- 1Centre de Recherche en Cancérologie de Lyon, INSERM U1052, CNRS UMR5286, Université de Lyon, Université Lyon 1, Centre Léon Bérard, Lyon, France
- 2Centre International de Recherche en Infectiologie, INSERM U1111 – CNRS UMR5308, Université de Lyon, ENS de Lyon, Université Lyon 1, Lyon, France
Inflammasomes are molecular complexes that trigger an inflammatory response upon detection of pathogens or danger signals. Recent studies suggest that they are also involved in cancer progression. However, their roles during tumorigenesis remain poorly understood and controversial. Here, we investigated whether inflammasome activation supports mammary tumor growth. Using mouse models of invasive breast cancer, our results demonstrate that the absence of a functional inflammasome impairs tumor growth. Importantly, tumors implanted into inflammasome-deficient mice recruited significantly less neutrophils and more natural killer (NK) cells, and these latter cells displayed a more active phenotype. Interestingly, NK cell depletion abolished the anti-tumoral effect observed in inflammasome-deficient mice, although inflammasome-regulated cytokine neutralization had no effect. Thus, our work identifies a novel role for the inflammasome in supporting mammary tumor growth by attenuating NK cell recruitment and activity. These results suggest that inflammasome inhibition could be a putative target for treating invasive breast cancers.
Introduction
Breast cancers are often infiltrated with immune cells that have pro- or anti-tumoral functions (1, 2). The composition of the immune infiltrate and the level of infiltration have been correlated with patient prognosis in several types of cancers (3–7). Chronic inflammation taking place within tumors can promote tumor progression by stimulating angiogenesis or inhibiting anti-tumoral immunity (8, 9).
Within injured tissues, innate immune cells sense pathogen- or danger-associated molecular patterns (PAMPs, DAMPs) using germline-encoded pattern recognition receptors (PRRs) that drive inflammation to restore homeostasis. Among these PRRs, specific NOD-like receptors (NLRs, such as NLRP3) and hematopoietic interferon-inducible nuclear antigens with 200 amino acid repeats (HIN200) protein families, operate by forming multiprotein complexes named inflammasomes. Besides PRRs, inflammasomes are composed of the adaptor protein associated speck-like containing a CARD protein (ASC) and of the cysteine protease caspase-1 (10). Once activated within the inflammasome complex, caspase-1 cleaves and activates two major pro-inflammatory cytokines namely the pro-IL-1β and the pro-IL-18, and drives an inflammatory cell death known as pyroptosis through gasdermin D cleavage (11, 12).
In the context of cancer, the role of the inflammasome is complex as it can both promote anti- and pro-tumoral responses. For instance, Nlrp3-, Nlrc4-, and Caspase-1-deficient mice are more sensitive to colorectal cancer induced by DSS-AOM treatment, suggesting an anti-tumoral role for the inflammasome in the gut (13–15). This protective role is mediated by the production of IL-18, which is involved in maintaining the intestinal epithelial barrier integrity. Conversely, IL-18 is a critical driver of immune suppression in a model of multiple myeloma, in which it fuels the development of myeloid-derived suppressor cells (MDSCs) (16). Moreover, the NLRP3 inflammasome was shown to promote tumor growth in models of carcinogen-induced sarcoma and skin papilloma through the release of IL-1β (17, 18). Thus, inflammasome activation may dampen or promote anti-tumor responses depending on the tumor type, the stage of tumorigenesis and the model studied. This intricacy is reinforced by the wide range of expression of some inflammasome components in immune and non-hematopoietic cells. For instance, in a carcinogen-induced skin cancer model, ASC depletion in keratinocytes facilitates tumor development, whereas its loss in myeloid cells impairs it (19).
With respect to breast cancer, the presence of IL-1β within the tumor microenvironment is frequently associated with poor prognosis, suggesting a pro-tumoral role for this cytokine (20–24). For instance, in the MMTV-NeuV664E BALB/c model, the invasive conversion of the mammary tumors was associated with an upregulation of the IL-1β transcriptional signature (25). In the 4T1 murine model, which is used as a preclinical model for invasive breast cancer, IL-1β promotes tumor growth and the capacity of cells to metastasize (26, 27). Yet, the role of inflammasomes is not limited to IL-1β production and the overall impact of this pathway in the anti-breast cancer response remains unclear. We thus tested whether the inflammasome supports invasive breast cancer development in vivo by using mice deficient in major inflammasome components.
Materials and Methods
Mouse Tumor Cell Lines
4T1 and YAC-1 cells were cultured in RPMI medium supplemented with 10% (v/v) heat-inactivated FBS (Life technologies), 1% (v/v) penicillin/streptomycin, 1% (v/v) L-glutamine, and 25 μM 2-mercaptoethanol (only 4T1 cells) at 37°C in a 5% CO2 incubator. 4T1 cells were proven to be mycoplasma-free (MycoAlert Mycoplasma detection kit, Lonza) before each injection and experiment. Cells were also proven to be free of mouse infectious agents by Taqman® PCR testing of mouse essential panel (Charles River).
Mice
Nlrp3 knockout (KO) mice were obtained from J. Tschopp (28), Asc KO mice from V. M. Dixit (29), and Caspase-1/Caspase-11 KO mice referred as Caspase-1 KO in the text from R. A. Flavell (30). MMTV-NeuV664E in the BALB/c from F Cavallo (31). The three transgenic KO strains were backcrossed with a BALB/c/Ola (Harlan strain) background for at least nine generations. WT animals were littermates of the Caspase-1/Caspase-11 knockout, Asc knockout, or Nlrp3 knockout colonies or imported from Harlan and maintained in the same cages as KO animals. Animals were housed in individually ventilated cages under specific pathogen-free conditions, fed with Harlan Teklad food pellets and studies were conducted in accordance with the regulations for animals used for scientific purposes governed by the European Directive 2010/63/EU. Protocols were validated by the local Animal Ethic Evaluation Committee (CECCAPP: C2EA-15, Comité d'Evaluation Commun au PBES, à AniCan, au laboratoire P4, à l'animalerie de transit de l'ENS, à l'animalerie de l'IGFL, au PRECI, à l'animalerie du Cours Albert Thomas, au CARRTEL INRA Thonon-les-Bains et à l'animalerie de transit de l'IBCP, CLB-2013-019, CLB-2015-015) and authorized by the French Ministry of Education and Research.
Bone Marrow Mouse Chimera
Five-week-old mice received antibiotics 2 days prior to being exposed to 6 Gy g-irradiations. The day of irradiation, bone marrow (BM) was flushed with 5 mL of RPMI from the hind legs of mice and CD3+ cells were depleted using the CD3 MicroBead Kit (Miltenyi biotec). 106 BM cells in PBS supplemented with 0.1% penicillin/streptomycin were re-injected intravenously (I.V.). Recipient animals recovered for 4 weeks before tumor injection.
Tumor Growth Assays
Only virgin female BALB/c/Ola mice aged 7 to 10 weeks were used for in vivo experiments. 20,000 4T1 tumor cells in 100 μL of sterile PBS were injected orthotopically into the 4th mammary fat pad. Primary tumor growth was monitored with a digital caliper measurement and expressed as a tumor volume (ellipsoidal formula, π/6 × length × width2). Mice were sacrificed when tumor size reached 1,200 mm3.
MMTV-NeuV664E mice were monitored over time for tumor appearance through palpation (~100 mm3).
Cell Suspensions From Spleens or Tumors
Spleens isolated from mice were crushed and filtered through a 40-μm filter and resuspended in FACS buffer (PBS supplemented with 5% (vol/vol) FBS, 2 mM EDTA). Red blood cells were lysed in 5 mL of erythrocyte lysis buffer (155 mM NH4Cl, 12 mM NaHCO3, 0.1 mM EDTA) for 5 min. After a PBS wash, cells were then resuspended in FACS buffer.
Seven or 14 days post-injection of 4T1 cells, tumors isolated from mice were cut into small pieces and incubated with 5 mL of DMEM supplemented with DNase 0.02 mg/mL (Sigma D4513)—Collagenase 1 mg/mL (Sigma C2674) for 30 min at 37°C. Digested tumors were then filtered through a 40-μm filter and re-suspended in FACS buffer and filtered again twice. Red blood cells were lysed in 5 mL of erythrocyte lysis buffer for 5 min. After a PBS wash, cells were then resuspended in FACS buffer.
