- 1Clinical Laboratory, Nanshan Maternity and Child Healthcare Hospital, Shenzhen, China
- 2Department of Science and Education, Nanshan Maternity and Child Healthcare Hospital, Shenzhen, China
- 3Department of Biochemistry and Molecular Biology, School of Basic Medical Sciences, Southern Medical University, Guangzhou, China
Increasing evidence indicates that the Aquaporin1 (AQP1) aberrant expression may be related to a wide variety of human cancers, including breast cancer (BC). In the present study, we explore the effects and possible mechanism of miR-3194-3p on the biological behaviors of BC. At first, miR-3194-3p is found to modulate AQP1 expression targeting the 3′-UTR using miRNA target prediction algorithms. MiR-3194-3p expression is markedly downregulated, and AQP1 expression is upregulated in BC tissues compared with adjacent normal breast tissues. Moreover, the differential expression of miR-3194-3p and AQP1 are observed in four BC cells with different malignancy degree. Meanwhile, a significant negative correlation between AQP1 and miR-3194-3p expressions in tumor tissues from 30 BC patients is revealed. miR-3194-3p mimic remarkably inhibits cell proliferation, migration, and invasion as well as promotes apoptosis in MDA-MB-231 cells while miR-3194-3p inhibitors exert an opposite role in MCF-7 cells. Dual-luciferase reporter system demonstrates that AQP1 is a direct target gene of miR-3194-3p. Overexpression of AQP1 by pBABE-puro-AQP1 vector partially abrogates the effect of miR-3194-3p mimic in MDA-MB-231 cells. In short, our results suggest that miR-3194-3p suppresses BC cell proliferation, migration, and invasion by targeting AQP1, providing a novel insight into BC tumorigenesis and treatment.
Introduction
The incidence and mortality of breast cancer (BC) have been high among female malignant tumors, which seriously threatens the health of women worldwide (1, 2). Despite advances in early screening and therapeutic strategy for BC in recent years, the 5-year survival rate of patients with BC is still not ideal due to high metastases and recurrences (3). Therefore, a deep understanding of the molecular mechanisms of BC is essential to develop new treatments and improve prognosis for BC.
Aquaporin1 (AQP1) is a kind of membrane channel protein widely existing in endothelial and epithelial cells of human tissues, which can efficiently and specifically transport water molecules (4). A growing number of studies have found that AQP1’s function is not limited to transporting water molecules; it is closely related to a wide variety of tumors, including tumors of brain, prostate, breast, ovary, colon, and lung (5). It has been reported that AQP1 is involved in cell migration (6), angiogenesis (7), and tumor growth (8). Especially in BC, AQP1 overexpression is significantly associated with poor prognosis in aggressive breast cancer (9). Furthermore, Esteva-Font et al. found that AQP1 deficiency reduces vessel density and lowers lung metastases in mice models (10). However, the possible regulation mechanisms involved in AQP1 overexpression in BC are still unclear.
MicroRNA (miRNA) is a class of endogenous non-coding RNAs with a length of 19–25 nucleotides that negatively regulate target gene expression at the post-transcriptional level (RNA cleavage or translation repression) mainly by binding to the complementary region on the 3′-untranslated regions (3′-UTR) of target gene mRNA (11, 12). miRNAs plays an important role in cell proliferation, differentiation, and apoptosis, and its abnormal expression may be closely related to tumorigenesis (13). Emerging research continues to focus on miRNAs as potential markers for BC targeted therapy and patient prognosis prediction (14). Recent studies reveal that miRNAs may act as oncogenes or suppressor genes in BC by participating in different cellular pathways (15, 16), including via targeting AQP1. Lately, miR-3194-3p was found significantly downregulated in hepatocellular carcinoma (HCC) tissues and cell lines, which was associated with metastasis and recurrence of HCC through targeting BCL9 (17). Nevertheless, the function of miR-3194-3p and its relationship with AQP1 in BC remain unknown.
To obtain a comprehensive understanding of the role and possible mechanism of miR-3194-3p in BC progression, we explored the effects of miR-3194-3p upregulation and downregulation on cellular biological process of BC and identified AQP1 as a direct target of miR-3194-3p. Moreover, the alteration of miR-3194-3p expression and its effect on BC cell growth were observed by promoting AQP1 expression. The expression pattern and biological role of miR-3194-3p and its target gene AQP1 in BC have not yet been elucidated, and our findings suggest that miR-3194-3p is a novel and promising molecular target for BC treatment.
Materials and Methods
Clinical Samples
BC tumor tissue and paired adjacent tissue were obtained from 30 patients with BC at the Nanfang Hospital of Southern Medical University (Guangzhou, China). Tissue specimens were collected from female patients with primary BC who underwent surgical resection between September 2014 and December 2016. The BC tissues removed surgically were placed in RNAlater (Ambion, Austin, TX, United States) immediately at 4°C for at least 24 h and stored at −80°C until used. This study was conducted in accordance with the Helsinki Declaration and was approved by the Ethics Committee of Nanfang Hospital of Southern Medical University.
Cell Culture and Transfection
Four BC cell lines (MDA-MB-231, MCF-7, ZR-75-1, SKBR3) and a normal human mammary epithelial cell line (MCF-10A) were obtained from the Chinese Academy of Sciences (Shanghai, China). All cell lines were cultured in DMEM medium (Thermo Fisher Scientific, United States) supplemented with 10% fetal bovine serum (Gibco, United States) and 1% penicillin, and incubated at 37°C and 5% CO2. The oligonucleotides of miR-3194-3p mimic (miR-3194-3p) and its negative control (mi-NC), miR-3194-3p inhibitor (anti-3194-3p) and its negative control (anti-NC) were purchased from GenePharma (Shanghai, China). A pBABE-puro-AQP1 expression vector was constructed and stored in the department of biochemistry and molecular Biology of Southern Medical University (18). For transfection, cells were seeded onto plates and incubated for 15 h and then transfected using Lipofectamine 2000 reagent (Invitrogen, Carlsbad, CA, United States) following the manufacturer’s instructions.
