- 1Department of Radiology, Mallinckrodt Institute of Radiology, Washington University School of Medicine, St. Louis, MO, United States
- 2Program in Quantitative Molecular Therapeutics, Washington University School of Medicine, St. Louis, MO, United States
- 3Radiology Cyclotron Facility, Mallinckrodt Institute of Radiology, Washington University in St. Louis, St. Louis, MO, United States
- 4Department of Biomedical Engineering, Johns Hopkins University, Baltimore, MD, United States
- 5Department of Biomedical Engineering, Washington University in St. Louis, St. Louis, MO, United States
- 6Oncologic Imaging Program, Siteman Cancer Center, Washington University School of Medicine, St. Louis, MO, United States
Metastatic prostate cancer is incurable, and novel methods to detect the disease earlier and to direct definitive treatment are needed. Molecularly specific tools to localize diagnostic and cytotoxic radionuclide payloads to cancer cells and the surrounding microenvironment are recognized as a critical component of new approaches to combat this disease. The implementation of theranostic approaches to characterize and personalize patient management is beginning to be realized for prostate cancer patients. This review article summarized clinically translated approaches to detect, characterize, and treat disease in this rapidly expanding field.
Introduction
Prostate cancer (PCa) is the most common malignancy in men. In 2019 there were ~175,000 new cases of PC with >30,000 deaths, yielding enormous personal, societal, and economic costs (1). For many low-risk patients with primary PCa, “active surveillance” to monitor indolent disease by serial biopsy and prostate specific antigen (PSA) measures is an appropriate option. If treatment is desired for primary PCa, standards of care may involve surgical resection, external beam or proton radiotherapy, and brachytherapy and often are curative. For patients diagnosed with primary PCa, 5-year survival rates exceed 90%. However, for patients with advanced prostate cancer with tumor cells present at distant sites outside of the prostate there are severe impacts on quality of life and a low (<30%) 5-year survival rate. Upon metastasis to the bone, the most common site of PCa metastasis, the 5-year survival rate falls to a dismal 3–5%, making the disease essentially incurable and the second leading cause of cancer death in men (2–6). Conventional treatments for later-stage and metastatic disease can involve anti-hormonal therapies, chemotherapies, further use of radiation, and the use of bone-targeted agents.
An emerging area with significant potential to combat this lethal disease is the use of theranostic agents that detect PCa with exquisite sensitivity and can precisely ablate these sites. The implementation of an expanding array of nuclear medicine approaches to accurately characterize disease status enabling personalized patient management for prostate cancer patients is beginning to be realized. Advances in radionuclide production and availability, chemical synthesis, and clinical trial implementation are rapid and ongoing, and new tools and approaches will undoubtedly emerge in the near future.
In this review, we concisely present molecular imaging and theranostic tools that have been developed to better delineate, monitor, and treat prostate cancer, with a focus on clinically implemented radionuclide theranostics. There have been numerous changes in the management for prostate cancer patients and the options for treatment in the previous decade. In Figure 1A, a general schematic for the progression of disease in a patient is presented, by following their hypothetical disease burden as measured by their secreted PSA (prostate specific antigen) values. Focal treatment options are available for primary disease sites, followed by different systemic treatments at different disease states (Figure 1B). Recent approvals of several different pharmacological and radiological entities by the FDA/EMA, often for the same indications, underline the value for molecularly targeted imaging and therapeutics to guide and enhance patient outcomes.
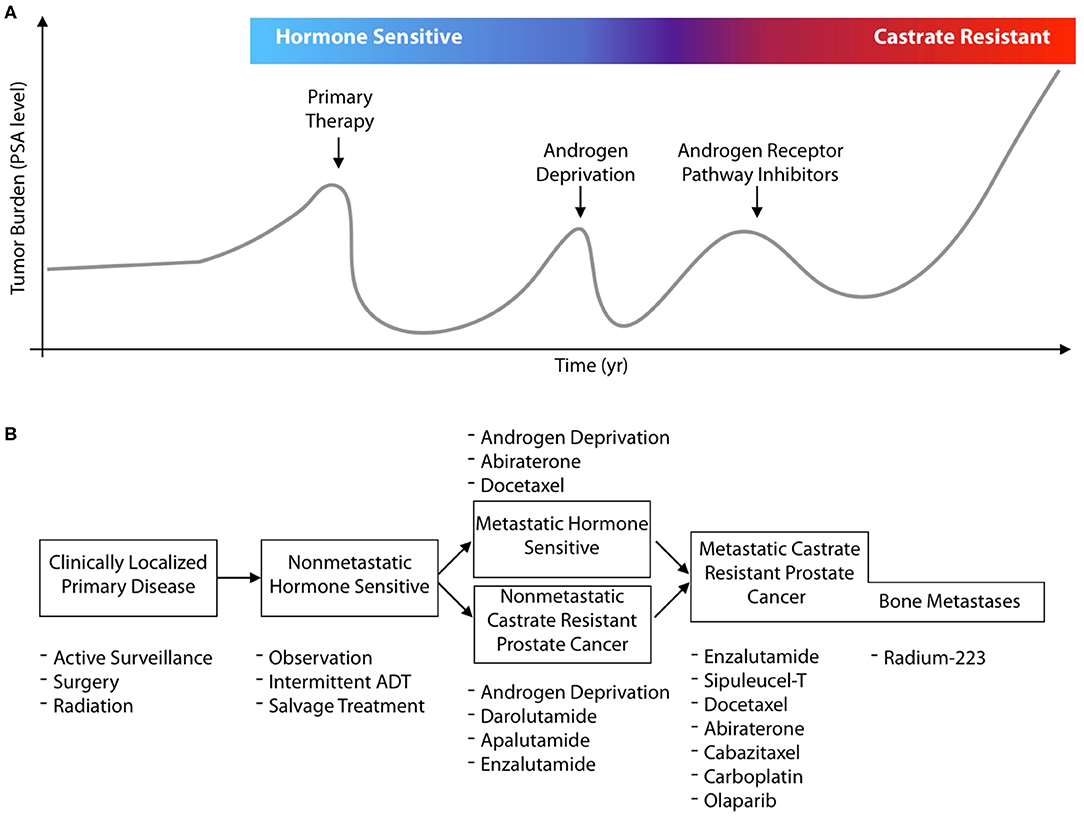
Figure 1. Contemporary prostate cancer states and systemic treatments. (A) Adenocarcinoma arising in the prostate gland is the second most common cancer in males, and can be cured with local intervention if detected when still localized to the organ. Disease is often detected by serum PSA measures which also enable tracking of disease recurrence should initial therapy and subsequent lines of treatment fail. Most commonly, disease control is attempted with hormonal control of the androgen receptor signaling access through (chemical) castration and the use of androgen receptor inhibitors. (B) The last decade has seen the approval of 8 new agents for prostate cancer across different disease states. These approvals result from significant survival benefits for patients at multiple lines of treatment. However, the eventual progression on novel antiandrogens, Radium-223, and chemotherapies leave considerable room for improvement. Despite the number of existing treatment options, efforts with molecularly targeted radiotherapies are under intense, global, evaluation; as well as to use new imaging agents to better guide drug development and more accurately characterize disease.
The present review helps provide context and an overview of the new options and methods leveraged to detect, characterize, and combat disease in this evolving clinical landscape. First, we describe methods using molecular imaging tools for the central oncological driver of the disease, the androgen receptor, to read out pharmacological properties of candidate hormonal therapy. Next, we describe efforts to target radiopharmaceuticals to receptors overexpressed on the surface of prostate cancer cells. Finally, we discuss efforts to target imaging and to direct treatment to the microenvironment of the bone-tropic metastases of this disease.
Androgen Receptor Imaging With [18F]-FDHT
The androgen receptor (AR), an intracellular DNA-binding, hormone-responsive transcription factor, is the key molecular driver for male organ development and is the oncological driver of PCa (7). Activated by binding androgens such as testosterone in the cytoplasm, the AR then translocates to the nucleus and stimulates the expression of genes involved in differentiation and proliferation (8). The effectiveness of repressing this central AR pathway by androgen-deprivation therapy was discovered by Huggins over 70-years ago (9, 10), and remains a mainstay of PCa treatment. However, after an initial response, AR pathway reactivation inevitably occurs, leading to disease progression. Recently developed, highly potent anti-androgen molecules can be employed to some effect, even in late-stage, castrate-resistant PCa [CRPC (11)]. This demonstrates that AR-signaling maintains its central role over the entire course of disease progression (9, 12), rather than CRPC becoming AR-signaling independent. Mechanisms of resistance to anti-androgen therapy in CRPC include AR-receptor gene amplification, AR-upregulation, local hormone production, and/or constitutively active AR-mutations (13–15). Counter intuitively, the term “castrate-resistant” most often reflects continued androgen dependence, rather than evolved AR-pathway independence. Thus, even in CRPC disease, the AR pathway thus remains an appropriate therapeutic and imaging target.
