (ECMO) due to the need for systemic anticoagulation; however, there have been several reported cases of its successful use in DAH due to systemic vasculitides as a rescue therapy (91). Morris et al. reported a case of the successful use of VA ECMO for DAH following HCT in a patient with Hurler Syndrome. The patient was systemically heparinized and was also treated with methylprednisolone and aminocaproic acid without further hemorrhage. He was able to be decannulated and survived to hospital discharge (92). In our institution, we also had a case of pulmonary hemorrhage following HSCT that was successfully supported on ECMO (93). Given reports of the successful use of ECMO for DAH as well as our experience, we advocate for its consideration in certain patients and propose that pulmonary hemorrhage and HSCT are not absolute contraindications for ECMO support.
Special Considerations
Thrombotic Microangiopathy
While uncommon, DAH has been reported in association with transplant-associated thrombotic microangiopathy (TA-TMA) (94). TA-TMA is as subset of the thrombotic microangiopathies characterized by Coombs negative hemolysis, proteinuria, increase in serum LDH, renal and/or neurologic dysfunction, and systemic serositis (95). Treatment options for TA-TMA include plasma exchange, the anti-CD20 monoclonal antibody rituximab, and the complement inhibitor eculizumab (96). Etanercept, a TNF-α antagonist, has also been suggested as a therapy option for TA-TMA (97). TA-TMA carries a significant mortality up to 75% alone, or higher when present with DAH (94, 96). Thus, in cases of suspected TA-TMA associated with DAH, the careful addition of one of these therapies should be considered.
Idiopathic Pneumonia Syndrome
Idiopathic pneumonia syndrome (IPS) is a non-infectious pulmonary complication of HCT which shares many clinical similarities with DAH, albeit without hemorrhage. It presents with findings of diffuse alveolar injury in the absence of lower respiratory tract infection, cardiac dysfunction, acute renal failure, or iatrogenic fluid overload (98). IPS is characterized by elevated levels of cytokines including IL-6, IL-8, Ang-2, and TNFR1, a surrogate marker for TNF-α (99). Etanercept, a TNF-α-binding protein, has been successfully used in combination with glucocorticoids (2 mg/kg/day MP equivalent) for the treatment of IPS in the pediatric population (99). In the DAH subset of IPS, higher doses of glucocorticoids may be considered.
Infection
While DAH is classically considered a non-infectious complication of HSCT, the presence of an occult infection must be considered in cases of recurrent hemorrhage or lack of response to treatment. Agusti et al. reported a high rate (6/11) of concurrent infections on post-mortem exam in areas separate from hemorrhage, including herpes pneumonia, CMV pneumonitis, bacterial pneumonia, and aspergillosis, though BAL studies were negative for infectious etiologies in the 7 patients who had a bronchoscopy within 7 days of death (15). In a report by Heggen et al. post-mortem exam of one patient who died from DAH-associated progressive respiratory failure showed lung cultures positive for both yeast and bacteria despite BAL culture negative for infectious organism at time of DAH diagnosis (5). Thus, we recommend a low threshold for antimicrobial coverage of occult infections in cases of DAH even when BAL studies do not identify an infectious etiology. In cases of diagnostic dilemma despite less invasive means such as CT and bronchoscopy, we recommend early consideration of lung biopsy.
Myelogenous Leukemia
Nanjappa et al. reported a case series of 5 patients with AML, two of whom had undergone allo-HCT, who developed DAH (100). While respiratory compromise in leukemia patients is recognized, it is likely that pulmonary hemorrhage is underestimated. On post-mortem exam of patients with leukemia, pulmonary hemorrhage was present in 74% of cases (101). Additionally, in patients with AML and acute respiratory failure of unknown etiology, pathologic findings of DAD/DAH were present in ~22% of cases (102). In a series of patients initially hospitalized for respiratory failure and subsequently diagnosed with M5 AML, BAL fluid was hemorrhagic in 7/15 patients undergoing bronchoscopy (103). In acute and chronic myelogenous leukemia, a pattern of DAD characterized by endothelial cell hyperplasia, interstitial edema, and the presence of interstitial lymphocytes, has been described following the initiation of chemotherapy. Lung injury occurs from lysis of leukemic cells and release of intracellular enzymes such as collagenases, cathepsin G, and lysozymes (104). In the setting of acute myelogenous leukemia, this mechanism may be responsible for the initial lung injury seen in the development of DAH.