RNA Isolation, RNA-Reverse Transcription, and qRT-PCR Analysis
Expression levels of miR-3194-3p and AQP1 mRNA in BC tissues and BC cells were detected by qRT-PCR analysis following the protocols of previous studies (15). Total RNA was isolated using TRIzol reagent (LifeTechnologies, Carlsbad, CA, United States). To quantify miR-3194-3p expression, reverse transcription was performed with the BestarTM qPCR RT Kit (DBI Bioscience, Inc., Germany). miRNA quantification was measured using the SYBR Green qPCR MasterMix (DBI, Bioscience Inc., Germany) on the ABI 7500 qPCR system (Applied Biosystems, Carlsbad, CA, United States) with U6 small nuclear RNA as an internal control. To quantify AQP1 mRNA expresssion, M-MLV reverse transcriptase (Clontech, Palo Alto, CA, United States) and SYBR Premix Ex Taq GC kit (DBI, Bioscience Inc., Germany) were applied. All primers were designed and synthesized by Invitrogen (Carlsbad, CA, United States). The relative expression of AQP1 was normalized to glyceraldehyde 3-phosphate dehydrogenase (GAPDH). The relative expression levels of miR-3194-3p and AQP1 mRNA were calculated using the 2–ΔΔCt approach.
Western Blot Analysis
Total protein from BC cells (1 × 106) was extracted with radio immunoprecipitation assay (RIPA) lysis buffer (Beyotime, China). Protein concentration was determined using the Bradford protein assay (Bio-Rad, United States). Take 40 μg/well of protein for 12.5% sodium dodecyl sulfate-polyacrylamide gel electrophoresis (SDS-PAGE), transfer the separated protein to polyvinylidenedifluoride (PVDF) membrane, and block the reaction with blocking solution containing 5% skimmed milk powder at room temperature for 2 h. Anti-AQP1 (1:2000; AF5231; Affinity) and anti-GAPDH (1:1000; ab8245; Abcam) antibodies were added and incubated at 4°C overnight. After washing the PVDF membrane three times with TBST, goat anti-rabbit IgG secondary antibody labeled with horseradish peroxidase (HRP) (1:20,000; BA1054; Boster) was added for 1 h. Protein bands were detected using an enhanced chemiluminescence (ECL) detection system (Amersham, United Kingdom). Image-Pro Plus 6.0 (Media Cybernetics, United States) was used to quantify protein bands, and GAPDH was used as a control.
Cell Counting Kit-8 Assay (CCK-8)
Cell proliferation was examined using a CCK-8 kit (Dojindo, Japan) according to the manufacturer’s protocol. Cells from each group were harvested at 24 h post-transfection and inoculated into 96-well plates (5 × 103 cells/well). The plates were placed in a cell culture incubator; CCK-8 (10 μL/well) was added after 0, 24, 48, and 72 h, respectively, and then cultured for another 2 h. The absorbance (A) value of each well at 450 nm was measured by microplate spectrophotometer (Bio-Rad, Hercules, CA, United States). Cell growth curves were plotted with time (T) as the horizontal axis and light absorption value (A) as the vertical coordinate.
5-Ethynyl-2′-Deoxyuridine (EdU) Assay
Cell proliferation ability was also measured by an EdU assay kit (Ribobio, China) as described previously (19). In 96-well plates, the post-transfected cells, in turn, were incubated with EdU (50 μM) for 2 h, 0.5% Triton X for 20 min, ApolloR reaction cocktail (100 μL) for 30 min, and Hoechst 33342 (100 μL) for 30 min. The cell images were taken using a fluorescence microscope (Olympus Corporation of Japan) at 100× magnification.
Cell Migration and Invasion Assays
The wound-healing assay was conducted to evaluate cell migration ability: 3 × 105 cells per well were seeded in triplicate into six-well plates and cultured for 24 h. The cell surface was scratched with a pipette tip. The suspended cells were washed off with PBS and cultured with fresh DMEM medium without serum for an additional 24 h. The area of the cell crawling from the scratch toward the center is observed under a microscope, and the size of the area indicates the cell’s ability to migrate (20). The migration distance was analyzed by Image-Pro Plus 6.0 software.
Cell invasion assays were performed using Transwell chambers (8-μm pore size; Corning, United States) coated with Matrigel matrix (BD Biosciences, United States). A detailed experiment was performed similar to that previously reported (21). The invaded cells were counted with a fluorescence inversion microscope (Olympus, Japan) at 200× magnification. Six fields per well were randomly selected under the microscope, and the average number of cells in each field was calculated.
Flow Cytometry Analysis of Cell Apoptosis
Cell apoptosis was evaluated using the Annexin V-FITC/PI Apoptosis Detection Kit (Kaiji, China) and flow cytometry (Becton Dickinson, United States). After 24 h transfection, cells were collected by digestion with 0.25% trypsin (without EDTA), rinsed, and resuspended in 500 μL binding buffer. Then, 5 μL Annexin V-FITC and 5 μL propidium iodide (PI) were added to the stain. After 10 min light-avoidance reaction, the apoptosis rate of the cells was interpreted by FlowJo software, version 10.0 (Flow Jo, United States).
Bioinformatic Analysis and Luciferase Reporter Assay
The online miRNA databases MiRanda1, miRBase2, and TargetScan3 were used to search for miRNAs that regulate AQP1 expression. The wild-type (WT) and mutant-type (MUT) AQP1 3′-UTR oligonucleotides miR-3194-3p were cloned into the psiCHECKTM-2 vector (Promega, United States). These constructed vectors were cotransfected with miR-3194-3p mimic or mi-NC into HEK293T cells using Lipofectamine 2000. Then, 24 h after transfection, the luciferase activity was detected using a dual-luciferase reporter assay system (Promega, United States).