16β-[18F]-fluoro-5α-dihydrotestosterone ([18F]-FDHT) is a positron-emitting analog of the native AR-binding dihydrotestosterone with a conjugated positron-emitting radionuclide ([18F]; Figure 2A) and has been used in small animals studies (17, 18) and in clinical studies to evaluate AR-expression levels and occupancy (19–21). [18F]-FDHT positron emission tomography (PET) enables detection of metastatic lesions, as indicated by increased concentrations of AR, and is being evaluated for its capacity to phenotype lesions in concert with other conventional imaging modalities (22).
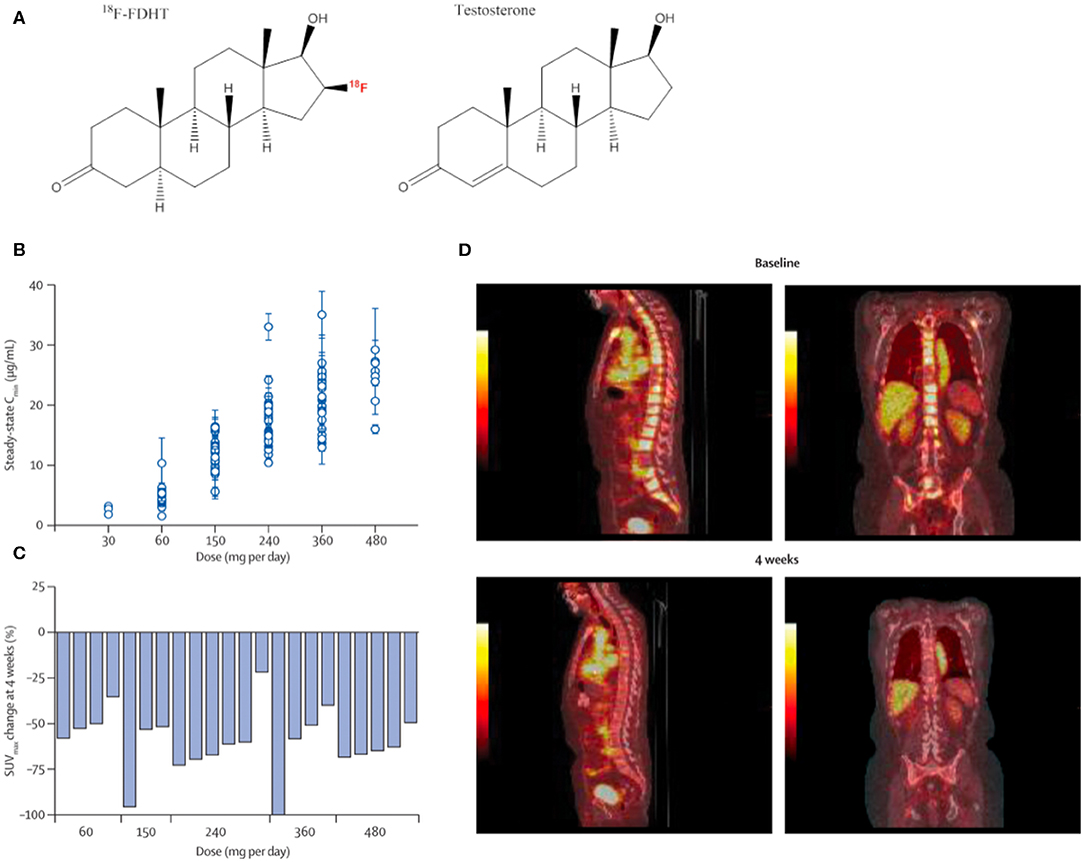
Figure 2. Quantitative imaging of the androgen receptor. [18F]-FDHT, which annotates the AR, the central molecular driver of prostate cancer development and progression, is an effective imaging tool to evaluate pharmacodynamic features of candidate therapies. MDV3100 treatment, a second-generation orally bioavailable anti-androgen, was tested in men with castrate-resistant prostate cancer. (A) Chemical structure of positron-emitting 18F-FDHT and testosterone. (B) MDV3100 serum concentration by dose level. (C) Representative baseline and treatment scans showing marked decrease in radio-androgen uptake in skeletal metastases. Reproduced from Scher et al. (16) (D) Change in standardized uptake value of [18F]-FDHT PET for men at baseline compared to 4 weeks of treatment.
The majority of CRPC escape anti-androgen therapy by AR-signaling amplification, and hormonal therapies that can inhibit AR-signaling are a mainstay of treatment for CRPC. Thus, imaging the expression levels of AR is a viable strategy to measure receptor density and the pharmacological response to these anti-androgen therapies (22, 23) (Figures 2B–D). These features have been exploited in the clinical evaluation of next generation anti-androgens in early clinical trial to directly quantitate AR-blockade. Reduction in [18F]-FDHT uptake and a plateau, consistent with saturation of AR binding, can be quantitated directly on a lesional or patient basis (16, 24).
Prostate Specific Membrane Antigen Imaging
Prostate Specific Membrane Antigen (PSMA) has emerged as the pre-eminent prostate cancer target for diagnostic imaging, assisting efforts to detect disease earlier, monitor recurrence, and track the progression of disease. PSMA is a robust target for PCa, with numerous pathological studies reporting elevated PSMA expression on 85–100% of prostate cancers (25–28). The number of clinical trials that use PSMA-targeted agents for diagnostic or therapeutic purposes is large and continues to expand: in the clinicaltrials.gov database, there are currently over 100 clinical trials that utilize PSMA for imaging or therapy.
PSMA is a type II transmembrane protein present on the cell surface, and, interestingly, PSMA contains an extracellular domain with glutamate carboxypeptidase activity (29). Although neither the exact physiological role of PSMA nor the reason for its overexpression on PCa cells is known, it has been used utilized in the targeting of multiple PSMA-binding small molecules, many of which are urea based and bind to the enzymatic site. We note that the use of PSMA as nomenclature to refer to this protein can be confusing, as this receptor is expressed in multiple non-prostate-derived tissues including the brain, peripheral nerves, salivary glands, gut, and kidney. Due to its independent discovery in multiple tissues, this protein, which we will refer to as PSMA, is also identified in the literature as glutamate carboxypeptidase II (GCPII) in the gut and as N-acetyl-L-aspartyl-L-glutamate peptidase I of NAAG peptidase I (NAALADase I) in the brain.
An array of agents have been developed that target PSMA with high affinity, including antibodies and small molecules. PSMA imaging agents for use in PET imaging and single-photon emitting computed tomography (SPECT) have been evaluated in preclinical and clinical settings, using an array of positron emitting radionuclides such as Fluorine-18, Gallium-68, Scandium-44 and Zirconium-89, or single-photon emitting radionuclides such as Technetium-99m and Iodine-125. Examples of widely tested structures and antibodies are found in Figure 3. Multiple PSMA imaging agents have been tested in humans and several have been evaluated in late stage, multicenter, clinical trials (Table 1).
