- 1Facultad de Ciencias Químico Biológicas, Universidad Autónoma de Sinaloa, Culiacán, Mexico
- 2Posgrado en Ciencias Genómicas, Universidad Autónoma de la Ciudad de México, Mexico City, Mexico
Gastrointestinal cancers (GICs) are the most common human tumors worldwide. Treatments have limited effects, and increasing global cancer burden makes it necessary to investigate alternative strategies such as drug repurposing. Interestingly, it has been found that psychiatric drugs (PDs) are promising as a new generation of cancer chemotherapies due to their anti-neoplastic properties. This review compiles the state of the art about how PDs have been redirected for cancer therapeutics in GICs. PDs, especially anti-psychotics, anti-depressants and anti-epileptic drugs, have shown effects on cell viability, cell growth, inhibition of proliferation (cell cycle arrest), apoptosis promotion by caspases activation or cytochrome C release, production of reactive oxygen species (ROS) and nuclear fragmentation over esophageal, gastric, colorectal, liver and pancreatic cancers. Additionally, PDs can inhibit neovascularization, invasion and metastasis in a dose-dependent manner. Moreover, they can induce chemosensibilization to 5-fluorouracil and cisplatin and can act synergistically with anti-neoplastic drugs such as gemcitabine, paclitaxel and oxaliplatin. All anti-cancer activities are given by activation or inhibition of pathways such as HDAC1/PTEN/Akt, EGFR/ErbB2/ErbB3, and PI3K/Akt; PI3K-AK-mTOR, HDAC1/PTEN/Akt; Wnt/β-catenin. Further investigations and clinical trials are needed to elucidate all molecular mechanisms involved on anti-cancer activities as well as adverse effects on patients.
Introduction
Gastrointestinal cancers (GICs) are the most common human tumors worldwide, affecting esophageal, stomach, colorectum, liver, pancreas, and gallbladder tissues (1). The treatment for these malignancies is surgical resection, nonetheless, at the time of diagnosis, most of the cases are unresectable (2–4). Additionally, chemotherapy and radiotherapy show a modest improvement in survival. These limited results and the increasing global cancer burden require investigating new strategies such as drug repurposing.
Mental disorders and substance abuse are at present the leading cause of disability worldwide (5), giving as a result a wide use of psychiatric drugs (PDs) for treatment. These long-term drug therapies have been studied in order to elucidate if its use is a risk factor, but at the same time it is possible that their molecular mechanisms could be used to treat different cancer types. On the one hand, Food and Drug Administration (FDA) preclinical-limited to animal studies showed that 63.6% of tested anti-depressants, 90% of anti-psychotic, 70% of benzodiazepines and sedative-hypnotics, 25% of amphetamines and stimulants, and 85.7% of anti-convulsants were associated with carcinogenicity (6). On the other hand, it has been found that PDs are promising as a new generation of cancer chemotherapies due to their anti-neoplastic properties (7), such as autophagy, which can inhibits migration and invasiveness in different types of cancer (8).
The repurposing potential of PDs has ignited interest in further exploration for treating GICs. The aim of this review is to compile the most recent and relevant information about how psychiatric drugs have been redirected due to their inhibitory potential on carcinogenic processes, such as, growth, proliferation, metastasis and cell survival in GICs (Figure 1A).
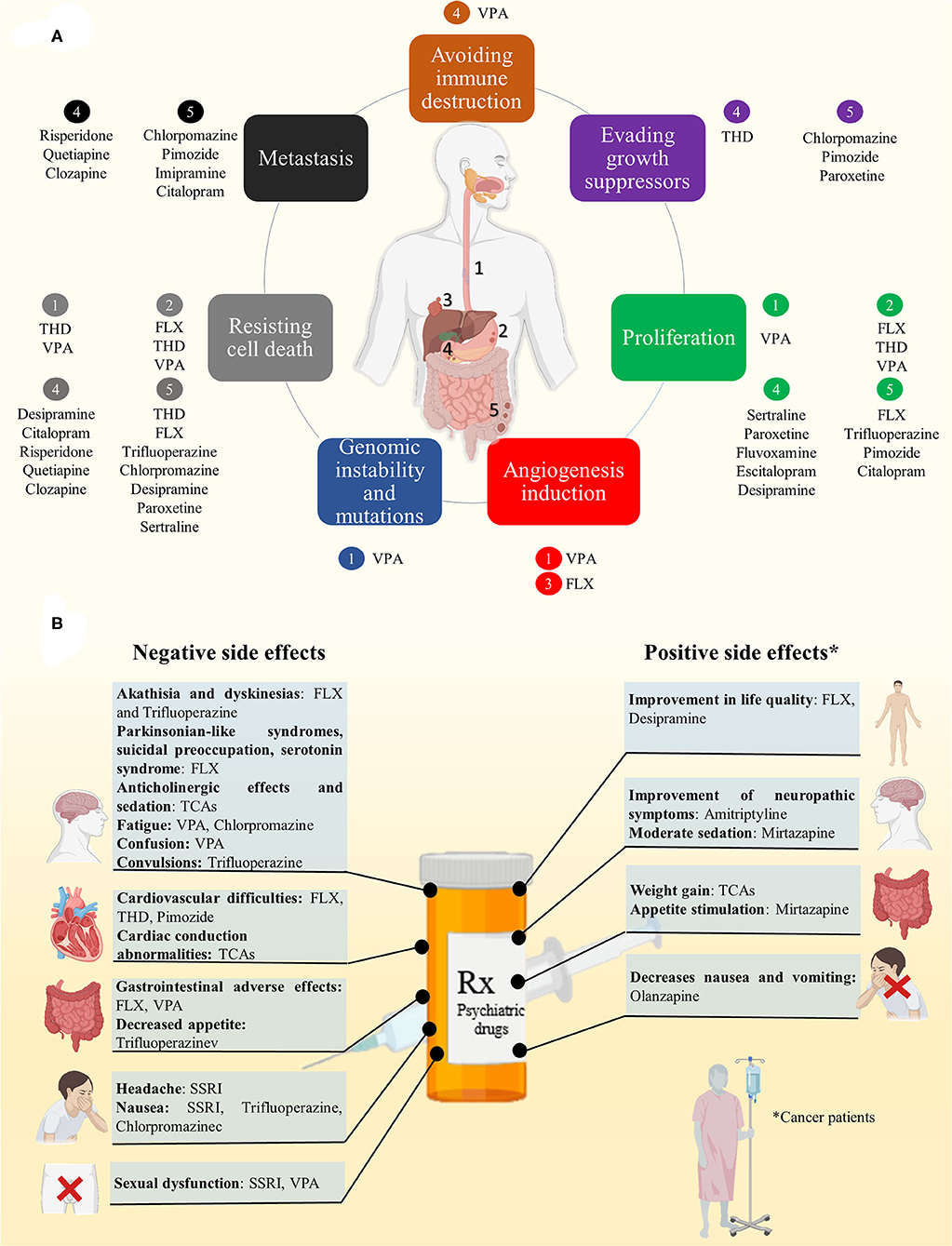
Figure 1. (A) Refocusing psychiatric drugs in GICs. PDs have shown inhibitory potential on carcinogenic processes, such as, growth, proliferation, angiogenesis, metastasis and cell survival on esophageal (1), gastric (2), liver (3), pancreatic (4), and colorectal cancer (5). (B) PDs exhibit positive and negative side effects that could affect the general state of patients and need to be considered.