Flow Cytometry
Cell suspensions from spleens or from tumors were washed in FACS buffer (PBS supplemented with 2 mM EDTA and 5% (vol/vol) FBS) and incubated for 5 min with purified anti-mouse CD16/32 FcBlocks (93; Biolegend). Cells were stained with fluorochrome-conjugated antibodies (Supplementary Table 1) at 4°C for 20 min LIVE/DEADTM Fixable Aqua Dead Cell Stain Kit, 405 nm excitation (Invitrogen L34965) was used to gate on live cells. For cell surface staining, cells were washed twice in FACS buffer, fixed in PBS 2% paraformaldehyde and stored in FACS buffer before analysis. For intracellular staining, cells were fixed/permeabilized with the Cytofix/Cytoperm kit (554714, BD Bioscience) for 20 min on ice. Cells were washed with PermWash buffer and stained with fluorochrome-conjugated antibodies diluted (Supplementary Table 1) in PermWash buffer for 30 min on ice. Cells were washed again in PermWash buffer and kept at 4°C before analysis. Data were collected on a LSR II Fortessa (BD Bioscience) and analyzed using the FlowJo software.
Luminex Assay
Tumors were prepared as described above. Digested tumors were centrifuged and supernatants were used for luminex assay according to manufacturer protocol (mouse pre-mixed multianalyte test reference LXAMSM-18 R&Dsystems).
NK Cell Depletion
Mice were injected I.V. (in the retro-orbital sinus) with 50 μL of the Ultra-LEAFTM Purified anti-Asialo-GM1 (clone: Poly21460, Biolegend) antibody 1 day prior to tumor cell injection. In order to maintain NK cell depletion during tumor growth, mice were injected I.V. every 10 days.
NK Cell Activation
3.106 splenic lymphocytes or tumor cell suspensions were prepared in complete medium (RPMI + glutamax, 10% SVF, 1% penicillin/streptomycin, 10 mM HEPES, 1 mM sodium-pyruvate, 50 μM 2-mercaptoethanol) and incubated for 4 h with cytokines [recombinant mouse IL-12 (Peprotech, 200-12) (final concentration: 100 ng/mL) and recombinant mouse IL-18 (R&D, B004-5) (final concentration: 20 ng/mL)] or on antibody-coated plates [anti-NKp46 (29A1; BD Biosciences), anti-Ly49D (4E5; BD Biosciences), anti-NKG2D (CX5; BD Biosciences), and GolgiStop (BD Biosciences) in the presence of anti-CD107a (2B6; BD Biosciences)] or co-cultured with Yac-1 or 4T1 cells (1:1 ratio).
In vivo Cytokine Depletion
Mice were injected intraperitoneally (I.P.) with 2.5 mg/kg of body weight (B.W.) of anti-IL-1β antibody (Clone B122, Biolegend, 503504), or 0.25 mg/kg of B.W. IL-18 binding protein (IL-18BPd-FC) (R&D systems, 122-BP), or both, or control IgG 1 day prior to the tumor cell injection and every 3 days after that. Anakinra (Kineret®) was administered I.P. (20 mg/kg of B.W.) prior to tumor cell inoculation and every 2 days after that. The second anti-IL-1b (AF-401-NA; R&D Systems) or control isotype was injected i.p. at a dose of 10 μg per mouse twice a week as described in (32).
Statistical Analysis
Statistical analysis of each experiment was conducted using the GraphPad Prism software. One-way or two-way ANOVA were used followed by Bonferroni's Post-test to compare tumor progression and immune cell infiltration.
Results
The Absence of a Functional Inflammasome Impairs Mammary Tumor Growth in Mice
To assess the impact of the inflammasome on mammary cancer progression in vivo, MMTV-NeuV664E mice were bred with Caspase-1 knock-out (KO) mice, the main inflammasome effector, and the number of tumor-free mice was monitored over time. As shown in the Figure 1, the absence of caspase-1 significantly delayed tumor onset in mice suggesting a pro-tumoral role for the inflammasome. The difference in the age of onset was however, modest and could be due to the fact that caspase-1 is well-expressed in different tissues including mammary cells and could display opposite functions as described above for ASC (19). We thus decided to use syngeneic 4T1 carcinoma cells to explore the effect of the presence of the inflammasome within the tumor microenvironment on tumor progression. The cells were injected into the mammary fat pad of WT, Caspase-1 KO and Asc KO BALB/c mice, respectively, and tumor growth was monitored over time. While inoculation of female WT BALB/c mice with cancer cells gave rise to large tumors within 30 days, the absence of caspase-1 or ASC resulted in significantly smaller tumors (Figures 2A,B), suggesting that inflammasomes likely support mammary tumor growth. To ascertain whether NLRP3 was also involved, we compared 4T1 cell growth in Nlrp3-deficient and Nlrp3-sufficient WT mice. Indeed, NLRP3 appeared to be a good candidate as this receptor is well-described to sense DAMPs, such as ATP or uric acid released by necrotic cells, and since necrosis of tumor cells is frequent during cancer progression (33, 34). As shown in Figures 2C,D, tumor sizes were similar between WT and Nlrp3 KO mice, indicating that NLRP3 does not support mammary tumor growth in vivo, unlike caspase-1 and ASC.
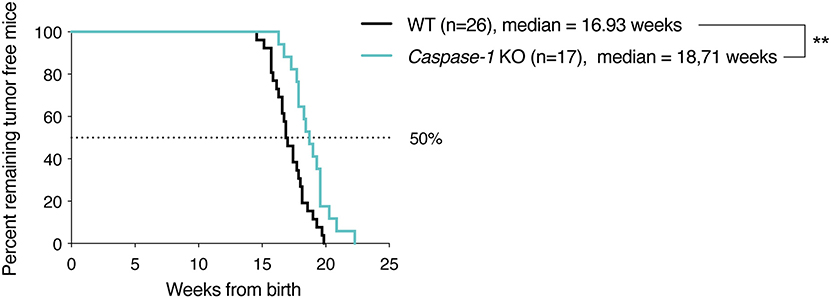
Figure 1. Caspase-1 deficiency delays tumor appearance in MMTV-NeuV664E BALB/c mouse model. Kaplan–Meier curves depicting tumor growth latency of MMTV-NeuV664E mice, defined as time from birth until appearance of the first palpable tumor. **P < 0.01 (Comparison of survival curves: Gehan–Breslow–Wilcoxon test).
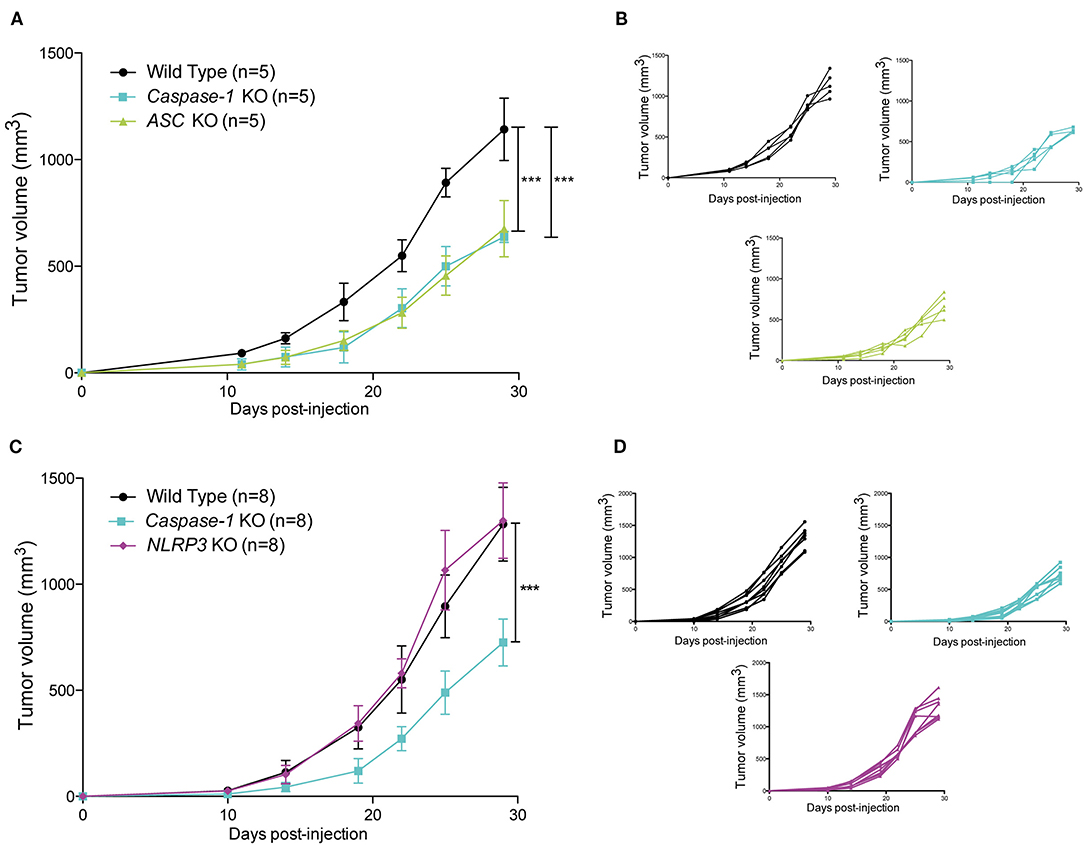
Figure 2. Loss of caspase-1 and ASC expression reduces 4T1 tumor growth in BALB/c mice. (A) WT, Caspase-1 KO, and Asc KO mice (n = 5) were injected orthotopically with 4T1 mammary tumor cells. Tumor growth was measured over 30 days. (B) Individual growth curves depicted in A. (C) WT, Caspase-1 KO, and Nlrp3 KO mice (n = 8) were injected orthotopically with 4T1 mammary tumor cells. Tumor growth was measured over 30 days. (D) Individual growth curves depicted in C. Data represent mean ± SD ***P < 0.001 (Two-way ANOVA analysis; n.s, non-significant).
