- 1Laboratory of Bone Tissue Engineering, Beijing Laboratory of Biomedical Materials, Beijing Research Institute of Traumatology and Orthopaedics, Beijing Jishuitan Hospital, Beijing, China
- 2Guangdong Provincial Key Laboratory of Orthopaedics and Traumatology, Guangzhou, China
- 3Department of Spinal Surgery, The First Affiliated Hospital of Sun Yat-sen University, Guangzhou, China
- 4Department of Orthopaedic Oncology Surgery, Beijing Jishuitan Hospital, Peking University, Beijing, China
Osteosarcoma is the most common malignant bone tumor in children and adolescents. Multiagent chemotherapy, together with surgical removal of all detectable lesions, has improved the long-term survival rate to 65–70% in patients with localized osteosarcoma and to 25–30% in patients with metastatic osteosarcoma since the 1970s. However, the conventional strategy has not improved in recent decades. With accumulating knowledge of the natural circular RNA (circRNA) pathogenesis of osteosarcoma, the diagnostic and therapeutic potential of some circRNAs has been explored. Meanwhile, artificial circular RNAs have been designed as onco-microRNA inhibitors to exert antitumor functions. Therefore, natural and artificial circular RNAs, like other RNA counterparts, are attractive new classes of therapeutic molecules for the treatment of osteosarcoma. This review summarizes the latest progress in the relationship between circRNAs and the malignant phenotype of osteosarcoma and sheds light on the therapeutic potential of the two types of circular RNA in the clinic.
Introduction
Osteosarcoma (OS) is a rare disease (incidence: 0.3 per 100,000 people per year) but has a high prevalence in children and adolescents. Most tumors arise in an extremity, while the proportion of axial tumor sites increases with age (1). It is a neoplasm that derives from primitive bone-forming mesenchymal cells that have histological evidence of osteoid production (2). The majority of primary OSs are high-grade bone tumors that account for 80–90% of all OSs (3). Due to the diverse molecular pathogeneses, lack of useful biomarkers, high local aggressiveness, and rapid metastasizing potential, traditional treatments have encountered serious setbacks (4). Although intensive adjuvant chemotherapy combined with surgery has achieved a 5-year survival rate of 60–70% in extremity-localized OS, the overall 5-year survival rate for patients with metastatic or relapsed OS has been around 20% for the past 30 years, and relapse rates have remained high at ~35% (5). Multidrug resistance and metastasis have long been two major obstacles in the treatment of OS and are also important causes of poor prognosis.
Initially thought to be the outcome of transcriptional noise, many non-coding RNAs (ncRNAs) have been found to participate in the pathogenesis of OS. Unlike microRNAs (miRNAs) and long non-coding RNAs (lncRNAs) that are already established research subjects, natural circular RNAs (circRNAs) are gaining increasing attention in the cancer research field. In general, the universal downregulation of circRNAs has been discovered in various tumors, which means that circRNAs may be diagnostic or therapeutic targets across cancer types (6, 7). Several reports have found that circRNAs are involved in the progression, metastasis, and multidrug resistance of OS (8, 9). As for the regulatory mechanism, it is widely accepted that circRNAs can act as competing endogenous RNAs (ceRNAs) to inhibit the function of certain endogenous miRNAs. Indeed, evidence has revealed that a ceRNA interacting network plays a vital role in regulating the pathogenic process of OS (10, 11). Additionally, newly developed artificial circular RNA molecules have been designed as exogenous miRNA inhibitors that effectively bind and block mature miRNAs, showing promise for the molecular therapy of cancer (12). While natural circRNAs found in the pathogenesis of OS may provide novel therapeutic targets, artificial circular RNAs designed for specific onco-microRNAs may also provide novel RNA-based therapy. Here, we review the role of circRNAs in the pathogenesis of OS with emphasis on the therapeutic potential of both natural and artificial circRNAs.
General Features of circRNAs
There are different types of circRNAs that are generated by distinct mechanisms, including circular RNA of the genomes of viroids, intermediates in rRNA processing or permuted tRNAs, and products of back-splicing of pre-mRNAs in eukaryotes (13). The most currently well-studied circRNAs generate their cyclic conformation from linear pre-mRNAs that back-splice or form exon lariats in which the 5′ splice site (splice donor) is joined to an upstream 3′ splice site (splice acceptor), resulting in a circular RNA with ends that are covalently ligated by a 3′-5′ phosphodiester bond (14).
CircRNAs have a unique circular structure that is resistant to degradation by most RNA decay machinery. Hansen et al. reported that the degradation of the circular CDR1 antisense transcript relies on the miR-671-mediated, Ago2-dependent cleavage pathway (15, 16). However, whether other circRNAs undergo a similar cleavage mechanism remains unknown. As opposed to their associated linear transcripts, circRNAs are characterized by a continuous covalently closed loop without 5′-3′ polarity or a 3′-poly A tail, giving them stronger resistance to RNase R and longer half-lives (17). It has been revealed that the median half-lives of circRNAs of mammary cells (18.8–23.7 h) are at least 2.5 times longer than their linear counterparts transcribed from the same host gene (18). CircRNAs exist stably in serum exosomes and human cell-free saliva, indicating their potential to be reliable biomarkers (19, 20). Such inherent stability makes this class of RNA a strong candidate to maintain homeostasis in the face of environmental challenge.