Statistical Analysis
All experiments were executed at least three independent times. The statistical data are presented as mean ± standard deviation and analyzed using GraphPad Prism 6.0 (CA, United States). Two-tailed Student’s t-tests were conducted to interpret the differences. The correlation between miR-3194-3p and AQP1 mRNA expressions in BC tissues was assessed with Spearman’s correlation test. P value <0.05 was considered statistically significant.
Results
MiR-3194-3p and AQP1 Expression in BC Tissues and Cell Lines
To investigate the potential role of miR-3194-3p in BC development, the expression levels of miR-3194-3p in BC tissue samples (n = 30) and cell lines (MDA-MB-231, MCF-7, ZR-75-1, SKBR3) were measured by qRT-PCR. The results show that the expression of miR-3194-3p is markedly downregulated in BC tissues as compared with that of adjacent non-tumor tissues (Figure 1A). Moreover, we found that miR-3194-3p expression is also significantly lowered in high invasive BC cell lines (MDA-MB-231 and SK-BR-3) compared with a normal human mammary epithelial cell (MCF-10A) (Figure 1B). Conversely, the mRNA and protein of AQP1 in BC tissues and cell lines are significantly higher than those in the adjacent non-tumor tissues (Figure 1C) and MCF-10A cells (Figures 1D,E). It is worth noting that miR-3194-3p expression was lowest and AQP1 mRNA and protein expression was highest in MDA-MB-231 cells. Spearman’s correlation analysis found a significant negative correlation between the expression levels of miR-3194-3p and AQP1 mRNA in BC tissues (Figure 1F). These results suggest that miR-3194-3p and AQP1 expressions are closely involved in BC progression.
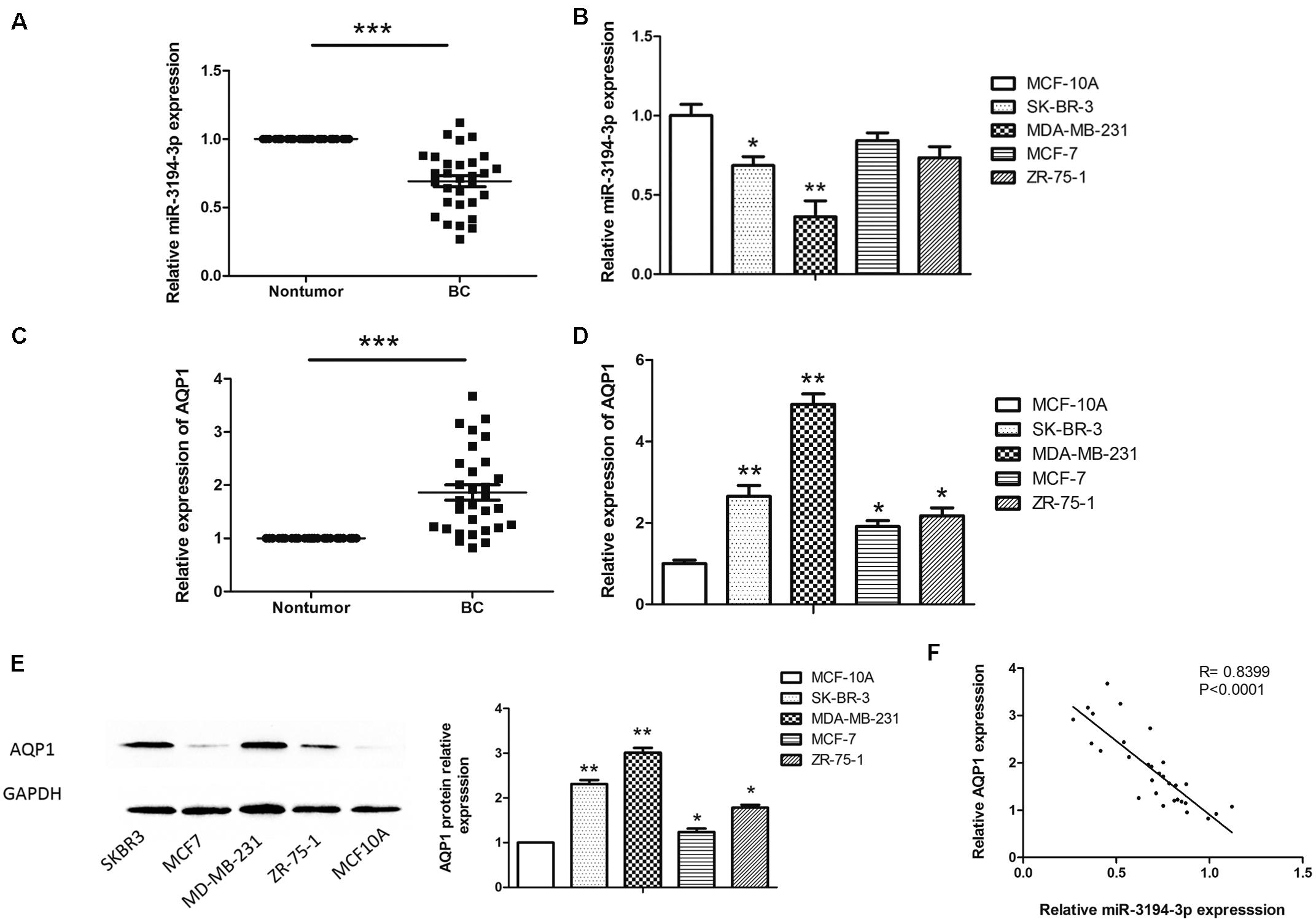
Figure 1. The miR-3194-3p and AQP1 expression levels in BC tissues and cell lines. Comparing differences in the expression levels of miR-3194-3p between (A) BC and adjacent non-tumor tissues (n = 30); (B) BC cell lines and the control breast epithelial cells (MCF-10A); differences in AQP1 mRNA level between (C) BC and adjacent non-tumor tissue; (D) BC cell lines and MCF-10A; differences in AQP1 protein level between (E) BC cell lines and MCF-10A. (F) A significant inverse correlation between miR-3194-3p and AQP1 expression was observed in BC tissues. All PCR reactions were performed independently in triplicate (*P < 0.05, **P < 0.01, and ***P < 0.001).