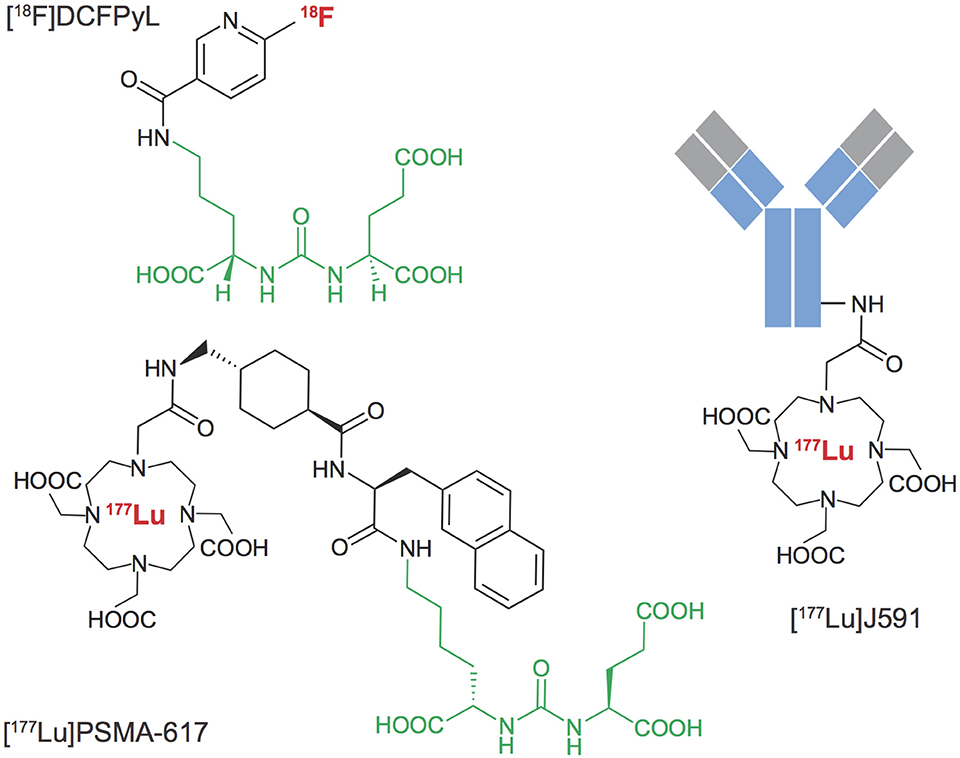
Figure 3. PSMA Targeted radiopharmaceuticals. Examples of diagnostic and therapeutic agents targeting Prostate Specific Membrane Antigen. A common urea-based motif (green) has been widely derivatized for localization at sites of prostate cancer metastases that over-express the membrane-localized PSMA metalloenzyme. Antibody targeting vectors with high affinity for PSMA have also been labeled with positron, beta-particle and alpha-particle emitting radionuclides.
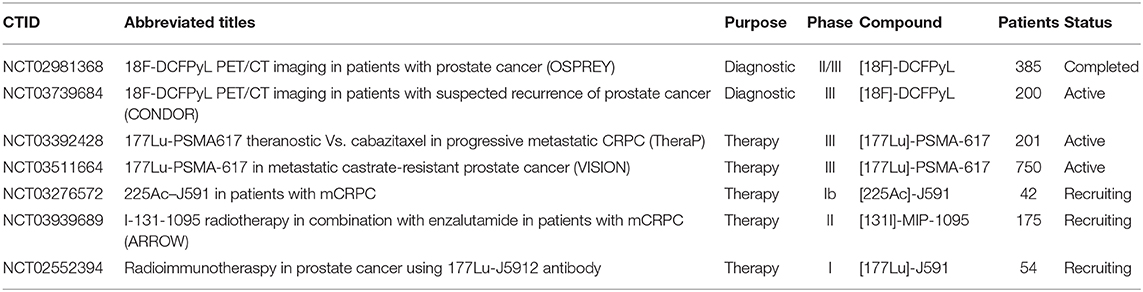
Table 1. Selected prostate cancer trials for disease detection and radiotherapy treatment of metastatic disease.
The first effort to target and image PSMA involved the 7E11-C5.3 murine antibody, which targets an intracellular epitope of PSMA (30, 31). Labeled with Indium-111, permitting imaging by SPECT, this agent was known as capromab pendetide or ProstaScint and was approved in 1996 for the detection of PCa lesions (32, 33). This initial PSMA-targeting agent found limited clinical implementation due to low contrast imaging, likely resulting from low accessibility in vivo to the intracellular PSMA epitope, concerns over human anti-murine immune responses, and competing conventional imaging methods.
A substantial improvement in PSMA imaging was achieved with the generation of J591, a humanized antibody targeting an epitope on the extracellular region of PSMA. J591 has been labeled with radionuclides for both PET and SPECT imaging of PCa lesions in humans (34–36). However, as antibody and antibody-fragment derived agents require administration of the radiolabeled agent several days before effective PSMA/tumor imaging can be performed, as this time is required for clearance of the tracer and optimal tumor/background signal. This relatively longer time frame from administration to imaging, which requires a return visit of the patient, has reduced enthusiasm for J591-based PSMA imaging.
Instead, much of the current intense interest in PCa-lesion imaging using PSMA revolves around PSMA-targeting small molecules (37). Small molecules can have exquisite targeting sensitivity and specificity, and they allow a much more rapid turnaround from agent injection to imaging (minutes to hours) than do antibodies (days). Also, small molecule agents that rapidly target PSMA allow the use of short-lived PET radionuclides, permitting high resolution, and sensitive detection with a reduced radiation dosed compared to antibody-based PET imaging methods (38, 39).
A large number of PSMA-targeted small molecules have been tested for PCa imaging in preclinical models and in humans. An example of a patient scan with the high affinity fluorinated agent is shown in Figure 4A. Multiple prospective clinical trials with targeted small-molecule PSMA imaging agents are underway, with encouraging results on the sensitivity of detection and the utility of these tracers in clinical settings (42–44). The most widely reported clinical imaging has been performed with PET imaging using radiometal-labeled [68Ga]PSMA 617 or [68Ga]PSMA-11 or using fluorinated [18F]-DCFPyL (45). There are currently many options for radionuclide and PSMA-targeting ligand, and several studies to compare imaging features have been undertaken. Optimal uptake time, magnitude of tumor and background organ uptake, and imaging resolution are dependent on ligand and radionuclide. Logistical and cost issues are also a consideration. Multiple doses of Fluorine-18 radiolabeled inhibitor are available at institutions with a medical cyclotron, with the ability to ship agent at greater distances. Lower upfront costs are required for Gallium-68 isolation on a 68Ge/68Ga generator system, however fewer doses are produced with lower specific activity, and decreased resolution (46–49).
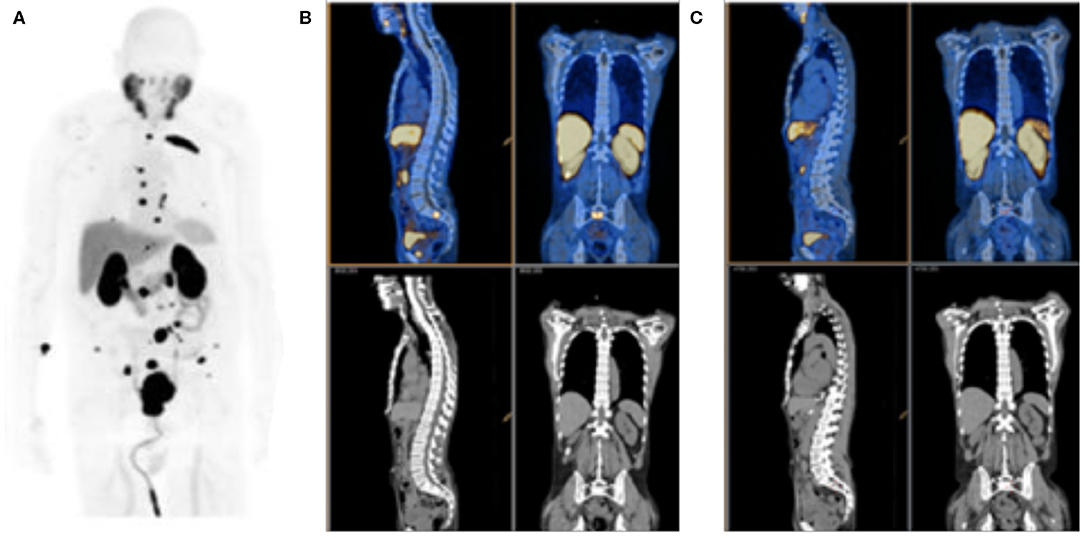
Figure 4. PSMA PET Imaging. (A) Detection of prostate cancer lesions with [18F]DCFPyL, along with uptake in kidneys, salivary gland and liver. (B) Pre-treatment [68Ga]PSMA-11 and (C) post-treatment PET/CT, top, and CT, bottom, scans obtained on day 9 after initiating combined anti-androgen hormone therapy. Reproduced from (A)(40) and (B,C) (41).
The capacity to perform highly sensitive molecular imaging of PSMA to detect minute foci of metastatic disease, led by PET-isotope labeled, PSMA-targeted small molecules, is motivating a paradigm shift in prostate cancer patient management. For example, the ability to rapidly determine lesion response on a given treatment regimen (41, 50, 51), as shown in Figures 4B,C, or to target external beam radiotherapy to sites of oligometastatic disease (52, 53), are means of precise disease control not previously possible. Of course, it should be noted that rigorous, prospective, controlled and multi-center trials, and statistical analyses are required before we can be confident that these new tools provide real-world benefit for patients.