Conclusions
Diffuse alveolar hemorrhage continues to be a recognized complication of HCT which has historically carried significant morbidity and mortality. While survival has seemingly improved with newer therapeutic options, there remains ample opportunity for optimization in the holistic care of these patients. In our institution, early recognition, a standard approach to diagnosis and treatment, and protection against additional organ dysfunction/endothelial damage are cornerstone to our treatment approach. Further research is needed to better understand the pathogenesis of this complex disease process, evaluate current treatment options, and develop new therapies in order to continue to improve outcomes.
Author Contributions
SG conceptualized the scope of manuscript and provided intellectual contributions. SG and KF performed the literature search, analysis, and wrote the original draft. SG, JM, and RM provided critical revisions and to the draft. All authors contributed to the manuscript revision, read, and approved the final submitted version.
Conflict of Interest
The authors declare that the research was conducted in the absence of any commercial or financial relationships that could be construed as a potential conflict of interest.
Acknowledgments
We would like to thank the library service at St. Jude Children's Research Hospital for assistance in literature search.
References
1. Gupta S, Jain A, Warneke CL, Gupta A, Shannon VR, Morice RC, et al. Outcome of alveolar hemorrhage in hematopoietic stem cell transplant recipients. Bone Marrow Transplant. (2007) 40:71–8. doi: 10.1038/sj.bmt.1705695
2. Afessa B, Tefferi A, Litzow MR, Krowka MJ, Wylam ME, Peters SG. Diffuse alveolar hemorrhage in hematopoietic stem cell transplant recipients. Am J Respir Crit Care Med. (2002) 166:641–5. doi: 10.1164/rccm.200112-141CC
3. Robbins RA, Linder J, Stahl MG, Thompson AB, Haire W, Kessinger A, et al. Diffuse alveolar hemorrhage in autologous bone marrow transplant recipients. Am J Med. (1989) 87:511–8. doi: 10.1016/S0002-9343(89)80606-0
4. Ben-Abraham R, Paret G, Cohen R, Szold O, Cividalli G, Toren A, et al. Diffuse alveolar hemorrhage following allogeneic bone marrow transplantation in children. Chest. (2003) 124:660–4. doi: 10.1378/chest.124.2.660
5. Heggen J, West C, Olson E, Olson T, Teague G, Fortenberry J, et al. Diffuse alveolar hemorrhage in pediatric hematopoietic cell transplant patients. Pediatrics. (2002) 109:965–71. doi: 10.1542/peds.109.5.965
6. Lewis ID, DeFor T, Weisdorf DJ. Increasing incidence of diffuse alveolar hemorrhage following allogeneic bone marrow transplantation: cryptic etiology and uncertain therapy. Bone Marrow Transplant. (2000) 26:539–43. doi: 10.1038/sj.bmt.1702546
7. Chao NJ, Duncan SR, Long GD, Horning SJ, Blume KG. Corticosteroid therapy for diffuse alveolar hemorrhage in autologous bone marrow transplant recipients. Ann Intern Med. (1991) 114:145–6. doi: 10.7326/0003-4819-114-2-145
8. Afessa B, Tefferi A, Litzow MR, Peters SG. Outcome of diffuse alveolar hemorrhage in hematopoietic stem cell transplant recipients. Am J Respir Crit Care Med. (2002) 166:1364–8. doi: 10.1164/rccm.200208-792OC
9. Jules-Elysee K, Stover DE, Yahalom J, White DA, Gulati SC. Pulmonary complications in lymphoma patients treated with high-dose therapy autologous bone marrow transplantation. Am Rev Respir Dis. (1992) 146:485–91. doi: 10.1164/ajrccm/146.2.485
10. Baker MS, Diab KJ, Carlos WG, Mathur P. Intrapulmonary recombinant factor VII as an effective treatment for diffuse alveolar hemorrhage: a case series. J Bronchology Interv Pulmonol. (2016) 23:255–8. doi: 10.1097/LBR.0000000000000286
