- 1Department of Clinical Microbiology and Immunology, Sackler Faculty of Medicine, Tel Aviv University, Tel Aviv-Yafo, Israel
- 2Alpha Tau Medical, Tel Aviv-Yafo, Israel
- 3Sackler Faculty of Exact Sciences, School of Physics and Astronomy, Tel Aviv University, Tel Aviv-Yafo, Israel
- 4Department of Otolaryngology, Head and Neck Surgery, Sheba Medical Center, Tel HaShomer, Israel
- 5Sackler Cellular and Molecular Imaging Center, Sackler Faculty of Medicine, Tel Aviv University, Tel Aviv-Yafo, Israel
- 6MedAustron, Wiener Neustadt, Austria
- 7Medical Faculty, Christian-Albrechts University, Kiel, Germany
Diffusing alpha-emitting radiation therapy (DaRT) employs intratumoral Ra-224-coated seeds that efficiently destroy solid tumors by slowly releasing alpha-emitting atoms inside the tumor. In immunogenic tumor models, DaRT was shown to activate systemic antitumor immunity. Agonists of the membrane-bound toll-like receptors (TLRs) enhanced these effects and led to tumor rejection. Here, we examined the combination of DaRT with agents that activate a different type of pattern recognition receptors, the cytoplasmatic RIG1-like receptors (RLRs). In response to cytoplasmatic viral dsRNA, RLRs activate an antiviral immune response that includes the elevation of antigen presentation. Thus, it was postulated that in low-immunogenic tumor models, RLR activation in tumor cells prior to the induction of their death by DaRT will be superior compared to TLR activation. Intratumoral cytoplasmatic delivery of the dsRNA mimic polyIC by polyethylenimine (PEI), was used to activate RLR, while polyIC without PEI was used to activate TLR. PolyIC(PEI) prior to DaRT synergistically retarded 4T1 triple-negative breast tumors and metastasis development more efficiently than polyIC and rejected panc02 pancreatic tumors in some of the treated mice. Splenocytes from treated mice, adoptively transferred to naive mice in combination with 4T1 tumor cells, delayed tumor development compared to naïve splenocytes. Low-dose cyclophosphamide, known to reduce T regulatory cell number, enhanced the effect of DaRT and polyIC(PEI) and led to high long-term survival rates under neoadjuvant settings, which confirmed metastasis clearance. The epigenetic drug decitabine, known to activate RLR in low doses, was given intraperitoneally prior to DaRT and caused tumor growth retardation, similar to local polyIC(PEI). The systemic and/or local administration of RLR activators was also tested in the squamous cell carcinoma (SCC) tumor model SQ2, in which a delay in tumor re-challenge development was demonstrated. We conclude that RIG-I-like activation prior to intratumoral alpha radiation may serve as a potent combination technique to reduce both tumor growth and the spread of distant metastases in low-immunogenic and metastatic tumor models.
Introduction
The destruction of the live tumor inside the host (namely, tumor ablation) releases tumor antigens to the tumor microenvironment and stimulates the activation of systemic and specific antitumor immune responses. Accordingly, tumor ablation can be considered as a form of “in situ vaccination” against tumor cells (1–4). Consequently, tumor ablation treatments may achieve two important goals simultaneously: [1] the destruction of the primary tumor and [2] the activation of antitumor immune responses against residual and distant tumor cells. This contrasts with surgical tumor resection, which achieves the first goal yet may suppress the second (5–7).
A unique radiotherapeutic tumor ablation technique utilizing the diffusion of alpha emitting atoms inside the tumor (diffusing alpha-emitters radiation therapy, referred to as DaRT henceforth) was shown to efficiently destroy a wide range of solid tumors, while sparing the adjacent tissues. This technique utilizes Radium (224Ra)-loaded stainless-steel wires or tubes (DaRT seeds) that release daughter atoms inside the tumor to a range of several millimeters (8–14). DaRT was shown to activate systemic immune memory when used as a monotherapy (15, 16). When combined with immunoadjuvants and inhibitors of immune suppressive cells, DaRT led to long-term rejection of immunogenic tumors in mice, whereas the same immunomodulatory treatment with a non-radioactive seed mostly led to tumor recurrence (17, 18). Long-term rejection of tumors was correlated with a specific immune memory against tumor antigens (18), suggesting that cell death by alpha radiation activates tumor antigen recognition at the ablation site. This agrees with reports showing that the cell response to radiation includes the elevation of damage-associated molecular patterns (DAMPs) (19), MHC class I expression (20), and interferon responses (21) that may contribute to antigen presentation, cross presentation, APC activation, and recruitment of effector T lymphocytes (22).
Another type of in situ vaccination employs the activation of cytoplasmatic viral sensors such as RIG-I-like receptors (RLRs). RLRs (e.g., RIG-I and MDA5) sense cytoplasmatic viral dsRNA as part of a conserved defense mechanism of the innate immune system (22–24). Upon activation, these sensors promote antigen presentation, a type-1 interferon response, pyroptosis, DAMPs secretion, and immunogenic cell death (25). Recently, RLR activation was found to boost the efficiency of anticancer vaccines and to be critical for responsiveness to immune checkpoint blockade (26, 27).
One way to activate RLRs is to deliver a dsRNA viral mimic, such as polyIC, directly into the cytoplasm of the cell, while bypassing endosomal recognition via toll-like receptors, such as TLR3 (28). This is done using a delivery agent, such as the cationic polymer polyethylenimine (PEI), which masks the viral dsRNA until it reaches the cytoplasm, where it is released and recognized (29, 30). PolyIC cytoplasmic delivery was previously tested as a targeted therapy (26, 31), a systemic therapy (32, 33), and a local therapy (34). However, the effects of this treatment on distant metastases or in combination with radiotherapy have not been well-investigated. Interestingly, hallmarks of immunogenic cell death were observed in tumor cells following treatment with polyIC complexed with PEI, including the elevation of MHC class I expression. However, identical concentrations of polyIC (a TLR3 agonist) without PEI failed to elevate MHC class I expression (3), suggesting that the RLR pathway is superior to the TLR pathway with regard to antigen presentation on tumor cells following activation.
Another way to stimulate RLRs is by using DNA methyltransferase (DNMT) inhibitors such as decitabine (35). DNMT inhibitors can stimulate endogenous retroviruses that are sensed by RLRs (36–38) or inhibit the methylation of RLR genes promoters (35). RLR activation by both cytoplasmatic delivery of dsRNA (34) and DNMT inhibitors (39–41) was shown to upregulate MHC class I and to potentiate interferon and cytotoxic T lymphocyte responses.
Both alpha radiation-based ablation (16) and RLR activation (34) were shown to induce local tumor cell killing and a systemic antitumor response. It was shown that radiation-mediated antitumor immunity requires a cytosolic DNA-sensing pathway, such as the stimulator of interferon genes (STING) pathway (42). The fact that DNA-sensing and RNA-sensing function via different pathways may increase the potential to achieve a synergy between DaRT and RLR activation.
The current study investigated a novel approach to combine alpha radiation-based ablation and RLR activation in low-immunogenic and metastatic tumor models, such as the triple negative breast cancer (TNBC) mouse model 4T1, the pancreatic carcinoma tumor model Panc02, and the squamous cell carcinoma (SCC) tumor model, SQ2. Aggressive tumors such as TNBC and pancreatic cancer demonstrate low immunogenicity, which correlates with low responsiveness to immunotherapy and is mainly determined by tumor antigenicity and antigen presentation efficiency (43). MHC class I molecules on the surface of tumor cells were identified as critical for the enhancement of immunotherapy effectiveness (44). In support of this, it was recently demonstrated that antigens presented in the context of MHC class I, pulled down from tumor cell lysate, can serve as an artificial antigen presenting cell and induce potent and specific effector CD8+ T cell responses against tumor cells (45). In the current study, RLR activation was used long enough prior to the induction of cell death by alpha radiation to allow the potential enhancement of antigen presentation on tumor cells, which may be crucial for achieving antigen-specific antitumor immunity in low-immunogenic tumors. The effect of the treatment on tumor development and on metastatic load was investigated by probing lung metastases at a late timepoint. In addition, long-term survival after local treatment and tumor resection was used to confirm clearance of metastases. Immune memory was investigated by employing the Winn and challenge assays. Finally, the treatment was combined with systemic immunomodulation.
Materials and Methods
Animals
All animal experiments were carried out in accordance with the government and institution guidelines and regulations (Ethics approval IDs 01-18-030, 01-19-039, 01-19-081) and with the National Institutes of Health guide for the care and use of Laboratory animals (NIH Publications No. 8023, revised 1978). BALB/c and C57BL/6 female mice (~20 g, 10 weeks old) were obtained from Envigo (Jerusalem, Israel) and were kept in the animal facility of Tel Aviv University. All surgical and invasive procedures were performed under anesthesia using ketamine (100 mg/kg, Bremer Pharma, Germany) and xylazine hydrochloride (10 mg/kg, Eurovet Animal Health B.V., Bladel, Netherlands) solution in PBS. Intraperitoneal inoculation was given 10 min before starting the treatment.