Inflammasome Expression in the Hematopoietic Compartment Supports Tumor Growth
As previously mentioned, caspase-1 is the main catalytic subunit of the inflammasome. Its expression is not restricted to immune cells as it is also expressed by many non-hematopoietic cell types such as epithelial cells or adipocytes (35, 36). To evaluate the role of inflammasome components in tumor growth in immune vs. non-immune cells, we first generated a series of bone marrow chimeric mice to obtain different combinations of caspase-1 expression in immune and non-immune cells as indicated in Figure 3A. Thirty days post reconstitution, mice were inoculated with 4T1 cells and tumor growth was monitored. The growth rate of 4T1 cells was determined by the expression of caspase-1 in immune cells, and independent of its expression in non-immune cells, as illustrated in Figures 3A,B in recipient mice with different genotypes. Thus, the absence of caspase-1 in the hematopoietic cell lineage is responsible for the delay in tumor growth, suggesting that caspase-1-expressing immune cells support cancer progression.
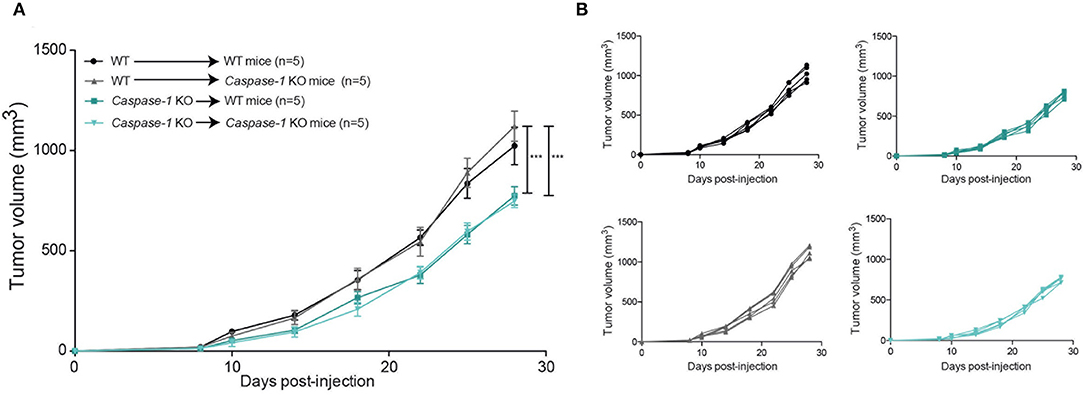
Figure 3. The presence of the inflammasome in the hematopoietic compartment promotes mammary tumor growth. Irradiated mice of indicated genotypes were grafted with bone marrow stem cells from WT or Caspase-1 KO mice and were injected with 4T1 mammary tumor cells 28 days later. (A) Tumor growth was measured over 30 days. (B) Individual growth curves depicted in A. Data represent mean ± SD ***P < 0.001 (Two-way ANOVA analysis).
The Inflammasome Impairs NK Cell Recruitment to the Tumor
Activation of the inflammasome has been shown to modulate the composition of the tumor immune infiltrate (37). To address this finding in our model, we analyzed the tumor immune infiltrate in the mammary gland at days 7 and 14 post-injection in WT, Asc-, and Caspase-1-deficient mice. Different myeloid and lymphoid cell subtypes were studied by flow cytometry.
With respect to the myeloid compartment, our analysis of the CD45+ infiltrate showed that the frequency of neutrophils (defined as Ly6Cint-Ly6Ghigh) was significantly decreased at both time points in Caspase-1- or Asc- deficient mice compared to control mice, while the abundance of monocytes, macrophages, dendritic cells (DC) and eosinophils was similar in all mouse groups (Figures 4A,B and Supplementary Figures 1A,C).
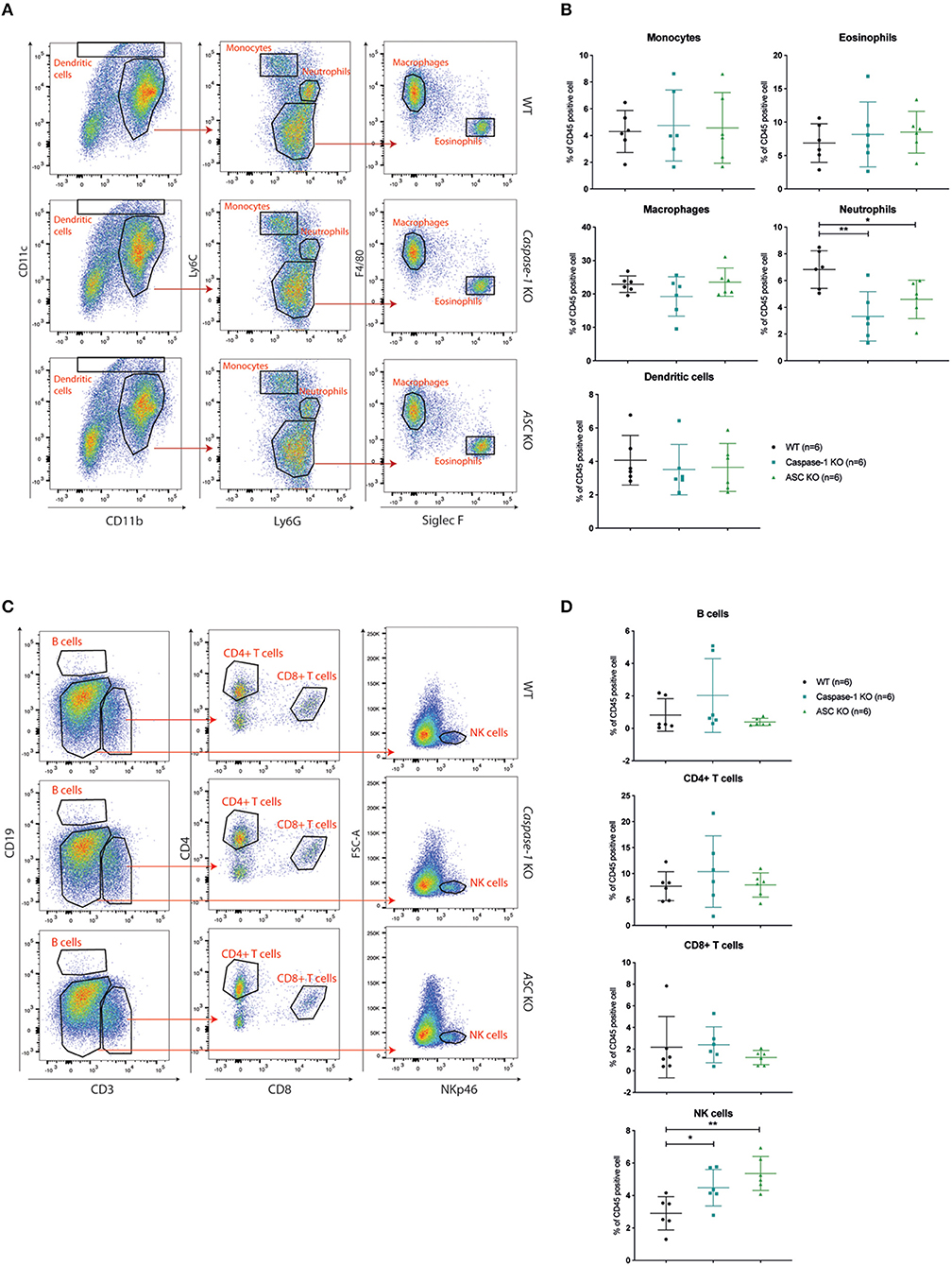
Figure 4. Caspase-1 or ASC deficiency improves NK cell recruitment 14 days post-injection. (A) Flow cytometry analysis of myeloid cell populations in 4T1 tumors at day 14 post-injection from WT (n = 6), Caspase-1 KO (n = 6), and Asc KO (n = 6) mice. The displayed dotplots were obtained by gating live and CD45+ cells. (B) Quantification and analysis of myeloid cell populations in the different mouse genotypes. (C) Flow cytometry analysis of lymphoid cell populations in 4T1 tumors from the same mice as in A. The displayed dotplots were gated from live and CD45+ cells. (D) Quantification and analysis of lymphoid cell populations in the different mouse genotypes. Data represent mean ± SD *P < 0.05; **P < 0.01 (One-way ANOVA test followed by Bonferroni's Multiple Comparison Test).
