- 1Department of Gastroenterology, Peking University People's Hospital, Beijing, China
- 2Clinical Center of Immune-Mediated Digestive Diseases, Peking University People's Hospital, Beijing, China
- 3Institute of Clinical Molecular Biology & Central Laboratory, Peking University People's Hospital, Beijing, China
Background: Although increasing evidences showed a correlation between cholecystectomy and the prevalence rate of colorectal cancer (CRC), and shed light on gut microbiota in colorectal pathogenesis, only a few studies focused on microbial alterations after cholecystectomy, and its sequent role in carcinogenesis and progression of CRC has not been reported. Thus, we aimed to investigate the bacterial alterations and tried to clarify their clinical significance.
Methods: 104 subjects were enrolled and divided into post-cholecystectomy patients (PC, n = 52) and healthy controls (HC, n = 52). To investigate the bacterial role in carcinogenesis, PC patients were further separated into preCA_CRC (patients with precancerous lesions and/or CRC, n = 9) and non-CA (patients without precancerous lesions and CRC, n = 43) based on the histopathology. Qualified stool samples were collected for 16S rRNA gene sequencing to analyze the bacterial profile.
Results: Our data showed noteworthy compositional and abundant alterations of bacterial microbiota in PC patients, characterized as Bacteroides ovatus, Prevotella copri, and Fusobacterium varium remarkably increased; Faecalibacterium prausnitzii, Roseburia faecis, and Bifidobacterium adolescentis significantly decreased. Additionally, the duration after cholecystectomy was the critical factor that affected bacterial composition. Machine learning-based analysis showed a pivotal role of Megamonas funiformis in discriminating PC from HC subjects and involving in the progression of CRC.
Conclusions: The bacterial dysbiosis may associate with CRC in PC patients, and the duration after cholecystectomy was highlighted as an important factor. Altered bacterial microbiota was likely to play a pivotal role in related-disease in the long-term follow-up of PC patients.
Introduction
With the changes of the modern lifestyle, the prevalence of cholelithiasis has steadily increased (1). Cholecystectomy is the gold standard for the treatment of symptomatic cholelithiasis (2), and the number of this surgery has also increased significantly. According to the information statistics, there are about 800,000 cases of cholecystectomy in the United States each year, and the number is also increasing in China annually (3, 4).
Cholecystectomy was considered nearly harmless in the past. However, growing evidences have shown that the prevalence of post-cholecystectomy syndrome (such as abdominal distention and abdominal pain) has increased in recent years, up to 10–47% (5). Meanwhile, it was reported that diabetic patients after cholecystectomy showed a slight deterioration in postprandial glycemic control in a clinical trial (6). Two large population-based studies suggested that cholecystectomy was likely to be one of the independent risk factors for non-alcoholic fatty liver disease (NAFLD) (7, 8). It was inferred that cholecystectomy might increase the risk of metabolic syndrome. In addition, some meta-analyses suggested that cholecystectomy probably raised the prevalence rate of colorectal cancer (CRC), particularly in the risk of right colon cancer (9–12).
Previous evidence showed that gut microbiome played crucial roles in colorectal carcinogenesis (13). Otherwise, a similar effect has not been reported in post-cholecystectomy (PC) patients complicated with CRC yet. There was a complex crosstalk between BAs and gut microbiome, BAs can modulate gut microbial composition (14–16), and BAs are stored in the gallbladder and metabolized by the gut microbiota. Therefore, we speculated that cholecystectomy likely had a huge impact on intestinal microbial homeostasis and facilitated CRC carcinogenesis and progression.
During the past decade, there were a few studies about gut microbiota after cholecystectomy. Keren et al. reported that cholecystectomy significantly affected the bacterial composition of cholelithiasis patients, with a remarkable increase in the abundance of Bacteroidetes (17). A study in China found that gut bacterial composition in healthy people changed with age, but the change was lost in PC patients, and the abundance of Bacteroidetes decreased in PC patients (18). Additionally, another study which contained 27 PC patients showed a subtle difference in the diversity of gut microbiota between the cholecystectomy and control groups (19). Nevertheless, these previous results were controversial, and the characterization in gut microbiota after cholecystectomy is still unclear. In addition, these studies analyzed at the genus level, ignored environmental factors and related diseases, and the character of bacterial alterations has not been further studied. Therefore, we intended to explore the alterations and roles of bacterial microbiota after cholecystectomy, and tried to clarify the clinical significance of the alterations.
Materials and Methods
Study Design and Sample Collection
Because there were few studies on the bacterial microbiota after cholecystectomy, and some existing studies had contradictory results on the abundance changes of the same genus of bacteria. In the early stage, we performed a pretest study for sample size evaluation, and considered the 10% loss of follow-up rate, therefore n was equal to 52 in each group.
Then a total of 52 PC patients were enrolled after cholecystectomy above 6 months and <25 years in Peking University People's Hospital from January 2018 to October 2018. Meanwhile, 52 healthy controls (HC) without any biliary diseases, tumors and traumatic ruptures were selected from health physical examination volunteers to match with PC patients. All involved subjects underwent colonoscopy within 6 months and were asked to avoid using probiotics and antibiotics at least 2 weeks before sampling. The demographic and basic clinical data of each group were recorded. Additionally, 9 of the PC patients had precancerous lesions and/or CRC, which confirmed by colonoscopy mucosal pathology. Firstly, we performed a 1:1 matched case-control group analysis. Subsequently, according to the absence and presence of precancerous lesions and/or CRC, we divided the PC patients into non-CA and preCA_CRC for subgroup analysis.
Fecal samples were collected in a Stool Collection Tube with Stool Stabilizer (German, Stratec Molecular) and then were separated and stored with ultra-hypothermia liquid nitrogen until microbial analysis.
DNA Extraction, 16S rRNA Gene Amplification and Sequencing
The total microbial genomic DNA was extracted from stool samples using the PSP® Spin Stool DNA Kit (German, Stratec Molecular). PCR amplification of 16S rRNA genes used barcoded primers specific to the V3–V4 variable region (357F, 806R) (20). Each PCR product was purified and amplified again to link with sample-specific barcodes. Sequencing was performed using the instrument secondary analysis of MiSeq Reporter software (MSR).
16S rDNA Sequence Analysis
The main software used for sequence analysis were Vsearch v2.8.1 (21) and Usearch v10 (bit 32) (22). The original data were merged by double-ended sequences by Vsearch, followed by data quality control, excision of primers and barcode, and the 164,772 sequence was removed, leaving 20,594,067 sequences. Then redundant sequences and sequences with <100 occurrences were removed by Vsearch. A total of 12,949,719 redundant sequences were removed and 9,012 high-quality sequences were obtained.
Exact sequence variants (ESVs) method was performed to filter chimeras (23), and 3,387 high-quality amplicons were obtained. Operational taxonomic units (OTUs) were aligned using the Vsearch and taxonomically classified using the reference sequence rdp_16s_v16_sp.fa. All specimens were sampled into the same amount of reads through Usearch V10, resulting in a total of 3,113,022 Reads and 3,179 OTUs. Among them, 0 OTUs appeared in all samples, 52 OTUs appeared in 90% of samples, and 1,637 OTUs appeared in 50% of samples.