11. Pathak V, Kuhn J, Gabriel D, Barrow J, Jennette JC, Henke DC. Use of activated factor VII in patients with diffuse alveolar hemorrhage: a 10 years institutional experience. Lung. (2015) 193:375–9. doi: 10.1007/s00408-015-9720-z
12. Bafaqih H, Chehab M, Almohaimeed S, Thabet F, Alhejaily A, AlShahrani M, et al. Pilot trial of a novel two-step therapy protocol using nebulized tranexamic acid and recombinant factor VIIa in children with intractable diffuse alveolar hemorrhage. Ann Saudi Med. (2015) 35:231–9. doi: 10.5144/0256-4947.2015.231
13. Park JA, Kim BJ. Intrapulmonary recombinant factor VIIa for diffuse alveolar hemorrhage in children. Pediatrics. (2015) 135:e216–20. doi: 10.1542/peds.2014-1782
14. Lara AR, Schwarz MI. Diffuse alveolar hemorrhage. Chest. (2010) 137:1164–71. doi: 10.1378/chest.08-2084
15. Agusti C, Ramirez J, Picado C, Xaubet A, Carreras E, Ballester E, et al. Diffuse alveolar hemorrhage in allogeneic bone marrow transplantation. A postmortem study. Am J Respir Crit Care Med. (1995) 151:1006–10. doi: 10.1164/ajrccm.151.4.7697223
16. Roychowdhury M, Pambuccian SE, Aslan DL, Jessurun J, Rose AG, Manivel JC, et al. Pulmonary complications after bone marrow transplantation: an autopsy study from a large transplantation center. Arch Pathol Lab Med. (2005) 129:366–71. doi: 10.1043/1543-21652005129<366:PCABMT>2.0.CO
17. Wojno KJ, Vogelsang GB, Beschorner WE, Santos GW. Pulmonary hemorrhage as a cause of death in allogeneic bone marrow recipients with severe acute graft-versus-host disease. Transplantation. (1994) 57:88–92. doi: 10.1097/00007890-199401000-00016
18. Graves PR, Siddiqui F, Anscher MS, Movsas B. Radiation pulmonary toxicity: from mechanisms to management. Semin Radiat Oncol. (2010) 20:201–7. doi: 10.1016/j.semradonc.2010.01.010
19. Slauson DO, Hahn FF, Chiffelle TL. The pulmonary vascular pathology of experimental radiation pneumonitis. Am J Pathol. (1977) 88:635–54.
20. Soubani AO, Pandya CM. The spectrum of noninfectious pulmonary complications following hematopoietic stem cell transplantation. Hematol Oncol Stem Cell Ther. (2010) 3:143–57. doi: 10.1016/S1658-3876(10)50025-6
21. Shankar G, Cohen DA. Idiopathic pneumonia syndrome after bone marrow transplantation: the role of pre-transplant radiation conditioning and local cytokine dysregulation in promoting lung inflammation and fibrosis. Int J Exp Pathol. (2001) 82:101–13. doi: 10.1111/j.1365-2613.2001.iep182.x
22. Vande Vusse LK, Wurfel MM, Madtes DM, Schoch HG, Harju-Baker S, Hill JA, et al. Alveolar levels of immuno-inflammatory mediators in diffuse alveolar hemorrhage after allogeneic transplant. Bone Marrow Transplant. (2018) 53:1206–9. doi: 10.1038/s41409-018-0168-7
23. Koh H, Nakamae H, Koh KR, Ohsawa M, Nakane T, Takeoka Y, et al. Serum cytokine profiles at the onset of severe, diffuse alveolar hemorrhage complicating allogeneic hematopoietic stem cell transplantation, treated successfully with pulse intravenous cyclophosphamide. Acta Haematol. (2010) 124:171–5. doi: 10.1159/000318013
24. Metcalf JP, Rennard SI, Reed EC, Haire WD, Sisson JH, Walter T, et al. Corticosteroids as adjunctive therapy for diffuse alveolar hemorrhage associated with bone marrow transplantation. Univ Nebraska Med Center Bone Marrow Transpl Grou Am J Med. (1994) 96:327–34. doi: 10.1016/0002-9343(94)90062-0
25. Carreras E, Diaz-Ricart M. The role of the endothelium in the short-term complications of hematopoietic SCT. Bone Marrow Transplant. (2011) 46:1495–502. doi: 10.1038/bmt.2011.65
26. Piguet PF, Grau GE, Collart MA, Vassalli, Kapanci Y. Pneumopathies of the graft-versus-host reaction. Alveolitis associated with an increased level of tumor necrosis factor mRNA and chronic interstitial pneumonitis. Lab Invest. (1989) 61:37–45.