MiR-3194-3 Overexpression Inhibits Proliferation, Migration, and Invasion and Promotes Apoptosis in BC Cells
To better understand the role of miR-3194-3p on the biological behaviors of BC cells, we subsequently transfected MDA-MB-231 cells that had the lowest miR-3194-3p expression with mimics and MCF-7 cells that had the highest miR-3194-3p expression with inhibitors and their corresponding miRNA controls. qRT-PCR assay revealed miR-3194-3p expression was effectively upregulated in MDA-MB-231 cells and downregulated in MCF-7 cells at 48 h post-transfection (Figure 2A).
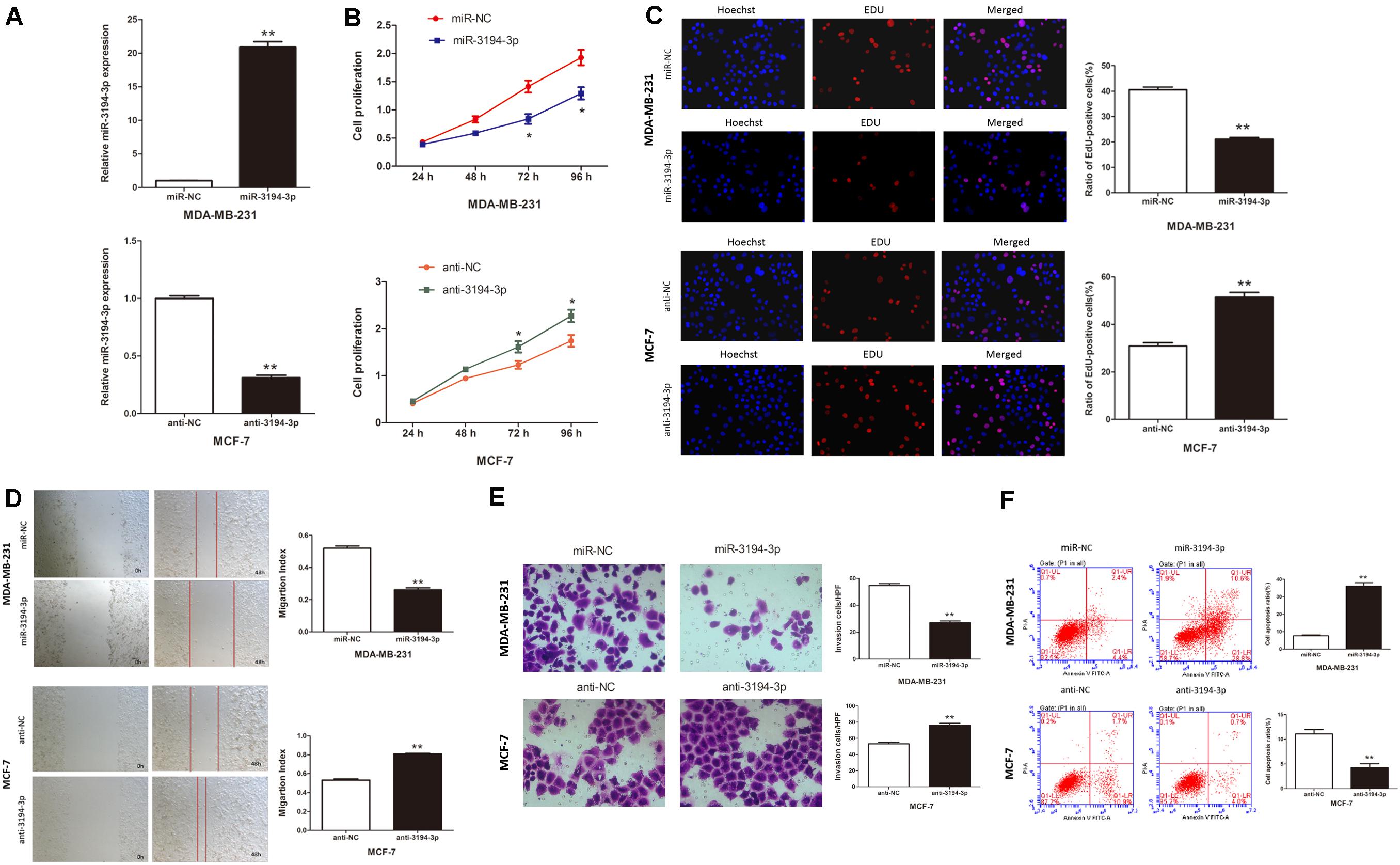
Figure 2. miR-3194-3p expression regulates cell proliferation, migration, invasion, and apoptosis in BC. (A) miR-3194-3p expression was measured by qRT-PCR in MDA-MB-231 and MCF-7 cells transfected with miR-3194-3p mimic/inhibitor and their negative controls. Cell proliferation was assessed with (B) CCK-8 assay and (C) Edu assay. (D) Cell migration was measured by wound-healing assay. (E) Cell invasion was measured by transwell assay. (F) Cell apoptosis was measured by Flow cytometry analysis (*P < 0.05 and **P < 0.01).
The effect of miR-3194-3p on BC cell proliferation was assessed by CCK-8 and EdU assay. The CCK-8 assay indicated that miR-3194-3p overexpression significantly reduced MDA-MB-231 cell viability (Figure 2B). The EdU assay displayed remarkably fewer EdU-positive cells in cells overexpressing miR-3194-3p than in control cells (Figure 2C). The wound-healing assay suggests that the migratory capacity of MDA-MB-231 cells transfected with miR-3194-3p mimics was much weaker than that of the miR-NC group cells (Figure 2D). Similarly, the transwell assay reveals that miR-3194-3p upregulation significantly undermined the invasion potential of MDA-MB-231 cells (Figure 2D). Flow cytometry analysis reveals that miR-3194-3p upregulation promotes apoptosis in MDA-MB-231 cells (Figure 2E). Conversely, miR-3194-3p downregulation promotes cell proliferation, migration, and invasion and inhibits apoptosis in MCF-7 cells transfected with miR-3194-3p inhibitors (Figures 2B–F). Taken together, these data indicate that miR-3194-3p overexpression inhibits BC cell proliferation, migration, and invasion and promotes cell apoptosis.