Statistical Analysis and Data Visualization
R 3.4.1 software with the ggplot2 package was used for visualization. The categorical variables were described by the number of cases, using the chi-square test or Fisher's exact test. The continuous variables were described by the mean ± standard deviation (Mean ± SD). The Mann-Whitney U non-parametric test and Kruskal-Wallis H non-parametric test was used for comparison. Correlation analysis was performed using Spearman's test; the p-value was corrected with a false discovery rate (FDR) and only a significant correlation was visualized with the pheatmap package. And p ≤ 0.05 was considered to be statistically significant.
Results
Characterization of Enrolled Subjects
A total of 104 subjects enrolled in our study, and their characterizations were showed in Table 1. PC patients, who suffered from acute and chronic cholecystitis, cholelithiasis, gallbladder polyps, and traumatic gallbladder rupture, were recruited after cholecystectomy at a mean duration of 9.48 ± 8.02 years. No significant differences were found between the two groups in terms of gender, age, and BMI. In addition, we compared complications ratios, for example, NAFLD, HBP, T2DM, etc., and there were no remarkable differences in the two groups. So did in laboratory indexes (for example TBA, ALT, AST, etc.). The above data indicated that there were no markedly alterations of clinical characteristics in PC patients, which were in accordance with the previous studies (24).
Cholecystectomy Altered Community Diversity of Bacterial Microbiota
Firstly, we compared the alpha diversity of the two groups by Shannon index (Figure 1A) and Chao1 index (Figure 1B), which represented species diversity and richness, respectively. In our study, there was a higher chao1 index and a similar Shannon index in PC patients. Then, principal coordinate analysis (PCoA) of beta diversity showed separated clusterings in PC and HC groups (Adonis test, p = 9.999e-05, Figure 1C). These data indicated that the species richness of bacterial microbiota increased, and the composition quite altered in PC patients.
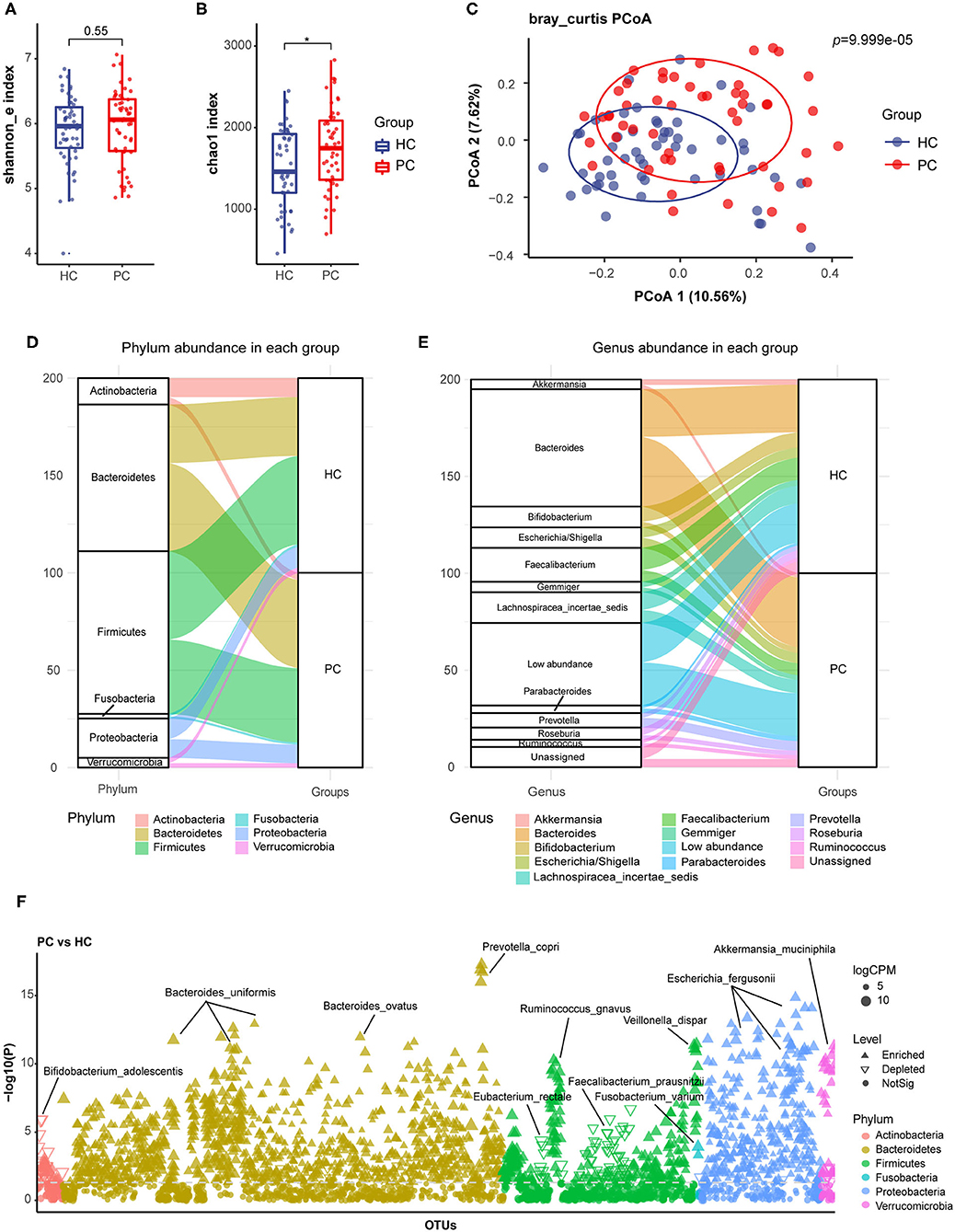
Figure 1. Alterations of fecal bacterial microbiota profile in PC patients. The alpha diversity of PC and HC group, (A), Shannon index; (B) Chao1 index. *p < 0.05. (C) Principal coordinate analysis (PCoA) of bacterial beta-diversity based on Bray Curtis distance. The Sankey diagram to visualize the bacterial composition at the phylum (D) and genus (E) level. (F) Bacterial species with abundance differentiation in PC group compared with HC group in the Manhattan diagram. Differences between two groups were shown as point shape indicated OTU enriched, depleted or not significant; point size indicated the abundance of OTU. FC, fold change; CPM, counts per million; HC, healthy controls; PC, post-cholecystectomy.
We further analyzed the bacterial composition in PC and HC groups, and delineated compositional structure which was mainly constituted by Bacteroidetes and Firmicutes at the phylum level (Figure 1D); Bacteroides, Bifidobacterium, Escherichia, and Faecalibacterium at the genus level (Figure 1E) of two groups. Additionally, the relative abundance of Bacteroides, Parabacteroides, and Prevotella increased; and the relative abundance of Faecalibacterium and Bifidobacterium decreased in PC patients at the genus level.