Refocusing Psychiatric Drugs on GICS
Esophageal Cancer
Thioridazine (THD), an antagonist of the dopamine receptor D2, is a potent anti-anxiety and anti-psychotic that in combination with radiotherapy promotes G0/G1 phase cell cycle arrest by CDK4 and cyclinD1 downregulation on the esophageal squamous cell carcinoma (ESCC) ECA-109 and TE-1 cell lines. It also induces apoptosis upregulating cleaved caspase-3 and 9 as well as Bax, Bak, and p53, and decreasing Bcl-2 and Bcl-xL expression. Besides, this treatment inhibits PI3K-AK-mTOR pathway. On xenograft mouse model, THD/radiotherapy reduces ESCC tumor growth (9).
Valproic acid (VPA), an anti-epileptic (10), inhibits radiation-induced double-strand break (DSB) reparation by non-homologous end joining (NHEJ) suppression, specifically through Ku70 acetylation on the human ESCC KES, TE9 and TE11 cell lines. Also, VPA after irradiation prolongs H2AX phosphorylation (γH2AX) levels that result on foci formation detection (11). In addition, VPA inhibits viability in a dose-dependent manner leading to apoptosis, and radiation-induced cytotoxicity by chromatin decondensation with H3 and H4 hyperacetylation/Rad51 downregulation on ESCC TE9, TE10, TE11, and TE14 cell lines (12). Interestingly, VPA leads to Epithelial Mesenchymal Transition (EMT) reversal induced by irradiation on ESCC TE9 cells, attenuating cell invasion, and migration (13).
Gastric Cancer
Fluoxetine (FLX) is a selective serotonin reuptake inhibitor (SSRI) to treat depression and panic disorder (14). In the human Gastric Adenocarcinoma cell line AGS, FLX inhibits proliferation in a dose-dependent manner. Moreover, FLX induces apoptosis increasing activated caspases, cleaved PARP, and promoting ROS production. Interestingly, FLX-treated AGS cells exhibit upregulation of CHOP (an endoplasmic reticulum stress marker) and its inhibition suppress partially apoptotic effects of FLX through death receptor 5 (DR5), cleaved caspase 3, and cleaved PARP downregulation (15). Besides, FLX induces both, protective autophagosome formation and apoptosis simultaneously in vitro. However, autophagy inhibition enhances FLX-induced apoptosis through decrease p-Akt and increase DR4 and DR5 expression (16). Moreover, it has been reported an improved anti-proliferative effect due to FLX/paclitaxel combination on GACs (AGS cell line). In addition, FLX/paclitaxel promotes cell death by early apoptotic and late apoptotic cell as well as necroptosis (17).
THD has cytotoxic effect on Gastric cancer (GC) cell lines NCI-N87 and AGS, inhibiting colony formation ability, and induces nuclear fragmentation and apoptosis in a caspase-dependent manner through downregulation of caspase-9, caspase-8, and caspase-3 precursors. Also, in vivo experiments using BALB/c nude mice showed an inhibition of tumor growth using pre-treated GC cells with THD (18).
VPA-treated AGS and SGC-7901 cells repress HDAC1/2 (histone deacetylase) activity and induces autophagy driving to apoptosis through HDAC1/PTEN/Akt signaling pathway inhibition, as well as Bcl-2 and Beclin-1 alterations. VPA treatment on xenograft models have shown significant inhibitory effect on cell growth via autophagy/apoptosis (19). VPA affects cell viability on GC OCUM-2MD3 cells, a derived cell line from human scirrhous GC. Besides, VPA increases acetyl-histone H3, acetyl-a-tubulin and p21WAF1 expression as well as p27, caspase 3, and caspase 9 upregulation. Furthermore, VPA downregulates bcl-2, cyclin D1, and survivin. In vivo experiments using xenograft models with previously VPA-treated cells inhibits tumor volume and apoptosis (20). Additionally, VPA inhibits proliferation by cell cycle arrest in G1 phase. Apoptosis activation induced by VPA is conducted mainly via intrinsic pathway through Caspase 9 and Caspase 3 activation and partially via extrinsic pathway by caspase-8 activation in BGC-823 gastric carcinoma cells. In addition, P21Waf/cip1, Mad1 upregulation and Cyclin A, c-Myc downregulation result in cell cycle arrest in G1 phase (21).