Prostate Specific Membrane Antigen Targeted Radiotherapy
Concurrent with the development of the PSMA-targeted diagnostic agents described above, the application of PSMA-directed targeted radiotherapy of PCa is an ongoing area of great potential. Here, PSMA-binding ligands are labeled with radionuclides that produce potent cytotoxic decay products, without or with the co-emission of imageable photons. Both beta-particle (Lutetium-177, Copper-67, Iodine-131) and alpha-particle (Bismuth-213 and Actinium-225) emitting PSMA-targeted agents are at various stages of drug development (54–58). It is noteworthy that PSMA-ligands are internalized by endocytosis after PSMA binding, allowing increased intracellular levels of residualizing therapeutic radionuclides and improved potential for tumor-cell killing. Several PSMA-targeted radiotherapeutic agents have begun to be applied in late stage metastatic prostate cancer (PCa) patients (58–62); Table 1.
The most developed agent in the PSMA-targeted radiotherapeutic class is [177Lu]PSMA-617. [177Lu]PSMA-617 is inherently theranostic in that the decay pathway of 177Lu emits both tumor-cell-killing beta particles and imageable photons, detectable by planar scintography, and SPECT imaging. Thus, [177Lu]PSMA-617 allows both therapy and imaging of agent distribution and uptake with the same agent. The majority of response data for this agent have been accrued from retrospective trials that have shown efficacy in reducing PSA levels with manageable hematological, renal, and salivary gland toxicity (63–69). Patient characteristics varied to a large degree with respect to previous treatment, disease stage, and radiographic and biochemical features (70). Thus, these results are encouraging as they indicate that there may be a benefit for patients along the spectrum of disease burden and stage, but also present a problem as informing to how these agents can be wielded most effectively. To aid in answering these questions, well-powered prospective trials with [177Lu]PSMA-617 are now underway.
Great interest has also been generated by the application of PSMA-617 labeled with 225Ac, which emits four cell-killing alpha particles. This agent has been studied in small cohorts of men in Germany and in South Africa and clinical findings have generated great interest, Figure 5 (58, 71, 72). Alpha particles emitted from heavy isotopes, such as Actinium-225 and Radium-223 (described below), have high energies, in the 5-8 MeV range, that produce extremely cytotoxic genomic damage. Alpha particles also exhibit a much shorter path length than β-particles, such as those emitted by Lutetium-177. Together, the radiobiological properties of alpha-particle emitting agents mean that even small deposits of cancer cells could be eradicated with appropriate uptake (57), while largely sparing adjacent and distant tissues because of their short path length. Investigations with additional alpha-particle emitting theranostics, including with generator-produced [213Bi]PSMA-617 and [212Pb]PSMA-617 (57, 73), are also being evaluated, which may alleviate sourcing issues regarding 225Ac.
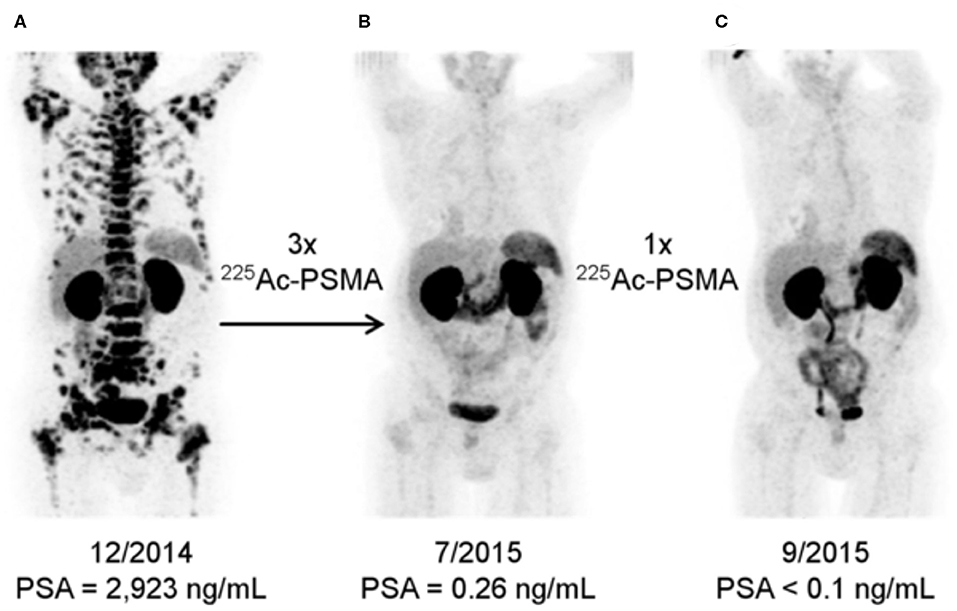
Figure 5. [68Ga]PSMA-11 Imaging Response to [225Ac]PSMA-617. Alpha-particle emitting Ac-225 bound to the DOTA chelator-bearing PSMA-617 scaffold was used to treat a patient following disease progression after several lines of conventional treatment and [177Lu]PSMA-617. Serial images during the treatment course reveal significantly less PSMA expression and potential lesion eradication. A decrease in salivary gland uptake is also noted, as it is a site of PSMA expression inadvertently treated by these agents. Reproduced from (71).
However, the expression of PSMA on non-prostate-derived cells as noted above leads to a major concern in the application of PSMA-targeted therapies: the on-target but off-tumor localization. Dosimetric evaluation of the distribution of the diagnostic and theranostic ligands provides a means to predict absorbed doses to sites of both healthy and diseased tissue uptake. Radiosensitive organs, such as the hematopoietic niche of the bone marrow, kidneys, nerves, and intestine often account for the dose-limiting activity that can safely be administered to a patient (74, 75). The major side-effect producing sites of undesired localization of PSMA-targeted agents identified at this time include the salivary glands and kidney, which highly express PSMA, are radiosensitive and are critical for survival and quality of life. Xerostomia from non-repairable damage to the salivary glands has been reported with 225Ac-PSMA-617, with varying degrees of glandular damage observed with 177Lu-PSMA-617. Interestingly, biological targeting vectors such as 177Lu-J591 which do not apparently accumulate in the salivary glands may have additional utility with the potential for a favoragle therapeutic window (76, 77). The much longer-onset effect of severe kidney toxicity has not yet been clinically noted (63). However, studies to date generally lack rigorous patient enrollment and follow-up criteria and have not followed patients for long periods of time. Prospective and randomized trials will be needed to compare patient benefit against conventional treatments and the effectiveness of means to reduce these off-tumor toxicities.
GRPR Bombesin
The gastrin-releasing peptide receptor (GRPR) is expressed on a wide range of cell types in higher mammals, especially in the nervous system and gastrointesinal tract (78, 79). Small peptides interact with GPRP to modulate a wide range of cell and organ functions (80). GRPR is aberrantly overexpressed on the cell surface of many cancers, including lung, breast, and prostate cancer (81, 82). The bombesin subfamily is the best studied GRPR, and a large number of mammalian and amphibian bombesin peptide analogs have been radiolabeled for cancer imaging and therapy (82).
Because of the frequent overexpression of GRPR in prostate cancer, several bombesin radiopharmaceuticals have been tested in humans for PCa disease detection, including [68Ga]RM2, [68Ga]BAY86-7548, and [64Cu]-CB-TE2A-AR06, among others. Studies reveal high-contrast detection of disseminated and primary disease (83–86). While GRPR expression may not be as ubiquitous on prostate cancer cells as PSMA, there is no background target expression in the kidneys or salivary glands. Thus, there may be utility for other prostate cancer targeted theranostics in addition to PSMA (87), especially in the GRPR class.
Theranostics for the Bone Metastasic Microenvironment
The skeletal compartment is the most frequent site of metastases in prostate cancer patients. These lesions are often painful, and may also further degrade quality of life through fracture, spinal cord compression, hypercalcemia, and impaired mobility (3, 88). Bone metastases occupy a nutrient-rich niche that enhances the treatment-resistant nature of disseminated tumor cells (89). Early detection and specific localized treatment of these disseminated sites are recognized as necessary components of a successful strategy to combat bone metastatic prostate cancer.
Conventional imaging modalities for PCa bone metastases include magnetic resonance and X-ray computed tomography, which are commonly applied in concert with nuclear medicine scans for accurate bone lesion detection. [99mTc]-bisphosphonates and [18F]-NaF are both bone-seeking agents that are incorporated at sites of active bone remodeling adjacent to metastatic foci and are used for imaging. Approved beta particle-emitting agents for bone pain palliation are [89Sr]chloride and [153Sm]EDTMP, an ion and phosphonic acid ligand, respectively, which are taken up at or near sites of bone metastasis. Both produce imageable emissions for planar imaging in order to evaluate uptake. Unfortunately, the long path length of these energetic beta particle emissions result in irradiation of the bone marrow, a dose limiting organ, and these agents have not produce survival improvements when evaluated in clinical trial.