Regarding lymphoid cells, no significant difference in CD4+ or CD8+ T lymphocyte recruitment was observed between WT and inflammasome-deficient mice at day 7 and day 14 post-injection and very few B cells had infiltrated the tumors (Figures 4C,D and Supplementary Figure 1D). However, the frequency of infiltrating NK cells (NKp46+ cells) was significantly higher in tumors implanted in Caspase-1 and Asc KO mice compared with WT mice at day 7 and day 14 (Figures 4C,D and Supplementary Figure 1B). Differences in tumor infiltrates between the groups of mice were not due to pre-existing differences in these mice, as the immune composition of the spleen of tumor-bearing mice was similar in WT, Asc, and Caspase-1 KO mice at day 14 (Supplementary Figures 1E,F).
Inhibition of Inflammasome-Regulated Cytokine Production Does Not Affect the Rate of Tumor Growth
The inflammasome controls the production of IL-1β and IL-18 and both cytokines are involved in tumor development or control. We wondered whether blocking IL-1β and IL-18 would impact the ability of 4T1 cells to grow in WT mice. Surprisingly, treatment with either the anti-IL-1β antibodies or the IL-18 binding protein (BP) or both did not affect the rate of tumor growth (Figure 5A, Supplementary Figure 2). Similarly, Anakinra (recombinant IL-1Ra) injection did not delay tumor growth in WT mice, suggesting that inflammasome-regulated cytokines are not essential for controlling 4T1 cell growth in vivo (Figure 5B) (24, 32, 38). Finally, cytokine measurement using multiplex technology of tumor supernatants at day 14 showed no difference in the amount of IL-1β, IL-33, CCL3/MIP1α, KC or β-FGF, between WT and Caspase-1 KO, while CCL5/RANTES was significantly increased in Caspase-1 KO (Supplementary Figure 3).
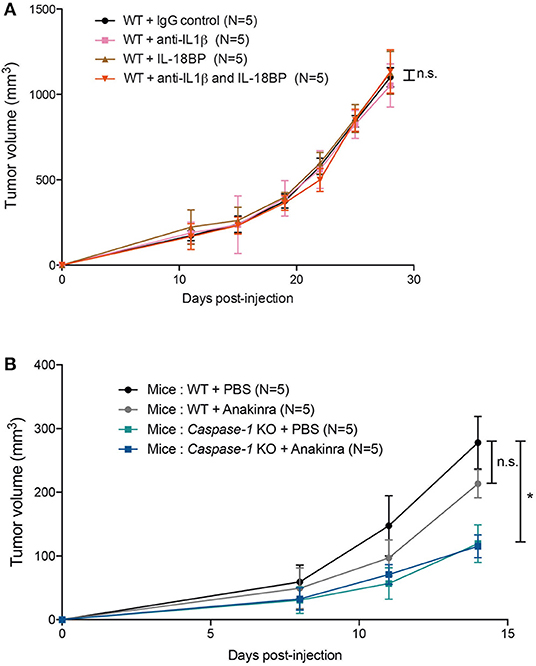
Figure 5. IL-1β, IL-18 or IL-1R inhibition does not affect 4T1 tumor growth in vivo. (A) WT (n = 5) were injected with IgG control, anti-IL1β antibody, IL-18BP, or both anti-IL1β and IL-18BP the day before tumor inoculation and then every 3 days. Treated mice were orthotopically injected with 4T1 mammary tumor cells. Tumor growth was measured over 28 days. (B) WT, caspase-1 KO were injected with PBS or Anakinra (IL-1 receptor inhibitor) the day before tumor inoculation and then every 2 days. Treated mice were orthotopically injected with 4T1 mammary tumor cells. Tumor growth was measured over 14 days. Data represent mean ± SD *P < 0.05 (Two-way ANOVA analysis; n.s, non-significant).
Caspase-1 Deficiency Improves NK Cell Anti-tumor Activity
Since NK cells, which are important anti-tumor effectors (39, 40), were preferentially recruited into the tumors of inflammasome-deficient mice, and since increased levels of CCL5 within the tumor microenvironment were detected, we tested whether NK cells were responsible for the reduction in tumor growth. Caspase-1 KO and WT mice were depleted of NK cells by I.V. injection of the anti-Asialo GM1 antibody before being inoculated with 4T1 cells. Upon NK cell depletion, tumors grew at the same rate in both groups of mice, demonstrating a major role for NK cells in mammary tumor growth control (Figures 6A,B).