Colorectal Cancer
Trifluoperazine, an anti-psychotic, inhibits cell viability and proliferation, induces apoptosis and promotes cell arrest at G0/G1 phase by repressing CDK2, CDK4, cyclin D1, cyclin E through the increasing p27 expression on HCT116. Also, this effect has a synergized effect with 5-fluorouracil and Oxaliplatin in CT26 and HCT116 colorectal cancer cells (22, 23).
THD treatment exhibits anti-tumor effect on CSCs EpCAM+/CD44+ subpopulations isolated from HCT116 through apoptosis induction via mitochondrial pathway since THD promotes upregulation of caspase-3 and Bax and downregulation of Bcl-2 suppressing proliferation and invasion (24).
Chlorpromazine reduces psychotic symptoms and has a potent anti-tumor activity (25–27). In CRC cell lines, such as, HCT116, LoVo, HT15, and HT29, chlorpromazine can suppresses cell growth, activates apoptosis by p53 acetylation and induces SIRT1 degradation acting as a cell-cycle regulator (28). Another anti-psychotic, Pimozide, dissociates the interaction between ARPC2 and vinculin suppressing focal adhesion, which blocks migration in DLD-1 CRC cells (29). Besides, Pimozide shows anti-proliferative features, suppresses migration in a dose-dependent manner, and increases N-cadherin, vimentin, and Snail expression as well as inhibits Wnt/β-catenin signaling pathway in HCT116 and SW480 cells. Also, inhibits tumor growth in xenografts mouse model (30).
Regarding tricyclic anti-depressants (TCAs), desipramine promotes apoptosis and modulates cell-cycle arrest at G0/G1-phase reducing a dose-dependent S-phase proportion cells in HT29 CRC cell line (31). Besides, FLX treatment target early colon carcinogenesis due to its anti-proliferative effect by affecting ROS production, DNA damage, cell viability, apoptosis, and cell-cycle in HT29 cell line. Besides, using C57BL/6 mice exposed to the carcinogen N-methyl-N'-nitro-N-nitrosoguanidine (MNNG) was demonstrated that FLX affects proliferation mainly in epithelial and stromal areas accompanied by reduction of VEGF expression and number of cells with angiogenic potential, such as CD133+, CD34+, and CD31+ (32).
Imipramine also compromises HCT116 cells viability at low concentrations and inhibits lamellipodium formation, cell migration and invasion by affecting fascin1 (33). On the other hand, has been reported that paroxetine reduces cell viability and increases apoptosis; it also inhibits colony and 3D spheroid formation in HCT116 and HT29 cell lines. Probably, paroxetine can act by the inhibition of MET and ERBB3 which are two major receptor tyrosine kinases, leading to the suppression of AKT, ERK and p38 activation and induction of JNK and caspase-3 pathways. Also, in vivo assays using athymic nude mice treated with paroxetine showed tumor growth suppression (34).
In the case of the SSRI Citalopram, it inhibits migration in HT29 and HCT116 cell lines. Besides, it has anti-proliferative effect on xenograft mouse model as well as in orthotopic mouse model, acting against metastatic progression (35). Another SSRI, Sertraline, arrest cells at the G0/G1 stage and stimulates DNA fragmentation in a dose-dependent manner and at low concentrations promotes apoptosis by increasing caspase-3 activation and c-Jun expression as well as a decrease in the anti-apoptotic protein Bcl-2 (36).
The dopamine inhibitor hydrochloride (IND), the dopamine receptor antagonists chlorpromazine hydrochloride (CPZ), and fluphenazine dihydrochloride (FPZ) are able to increase LC3-II in a dose- and time-dependent manner in HCT116 cells. Moreover, FPZ induces autophagy by mTOR signaling inhibition, meanwhile IND and CPZ induces autophagy in an mTOR-independent manner. However, in this context autophagy does not stimulates apoptosis, rather offers beneficial effects for cell survival in HCT116 treated with IND, CPZ and FPZ (37).
Liver Cancer
SSRIs including FLX, sertraline, paroxetine, citalopram, escitalopram, and fluvoxamine are associated with a lower risk of hepatocellular carcinoma (HCC) in a dose-dependent manner (38). Also, sertraline, paroxetine, fluvoxamine, and escitalopram have shown significant decrease of cell viability in HCC, being sertraline the most efficient to induce caspase-3/7 activity in HepG2 cells (39). Another anti-depressant, desipramine reduces cell viability by increasing ROS production, inhibits MMP activity in Hep3B cells. Besides, it is able to induce apoptosis through MAPKs activation (ERK 1/2, JNK, and p38), companied of intracellular Ca2+ levels increase (40). In addition, citalopram activates NF-kB and is a potent apoptosis-inductor since increases mitochondrial Bax levels, decreases in Bcl2 levels, increases ROS and releases cytochrome c release in HepG2 cells (41).
The use of risperidone, quetiapine, and clozapine, have shown reduction of cell viability, cell proliferation, invasion, and induction of apoptosis in vitro, in Huh7, and HepG2 cells (42). Meanwhile, several HCC cell lines treated with the anti-psychotic pimozide exhibit inhibition of cell proliferation and sphere formation through G0/G1 phase cell cycle arrest as well as cell migration. In addition, it suppresses STAT3 signaling. Besides, pimozide inhibits stemness properties of HCC stem-like cells as colony formation, sphere formation, migration and has the capacity to reverses the stem phenotype. In vivo, pimozide reduces the tumor burden in the nude mice xenograft model (43) and affects cell viability on Hep3B and HepG2 cells by inhibition of·Wnt/β-catenin signaling and EpCAM gene and protein expression (44).