The first bone-targeted radionuclide that aids in pain palliation and also achieves an overall survival benefit over standard of care is the alpha-particle emitting [223Ra]Cl2 citrate, tradename Xofigo (90). Radium-223 is a calcium-mimetic and localizes to sites of active bone turnover, where it subsequently decays, irradiating nearby prostate cancer cells. The short path lengths of the alpha particles do not result in anemic responses and the drug is well-tolerated. While difficult to image, efforts are underway to provide quantitative assessment of 223RaCl2 distribution to inform absorbed dose measures at sites of disease and background organs (91–94).
Conclusion
Agents that specifically and sensitively target molecular features of prostate cancer are being brought to bear to detect, guide, and deliver treatments for men with advanced prostate cancer. The underlying initiator of PCa, the androgen receptor, is the driver of prostate adenocarcinoma development, can be visualized with [18F]-FDHT. This imaging tool can evaluate the pharmacological impact of anti-androgens and their efficacy. Cell surface antigen targeted agents, in particular PSMA targeted urea-based ligands and antibodies, have now been assessed in a wide range of scenarios to detect and treat metastatic prostate cancer. Prospective clinical trials that are currently recruiting or underway will provide clearer information on the utility of these new theranostic approaches to improve quality of life and overall survival. These agents have the capacity to deliver ablative doses to sites of disease throughout the body with the potential to overcome this currently incurable disease. Differences in the imaging properties and therapeutic niche for different molecular entities and radionuclides are of continuing research interest to provide optimal patient-specific diagnostic information and therapeutic outcome. These ongoing trials may also shed light on the important question of how these novel imaging and therapy agents will integrate with current treatment modalities and approved imaging methods, including conventional imaging and imaging of prostate cancer cell metabolism with established agents such as [11C/18F]-choline, [18F]-fluorodeoxyglucose and [18F]-fluciclovine.
Author Contributions
All authors listed have made a substantial, direct and intellectual contribution to the work, and approved it for publication.
Funding
This work was supported in part by NIH NCI R01CA229893, R01CA201035, and R01CA240711 (DT); and the American Cancer Society (HZ).
Conflict of Interest
The authors declare that the research was conducted in the absence of any commercial or financial relationships that could be construed as a potential conflict of interest.
References
1. Siegel RL, Miller KD, Jemal A. Cancer statistics, 2018. CA Cancer J Clin. (2018) 68:7–30. doi: 10.3322/caac.21442
2. Nørgaard M, Jensen AØ, Jacobsen JB, Cetin K, Fryzek JP, Sørensen HT. Skeletal related events, bone metastasis and survival of prostate cancer: a population based cohort study in Denmark (1999 to 2007). J Urol. (2010) 184:162–7. doi: 10.1016/j.juro.2010.03.034
3. Bubendorf L, Schöpfer A, Wagner U, Sauter G, Moch H, Willi N, et al. Metastatic patterns of prostate cancer: an autopsy study of 1,589 patients. Hum Pathol. (2000) 31:578–83. doi: 10.1053/hp.2000.6698
4. Rajpar S, Fizazi K. Bone targeted therapies in metastatic castration-resistant prostate cancer. Cancer J. (2013) 19:66–70. doi: 10.1097/PPO.0b013e31827f123e
5. Mehra R, Kumar-Sinha C, Shankar S, Lonigro RJ, Jing X, Philips NE, et al. Characterization of bone metastases from rapid autopsies of prostate cancer patients. Clin Cancer Res. (2011) 17:3924–32. doi: 10.1158/1078-0432.CCR-10-3120
6. Lilja H, Ulmert D, Vickers AJ. Prostate-specific antigen and prostate cancer: prediction, detection and monitoring. Nat Rev Cancer. (2008) 8:268–78. doi: 10.1038/nrc2351
7. Augello MA, Den RB, Knudsen KE. AR function in promoting metastatic prostate cancer. Cancer Metastasis Rev. (2014) 33:399–411. doi: 10.1007/s10555-013-9471-3
8. Carver BS. Strategies for targeting the androgen receptor axis in prostate cancer. Drug Discov Today. (2014) 19:1493–97. doi: 10.1016/j.drudis.2014.07.008
9. Huggins C, Hodges CV. Studies on prostatic cancer. I. The effect of castration, of estrogen and of androgen injection on the normal and on the hyperplastic prostate glands of dogs. Cancer Res. (1941) 1:293–8.
10. Huggins C, Hodges CV. Studies on prostatic cancer: I. The effect of castration, of estrogen and of androgen injection on serum phosphatases in metastatic carcinoma of the prostate. 1941. J Urol. (2002) 168:9–12. doi: 10.1016/S0022-5347(05)64820-3
11. Teo MY, Rathkopf DE, Kantoff P. Treatment of advanced prostate cancer. Annu Rev Med. (2019) 70:479–99. doi: 10.1146/annurev-med-051517-011947
12. Ferraldeschi R, Welti J, Luo J, Attard G, de Bono JS. Targeting the androgen receptor pathway in castration-resistant prostate cancer: progresses and prospects. Oncogene. (2015) 34:1745–57. doi: 10.1038/onc.2014.115
13. Pfitzenmaier J, Ellis WJ, Hawley S, Arfman EW, Klein JR, Lange PH, et al. The detection and isolation of viable prostate-specific antigen positive epithelial cells by enrichment: a comparison to standard prostate-specific antigen reverse transcriptase polymerase chain reaction and its clinical relevance in prostate cancer. In: Urologic Oncology: Seminars and Original Investigations. New York, NY: Elsevier (2007). doi: 10.1016/j.urolonc.2006.09.018
14. Koivisto P, Kononen J, Palmberg C, Tammela T, Hyytinen E, Isola J, et al. Androgen receptor gene amplification: a possible molecular mechanism for androgen deprivation therapy failure in prostate cancer. Cancer Res. (1997) 57:314–9.
15. Pedersen EA, Shiozawa Y, Pienta KJ, Taichman RS. The prostate cancer bone marrow niche: more than just “fertile soil”. Asian J Androl. (2012) 14:423–7. doi: 10.1038/aja.2011.164
16. Scher HI, Beer TM, Higano CS, Anand A, Taplin ME, Efstathiou E, et al. Prostate cancer foundation/department of defense prostate cancer clinical trials consortium. antitumour activity of MDV3100 in castration-resistant prostate cancer: a phase 1-2 study. Lancet. (2010) 375:1437–46. doi: 10.1016/S0140-6736(10)60172-9
17. Larimer BM, Dubois F, Bloch E, Nesti S, Placzek M, Zadra G, et al. Specific 18F-FDHT accumulation in human prostate cancer xenograft murine models is facilitated by prebinding to sex hormone-binding globulin. J Nucl Med. (2018) 59:1538–43. doi: 10.2967/jnumed.118.208785
18. Bonasera TA, O'Neil JP, Xu M, Dobkin JA, Cutler PD, Lich LL, et al. Preclinical evaluation of fluorine-18-labeled androgen receptor ligands in baboons. J Nucl Med. (1996) 37:1009–15.
19. Miederer M, McDevitt MR, Sgouros G, Kramer K, Cheung NKV, Scheinberg DA. Pharmacokinetics, dosimetry, and toxicity of the targetable atomic generator, 225Ac-HuM195, in nonhuman primates. J Nucl Med. (2004) 45:129–37.
20. Dehdashti F, Picus J, Michalski JM, Dence CS, Siegel BA, Katzenellenbogen JA, et al. Positron tomographic assessment of androgen receptors in prostatic carcinoma. Eur J Nucl Med Mol Imaging. (2005) 32:344–50. doi: 10.1007/s00259-005-1764-5
21. Larson SM, Morris M, Gunther I, Beattie B, Humm JL, Akhurst TA, et al. Tumor localization of 16β-18F-fluoro-5α-dihydrotestosterone versus 18F-FDG in patients with progressive, metastatic prostate cancer. J Nucl Med. (2004) 45:366–73.