27. Krishnagopalan S, Kumar A, Parrillo JE, Kumar A. Myocardial dysfunction in the patient with sepsis. Curr Opin Criti Care. (2002) 8:376–88. doi: 10.1097/00075198-200210000-00003
28. Semple JW, Rebetz J, Kapur R. Transfusion-associated circulatory overload and transfusion-related acute lung injury. Blood. (2019) 133:1840–53. doi: 10.1182/blood-2018-10-860809
29. Yang G, Hamacher J, Gorshkov B, White R, Sridhar S, Verin A, et al. The dual role of TNF in pulmonary edema. J Cardiovasc Dis Res. (2010) 1:29–36. doi: 10.4103/0975-3583.59983
30. Krause ML, Cartin-Ceba R, Specks U, Peikert T. Update on diffuse alveolar hemorrhage and pulmonary vasculitis. Immunol Allergy Clin North Am. (2012) 32:587–600. doi: 10.1016/j.iac.2012.08.001
31. Majhail NS, Parks K, Defor TE, Weisdorf DJ. Diffuse alveolar hemorrhage and infection-associated alveolar hemorrhage following hematopoietic stem cell transplantation: related and high-risk clinical syndromes. Biol Blood Marrow Transplant. (2006) 12:1038–46. doi: 10.1016/j.bbmt.2006.06.002
32. Keklik F, Alrawi EB, Cao Q, Bejanyan N, Rashidi A, Lazaryan A, et al. Diffuse alveolar hemorrhage is most often fatal and is affected by graft source, conditioning regiment toxicity, engraftment kinetics. Haematologica. (2018) 103:2109–15. doi: 10.3324/haematol.2018.189134
33. Raptis A, Mavroudis D, Suffredini A, Molldrem J, Rhee FV, Childs R, et al. High-dose corticosteroid therapy for diffuse alveolar hemorrhage in allogeneic bone marrow stem cell transplant recipients. Bone Marrow Transplant. (1999) 24:879–83. doi: 10.1038/sj.bmt.1701995
34. Kernan NA, Grupp S, Smith AR, Arai S, Triplett B, Antin JH, et al. Final results from a defibrotide treatment-IND study for patients with hepatic veno-occlusive disease/sinusoidal obstruction syndrome. Br J Haematol. (2018) 181:816–27. doi: 10.1111/bjh.15267
35. Patel AV, Hahn T, Bogner PN, Loud PA, Brown K, Paplham, et al. Fatal diffuse alveolar hemorrhage associated with sirolimus after allogeneic hematopoietic cell transplantation. Bone Marrow Transplant. (2010) 45:1363–4. doi: 10.1038/bmt.2009.339
36. Witte RJ, Gurney JW, Robbins RA, Linder J, Rennard SI, Arneson M, et al. Diffuse pulmonary alveolar hemorrhage after bone marrow transplantation: radiographic findings in 39 patients. AJR Am J Roentgenol. (1991) 157:461–4. doi: 10.2214/ajr.157.3.1872226
37. Broglie L, Fretham C, Al-Seraihy A, George B, Kurtzberg J, Loren A, et al. Pulmonary complications in pediatric and adolescent patients following allogeneic hematopoietic cell transplantation. Biol Blood Marrow Transplant. (2019) 25:2024–30. doi: 10.1016/j.bbmt.2019.06.004
38. Heslet L, Nielsen JD, Levi M, Sengelov H, Johansson PI. Successful pulmonary administration of activated recombinant factor VII in diffuse alveolar hemorrhage. Crit Care. (2006) 10:R177. doi: 10.1186/cc5132
39. Haselton DJ, Klekamp JG, Christman BW, Barr FE. Use of high-dose corticosteroids and high-frequency oscillatory ventilation for treatment of a child with diffuse alveolar hemorrhage after bone marrow transplantation: case report and review of the literature. Crit Care Med. (2000) 28:245–8. doi: 10.1097/00003246-200001000-00042
40. Yen KT, Lee AS, Krowka MJ, Burger CD. Pulmonary complications in bone marrow transplantation: a practical approach to diagnosis and treatment. Clin Chest Med. (2004) 25:189–201. doi: 10.1016/S0272-5231(03)00121-7
41. De Lassence A, Fleury-Feith J, Escudier E, Beaune J, Bernaudin JF, Cordonnier C. Alveolar hemorrhage. Diagnostic criteria and results in 194 immunocompromised hosts. Am J Respir Crit Care Med. (1995) 151:157–63. doi: 10.1164/ajrccm.151.1.7812547