Tumor Cell Lines
All cell lines were incubated in a humid incubator at a temperature of 37°C and 5% CO2. M-cherry-labeled 4T1 mammary adenocarcinoma tumor cells (kindly provided by Prof. Satchi-Fainaro, Faculty of Medicine, Tel Aviv University, Tel Aviv, Israel) were grown in RPMI-1640 containing L-glutamine, supplemented with 10% fetal calf serum, penicillin (100 U/ml), streptomycin (100 μg/ml), nystatin (12.5 U/ml), sodium pyruvate (1 mM), and HEPES buffer 1 M (Biological Industries, Kibbutz Beit Haemek, Israel). Panc02 murine pancreatic carcinoma (kindly provided by Dr. Hollingsworth, Eppley Institute, Nebraska University Medical Center, USA) were grown in Dulbecco's modified eagle medium (DMEM) supplemented with 10% fetal calf serum, penicillin (100 U/ml), streptomycin (100 μg/ml), nystatin (12.5 U/ml), sodium pyruvate (1 mM), and MEM Non-Essential Amino Acids (Biological Industries, Kibbutz Beit Haemek, Israel). SQ2 murine squamous cell carcinoma (kindly provided by Dr. Gad Lavie from the Sheba Medical Center, Tel HaShomer, Israel) were grown in Dulbecco's modified eagle medium (DMEM) supplemented with 10% fetal calf serum, penicillin (100 U/ml), streptomycin (100 μg/ml), and nystatin (12.5 U/ml) (Biological Industries, Kibbutz Beit Haemek, Israel).
Tumor Cell Inoculation
4T1MCherry, SQ2, and panc02 tumor cells were inoculated in doses of 2.5 × 105, 5 × 105, and 6 × 105, respectively. Mice were inoculated intracutaneously into the right (unless stated otherwise) low lateral side of the back in 0.05 mL Hanks' balanced salt solution (HBSS, Biological Industries, Kibbutz Beit Haemek, Israel).
Drug Preparations
According to previous studies, high-molecular-weight (HMW) polyIC induced stronger immune activation than low-molecular-weight (LMW) polyIC (46) and was therefore chosen to be delivered into tumor cells in the current study. PolyIC HMW VacciGrade™ (InvivoGen, USA) was prepared in aliquots according to manufacturer instructions and kept at −20°C. At the day of treatment, polyIC was mixed with in vivo-jetPEI® (Polyplus, France) according to manufacturer instructions. Briefly, polyIC and PEI were diluted in 5% glucose solution and incubated at a ratio of N:P = 6 for 15 min at room temperature. PolyIC was intratumorally injected to the tumor 72 and 24 h prior to DaRT insertion. 5% glucose served as vehicle unless mentioned otherwise. Cyclophosphamide (Sigma C0768, Israel) was prepared at the indicated concentrations in saline solution. CP was administrated i.p. in the dose of 100 mg/kg 24 h prior to polyIC. Decitabine (Tocris, UK) was prepared in PBS. Decitabine was administrated i.p. in the dose of 1 mg/kg daily for 4 consecutive days prior to DaRT insertion.
224Ra-Loaded Seed Preparation and Insertion
Stainless steel (316 LVM) 0.7-mm-diameter tubes in the length of 6.5 mm (unless mentioned otherwise) were loaded with 224Ra atoms, following an electrostatic collection process similar to that described in (12). To prevent radium detachment from the surface, the seeds were coated, in this study, with a 250-nm (nanometer) polymeric layer (Nusil, med2-4213 model). The 220Rn desorption probability (the probability that a 220Rn atom is emitted from the seed following a decay of 224Ra) was 45% (unless mentioned otherwise). The 224Ra activity in kBq is indicated for each experiment in the Results section. Seeds, either loaded with 224Ra or inert, were placed near the tip of a 19-gauge needle, which is attached to an insertion applicator. The radioactive and inert seeds were inserted into the tumor under anesthesia.
In vivo Tumor Measurements
Local tumor growth was determined by measuring 3 mutually orthogonal tumor dimensions 2–3 times per week, according to the following formula: Tumor volume = π/6 × Diameter 1 × Diameter 2 × Height. Daily survival monitoring was performed and recorded.
Tumor and Metastasis Imaging and Analysis
CRI Maestro™ (Cambridge Research and Instrumentation, USA) was used to measure M-Cherry signal. Multispectral image cubes were acquired through a 550–800-nm spectral range in 10-nm steps using an excitation (595 nm longpass) and emission (645 nm longpass) filter set, under exposure time of 2,000 ms. Autofluorescence signals were eliminated by spectral analysis and linear unmixing algorithm of the CRI-Maestro software. Computed tomography (CT) scan was performed using the TomoScope Synergy microCT scanner (CT imaging, Erlangen, Germany) under anesthesia. Data was acquired using 360° individual projection collected every 1° to complete one rotation around the animal, with X-ray tube voltage of 40 kV. Cross-sectional images (DICOM format) were generated using TomoScope image reconstruction software (CT imaging, Germany) and were analyzed using “RadiAnt” software.
Histology
For histological H&E staining, lungs were washed in PBS and fixed in a 4% formaldehyde solution (Bio-Lab, Jerusalem, Israel) for at least 24 h. The preserved specimens were processed in ethanol and xylene and then embedded in paraffin. Six-μm sections were then stained with hematoxylin (Sigma, Rehovot, Israel) and eosin (Surgipath, Richmond, VA, USA).
Winn Assay
Spleens were harvested, immersed in PBS, ground with the flat end of a syringe, and passed through a cell strainer. Cells were washed in RPMI/HBSS and centrifuged at 394 × g for 7 min. The supernatant was removed, and cells were resuspended and pooled. Red blood cells were lysed, and cells were washed in HBSS. Cells were then mixed with tumor cells in the indicated ratio and immediately injected in a volume of 0.15 ml.
Statistical Analysis
The difference between the mean values of two groups was determined by two-sided Student's T-test on the last day of the experiment, unless mentioned otherwise. The difference in the proportion of an event between two groups was determined by χ2 test. Differences in the survival period between two groups were determined by log-rank test. p < 0.05 was considered as significant difference between groups.
Results
Intratumoral polyICPEI and DaRT Synergistically Inhibit the Development of 4T1 Solid Tumors and Metastases
A previous study done in the immunogenic tumor model CT26 has shown that combining DaRT with TLR agonists led to long-term tumor rejection, which was not observed when DaRT was used alone (18). Here, it was investigated whether in the low-immunogenic tumor model 4T1 using RLR activation in combination with DaRT is superior to TLR activation, and whether this combination is synergistic in terms of long-term local and systemic retardation of tumor development. To answer these questions, intratumoral administration of the dsRNA viral mimic, polyIC, was used in two forms, as follows. Either complexed with the delivery polymer PEI (polyICPEI) to enable the cytoplasmatic delivery of polyIC and the activation of the RIG-1 receptor MDA5 (47) or “Naked” polyIC (polyICnaked), which agonizes the toll-like receptor TLR3 (48).
Mice bearing 4T1 tumors were treated by an intratumoral injection of 20 μg/40 μl polyICPEI, polyICnaked, or PBS followed by the insertion of a single DaRT seed (length = 8 mm, activity =70 kBq) or a non-radioactive (inert) seed. Twenty-nine days after treatment started, lungs were scanned by computed tomography (CT). The experiment was terminated 37–38 days following tumor cell inoculation, and lungs were imaged for M-Cherry fluorescent signal (Figures 1A,B).
