- 1Hematology and Clinical Immunology Section, Department of Medicine (DIMED), Padova University School of Medicine, Padova, Italy
- 2Veneto Institute of Molecular Medicine (VIMM), Padova, Italy
Large granular lymphocyte leukemia (LGLL) is a chronic proliferation of clonal cytotoxic lymphocytes, usually presenting with cytopenias and yet lacking a specific therapy. The disease is heterogeneous, including different subsets of patients distinguished by LGL immunophenotype (CD8+ Tαβ, CD4+ Tαβ, Tγδ, NK) and the clinical course of the disease (indolent/symptomatic/aggressive). Even if the etiology of LGLL remains elusive, evidence is accumulating on the genetic landscape driving and/or sustaining chronic LGL proliferations. The most common gain-of-function mutations identified in LGLL patients are on STAT3 and STAT5b genes, which have been recently recognized as clonal markers and were included in the 2017 WHO classification of the disease. A significant correlation between STAT3 mutations and symptomatic disease has been highlighted. At variance, STAT5b mutations could have a different clinical impact based on the immunophenotype of the mutated clone. In fact, they are regarded as the signature of an aggressive clinical course with a poor prognosis in CD8+ T-LGLL and aggressive NK cell leukemia, while they are devoid of negative prognostic significance in CD4+ T-LGLL and Tγδ LGLL. Knowing the specific distribution of STAT mutations helps identify the discrete mechanisms sustaining LGL proliferations in the corresponding disease subsets. Some patients equipped with wild type STAT genes are characterized by less frequent mutations in different genes, suggesting that other pathogenetic mechanisms are likely to be involved. In this review, we discuss how the LGLL mutational pattern allows a more precise and detailed tumor stratification, suggesting new parameters for better management of the disease and hopefully paving the way for a targeted clinical approach.
Introduction
The 2017 world health organization (WHO) classification includes the large granular lymphocyte (LGL) leukemia in the category of cytotoxic T and NK cell leukemia and lymphoma. LGL leukemia is a lymphoproliferative disorder, sustained by clonal mature T or NK cells, that configures T-LGL leukemia (T-LGLL) or the chronic lymphoproliferative disease of NK cells (CLPD-NK), respectively (1). T-LGLL is the most frequent form (about 85% of cases), whereas, CLPD-NK is less represented (10% of cases) (2). A third group of rare (incidence 5%) diseases accounts for aggressive T-LGLL and aggressive NK cell leukemia (ANKL), characterized by very poor prognosis (2). T-LGLs usually express the TCR αβ+, CD4–, CD8+ phenotype and the disease is referred to CD8+ T-LGLL. The heterogeneity of the disease is emphasized by the presence, in 10–15% cases, of a disorder sustained by TCR αβ+, CD4+, CD8+/– LGLs, defining the CD4+ T-LGLL. Beyond the expansions of T cells bearing the TCR αβ+, a minority of cases originates from TCRγδ+ cells (Tγδ LGLL) (3). In addition, some patients are characterized by a bi-phenotypical variant, identified by a concomitant T/NK cell clone, or by a switch from T to NK phenotype or vice versa (4).
The disease is asymptomatic in nearly 30% of cases, with lymphocytosis representing the only observed hematological abnormality (5). However, during the disease course in 60% of cases therapy is needed, mostly for cytopenia-related manifestations, symptomatic patients showing clinical features often related to neutropenia (2). Currently, no specific treatment is available for LGL disorders and the current therapy is based on immunosuppressive drugs (i.e., Methotrexate, Cyclophosphamide or Cyclosporine A) giving unsatisfying responses (6, 7).
The etiopathogenesis of LGL leukemia has not been established. A viral or autologous antigen has been claimed to trigger the initial lymphocytosis whose survival over the time is then maintained by the upregulation of several cell activating pathways (8, 9). Among these, the Janus kinase/signal transducer and activator of transcription (JAK/STAT) signaling is central to direct the cell toward survival, being STAT an inducer of the transcription of many pro-survival genes (10). Supporting the role of these activatory pathways, in about 40% of patients, mutations on STAT3 and STAT5b have been recognized as the most common gain-of-function genetic lesions up to now identified in LGLL patients. The resulting constitutive activation of STAT3 and STAT5b promotes an upregulation of the expression of genes that are required for cell proliferation and survival, i.e., c-Myc, cyclin D1 and cyclin D2, Bcl-xl, Mcl1, and survivin (11). STAT3 and STAT5b mutations have been included in the 2017 WHO LGLL classification (12).