Bacterial Species With Abundance Differentiation in PC Patients vs. HC Subjects
By analysis and identification bacterial species with abundance differentiation in each group, we found that the abundance of Bacteroides ovatus (B. ovatus), Parabacteroides distasonis (P. distasonis), Prevotella copri (P. copri), and Fusobacterium varium (F. varium) remarkably increased; additionally, a significant reduction in the abundance of Faecalibacterium prausnitzii (F. prausnitzii), Roseburia faecis (R. faecis), Eubacterium rectale (E. rectale), and Bifidobacterium adolescentis (B. adolescentis) was observed in PC patients compared with HC subjects (Figure 1F).
B. ovatus and P. distasonis were reported to express bile salt hydrolase (BSH) and take part in bile acid metabolism (25–27). P. copri and F. varium were proved to promote inflammation (28, 29). However, F. prausnitzii and R. faecis were confirmed to participate in short-chain fatty acids (SCFAs) biosynthesis. B. adolescentis could secrete small molecule anti-inflammatory substances and inhibit inflammation (30). Eubacterium rectale could degrade starch in the human intestine and competitively inhibited the growth of harmful bacteria (31).
Based on these results, we inferred that fecal bacterial microbiota underwent a remarkable alteration after cholecystectomy, which was characterized as the accumulation of species with pro-inflammatory effects involved in bile acid metabolism, and the depletion of protective species with anti-inflammatory effects or producing SCFAs.
Group-Specific Microbiota in PC Patients Differentiated Disease Status
We next performed a machine learning analysis to confirm the group-specific microbiota, randomly selected half of the samples from each group to make up the model in the exploration step, and screened out the 20 most important species which contributed a lot to the disease classification to visualize (Figure 2A). According to the curve in Figure 2A, the prediction error rate going down to 4% when the top two species were enrolled in. Therefore, Megamonas funiformis (M. funiformis), and Lactobacillus mucosae (L. mucosae) contributed largely to the sample classification, and were selected as biomarkers of PC patients. Then, using biomarkers for further validated in the remaining subjects of each group, the prediction accuracy rate was 96.2% (Figure 2B). Taking the two biomarkers into the validation step (Figure 2C), the area under the curve (AUC) of L. mucosae was 0.62, and the AUC of M. funiformis was 0.85. Combined with the positive test rate in two groups, respectively (Figure 2D), we verified that M. funiformis and L. mucosae were characteristic species which could differentiate PC patients from HC subjects.
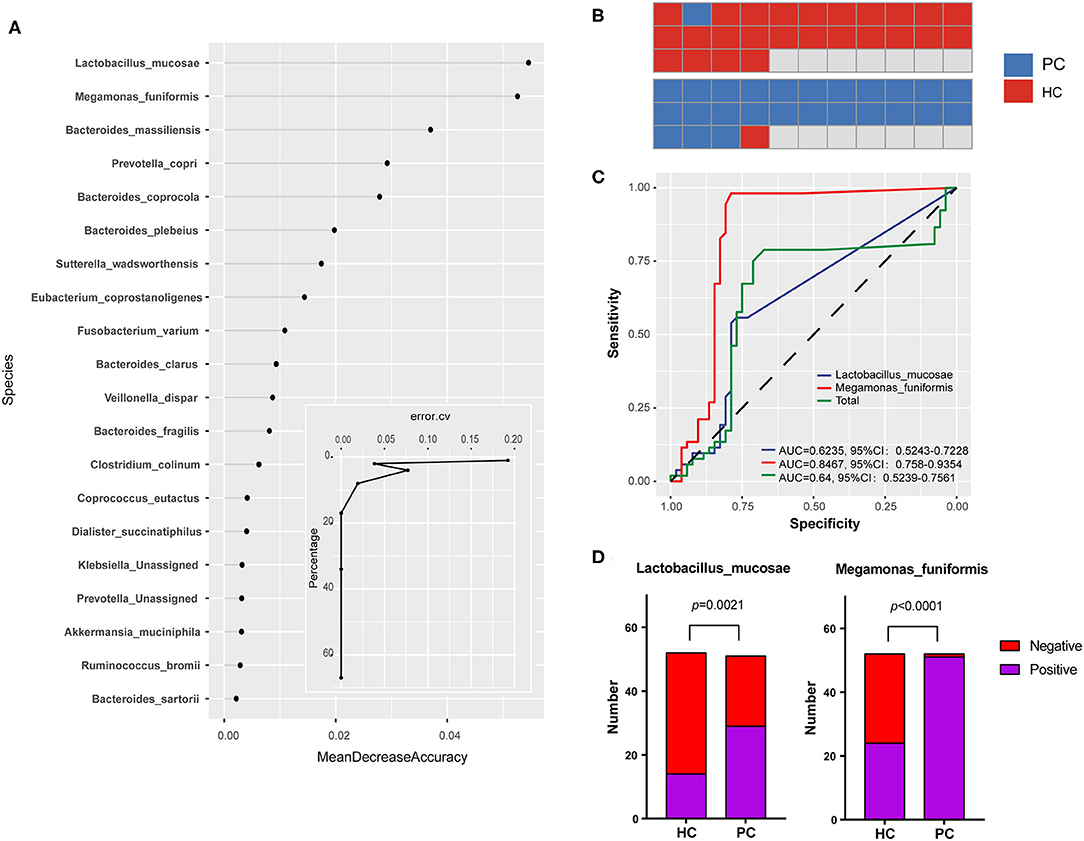
Figure 2. Group-specific microbiota in PC patients. (A) Bacterial contribution values to the disease classification at the species level and the curve of prediction error rate. Random Forest package was used for comparative analysis and took random seeds as 315. (B) The prediction accuracy rate of biomarkers in the validation step. (C) Receiver operating characteristic (ROC) curve for biomarkers in the validation step. (D) The comparison for positive test rates of biomarkers in PC and HC groups, respectively. HC, healthy controls; PC, post-cholecystectomy.
L. mucosae was a protective strain colonized on mucosa and also involved in bile acid metabolism (32–36). M. funiformis was classified into the genus Megamonas of the family Verimellaceae in the phylum of Firmicutes (37), and its function is still unclear at present. In addition, as PC biomarkers, the alterations and more characters of the two species need further studies.
Correlations Between Environmental Factors and Bacterial Composition
Taking nine clinical characteristics (such as TBA and ALT) and 10 environmental factors (such as BMI and the duration after cholecystectomy) into account, no correlation was observed between the model of overall clinical characteristics and the bacterial composition of all samples (p = 0.075, Figure 3A); whereas the model of overall environmental factors was significantly correlated with the bacterial composition (p = 0.046, Figure 3B). Furthermore, we examined every environmental factor and found that only the duration after cholecystectomy demonstrated the most pronounced correlation with bacterial composition (p = 0.002, Figure 3C). With the increase of the duration, bacterial dysbiosis after cholecystectomy was more obvious. These findings suggested that the long-term follow-up of PC patients should attract more attention.