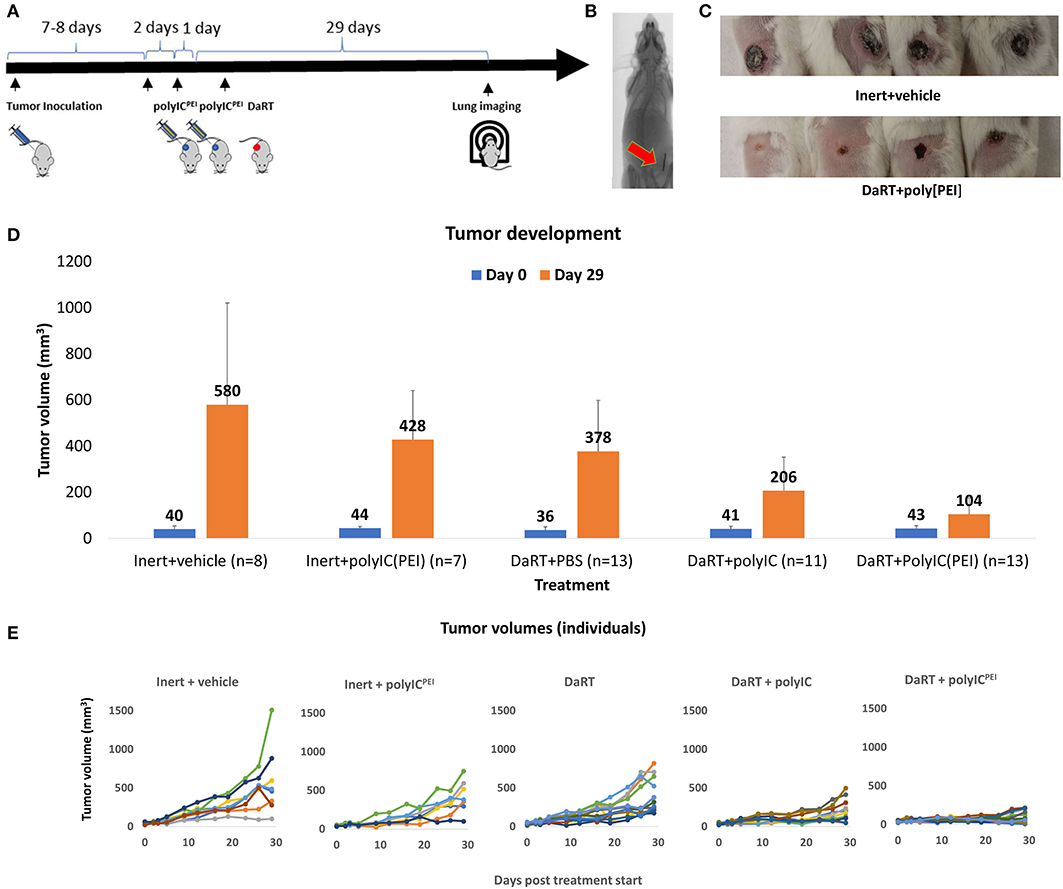
Figure 1. Primary tumor growth following treatment with DaRT+polyICPEI. 4T1-bearing mice (40 mm3) were treated intratumorally with polyICPEI (20 μg/40 μl) or polyICnaked (20 μg/40 μl) or vehicle, 72 and 24 h prior to the insertion of a single DaRT seed (length = 8 mm, activity = 70 kBq) or an inert seed (for 29 days). (A) Schematic representation of the treatment protocol. (B) CT image of a DaRT seed inside the tumor. (C) Representative primary tumors 29 days after tumor cell inoculation. (D) Mean tumor volumes ± SEM on the day of treatment start (0) and on the day of experiment termination (29). pt−test < 0.005 for DaRT+ polyICPEI vs. control; pt−test < 0.0005 for DaRT+ polyICPEI vs. DaRT alone; pt−test < 0.0005 for DaRT+ polyICPEI vs. polyICPEI alone; pt−test < 0.05 for DaRT+ polyICPEI vs. DaRT+polyICnaked. (E) Individual tumor growth curves for each treatment, up to 29 days from treatment start. Each line represents an individual mouse. The results are based on cumulative data from two different experiments.
The results indicated that DaRT combined with polyICPEI significantly retarded tumor growth (pt−test < 0.05) compared to all other groups (Figures 1C–E). The percent reduction in tumor volume compared to inert+vehicle control was calculated for each treatment (according to the following formula: [(mean tumor volume at day 29 in the treatment group)/(mean tumor volume at day 29 of control group)−1] × 100). The cytoplasmatic delivery of polyIC treatment on its own reduced tumor size by 26% compared to inert+vehicle (control). Alpha radiation treatment on its own reduced tumor size by up to about 34% compared to control. The combination of alpha radiotherapy and cytoplasmatic delivery of the viral mimic polyIC reduced the tumor size by 82%, demonstrating a synergistic effect between the treatments. Treatment with DaRT+polyICnaked significantly retarded tumor growth compared to DaRT alone or inert+vehicle control (Figures 1C,E). However, the treatment was significantly less effective compared to DaRT combined with polyICPEI.
Analysis of lung metastases by CT scan or M-Cherry fluorescence imaging (see Methods, Figure 2A) revealed that 37 days after tumor inoculation, the percent of animals bearing lung metastases was significantly smaller in the polyICPEI+DaRT group (23%) compared to DaRT alone (77%) or inert+vehicle control (75%), p (χ2 test) <0.05 (Figure 2B). DaRT+polyICnaked also reduced metastatic burden, as demonstrated by total M-Cherry signal in the lungs (Figure 2C). However, a higher number of mice treated with DaRT+polyICnaked were positive for metastases than those treated with DaRT+polyICPEI (55% compared with 23%). Histology sections of lungs correlated with the findings obtained by M-Cherry and CT (Figure 2D).
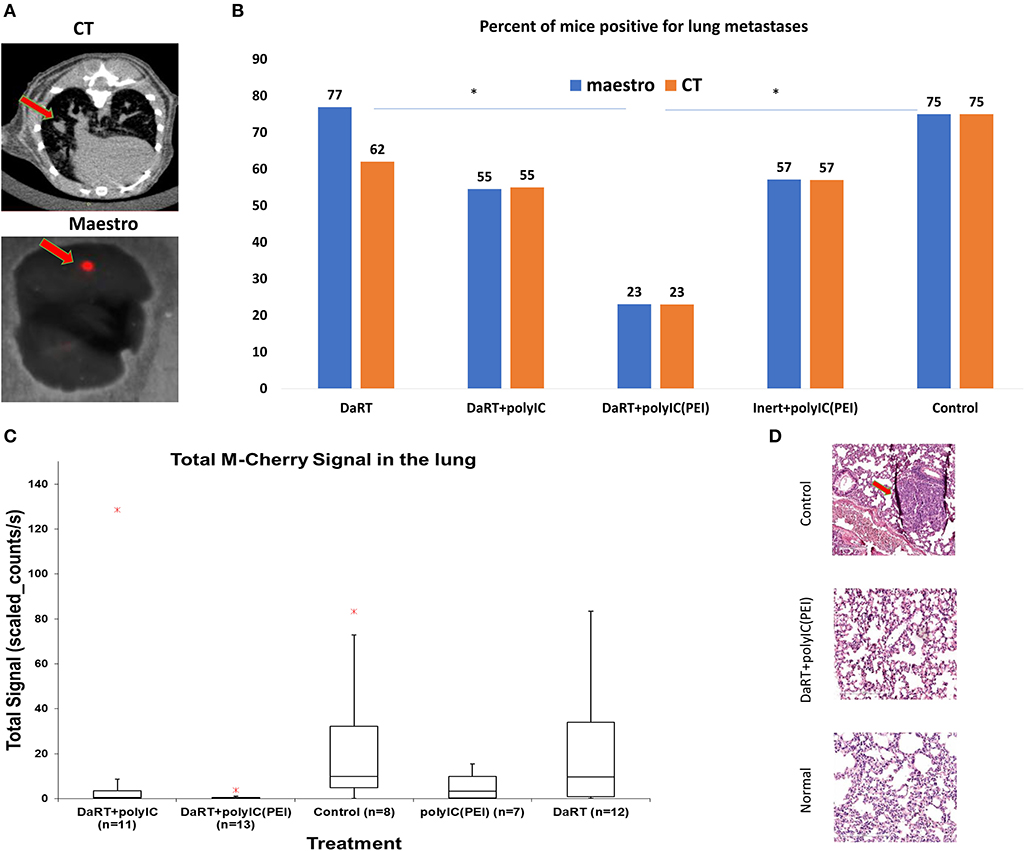
Figure 2. Lung metastases following treatment with DaRT+polyICPEI. 4T1MCherry-bearing mice were treated intratumorally with polyICPEI, polyICnaked, or vehicle, prior the insertion of a single DaRT seed or an inert seed (see Figure 1A). Lungs were imaged by CT and fluorescence imaging using Maestro, 37 days following tumor cell inoculation. (A) Illustrative pictures of metastases as imaged by CT scan or M-Cherry fluorescence imaging. (B) Percent of mice that were positive for lung metastases according to CT scan and M-Cherry fluorescence imaging. (C) Box plot of M-cherry total signal in the lungs. Red cross denotes an outlier (the graph does not include a DaRT outlier with the value of 362 counts/s). (D) Representative histology sections of a normal lung, a lung of a 4T1-bearing mouse treated with DaRT+polyICPEI, and a lung of a 4T1-bearing mouse treated with inert+vehicle. The results are based on cumulative data from two different experiments.