42. Spira D, Wirths S, Skowronski F, Pintoffl J, Kaufmann S, Brodoefel H, et al. Diffuse alveolar hemorrhage in patients with hematological malignancies: HRCT patterns of pulmonary involvement and disease course. Clin Imaging. (2013) 37:680–6. doi: 10.1016/j.clinimag.2012.11.005
43. Wanko SO, Broadwater G, Folz RJ, Chao NJ. Diffuse alveolar hemorrhage: retrospective review of clinical outcome in allogeneic transplant recipients treated with aminocaproic acid. Biol Blood Marrow Transplant. (2006) 12:949–53. doi: 10.1016/j.bbmt.2006.05.012
44. Huaringa AJ, Leyva FJ, Giralt SA, Blanco J, Signes-Costa J, Velarde H, et al. Outcome of bone marrow transplantation patients requiring mechanical ventilation. Crit Care Med. (2000) 28:1014–7. doi: 10.1097/00003246-200004000-00017
45. Rathi NK, Tanner AR, Dinh A, Dong W, Feng L, Ensor J, et al. Low-, medium- and high-dose steroids with or without aminocaproic acid in adult hematopoietic SCT patients with diffuse alveolar hemorrhage. Bone Marrow Transplant. (2015) 50:420–6. doi: 10.1038/bmt.2014.287
46. Estella A, Jareno A, Perez-Bello Fontaina L. Intrapulmonary administration of recombinant activated factor VII in diffuse alveolar haemorrhage: a report of two case stories. Cases J. (2008) 1:150. doi: 10.1186/1757-1626-1-150
47. Khorana AA, Francis CW, Blumberg N, Culakova E, Refaai MA, Lyman GH. Blood transfusions, thrombosis, and mortality in hospitalized patients with cancer. Arch Intern Med. (2008) 168:2377–81. doi: 10.1001/archinte.168.21.2377
48. Stolla M, Refaai MA, Heal JM, Spinelli SL, Garraud O, Phipps R, et al. Platelet transfusion - the new immunology of an old therapy. Front Immunol. (2015) 6:28. doi: 10.3389/fimmu.2015.00028
49. Cloutier N, Pare A, Farndale RW, Schumacher HR, Nigrovic PA, Lacroix S, et al. Platelets can enhance vascular permeability. Blood. (2012) 120:1334–43. doi: 10.1182/blood-2012-02-413047
50. Andreu G, Boudjedir K, Muller JY, Pouchol E, Ozier Y, Fevre G, et al. Analysis of transfusion-related acute lung injury and possible transfusion-related acute lung injury reported to the French hemovigilance network from 2007 to 2013. Transfus Med Rev. (2018) 32:16–27. doi: 10.1016/j.tmrv.2017.07.001
51. Nogami K. The utility of thromboelastography in inherited and acquired bleeding disorders. Br J Haematol. (2016) 174:503–14. doi: 10.1111/bjh.14148
52. Wikkelso A, Wetterslev J, Moller AM, Afshari A. Thromboelastography (TEG) or thromboelastometry (ROTEM) to monitor haemostatic treatment versus usual care in adults or children with bleeding. Cochrane Database Syst Rev. (2016) 2016:Cd007871. doi: 10.1002/14651858.CD007871.pub3
53. Bateman ST, Lacroix J, Boven K, Forbes, Barton R, Thomas NJ, et al. Anemia, blood loss, and blood transfusions in North American children in the intensive care unit. Am J Respir Crit Care Med. (2008) 178:26–33. doi: 10.1164/rccm.200711-1637OC
54. Doctor A, Cholette JM, Remy KE, Argent A, Carson JL, Valentine SL, et al. Recommendations on RBC transfusion in general critically ill children based on hemoglobin and/or physiologic thresholds from the pediatric critical care transfusion and anemia expertise initiative. Pediatr Crit Care Med. (2018) 19(Suppl. 1):S98–113. doi: 10.1097/PCC.0000000000001590
55. Hendrickson JE, Hillyer CD. Noninfectious serious hazards of transfusion. Anesth Analg. (2009) 108:759–69. doi: 10.1213/ane.0b013e3181930a6e
56. Lacroix J, Hebert PC, Hutchison JS, Hume HA, Tucci M, Ducruet T, et al. Transfusion strategies for patients in pediatric intensive care units. N Engl J Med. (2007) 356:1609–19. doi: 10.1056/NEJMoa066240
57. Valentine SL, Bembea MM, Muszynski JA, Cholette JM, Doctor A, Spinella PC, et al. Consensus recommendations for RBC transfusion practice in critically Ill children from the pediatric critical care transfusion and anemia expertise initiative. Pediatr Crit Care Med. (2018) 19:884–98. doi: 10.1097/PCC.0000000000001613