MiR-3194-3p Directly Targets and Inhibits AQP1 Expression
Our previous study shows that AQP1 has different roles in human cancers, including chronic myeloid leukemia (CML) (18), hepatocellular carcinoma (22), and cervical cancer (23). Another study finds AQP1 is overexpressed in BC tissues and participates in the pathogenesis of BC (24). To further clarify the regulatory mechanism of high AQP1 expression in BC tumorigenesis, we used three bioinformatic algorithms (MiRanda, miRBase, and TargetScan) to search for potential miRNAs that regulate AQP1 expression. As shown in Figure 3A, miR-3194-3p can directly bind with the AQP1 3′-UTR. The MiR-3194-3p target region within AQP1 3′-UTR ranges from 30,924,372 to 30,924,393 bp in the genomic sequence of AQP1 (NC_000007.14, location: 30,911,694–30,925,517).
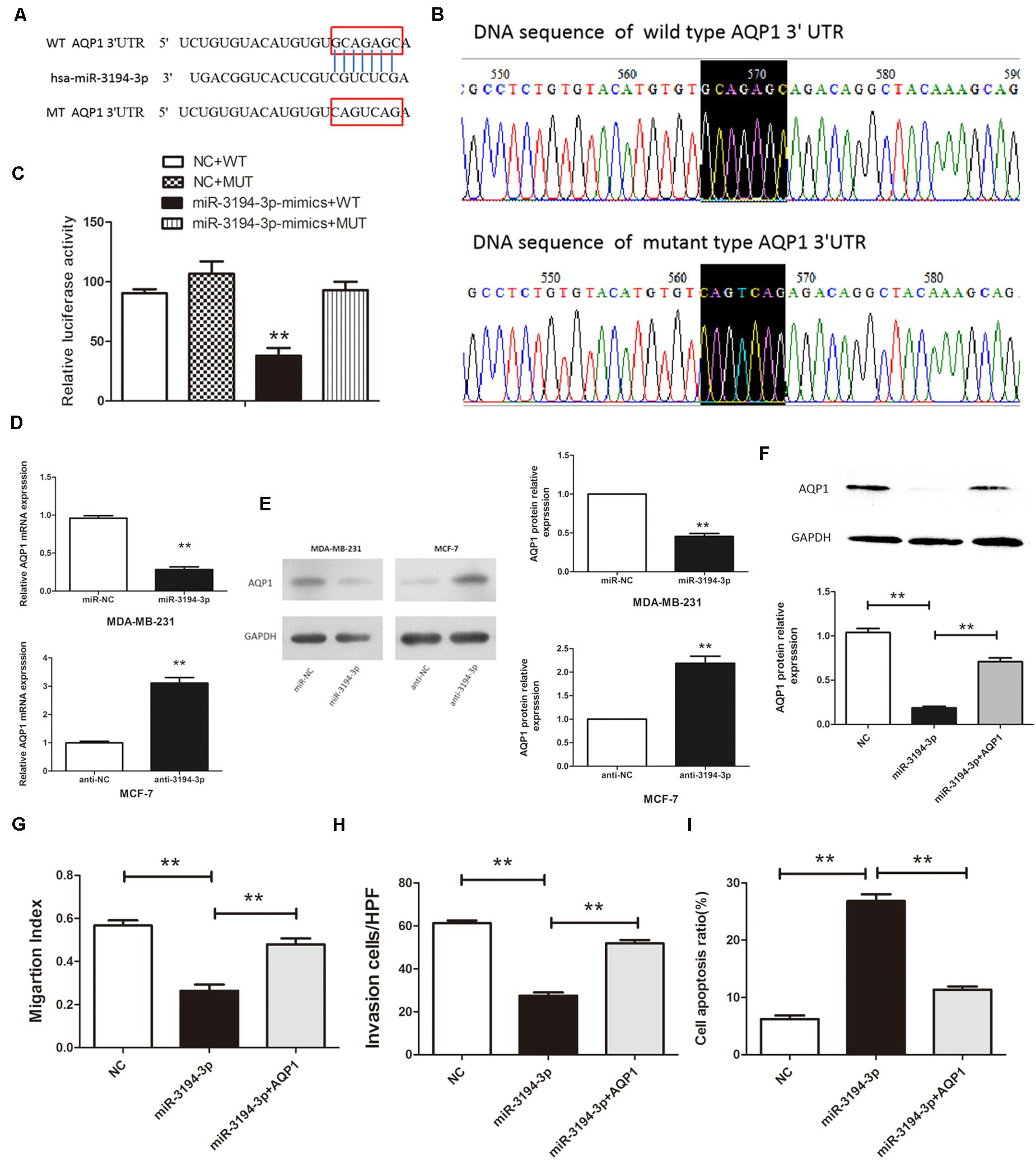
Figure 3. miR-3194-3p negatively regulates the expresssion of AQP1 by binding to the 3′-UTR of its mRNA. (A) The predicted miR-3194-3p-binding site in the AQP1 3′-UTR. (B) Mutations were generated and sequenced at the putative miR-3194-3p-binding site located in the AQP1 3′-UTR. (C) Either the WT or MUT reporter plasmid was cotransfected with either miR-3194-3p or NC oligo into HEK293T cells, and the luciferase activity was determined. (D) qRT-PCR analysis and (E) Western blot analysis of AQP1 expression in MDA-MB-231 and MCF-7 cells transfected with miR-3194-3p mimic/inhibitor and their negative controls. (F) Western blot analysis of AQP1 expression in MDA-MB-231 cells cotransfected with pBABE-puro-AQP1 and miR-3194-3p mimic. (G) Cell migration, (H) cell invasion, and (I) cell apoptosis was measured in MDA-MB-231 cells cotransfected with pBABE-puro-AQP1 and miR-3194-3p mimic (**P < 0.01).