Treatment With Intratumoral polyICPEI Prior to DaRT Caused Rejection of Panc02 Solid Tumors
The robustness of this treatment was tested by applying it to another aggressive and metastatic tumor model, the pancreatic tumor cell line Panc02. Mice bearing Panc02 tumors were treated with polyICPEI (25 μg/50 μl), followed by the insertion of a DaRT seed (75 kBq). On the seed insertion day, average tumor volume was ~35 mm3.
DaRT+polyICPEI significantly retarded tumor growth compared with DaRT (12-fold change on day 24 post-DaRT) (Figure 3A). Moreover, the treatment caused tumor rejection in 42.9% (3 out of 7) of the animals for up to 38 days following DaRT upper panel (Figure 3B). At this timepoint, one tumor recurred and 2 out of 7 mice remained tumor-free and survived from this timepoint on, with no signs of illness. Tumors that were not rejected developed more slowly in the DaRT+polyICPEI group relative to the DaRT group (Figures 3C,D).
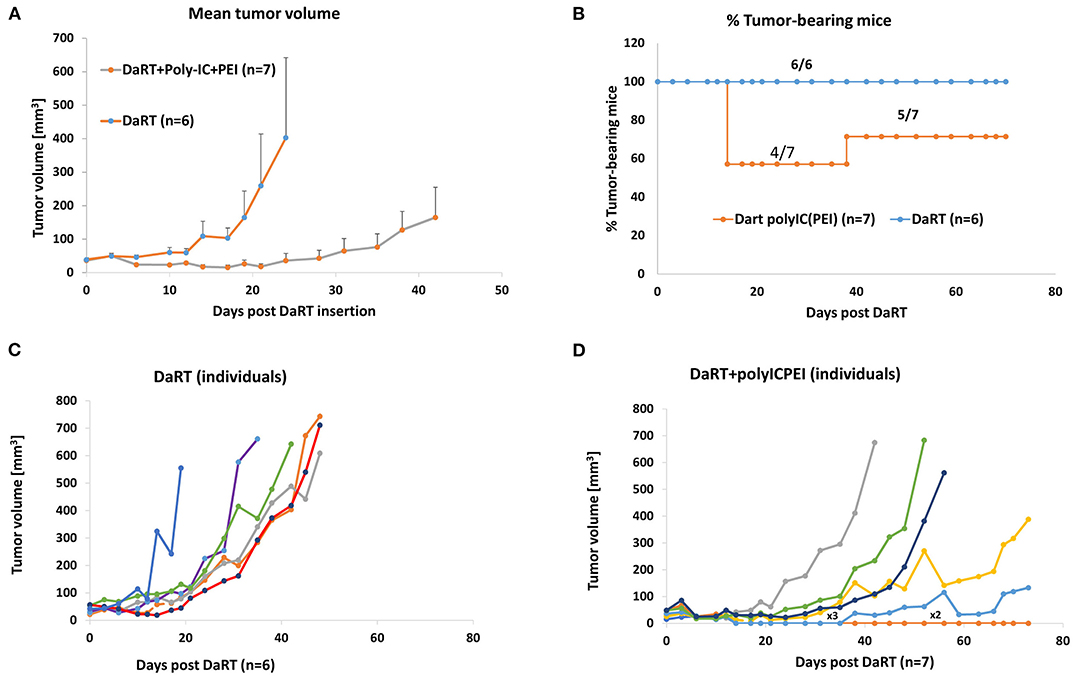
Figure 3. DaRT+polyICPEI treatment rejected Panc02 tumors. Panc02-bearing mice were treated with an intratumoral injection of polyICPEI (25 μg /50 μl) 72 and 24 h prior DaRT seed insertion (activity=75 kBq). (A) Tumor volume ± SEM. (B) The percent of tumor-bearing mice. (C,D) Individual tumor development of mice treated with DaRT+polyICPEI (left) or DaRT+vehicle (right). Each line represents an individual mouse. “x3” denotes a line representing three individuals; “x2” denotes a line representing two individuals.
Splenocytes From Mice Pretreated With Intratumoral polyICPEI and DaRT Inhibit 4T1 Tumor Development When Adoptively Transferred to Naïve Mice
The results above showed that even though polyICPEI+DaRT therapy was administrated locally, at the primary tumor site only, it led to both long-term tumor growth retardation and clearance of distant metastases. The Winn assay was employed to investigate whether the treatment activated a long-term systemic immune memory against tumor antigens. In this in vivo cytotoxic test, splenocytes from treated mice or from naïve mice are adoptively transferred to naïve mice in combination with tumor cells, and tumor development is monitored.
Mice (n = 16) bearing 4T1 tumors (30 mm3) were treated with polyICPEI+DaRT (as depicted in Figure 1A). Residual tumors were resected 24 days following tumor cell inoculation (at a time in which metastases were already present in the lungs), and animals were observed for long-term survival (namely, metastases-related death). Mice surviving for 9 months after tumor inoculation were considered as cured, and their splenocytes were used for an adoptive cell transfer assay (Winn assay). Autopsy of non-surviving mice confirmed lung metastases in all animals (except one animal which had an inflamed lung without visible metastases). Lymphocytes from the spleens of cured mice (n = 4) were harvested, pooled, mixed with 2.5 × 105 4T1 tumor cells in a ratio of 100:1 (splenocytes: tumor cells), and inoculated into naïve mice. Splenocytes of naïve mice (n = 4) served as control (Figure 4A).
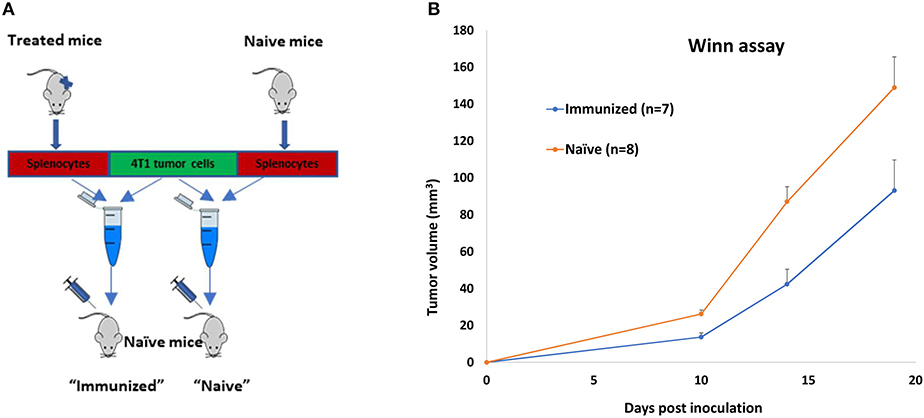
Figure 4. Long-term immune memory in 4T1-bearing mice treated by polyICPEI+DaRT and surgery. 4T1-bearing mice (n = 16) were treated by intratumoral 20 μg/40 μl polyICPEI or vehicle 72 and 24 h prior the insertion of a single DaRT seed (7 mm, 80 kBq). Twenty-four days after tumor cell inoculation, tumors were resected. Mice surviving 9 months after initial tumor cell inoculation (n = 4) or naïve mice (n = 4) were sacrificed, and their splenocytes were pooled and mixed with 4T1 tumor cells in the ratio of 100:1 (splenocytes: tumor cells). The combined suspension was inoculated into naïve mice. (A) Winn assay scheme. (B) Mean tumor volume ± SEM, pt−test < 0.05 for immunized vs. naïve groups.
Splenocytes of treated mice significantly retarded tumor development compared to splenocytes of naïve mice (Figure 4B). A significant reduction in tumor size lasted for 19 days after co-inoculation with tumor cells (52% reduction was evident 14 days after co-inoculation, pt−test= 0.002: 42 ± 8 and 87 ± 8 mm3 for immune vs. naïve splenocytes, respectively), demonstrating that the treatment induced a long-term antitumor immune memory that is efficient even 9 months following the initial tumor cell inoculation.
Systemic Low-Dose Cyclophosphamide Combined With Intratumoral polyICPEI Synergizes With DaRT in Preventing Lung Metastases-Related Death
Next, it was investigated whether systemic immunomodulation could further augment tumor growth retardation caused by polyICPEI+DaRT treatment or prolong mouse survival by preventing metastasis-related death. To reduce the number of T regulatory cells (Tregs), a previously proven treatment regimen of low-dose CP was employed (49). 4T1-bearing mice were treated with CP 1 day before the first polyICPEI injection (30 μg/60 μl), which is 4 days prior to DaRT insertion (activity = 65 kBq), at a time in which tumor size was ~24 mm3. DaRT+polyICPEI+vehicle served as a control. Tumor development was followed for 14 days after DaRT insertion, and the tumors were resected thereafter (Figure 5A). In order to examine the effect of the treatments on lung metastases in the above treated animals, monitoring was done for metastases-related death for ~6 months post DaRT, and animal death and the presence of lung metastases were recorded.