The potential mechanisms of circRNAs as miRNA inhibitors have been extensively explored. Pandolfi et al. proposed a ceRNA hypothesis in which different RNA transcripts interact with each other by competing for miRNAs through miRNA recognition elements (MREs) (21). Endogenous circRNAs could regulate gene expression by sequestering miRNAs that mediate negative regulation of their target genes (16, 22, 23). Rajewsky's and Kjems's labs have provided evidence supporting this idea, focusing on a natural antisense transcript (CDR1as) to cerebellar degeneration related protein 1 (CDR1) (16, 24). In contrast to other circular natural antisense transcriptional products, CDR1as contains about 70 MREs for miR-7 and 1 for miR-671. CDR1as functions as an miRNA antagonist, similar to knocking-down miR-7, impairing midbrain development in a zebrafish model (24). Knockdown of CDR1as in OS, hepatocellular carcinoma, and colorectal cancer cell lines leads to de-repressed miR-7 levels, downregulating miR-7-target genes and impairing cell vitality (25–27). Piwecka et al. reported the first CDR1as knockout mouse model and surprisingly observed that miR-7 was markedly downregulated, while its target genes were specifically upregulated in the brain (28). Bezzi et al. found that the prolonged absence of “sponge” circRNAs leads to destabilization of their binding miRNAs and de-repression of their targets (22). These studies imply that circRNAs may also serve as competing endogenous RNAs to store and transport miRNAs like a storage pool, indicating that they may serve a regulatory function in OS.
CircRNAs Involved in Malignant Phenotypes of OS
Although the fundamental molecular mechanisms underlying tumorigenesis, drug resistance, and metastasis of OS remain obscure, some studies have shown a clear relationship between certain circRNAs and malignant phenotypes (Table 1), which may provide new therapeutic targets for OS patients.
CircRNA-Mediated Chemoresistance of OS
A major obstacle for OS therapy is resistance to chemotherapy, which occurs in 35–45% of patients (39). The prognosis of patients with metastatic disease remains poor, with the 5-year survival rate less than 20% despite aggressive therapy. Therefore, understanding the mechanisms of chemoresistance is conducive to developing novel anti-OS strategies. CircRNA dysregulation related to chemotherapeutic resistance in OS has been reported with the help of high-throughput RNA sequencing, and the underlying mechanisms have been illustrated.
Zhang et al. reported that hsa_circ_001569 is significantly upregulated in OS tissues and facilitates enhanced resistance to cisplatin by activating the Wnt/β-catenin pathway (32). Zhu et al. found that knocking-down circPVT1 partly reversed the chemoresistance to doxorubicin and cisplatin by reducing the expression of ABCB1, a typical multidrug resistance-related gene (31). Zhu et al. further revealed the possible mechanisms of chemoresistance in OS in novel ways. They compared three paired multidrug-resistant and -sensitive OS cell lines by next-generation sequencing and found that hsa_circ_0004674 is distinctly upregulated in drug-resistant OS cell lines and in chemoresistant OS tissue. Furthermore, they speculated that hsa_circ_0004674 mediates chemoresistance by regulating the circRNA/miR-490-3p/ABCC2 or circRNA/miR-1254/EGFR axis by searching bioinformatics databases (Target Scan and miRanda) (33). To further elucidate the circRNA drug-resistance mechanism, Zhu et al. developed whole-transcriptome sequencing in multiple drug-resistant OS cell lines to identify differentially expressed ncRNAs and mRNAs, validating two ceRNA regulatory pathways, namely lncRNAMEG3/hsa-miR-200b-3p/AKT2 and hsa_circ_0001258/hsa-miR-744-3p/GSTM2 regulatory axes. In conclusion, circRNAs participate in the development of chemoresistance in OS by modulating signaling pathways or by their involvement in ceRNA regulatory networks, and therefore could potentially serve as novel therapeutic targets.
CircRNA-Mediated Metastasis of OS
OS is prone to metastases, which mostly arise in lungs (85–90%) but also develop in bones (8–10%) and rarely in lymph nodes (3). Approximately 20–25% of patients are diagnosed with lung metastasis before the initial treatment, and about 80% of patients progress with metastases following surgical resection (40, 41). Current treatment for patients with pulmonary metastasis is radical metastasectomy combined with chemotherapy. However, it fails to prolong the long-term survival rate. Once the disease has advanced, prognosis is poor with a survival rate of less than 20%. Recent work has shown that circRNAs crosstalk with regulatory miRNAs in OS metastasis. Wu et al. systemically elucidated the role of circTADA2A in the progression and metastasis of OS. They found that circTADA2A acted as an oncogene by sponging miR-203a-3p and upregulating the expression of CREB3. CREB3, a driver gene, enhances the expression of mmp-9 and Bcl-2, resulting in progression and metastasis in OS (34). In a similar pro-metastatic mechanism, Huang et al. reported that the expression level of circNASP is positively correlated with metastasis in OS patients and modulates malignant behaviors via the miR-1253/FOXF1 axis (36). As illustrated in Table 1, the aberrant expression of circRNAs is closely related to metastatic tendencies.