Pancreatic Cancer
Mirtazapine is a novel noradrenergic and specific serotonergic anti-depressant (NaSSA) (45) that helps during gemcitabine-induced mild cachexia in pancreatic tumor-induced on mice (46). Olanzapine, an anti-psychotic, inhibits survivin expression on pancreatic CSCs which results on chemosensibilization to 5-fluorouracil, gemcitabine and cisplatin (47) (Table 1). On the other hand, 5-HT uptake promotes activation of Rac1 resulting in trans-differentiation of primary acinar cells into acinar-to-ductal metaplasia (ADM), a determinant step to Pancreatic Ductal Adenocarcinoma (PDAC). However, FLX inhibits to Slc6a4, a 5-HT transporter Sert, reducing ADM formation both in vitro and in vivo on mice model. Besides, FLX treatment inhibits proliferation, tumor microenvironment and alters lipid metabolism of several PDAC cell lines (49).
VPA inhibits cell proliferation and cell attachment to the endothelium on DanG cells and enhances β1 integrin subunits expression such as α4, α5, and α6 (50). Besides, VPA inhibits cell survival and induces apoptosis via EGFR/ErbB2/ErbB3 downregulation on HPAF-II, MPanc96 PC cells and inhibits tumor growth on xenograft models (51). Moreover, VPA increases susceptibility of PANC-1, MIA PaCa-2, and BxPC-3 PC cell lines to NK cell-mediated lysis both in vitro and in vivo (xenografts in NOD/SCID mice) by MICA and MICB upregulation in a dependent mechanism of PI3K/Akt signaling pathway (52). Interestingly, high-dose VPA in combination with gemcitabine has a synergic effect enhancing the sensitivity of PANC-1 and Patu8988 PC cell lines to gemcitabine, meanwhile low-dose of VPA potentiates migration, invasion, and promotes ROS production, activating AKT that induces STAT3/Bmi1 signaling cascade activation as well as migration and invasion of PC cells induced by gemcitabine. However, high-dose VPA stimulates ROS accumulation promoting p38 activation and suppressing STAT3/Bmi1 activation (53). Combination of VPA with PEG-IFNα inhibits cell proliferation on BxPC3 PC cell line by caspase-3/7 activity promotion. In addition, the combination sensibilizes BxPC3 and SUIT-2 PC cells to interferon-α, promoting IFN-related genes expression, IFNAR1, IFNAR2, and IRF8 (54).
In the case of the anti-psychotic drugs penfluridol, pimozide, fluspirilene, and promethazine have an antagonist effect on proliferation and induce autophagy by LC3II and p62 upregulation in Panc-1, and MiaPaCa-2 human PDAC cell lines. Also, penfluridol suppress colony and spheroid formation and decreases PRL-induced JAK2 signaling by binding to PRLR. In vivo, using NSG mice, penfluridol have shown tumor growth suppression (55). Additionally, penfluridol shows cytotoxic effects as well as autophagy-mediated apoptosis in the pancreatic cancer Panc-1, AsPC-1, and BxPC-3 cell lines and is able to suppress subcutaneously implanted pancreatic tumor growth through autophagy induction on athymic nude mice. Finally, Panc-1 cells implanted into the pancreas of mice shows that penfluridol increases p62 and LC3B as well as cleavage caspase3 and PARP (56).
Clinical Implications and Side Effects
PDs exhibit side effects that need to be considered (Figure 1B):
• SSRI anti-depressants: headache, nausea, and sexual dysfunction, as well as several cytochrome P450 drug interaction (57).
• FLX: cardiovascular difficulties, akathisia, dyskinesias, parkinsonian-like syndromes, suicidal preoccupation, “serotonin syndrome” (58), gastrointestinal adverse effects compared to other anti-depressants (59).
• FLX and Desipramine offer benefits on quality of life issues in patients with advanced cancer (60).
• TCAs: cardiac conduction abnormalities, anti-cholinergic effects, weight gain and sedation. Specifically, Amitriptyline and Imipramine induce sedation, anti-cholinergic effects, weight gain, sexual dysfunction, sedation, and cardiac effects (61). However, weight gain associated to TCAs can be a well feature on cancer patients. Besides, low doses of Amitriptyline improve several neuropathic symptoms which result in a best quality of life on cancer patient (62).
• VPA: fatigue, confusion, neuroconstipation, and somnolence (63).
• Trifluoperazine: decreased appetite and induces nausea, insomnia, akathisia, dyskinesias, skin disorders, and tremors including convulsions, as well as, hyperprolactinaemia and galacthorrea (64–67).
• Chlorpromazine: nausea, drowsiness, and tiredness (68).
• THD and Pimozide: cardiac effects (69).
• Mirtazapine: can induces moderate sedation and appetite stimulation that can enhances patients' wellness (70).
• Olanzapine: decrease chemotherapy side effects as nausea and vomiting in advanced cancer patients (71).
Concluding Remarks
GICs still rank among the leading causes of cancer related death because of late-stage detection and poor survival following metastasis. Treatments depend on multiple factors and constantly fail. Psychiatric drugs (PDs) are promising as a new generation of cancer chemotherapies due to their anti-neoplastic properties in a plethora of cancer types.
Drug repurposing potential of PDs has been studied for the treatment of GICs founding that these drugs can modulate several cellular processes as cell viability, cell growth, proliferation (cell cycle arrest), apoptosis, autophagy, ROS production, and DNA fragmentation. Besides, PDs can inhibit neovascularization, invasion, and metastasis and effects can be in a dose-dependent manner, such as, VPA, FLX, Pimozide, Desipramine, and Sertraline. Moreover, PDs can induce chemosensibilization as Olanzapine combined with 5-fluorouracil, gemcitabine, and cisplatin induce cell death. In addition, low-dose of VPA enhances cytotoxicity to gemcitabine. FLX also shows anti-proliferative effect in combination with paclitaxel, while, trifluoroperazine exerts viability inhibition when combined with 5-fluorouracil and oxaliplatin.