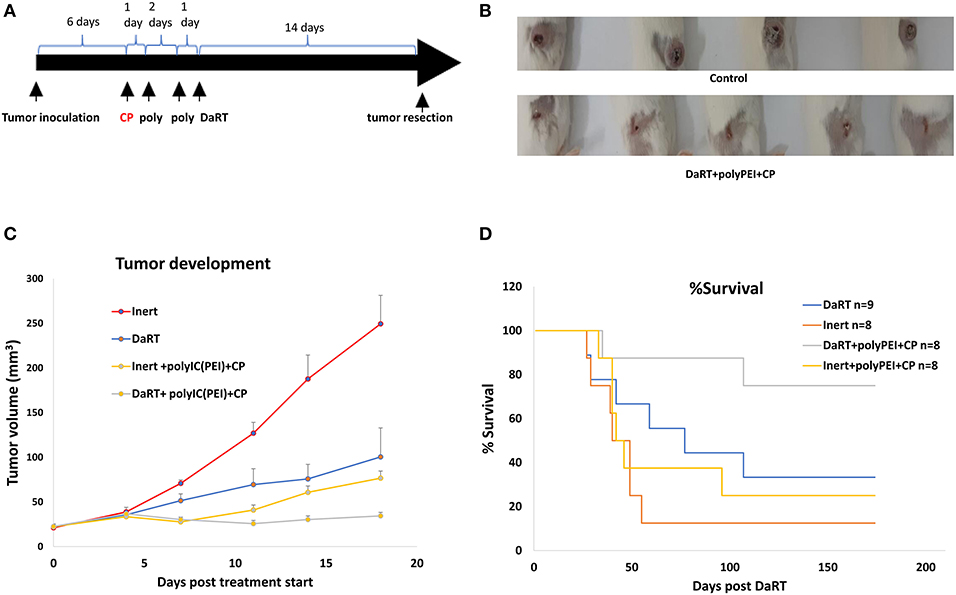
Figure 5. The effect of systemic low-dose CP in combination with local polyICPEI+DaRT on tumor development and metastasis-related death. (A) Schematic representation of the treatments with low-dose cyclophosphamide combined with DaRT+polyICPEI and tumor resection. (B) Representative tumors on the day of tumor resection. (C) Mice were treated with CP (100 mg/kg, i.p.) combined with polyICPEI (30 μg/60 μl i.t.) + DaRT (activity = 85 kBq). Presented are tumor volume ± SEM. Pt−test < 0.05 for DaRT+polyICPEI+CP compared all other treatments. (D) Kaplan–Meier survival curves of tumor-resected mice following treatment. Plog−ranktest < 0.01; < 0.05, for DaRT+ polyICPEI+CP vs. inert+vehicle control or polyICPEI+CP, respectively.
Adding CP to DaRT+polyICPEI treatment significantly reduced tumor volume on the day of tumor resection (47 ± 5, 29 ± 3 mm3, pt−test < 0.05). The manual measurements of tumor dimensions by a caliper were corroborated with M-cherry fluorescence imaging of the resected tumors. This analysis confirmed that total M-cherry signal and the tumor area (according to M-Cherry fluorescence) where smaller in the polyICPEI+DaRT+CP group compared to the polyICPEI+DaRT group (83 ± 23 vs. 693 ± 280 scaled counts/s, pt−test = 0.051; 65 ± 14 vs. 163 ± 20 mm2, pt−test < 0.005, respectively).
Adding CP to polyICPEI+DaRT treatment extended the survival period relative to treatment with polyICPEI+DaRT without CP. During the 143 days post DaRT insertion, 100% of the animals treated with polyICPEI+DaRT+CP were still alive, at the same timepoint only 71% of the mice treated with polyICPEI+DaRT survived. Nonetheless, the difference between the groups according to log-rank test was not significant and at the end of the experiment identical survival rates (71.4%) were observed in both groups.
Next, an additional experiment was conducted to explore the contribution of DaRT alone (85 kBq) or immunotherapy alone by CP+polyICPEI (30 μg/60 μl i.t.) relative to the combined treatment. PolyICPEI+CP+inert seed or DaRT+vehicle significantly retarded tumor development compared to inert+vehicle control (pt−test < 0.05, on the day of resection) (Figures 5B,C). The combined treatment using DaRT+polyICPEI+CP was significantly more effective than all other treatments (Figure 5C). Ten days post DaRT, for example, tumor volume in the control (inert+vehicle) group was 2.5-fold higher than DaRT only group (DaRT+vehicle), and 3.1-fold higher than that in the immunotherapy-only group (polyICPEI+CP+inert). On that same day, the control group was 6.2-fold higher than the combination treatment (DaRT+polyICPEI+CP), which is more than the expected additive effect (5.6-fold).
At the end of the follow-up period, 75% (6/8) of the mice treated with DaRT+polyICPEI+CP survived, whereas lower survival rates were obtained by DaRT+vehicle (33%, 3/9), inert+polyICPEI+CP (25%, 2/8), or inert+vehicle (12%, 1/8) treatments (Figure 5D). Autopsies of non-surviving animals confirmed metastasis-related death. The effect of DaRT+polyICPEI+CP was significant compared to inert+vehicle (plog−rank test < 0.01) and compared to inert+polyICPEI+CP (plog−rank test < 0.05), but not compared to DaRT alone (plog−rank test =0.081).
Systemic Low-Dose Decitabine Combined With DaRT Retarded the Growth of 4T1 Tumors
It was then investigated whether systemic low-dose administration of the epigenetic drug decitabine (37), which is known to activate RLR, will strengthen tumor growth retardation induced by DaRT, similar to locally administered polyICPEI. This question is of special therapeutic importance, because decitabine can be administrated systematically to patients.
Mice bearing 4T1 tumors (40 mm3) were treated with polyICPEI (30 μg/60 μl 72 h prior to DaRT and 50 μg/100 μl 24 h prior to DaRT) and/or decitabine (1 mg/kg i.p. daily for 4 consecutive days) prior to the insertion of a DaRT seed (activity = 75 kBq) (Figure 6A).
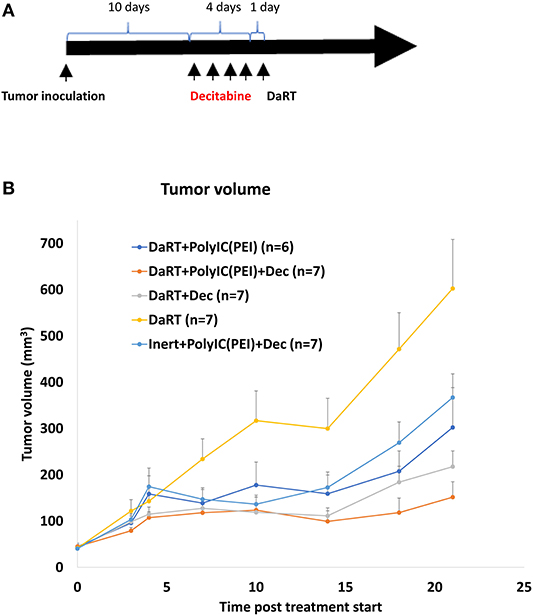
Figure 6. Effect of DaRT combined with decitabine and/or polyICPEI on 4T1 tumor development. Mice bearing 4T1 tumors (40 mm3) were treated with a DaRT seed (75 kBq) in combination with decitabine (1 mg/kg i.p. for 4 consecutive days prior to DaRT) and/or polyICPEI (30 μg/60 μl, 72 h prior to DaRT and 50 μg/100 μl polyICPEI, 24 h prior DaRT). (A) Schematic representation of the treatment by decitabine. (B) Presented are the tumor volumes ± SEM.
DaRT combined with decitabine significantly reduced tumor size by 64% compared to DaRT alone, similar to the 50% reduction achieved by DaRT+polyICPEI (Figure 6B). DaRT combined with both decitabine and polyICPEI achieved the strongest effect (75% reduction compared with DaRT alone, pt−test = 0.001), yet it was only marginally better than DaRT with each stimulator alone. In addition, it was demonstrated that DaRT+decitabine+polyICPEI was significantly stronger (2.5-fold) compared to the same treatment with a non-radioactive seed (Figure 6B). These results were confirmed in an additional experiment in which mice were bearing larger tumors (85 mm3 at the day of treatment start). Tumor volume determined at the same timepoint for inert or DaRT, combined with polyICPEI and decitabine, was 194 ± 25 vs. 115 ± 16 mm3, respectively, pt−test < 0.05).
DaRT Combined With Systemic Low-Dose Decitabine or Intratumoral polyICPEI Inhibited the Growth of SQ2 Solid Tumors and Induced Antitumor Immune Response Against Tumor Cell Re-challenge
To further test the robustness of these treatment regimens, including their ability to induce an antitumor systemic immune memory, a tumor model of squamous cell carcinoma (SCC), SQ2, was investigated. SCC was the first type of tumor for which DaRT was tested in human patients (50). SQ2-bearing mice were treated with DaRT (85 kBq) combined with polyICPEI (25 μg /50 μl), decitabine, or both. Residual tumors were resected 24 days after DaRT, and mice were subjected to tumor re-challenge of the same number of cells (5 × 105 tumor cells), 22 days after tumor resection.