58. Rhen T, Cidlowski JA. Antiinflammatory action of glucocorticoids–new mechanisms for old drugs. N Engl J Med. (2005) 353:1711–23. doi: 10.1056/NEJMra050541
59. Strehl C, Ehlers L, Gaber T, Buttgereit F. Glucocorticoids-All-Rounders Tackling the Versatile Players of the Immune System. Front Immunol. (2019) 10:1744. doi: 10.3389/fimmu.2019.01744
60. Stahn C, Buttgereit F. Genomic and nongenomic effects of glucocorticoids. Nat Clin Pract Rheumatol. (2008) 4:525–33. doi: 10.1038/ncprheum0898
61. Saklatvala J. Glucocorticoids: do we know how they work? Arthritis Res. (2002) 4:146–50. doi: 10.1186/ar398
62. de Benedictis FM, Bush A. Corticosteroids in respiratory diseases in children. Am J Respir Crit Care Med. (2012) 185:12–23. doi: 10.1164/rccm.201107-1174CI
63. Lipworth BJ. Therapeutic implications of non-genomic glucocorticoid activity. Lancet. (2000) 356:87–9. doi: 10.1016/S0140-6736(00)02463-6
64. Buttgereit F, da Silva JA, Boers M, Burmester GR, Cutolo M, Jacobs J, et al. Standardised nomenclature for glucocorticoid dosages and glucocorticoid treatment regimens: current questions and tentative answers in rheumatology. Ann Rheum Dis. (2002) 61:718–22. doi: 10.1136/ard.61.8.718
65. Wanner A, Horvath G, Brieva JL, Kumar SD, Mendes ES. Nongenomic actions of glucocorticosteroids on the airway vasculature in asthma. Proc Am Thorac Soc. (2004) 1:235–8. doi: 10.1513/pats.200402-013MS
66. Liu L, Wang YX, Zhou J, Long F, Sun HW, Liu Y, et al. Rapid non-genomic inhibitory effects of glucocorticoids on human neutrophil degranulation. Inflamm Res. (2005) 54:37–41. doi: 10.1007/s00011-004-1320-y
67. Panettieri RA, Schaafsma D, Amrani Y, Koziol-White C, Ostrom R, Tliba O. Non-genomic effects of glucocorticoids: an updated view. Trends Pharmacol Sci. (2019) 40:38–49. doi: 10.1016/j.tips.2018.11.002
68. Zhang Y, Wang SJ, Han ZH, Li YQ, Xue JH, Gao DF, et al. PI3K/AKT signaling pathway plays a role in enhancement of eNOS activity by recombinant human angiotensin converting enzyme 2 in human umbilical vein endothelial cells. Int J Clin Exp Pathol. (2014) 7:8112–7.
69. Boschetto P, Rogers DF, Fabbri LM, Barnes PJ. Corticosteroid inhibition of airway microvascular leakage. Am Rev Respir Dis. (1991) 143:605–9. doi: 10.1164/ajrccm/143.3.605
70. de Benedictis FM, Canny GJ, Levison H. The role of corticosteroids in respiratory diseases of children. Pediatr Pulmonol. (1996) 22:44–57. doi: 10.1002/(SICI)1099-0496(199607)22:1<44::AID-PPUL7>3.0.CO;2-K
71. Jantz MA, Sahn SA. Corticosteroids in acute respiratory failure. Am J Respir Crit Care Med. (1999) 160:1079–100. doi: 10.1164/ajrccm.160.4.9901075
72. Sethi GR, Singhal KK. Pulmonary diseases and corticosteroids. Ind J Pediatr. (2008) 75:1045–56. doi: 10.1007/s12098-008-0209-0
73. Sinha A, Bagga A. Pulse steroid therapy. Ind J Pediatr. (2008) 75:1057–66. doi: 10.1007/s12098-008-0210-7
74. Hari, Srivastava RN. Pulse corticosteroid therapy with methylprednisolone or dexamethasone. Ind J Pediatr. (1998) 65:557–60. doi: 10.1007/BF02730894
75. Buttgereit F, Brand MD, Burmester GR. Equivalent doses and relative drug potencies for non-genomic glucocorticoid effects: a novel glucocorticoid hierarchy. Biochem Pharmacol. (1999) 58:363–8. doi: 10.1016/S0006-2952(99)00090-8
76. Liu D, Ahmet A, Ward L, Krishnamoorthy, Mandelcorn ED, Leigh R, et al. A practical guide to the monitoring and management of the complications of systemic corticosteroid therapy. Allergy Asthma Clin Immunol. (2013) 9:30. doi: 10.1186/1710-1492-9-30
77. Saag KG. Short-term and long-term safety of glucocorticoids in rheumatoid arthritis. Bull NYU Hosp Jt Dis. (2012) 70(Suppl. 1):21–5.