The modulation of several processes by PDs can be through activation or inhibition of multiple pathways such as HDAC1/PTEN/Akt, EGFR/ErbB2/ErbB3, and PI3K/Akt (VPA); PI3K-AK-mTOR, HDAC1/PTEN/Akt (THD); Wnt/β-catenin (pimozide).
Finally, PDs as repurposed drugs, could be more affordable for patients and could also reduce costs for drugs developers. PDs might improve cancer treatments as well as life quality of patients in long therm. Nevertheless, further investigations and clinical trials are needed to elucidate all molecular mechanisms involved on anti-cancer activities of PDs as well as negative side effects on patients.
Author Contributions
MA-F, MA-M, MB, EL-V, and RR-P conceived and designed the content of this review and wrote the paper. CL-C contributed to the final version of the manuscript. All authors contributed to the article and approved the submitted version.
Funding
The authors acknowledge Consejo Nacional de Ciencia y Tecnología CONACYT (grant 290311) for funding.
Conflict of Interest
The authors declare that the research was conducted in the absence of any commercial or financial relationships that could be construed as a potential conflict of interest.
Acknowledgments
The authors acknowledge CONACyT for MA-F (575985) and EL-V (304939) fellowships.
References
1. Dizdar Ö, Kiliçkap S. Global epidemiology of gastrointestinal cancers. In: Yalcin S, Philip PA, editors. Textbook of Gastrointestinal Oncology. Cham: Springer International Publishing (2019). p. 1–12.
2. Petrelli F, Coinu A, Borgonovo K, Cabiddu M, Barni S. Progression-free survival as surrogate endpoint in advanced pancreatic cancer: meta-analysis of 30 randomized first-line trials. Hepatobiliary Pancreat Dis Int. (2015) 14:124–31. doi: 10.1016/S1499-3872(15)60344-7
3. Chen D, Wang W, Chen Y, Hu J, Yang M, Mo J, et al. Recurrence and prognostic model for identifying patients at risk for esophageal cancer after surgery. Cancer Manag Res. (2018) 10:6109–20. doi: 10.2147/CMAR.S186194
4. Saadati K, Moghimi M, Baba Ali S, Eghdam Zamiri R. A study on comparison of overall survival and disease- free survival among gastric cancer patients treated with two adjuvant and neoadjuvant methods. J Med Life. (2015) 8:186–8. Available online at: https://medandlife.org/all-issues/2015/special-issue-4-2015/original-articles-special-issue-4-2015/a-study-on-comparison-of-overall-survival-and-disease-free-survival-among-gastric-cancer-patients-treated-with-two-adjuvant-and-neoadjuvant-methods/
5. Whiteford HA, Degenhardt L, Rehm J, Baxter AJ, Ferrari AJ, Erskine HE, et al. Global burden of disease attributable to mental and substance use disorders: findings from the global burden of disease study 2010. Lancet. (2013) 382:1575–86. doi: 10.1016/S0140-6736(13)61611-6
6. Amerio A, Gálvez JF, Odone A, Dalley SA, Ghaemi SN. Carcinogenicity of psychotropic drugs: a systematic review of US food and drug administration–required preclinical in vivo studies. Aust N Z J Psychiatry. (2015) 49:686–96. doi: 10.1177/0004867415582231
7. Huang J, Zhao D, Liu Z, Liu F. Repurposing psychiatric drugs as anti-cancer agents. Cancer Lett. (2018) 419:257–65. doi: 10.1016/j.canlet.2018.01.058
8. Vucicevic L, Misirkic-Marjanovic M, Harhaji-Trajkovic L, Maric N, Trajkovic V. Mechanisms and therapeutic significance of autophagy modulation by antipsychotic drugs. Cell Stress. (2018) 2:282–91. doi: 10.15698/cst2018.11.161
9. Li H, Juan L, Xia L, Wang Y, Bao Y, Sun G. Thioridazine sensitizes esophageal carcinoma cell lines to radiotherapy-induced apoptosis in vitro and in vivo. Med Sci Monit. (2016) 22:2624–34. doi: 10.12659/MSM.899950
10. Zhu MM, Li HL, Shi LH, Chen XP, Luo J, Zhang ZL. The pharmacogenomics of valproic acid. J Hum Genet. (2017) 62:1009–14. doi: 10.1038/jhg.2017.91
11. Makita N, Ninomiya I, Tsukada T, Okamoto K, Harada S, Nakanuma S, et al. Inhibitory effects of valproic acid in DNA double-strand break repair after irradiation in esophageal squamous carcinoma cells. Oncol Rep. (2015) 34:1185–92. doi: 10.3892/or.2015.4089
12. Shoji M, Ninomiya I, Makino I, Kinoshita J, Nakamura K, Oyama K, et al. Valproic acid, a histone deacetylase inhibitor, enhances radiosensitivity in esophageal squamous cell carcinoma. Int J Oncol. (2012) 40:2140–6. doi: 10.3892/ijo.2012.1416
13. Kanamoto A, Ninomiya I, Harada S, Tsukada T, Okamoto K, Nakanuma S, et al. Valproic acid inhibits irradiation-induced epithelial-mesenchymal transition and stem cell-like characteristics in esophageal squamous cell carcinoma. Int J Oncol. (2016) 49:1859–69. doi: 10.3892/ijo.2016.3712
14. Wong DT, Horng JS, Bymaster FP, Hauser KL, Molloy BB. A selective inhibitor of serotonin uptake: lilly 110140, 3-(p-trifluoromethylphenoxy)-N-methyl-3-phenylpropylamine. Life Sci. (1974) 15:471–9. doi: 10.1016/0024-3205(74)90345-2
15. Khin PP, Po WW, Thein W, Sohn UD. Apoptotic effect of fluoxetine through the endoplasmic reticulum stress pathway in the human gastric cancer cell line AGS. Naunyn Schmiedebergs Arch Pharmacol. (2020) 393:537–49. doi: 10.1007/s00210-019-01739-7