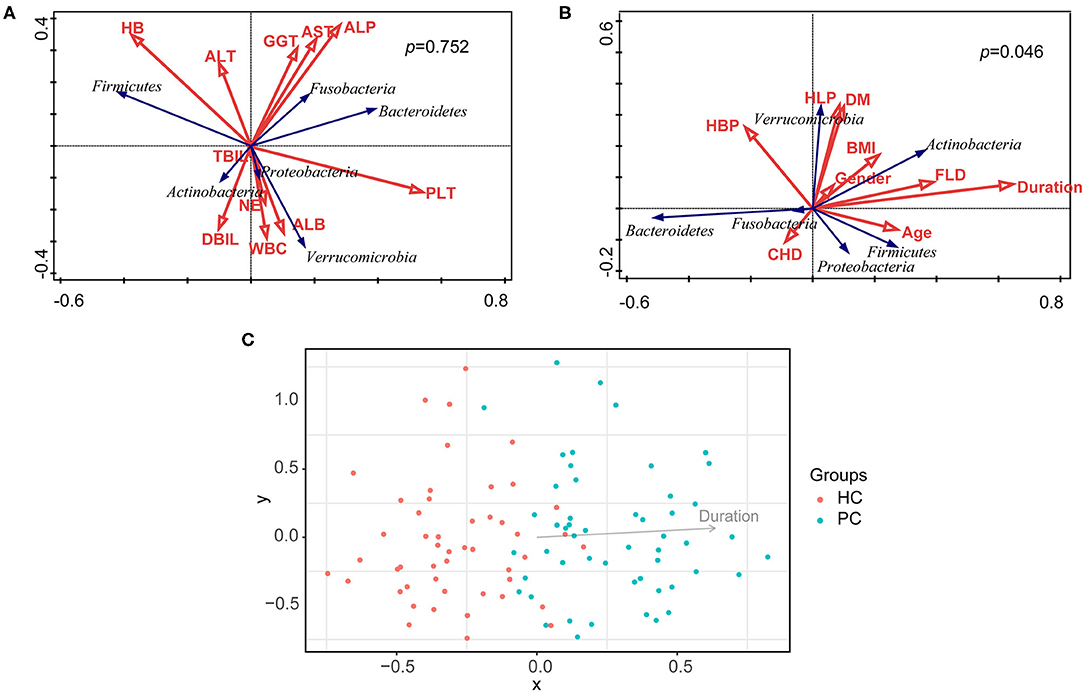
Figure 3. Correlation analysis of clinical characteristics, environmental factors, and bacterial composition. (A) Correlation analysis of clinical characteristics and bacterial composition of all samples. (B) Correlation analysis of environmental factors and bacterial composition of all samples. (C) Correlation analysis of the duration after cholecystectomy and bacterial composition of all samples. HC, healthy controls; PC, post-cholecystectomy.
Community Diversity and Bacterial Species With Abundance Differentiation in preCA_CRC vs. Non-CA Patients
Similarly, we compared the alpha diversity indexes of preCA_CRC and non-CA patients. No pronounced differences were found between the two groups but a lower tendency was seen in both Shannon and Chao1 indexes of preCA_CRC patients (Figures 4A,B). Analysis of beta diversity with PCoA showed parallel clusterings in two groups (Adonis test, p = 0.55, Figure 4C).
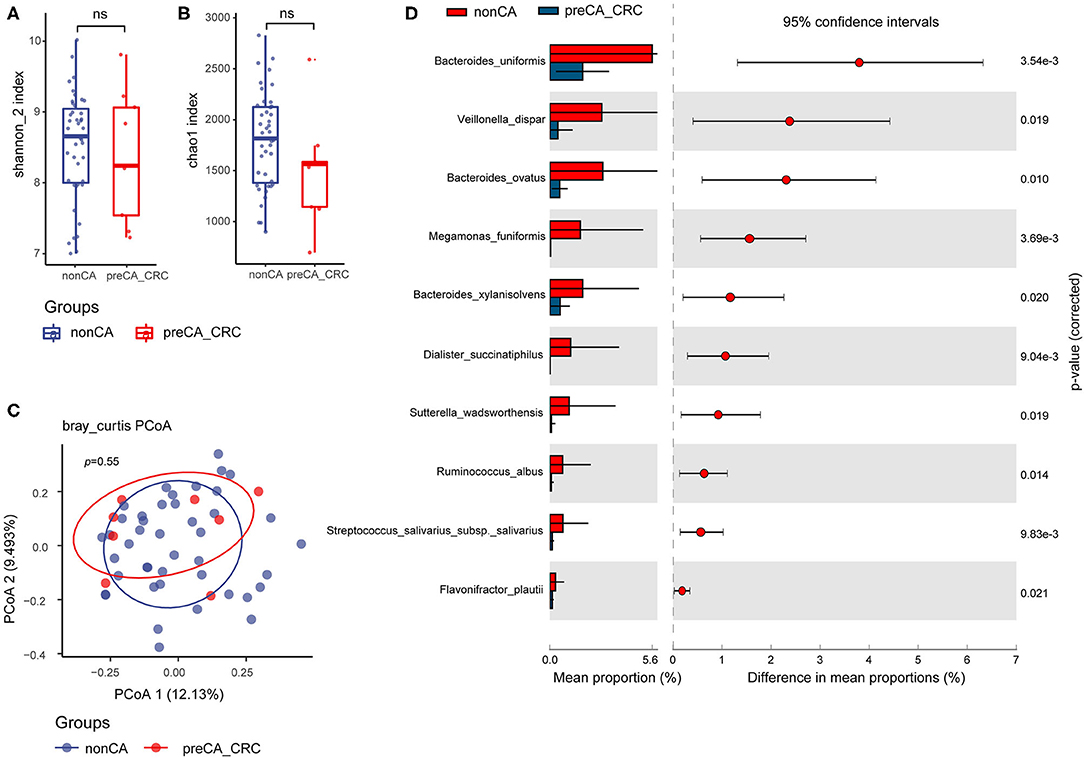
Figure 4. Compared with non-CA patients, alteration of fecal bacterial microbiota profile in preCA_CRC patients. The alpha diversity of preCA_CRC and non-CA patients, (A) Shannon index; (B) Chao1 index. ns, not significant. (C) PCoA of bacterial beta-diversity based on Bray Curtis distance. (D) Bacterial species with abundance differentiation in preCA_CRC patients compared with non-CA patients by STAMP. preCA_CRC, PC patients with precancerous lesions and/or CRC; non-CA, non-cancerous PC patients.
To identify differentially abundant taxa, we performed statistical analysis of taxonomic and functional profiles (STAMP), found that the abundance of bacterial microbiota was generally reduced in preCA_CRC patients. The abundance of some protective species, such as Sutterella wadsworthensis (anti-inflammation) (38) and Flavonifractor plautii (SCFAs biosynthesis) (39) further decreased in preCA_CRC patients, and the abundance of biomarker M. funiformis decreased as well (Figure 4D).
Correlations Between the Progression of CRC and Bacterial Microbiota
In this study, we set up a new scoring method for colonoscopic biopsy and applied it as a factor of the progression of CRC (Table 2). With the increase of score, the cancer progressed to a later stage. Taking the duration after cholecystectomy, results of colonoscopy, complicating with preCA_CRC and the score of colonoscopic biopsy as variables of the progression of CRC into account, we found that the abundance of M. funiformis, Veillonella dispar (V. dispar), and Bifidobacterium longum subsp. longum (B. longum subsp. longum) was significantly correlated with the progression of CRC after cholecystectomy (Figure 5A).