22. Fox JJ, Gavane SC, Blanc-Autran E, Nehmeh S, Gönen M, Beattie B, et al. Positron emission tomography/computed tomography-based assessments of androgen receptor expression and glycolytic activity as a prognostic biomarker for metastatic castration-resistant prostate cancer. JAMA Oncol. (2018) 4:217–24. doi: 10.1001/jamaoncol.2017.3588
23. Hoving H, Palthe S, Vallinga M, Dost R, Gietema JA, Antunes I, et al. Early 18F-FDHT PET/CT as a predictor of treatment response in mCRPC treated with enzalutamide. J Clin Orthod. (2019) 37:232. doi: 10.1200/JCO.2019.37.7_suppl.232
24. Rathkopf DE, Morris MJ, Fox JJ, Danila DC, Slovin SF, Hager JH, et al. Phase I study of ARN-509, a novel antiandrogen, in the treatment of castration-resistant prostate cancer. J Clin Oncol. (2013) 31:3525–30. doi: 10.1200/JCO.2013.50.1684
25. Wright GL Jr, Haley C, Beckett ML, Schellhammer PF. Expression of prostate-specific membrane antigen in normal, benign, and malignant prostate tissues. Urol Oncol. (1995) 1:18–28. doi: 10.1016/1078-1439(95)00002-Y
26. Bostwick DG, Pacelli A, Blute M, Roche P, Murphy GP. Prostate specific membrane antigen expression in prostatic intraepithelial neoplasia and adenocarcinoma: a study of 184 cases. Cancer. (1998) 82:2256–61. doi: 10.1002/(SICI)1097-0142(19980601)82:11<2256::AID-CNCR22>3.0.CO;2-S
27. Kusumi T, Koie T, Tanaka M, Matsumoto K, Sato F, Kusumi A, et al. Immunohistochemical detection of carcinoma in radical prostatectomy specimens following hormone therapy. Pathol Int. (2008) 58:687–94. doi: 10.1111/j.1440-1827.2008.02294.x
28. Mannweiler S, Amersdorfer P, Trajanoski S, Terrett JA, King D, Mehes G. Heterogeneity of prostate-specific membrane antigen (PSMA) expression in prostate carcinoma with distant metastasis. Pathol Oncol Res. (2009) 15:167–72. doi: 10.1007/s12253-008-9104-2
29. Barinka C, Rojas C, Slusher B, Pomper M. Glutamate carboxypeptidase II in diagnosis and treatment of neurologic disorders and prostate cancer. Curr Med Chem. (2012) 19:856–70. doi: 10.2174/092986712799034888
30. Horoszewicz JS, Kawinski E, Murphy GP. Monoclonal antibodies to a new antigenic marker in epithelial prostatic cells and serum of prostatic cancer patients. Anticancer Res. (1987) 7:927–35
31. Wynant GE, Murphy GP, Horoszewicz JS, Neal CE, Collier BD, Mitchell E, et al. Immunoscintigraphy of prostatic cancer: preliminary results with 111In-labeled monoclonal antibody 7E11-C5.3 (CYT-356). Prostate. (1991) 18:229–41. doi: 10.1002/pros.2990180305
32. Hinkle GH, Burgers JK, Neal CE, Texter JH, Kahn D, Williams RD, et al. Multicenter radioimmunoscintigraphic evaluation of patients with prostate carcinoma using indium-111 capromab pendetide. Cancer: Interdiscipl Int J Am Cancer Soc. (1998) 83:739–47. doi: 10.1002/(SICI)1097-0142(19980815)83:4<739::AID-CNCR16>3.0.CO;2-T
33. Manyak MJ, Hinkle GH, Olsen JO, Chiaccherini RP, Partin AW, Piantadosi S, et al. Immunoscintigraphy with indium-111-capromab pendetide: evaluation before definitive therapy in patients with prostate cancer. Urology. (1999) 54:1058–63. doi: 10.1016/S0090-4295(99)00314-3
34. Osborne JR, Green DA, Spratt DE, Lyashchenko S, Fareedy SB, Robinson BD, et al. A Prospective pilot study of 89Zr-J591/prostate specific membrane antigen positron emission tomography in men with localized prostate cancer undergoing radical prostatectomy. J Urol. (2014) 191:1439–45. doi: 10.1016/j.juro.2013.10.041
35. Bander NH, Trabulsi EJ, Kostakoglu L, Yao D, Vallabhajosula S, Smith-Jones P, et al. Targeting metastatic prostate cancer with radiolabeled monoclonal antibody J591 to the extracellular domain of prostate specific membrane antigen. J Urol. (2003) 170:1717–21. doi: 10.1097/01.ju.0000091655.77601.0c
36. Morris MJ, Divgi CR, Pandit-Taskar N, Batraki M, Warren N, Nacca A, et al. Pilot trial of unlabeled and indium-111-labeled anti-prostate-specific membrane antigen antibody J591 for castrate metastatic prostate cancer. Clin Cancer Res. (2005) 11:7454–61. doi: 10.1158/1078-0432.CCR-05-0826
37. Rowe SP, Gorin MA, Pomper MG. Imaging of prostate-specific membrane antigen with small-molecule PET radiotracers: from the bench to advanced clinical applications. Annu Rev Med. (2019) 70:461–77. doi: 10.1146/annurev-med-062117-073027
38. Pandit-Taskar N, O'Donoghue JA, Beylergil V, Lyashchenko S, Ruan S, Solomon SB, et al. 89Zr-huJ591 immuno-PET imaging in patients with advanced metastatic prostate cancer. Eur J Nucl Med Mol Imaging. (2014) 41:2093–105. doi: 10.1007/s00259-014-2830-7
39. Pandit-Taskar N, O'Donoghue JA, Durack JC, Lyashchenko SK, Cheal SM, Beylergil V, et al. A phase I/II study for analytic validation of 89Zr-J591 immunoPET as a molecular imaging agent for metastatic prostate cancer. Clin Cancer Res. (2015) 21:5277–85. doi: 10.1158/1078-0432.CCR-15-0552
40. Szabo Z, Mena E, Rowe SP, Plyku D, Nidal R, Eisenberger MA, et al. Initial evaluation of [(18)F]DCFPyL for prostate-specific membrane antigen (PSMA)-targeted PET imaging of prostate cancer. Mol Imaging Biol. (2015) 17:565–74. doi: 10.1007/s11307-015-0850-8
41. Emmett L, Yin C, Crumbaker M, Hruby G, Kneebone A, Epstein R, et al. Rapid modulation of PSMA expression by androgen deprivation: serial 68Ga-PSMA-11 PET in men with hormone-sensitive and castrate-resistant prostate cancer commencing androgen blockade. J Nucl Med. (2019) 60:950–4. doi: 10.2967/jnumed.118.223099
42. Rousseau E, Wilson D, Lacroix-Poisson F, Krauze A, Chi K, Gleave M, et al. A prospective study on 18F-DCFPyL PSMA PET/CT imaging in biochemical recurrence of prostate cancer. J Nucl Med. (2019) 60:1587–93. doi: 10.2967/jnumed.119.226381
43. Calais J, Czernin J, Fendler WP, Elashoff D, Nickols NG. Randomized prospective phase III trial of 68Ga-PSMA-11 PET/CT molecular imaging for prostate cancer salvage radiotherapy planning [PSMA-SRT]. BMC Cancer. (2019) 19:18. doi: 10.1186/s12885-019-5297-x
44. McCarthy M, Francis R, Tang C, Watts J, Campbell A. A multicenter prospective clinical trial of 68gallium PSMA HBED-CC PET-CT restaging in biochemically relapsed prostate carcinoma: oligometastatic rate and distribution compared with standard imaging. Int J Radiat Oncol Biol Phys. (2019) 104:801–8. doi: 10.1016/j.ijrobp.2019.03.014
45. Chen Y, Pullambhatla M, Foss CA, Byun Y, Nimmagadda S, Senthamizhchelvan S, et al. 2-(3-{1-Carboxy-5-[(6-[18F]fluoro-pyridine-3-carbonyl)-amino]-pentyl}-ureido)-pentanedioic acid, [18F]DCFPyL, a PSMA-based PET imaging agent for prostate cancer. Clin Cancer Res. (2011) 17:7645–53. doi: 10.1158/1078-0432.CCR-11-1357
46. Rosar F, Buchholz HG, Michels S, Hoffmann MA, Piel M, Waldmann CM, et al. Image quality analysis of 44Sc on two preclinical PET scanners: a comparison to 68Ga. EJNMMI Phys. (2020) 7:16. doi: 10.1186/s40658-020-0286-3
47. Eppard E, de la Fuente A, Benešová M, Khawar A, Bundschuh RA, Gärtner FC, et al. Clinical translation and first in-human use of [44Sc]Sc-PSMA-617 for PET imaging of metastasized castrate-resistant prostate cancer. Theranostics. (2017) 7:4359–69. doi: 10.7150/thno.20586