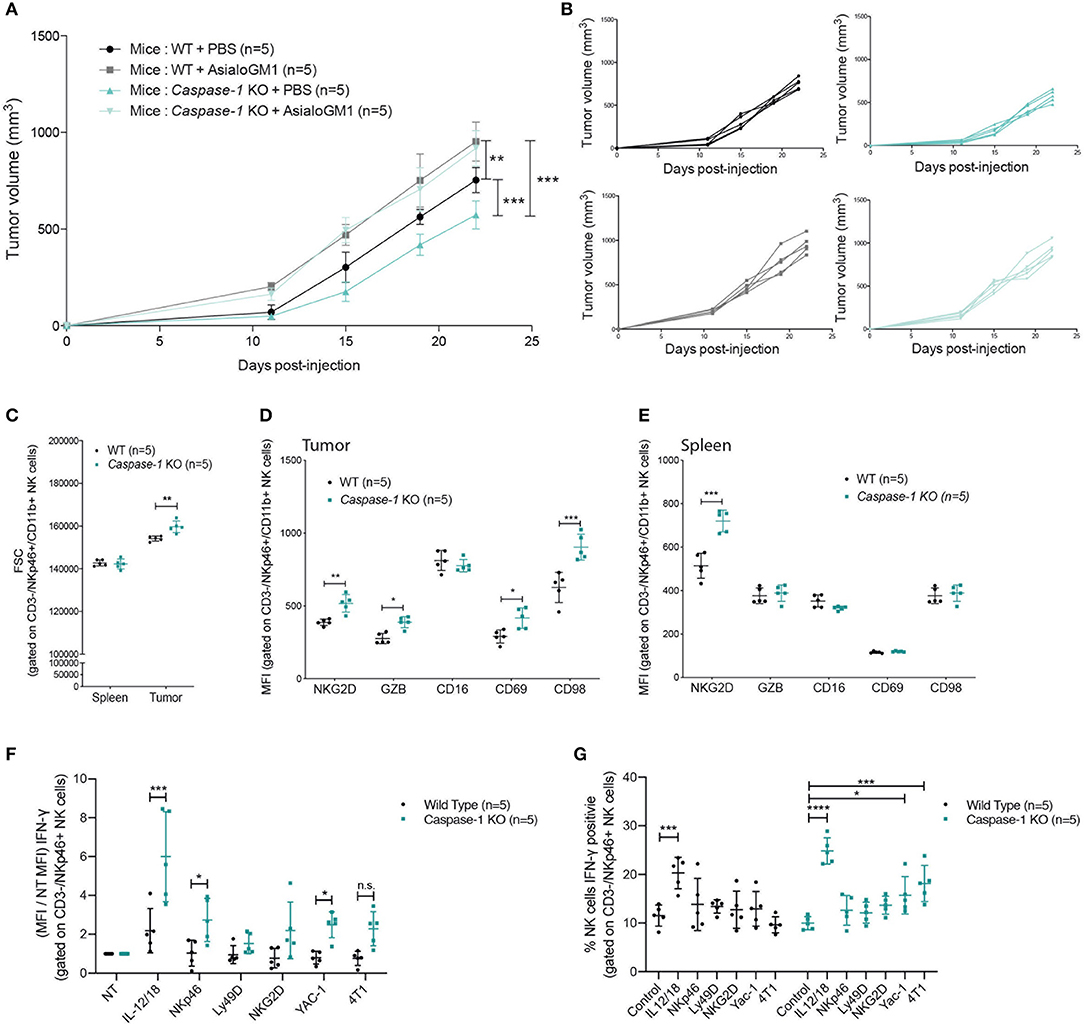
Figure 6. NK cells control tumor growth and are more active in caspase-1 KO mice. (A) Tumor growth of 4T1 cells was measured in NK-depleted (anti-asialo GM1) or PBS-treated WT and caspase-1 KO mice (n = 5). Each value represents mean ± SD **P < 0.01; ***P < 0.001 (Two-way ANOVA analysis). (B) Individual growth curves depicted in (A). (C–E) Flow cytometry analysis of NK cell size in tumors and spleens (C) and activation markers in 4T1 tumors (D) and in spleen (E) from WT (n = 5) and Caspase-1 KO (n = 5) mice at day 7 post-injection. (F) Cell suspensions from digested tumors of the indicated mouse genotype were cultured in the presence of cytokines (IL-12/IL-18), antibodies (NKp46, Ly49D, NKG2D) or tumor cells (YAC-1, 4T1) and NK cell IFN-γ production (F) or IFN-γpositive cells (G) were measured by flow cytometry. Data from (C–G) represent mean ± SD *P < 0.05; **P < 0.01; ***P < 0.001; ****P < 0.0001 (Two-way ANOVA test followed by Bonferroni's Post-test; ns, non-significant).
We then asked whether caspase-1 expression within the immune compartment could affect NK cell phenotype and activation. As shown in Figure 6C, NK cells infiltrating the mammary tumors of Caspase-1 KO were larger (according to FSC parameters) than those infiltrating WT mice, a feature which is commonly associated with a stronger activation status (41). Furthermore, NK cells present in the tumor of Caspase-1-deficient hosts expressed higher levels of NK cell activation markers NKG2D, Granzyme B (GZB), CD69 and CD98 (Figure 6D) (42). With the exception of NKG2D, few differences were detected between splenic NK cells from WT and Caspase-1-deficient tumor-bearing mice, indicating that the tumor microenvironment directly impacts NK cell phenotype (Figure 6E).
We then investigated the ability of tumor infiltrating NK cells to be activated when re-stimulated in vitro. Tumor cell suspensions from WT or Caspase-1 KO mice were incubated with a combination of IL-12+IL-18 cytokines, or with crosslinking antibodies against activating receptors (NKp46, Ly49D, NKG2D) or with different tumor cell lines (4T1, and YAC1 cells, a classical NK cell target). Interestingly, NK cells from tumors growing in Caspase-1 KO mice displayed an increased expression of IFN-γ following activation compared to those from tumors growing in WT mice, irrespective of the stimulus used (Figure 6F and Supplementary Figure 4A). In addition, in the tumor cell suspension from Caspase-1 KO mice, significantly more NK cells were activated (positive to IFN-γ) in response to YAC-1 or 4T1 stimulation compared with WT (Figure 6G and Supplementary Figure 4B). These results suggest that the absence of caspase-1 from the tumor immune microenvironment promotes NK cell activation.
Discussion
The role of inflammasome activation in cancer (15, 43) remains largely undefined and can be either pro-tumoral or anti-tumoral. The data presented here suggest that the inflammasome promotes the growth of invasive breast cancer in two mouse models, the MMTV-Neu and 4T1 cells. Interestingly, bone marrow chimeric mouse experiments demonstrated that caspase-1-expressing immune cells promote mammary tumor progression.
Despite the fact that necrotic cell death occurs during tumor progression releasing DAMPs, such as ATP, or uric acid, tumor growth was independent of NLRP3, suggesting either redundancy or the involvement of another PRR. Other innate immune receptors such as AIM2 or NLRC4 can form inflammasome platforms. AIM2 is a DNA sensor, which can be activated by circulating-free DNA released by dying cells (44, 45). And NLRC4 was recently shown to promote mammary tumor growth in a model of high fat diet-induced obesity via the production of IL-1β (46). Further experiments would be required using KO animals in the BALB/c background to determine their putative involvement in tumor progression of invasive breast cancer.
NK cells are an important aspect of the anti-tumor arsenal and their presence is associated with good prognosis in several types of cancers (47, 48). However, during cancer progression malignant cells develop different strategies to escape or to dampen NK cell functions (39, 49). Indeed, NK cell activity was shown to be reduced in the blood of primary and metastatic breast cancer patients (50).
With respect to the role of the inflammasome on NK cell function, former studies mostly addressed its involvement in mouse models of cancer metastasis (15). For instance, Nlrp3-deficient mice displayed reduced number of melanoma lung metastasis due to more active NK cells in a caspase-1-independent way, while in the context of colon metastasis to the liver, caspase-1 and NLRP3 were protective by promoting more active NK cells in the livers of WT mice compared with Caspase-1-deficient mice (17, 37). Here, we showed for the first time that the absence of a functional inflammasome improved NK cell recruitment and activation in the mammary tumor microenvironment. Higher levels of CCL5 were also detected in the tumor supernatant of Caspase-1-deficient tumors consistent with an increase in NK cells recruitment and activation (51, 52). We further showed that NK cells from Caspase-1-deficient mice responded better to ex vivo re-stimulations, and NK cell depletion in WT and Caspase-1-deficient mice resulted in similar tumor growth rates, demonstrating the major role of NK cells on tumor growth control. Since the phenotype of NK cells was similar in WT and in Caspase-1 KO mouse spleens, our results suggest that the tumor microenvironment directly modulates NK cell anti-tumor response, as previously described in invasive breast cancer and non-small cell lung cancer (NSCLC) models (53, 54).
Our observations also revealed that Ly6Cint-Ly6Ghigh neutrophils were less abundant in the tumor microenvironment of inflammasome-deficient mice. Several studies have described these tumor infiltrating cells as myeloid-derived suppressor cell (MDSC) populations, due to their ability to down-regulate the anti-cancer immune response (55, 56). For instance, MDSC recruited within the tumor bed are able to suppress NK cell cytotoxicity, IFN-γ production and NKG2D expression (57). MDSCs promote primary and metastatic 4T1 tumor progression (26), and impairing their recruitment to the tumor microenvironment limits tumor growth (22, 58). Intriguingly, Chow et al. reported in Nlrp3 KO lungs the presence of a CD11b+ Gr-1int population which, upon adoptive transfer into WT animals, suppressed lung metastasis of melanoma cells (17). Moreover, those cells secreted CCL5. However, we did not observe the recruitment of a similar population in the mammary immune infiltrate of Caspase-1 or Asc KO mice. Thus, our data suggest that the inflammasome supports tumor growth by recruiting Ly6Cint-Ly6Ghigh cells to the tumor bed preventing NK cell infiltration and activation.