78. Mannucci PM. Hemostatic drugs. N Engl J Med. (1998) 339:245–53. doi: 10.1056/NEJM199807233390407
79. Solomonov A, Fruchter O, Zuckerman T, Brenner B, Yigla M. Pulmonary hemorrhage: a novel mode of therapy. Respir Med. (2009) 103:1196–200. doi: 10.1016/j.rmed.2009.02.004
80. Sindet-Pedersen S, Ramstrom G, Bernvil S, Blomback M. Hemostatic effect of tranexamic acid mouthwash in anticoagulant-treated patients undergoing oral surgery. N Engl J Med. (1989) 320:840–3. doi: 10.1056/NEJM198903303201305
81. O'Neil ER, Schmees LR, Resendiz K, Justino H, Anders MM. Inhaled tranexamic acid as a novel treatment for pulmonary hemorrhage in critically Ill pediatric patients: an observational study. Criti Care Explorations. (2020) 2:e0075. doi: 10.1097/CCE.0000000000000075
82. Von Depka M. NovoSeven: mode of action and use in acquired haemophilia. Intensive Care Med. (2002) 28(Suppl. 2):S222–7. doi: 10.1007/s00134-002-1469-1
83. Hedner U, Kisiel W. Use of human factor VIIa in the treatment of two hemophilia A patients with high-titer inhibitors. J Clin Invest. (1983) 71:1836–41. doi: 10.1172/JCI110939
84. Mathew P. The use of rFVIIa in non-haemophilia bleeding conditions in paediatrics. A systematic review. Thromb Haemost. (2004) 92:738–46. doi: 10.1160/TH04-03-0163
85. Henke D, Falk RJ, Gabriel DA. Successful treatment of diffuse alveolar hemorrhage with activated factor VII. Ann Intern Med. (2004) 140:493–4. doi: 10.7326/0003-4819-140-6-200403160-00033
86. Hicks K, Peng D, Gajewski JL. Treatment of diffuse alveolar hemorrhage after allogeneic bone marrow transplant with recombinant factor VIIa. Bone Marrow Transplant. (2002) 30:975–8. doi: 10.1038/sj.bmt.1703731
87. Pastores SM, Papadopoulos E, Voigt L, Halpern NA. Diffuse alveolar hemorrhage after allogeneic hematopoietic stem-cell transplantation: treatment with recombinant factor VIIa. Chest. (2003) 124:2400–3. doi: 10.1016/S0012-3692(15)31709-8
88. Colin AA, Shafieian M, Andreansky M. Bronchoscopic instillation of activated recombinant factor VII to treat diffuse alveolar hemorrhage in a child. Pediatr Pulmonol. (2010) 45:411. doi: 10.1002/ppul.21178
89. Larcombe PJ, Kapur N, Fraser CJ, Coulthard MG, Schlapbach LJ. Intrabronchial administration of activated recombinant factor VII in a young child with diffuse alveolar hemorrhage. Pediatr Blood Cancer. (2014) 61:570–1. doi: 10.1002/pbc.24841
90. Hurley C, Ghafoor S, Hall E, Qudeimat A, McArthur J. Intrapulmonary administration of recombinant activated factore VII for diffuse alveolar hemorrhage. In: The American Society of Pediatric Hematology/Oncology Conference. New Orleans, LA. (2019).
91. Delvino P, Monti S, Balduzzi S, Belliato M, Montecucco C, Caporali R. The role of extra-corporeal membrane oxygenation (ECMO) in the treatment of diffuse alveolar haemorrhage secondary to ANCA-associated vasculitis: report of two cases and review of the literature. Rheumatol Int. (2019) 39:367–75. doi: 10.1007/s00296-018-4116-z
92. Morris SH, Haight AE, Kamat, Fortenberry JD. Successful use of extracorporeal life support in a hematopoietic stem cell transplant patient with diffuse alveolar hemorrhage. Pediatr Crit Care Med. (2010) 11:e4–7. doi: 10.1097/PCC.0b013e3181b00e63
93. Ransone S, Hurley C, Sandhu H, Ghafoor S, Morrison RR, Shah S. Extracorporeal membrane oxygenation in a pediatric HSCT recipient: a case report. In: 27th Annual Extracoporeal Life Support Organization/AmSET meeting. San Diego: CA (2016).