DaRT combined with polyICPEI, decitabine, or both significantly retarded tumor development compared to DaRT alone. DaRT+decitabine significantly retarded tumor development similar to DaRT+polyICPEI, leading to a ~65% reduction in tumor size compared to DaRT+vehicle treatment, for up to 27 days from treatment initiation. In this tumor model, the combination of DaRT+polyICPEI+decitabine provided the best results with 92% reduction (20-fold change) compared to DaRT alone and was significantly superior to both DaRT+decitabine or DaRT+polyICPEI (Figure 7A). DaRT combined with polyICPEI, decitabine, or both preserved the ability to induce long-term immune memory, as demonstrated by a significant reduction (~80%) in tumor size after re-challenge, compared to naïve mice inoculated with the same number of tumor cells (Figure 7B).
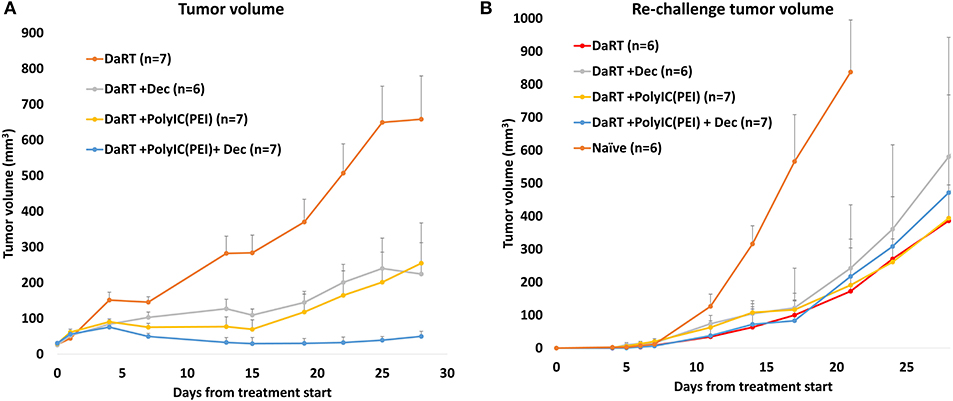
Figure 7. Tumor control and long-term immune memory following treatment with DaRT, decitabine, polyICPEI, or both, in SQ2 tumor model. (A) SQ2-bearing mice (30 mm3 on first decitabine dose day) were treated with decitabine (1 mg/kg i.p. daily for 4 consecutive days prior to the DaRT) and/or an intratumoral injection of polyICPEI (25 μg/50 μl 72 and 24 h prior to DaRT). A DaRT (activity=85 kBq) seed was inserted into the tumor 24 h later. Presented are the tumor volumes ± SEM. Pt−test < 0.05 for all treatments vs. DaRT and for DaRT+decitabine+polyICPEI vs. DaRT+decitabine. (B) Residual tumors were resected 24 days after DaRT and mice were subjected to tumor re-challenge of 5 × 105 tumor cells 21 days after tumor resection. Presented are the tumor volumes ± SEM of the re-challenged tumors. Significant difference was observed for all groups vs. naïve mice (Pt−test < 0.05, on day 17). *On day 17 one mouse from the DaRT+decitabine+polyICPEI group died from unknown reason and was not included in the mean tumor volume calculation from this time point on.
Discussion
In the present study, we examined the possible synergy between the activation of cytoplasmatic dsRNA sensors and tumor ablation by intratumoral diffusion of alpha emitting atoms, both at the local and systemic levels. Treatment with DaRT in combination with cytoplasmatic delivery of polyIC synergistically retarded the development of mouse TNBC tumors and demonstrated rejection of mouse pancreatic tumors. Although the treatment was administrated locally, it also reduced the metastatic load in the lungs and induced a long-term systemic antitumor immune response. Low-dose CP, which was previously shown to reduce the number of Tregs (49), enhanced the tumor control achieved by the local treatment and led to high long-term survival rates that confirmed the reduction in metastatic load.
DaRT-related antitumor immunity (16) was previously attributed to the in situ dispersion of tumor antigens, processed by APCs (4). Addition of TLR agonists (16–18) that activate APCs enhanced DaRT's effect. In the current study, combining DaRT with polyIC, complexed with the delivery reagent PEI (PolyICPEI), led to more robust solid tumor control and greater clearance of metastases relative to the same treatment with polyIC only (a TLR3 agonist by itself). This finding suggests that polyICPEI may exhibit a dual effect, both augmenting antigen presentation by tumor cells (via RLR) and antigen presentation by dendritic cells (via TLR).
The use of DaRT after polyICPEI may consequently lead to the release of DAPMs after DNA damage, pathogen-associated molecular patterns (PAMPs) from radiation killed cells containing dsRNA, and a massive amount of tumor antigens in the context of MHC class I. This may support important processes such as cross-presentation and cross-dressing (51). In addition, the potential elevation of MHC class I on tumor cells by PolyICPEI (34) prior to cell death by DaRT may increase the probability to present yet non-presented tumor antigens in the context of MHC class I. Thus, it can be speculated that PolyICPEI-treated, and alpha-radiation-killed, tumor cells may release such MHC class I-antigen complexes, which can be picked up by DCs that in turn present them to CD8+ T cells and help to expand the number of clones recognizing tumor antigens.
In this study, it was shown that DaRT combined with different types of agents known to activate RLR achieved robust antitumor effects in three tumor models. Low-dose decitabine resulted in tumor retardation, similar to polyICPEI. In the SCC tumor model, adding decitabine, polyICPEI, or both reduces tumor size compared to DaRT, yet in the challenge assay, the addition of RLR activation did not affect the power of the long-term immune response relative to DaRT alone (Figure 7B). This may be due to the relatively high number of tumor cells used in the assay. Another possibility is that cells inoculated in the challenge assay were not subjected to a treatment that elevates antigen presentation before inoculation. Namely, antigens that were potentially unmasked by RLR activation in situ were not presented by the tumor cells inoculated in the challenge assay, since they were not exposed to the RLR activator and no elevation of MHC class I was induced. Further study is needed to clarify these mechanisms.
The synergy between DaRT and RLR activation can be attributed to additional non-immune-related potential mechanisms. For example, the cellular response to a viral attack may promote transcription related to programmed cell death (52), and thus when DNA damage is induced by alpha radiation, the cellular stress response is already biased to favor cellular death over DNA repair. Indeed, RLR activation by cytoplasmatic delivery of polyIC was found to sensitize tumor cells to ionizing radiation also in vitro (53). In the case of decitabine, sensitization to alpha radiation may also be due to chromatin de-condensation (54).
In its first-in-human clinical trial, DaRT was used to treat SCC patients. All patients responded to DaRT, with almost 80% showing complete responses with minor adverse effects (50). In one case, evidence suggests the possible induction of an abscopal effect (55). The treatment regimens presented here efficiently affected both the tumor and distant metastases and extended long-term survival. Low-dose cyclophosphamide, previously found to reduce the number of Tregs, demonstrated the potential of immunomodulating therapies used in clinical practice (56) to further enhance these effects. Taken together, the results presented here may suggest future directions for improved therapeutic protocols for treating patients with metastatic cancer.
Data Availability Statement
The datasets generated for this study are available on request to the corresponding author.
Ethics Statement
The animal study was reviewed and approved by Tel Aviv University ethics committee.
Author Contributions
VD: conceptualization, formal analysis, investigation, methodology, project administration, resources, supervision, validation, visualization, writing—original draft, and writing—review & editing. ME and MS: investigation, methodology, and resources. EG: formal analysis, investigation, visualization, and writing—original draft. FM, AS, AC, and EF: investigation. YZ: investigation and resources. RG: conceptualization and writing—review & editing. IK: conceptualization, funding acquisition, methodology, supervision, and writing—review & editing. YK: conceptualization, funding acquisition, methodology, project administration, supervision, validation, visualization, writing—original draft, and writing—review & editing. All authors contributed to the article and approved the submitted version.
Funding
This study was partially funded by a grant from the Israel Innovation Authority.
Conflict of Interest
YK and IK serve as consultants for Alpha Tau Medical LTD. Tel Aviv, Israel. VD, ME, MS, EF, and AS are employees of Alpha Tau Medical LTD. Tel Aviv, Israel. YK, IK, VD, ME, and MS hold stock options in Alpha Tau Medical LTD. Tel Aviv, Israel. YK, IK, and VD are the inventors of a patent application submitted by Alpha Tau Medical LTD, which is related to the results of the current study.