To confirm that AQP1 is a direct target of miR-3194-3p, WT and MUT AQP1 3′-UTR transcription reporter vectors were constructed and transfected into HEK293T cells (Figure 3B). As pointed out in Figure 3C, high expression of miR-3194-3p significantly inhibits the luciferase activity of WT-AQP1-3′-UTR, whereas the luciferase activity of MUT-AQP1-3′-UTR is unaffected. Meanwhile, qRT-PCR and Western blot analyses prove that both mRNA and protein levels of AQP1 are significantly lowered in miR-3194-3p overexpressing MDA-MB-231 cells and higher in miR-3194-3p knockdown MCF-7 cells (Figures 3D,E). Altogether, AQP1 is the direct target gene of miR-3194-3p, and miR-3194-3p affects the progression of BC cells by downregulating AQP1 expression.
AQP1 Overexpression Partly Abrogates the Effect of MiR-3194-3p Mimic
To further elucidate that miR-3194-3p affects BC cell migration and invasion capacity is mediated by AQP1, we restored AQP1 in miR-3194-3p overexpressing MDA-MB-231 cells. Cells were transfected with pBABE-puro-AQP1 retroviral vector and miR-3194-3p mimic at the same time. As shown in Figure 3F, the suppression effect of miR-3194-3p mimic on AQP1 gene expression was reversed by pBABE-puro-AQP1. As expected, the effect of the miR-3194-3p mimic on cell migration, invasion, and apoptosis was significantly reversed by AQP1 overexpression (Figures 3G–I). Our findings support that miR-3194-3p regulates BC cell migration, invasion, and apoptosis through the AQP1 signaling pathway.
Discussion
Accumulating research has found that AQP1 can affect multiple important biological processes, including angiogenesis, wound healing, tumor metastasis, and invasion, etc. (25). AQP1-mediated rapid transmembrane transport of water flux is one of the important mechanisms to promote tumor cell migration (26). Concrete evidence shows that, after subcutaneous or intracranial tumor cell implantation, tumor growth is significantly inhibited in AQP1-deficient mice with reduced tumor vasculature and extensive necrosis (27). By establishing a mouse melanoma model, specific AQP1 siRNAs can effectively reduce the density of tumor microvessels, showing less obvious local tumor invasion and tumor metastasis in in vivo experiments (28). Otterbach et al. reports that high expression of AQP1 in BC is significantly associated with basal-like phenotype and poor survival (9). And in a long-term follow-up, AQP1 expression in 203 patients with aggressive BC shows a significant correlation with high tumor grade, medullary-like histology, and “triple-negativity” (29). Although evidence suggests that AQP1 may be a potentially promising target for inhibiting metastasis and aggressiveness in BC, the regulatory mechanisms of its aberrant expression have not been fully elucidated (7).
So far, approximately 50% of annotated miRNAs in humans are located in cancer-associated fragile loci and other genomic regions, and those miRNAs may act as both oncogenes or anti-oncogenes. Furthermore, it is estimated that more than 5,000 human genes, 30% of which have been validated, can serve as target genes of those miRNAs (30) and are mainly involved in the cellular signaling pathways regulating diverse biological processes, including metabolism (31), apoptosis (32), and the cell cycle (33).
To date, some miRNAs targeting AQP1 have been verified in a variety of tumors. For instance, miR320 is downregulated in plasma and tumor tissues of BC patients, and its target gene AQP1 is highly expressed, both of which are associated with poor prognosis (24). Overexpression of miR-495 may activate the p38 MAPK signaling pathway, inhibit AQP1, and promote the proliferation and differentiation of osteoblasts in tibial fracture mice (34). In thyroid cancer, it is reported that miR-223 inhibits cell proliferation and induces apoptosis by regulating AQP1 expression (35).
Here, we find miR-3194-3p regulates cell proliferation, migration, and apoptosis in BC by targeting AQP1. First, we predicted that miR-3194-3p may have a specific regulation on AQP1 through bioinformatics methods, which next was verified by qRT-PCR and luciferase reporter assay. We also searched gene databases, such as NCBI and PolymiRTS, and found no gene polymorphism in the region of miR-3194-3p-AQP1 interaction. The presence/absence of these polymorphisms in the miRNA seed region or target gene region may influence the loss/creation of miRNA binding sites, thus affecting the regulatory role of miRNA. The genetic variants of AQP1 3′-UTR and their effects on miR-3194-3p regulation remain to be further discovered.
In our study, miR-3194-3p expressions are remarkably lower in BC tissues, and the expression of AQP1 mRNA and protein are significantly higher in BC tissues compared with adjacent normal tissues. There is a significant negative correlation between miR-3194-3p and AQP1 expression in 30 cases of breast cancer and adjacent tissues (r = −0.84, P < 0.01). As is well known, BC with different molecular subtypes has significant differences in treatment effects and prognosis. Therefore, we compared the expression of miR-3194-3p and AQP1 in multiple BC cell lines. The results show that miR-3194-3p expression is lowest and AQP1 expression is highest in triple-negative MDA-MB-231 cells. All these findings suggest that miR-3194-3p and AQP1 are involved in carcinogenesis and may be associated with the tumor malignancy of BC. The relationship between miR-3194-3p and clinicopathological characteristics and prognosis of BC remains to be further investigated.
To further investigate whether miR-3194-3p suppresses BC progression via regulating AQP1, MDA-MB-231 and MCF-7 cells were transfected with miR-3194-3p mimic or inhibitors, and the ability of BC cells to grow, migrate, and invade and apoptosis were measured. Gain- and loss-of-function experiments reveal that miR-3194-3p overexpression prominently inhibits the proliferation, migration, and invasion of BC cells, and miR-3194-3p knockdown increases these cellular biological behaviors in vitro. Moreover, the cell apoptotic rate was notably increased by miR-3194-3p mimic. Importantly, we subsequently applied the AQP1 overexpressed vector in MDA-MB-231 cells and found that AQP1 upregulation partly abrogated the effect of miR-3194-3p mimic on cell migratory ability. These results strongly suggest that miR-3194-3p serves as a novel tumor suppressor through regulating AQP1. However, to fully understand the regulatory mechanism of miR-3194-3p in the pathogenesis of BC, further research is needed.