Genetics of T-LGLL
STAT3 Mutations
Currently, STAT3 mutations are the most commonly recognized genetic lesions in T-LGLL. Somatic STAT3 mutations are preferentially located in the Src homology 2 (SH2) domain of the gene, leading to an increase of the stability of STAT3 protein dimerization that results in an enhanced transcriptional activity of pro-survival proteins (13). STAT3 mutation is preferentially found in CD8+ T-LGLL (14) and some TCRγδ LGLL cases (15), its incidence among the entire cohort of T-LGLL ranging from 11 up to 75% based on different reports (13–26). Y640F and D661Y are the most frequent STAT3 genetic lesions, accounting for about 60% of the recognized mutations. The remnant other less frequent mutations include both point mutations and insertion or deletions and are mostly found in SH2 domain (13–26), although some missense substitutions were described in DNA-binding and coiled coil domains [(27); Figure 1]. All T-LGLL patients are characterized by STAT3 activation, that is the hallmark of every T-LGLL, but a higher amount of the phosphorylated protein has been observed in cases with STAT3 mutations (13, 14, 17). Functional studies revealed that even if in different locations, most of the reported mutations lead to a higher protein transcriptional activity and cytokine responsiveness (13, 27). Nevertheless, deep transcriptional expression studies in T-LGLL did not find significant differences that distinguish patients with and without STAT3 mutations, which showed similar overexpression of STAT3-responsive genes (13, 17, 28, 33). These findings suggest that in patients devoid of STAT3 mutations, other mechanisms or lesions can be responsible of the activation of STAT3 pathway.
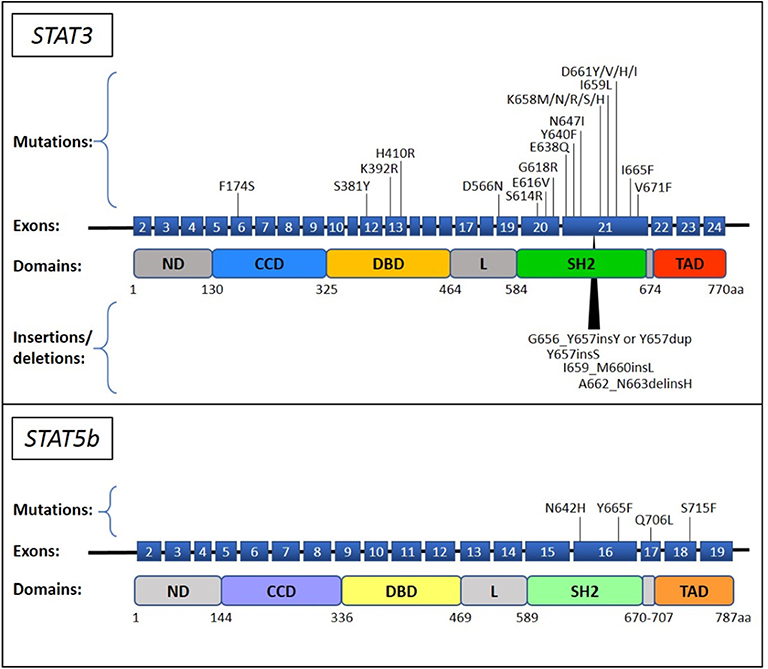
Figure 1. STAT3 and STAT5b mutations described in T-LGLL and CLPD-NK. The STAT3 and STAT5b mutations, reported up to now in literature (13–32), are indicated on their position in the exon upstream the corresponding protein domain. The point mutations and the insertions/deletions are reported above and below the schematic representation of the gene, respectively. ND, N-terminal domain; CCD, coiled-coil domain; DBD, DNA binding domain; L, linker; SH2, Src Homology 2; TAD, transactivation domain.
STAT5b Mutations
Another member of STAT protein family has been reported to carry gain-of-function mutations, namely STAT5b. Initially discovered in only 2% of CD8+ T-LGLL, specifically found in the aggressive form of LGLL (28), STAT5b mutations were subsequently identified in 15–55% of CD4+ T-LGLL (14, 15, 29), and in 19% of TCRγδ LGLL (15). To date, genetic alterations discovered in STAT5b are all point mutations located in the SH2 and in the transactivation domain of the gene (Figure 1). The most recurrent mutations are N642H and Y665F, both deemed to increase the protein activity. At variance with STAT3, STAT5b activation has been observed only in cell samples carrying the mutated protein, whereas the wild type protein is unphosphorylated, similarly to healthy controls (28). Notably, functional studies and transcriptional analysis verified that N642H is able to induce a strong protein activation and to characterize patients with a peculiar gene expression distinguishing them from other patients who are not equipped with this mutation (including patients with STAT wild type, STAT3 mutation and with STAT5b Y665F) (28).