The remaining authors declare that the research was conducted in the absence of any commercial or financial relationships that could be construed as a potential conflict of interest.
Acknowledgments
We thank the staff of the animal facility at the Tel University Medical School, headed by Dr. Michael Harlev, for their assistance and support.
References
1. Den Brok MH, Sutmuller RP, Van Der Voort R, Bennink EJ, Figdor CG, Ruers TJ, et al. In situ tumor ablation creates an antigen source for the generation of antitumor immunity. Cancer Res. (2004) 64:4024–9. doi: 10.1158/0008-5472.CAN-03-3949
2. Pierce RH, Campbell JS, Pai SI, Brody JD, Kohrt HE. In-situ tumor vaccination: bringing the fight to the tumor. Hum Vaccin Immunother. (2015) 11:1901–9. doi: 10.1080/21645515.2015.1049779
3. Aznar MA, Tinari N, Rullan AJ, Sanchez-Paulete AR, Rodriguez-Ruiz ME, Melero I. Intratumoral delivery of immunotherapy-act locally, think globally. J Immunol. (2017) 198:31–9. doi: 10.4049/jimmunol.1601145
4. Keisari Y. Tumor abolition and antitumor immunostimulation by physico-chemical tumor ablation. Front Biosci. (2017) 22:310–47. doi: 10.2741/4487
5. Tohme S, Simmons RL, Tsung A. Surgery for cancer: a trigger for metastases. Cancer Res. (2017) 77:1548–52. doi: 10.1158/0008-5472.CAN-16-1536
6. Ben-Eliyahu S, Golan T. Harnessing the perioperative period to improve long-term cancer outcomes. J Natl Cancer Inst. (2018) 110:1137–8. doi: 10.1093/jnci/djy055
7. Chen Z, Zhang P, Xu Y, Yan J, Liu Z, Lau WB, et al. Surgical stress and cancer progression: the twisted tango. Mol Cancer. (2019) 18:132. doi: 10.1186/s12943-019-1058-3
8. Arazi L, Cooks T, Schmidt M, Keisari Y, Kelson I. Treatment of solid tumors by interstitial release of recoiling short-lived alpha emitters. Phys Med Biol. (2007) 52:5025–42. doi: 10.1088/0031-9155/52/16/021
9. Cooks T, Arazi L, Schmidt M, Marshak G, Kelson I, Keisari Y. Growth retardation and destruction of experimental squamous cell carcinoma by interstitial radioactive wires releasing diffusing alpha-emitting atoms. Int J Cancer. (2008) 122:1657–64. doi: 10.1002/ijc.23268
10. Cooks T, Arazi L, Efrati M, Schmidt M, Marshak G, Kelson I, et al. Interstitial wires releasing diffusing alpha emitters combined with chemotherapy improved local tumor control and survival in squamous cell carcinoma-bearing mice. Cancer. (2009) 115:1791–801. doi: 10.1002/cncr.24191
11. Cooks T, Schmidt M, Bittan H, Lazarov E, Arazi L, Kelson I, et al. Local control of lung derived tumors by diffusing alpha-emitting atoms released from intratumoral wires loaded with radium-224. Int J Radiat Oncol Biol Phys. (2009) 74:966–73. doi: 10.1016/j.ijrobp.2009.02.063
12. Arazi L, Cooks T, Schmidt M, Keisari Y, Kelson I. The treatment of solid tumors by alpha emitters released from (224)Ra-loaded sources-internal dosimetry analysis. Phys Med Biol. (2010) 55:1203–18. doi: 10.1088/0031-9155/55/4/020
13. Cooks T, Tal M, Raab S, Efrati M, Reitkopf S, Lazarov E, et al. Intratumoral 224Ra-loaded wires spread alpha-emitters inside solid human tumors in athymic mice achieving tumor control. Anticancer Res. (2012) 32:5315–21.
14. Horev-Drori G, Cooks T, Bittan H, Lazarov E, Schmidt M, Arazi L, et al. Local control of experimental malignant pancreatic tumors by treatment with a combination of chemotherapy and intratumoral 224radium-loaded wires releasing alpha-emitting atoms. Transl Res. (2012) 159:32–41. doi: 10.1016/j.trsl.2011.08.009
15. Keisari Y, Hochman I, Confino H, Korenstein R, Kelson I. Activation of local and systemic anti-tumor immune responses by ablation of solid tumors with intratumoral electrochemical or alpha radiation treatments. Cancer Immunol Immunother. (2014) 63:1–9. doi: 10.1007/s00262-013-1462-2
16. Confino H, Hochman I, Efrati M, Schmidt M, Umansky V, Kelson I, et al. Tumor ablation by intratumoral Ra-224-loaded wires induces anti-tumor immunity against experimental metastatic tumors. Cancer Immunol Immunother. (2015) 64:191–9. doi: 10.1007/s00262-014-1626-8
17. Confino H, Schmidt M, Efrati M, Hochman I, Umansky V, Kelson I, et al. Inhibition of mouse breast adenocarcinoma growth by ablation with intratumoral alpha-irradiation combined with inhibitors of immunosuppression and CpG. Cancer Immunol Immunother. (2016) 65:1149–58. doi: 10.1007/s00262-016-1878-6
18. Domankevich V, Cohen A, Efrati M, Schmidt M, Rammensee HG, Nair SS, et al. Combining alpha radiation-based brachytherapy with immunomodulators promotes complete tumor regression in mice via tumor-specific long-term immune response. Cancer Immunol Immunother. (2019) 68:1949–58. doi: 10.1007/s00262-019-02418-5
19. Iyer SP, Hunt CR, Pandita TK. Cross Talk between Radiation and Immunotherapy: The Twain Shall Meet. Radiat Res. (2018) 189:219–24. doi: 10.1667/RR14941.1
20. Reits EA, Hodge JW, Herberts CA, Groothuis TA, Chakraborty M, Wansley EK, et al. Radiation modulates the peptide repertoire, enhances MHC class I expression, and induces successful antitumor immunotherapy. J Exp Med. (2006) 203:1259–71. doi: 10.1084/jem.20052494
21. Lim JY, Gerber SA, Murphy SP, Lord EM. Type I interferons induced by radiation therapy mediate recruitment and effector function of CD8(+) T cells. Cancer Immunol Immunother. (2014) 63:259–71. doi: 10.1007/s00262-013-1506-7
22. Lhuillier C, Rudqvist NP, Elemento O, Formenti SC, Demaria S. Radiation therapy and anti-tumor immunity: exposing immunogenic mutations to the immune system. Genome Med. (2019) 11:40. doi: 10.1186/s13073-019-0653-7
23. Reikine S, Nguyen JB, Modis Y. Pattern Recognition and Signaling Mechanisms of RIG-I and MDA5. Front Immunol. (2014) 5:342. doi: 10.3389/fimmu.2014.00342
24. Brisse M, Ly H. Comparative Structure and Function Analysis of the RIG-I-Like Receptors: RIG-I and MDA5. Front Immunol. (2019) 10:1586. doi: 10.3389/fimmu.2019.01586
25. Elion DL, Cook RS. Harnessing RIG-I and intrinsic immunity in the tumor microenvironment for therapeutic cancer treatment. Oncotarget. (2018) 9:29007–17. doi: 10.18632/oncotarget.25626
26. Heidegger S, Kreppel D, Bscheider M, Stritzke F, Nedelko T, Wintges A, et al. RIG-I activating immunostimulatory RNA boosts the efficacy of anticancer vaccines and synergizes with immune checkpoint blockade. EBioMedicine. (2019) 41:146–55. doi: 10.1016/j.ebiom.2019.02.056
27. Heidegger S, Wintges A, Stritzke F, Bek S, Steiger K, Koenig PA, et al. RIG-I activation is critical for responsiveness to checkpoint blockade. Sci Immunol. (2019) 4:eaau8943. doi: 10.1126/sciimmunol.aau8943
28. Chattopadhyay S, Sen GC. dsRNA-activation of TLR3 and RLR signaling: gene induction-dependent and independent effects. J Interferon Cytokine Res. (2014) 34:427–36. doi: 10.1089/jir.2014.0034
29. Bhoopathi P, Quinn BA, Gui Q, Shen XN, Grossman SR, Das SK, et al. Pancreatic cancer-specific cell death induced in vivo by cytoplasmic-delivered polyinosine-polycytidylic acid. Cancer Res. (2014) 74:6224–35. doi: 10.1158/0008-5472.CAN-14-0819
30. Duewell P, Steger A, Lohr H, Bourhis H, Hoelz H, Kirchleitner SV, et al. RIG-I-like helicases induce immunogenic cell death of pancreatic cancer cells and sensitize tumors toward killing by CD8(+) T cells. Cell Death Differ. (2014) 21:1825–37. doi: 10.1038/cdd.2014.96