Clinical Implications of circRNA
OS is an aggressive malignancy with poor clinical outcomes. Thus, it is urgent to identify reliable and non-invasive biomarkers to detect the disease at a very early stage. Endogenous circRNAs are ideal biomarkers for the diagnosis and prognosis of OS due to their stability in serum exosomes.
MiRNAs are endogenous noncoding RNAs closely related to the development of OS, and their aberrant expression results in carcinogenesis and cancer progression (42–44). CircRNAs have been proven to be effective inhibitors of miRNAs and can rescue their abnormal expression, ultimately stopping the cancer process.
Endogenous circRNAs as Potential Biomarkers
Histological evaluation is the gold standard for diagnosing OS, but the method is invasive and sometimes too late (45). CircRNAs are highly dysregulated in OS and can be detected in tissues and even in plasma samples, which make them ideal candidates for biomarkers. For instance, Zhu et al. confirmed that the upregulation of serum circPVT1 can distinguish OS patients from healthy individuals, finding that circPVT1 is more reliable than alkaline phosphatase for diagnosis (31). In another study, Zhang et al. revealed that circUBAP2 expression is significantly positively correlated with the tumor stages of OS, and Kaplan-Meier survival analysis showed that increased circUBAP2 correlates with reduced survival and poor prognosis (46). Huang et al. conducted a meta-analysis of abnormal circRNAs in OS patients (47). In terms of prognosis, both oncogenic and tumor-suppressor circRNAs had effects on overall survival. In the diagnosis of OS, they calculated an area under the curve of 0.85, with 80% sensitivity, and 77% specificity, using circRNAs.
Endogenous circRNAs as Targets for OS Therapy
CircRNAs, as ceRNAs, are natural miRNA inhibitors that bind to a limited miRNA pool to relieve the repression of target RNAs. Endogenous circRNAs function as either tumor activators or tumor suppressors in OS (25, 38). These opposing effects give circRNAs great potential for therapeutic strategies. Most reported circRNAs are carcinogenic, playing essential roles in chemoresistance and metastasis of OS (Table 1). These can be targeted and knocked down to impair the development of OS and have long been used for rescue experiments. RNA interference (RNAi) is the most common approach via targeting back-splicing junction sites in order to correct the expression of carcinogenic circRNAs (48). Some circRNAs are downregulated in OS, and overexpressing them by transfection significantly suppresses OS progression. Hsa_circ_0002052 and circHIPK3 are significantly deregulated in OS tissues and cell lines and act as tumor suppressor genes that may serve as therapeutic molecules for OS (37, 38). Taken together, circRNAs can either serve as oncogenic stimuli or tumor suppressors in OS and are therefore potential therapeutic targets for OS intervention.
Artificial circRNAs as Novel miRNA Inhibitors
With the increasing demand for regulating miRNA activity for cancer therapies, a newly designed artificial miRNA sponge has been developed. Inspired by natural circRNAs reported as endogenous miRNA sponges, a practical artificial circRNA sponge has been synthesized using simple enzymatic ligation steps (12). The artificial circRNA has five bulged miR-21 binding sites that can sponge multiple miR-21 molecules, and better restoration of luciferase activity has been observed as compared with a conventional linear miR-21 inhibitor. Its linear counterparts degrade by 92% in a condition of 4% FBS after 30 min, while the synthetic circRNA only degrades by 9% (12). The sponge not only avoids degradation by exonucleases but also upregulates the expression of the tumor suppressor gene DAXX targeted by miR-21, thus significantly inhibiting the proliferation of gastric cancer cells (12). Liu et al. constructed circRNA sponges for miR-21 and miR-221 using a circular sponge-producing vector, and these were found to be more effective in repressing miRNA targets and anticancer activities compared to typical linear miRNA sponges in malignant melanoma cell lines (49). Shu et al. developed an intracellular vector to express circular miRNA inhibitors, anti-miR223 and anti-miR21, which inhibit the function of carcinogenic miRNAs more effectively than their linear counterparts (50). These custom circular sponges provide a new means of inhibiting the function of targeted miRNAs in vitro.
The Advantages of circRNAs
Their circular conformation gives circRNA molecules some advantages over other miRNA inhibitors. (1) CircRNAs are stable in the cytoplasm and resist degradation by RNA enzymes and miRNAs (17, 24, 48). Moreover, circRNAs function rapidly and efficiently by affecting hundreds of transcription products by regulating their matching miRNAs (51). (2) Compared with manmade anti-miRNA oligonucleotides (AMO), artificial circRNA sponges offer greater clinical value. AMOs are 17–22 nt single-stranded antisense oligonucleotides that act against natural miRNAs and are chemically modified and optimized for in vivo delivery (52). However, they fail to maintain a relatively high concentration for satisfactory suppression in the absence of continuous supply. In contrast, miRNA sponges can exert prolonged or permanent suppression against targeted individual miRNAs or against an entire miRNA family by recognizing shared MREs (53–55). In 2018, Liu et al. and Josta et al. provided a proof-of-principle that artificial circRNAs can sponge targeted miRNAs and reduce a series of post-transcriptional products more efficiently compared with conventional AMOs (12, 56, 57).