TNFAIP3 Mutations
TNFα-induced protein 3 (TNFAIP3) is another gene recurrently mutated in T-LGLL, that has been found altered in 4 cases within a cohort of 39 patients (24). This gene is a tumor suppressor that encodes A20, a negative regulator of nuclear factor kappa B (NF-kB), whose mutated form likely contributes to deregulate NF-kB activity. Moreover, the association between TNFAIP3 and STAT3 mutations (3 out of 4 cases simultaneously carry both mutations) suggests that TNFAIP3 lesion is not only an alternative genetic mechanism to STAT alterations, but rather an additional event concurring to induce the LGLL phenotype.
Other Less Frequent Gene Mutations
Through deep sequencing, some authors discovered other genes occasionally mutated in T-LGLL, many of them linked to STAT3 signaling pathway and cytotoxic T lymphocyte activation, including PTPRT, BCL11B, PTPN14, PTPN23 (13, 27–29, 33–35). Consistently, a systems genetic assay showed that 5 out of 8 patients devoid of STAT mutations carried alterations on genes “STAT related” or connecting STAT with Ras/MAPK/ERK and IL-15 signaling, including FLT3, ANGPT2, KDR/VEGFR2, and CD40LG (36). These data emphasize the central role of JAK/STAT pathway in T-LGLL patients regardless of STAT mutational pattern. In addition, this analysis demonstrates that several genetic lesions affect different functionally connected genes that can concur to drive a similar phenotype.
Genetics of CLPD-NK
STAT3 and STAT5b Mutations
Although sharing many similarities (37), CLPD-NK is only partially reminiscent of the genetic background of T-LGLL. Since this disease is rarer than the T related entity, less data are available and appropriate analyses are lacking to precisely define this disorder. The similarity with T-LGLL involves STAT3 gene, that is reported mutated also in CLPD-NK [(17, 38); Figure 1]. To note, our data indicate that STAT3 mutations seem to have a lower incidence, from 5.9 to 8.3%, in this disease as compared to T-LGLL (15, 39). Other studies report a frequency of STAT3 mutations from 11 up to 40%; the two studies with the largest number of CLPD-NK patients indicate 15/50 (30%) and 5/40 (13%) cases with STAT3 mutation (17, 40).
Differently from T-LGLL, CLPD-NK appears to be devoid of STAT5b genetic lesions (15, 38, 39), with the only exception of the aggressive case discussed by Rajala et al., who subsequently developed ANKL (28).
Other Less Frequent Gene Mutations
In line with T-LGLL, TNFAIP3 mutation has been found in one out of 17 CLPD-NK patients (5.9%) (38).
Other genetic alterations have been detected through whole exome sequencing (WES) on 3 CLPD-NK patients (all STAT-mutation-negative) by Coppe et al. (36). From this analysis, 31 genes harbored somatic mutations including several “cancer genes” i.e., KRAS, PTK2, NOTCH2, CDC25B, HRASLS, RAB12, PTPRT, and LRBA. More recently, the same authors reported WES data obtained in a larger series of patients indicating that the involvement of JAK/STAT pathway resulted to be not so central as observed in T-LGLL. Otherwise, other pathways and genes were hit by genetic alterations potentially impacting on cell survival, proliferation, chromatin-remodeling, innate immunity, and NK cells activation (41).
Currently, more information is available on ANKL rather than CLPD-NK. Through WES on 14 ANKL patients, Dufva et al. identified alterations in JAK/STAT, RAS/MAPK, and epigenetic modifier genes (42). They found JAK/STAT signaling components frequently altered, with 21% of cases carrying STAT3 mutations, showing that some features are shared by ANKL and the relatively indolent LGLL. The same authors demonstrated an overlapping genetic landscape between ANKL and extranodal NK/T cell lymphoma, nasal type (NKTCL) rather than with CLPD-NK, suggesting that ANKL might represent a more advanced form of NKTCL (42).
STAT Mutation: Founding or Late Event?
The current pathogenetic hypothesis on LGLL development rests on an initial antigen-triggered oligoclonal LGL expansion that only successively develops into a monoclonal lymphocytosis. In this context, STAT mutation seems not to be an inciting but rather an acquired event during the disease, conferring an advantage on the clone development. Indeed, STAT mutations are more frequently found in large clones (18). Interestingly, STAT5b mutations are more often reported on large monoclonal TCR-Vβ expansions (28), whereas, STAT3 mutations are also detected in small subclones (18). Kerr et al., evaluating the relationship between STAT3 mutation and T-cell clone burden, showed that STAT3 mutation frequency can be lower than the T-cell clone entity thus confirming that the mutation is likely to occur as a secondary event within a pre-expanded immunodominant clone (43). The above authors also observed that STAT3 mutation may contribute to an autonomous antigen-independent clonal expansion (43).