48. Werner RA, Derlin T, Lapa C, Sheikbahaei S, Higuchi T, Giesel FL, et al. 18F-labeled, PSMA-targeted radiotracers: leveraging the advantages of radiofluorination for prostate cancer molecular imaging. Theranostics. (2020) 10:1–16. doi: 10.7150/thno.37894
49. Ferreira G, Iravani A, Hofman MS, Hicks RJ. Intra-individual comparison of 68Ga-PSMA-11 and 18F-DCFPyL normal-organ biodistribution. Cancer Imaging. (2019) 19:23. doi: 10.1186/s40644-019-0211-y
50. Siva S, Murphy D, Hofman MS, Tran B. 223P Using PSMA PET/CT to assess response in metastatic prostate cancer (mPC) patients (pts) receiving upfront chemohormonal therapy. Ann Oncol. (2018) 29:mdy434–011. doi: 10.1093/annonc/mdy434.011
51. Plouznikoff N, Artigas C, Sideris S, Martinez Chanza N, Gil T, Peltier A, et al. Evaluation of PSMA expression changes on PET/CT before and after initiation of novel antiandrogen drugs (enzalutamide or abiraterone) in metastatic castration-resistant prostate cancer patients. Ann Nucl Med. (2019) 33:945–54. doi: 10.1007/s12149-019-01404-2
52. Phillips RM, Gorin MA, Rowe SP, Pomper MG, Pienta KJ, Ross AE, et al. Complete biochemical response after stereotactic ablative radiotherapy of an isolated prostate cancer pelvic soft tissue recurrence detected by 18F-DCFPyL PET/CT. Urol Case Rep. (2018) 16:86–8. doi: 10.1016/j.eucr.2017.11.021
53. Phillips R, Lim SJ, Shi WY, Antonarakis ES, Rowe S, Gorin M, et al. Primary outcomes of a phase II randomized trial of observation versus stereotactic ablative radiation for oligometastatic prostate cancer (ORIOLE). Int J Radiat Oncol Biol Phys. (2019) 105:681. doi: 10.1016/j.ijrobp.2019.08.031
54. Rahbar K, Ahmadzadehfar H, Kratochwil C, Haberkorn U, Schäfers M, Essler M, et al. German multicenter study investigating 177Lu-PSMA-617 radioligand therapy in advanced prostate cancer patients. J Nucl Med. (2017) 58:85–90. doi: 10.2967/jnumed.116.183194
55. Kelly JM, Amor-Coarasa A, Ponnala S, Nikolopoulou A, Williams C Jr, Thiele NA, et al. A single dose of 225Ac-RPS-074 induces a complete tumor response in an LNCaP xenograft model. J Nucl Med. (2019) 60:649–55. doi: 10.2967/jnumed.118.219592
56. Zechmann CM, Afshar-Oromieh A, Armor T, Stubbs JB, Mier W, Hadaschik B, et al. Radiation dosimetry and first therapy results with a 124I/131I-labeled small molecule (MIP-1095) targeting PSMA for prostate cancer therapy. Eur J Nucl Med Mol Imaging. (2014) 41:1280–92. doi: 10.1007/s00259-014-2713-y
57. Sathekge M, Knoesen O, Meckel M, Modiselle M, Vorster M, Marx S. 213Bi-PSMA-617 targeted alpha-radionuclide therapy in metastatic castration-resistant prostate cancer. Eur J Nucl Med Mol Imaging. (2017) 44:1099–100. doi: 10.1007/s00259-017-3657-9
58. Kratochwil C, Bruchertseifer F, Rathke H, Hohenfellner M, Giesel FL, Haberkorn U, et al. Targeted α-therapy of metastatic castration-resistant prostate cancer with 225Ac-PSMA-617: swimmer-plot analysis suggests efficacy regarding duration of tumor control. J Nucl Med. (2018) 59:795–802. doi: 10.2967/jnumed.117.203539
59. Tagawa ST, Milowsky MI, Morris M, Vallabhajosula S, Christos P, Akhtar NH, et al. Phase II study of lutetium-177–labeled anti-prostate-specific membrane antigen monoclonal antibody J591 for metastatic castration-resistant prostate cancer. Clin Cancer Res. (2013) 19:5182–91. doi: 10.1158/1078-0432.CCR-13-0231
60. Kratochwil C, Giesel FL, Stefanova M, Benešová M, Bronzel M, Afshar-Oromieh A, et al. PSMA-targeted radionuclide therapy of metastatic castration-resistant prostate cancer with 177Lu-labeled PSMA-617. J Nucl Med. (2016) 57:1170–6. doi: 10.2967/jnumed.115.171397
61. Bander NH, Milowsky MI, Nanus DM, Kostakoglu L, Vallabhajosula S, Goldsmith SJ. Phase I trial of 177lutetium-labeled J591, a monoclonal antibody to prostate-specific membrane antigen, in patients with androgen-independent prostate cancer. J Clin Oncol. (2005) 23:4591–601. doi: 10.1200/JCO.2005.05.160
62. Milowsky MI, Nanus DM, Kostakoglu L, Vallabhajosula S, Goldsmith SJ, Bander NH. Phase I trial of yttrium-90-labeled anti-prostate-specific membrane antigen monoclonal antibody J591 for androgen-independent prostate cancer. J Clin Oncol. (2004) 22:2522–31. doi: 10.1200/JCO.2004.09.154
63. Yadav MP, Ballal S, Tripathi M, Damle NA, Sahoo RK, Seth A, et al. 177Lu-DKFZ-PSMA-617 therapy in metastatic castration resistant prostate cancer: safety, efficacy, and quality of life assessment. Eur J Nucl Med Mol Imaging. (2017) 44:81–91. doi: 10.1007/s00259-016-3481-7
64. Rahbar K, Schmidt M, Heinzel A, Eppard E, Bode A, Yordanova A, et al. Response and tolerability of a single dose of 177Lu-PSMA-617 in patients with metastatic castration-resistant prostate cancer: a multicenter retrospective analysis. J Nucl Med. (2016) 57:1334–8. doi: 10.2967/jnumed.116.173757
65. Rahbar K, Boegemann M, Yordanova A, Eveslage M, Schäfers M, Essler M, et al. PSMA targeted radioligandtherapy in metastatic castration resistant prostate cancer after chemotherapy, abiraterone and/or enzalutamide. a retrospective analysis of overall survival. Eur J Nucl Med Mol Imaging. (2018) 45:12–9. doi: 10.1007/s00259-017-3848-4
66. Ferdinandus J, Eppard E, Gaertner FC, Kürpig S, Fimmers R, Yordanova A, et al. Predictors of response to radioligand therapy of metastatic castrate-resistant prostate cancer with 177Lu-PSMA-617. J Nucl Med. (2017) 58:312–9. doi: 10.2967/jnumed.116.178228
67. Ahmadzadehfar H, Wegen S, Yordanova A, Fimmers R, Kürpig S, Eppard E, et al. Overall survival and response pattern of castration-resistant metastatic prostate cancer to multiple cycles of radioligand therapy using [177Lu]Lu-PSMA-617. Eur J Nucl Med Mol Imaging. (2017) 44:1448–54. doi: 10.1007/s00259-017-3716-2
68. Ahmadzadehfar H, Schlolaut S, Fimmers R, Yordanova A, Hirzebruch S, Schlenkhoff C, et al. Predictors of overall survival in metastatic castration-resistant prostate cancer patients receiving [177Lu]Lu-PSMA-617 radioligand therapy. Oncotarget. (2017) 8:103108–16. doi: 10.18632/oncotarget.21600
69. Bräuer A, Grubert LS, Roll W, Schrader AJ, Schäfers M, Bögemann M, et al. 177Lu-PSMA-617 radioligand therapy and outcome in patients with metastasized castration-resistant prostate cancer. Eur J Nucl Med Mol Imaging. (2017) 44:1663–70. doi: 10.1007/s00259-017-3751-z
70. Yadav MP, Ballal S, Sahoo RK, Dwivedi SN, Bal C. Radioligand therapy with 177Lu-PSMA for metastatic castration-resistant prostate cancer: a systematic review and meta-analysis. AJR Am J Roentgenol. (2019) 213:275–85. doi: 10.2214/AJR.18.20845
71. Kratochwil C, Bruchertseifer F, Giesel FL, Weis M, Verburg FA, Mottaghy F, et al. 225Ac-PSMA-617 for PSMA-targeted α-radiation therapy of metastatic castration-resistant prostate cancer. J Nucl Med. (2016) 57:1941–4. doi: 10.2967/jnumed.116.178673