Activation and secretion of the two pro-inflammatory cytokines IL-1β and IL-18 are mostly regulated through the inflammasome. According to previous studies, IL-1β promotes 4T1 tumor growth (26, 27). However, we did no detect any difference in intra-tumoral IL-1β concentration and the inhibition of the IL-1R pathway by IL-1Ra administration or through the immune-depletion of IL-1β in vivo did not impair 4T1 growth. Moreover, injecting the same anti-IL-1β used by Kaplanov et al. in WT mice did not affect 4T1 growth, although tumor volumes were globally much smaller in their study compared with ours (32). In addition, Bruchard et al. described no decrease in tumor growth in the presence of IL-1Ra (59). IL-18 inhibition, and the combination of both IL-1β and IL-18 inhibition also had no impact on tumor cell growth. The discrepancies observed these different studies could be explained by the BALB/c strain that we used or by specific in-house microbiota. Of note, using the aggressive PyMT mouse model of invasive breast carcinoma inter-crossed with the Il-1r KO background, Dagenais and colleagues observed an increase in tumor burden and aggressiveness, while no effect on the composition of the tumor immune microenvironment was noted, minimizing the role of the IL-1R pathway as a main modulator of breast cancer progression through the modulation of the immune composition (60). Thus, in our model, the inflammasome may support tumor growth through as yet understudied effector mechanisms. They could be pyroptosis, which induces pore formation in the plasma membrane and the release of the intracellular content, or caspase-1 mediated eicosanoid storm (12, 61). Eicosanoids, and especially prostaglandin E2 (PGE2) synthesized by cyclooxygenases, were known to suppress anti-tumor immunity by inhibiting NK cell viability and activation, and to promote cancer growth (51, 62, 63).
Altogether, our study highlights a new role for the inflammasome in promoting invasive breast cancer progression by facilitating tumor infiltration with neutrophils, while impeding the NK cell-associated anti-tumoral response independently of IL-1β and IL-18. These results suggest that inflammasome catalytic inhibition could be an interesting therapeutic approach for breast cancer.
Data Availability Statement
The raw data supporting the conclusions of this article will be made available by the authors, without undue reservation.
Ethics Statement
The animal study was reviewed and approved by the local Animal Ethic Evaluation Committee (CECCAPP: C2EA-15, Comité d'Evaluation Commun au PBES, à AniCan, au laboratoire P4, à l'animalerie de transit de l'ENS, à l'animalerie de l'IGFL, au PRECI, à l'animalerie du Cours Albert Thomas, au CARRTEL INRA Thonon-les-Bains et à l'animalerie de transit de l'IBCP, CLB-2013-019, CLB-2015-015) and authorized by the French Ministry of Education and Research.
Author Contributions
BG, TW, and VP designed experiments. BG, MB-W, AD, MP, IP, AE, and VP performed research. NG, NB-V, CC, and TW contributed to agents/analytic tools. BG, TW, and VP analyzed data. BG and VP wrote the paper. All authors contributed to the article and approved the submitted version.
Funding
This work was supported by the Ligue contre le Cancer comité de l'Ain and the Plan Cancer FP7-PEOPLE-ERG2-2008 n°239479. BG was supported by the Fondation ARC pour la recherche sur le cancer. TW is supported by the Fondation ARC (équipe labellisée).
Conflict of Interest
The authors declare that the research was conducted in the absence of any commercial or financial relationships that could be construed as a potential conflict of interest.
Acknowledgments
We thank V. M. Dixit (Genentech) for the Asc−/− mice; the late J. Tschopp (University of Lausanne) for the Nlrp3−/− mice; R. A. Flavell (Yale School of Medicine) for the Caspase-1/caspase-11−/− mice; Uzma Hasan for providing Anakinra; the Plateforme d'Expérimentation Animale en Cancérologie (AniCan) facility and Julie Gorry for technical help. We thank Brigitte Manship for English editing of the manuscript. The content of this manuscript has been partly published as part of the Ph.D., thesis of BG (64).
Supplementary Material
The Supplementary Material for this article can be found online at: https://www.frontiersin.org/articles/10.3389/fonc.2020.01683/full#supplementary-material
Supplementary Figure 1. Caspase-1 and ASC deficiency alters the composition of the tumor immune infiltrate in tumors but not that of the spleen. (A) Flow cytometry quantification of CD45 positive cells in 4T1 tumors isolated from WT (n = 6), Caspase-1 KO (n = 6), and Asc KO (n = 6) mice 14 days post-injection. (B) Correlation graph of NK cells vs. neutrophils infiltrated in 4T1 tumors isolated from WT (n = 6), Caspase-1 KO (n = 6), and Asc KO (n = 6) mice 14 days post-injection. (C,D) Flow cytometry quantification and analysis of myeloid (C) and lymphoid (D) cell populations from WT, Caspase-1 KO, and Asc KO mice 7 days post-injection with 4T1 mammary tumor cells. (E,F) Flow cytometry quantification and analysis of myeloid (E) and lymphoid (F) cell populations in spleens from WT (n = 4), Caspase-1 KO (n = 4), and Asc KO (n = 4) mice injected with 4T1 mammary tumor cells at day 14 post 4T1 tumor cell injection. Data represent mean ± SD *P < 0.05, **P < 0.01 (One-way ANOVA test followed by Bonferroni's Multiple Comparison Test).
Supplementary Figure 2. IL-1β inhibition does not affect 4T1 tumor growth in vivo. WT mice were injected with IgG control (n = 7) or anti-IL1β antibody (n = 8) the day before tumor inoculation and then twice a week. Treated mice were orthotopically injected with 4T1 mammary tumor cells. Tumor growth was measured over 28 days.
Supplementary Figure 3. Cytokine measurements in tumor cell supernatants of WT or caspase-1 KO mice by Luminex technology. Supernatants from tumor dilacerations of WT (N = 8) and caspase-1 KO (N = 8) mice were analyzed by Luminex assay for CCL5 IL-1β, CCL3, IL-33, KC, and FGF-b. Data represent mean ± SD (p from unpaired t-test).
Supplementary Figure 4. NK cells control tumor growth and are more activated in caspase-1 KO mice. (A) Cytometric profiles of data shown in Figure 6F. Cell suspensions from digested tumors of the indicated mouse genotype were cultured in the presence of cytokines (IL-12/IL-18), antibodies (NKp46, Ly49D, NKG2D), or tumor cells (YAC-1, 4T1) and NK cell IFN-γ production was measured by flow cytometry. (B) Comparison of IFN-γ-positive NK cells from tumor of WT and Caspase-1 KO mice exposed or not to 4T1 cells.
Supplementary Table 1. Inventory of fluorochrome conjugated-antibodies used for cytometry analysis.