Data Availability Statement
The raw data supporting the conclusions of this article will be made available by the authors, without undue reservation.
Ethics Statement
The studies involving human participants were reviewed and approved by the Ethics Committee of NanFang Hospital of Southern Medical University. The patients/participants provided their written informed consent to participate in this study.
Author Contributions
MW, HY, and CC took part in research design, conducted experiments, and drafted the manuscript. RG, XL, and HZ participated in the data analysis and helped to draft the manuscript. All authors read and approved the final manuscript.
Funding
This work was supported by grants from National Natural Science Foundation of China (grant nos. 81672754 and 30901721) and by grants from Shenzhen Natural Science Foundation.
Conflict of Interest
The authors declare that the research was conducted in the absence of any commercial or financial relationships that could be construed as a potential conflict of interest.
Footnotes
References
1. O’Grady S, Morgan MP. Microcalcifications in breast cancer: from pathophysiology to diagnosis and prognosis. Biochim Biophys Acta Rev Cancer. (2018) 1869:310–20. doi: 10.1016/j.bbcan.2018.04.006
2. Roumeliotis GA, Dostaler G, Boyd KU. Complementary and Alternative medicines and patients with breast cancer: a case of mortality and systematic review of patterns of use in patients with breast cancer. Plast Surg (Oakv). (2017) 25:275–83. doi: 10.1177/2292550317716126
3. DeSantis CE, Ma J, Gaudet MM, Newman LA, Miller KD, Goding SA, et al. Breast cancer statistics, 2019. CA Cancer J Clin. (2019) 69:438–51. doi: 10.3322/caac.21583
4. Ishibashi K, Hara S, Kondo S. Aquaporin water channels in mammals. Clin Exp Nephrol. (2009) 13:107–17. doi: 10.1007/s10157-008-0118-6
5. Saadoun S, Papadopoulos MC, Davies DC, Bell BA, Krishna S. Increased aquaporin 1 water channel expression in human brain tumours. Br J Cancer. (2002) 87:621–3. doi: 10.1038/sj.bjc.6600512
6. Kang BW, Kim JG, Lee SJ, Chae YS, Jeong JY, Yoon GS, et al. Expression of aquaporin-1, aquaporin-3, and aquaporin-5 correlates with nodal metastasis in colon cancer. Oncology. (2015) 88:369–76. doi: 10.1159/000369073
7. Hu J, Verkman AS. Increased migration and metastatic potential of tumor cells expressing aquaporin water channels. Faseb J. (2006) 20:1892–4. doi: 10.1096/fj.06-5930fje
8. Shi Z, Zhang T, Luo L, Zhao H, Cheng J, Xiang J, et al. Aquaporins in human breast cancer: identification and involvement in carcinogenesis of breast cancer. J Surg Oncol. (2012) 106:267–72. doi: 10.1002/jso.22155
9. Otterbach F, Callies R, Adamzik M, Kimmig R, Siffert W, Schmid KW, et al. Aquaporin 1 (AQP1) expression is a novel characteristic feature of a particularly aggressive subgroup of basal-like breast carcinomas. Breast Cancer Res Treat. (2010) 120:67–76. doi: 10.1007/s10549-009-0370-9
10. Esteva-Font C, Jin BJ, Verkman AS. Aquaporin-1 gene deletion reduces breast tumor growth and lung metastasis in tumor-producing MMTV-PyVT mice. Faseb J. (2014) 28:1446–53. doi: 10.1096/fj.13-245621
11. Shukla GC, Singh J, Barik S. MicroRNAs: processing, maturation, target recognition and regulatory functions. Mol Cell Pharmacol. (2011) 3:83–92.
12. Bartel DP. MicroRNAs: target recognition and regulatory functions. Cell. (2009) 136:215–33. doi: 10.1016/j.cell.2009.01.002
13. Wang L, Sun J, Cao H. MicroRNA−384 regulates cell proliferation and apoptosis through directly targeting WISP1 in laryngeal cancer. J Cell Biochem. (2018) 120:3018–26. doi: 10.1002/jcb.27323
14. Luengo-Gil G, García-Martínez E, Chaves-Benito A, Conesa-Zamora P, Navarro-Manzano E, González-Billalabeitia E, et al. Clinical and biological impact of miR-18a expression in breast cancer after neoadjuvant chemotherapy. Cell Oncol. (2019) 42:627–44. doi: 10.1007/s13402-019-00450-2
15. Long X, Shi Y, Ye P, Guo J, Zhou Q, Tang Y. MicroRNA-99a suppresses breast cancer progression by targeting FGFR3. Front Oncol. (2019) 9:1473. doi: 10.3389/fonc.2019.01473
16. Xiao S, Zhu H, Luo J, Wu Z, Xie M. miR4255p is associated with poor prognosis in patients with breast cancer and promotes cancer cell progression by targeting PTEN. Oncol Rep. (2019) 42:2550–60. doi: 10.3892/or.2019.7371
17. Yao B, Li Y, Wang L, Chen T, Niu Y, Liu Q, et al. MicroRNA-3194-3p inhibits metastasis and epithelial-mesenchymal transition of hepatocellular carcinoma by decreasing Wnt/beta-catenin signaling through targeting BCL9. Artif Cells Nanomed Biotechnol. (2019) 47:3885–95. doi: 10.1080/21691401.2019.1670190
18. Wei M, Shi R, Zeng J, Wang N, Zhou J, Ma W. The over-expression of aquaporin-1 alters erythroid gene expression in human erythroleukemia K562 cells. Tumour Biol. (2015) 36:291–302. doi: 10.1007/s13277-014-2614-5