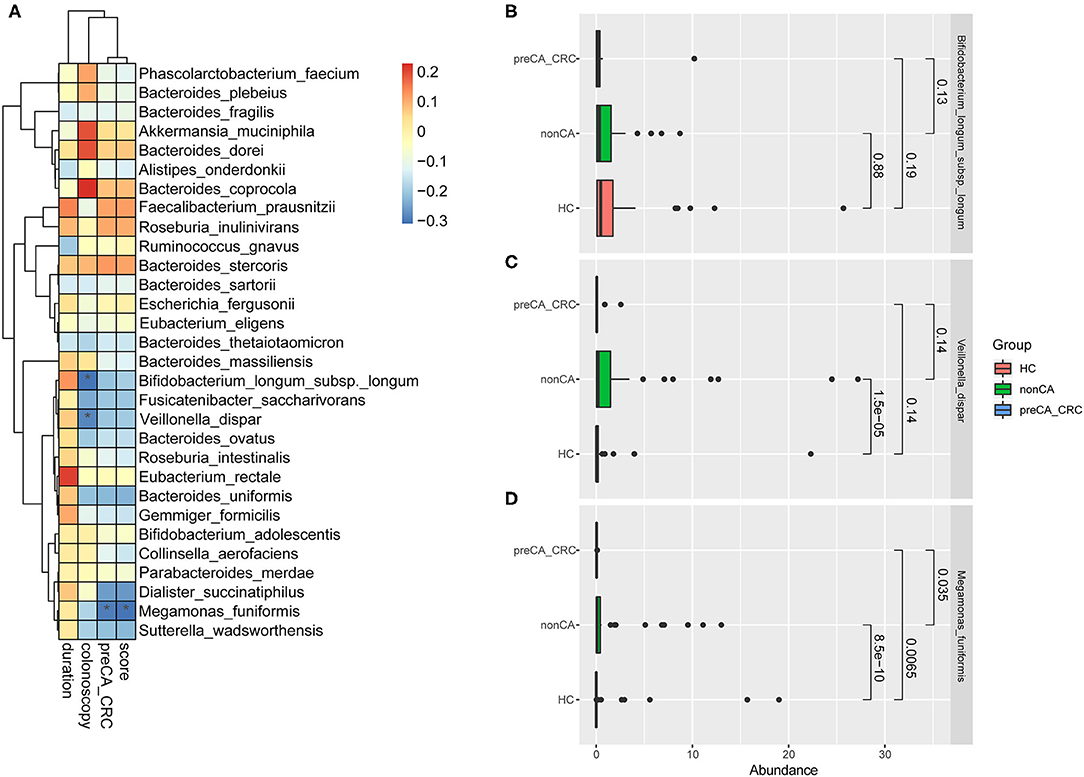
Figure 5. Correlation analysis of the progression of CRC and bacterial abundance. (A) Correlation analysis about bacterial microbiota and the progression of CRC based on duration after cholecystectomy, results of colonoscopy, complicating with preCA_CRC and the score of mucosal pathology. Spearman's correlation was performed. *p < 0.05. R 3.5.1 with the pheatmap package was used for data visualization. Abundance of (B) Bifidobacterium longum subsp. longum, (C) Veillonella dispar and (D) Megamonas funiformis in HC, non-CA and preCA_CRC groups, respectively. HC, healthy controls; non-CA, non-cancerous PC patients; preCA_CRC, PC patients with precancerous lesions and/or CRC.
Figures 5B–D detailed the abundant alterations of the above three species in HC, non-CA and preCA_CRC groups. The charts showed that the abundance of M. funiformis remarkably increased at first in non-CA group compared with healthy controls (p = 8.5e-10), and significantly decreased later in preCA_CRC group compared with non-CA group (p = 0.0065); additionally, V. dispar also had a semblable trend. In contrast, B. longum subsp. longum showed a continuous declination trend in abundance from HC to preCA_CRC group. Based on the above data, we inferred that bacterial dysbiosis might affected the progression of CRC after cholecystectomy.
Discussion
In the past, the impact of cholecystectomy on health was insufficiently understood. However, more patients experienced gastrointestinal symptoms such as dyspepsia and diarrhea, which were difficult to relieve after cholecystectomy. Meanwhile, several meta-analysis suggested that the prevalence rate of CRC was increased (9–12). It was proved that gut microbiota was involved in the pathogenesis of many diseases such as CRC (13), inflammatory bowel disease (IBD) and irritable bowel syndrome (IBS) (40). To fully realize the potential of cholecystectomy, we need to make further careful studies on the alterations of gut microbiota in PC patients, which may provide new ideas for the study of related-diseases after cholecystectomy and new treatment strategies for PC patients.
Herein, we depicted the overall structure of bacterial microbiota by 16S rRNA gene sequencing, demonstrated that bacterial dysbiosis after cholecystectomy was characterized by distinct microbial composition and altered relative abundances in species with specific functions. Based on the data, two biomarkers were screened out for distinguishing PC patients from HC subjects, and the duration after cholecystectomy mainly affected bacterial composition. Note that bacterial dysbiosis after cholecystectomy was likely associated with CRC in PC patients.
Previous studies found that the circadian excretion rhythm of BAs disappeared after cholecystectomy, along with increases of bile acid synthesis and bile acid enterohepatic recirculation rate (24, 41–43). In our study, the bacterial species involved in bile acid metabolism had quite high abundance in PC patients, and it was probably ascribed to the enriched BAs exposure. Butyrate was the main energy source for colon cells and had protective effects against CRC and IBD (44, 45). Furthermore, a reduced abundance of protective bacteria which participated in SCFAs biosynthesis including butyrate (46–48), such as F. prausnitzii and R. faecis, were observed in our study, and it was surmised that decreased production of SCFAs by microbiota may affect carcinogenesis of CRC. Other than the characters above-mentioned, PC biomarker L. mucosae was reported to be interfered with by surfactant protein D (SP-D), which was synthesized and secreted by gallbladder in mice (49). Whether there were similar bacteria-protein interactions in the human gallbladder still needed to be verified.
Bacterial compositional state was shaped by many environmental factors but also potentially self-reinforcing. Duration after cholecystectomy was observed as a vital factor which affected bacterial composition in PC patients, and with the increase of duration after cholecystectomy, the bacterial composition changed more obviously. The external influence might trigger a compositional shift which gradually formed an adapted bacterial composition, and then bacterial composition possibly fed back to the host in multifarious ways, for example, via the production of certain metabolites like bile acids (50).