16. Po WW, Thein W, Khin PP, Khing TM, Han KWW, Park CH, et al. Fluoxetine simultaneously induces both apoptosis and autophagy in human gastric adenocarcinoma cells. Biomol Ther. (2020) 28:202–10. doi: 10.4062/biomolther.2019.103
17. Khing TM, Po WW, Sohn UD. Fluoxetine enhances anti-tumor activity of paclitaxel in gastric adenocarcinoma cells by triggering apoptosis and necroptosis. Anticancer Res. (2019) 39:6155–63. doi: 10.21873/anticanres.13823
18. Mu J, Xu H, Yang Y, Huang W, Xiao J, Li M, et al. Thioridazine, an antipsychotic drug, elicits potent antitumor effects in gastric cancer. Oncol Rep. (2014) 31:2107–14. doi: 10.3892/or.2014.3068
19. Sun J, Piao J, Li N, Yang Y, Kim KY, Lin Z. Valproic acid targets HDAC1/2 and HDAC1/PTEN/Akt signalling to inhibit cell proliferation via the induction of autophagy in gastric cancer. FEBS J. (2019) 287:2118–33. doi: 10.1111/febs.15122
20. Yagi Y, Fushida S, Harada S, Kinoshita J, Makino I, Oyama K, et al. Effects of valproic acid on the cell cycle and apoptosis through acetylation of histone and tubulin in a scirrhous gastric cancer cell line. J Exp Clin Cancer Res. (2010) 29:149. doi: 10.1186/1756-9966-29-149
21. Zhao X, Yang W, Shi C, Ma W, Liu J, Wang Y, et al. The G1 phase arrest and apoptosis by intrinsic pathway induced by valproic acid inhibit proliferation of BGC-823 gastric carcinoma cells. Tumour Biol. (2011) 32:335–46. doi: 10.1007/s13277-010-0126-5
22. Qian K, Sun L, Zhou G, Ge H, Meng Y, Li J, et al. Trifluoperazine as an alternative strategy for the inhibition of tumor growth of colorectal cancer. J Cell Biochem. (2019) 120:15756–65. doi: 10.1002/jcb.28845
23. Xia Y, Jia C, Xue Q, Jiang J, Xie Y, Wang R, et al. Antipsychotic drug trifluoperazine suppresses colorectal cancer by inducing G0/G1 arrest and apoptosis. Front Pharmacol. (2019) 10:1029. doi: 10.3389/fphar.2019.01029
24. Zhang C, Gong P, Liu P, Zhou N, Zhou Y, Wang Y. Thioridazine elicits potent antitumor effects in colorectal cancer stem cells. Oncol Rep. (2017) 37:1168–74. doi: 10.3892/or.2016.5313
25. Ban TA. Fifty years chlorpromazine: a historical perspective. Neuropsychiatr Dis Treat. (2007) 3:495–500. Available online at: https://www.dovepress.com/fifty-years-chlorpromazine-a-historical-perspective-peer-reviewed-article-NDT
26. Shin SY, Kim CG, Kim SH, Kim YS, Lim Y, Lee YH. Chlorpromazine activates p21Waf1/Cip1 gene transcription via early growth response-1 (Egr-1) in C6 glioma cells. Exp Mol Med. (2010) 42:395–405. doi: 10.3858/emm.2010.42.5.041
27. Yde CW, Clausen MP, Bennetzen MV, Lykkesfeldt AE, Mouritsen OG, Guerra B. The antipsychotic drug chlorpromazine enhances the cytotoxic effect of tamoxifen in tamoxifen-sensitive and tamoxifen-resistant human breast cancer cells. Anticancer Drugs. (2009) 20:723–35. doi: 10.1097/CAD.0b013e32832ec041
28. Lee WY, Lee WT, Cheng CH, Chen KC, Chou CM, Chung CH, et al. Repositioning antipsychotic chlorpromazine for treating colorectal cancer by inhibiting sirtuin 1. Oncotarget. (2015) 6:27580–95. doi: 10.18632/oncotarget.4768
29. Choi J, Lee YJ, Yoon YJ, Kim CH, Park SJ, Kim SY, et al. Pimozide suppresses cancer cell migration and tumor metastasis through binding to ARPC2, a subunit of the Arp2/3 complex. Cancer Sci. (2019) 110:3788–801. doi: 10.1111/cas.14205
30. Ren Y, Tao J, Jiang Z, Guo D, Tang J. Pimozide suppresses colorectal cancer via inhibition of Wnt/beta-catenin signaling pathway. Life Sci. (2018) 209:267–73. doi: 10.1016/j.lfs.2018.08.027
31. Arimochi H, Morita K. Characterization of cytotoxic actions of tricyclic antidepressants on human HT29 colon carcinoma cells. Eur J Pharmacol. (2006) 541:17–23. doi: 10.1016/j.ejphar.2006.04.053
32. Kannen V, Hintzsche H, Zanette DL, Silva WA Jr, Garcia SB, Waaga-Gasser AM, et al. Antiproliferative effects of fluoxetine on colon cancer cells and in a colonic carcinogen mouse model. PLoS ONE. (2012) 7:e50043. doi: 10.1371/journal.pone.0050043
33. Alburquerque-Gonzalez B, Bernabe-Garcia M, Montoro-Garcia S, Bernabe-Garcia A, Rodrigues PC, Ruiz Sanz J, et al. New role of the antidepressant imipramine as a Fascin1 inhibitor in colorectal cancer cells. Exp Mol Med. (2020) 52:281–92. doi: 10.1038/s12276-020-0389-x
34. Jang WJ, Jung SK, Vo TTL, Jeong CH. Anticancer activity of paroxetine in human colon cancer cells: involvement of MET and ERBB3. J Cell Mol Med. (2019) 23:1106–15. doi: 10.1111/jcmm.14011
35. Iskar M, Bork P, van Noort V. Discovery and validation of the antimetastatic activity of citalopram in colorectal cancer. Mol Cell Oncol. (2015) 2:e975080. doi: 10.4161/23723556.2014.975080
36. Gil-Ad I, Zolokov A, Lomnitski L, Taler M, Bar M, Luria D, et al. Evaluation of the potential anti-cancer activity of the antidepressant sertraline in human colon cancer cell lines and in colorectal cancer-xenografted mice. Int J Oncol. (2008) 33:277–86. doi: 10.3892/ijo_00000007