94. Srivastava A, Gottlieb D, Bradstock KF. Diffuse alveolar haemorrhage associated with microangiopathy after allogeneic bone marrow transplantation. Bone Marrow Transplant. (1995) 15:863–7.
95. Ho VT, Cutler C, Carter S, Martin, Adams R, Horowitz M, et al. Blood and marrow transplant clinical trials network toxicity committee consensus summary: thrombotic microangiopathy after hematopoietic stem cell transplantation. Biol Blood Marrow Transplant. (2005) 11:571–5. doi: 10.1016/j.bbmt.2005.06.001
96. Seaby EG, Gilbert RD. Thrombotic microangiopathy following haematopoietic stem cell transplant. Pediatr Nephrol. (2018) 33:1489–500. doi: 10.1007/s00467-017-3803-4
97. Daly AS, Xenocostas A, Lipton JH. Transplantation-associated thrombotic microangiopathy: twenty-two years later. Bone Marrow Transplant. (2002) 30:709–15. doi: 10.1038/sj.bmt.1703710
98. Panoskaltsis-Mortari A, Griese M, Madtes DK, Belperio JA, Haddad IY, Folz RJ, et al. An official American thoracic society research statement: noninfectious lung injury after hematopoietic stem cell transplantation: idiopathic pneumonia syndrome. Am J Respir Crit Care Med. (2011) 183:1262–79. doi: 10.1164/rccm.2007-413ST
99. Yanik GA, Grupp SA, Pulsipher MA, Levine JE, Schultz KR, Wall DA, et al. TNF-receptor inhibitor therapy for the treatment of children with idiopathic pneumonia syndrome. A joint pediatric blood and marrow transplant consortium and children's oncology group study (ASCT0521). Biol Blood Marrow Transplant. (2015) 21:67–73. doi: 10.1016/j.bbmt.2014.09.019
100. Nanjappa S, Jeong DK, Muddaraju M, Jeong K, Hill ED, Greene JN. Diffuse alveolar hemorrhage in acute myeloid leukemia. Cancer Control. (2016) 23:272–7. doi: 10.1177/107327481602300310
101. Maile CW, Moore AV, Ulreich S, Putman CE. Chest radiographic-pathologic correlation in adult leukemia patients. Invest Radiol. (1983) 18:495–9. doi: 10.1097/00004424-198311000-00002
102. Van de Louw A, Lewis AM, Yang Z. Autopsy findings in patients with acute myeloid leukemia and non-Hodgkin lymphoma in the modern era: a focus on lung pathology and acute respiratory failure. Ann Hematol. (2019) 98:119–29. doi: 10.1007/s00277-018-3494-3
103. Azoulay É, Fieux F, Moreau D, Thiery G, Rousselot, Parrot A, et al. Acute monocytic leukemia presenting as acute respiratory failure. Am J Respir Criti Care Med. (2003) 167:1329–33. doi: 10.1164/rccm.200206-554OC
Keywords: diffuse alveolar hemorrhage, hematopoietic cell transplant (HCT) complications, pediatric oncology, glucocorticoids, thrombotic microangiopathy, graft vs. host disease, pulmonary hemorrhage, extracorporeal membrane oxygenation (ECMO)
Citation: Fan K, McArthur J, Morrison RR and Ghafoor S (2020) Diffuse Alveolar Hemorrhage After Pediatric Hematopoietic Stem Cell Transplantation. Front. Oncol. 10:1757. doi: 10.3389/fonc.2020.01757
Received: 06 June 2020; Accepted: 05 August 2020;
Published: 09 September 2020.
Edited by:
Giuseppe Maria Milano, Bambino Gesù Children Hospital (IRCCS), ItalyReviewed by:
Joseph Louis Lasky, Cure 4 the Kids, United StatesHema Dave, Children's National Hospital, United States
Copyright © 2020 Fan, McArthur, Morrison and Ghafoor. This is an open-access article distributed under the terms of the Creative Commons Attribution License (CC BY). The use, distribution or reproduction in other forums is permitted, provided the original author(s) and the copyright owner(s) are credited and that the original publication in this journal is cited, in accordance with accepted academic practice. No use, distribution or reproduction is permitted which does not comply with these terms.
*Correspondence: Saad Ghafoor, saad.ghafoor@stjude.org