To investigate the role of bacterial microbiota in the pathogenesis and progression of CRC, we further analyzed the bacterial differences between the two subgroups of PC patients. preCA_CRC patients were enrolled after cholecystectomy at a mean duration of 11.17 (±10.34) years, and the mean duration was 9.13 (±7.55) years in non-CA patients. There was no significant difference in the mean duration after cholecystectomy (t = −0.690, p = 0.493), but a higher tendency was seen in preCA_CRC patients. In addition, basic clinical data, such as gender, clinical comorbidities, and laboratory tests, were not significantly different between preCA_CRC and non-CA groups. Furthermore, 66.7% of the preCA_CRC patients (n = 6) had mucosal lesions located in the right colon (Table 3), and these findings supported some conclusions in previous meta-analyses (9–12). Low bacterial diversity and reduced abundance of protective bacteria (mainly producing SCFAs) were considered to be major types of gut dysbiosis of CRC (51, 52); and it was widely reported that Bacteroides fragilis, Fusobacterium nucleatum, and Prevotella intermedia had increased in abundance of sporadic CRC patients (53, 54). Our findings were slightly different from bacterial alterations of sporadic CRC patients, and the reasons may lie in the small samples of preCA_CRC patients. We found the accumulation of most Bacteroides species, Prevotella copri, and Fusobacterium varium after cholecystectomy, and these species were from the same genera with the characteristic species of sporadic CRC. Some studies reported that the dominant species of Bacteroides, Paraprevotella, Eubacterium in fecal samples of CRC patients were often different (53). Additionally, the abundance of M. funiformis, V. dispar, and B. longum subsp. longum was correlated with the progression of CRC, which might play a pivotal role in related-disease studies. Based on these data, we speculated that CRC patients deduced from PC patients might have a distinctive bacterial profile when compared to sporadic CRC patients.
Additionally, the guideline suggested that the general-risk population recommended will start screening colonoscopy at the age of 50 to find early CRC and precancerous lesions (55). According to previous studies and the results of the functional annotation of bacterial microbiota in our study, cholecystectomy may increase the risk of CRC in postoperative patients compared with healthy people. The age distribution during cholecystectomy is wide due to various causes such as acute cholecystitis, chronic cholecystitis, gallstones, and traumatic cholecyst rupture. Therefore, the results of our study suggested that regardless of age, patients after cholecystectomy were recommended to screen colonoscopy to detect early CRC and precancerous lesions.
Even their tentative conclusions were debatable, previous studies had partly reported the changes of bacterial microbiota after cholecystectomy by sequencing of fecal samples (17–19). Nevertheless, the impact of environmental factors on these bacterial alterations has not been analyzed, and the link between bacterial alterations and postoperative related-diseases has not received enough attention. The major advantages of our study contained subjects enrolled with colonoscopic biopsy, considerations of clinical characteristics and environmental factors, bacterial screening at the species level, and correlation analysis on bacterial alterations after cholecystectomy and CRC. However, our data provide evidence of association, not causality, and further studies are guaranteed to clarify how the disease-associated bacteria play a role in carcinogenesis and progression of CRC. The small sample size of preCA_CRC patients was indeed a limitation of our study. In the future, we plan to conduct a controlled study on patients with sporadic CRC and post-cholecystectomy patients with CRC to better explain the impact of cholecystectomy on the incidence of CRC.
In conclusion, our study showed specific bacterial alterations after cholecystectomy, and bacterial dysbiosis likely associated with carcinogenesis and progression of CRC in PC patients. Furthermore, duration after cholecystectomy notably affected bacterial composition in PC patients. Our findings provide novel insights into related-disease studies after cholecystectomy, and the long-term follow-up of PC patients should attract more attention. It reminds us of broad considerations when implementing cholecystectomy in clinic.
Data Availability Statement
The datasets presented in this study can be found in online repositories. The names of the repository/repositories and accession number(s) can be found below: https://www.ncbi.nlm.nih.gov/, PRJNA541484.
Ethics Statement
The studies involving human participants were reviewed and approved by the Institutional Medical Ethics Review Board of Peking University People's Hospital (Document No. 2018PHB035-01). The patients/participants provided their written informed consent to participate in this study.
Author Contributions
YL, XR, and JX designed this study. XR, YuZ, GC, YiZ, and QH performed sample and clinical information collection. XR and JX performed data analysis and visualization. XR performed manuscript writing. YL and JX revised this article. All authors contributed to the article and approved the submitted version.
Funding
This work was supported by the national key research and development program of China (2017YFC0908900), National Natural Science Foundation of China (81670499), and Beijing Municipal Science and Technology Project (Z171100000417022).
Conflict of Interest
The authors declare that the research was conducted in the absence of any commercial or financial relationships that could be construed as a potential conflict of interest.
Acknowledgments
The authors are grateful for all the subjects who participated in the study.
Abbreviations
ALP, alkaline phosphatase; ALT, alanine aminotransferase; AST, aspartate aminotransferase; AUC, area under the curve; BAs, bile acids; BMI, body mass index; BSH, bile salt hydrolase; CHD, coronary atherosclerotic heart disease; CRC, colorectal cancer; GGT, glutamyl transpeptidase; HB, hemoglobin; HBP, hypertension; HC, healthy control; HLP, hyperlipemia; IBD, inflammatory bowel disease; IBS, irritable bowel syndrome; NAFLD, non-alcoholic fatty liver disease; NE%, neutrophilic granulocyte percentage; OTU, Operational Taxonomic Unit; PC, post-cholecystectomy; PCoA, principal coordinate analysis; PLT, blood platelet count; ROC, receiver operating characteristic curve; SCFAs, short-chain fatty acids; SP-D, surfactant protein D; STAMP, statistical analysis of taxonomic and functional Profiles; T2DM, type 2 diabetes mellitus; TBA, total bile acid; WBC, white blood cell count.