References
1. DeNardo DG, Barreto JB, Andreu P, Vasquez L, Tawfik D, Kolhatkar N, et al. CD4(+) T cells regulate pulmonary metastasis of mammary carcinomas by enhancing protumor properties of macrophages. Cancer Cell. (2009) 16:91–102. doi: 10.1016/j.ccr.2009.06.018
2. Coussens LM, Zitvogel L, Paluckaa K. Neutralizing tumor-promoting chronic inflammation: a magic bullet? Science. (2013) 339:286–91. doi: 10.1126/science.1232227
3. Fridman WH, Pagès F, Sautès-Fridman C, Galon J. The immune contexture in human tumours: impact on clinical outcome. Nat Rev Cancer. (2012) 12:298–306. doi: 10.1038/nrc3245
4. Fridman W-H, Dieu-Nosjean M-C, Pagès F, Cremer I, Damotte D, Sautès-Fridman C, et al. The immune microenvironment of human tumors: general significance and clinical impact. Cancer Microenviron. (2013) 6:117–22. doi: 10.1007/s12307-012-0124-9
5. Kristensen VN, Vaske CJ, Ursini-Siegel J, Van Loo P, Nordgard SH, Sachidanandam R, et al. Integrated molecular profiles of invasive breast tumors and ductal carcinoma in situ (DCIS) reveal differential vascular and interleukin signaling. Proc Natl Acad Sci USA. (2012) 109:2802–7. doi: 10.1073/pnas.1108781108
6. Nagalla S, Chou JW, Willingham MC, Ruiz J, Vaughn JP, Dubey P, et al. Interactions between immunity, proliferation and molecular subtype in breast cancer prognosis. Genome Biol. (2013) 14:R34. doi: 10.1186/gb-2013-14-4-r34
7. Giraldo NA, Becht E, Remark R, Damotte D, Sautès-Fridman C, Fridman WH. The immune contexture of primary and metastatic human tumours. Curr Opin Immunol. (2014) 27:8–15. doi: 10.1016/j.coi.2014.01.001
9. Shalapour S, Karin M. Immunity, inflammation, and cancer: an eternal fight between good and evil. J Clin Invest. (2015) 125:3347–55. doi: 10.1172/JCI80007
10. Schroder K, Tschopp J. The inflammasomes. Cell. (2010) 140:821–32. doi: 10.1016/j.cell.2010.01.040
11. Rathinam VAK, Fitzgerald KA. Inflammasome complexes: emerging mechanisms and effector functions. Cell. (2016) 165:792–800. doi: 10.1016/j.cell.2016.03.046
12. Kovacs SB, Miao EA. Gasdermins: effectors of pyroptosis. Trends Cell Biol. (2017) 27:673–84. doi: 10.1016/j.tcb.2017.05.005
13. Allen IC, TeKippe EM, Woodford R-MT, Uronis JM, Holl EK, Rogers AB, et al. The NLRP3 inflammasome functions as a negative regulator of tumorigenesis during colitis-associated cancer. J Exp Med. (2010) 207:1045–56. doi: 10.1084/jem.20100050
14. Hu B, Elinav E, Huber S, Booth CJ, Strowig T, Jin C, et al. Inflammation-induced tumorigenesis in the colon is regulated by caspase-1 and NLRC4. Proc Natl Acad Sci USA. (2010) 107:21635–40. doi: 10.1073/pnas.1016814108
15. Petrilli V. The multifaceted roles of inflammasome proteins in cancer. Curr Opin Oncol. (2017) 29:35–40. doi: 10.1097/CCO.0000000000000346
16. Nakamura K, Kassem S, Cleynen A, Chrétien M-L, Guillerey C, Putz EM, et al. Dysregulated IL-18 is a key driver of immunosuppression and a possible therapeutic target in the multiple myeloma microenvironment. Cancer Cell. (2018) 33:634–48.e5. doi: 10.1016/j.ccell.2018.02.007
17. Chow MT, Sceneay J, Paget C, Wong CSF, Duret H, Tschopp J, et al. NLRP3 suppresses NK cell-mediated responses to carcinogen-induced tumors and metastases. Cancer Res. (2012) 72:5721–32. doi: 10.1158/0008-5472.CAN-12-0509
18. Chow MT, Tschopp J, Möller A, Smyth MJ. NLRP3 promotes inflammation-induced skin cancer but is dispensable for asbestos-induced mesothelioma. Immunol Cell Biol. (2012) 90:983–6. doi: 10.1038/icb.2012.46
19. Drexler SK, Bonsignore L, Masin M, Tardivel A, Jackstadt R, Hermeking H, et al. Tissue-specific opposing functions of the inflammasome adaptor ASC in the regulation of epithelial skin carcinogenesis. Proc Natl Acad Sci USA. (2012) 109:18384–9. doi: 10.1073/pnas.1209171109
20. Miller LJ, Kurtzman SH, Anderson K, Wang Y, Stankus M, Renna M, et al. Interleukin-1 family expression in human breast cancer: interleukin-1 receptor antagonist. Cancer Invest. (2000) 18:293–302. doi: 10.3109/07357900009012171
21. Pantschenko AG, Pushkar I, Anderson KH, Wang Y, Miller LJ, Kurtzman SH, et al. The interleukin-1 family of cytokines and receptors in human breast cancer: implications for tumor progression. Int J Oncol. (2003) 23:269–84. doi: 10.3892/ijo.23.2.269
22. Bunt SK, Yang L, Sinha P, Clements VK, Leips J, Ostrand-Rosenberg S. Reduced inflammation in the tumor microenvironment delays the accumulation of myeloid-derived suppressor cells and limits tumor progression. Cancer Res. (2007) 67:10019–26. doi: 10.1158/0008-5472.CAN-07-2354
23. Dirat B, Bochet L, Dabek M, Daviaud D, Dauvillier S, Majed B, et al. Cancer-associated adipocytes exhibit an activated phenotype and contribute to breast cancer invasion. Cancer Res. (2011) 71:2455–65. doi: 10.1158/0008-5472.CAN-10-3323
24. Guo B, Fu S, Zhang J, Liu B, Li Z. Targeting inflammasome/IL-1 pathways for cancer immunotherapy. Sci Rep. (2016) 6:36107. doi: 10.1038/srep36107
25. Calogero RA, Cordero F, Forni G, Cavallo F. Inflammation and breast cancer. Inflammatory component of mammary carcinogenesis in ErbB2 transgenic mice. Breast Cancer Res. (2007) 9:211. doi: 10.1186/bcr1745
26. Bunt SK, Sinha P, Clements VK, Leips J, Ostrand-Rosenberg S. Inflammation induces myeloid-derived suppressor cells that facilitate tumor progression. J Immunol. (2006) 176:284–90. doi: 10.4049/jimmunol.176.1.284
27. Elkabets M, Ribeiro VSG, Dinarello CA, Ostrand-Rosenberg S, Di Santo JP, Apte RN, et al. IL-1β regulates a novel myeloid-derived suppressor cell subset that impairs NK cell development and function. Eur J Immunol. (2010) 40:3347–57. doi: 10.1002/eji.201041037
28. Martinon F, Pétrilli V, Mayor A, Tardivel A, Tschopp J. Gout-associated uric acid crystals activate the NALP3 inflammasome. Nature. (2006) 440:237–41. doi: 10.1038/nature04516
29. Mariathasan S, Newton K, Monack DM, Vucic D, French DM, Lee WP, et al. Differential activation of the inflammasome by caspase-1 adaptors ASC and Ipaf. Nature. (2004) 430:213–8. doi: 10.1038/nature02664
30. Kuida K, Lippke JA, Ku G, Harding MW, Livingston DJ, Su MS, et al. Altered cytokine export and apoptosis in mice deficient in interleukin-1 beta converting enzyme. Science. (1995) 267:2000–3. doi: 10.1126/science.7535475
31. Boggio K, Nicoletti G, Di Carlo E, Cavallo F, Landuzzi L, Melani C, et al. Interleukin 12-mediated prevention of spontaneous mammary adenocarcinomas in two lines of Her-2/neu transgenic mice. J Exp Med. (1998) 188:589–96. doi: 10.1084/jem.188.3.589
32. Kaplanov I, Carmi Y, Kornetsky R, Shemesh A, Shurin GV, Shurin MR, et al. Blocking IL-1β reverses the immunosuppression in mouse breast cancer and synergizes with anti–PD-1 for tumor abrogation. Proc Natl Acad Sci USA. (2019) 116:1361–9. doi: 10.1073/pnas.1812266115
33. Shi Y, Evans JE, Rock KL. Molecular identification of a danger signal that alerts the immune system to dying cells. Nature. (2003) 425:516–21. doi: 10.1038/nature01991
34. Tesniere A, Panaretakis T, Kepp O, Apetoh L, Ghiringhelli F, Zitvogel L, et al. Molecular characteristics of immunogenic cancer cell death. Cell Death Differ. (2008) 15:3–12. doi: 10.1038/sj.cdd.4402269
35. Stienstra R, Joosten LAB, Koenen T, van Tits B, van Diepen JA, van den Berg SAA, et al. The inflammasome-mediated caspase-1 activation controls adipocyte differentiation and insulin sensitivity. Cell Metab. (2010) 12:593–605. doi: 10.1016/j.cmet.2010.11.011
36. Yazdi AS, Drexler SK, Tschopp J. The role of the inflammasome in nonmyeloid cells. J Clin Immunol. (2010) 30:623–7. doi: 10.1007/s10875-010-9437-y