72. Sathekge M, Bruchertseifer F, Knoesen O, Reyneke F, Lawal I, Lengana T, et al. 225Ac-PSMA-617 in chemotherapy-naive patients with advanced prostate cancer: a pilot study. Eur J Nucl Med Mol Imaging. (2019) 46:129–38. doi: 10.1007/s00259-018-4167-0
73. dos Santos JC, Schäfer M, Bauder-Wüst U, Lehnert W, Leotta K, Morgenstern A, et al. Development and dosimetry of 203Pb/212Pb-labelled PSMA ligands: bringing “the lead” into PSMA-targeted alpha therapy? Eur J Nucl Med Mol Imaging. (2019) 46:1081–91. doi: 10.1007/s00259-018-4220-z
74. Lim A, Joseph KJ. Predicting radiation-adverse effects using three-dimensional dose and fractionation data: radiation dermatitis. Int J Radiat Oncol Biol Phys. (2019) 105:E130. doi: 10.1016/j.ijrobp.2019.06.2259
75. Loke KSH, Padhy AK, Ng DCE, Goh ASW, Divgi C. Dosimetric considerations in radioimmunotherapy and systemic radionuclide therapies: a review. World J Nucl Med. (2011) 10:122–38. doi: 10.4103/1450-1147.89780
76. Tagawa ST, Vallabhajosula S, Christos PJ, Jhanwar YS, Batra JS, Lam L, et al. Phase 1/2 study of fractionated dose lutetium-177-labeled anti-prostate-specific membrane antigen monoclonal antibody J591 (177 Lu-J591) for metastatic castration-resistant prostate cancer. Cancer. (2019) 125:2561–9. doi: 10.1002/cncr.32072
77. Niaz MJ, Batra JS, Walsh RD, Ramirez-Fort MK, Vallabhajosula S, Jhanwar YS, et al. Pilot study of hyperfractionated dosing of lutetium-177-labeled antiprostate-specific membrane antigen monoclonal antibody J591 (177 Lu-J591) for metastatic castration-resistant prostate cancer. Oncologist. (2020) 2020–0028. doi: 10.1634/theoncologist.2020-0028
78. Sunday ME, Kaplan LM, Motoyama E, Chin WW, Spindel ER. Gastrin-releasing peptide (mammalian bombesin) gene expression in health and disease. In: Pathology Reviews. Totawa, NJ: Humana Press (1989). p. 54–7. doi: 10.1007/978-1-4612-4502-5_4
79. Penman E, Wass JA, Butler MG, Penny ES, Price J, Wu P, et al. Distribution and characterisation of immunoreactive somatostatin in human gastrointestinal tract. Regul Pept. (1983) 7:53–65. doi: 10.1016/0167-0115(83)90281-1
80. Jensen RT, Battey JF, Spindel ER, Benya RV. International union of pharmacology. LXVIII. mammalian bombesin receptors: nomenclature, distribution, pharmacology, signaling, and functions in normal and disease states. Pharmacol Rev. (2008) 60:1–42. doi: 10.1124/pr.107.07108
81. Cuttitta F, Carney DN, Mulshine J, Moody TW, Fedorko J, Fischler A, et al. Bombesin-like peptides can function as autocrine growth factors in human small-cell lung cancer. Nature. (1985) 316:823–6. doi: 10.1038/316823a0
82. Ramos-Álvarez I, Moreno P, Mantey SA, Nakamura T, Nuche-Berenguer B, Moody TW, et al. Insights into bombesin receptors and ligands: highlighting recent advances. Peptides. (2015) 72:128–44. doi: 10.1016/j.peptides.2015.04.026
83. Touijer KA, Michaud L, Alvarez HAV, Gopalan A, Kossatz S, Gonen M, et al. Prospective study of the radiolabeled GRPR antagonist BAY86-7548 for positron emission tomography/computed tomography imaging of newly diagnosed prostate cancer. Eur Urol Oncol. (2019) 2:166–73. doi: 10.1016/j.euo.2018.08.011
84. Roivainen A, Kahkonen E, Borkowski S, Hofmann B, Lehtio K, Luoto P, et al. Radiometabolism, whole-body distribution and radiation dosimetry of Ga-68-bombesin antagonist BAY86-7548 in healthy men. In: European Journal Of Nuclear Medicine And Molecular Imaging. Springer, NY: Spring (2012). p. 10013. doi: 10.2967/jnumed.112.114082
85. Stephens A, Loid I WC, Beheshti M, Jambor I, Kemppainen J, Bostrom P, et al. Detection of prostate cancer with the [68Ga]-labeled bombesin antagonist RM2 in patients undergoing radical prostatectomy. J Clin Oncol. (2016) 34 (Suppl. 80):80. doi: 10.1200/jco.2016.34.2_suppl.80
86. Wieser G, Popp I, Rischke HC, Drendel V, Grosu AL, Bartholomä M, et al. Diagnosis of recurrent prostate cancer with PET/CT imaging using the gastrin-releasing peptide receptor antagonist 68 Ga-RM2: Preliminary results in patients with negative or inconclusive [18 F] Fluoroethylcholine-PET/CT. Eur J Nucl Med Mol Imaging. (2017) 44:1463–72. doi: 10.1007/s00259-017-3702-8
87. Iagaru A. Will GRPR compete with PSMA as a target in prostate cancer? J Nucl Med. (2017) 58:1883–4. doi: 10.2967/jnumed.117.198192
88. Coleman RE. Metastatic bone disease: clinical features, pathophysiology and treatment strategies. Cancer Treat Rev. (2001) 27:165–76. doi: 10.1053/ctrv.2000.0210
89. Ren G, Esposito M, Kang Y. Bone metastasis and the metastatic niche. J Mol Med. (2015) 93:1203–12. doi: 10.1007/s00109-015-1329-4
90. Parker C, Nilsson S, Heinrich D, Helle SI, O'sullivan JM, Fosså SD, et al. Alpha emitter radium-223 and survival in metastatic prostate cancer. N Engl J Med. (2013) 369:213–23. doi: 10.1056/NEJMoa1213755
91. Flux GD. Imaging and dosimetry for radium-223: the potential for personalized treatment. Br J Radiol. (2017) 90:20160748. doi: 10.1259/bjr.20160748
92. Abou DS, Rittenbach A, Tomlinson RE, Finley PA, Tsui B, Simons BW, et al. Preclinical single photon emission computed tomography of alpha particle-emitting radium-223. Cancer Biother Radiopharm. (2020). doi: 10.1089/cbr.2019.3308. [Epub ahead of print].
93. Benabdallah N, Bernardini M, Bianciardi M, de Labriolle-Vaylet C, Franck D, Desbrée A. 223Ra-dichloride therapy of bone metastasis: optimization of SPECT images for quantification. EJNMMI Res. (2019) 9:20. doi: 10.1186/s13550-019-0488-7
94. Umeda T, Koizumi M, Fukai S, Miyaji N, Motegi K, Nakazawa S, et al. Evaluation of bone metastatic burden by bone SPECT/CT in metastatic prostate cancer patients: defining threshold value for total bone uptake and assessment in radium-223 treated patients. Ann Nucl Med. (2018) 32:105–13. doi: 10.1007/s12149-017-1224-x
Keywords: peptide, radionuclide, radiotherapy, alpha particle, prostate cancer
Citation: Abou D, Benabdallah N, Jiang W, Peng L, Zhang H, Villmer A, Longtine MS and Thorek DLJ (2020) Prostate Cancer Theranostics - An Overview. Front. Oncol. 10:884. doi: 10.3389/fonc.2020.00884
Received: 16 October 2019; Accepted: 05 May 2020;
Published: 05 June 2020.
Edited by:
Georgios S. Limouris, National and Kapodistrian University of Athens, GreeceReviewed by:
Di Dong, Institute of Automation (CAS), ChinaCaroline Rousseau, Institut de Cancérologie de l'Ouest (ICO), France
Copyright © 2020 Abou, Benabdallah, Jiang, Peng, Zhang, Villmer, Longtine and Thorek. This is an open-access article distributed under the terms of the Creative Commons Attribution License (CC BY). The use, distribution or reproduction in other forums is permitted, provided the original author(s) and the copyright owner(s) are credited and that the original publication in this journal is cited, in accordance with accepted academic practice. No use, distribution or reproduction is permitted which does not comply with these terms.
*Correspondence: Diane Abou, ZGFib3UxQHd1c3RsLmVkdQ==; Daniel L. J. Thorek, dGhvcmVrLmxhYkB3dXN0bC5lZHU=