Many data have been reported demonstrating that STAT3 mutation does not represent the only factor, itself mandatory, to trigger LGL clonal expansion. In vitro inhibition of STAT3 was observed to restore LGL apoptosis independently from STAT3 mutational status and STAT3 was found activated also in STAT3 wild type LGLL patients (17). Furthermore, analysis on murine cells transduced with retrovirus showed that STAT3 mutants (D661V, Y640F) do not provide any cell growth advantage (44). Similarly, a mouse model demonstrated that the expression of STAT3 mutant alone is not enough to induce LGL leukemia (44), at variance to what had been observed in the mouse model with over-production of IL15 (45). In addition, in a murine bone marrow transplantation model, also Couronne et al. showed that the expression of Y640F mutated STAT3 primarily induces myeloid malignancy rather than LGL disease (46). All these results suggest that additional gene mutations or deregulation due to other signaling molecules or pathways associated with STAT3 mutations might be involved in LGLL pathogenesis (47).
Several points should be made in terms of the STAT5b mutations. STAT5b N642H has been indeed identified as an oncogenic driver in innate-like lymphocytes (48), and a mouse model expressing human N642H mutated STAT5b has been described to develop severe CD8+ T cell neoplasia (49). Provided that IL-15 is an upstream factor of STAT5b and that IL-15 transgenic mice develops the aggressive variant of T or NK cell leukemia (50), the IL-15-STAT5 axis might be considered crucial for neoplastic transformation. The requirement of additional cytokine signals on STAT5b genetic lesions suggests that the indolent course of CD4+ T-LGLL or Tγδ LGLL carrying these genetic lesions might be due to the lack of one or more concurring events together with STAT5b mutations, e.g., cytokine stimulation.
The Clinical Impact of STAT3 and STAT5b Mutations
Isolated neutropenia represents the clinical hallmark of the disease, observed in 40–60% of patients, with approximately half of them developing severe neutropenia. A significant correlation between the presence of STAT3 mutations and neutropenia/symptomatic disease has already been highlighted in several studies (13–16, 22, 25). We hypothesized that the mechanism accounting for neutropenia development involves high levels of STAT3 activation (14). More in detail, we recently demonstrated the presence of a STAT3-miR-146b-FasL axis in neutropenic T-LGLL patients, that, once triggered, leads to high production of Fas Ligand, which in turn is responsible of neutrophil apoptosis (51). These data emphasize the role of STAT3 activation in the pathogenesis of LGLL neutropenia, with STAT3 mutations likely being involved in further boosting this mechanism.
Besides neutropenia, several other clinical features have been described to be more frequent in patients with STAT3 mutations, including different cytopenias or autoimmune diseases. Interestingly, T-LGLL patients with multiple STAT3 mutations have been reported to associate with concomitant rheumatoid arthritis (RA) (52). On the contrary, the association with pure red cell aplasia (PRCA) remains a controversial issue (17, 21, 22, 26).
At variance with STAT3, STAT5b mutation has been reported to have a very different clinical impact. Depending on the immunophenotype of the mutated clone, the presence of STAT5b mutations in the same hotspot positions represents a signature of aggressive clinical course with a poor prognosis in aggressive CD8+ T-LGLL patients (28), while it is devoid of negative prognostic significance in CD4+ T-LGLL and Tγδ LGLL patients (15, 29, 53). The issue is quite intriguing since STAT5b N642H behaves as a driver mutation in several T-cell lymphomas and in the mice model it is enough to induce a leukemic phenotype (48).
The association between STATs mutations and patients' clinical features has been recently confirmed by our data obtained in a large cohort of 205 LGLL patients including all LGLL subtypes, but aggressive T-LGLL and ANKL. We observed that STAT3 mutations were significantly associated with absolute neutrophils count <500/mm3, hemoglobin level <90 g/L and treatment requirement, while STAT5b mutations were found in 15/152 asymptomatic patients. Moreover, by univariate and multivariate analysis, STAT3 mutated status resulted to be associated with reduced overall survival, firstly demonstrating the adverse impact of STAT3 mutations in LGLL patients (15).