37. Li Y, McGreal S, Zhao J, Huang R, Zhou Y, Zhong H, et al. A cell-based quantitative high-throughput image screening identified novel autophagy modulators. Pharmacol Res. (2016) 110:35–49. doi: 10.1016/j.phrs.2016.05.004
38. Chan HL, Chiu WC, Chen VC, Huang KY, Wang TN, Lee Y, et al. SSRIs associated with decreased risk of hepatocellular carcinoma: a population-based case-control study. Psychooncology. (2018) 27:187–92. doi: 10.1002/pon.4493
39. Kuwahara J, Yamada T, Egashira N, Ueda M, Zukeyama N, Ushio S, et al. Comparison of the anti-tumor effects of selective serotonin reuptake inhibitors as well as serotonin and norepinephrine reuptake inhibitors in human hepatocellular carcinoma cells. Biol Pharm Bull. (2015) 38:1410–4. doi: 10.1248/bpb.b15-00128
40. Yang DK, Kim SJ. Desipramine induces apoptosis in hepatocellular carcinoma cells. Oncol Rep. (2017) 38:1029–34. doi: 10.3892/or.2017.5723
41. Elham A, Aziz E, Hossein B, Alireza MN. Anti-cancer effects of citalopram on hepatocellular carcinoma cells occur via cytochrome C release and the activation of NF-kB. Anticancer Agents Med Chem. (2017) 17:1570–7. doi: 10.2174/1871520617666170327155930
42. Chen VC, Chan HL, Hsu TC, Lu ML, Lee YC, Lee Y, et al. New use for old drugs: the protective effect of atypical antipsychotics on hepatocellular carcinoma. Int J Cancer. (2019) 144:2428–39. doi: 10.1002/ijc.31980
43. Chen JJ, Cai N, Chen GZ, Jia CC, Qiu DB, Du C, et al. The neuroleptic drug pimozide inhibits stem-like cell maintenance and tumorigenicity in hepatocellular carcinoma. Oncotarget. (2017) 8:17593–609. doi: 10.18632/oncotarget.4307
44. Fako V, Yu Z, Henrich CJ, Ransom T, Budhu AS, Wang XW. Inhibition of wnt/β-catenin signaling in hepatocellular carcinoma by an antipsychotic drug pimozide. Int J Biol Sci. (2016) 12:768–75. doi: 10.7150/ijbs.14718
45. Croom KF, Perry CM, Plosker GL. Mirtazapine: a review of its use in major depression and other psychiatric disorders. CNS Drugs. (2009) 23:427–52. doi: 10.2165/00023210-200923050-00006
46. Jiang S-M, Wu J-H, Jia L. Intervention of mirtazapine on gemcitabine-induced mild cachexia in nude mice with pancreatic carcinoma xenografts. World J Gastroenterol. (2012) 18:2867–71. doi: 10.3748/wjg.v18.i22.2867
47. Sanomachi T, Suzuki S, Kuramoto K, Takeda H, Sakaki H, Togashi K, et al. Olanzapine, an atypical antipsychotic, inhibits survivin expression and sensitizes cancer cells to chemotherapeutic agents. Anticancer Res. (2017) 37:6177–88. doi: 10.21873/anticanres.12067
48. Li H, Zhang Z, Gao C, Wu S, Duan Q, Wu H, et al. Combination chemotherapy of valproic acid (VPA) and gemcitabine regulates STAT3/Bmi1 pathway to differentially potentiate the motility of pancreatic cancer cells. Cell Biosci. (2019) 9:50. doi: 10.1186/s13578-019-0312-0
49. Saponara E, Visentin M, Baschieri F, Seleznik G, Martinelli P, Esposito I, et al. Serotonin uptake is required for Rac1 activation in Kras-induced acinar-to-ductal metaplasia in the pancreas. J Pathol. (2018) 246:352–65. doi: 10.1002/path.5147
50. Jones J, Bentas W, Blaheta RA, Makarevic J, Hudak L, Wedel S, et al. Modulation of adhesion and growth of colon and pancreatic cancer cells by the histone deacetylase inhibitor valproic acid. Int J Mol Med. (2008) 22:293–9. doi: 10.3892/ijmm_00000022
51. Lin T, Ren Q, Zuo W, Jia R, Xie L, Lin R, et al. Valproic acid exhibits anti-tumor activity selectively against EGFR/ErbB2/ErbB3-coexpressing pancreatic cancer via induction of ErbB family members-targeting microRNAs. J Exp Clin Cancer Res. (2019) 38:150. doi: 10.1186/s13046-019-1160-9
52. Shi P, Yin T, Zhou F, Cui P, Gou S, Wang C. Valproic acid sensitizes pancreatic cancer cells to natural killer cell-mediated lysis by upregulating MICA and MICB via the PI3K/Akt signaling pathway. BMC Cancer. (2014) 14:370. doi: 10.1186/1471-2407-14-370
53. Li H, Zhang Z, Gao C, Wu S, Duan Q, Wu H, et al. Combination chemotherapy of valproic acid (VPA) and gemcitabine regulates STAT3/Bmi1 pathway to differentially potentiate the motility of pancreatic cancer cells. Cell Biosci. (2019) 9:50. doi: 10.1186/s13578-019-0312-0
54. Sugimoto K, Shimada M, Utsunomiya T, Morine Y, Imura S, Ikemoto T, et al. Valproic acid enhances the anti-tumor effect of pegylated interferon-alpha towards pancreatic cancer cell lines. Anticancer Res. (2014) 34:3403–9. Available online at: http://ar.iiarjournals.org/content/34/7/3403.long
55. Dandawate P, Kaushik G, Ghosh C, Standing D, Ali Sayed AA, Choudhury S, et al. Diphenylbutylpiperidine antipsychotic drugs inhibit prolactin receptor signaling to reduce growth of pancreatic ductal adenocarcinoma in mice. Gastroenterology. (2020) 158:1433–49.e27. doi: 10.1053/j.gastro.2019.11.279
56. Ranjan A, Srivastava SK. Penfluridol suppresses pancreatic tumor growth by autophagy-mediated apoptosis. Sci Rep. (2016) 6:26165. doi: 10.1038/srep26165
57. Bruera E, Neumann CM. The uses of psychotropics in symptom management in advanced cancer. Psycho Oncol. (1998) 7:346–58.