31. Levitzki A, Klein S. My journey from tyrosine phosphorylation inhibitors to targeted immune therapy as strategies to combat cancer. Proc Natl Acad Sci USA. (2019) 116:11579–86. doi: 10.1073/pnas.1816012116
32. Besch R, Poeck H, Hohenauer T, Senft D, Hacker G, Berking C, et al. Proapoptotic signaling induced by RIG-I and MDA-5 results in type I interferon-independent apoptosis in human melanoma cells. J Clin Invest. (2009) 119:2399–411. doi: 10.1172/JCI37155
33. Duewell P, Beller E, Kirchleitner SV, Adunka T, Bourhis H, Siveke J, et al. Targeted activation of melanoma differentiation-associated protein 5 (MDA5) for immunotherapy of pancreatic carcinoma. Oncoimmunology. (2015) 4:e1029698. doi: 10.1080/2162402X.2015.1029698
34. Aznar MA, Planelles L, Perez-Olivares M, Molina C, Garasa S, Etxeberria I, et al. Immunotherapeutic effects of intratumoral nanoplexed poly I:C. J Immunother Cancer. (2019) 7:116. doi: 10.1186/s40425-019-0568-2
35. Gonzalez-Cao M, Karachaliou N, Santarpia M, Viteri S, Meyerhans A, Rosell R. Activation of viral defense signaling in cancer. Ther Adv Med Oncol. (2018) 10:1758835918793105. doi: 10.1177/1758835918793105
36. Chiappinelli KB, Strissel PL, Desrichard A, Li H, Henke C, Akman B, et al. Inhibiting DNA Methylation Causes an Interferon Response in Cancer via dsRNA Including Endogenous Retroviruses. Cell. (2015) 162:974–86. doi: 10.1016/j.cell.2015.07.011
37. Roulois D, Loo Yau H, Singhania R, Wang Y, Danesh A, Shen SY, et al. DNA-demethylating agents target colorectal cancer cells by inducing viral mimicry by endogenous transcripts. Cell. (2015) 162:961–73. doi: 10.1016/j.cell.2015.07.056
38. Daskalakis M, Brocks D, Sheng YH, Islam MS, Ressnerova A, Assenov Y, et al. Reactivation of endogenous retroviral elements via treatment with DNMT- and HDAC-inhibitors. Cell Cycle. (2018) 17:811–22. doi: 10.1080/15384101.2018.1442623
39. Adair SJ, Hogan KT. Treatment of ovarian cancer cell lines with 5-aza-2'-deoxycytidine upregulates the expression of cancer-testis antigens and class I major histocompatibility complex-encoded molecules. Cancer Immunol Immunother. (2009) 58:589–601. doi: 10.1007/s00262-008-0582-6
40. Stone ML, Chiappinelli KB, Li H, Murphy LM, Travers ME, Topper MJ, et al. Epigenetic therapy activates type I interferon signaling in murine ovarian cancer to reduce immunosuppression and tumor burden. Proc Natl Acad Sci USA. (2017) 114:E10981–E10990. doi: 10.1073/pnas.1712514114
41. Luo N, Nixon MJ, Gonzalez-Ericsson PI, Sanchez V, Opalenik SR, Li H, et al. DNA methyltransferase inhibition upregulates MHC-I to potentiate cytotoxic T lymphocyte responses in breast cancer. Nat Commun. (2018) 9:248. doi: 10.1038/s41467-017-02630-w
42. Deng L, Liang H, Xu M, Yang X, Burnette B, Arina A, et al. STING-dependent cytosolic dna sensing promotes radiation-induced type i interferon-dependent antitumor immunity in immunogenic tumors. Immunity. (2014) 41:843–52. doi: 10.1016/j.immuni.2014.10.019
43. Wang S, He Z, Wang X, Li H, Liu XS. Antigen presentation and tumor immunogenicity in cancer immunotherapy response prediction. Elife. (2019) 8:e03401. doi: 10.7554/eLife.49020.036
44. Garrido F, Aptsiauri N, Doorduijn EM, Garcia Lora AM, Van Hall T. The urgent need to recover MHC class I in cancers for effective immunotherapy. Curr Opin Immunol. (2016) 39:44–51. doi: 10.1016/j.coi.2015.12.007
45. Su Q, Igyarto BZ. One-step artificial antigen presenting cell-based vaccines induce potent effector CD8 T cell responses. Sci Rep. (2019) 9:18949. doi: 10.1038/s41598-019-55286-5
46. Zhou Y, Guo M, Wang X, Li J, Wang Y, Ye L, et al. TLR3 activation efficiency by high or low molecular mass poly I:C. Innate Immun. (2013) 19:184–92. doi: 10.1177/1753425912459975
47. Alonso-Curbelo D, Soengas MS. Self-killing of melanoma cells by cytosolic delivery of dsRNA: wiring innate immunity for a coordinated mobilization of endosomes, autophagosomes and the apoptotic machinery in tumor cells. Autophagy. (2010) 6:148–50. doi: 10.4161/auto.6.1.10464
48. Bianchi F, Pretto S, Tagliabue E, Balsari A, Sfondrini L. Exploiting poly(I:C) to induce cancer cell apoptosis. Cancer Biol Ther. (2017) 18:747–56. doi: 10.1080/15384047.2017.1373220
49. Hughes E, Scurr M, Campbell E, Jones E, Godkin A, Gallimore A. T-cell modulation by cyclophosphamide for tumour therapy. Immunology. (2018) 154:62–8. doi: 10.1111/imm.12913
50. Popovtzer A, Rosenfeld E, Mizrachi A, Bellia SR, Ben-Hur R, Feliciani G, et al. Initial safety and tumor control results from a “first-in-human” multicenter prospective trial evaluating a novel alpha-emitting radionuclide for the treatment of locally advanced recurrent squamous cell carcinomas of the skin and head and neck. Int J Radiat Oncol Biol Phys. (2019) 106:571–78. doi: 10.1016/j.ijrobp.2019.10.048
51. Nakayama M. Antigen presentation by MHC-dressed cells. Front Immunol. (2014) 5:672. doi: 10.3389/fimmu.2014.00672
52. Barber GN. Host defense, viruses and apoptosis. Cell Death Differ. (2001) 8:113–26. doi: 10.1038/sj.cdd.4400823
53. Yoshino H, Iwabuchi M, Kazama Y, Furukawa M, Kashiwakura I. Effects of retinoic acid-inducible gene-I-like receptors activations and ionizing radiation cotreatment on cytotoxicity against human non-small cell lung cancer in vitro. Oncol Lett. (2018) 15:4697–705. doi: 10.3892/ol.2018.7867
54. Poplineau M, Doliwa C, Schnekenburger M, Antonicelli F, Diederich M, Trussardi-Regnier A, et al. Epigenetically induced changes in nuclear textural patterns and gelatinase expression in human fibrosarcoma cells. Cell Prolif . (2013) 46:127–36. doi: 10.1111/cpr.12021
55. Bellia SR, Feliciani G, Duca MD, Monti M, Turri V, Sarnelli A, et al. Clinical evidence of abscopal effect in cutaneous squamous cell carcinoma treated with diffusing alpha emitters radiation therapy: a case report. J Contemp Brachytherapy. (2019) 11:449–57. doi: 10.5114/jcb.2019.88138
Keywords: PolyIC, polyethylenimine, decitabine, radiotherapy, immunotherapy, alpha radiation, triple-negative, breast carcinoma
Citation: Domankevich V, Efrati M, Schmidt M, Glikson E, Mansour F, Shai A, Cohen A, Zilberstein Y, Flaisher E, Galalae R, Kelson I and Keisari Y (2020) RIG-1-Like Receptor Activation Synergizes With Intratumoral Alpha Radiation to Induce Pancreatic Tumor Rejection, Triple-Negative Breast Metastases Clearance, and Antitumor Immune Memory in Mice. Front. Oncol. 10:990. doi: 10.3389/fonc.2020.00990
Received: 20 February 2020; Accepted: 19 May 2020;
Published: 17 July 2020.
Edited by:
Carsten Herskind, University of Heidelberg, GermanyReviewed by:
Gabi Niedermann, University of Freiburg, GermanyZhenkun Lou, Mayo Clinic, United States
Copyright © 2020 Domankevich, Efrati, Schmidt, Glikson, Mansour, Shai, Cohen, Zilberstein, Flaisher, Galalae, Kelson and Keisari. This is an open-access article distributed under the terms of the Creative Commons Attribution License (CC BY). The use, distribution or reproduction in other forums is permitted, provided the original author(s) and the copyright owner(s) are credited and that the original publication in this journal is cited, in accordance with accepted academic practice. No use, distribution or reproduction is permitted which does not comply with these terms.
*Correspondence: Yona Keisari, ykeisari@tauex.tau.ac.il