37. Dupaul-Chicoine J, Arabzadeh A, Dagenais M, Douglas T, Champagne C, Morizot A, et al. The Nlrp3 inflammasome suppresses colorectal cancer metastatic growth in the liver by promoting natural killer cell tumoricidal activity. Immunity. (2015) 43:751–63. doi: 10.1016/j.immuni.2015.08.013
38. Terme M, Ullrich E, Aymeric L, Meinhardt K, Desbois M, Delahaye N, et al. IL-18 induces PD-1-dependent immunosuppression in cancer. Cancer Res. (2011) 71:5393–9. doi: 10.1158/0008-5472.CAN-11-0993
39. Roberti MP, Mordoh J, Levy EM. Biological role of NK cells and immunotherapeutic approaches in breast cancer. Front Immunol. (2012) 3:375. doi: 10.3389/fimmu.2012.00375
40. Chiossone L, Dumas PY, Vienne M, Vivier E. Natural killer cells and other innate lymphoid cells in cancer. Nat Rev Immunol. (2018) 18:671–88. doi: 10.1038/s41577-018-0061-z
41. Marçais A, Cherfils-Vicini J, Viant C, Degouve S, Viel S, Fenis A, et al. The metabolic checkpoint kinase mTOR is essential for IL-15 signaling during the development and activation of NK cells. Nat Immunol. (2014) 15:749–57. doi: 10.1038/ni.2936
42. Cichocki F, Miller JS, Anderson SK, Bryceson YT. Epigenetic regulation of NK cell differentiation and effector functions. Front Immunol. (2013) 4:55. doi: 10.3389/fimmu.2013.00055
43. Mangan MSJ, Olhava EJ, Roush WR, Seidel HM, Glick GD, Latz E. Targeting the NLRP3 inflammasome in inflammatory diseases. Nat Rev Drug Discov. (2018) 17:588–606. doi: 10.1038/nrd.2018.97
44. Jakobs C, Perner S, Hornung V. AIM2 drives joint inflammation in a self-DNA triggered model of chronic polyarthritis. PLoS ONE. (2015) 10:e0131702. doi: 10.1371/journal.pone.0131702
45. Schattgen SA, Gao G, Kurt-Jones EA, Fitzgerald KA. Cutting edge: DNA in the lung microenvironment during influenza virus infection tempers inflammation by engaging the DNA sensor AIM2. J Immunol. (2016) 196:29–33. doi: 10.4049/jimmunol.1501048
46. Kolb R, Phan L, Borcherding N, Liu Y, Yuan F, Janowski AM, et al. Obesity-associated NLRC4 inflammasome activation drives breast cancer progression. Nat Commun. (2016) 7:13007. doi: 10.1038/ncomms13007
47. Coca S, Perez-Piqueras J, Martinez D, Colmenarejo A, Saez MA, Vallejo C, et al. The prognostic significance of intratumoral natural killer cells in patients with colorectal carcinoma. Cancer. (1997) 79:2320–8. doi: 10.1002/(SICI)1097-0142(19970615)79:12<2320::AID-CNCR5>3.0.CO;2-P
48. Villegas FR, Coca S, Villarrubia VG, Jiménez R, Chillón MJ, Jareño J, et al. Prognostic significance of tumor infiltrating natural killer cells subset CD57 in patients with squamous cell lung cancer. Lung Cancer. (2002) 35:23–8. doi: 10.1016/S0169-5002(01)00292-6
49. Mamessier E, Sylvain A, Thibult ML, Houvenaeghel G, Jacquemier J, Castellano R, et al. Human breast cancer cells enhance self tolerance by promoting evasion from NK cell antitumor immunity. J Clin Invest. (2011) 121:3609–22. doi: 10.1172/JCI45816
50. Verronèse E, Delgado A, Valladeau-Guilemond J, Garin G, Guillemaut S, Tredan O, et al. Immune cell dysfunctions in breast cancer patients detected through whole blood multi-parametric flow cytometry assay. Oncoimmunology. (2016) 5:e1100791. doi: 10.1080/2162402X.2015.1100791
51. Böttcher JP, Bonavita E, Chakravarty P, Blees H, Cabeza-Cabrerizo M, Sammicheli S, et al. NK cells stimulate recruitment of cDC1 into the tumor microenvironment promoting cancer immune control. Cell. (2018) 172:1022–37.e14. doi: 10.1016/j.cell.2018.01.004
52. Bezman NA, Kim CC, Sun JC, Min-Oo G, Hendricks DW, Kamimura Y, et al. Molecular definition of the identity and activation of natural killer cells. Nat Immunol. (2012) 13:1000–9. doi: 10.1038/ni.2395
53. Mamessier E, Sylvain A, Bertucci F, Castellano R, Finetti P, Houvenaeghel G, et al. Human breast tumor cells induce self-tolerance mechanisms to avoid NKG2D-mediated and DNAM-mediated NK cell recognition. Cancer Res. (2011) 71:6621–32. doi: 10.1158/0008-5472.CAN-11-0792
54. Cremer I, Fridman WH, Sautès-Fridman C. Tumor microenvironment in NSCLC suppresses NK cells function. Oncoimmunology. (2012) 1:244–6. doi: 10.4161/onci.1.2.18309
55. Gabrilovich DI, Ostrand-Rosenberg S, Bronte V. Coordinated regulation of myeloid cells by tumours. Nat Rev Immunol. (2012) 12:253–68. doi: 10.1038/nri3175
56. Keskinov AA, Shurin MR. Myeloid regulatory cells in tumor spreading and metastasis. Immunobiology. (2015) 220:236–42. doi: 10.1016/j.imbio.2014.07.017
57. Li H, Han Y, Guo Q, Zhang M, Cao X. Cancer-expanded myeloid-derived suppressor cells induce anergy of NK cells through membrane-bound TGF-beta 1. J Immunol. (2009) 182:240–9. doi: 10.4049/jimmunol.182.1.240
58. Sinha P, Clements VK, Fulton AM, Ostrand-Rosenberg S. Prostaglandin E2 promotes tumor progression by inducing myeloid-derived suppressor cells. Cancer Res. (2007) 67:4507–13. doi: 10.1158/0008-5472.CAN-06-4174
59. Bruchard M, Mignot G, Derangère V, Chalmin F, Chevriaux A, Végran F, et al. Chemotherapy-triggered cathepsin B release in myeloid-derived suppressor cells activates the Nlrp3 inflammasome and promotes tumor growth. Nat Med. (2012) 19:57–64. doi: 10.1038/nm.2999
60. Dagenais M, Dupaul-Chicoine J, Douglas T, Champagne C, Morizot A, Saleh M. The Interleukin (IL)-1R1 pathway is a critical negative regulator of PyMT-mediated mammary tumorigenesis and pulmonary metastasis. Oncoimmunology. (2017) 6:e1287247. doi: 10.1080/2162402X.2017.1287247
61. von Moltke J, Trinidad NJ, Moayeri M, Kintzer AF, Wang SB, van Rooijen N, et al. Rapid induction of inflammatory lipid mediators by the inflammasome in vivo. Nature. (2012) 490:107–11. doi: 10.1038/nature11351
62. Howe LR, Chang SH, Tolle KC, Dillon R, Young LJT, Cardiff RD, et al. HER2/neu-induced mammary tumorigenesis and angiogenesis are reduced in cyclooxygenase-2 knockout mice. Cancer Res. (2005) 65:10113–10119. doi: 10.1158/0008-5472.CAN-05-1524
63. Zelenay S, van der Veen AG, Böttcher JP, Snelgrove KJ, Rogers N, Acton SE, et al. Cyclooxygenase-dependent tumor growth through evasion of immunity. Cell. 162:1257–70. (2015). doi: 10.1016/j.cell.2015.08.015
Keywords: inflammasome, breast cancer, NK cells, inflammation, Caspase-1, ASC
Citation: Guey B, Bodnar-Wachtel M, Drouillard A, Eberhardt A, Pratviel M, Goutagny N, Bendriss-Vermare N, Puisieux I, Caux C, Walzer T and Petrilli V (2020) Inflammasome Deletion Promotes Anti-tumor NK Cell Function in an IL-1/IL-18 Independent Way in Murine Invasive Breast Cancer. Front. Oncol. 10:1683. doi: 10.3389/fonc.2020.01683
Received: 22 May 2020; Accepted: 29 July 2020;
Published: 16 September 2020.
Edited by:
Francois Ghiringhelli, Institut National de la Santé et de la Recherche Médicale (INSERM), FranceReviewed by:
Limagne Emeric, Centre Georges François Leclerc, FranceFrederique Vegran, INSERM U1231 Lipides, Nutrition, Cancer (LNC), France
Antonino Bruno, MultiMedica (IRCCS), Italy
Copyright © 2020 Guey, Bodnar-Wachtel, Drouillard, Eberhardt, Pratviel, Goutagny, Bendriss-Vermare, Puisieux, Caux, Walzer and Petrilli. This is an open-access article distributed under the terms of the Creative Commons Attribution License (CC BY). The use, distribution or reproduction in other forums is permitted, provided the original author(s) and the copyright owner(s) are credited and that the original publication in this journal is cited, in accordance with accepted academic practice. No use, distribution or reproduction is permitted which does not comply with these terms.
*Correspondence: Virginie Petrilli, virginie.petrilli@lyon.unicancer.fr
†Present address: Baptiste Guey, Global Health Institute, École Polytechnique Fédérale de Lausanne, Lausanne, Switzerland
Isabelle Puisieux, Institut Curie, Research Center U932 26, rue d'ULM 75248, Paris, France