Perspectives and Conclusion
Since the first RNAi-based oligonucleotide was approved by the United States Food and Drug Administration in 2018, many pharmaceutical companies have raced to develop RNAi-based drugs (58). RNAi-based drugs directly bind to their targets with perfect complementarity and mediate the cleavage of specific transcripts, while miRNA-based therapies aim to reestablish normal expression and function of key miRNAs (59). Even though no miRNA-based therapeutics have been approved for clinical use, clinical trials support the validity of miRNA inhibition in clinical applications and might inspire future attempts to develop antagomiRs or miRNA mimics for use in cancer therapy (60, 61).
In terms of miRNA-based agents in OS, many miRNAs with great therapeutic properties have been developed in preclinical research. However, none of them have yet reached the clinical evaluation stage. For instance, miR-21 is a typical oncogenic miRNA that is overexpressed in many types of tumors and is involved in all tumor stages ranging from initiation to metastasis (62, 63), including in OS. Chen et al. reported that miR-21 promotes proliferation and invasion by activating the PTEN/Akt pathway (64). Wu et al. revealed that miR-21 negatively regulates the tumor suppressor gene RECK, leading to cell invasion and migration (65). Yang et al. showed that miR-21 regulates sensitivity to cisplatin (66), and Xu et al. discovered that miR-21 inhibits the apoptosis of OS by downregulating caspase 8 (67). Ren et al. reported that high miR-21 expression in tumor tissues is associated with poor prognosis and shorter survival (68). These findings raise concerns about the use of anti-miR-21 as a therapeutic agent in OS.
Artificial circRNAs engineered to contain an array of MREs can act as an miR-21 sponge. This kind of circRNA sponge sequesters miR-21 from targeted genes in a manner similar to antagomiRs, suppressing gastric carcinoma cell proliferation in vitro and inhibiting esophageal carcinoma tumorigenesis in vivo (12, 69). Preliminary data suggest that artificial circRNAs are a new class of miRNA inhibitors that can provide novel future miRNA-based therapy in OS (Figure 1).
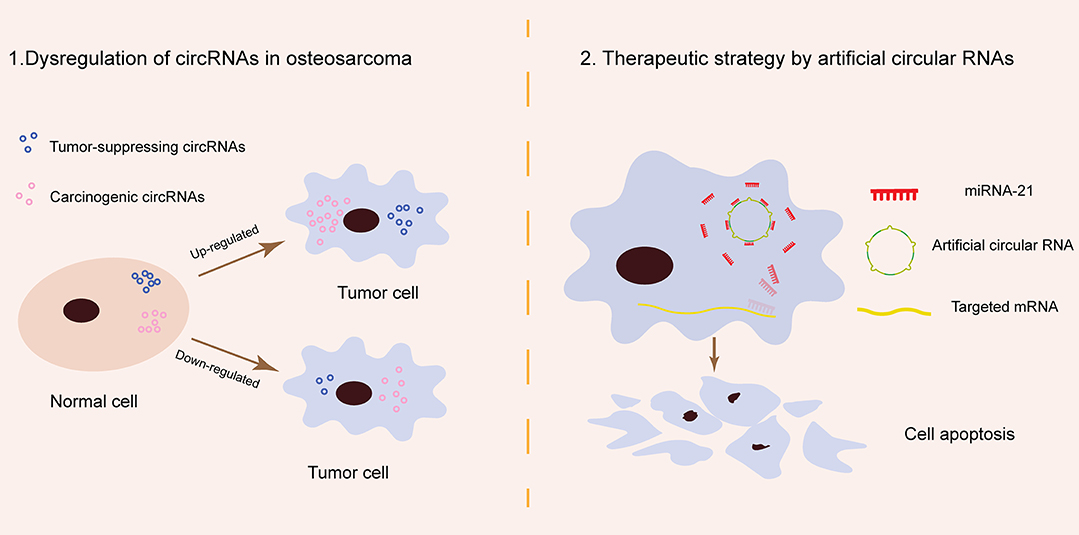
Figure 1. Schematic of circRNA pathogenesis in osteosarcoma and their potential role in osteosarcoma therapy. (1) Tumor-suppressing and carcinogenic circRNAs are naturally present in normal cells and remain in relatively stable amounts. In osteosarcoma, tumor-suppressing circRNAs are downregulated while carcinogenic circRNAs are upregulated. The dysregulation of specific natural circRNAs contributes to osteosarcoma carcinogenesis. (2) miR-21 is a typical oncogenic miRNA in osteosarcoma. Artificial circular RNAs produced by chemical synthesis may block osteosarcoma progress by sponging miRNA-21 in the cytoplasm.
Natural circRNAs may be secreted and circulate in bodily fluids, and their circular structure gives them strong resistance to ribonucleases. Studies have partially proven that dysregulated circRNA expression can serve as a novel biomarker for OS. However, there are still some limitations before clinical application. First, potential circRNA candidates should be confirmed using larger sample sizes. Second, high specificity and high sensitivity must be emphasized. Third, the mechanism by which circRNAs are dysregulated in OS needs to be explored.