References
1. Stokes CS, Krawczyk M, Lammert F. Gallstones: environment, lifestyle and genes. Dig Dis. (2011) 29:191–201. doi: 10.1159/000323885
2. European Association for the Study of the Liver (EASL). EASL Clinical Practice Guidelines on the prevention, diagnosis and treatment of gallstones. J Hepatol. (2016) 65:146–81. doi: 10.1016/j.jhep.2016.03.005
3. Brescia A, Gasparrini M, Nigri G, Cosenza UM, Dall'Oglio A, Pancaldi A, et al. Laparoscopic cholecystectomy in day surgery: feasibility and outcomes of the first 400 patients. Surgeon. (2013) 11(Suppl 1):S14–8. doi: 10.1016/j.surge.2012.09.006
4. Lamberts MP, Kievit W, Ozdemir C, Westert GP, van Laarhoven CJ, Drenth JP. Value of EGD in patients referred for cholecystectomy: a systematic review and meta-analysis. Gastrointest Endosc. (2015) 82:24–31. doi: 10.1016/j.gie.2015.01.024
5. Whitby J, Thomson A, Gananadha S. Mucocele of the gall bladder stump: a cause of post-cholecystectomy syndrome. ANZ J Surg. (2016) 86:725–6. doi: 10.1111/ans.12745
6. Sonne DP, Hare KJ, Martens P, Rehfeld JF, Holst JJ, Vilsboll T, et al. Postprandial gut hormone responses and glucose metabolism in cholecystectomized patients. Am J Physiol Gastrointest Liver Physiol. (2013) 304:G413–9. doi: 10.1152/ajpgi.00435.2012
7. Ruhl CE, Everhart JE. Relationship of non-alcoholic fatty liver disease with cholecystectomy in the US population. Am J Gastroenterol. (2013) 108:952–8. doi: 10.1038/ajg.2013.70
8. Kwak MS, Kim D, Chung GE, Kim W, Kim YJ, Yoon JH. Cholecystectomy is independently associated with nonalcoholic fatty liver disease in an Asian population. World J Gastroenterol. (2015) 21:6287–95. doi: 10.3748/wjg.v21.i20.6287
9. Schernhammer ES, Leitzmann MF, Michaud DS, Speizer FE, Giovannucci E, Colditz GA, et al. Cholecystectomy and the risk for developing colorectal cancer and distal colorectal adenomas. Br J Cancer. (2003) 88:79–83. doi: 10.1038/sj.bjc.6600661
10. Altieri A, Pelucchi C, Talamini R, Bosetti C, Franceschi S, La Vecchia C. Cholecystectomy and the risk of colorectal cancer in Italy. Br J Cancer. (2004) 90:1753–5. doi: 10.1038/sj.bjc.6601721
11. Shao T, Yang YX. Cholecystectomy and the risk of colorectal cancer. Am J Gastroenterol. (2005) 100:1813–20. doi: 10.1111/j.1572-0241.2005.41610.x
12. Zhang Y, Liu H, Li L, Ai M, Gong Z, He Y, et al. Cholecystectomy can increase the risk of colorectal cancer: A meta-analysis of 10 cohort studies. PLoS ONE. (2017) 12:e0181852. doi: 10.1371/journal.pone.0181852
13. Saus E, Iraola-Guzmán S, Willis JR, Brunet-Vega A, Gabaldón T. Microbiome and colorectal cancer: roles in carcinogenesis and clinical potential. Mol Aspects Med. (2019) 69:93–106. doi: 10.1016/j.mam.2019.05.001
14. De Boever P, Wouters R, Verschaeve L, Berckmans P, Schoeters G, Verstraete W. Protective effect of the bile salt hydrolase-active Lactobacillus reuteri against bile salt cytotoxicity. Appl Microbiol Biotechnol. (2000) 53:709–14. doi: 10.1007/s002530000330
15. Begley M, Gahan CG, Hill C. The interaction between bacteria and bile. FEMS Microbiol Rev. (2005) 29:625–51. doi: 10.1016/j.femsre.2004.09.003
16. Staley C, Weingarden AR, Khoruts A, Sadowsky MJ. Interaction of gut microbiota with bile acid metabolism and its influence on disease states. Appl Microbiol Biotechnol. (2017) 101:47–64. doi: 10.1007/s00253-016-8006-6
17. Keren N, Konikoff FM, Paitan Y, Gabay G, Reshef L, Naftali T, et al. Interactions between the intestinal microbiota and bile acids in gallstones patients. Environ Microbiol Rep. (2015) 7:874–80. doi: 10.1111/1758-2229.12319
18. Wang W, Wang J, Li J, Yan P, Jin Y, Zhang R, et al. Cholecystectomy damages aging-associated intestinal microbiota construction. Front Microbiol. (2018) 9:1402. doi: 10.3389/fmicb.2018.01402
19. Yoon WJ, Kim HN, Park E, Ryu S, Chang Y, Shin H, et al. The impact of cholecystectomy on the gut microbiota: a case-control study. J Clin Med. (2019) 8:79. doi: 10.3390/jcm8010079
20. Klindworth A, Pruesse E, Schweer T, Peplies J, Quast C, Horn M, et al. Evaluation of general 16S ribosomal RNA gene PCR primers for classical and next-generation sequencing-based diversity studies. Nucl Acids Res. (2013) 41:e1. doi: 10.1093/nar/gks808
21. Rognes T, Flouri T, Nichols B, Quince C, Mahe F. VSEARCH: a versatile open source tool for metagenomics. PeerJ. (2016) 4:e2584. doi: 10.7717/peerj.2584
22. Alloui T, Boussebough I, Chaoui A, Nouar AZ, Chettah MC. Usearch: a meta search engine based on a new result merging strategy. J South Central Coll Natl. (2015) 269–272. doi: 10.1109/ICIG.2013.57
23. Edgar R.C. (2016). UNOISE2: improved error-correction for Illumina 16S and ITS amplicon sequencing. bioRxiv 081257. doi: 10.1101/081257
24. Kullak-Ublick GA, Paumgartner G, Berr F. Long-term effects of cholecystectomy on bile acid metabolism. Hepatology. (1995) 21:41–5. doi: 10.1002/hep.1840210109
25. Ridlon JM, Kang DJ, Hylemon PB. Bile salt biotransformations by human intestinal bacteria. J Lipid Res. (2006) 47:241–59. doi: 10.1194/jlr.R500013-JLR200
26. Chiang JYL. Thematic review series: bile acids: bile acids: regulation of synthesis. J Lipid Res. (2009) 50:1955. doi: 10.1194/jlr.R900010-JLR200
27. Gerard P. Metabolism of cholesterol and bile acids by the gut microbiota. Pathogens. (2013) 3:14–24. doi: 10.3390/pathogens3010014
28. Allen-Vercoe E. Fusobacterium varium in ulcerative colitis: is it population-based? Dig Dis Sci. (2015) 60:7–8. doi: 10.1007/s10620-014-3390-1
29. Pianta A, Arvikar S, Strle K, Drouin EE, Wang Q, Costello CE, et al. Evidence of the immune relevance of prevotella copri, a gut microbe, in patients with rheumatoid arthritis. Arthritis Rheumatol. (2017) 69:964–75. doi: 10.1002/art.40003
30. Kawabata K, Baba N, Sakano T, Hamano Y, Taira S, Tamura A, et al. Functional properties of anti-inflammatory substances from quercetin-treated Bifidobacterium adolescentis. Biosci Biotechnol Biochem. (2018) 82:689–97. doi: 10.1080/09168451.2017.1401916
31. Cockburn DW, Orlovsky NI, Foley MH, Kwiatkowski KJ, Bahr CM, Maynard M, et al. Molecular details of a starch utilization pathway in the human gut symbiont Eubacterium rectale. Mol Microbiol. (2015) 95:209–30. doi: 10.1111/mmi.12859
32. Vizoso Pinto MG, Franz CM, Schillinger U, Holzapfel WH. Lactobacillus spp. with in vitro probiotic properties from human faeces and traditional fermented products. Int J Food Microbiol. (2006) 109:205–14. doi: 10.1016/j.ijfoodmicro.2006.01.029