58. Messiha FS. Fluoxetine: adverse effects and drug-drug interactions. J Toxicol Clin Toxicol. (1993) 31:603–30. doi: 10.3109/15563659309025765
59. Brambilla P, Cipriani A, Hotopf M, Barbui C. Side-effect profile of fluoxetine in comparison with other SSRIs, tricyclic and newer antidepressants: a meta-analysis of clinical trial data. Pharmacopsychiatry. (2005) 38:69–77. doi: 10.1055/s-2005-837806
60. Holland JC, Romano SJ, Heiligenstein JH, Tepner RG, Wilson MG. A controlled trial of fluoxetine and desipramine in depressed women with advanced cancer. Psycho Oncol. (1998) 7:291–300.
61. Valentine AD. Cancer pain and depression: management of the dual-diagnosed patient. Curr Pain Headache Rep. (2003) 7:262–9. doi: 10.1007/s11916-003-0046-9
62. Kautio AL, Haanpää M, Leminen A, Kalso E, Kautiainen H, Saarto T. Amitriptyline in the prevention of chemotherapy-induced neuropathic symptoms. Anticancer Res. (2009) 29:2601–6. Available online at: http://ar.iiarjournals.org/content/29/7/2601.long
63. Ryan QC, Headlee D, Acharya M, Sparreboom A, Trepel JB, Ye J, et al. Phase I and pharmacokinetic study of MS-275, a histone deacetylase inhibitor, in patients with advanced and refractory solid tumors or lymphoma. J Clin Oncol. (2005) 23:3912–22. doi: 10.1200/JCO.2005.02.188
64. Koch K, Mansi K, Haynes E, Adams CE, Sampson S, Furtado VA. Trifluoperazine versus placebo for schizophrenia. Cochrane Database Syst Rev. (2014) 2014:CD010226. doi: 10.1002/14651858.CD010226.pub2
66. Chouinard G, Boisvert D, Bradwejn J. Tardive dyskinesia in a nonpsychiatric patient due to short-term use of a neuroleptic/anticholinergic combination drug. Can Med Assoc J. (1982) 126:821–2.
67. Hussain MZ, Harinath M, Murphy J. Tranquillizer-induced galactorrhea. Can Med Assoc J. (1972) 106:1107–8.
68. Glaser EM, Newling PS. Side effects of chlorpromazine hydrochloride. Br J Pharmacol Chemother. (1955) 10:429–33. doi: 10.1111/j.1476-5381.1955.tb00098.x
69. Thanacoody RH. Thioridazine: the good and the bad. Recent Patents Anti Infect Drug Discov. (2011) 6:92–8. doi: 10.2174/157489111796064588
70. Theobald DE, Kirsh KL, Holtsclaw E, Donaghy K, Passik SD. An open-label, crossover trial of mirtazapine (15 and 30 mg) in cancer patients with pain and other distressing symptoms. J Pain Symptom Manag. (2002) 23:442–7. doi: 10.1016/S0885-3924(02)00381-0
Keywords: psychiatric drugs, cancer, esophageal, gastric, colorectal, hepatic, pancreatic
Citation: Avendaño-Félix M, Aguilar-Medina M, Bermudez M, Lizárraga-Verdugo E, López-Camarillo C and Ramos-Payán R (2020) Refocusing the Use of Psychiatric Drugs for Treatment of Gastrointestinal Cancers. Front. Oncol. 10:1452. doi: 10.3389/fonc.2020.01452
Received: 22 May 2020; Accepted: 08 July 2020;
Published: 14 August 2020.
Edited by:
Teresita Padilla-Benavides, Wesleyan University, United StatesReviewed by:
Marco Cordani, IMDEA Nanociencia, SpainIsaac Cervantes Sandoval, Georgetown University, United States
Copyright © 2020 Avendaño-Félix, Aguilar-Medina, Bermudez, Lizárraga-Verdugo, López-Camarillo and Ramos-Payán. This is an open-access article distributed under the terms of the Creative Commons Attribution License (CC BY). The use, distribution or reproduction in other forums is permitted, provided the original author(s) and the copyright owner(s) are credited and that the original publication in this journal is cited, in accordance with accepted academic practice. No use, distribution or reproduction is permitted which does not comply with these terms.
*Correspondence: Rosalío Ramos-Payán, cm9zYWxpb3JwJiN4MDAwNDA7dWFzLmVkdS5teA==