In conclusion, the dysregulation of some circRNAs plays a pathogenic role in OS. CircRNAs can function as tumor activators or suppressors in OS and have been further implicated in regulating OS chemoresistance and metastasis. Current treatments fail to further benefit patients with progressed OS due to chemoresistance, early metastasis, and a high recurrence rate. The use of RNAs as therapeutics has advanced tremendously and may provide a novel way to treat OS. For one thing, natural circRNAs can serve as tumor-suppressive molecules, such as hsa_circ_0002052, and could be natural anticancer agents when overexpressed in OS. For another, exogenous circRNAs can function as miRNA sponges to stop the cancer process, whether chemically synthesized or expressed by gene vectors. Hence, we are convinced that well-engineered circRNAs with multiple miR-21 binding sites may inhibit OS progression, since miRNA-21 is an essential onco-miRNA in OS. Although circRNA-based therapies for OS are still in the early stages, further development of circRNAs as biomarkers and miRNA inhibitors has a bright future.
Author Contributions
DC, RW, and WL conceptualized the ideas. BW and HH contributed equally to this review. BW summarized related publications and drafted the manuscript. BW and HH created the figure and table. DC, RW, HH, and WL reviewed the manuscript and provided feedback. All authors have read and approved the final manuscript.
Funding
This work was supported by the National Natural Science Foundation of China (Grant no. 51973021), Beijing Municipal Health Commission (Grant no. BMHC-2019-9 and BMHC-2018-4).
Conflict of Interest
The authors declare that the research was conducted in the absence of any commercial or financial relationships that could be construed as a potential conflict of interest.
References
1. Casali PG, Bielack S, Abecassis N, Aro HT, Bauer S, Biagini R, et al. Bone sarcomas: ESMO–PaedCan–EURACAN Clinical Practice Guidelines for diagnosis, treatment and follow-up. Ann Oncol. (2018) 29:iv79–95. doi: 10.1093/annonc/mdy310
2. Ottaviani G, Jaffe N. The epidemiology of osteosarcoma. In: Jaffe N, Bruland OS, Bielack S, editors. Pediatric and Adolescent Osteosarcoma. Springer (2009). p. 3–13.
3. Luetke A, Meyers PA, Lewis I, Juergens H. Osteosarcoma treatment - where do we stand? A state of the art review. Cancer Treat Rev. (2014) 40:523–32. doi: 10.1016/j.ctrv.2013.11.006
4. Lindsey BA, Markel JE, Kleinerman ES. Osteosarcoma overview. Rheumatol Ther. (2017) 4:25–43. doi: 10.1007/s40744-016-0050-2
5. Dean DC, Shen S, Hornicek FJ, Duan Z. From genomics to metabolomics: emerging metastatic biomarkers in osteosarcoma. Cancer Metastasis Rev. (2018) 37:719–31. doi: 10.1007/s10555-018-9763-8
6. Patop IL, Kadener S. circRNAs in Cancer. Curr Opin Genet Dev. (2018) 48:121–7. doi: 10.1016/j.gde.2017.11.007
7. Vo JN, Cieslik M, Zhang Y, Shukla S, Xiao L, Zhang Y, et al. The landscape of circular RNA in cancer. Cell. (2019) 176:869–81.e13. doi: 10.1016/j.cell.2018.12.021
8. Wang C, Jing J, Cheng L. Emerging roles of non-coding RNAs in the pathogenesis, diagnosis and prognosis of osteosarcoma. Invest New Drugs. (2018) 36:1116–32. doi: 10.1007/s10637-018-0624-7
9. Wang C, Ren M, Zhao X, Wang A, Wang J. Emerging roles of circular RNAs in osteosarcoma. Med Sci Monit. (2018) 24:7043–50. doi: 10.12659/MSM.912092
10. Zhu K-P, Zhang C-L, Ma X-L, Hu J-P, Cai T, Zhang L. Analyzing the interactions of mRNAs and ncRNAs to predict competing endogenous RNA networks in osteosarcoma chemo-resistance. Mol Ther. (2019) 27:518–30. doi: 10.1016/j.ymthe.2019.01.001
11. Zhong Y, Du Y, Yang X, Mo Y, Fan C, Xiong F, et al. Circular RNAs function as ceRNAs to regulate and control human cancer progression. Mol Cancer. (2018) 17:79. doi: 10.1186/s12943-018-0827-8
12. Liu X, Abraham JM, Cheng Y, Wang Z, Wang Z, Zhang G, et al. Synthetic circular RNA functions as a miR-21 sponge to suppress gastric carcinoma cell proliferation. Mol Ther Nucleic Acids. (2018) 13:312–21. doi: 10.1016/j.omtn.2018.09.010