19. Li T, Zhang C, Ding Y, Zhai W, Liu K, Bu F, et al. Umbilical cord-derived mesenchymal stem cells promote proliferation and migration in MCF-7 and MDA-MB-231 breast cancer cells through activation of the ERK pathway. Oncol Rep. (2015) 34:1469–77. doi: 10.3892/or.2015.4109
20. Wu Y, Chen X, Liang Y, Li J, Zhang K, Dai L, et al. Overexpression of long non-coding RNA SOX2OT promotes esophageal squamous cell carcinoma growth. Cancer Cell Int. (2018) 18:76. doi: 10.1186/s12935-018-0570-7
21. Liu Z, Wang Y, Wang L, Yao B, Sun L, Liu R, et al. Long non-coding RNA AGAP2-AS1, functioning as a competitive endogenous RNA, upregulates ANXA11 expression by sponging miR-16-5p and promotes proliferation and metastasis in hepatocellular carcinoma. J Exp Clin Cancer Res. (2019) 38:194. doi: 10.1186/s13046-019-1188-x
22. Luo LM, Xia H, Shi R, Zeng J, Liu XR, Wei M. The association between aquaporin-1 expression, microvessel density and the clinicopathological features of hepatocellular carcinoma. Oncol Lett. (2017) 14:7077–84. doi: 10.3892/ol.2017.7106
23. Wei M, Yu H, Zhang Y, Zeng J, Cai C, Shi R. Decreased expression of aquaporin 1 correlates with clinicopathological features of patients with cervical cancer. Onco Targets Ther. (2019) 12:2843–51. doi: 10.2147/OTT.S194650
24. Luo L, Yang R, Zhao S, Chen Y, Hong S, Wang K, et al. Decreased miR-320 expression is associated with breast cancer progression, cell migration, and invasiveness via targeting Aquaporin 1. Acta Biochim Biophys Sin (Shanghai). (2018) 50:473–80. doi: 10.1093/abbs/gmy023
25. Yamazato Y, Shiozaki A, Ichikawa D, Kosuga T, Shoda K, Arita T, et al. Aquaporin 1 suppresses apoptosis and affects prognosis in esophageal squamous cell carcinoma. Oncotarget. (2018) 9:29957. doi: 10.18632/oncotarget.25722
26. Stigliano C, Aryal S, de Tullio MD, Nicchia GP, Pascazio G, Svelto M, et al. siRNA-chitosan complexes in poly(lactic-co-glycolic acid) nanoparticles for the silencing of aquaporin-1 in cancer cells. Mol Pharm. (2013) 10:3186–94. doi: 10.1021/mp400224u
27. Saadoun S, Papadopoulos MC, Hara-Chikuma M, Verkman AS. Impairment of angiogenesis and cell migration by targeted aquaporin-1 gene disruption. Nature. (2005) 434:786–92. doi: 10.1038/nature03460
28. Nicchia GP, Stigliano C, Sparaneo A, Rossi A, Frigeri A, Svelto M. Inhibition of aquaporin-1 dependent angiogenesis impairs tumour growth in a mouse model of melanoma. J Mol Med (Berl). (2013) 91:613–23. doi: 10.1007/s00109-012-0977-x
29. Otterbach F, Callies R, Kimmig R, Schmid KW, Bankfalvi A. Aquaporin 1 expression in invasive breast carcinomas. Pathologe. (2008) 29(Suppl. 2):357–62. doi: 10.1007/s00292-008-1039-6
30. Cui Q, Yu Z, Purisima EO, Wang E. Principles of microRNA regulation of a human cellular signaling network. Mol Syst Biol. (2006) 2:46. doi: 10.1038/msb4100089
31. Poy MN, Eliasson L, Krutzfeldt J, Kuwajima S, Ma X, Macdonald PE, et al. A pancreatic islet-specific microRNA regulates insulin secretion. Nature. (2004) 432:226–30. doi: 10.1038/nature03076
32. Brennecke J, Hipfner DR, Stark A, Russell RB, Cohen SM. Bantam encodes a developmentally regulated microRNA that controls cell proliferation and regulates the proapoptotic gene hid in Drosophila. Cell. (2003) 113:25–36. doi: 10.1016/s0092-8674(03)00231-9
33. Yu Z, Baserga R, Chen L, Wang C, Lisanti MP, Pestell RG. microRNA, cell cycle, and human breast cancer. Am J Pathol. (2010) 176:1058–64. doi: 10.2353/ajpath.2010.090664
34. Zhu L, Lin ZW, Wang G, Zhang H, Liu B, Xu QJ. MicroRNA-495 downregulates AQP1 and facilitates proliferation and differentiation of osteoblasts in mice with tibial fracture through activation of p38 MAPK signaling pathway. Sci Rep. (2019) 9:16171. doi: 10.1038/s41598-019-50013-6
Keywords: miR-3194-3p, AQP1, proliferation, migration, invasion, apoptosis, breast cancer
Citation: Wei M, Yu H, Cai C, Gao R, Liu X and Zhu H (2020) MiR-3194-3p Inhibits Breast Cancer Progression by Targeting Aquaporin1. Front. Oncol. 10:1513. doi: 10.3389/fonc.2020.01513
Received: 28 April 2020; Accepted: 14 July 2020;
Published: 07 August 2020.
Edited by:
Kartiki V. Desai, National Institute of Biomedical Genomics (NIBMG), IndiaReviewed by:
Chandramani Pathak, Indian Institute of Advanced Research, IndiaTanja Kunej, University of Ljubljana, Slovenia
Copyright © 2020 Wei, Yu, Cai, Gao, Liu and Zhu. This is an open-access article distributed under the terms of the Creative Commons Attribution License (CC BY). The use, distribution or reproduction in other forums is permitted, provided the original author(s) and the copyright owner(s) are credited and that the original publication in this journal is cited, in accordance with accepted academic practice. No use, distribution or reproduction is permitted which does not comply with these terms.
*Correspondence: Min Wei, weiminphd@126.com