33. Zago M, Fornasari ME, Carminati D, Burns P, Suarez V, Vinderola G, et al. Characterization and probiotic potential of Lactobacillus plantarum strains isolated from cheeses. Food Microbiol. (2011) 28:1033–40. doi: 10.1016/j.fm.2011.02.009
34. Munoz-Atienza E, Gomez-Sala B, Araujo C, Campanero C, del Campo R, Hernandez PE, et al. Antimicrobial activity, antibiotic susceptibility and virulence factors of Lactic Acid Bacteria of aquatic origin intended for use as probiotics in aquaculture. BMC Microbiol. (2013) 13:15. doi: 10.1186/1471-2180-13-15
35. Etzold S, Kober OI, Mackenzie DA, Tailford LE, Gunning AP, Walshaw J, et al. Structural basis for adaptation of lactobacilli to gastrointestinal mucus. Environ Microbiol. (2014) 16:888–903. doi: 10.1111/1462-2920.12377
36. London LEE, Price NPJ, Ryan P, Wang L, Auty MAE, Fitzgerald GF, et al. Characterization of a bovine isolate Lactobacillus mucosae? DPC 6426 which produces an exopolysaccharide composed predominantly of mannose residues. J Appl Microbiol. (2014) 117:509–17. doi: 10.1111/jam.12542
37. Sakon H, Nagai F, Morotomi M, Tanaka R. Sutterella parvirubra sp. nov. and Megamonas funiformis sp. nov., isolated from human faeces. Int J Syst Evol Microbiol. (2008) 58:970–5. doi: 10.1099/ijs.0.65456-0
38. Hiippala K, Kainulainen V, Kalliomaki M, Arkkila P, Satokari R. Mucosal prevalence and interactions with the epithelium indicate commensalism of Sutterella spp. Front Microbiol. (2016) 7:1706. doi: 10.3389/fmicb.2016.01706
39. Engels C, Ruscheweyh HJ, Beerenwinkel N, Lacroix C, Schwab C. The common gut microbe Eubacterium hallii also contributes to intestinal propionate formation. Front Microbiol. (2016) 7:713. doi: 10.3389/fmicb.2016.00713
40. Vich Vila A, Imhann F, Collij V, Jankipersadsing SA, Gurry T, Mujagic Z, et al. Gut microbiota composition and functional changes in inflammatory bowel disease and irritable bowel syndrome. Sci Transl Med. (2018) 10:eaap8914. doi: 10.1126/scitranslmed.aap8914
41. Roda E, Aldini R, Mazzella G, Roda A, Sama C, Festi D, et al. Enterohepatic circulation of bile acids after cholecystectomy. Gut. (1978) 19:640–9. doi: 10.1136/gut.19.7.640
42. Amigo L, Husche C, Zanlungo S, Lutjohann D, Arrese M, Miquel JF, et al. Cholecystectomy increases hepatic triglyceride content and very-low-density lipoproteins production in mice. Liver Int. (2011) 31:52–64. doi: 10.1111/j.1478-3231.2010.02361.x
43. Barrera F, Azócar L, Molina H, Schalper KA, Ocares M, Liberona J, et al. Effect of cholecystectomy on bile acid synthesis and circulating levels of fibroblast growth factor. Ann Hepatol. (2015) 14:710. doi: 10.1016/S1665-2681(19)30766-5
44. Christl SU, Eisner HD, Dusel G, Kasper H, Scheppach W. Antagonistic effects of sulfide and butyrate on proliferation of colonic mucosa: a potential role for these agents in the pathogenesis of ulcerative colitis. J Digest Dis Sci. (1996) 41:2477–81. doi: 10.1007/BF02100146
45. Archer S, Meng S, Wu J, Johnson J, Tang R, Hodin R. Butyrate inhibits colon carcinoma cell growth through two distinct pathways. J Surg. (1998) 124:248–53. doi: 10.1016/S0039-6060(98)70127-8
46. Barcenilla A, Pryde SE, Martin JC, Duncan SH, Stewart CS, Henderson C, et al. Phylogenetic relationships of butyrate-producing bacteria from the human gut. Appl Environ Microbiol. (2000) 66:1654–61. doi: 10.1128/AEM.66.4.1654-1661.2000
47. Duncan SH, Hold GL, Harmsen HJ, Stewart CS, Flint HJ. Growth requirements and fermentation products of Fusobacterium prausnitzii, and a proposal to reclassify it as Faecalibacterium prausnitzii gen. nov., comb. nov. J Int J Syst Evol Microbiol. (2002) 52:2141–6. doi: 10.1099/00207713-52-6-2141
48. Tamanai-Shacoori Z, Smida I, Bousarghin L, Loreal O, Meuric V, Fong SB, et al. Roseburia spp.: a marker of health? Future Microbiol. (2017) 12:157–70. doi: 10.2217/fmb-2016-0130
49. Sarashina-Kida H, Negishi H, Nishio J, Suda W, Nakajima Y, Yasui-Kato M, et al. Gallbladder-derived surfactant protein D regulates gut commensal bacteria for maintaining intestinal homeostasis. Proc Natl Acad Sci USA. (2017) 114:10178–83. doi: 10.1073/pnas.1712837114
50. Wahlstrom A, Sayin SI, Marschall HU, Backhed F. Intestinal crosstalk between bile acids and microbiota and its impact on host metabolism. Cell Metab. (2016) 24:41–50. doi: 10.1016/j.cmet.2016.05.005
51. Ahn J, Sinha R, Pei Z, Dominianni C, Wu J, Shi J, et al. Human gut microbiome and risk for colorectal cancer. J Natl Cancer Inst. (2013) 105:1907–11. doi: 10.1093/jnci/djt300
52. Tilg H, Adolph TE, Gerner RR, Moschen AR. The intestinal microbiota in colorectal cancer. Cancer Cell. (2018) 33:954–64. doi: 10.1016/j.ccell.2018.03.004
53. Chen W, Liu F, Ling Z, Tong X, Xiang C. Human intestinal lumen and mucosa-associated microbiota in patients with colorectal cancer. PLoS ONE. (2012) 7:e39743. doi: 10.1371/journal.pone.0039743
54. Viljoen KS, Dakshinamurthy A, Goldberg P, Blackburn JM. Quantitative profiling of colorectal cancer-associated bacteria reveals associations between Fusobacterium spp., enterotoxigenic Bacteroides fragilis (ETBF) and clinicopathological features of colorectal cancer. PLoS ONE. (2015) 10:e0119462. doi: 10.1371/journal.pone.0119462
Keywords: cholecystectomy, the duration after cholecystectomy, 16S rRNA gene sequencing, bacterial alterations, colorectal cancer
Citation: Ren X, Xu J, Zhang Y, Chen G, Zhang Y, Huang Q and Liu Y (2020) Bacterial Alterations in Post-Cholecystectomy Patients Are Associated With Colorectal Cancer. Front. Oncol. 10:1418. doi: 10.3389/fonc.2020.01418
Received: 15 April 2020; Accepted: 06 July 2020;
Published: 12 August 2020.
Edited by:
Tuanjie Li, Georgetown University, United StatesReviewed by:
Liming Wang, Saitama Medical University, JapanJingping Zhang, Cornell University, United States
Copyright © 2020 Ren, Xu, Zhang, Chen, Zhang, Huang and Liu. This is an open-access article distributed under the terms of the Creative Commons Attribution License (CC BY). The use, distribution or reproduction in other forums is permitted, provided the original author(s) and the copyright owner(s) are credited and that the original publication in this journal is cited, in accordance with accepted academic practice. No use, distribution or reproduction is permitted which does not comply with these terms.
*Correspondence: Yulan Liu, liuyulan@pkuph.edu.cn
†These authors have contributed equally to this work