13. Lasda E, Parker R. Circular RNAs: diversity of form and function. RNA. (2014) 20:1829–42. doi: 10.1261/rna.047126.114
14. Wilusz JE. A 360 view of circular RNAs: from biogenesis to functions. Wiley Interdiscip Rev RNA. (2018) 9:e1478. doi: 10.1002/wrna.1478
15. Hansen TB, Wiklund ED, Bramsen JB, Villadsen SB, Statham AL, Clark SJ, et al. miRNA-dependent gene silencing involving Ago2-mediated cleavage of a circular antisense RNA. EMBO J. (2011) 30:4414–22. doi: 10.1038/emboj.2011.359
16. Hansen TB, Jensen TI, Clausen BH, Bramsen JB, Finsen B, Damgaard CK, et al. Natural RNA circles function as efficient microRNA sponges. Nature. (2013) 495:384–8. doi: 10.1038/nature11993
17. Jeck WR, Sorrentino JA, Wang K, Slevin MK, Burd CE, Liu J, et al. Circular RNAs are abundant, conserved, and associated with ALU repeats. RNA. (2013) 19:141–57. doi: 10.1261/rna.035667.112
18. Enuka Y, Lauriola M, Feldman ME, Sas-Chen A, Ulitsky I, Yarden Y. Circular RNAs are long-lived and display only minimal early alterations in response to a growth factor. Nucleic Acids Res. (2016) 44:1370–83. doi: 10.1093/nar/gkv1367
19. Li Y, Zheng Q, Bao C, Li S, Guo W, Zhao J, et al. Circular RNA is enriched and stable in exosomes: a promising biomarker for cancer diagnosis. Cell Res. (2015) 25:981–4. doi: 10.1038/cr.2015.82
20. Bahn JH, Zhang Q, Li F, Chan TM, Lin X, Kim Y, et al. The landscape of microRNA, Piwi-interacting RNA, and circular RNA in human saliva. Clin Chem. (2015) 61:221–30. doi: 10.1373/clinchem.2014.230433
21. Salmena L, Poliseno L, Tay Y, Kats L, Pandolfi PP. A ceRNA hypothesis: the Rosetta Stone of a hidden RNA language? Cell. (2011) 146:353–8. doi: 10.1016/j.cell.2011.07.014
22. Bezzi M, Guarnerio J, Pandolfi PP. A circular twist on microRNA regulation. Cell Res. (2017) 27:1401–2. doi: 10.1038/cr.2017.136
23. Catalanotto C, Cogoni C, Zardo G. MicroRNA in control of gene expression: an overview of nuclear functions. Int J Mol Sci. (2016) 17:1712. doi: 10.3390/ijms17101712
24. Memczak S, Jens M, Elefsinioti A, Torti F, Krueger J, Rybak A, et al. Circular RNAs are a large class of animal RNAs with regulatory potency. Nature. (2013) 495:333–8. doi: 10.1038/nature11928
25. Xu B, Yang T, Wang Z, Zhang Y, Liu S, Shen M. CircRNA CDR1as/miR-7 signals promote tumor growth of osteosarcoma with a potential therapeutic and diagnostic value. Cancer Manag Res. (2018) 10:4871–80. doi: 10.2147/CMAR.S178213
26. Yu L, Gong X, Sun L, Zhou Q, Lu B, Zhu L. The circular RNA Cdr1as act as an oncogene in hepatocellular carcinoma through targeting miR-7 expression. PLoS ONE. (2016) 11:e0158347. doi: 10.1371/journal.pone.0158347
27. Tang W, Ji M, He G, Yang L, Niu Z, Jian M, et al. Silencing CDR1as inhibits colorectal cancer progression through regulating microRNA-7. Onco Targets Ther. (2017) 10:2045–56. doi: 10.2147/OTT.S131597
28. Piwecka M, GlaŽar P, Hernandez-Miranda LR, Memczak S, Wolf SA, Rybak-Wolf A, et al. Loss of a mammalian circular RNA locus causes miRNA deregulation and affects brain function. Science. (2017) 357:eaam8526. doi: 10.1126/science.aam8526
29. Song YZ, Li JF. Circular RNA hsa_circ_0001564 regulates osteosarcoma proliferation and apoptosis by acting miRNA sponge. Biochem Biophys Res Commun. (2018) 495:2369–75. doi: 10.1016/j.bbrc.2017.12.050
30. Deng N, Li L, Gao J, Zhou J, Wang Y, Wang C, et al. Hsa_circ_0009910 promotes carcinogenesis by promoting the expression of miR-449a target IL6R in osteosarcoma. Biochem Biophys Res Commun. (2018) 495:189–96. doi: 10.1016/j.bbrc.2017.11.028
31. Kun-Peng Z, Xiao-Long M, Chun-Lin Z. Overexpressed circPVT1, a potential new circular RNA biomarker, contributes to doxorubicin and cisplatin resistance of osteosarcoma cells by regulating ABCB1. Int J Biol Sci. (2018) 14:321–30. doi: 10.7150/ijbs.24360
32. Zhang H, Yan J, Lang X, Zhuang Y. Expression of circ_001569 is upregulated in osteosarcoma and promotes cell proliferation and cisplatin resistance by activating the Wnt/β-catenin signaling pathway. Oncol Lett. (2018) 16:5856–62. doi: 10.3892/ol.2018.9410
33. Kun-Peng Z, Xiao-Long M, Lei Z, Chun-Lin Z, Jian-Ping H, Tai-Cheng Z. Screening circular RNA related to chemotherapeutic resistance in osteosarcoma by RNA sequencing. Epigenomics. (2018) 10:1327–46. doi: 10.2217/epi-2018-0023
34. Wu Y, Xie Z, Chen J, Chen J, Ni W, Ma Y, et al. Circular RNA circTADA2A promotes osteosarcoma progression and metastasis by sponging miR-203a-3p and regulating CREB3 expression. Mol Cancer. (2019) 18:73. doi: 10.1186/s12943-019-1007-1
35. Jin H, Jin X, Zhang H, Wang W. Circular RNA hsa-circ-0016347 promotes proliferation, invasion and metastasis of osteosarcoma cells. Oncotarget. (2017) 8:25571–81. doi: 10.18632/oncotarget.16104
36. Huang L, Chen M, Pan J, Yu W. Circular RNA circNASP modulates the malignant behaviors in osteosarcoma via miR-1253/FOXF1 pathway. Biochem Biophys Res Commun. (2018) 500:511–7. doi: 10.1016/j.bbrc.2018.04.131
37. Xiao-Long M, Kun-Peng Z, Chun-Lin Z. Circular RNA circ_HIPK3 is down-regulated and suppresses cell proliferation, migration and invasion in osteosarcoma. J Cancer. (2018) 9:1856–62. doi: 10.7150/jca.24619
38. Wu Z, Shi W, Jiang C. Overexpressing circular RNA hsa_circ_0002052 impairs osteosarcoma progression via inhibiting Wnt/β-catenin pathway by regulating miR-1205/APC2 axis. Biochem Biophys Res Commun. (2018) 502:465–71. doi: 10.1016/j.bbrc.2018.05.184
39. Chou AJ, Gorlick R. Chemotherapy resistance in osteosarcoma: current challenges and future directions. Expert Rev Anticancer Ther. (2006) 6:1075–85. doi: 10.1586/14737140.6.7.1075
40. Marina N, Gebhardt M, Teot L, Gorlick R. Biology and therapeutic advances for pediatric osteosarcoma. Oncologist. (2004) 9:422–41. doi: 10.1634/theoncologist.9-4-422
41. Hattinger CM, Pasello M, Ferrari S, Picci P, Serra M. Emerging drugs for high-grade osteosarcoma. Expert Opin Emerg Drugs. (2010) 15:615–34. doi: 10.1517/14728214.2010.505603
42. Esquela-Kerscher A, Slack FJ. Oncomirs—microRNAs with a role in cancer. Nat Rev Cancer. (2006) 6:259–69. doi: 10.1038/nrc1840
43. Di Leva G, Garofalo M, Croce CM. MicroRNAs in cancer. Annu Rev Pathol. (2014) 9:287–314. doi: 10.1146/annurev-pathol-012513-104715
44. Jones KB, Salah Z, Del Mare S, Galasso M, Gaudio E, Nuovo GJ, et al. miRNA signatures associate with pathogenesis and progression of osteosarcoma. Cancer Res. (2012) 72:1865–77. doi: 10.1158/0008-5472.CAN-11-2663
45. Kong C, Hansen MF. Biomarkers in osteosarcoma. Expert Opin Med Diagn. (2009) 3:13–23. doi: 10.1517/17530050802608496
46. Zhang H, Wang G, Ding C, Liu P, Wang R, Ding W, et al. Increased circular RNA UBAP2 acts as a sponge of miR-143 to promote osteosarcoma progression. Oncotarget. (2017) 8:61687–97. doi: 10.18632/oncotarget.18671
47. Huang X, Yang W, Zhang Z, Shao Z. Dysregulated circRNAs serve as prognostic and diagnostic markers in osteosarcoma by sponging microRNA to regulate the downstream signaling pathway. J Cell Biochem. (2020) 121:1834–41. doi: 10.1002/jcb.29418
48. Li X, Yang L, Chen LL. The biogenesis, functions, and challenges of circular RNAs. Mol Cell. (2018) 71:428–42. doi: 10.1016/j.molcel.2018.06.034
49. Liu Y, Cui H, Wang W, Li L, Wang Z, Yang S, et al. Construction of circular miRNA sponges targeting miR-21 or miR-221 and demonstration of their excellent anticancer effects on malignant melanoma cells. Int J Biochem Cell Biol. (2013) 45:2643–50. doi: 10.1016/j.biocel.2013.09.003
50. Shu Y, Wu K, Zeng Z, Huang S, Ji X, Yuan C, et al. A simplified system to express circularized inhibitors of miRNA for stable and potent suppression of miRNA functions. Mol Ther Nucleic Acids. (2018) 13:556–67. doi: 10.1016/j.omtn.2018.09.025
51. Taulli R, Loretelli C, Pandolfi PP. From pseudo-ceRNAs to circ-ceRNAs: a tale of cross-talk and competition. Nat Struct Mol Biol. (2013) 20:541–3. doi: 10.1038/nsmb.2580
52. Biswas S. MicroRNAs as therapeutic agents: the future of the battle against cancer. Curr Top Med Chem. (2018) 18:2544–54. doi: 10.2174/1568026619666181120121830
53. Tang L, Chen HY, Hao NB, Tang B, Guo H, Yong X, et al. microRNA inhibitors: natural and artificial sequestration of microRNA. Cancer Lett. (2017) 407:139–47. doi: 10.1016/j.canlet.2017.05.025
54. Thomson DW, Dinger ME. Endogenous microRNA sponges: evidence and controversy. Nat Rev Genet. (2016) 17:272–83. doi: 10.1038/nrg.2016.20
55. Cohen SM. Use of microRNA sponges to explore tissue-specific microRNA functions in vivo. Nat Methods. (2009) 6:873–4. doi: 10.1038/nmeth1209-873
56. Jost I, Shalamova LA, Gerresheim GK, Niepmann M, Bindereif A, Rossbach O. Functional sequestration of microRNA-122 from Hepatitis C Virus by circular RNA sponges. RNA Biol. (2018) 15:1032–9. doi: 10.1080/15476286.2018.1435248
57. Rossbach O. Artificial circular RNA sponges targeting MicroRNAs as a novel tool in molecular biology. Mol Ther Nucleic Acids. (2019) 17:452–4. doi: 10.1016/j.omtn.2019.06.021
58. Hoy SM. Patisiran: first global approval. Drugs. (2018) 78:1625–31. doi: 10.1007/s40265-018-0983-6
59. Bajan S, Hutvagner G. RNA-based therapeutics: from antisense oligonucleotides to miRNAs. Cells. (2020) 9:137. doi: 10.3390/cells9010137
60. Querfeld C, Pacheco T, Foss FM, Halwani AS, Porcu P, Seto AG, et al. Preliminary results of a phase 1 trial evaluating MRG-106, a synthetic microRNA antagonist (LNA antimiR) of microRNA-155, in patients with TCL. Blood. (2016) 128:1829. doi: 10.1182/blood.V128.22.1829.1829
61. van Zandwijk N, Pavlakis N, Kao SC, Linton A, Boyer MJ, Clarke S, et al. Safety and activity of microRNA-loaded minicells in patients with recurrent malignant pleural mesothelioma: a first-in-man, phase 1, open-label, dose-escalation study. Lancet Oncol. (2017) 18:1386–96. doi: 10.1016/S1470-2045(17)30621-6
62. Si ML, Zhu S, Wu H, Lu Z, Wu F, Mo YY. miR-21-mediated tumor growth. Oncogene. (2007) 26:2799–803. doi: 10.1038/sj.onc.1210083
63. Selcuklu SD, Donoghue MT, Spillane C. miR-21 as a key regulator of oncogenic processes. Biochem Soc Trans. (2009) 37(Pt 4):918–25. doi: 10.1042/BST0370918
64. Lv C, Hao Y, Tu G. MicroRNA-21 promotes proliferation, invasion and suppresses apoptosis in human osteosarcoma line MG63 through PTEN/Akt pathway. Tumour Biol. (2016) 37:9333–42. doi: 10.1007/s13277-016-4807-6
65. Ziyan W, Shuhua Y, Xiufang W, Xiaoyun L. MicroRNA-21 is involved in osteosarcoma cell invasion and migration. Med Oncol. (2011) 28:1469–74. doi: 10.1007/s12032-010-9563-7
66. Ziyan W, Yang L. MicroRNA-21 regulates the sensitivity to cisplatin in a human osteosarcoma cell line. Ir J Med Sci. (2016) 185:85–91. doi: 10.1007/s11845-014-1225-x
67. Xu B, Xia H, Cao J, Wang Z, Yang Y, Lin Y. MicroRNA-21 inhibits the apoptosis of osteosarcoma cell line SAOS-2 via targeting caspase 8. Oncol Res. (2017) 25:1161–8. doi: 10.3727/096504017X14841698396829
68. Ren X, Shen Y, Zheng S, Liu J, Jiang X. miR-21 predicts poor prognosis in patients with osteosarcoma. Br J Biomed Sci. (2016) 73:158–62. doi: 10.1080/09674845.2016.1220710
Keywords: natural circular RNA, artificial circular RNA, osteosarcoma, RNA-based therapy, cancer therapeutic strategy
Citation: Wan B, Hu H, Wang R, Liu W and Chen D (2020) Therapeutic Potential of Circular RNAs in Osteosarcoma. Front. Oncol. 10:370. doi: 10.3389/fonc.2020.00370
Received: 08 October 2019; Accepted: 03 March 2020;
Published: 15 April 2020.
Edited by:
Ondrej Slaby, Brno University of Technology, CzechiaReviewed by:
Pierpaolo Correale, Azienda Ospedaliera ‘Bianchi-Melacrino-Morelli’, ItalyPirjo Spuul, Tallinn University of Technology, Estonia
Copyright © 2020 Wan, Hu, Wang, Liu and Chen. This is an open-access article distributed under the terms of the Creative Commons Attribution License (CC BY). The use, distribution or reproduction in other forums is permitted, provided the original author(s) and the copyright owner(s) are credited and that the original publication in this journal is cited, in accordance with accepted academic practice. No use, distribution or reproduction is permitted which does not comply with these terms.
*Correspondence: Dafu Chen, Y2hlbmRhZnUmI3gwMDA0MDtqc3Rob3NwaXRhbC5vcmc=
†These authors share first authorship