Considering that a specific therapy is still missing in LGLL and that current immunosuppressive drugs do not provide satisfying responses, the above-mentioned clinical impact of STAT signaling in LGLL makes these molecules attractive new targets for drug development. Several direct STAT inhibitors interacting with protein domains are available, including Stattic, S3I-201, STA-21 for STAT3 (54) and Pimozide, Stafib2, and Cpd17f for STAT5b (55). However, these compounds induce several off-targets toxicities and severe side-effects that for the time being prevent their use in the clinical setting. To date, no direct STAT3/5b inhibitors of clinical grade are available. However, early-phase clinical trials with drugs targeting STAT3 are ongoing in solid and hematologic malignancies other than LGLL, namely AZD9150, a STAT3 antisense oligonucleotide, and Napabucasin, an inhibitor of gene expression driven by STAT3 (56). In LGLL some compounds against the upstream signaling to STAT have been tested, with preliminary promising results. Tofacitinib citrate, a JAK3-specific inhibitor, showed good response in patients with refractory LGLL associated with RA (30); furthermore, BNZ-1, a multicytokine inhibitor, is currently being tested in LGLL patients in a phase I/II trial (57). Additional studies are needed to confirm these data and the inclusion of STAT mutational status in the work-up is suggested to achieve a personalized treatment of LGLL. Consistently, STAT3 Y640F mutations have been shown to predict response to methotrexate in a small series of patients (7), representing a putative, potential parameter to select the initial best therapy for LGLL patients.
STAT3 and STAT5b Mutations Occur in Phenotypically Distinct LGLL
The correlation found between STAT mutations and LGL immunophenotype has been highlighted, in fact STAT3 and STAT5b mutations are mutually exclusive and preferentially occur in phenotypically distinct leukemic LGLs.
Within Tαβ-LGL disorders, STAT3 mutations characterize the CD8+ T-LGLL and have never been observed in the CD4+ subset (14, 29). Instead, STAT5b mutations have been mainly found in CD4+ TLGLL and also in the rare aggressive form of CD8+ T-LGLL (28), whereas in indolent CD8+ T-LGLL these genetic lesions seem to be rare (14, 29).
Besides this preliminary distinction, a more precise definition of patients harboring STAT mutation can be described, considering the differential immunophenotypic combination of the LGL markers, i.e., CD16, CD56, and CD57. In CD8+ T-LGLL, the CD16+/CD56– phenotype, with or without CD57, is strongly linked to patients characterized by the presence of STAT3 mutation (14). The rare aggressive form of T-LGLL, frequently carrying STAT5b mutations, is discretely characterized by the proliferation of CD8+/CD56+/CD16–/CD57– LGLs (28). Interestingly, STAT5b mutation is found among CD4+ TLGLL patients whose LGL clone is always CD56+, CD16– (14, 29). Similarly, even though CD16 frequently characterizes Tγδ LGLs, in Tγδ LGLL we observed STAT3 mutations in CD56– LGLs, whereas STAT5b mutations were detected in CD56+ phenotype (53).
Also in CLPD-NK discrete subtypes can be identified by flow analysis. We observed that patients with CD56−/dim/CD16high/CD57− cytotoxic NK cells expansion include a subgroup characterized by a more symptomatic disease and the presence of STAT3 mutation (39). For ANKL, otherwise, no STAT mutation-linked phenotypes have been reported.
Taken together, these data suggest that STAT3 mutation can occur in CD16+/CD56– LGLs, whereas STAT5b mutation may be detectable in CD56+ LGL (Figure 2). This link suggests that CD16+/CD56– LGLs and CD56+ LGLs preferentially use STAT3 or STAT5b signaling, respectively, to develop LGL expansion and consequently they are differentially predisposed to be genetically hit. The nature of this relationship remains an open issue that needs to be elucidated. A larger analysis on CLPD-NK and Tγδ LGLL cases is mandatory to get insights also in these less frequent disorders.
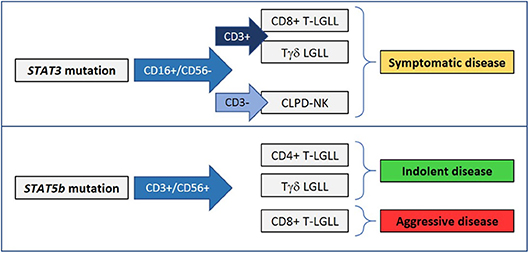
Figure 2. STAT3 and STAT5b mutations are preferentially found in phenotypically distinct LGL disorders and can correlate with different clinical presentations.
Conclusions
The gain-of-function mutations in STAT3 and STAT5b genes up to now remain the most frequent abnormalities in LGLL, even if additional genetic lesions in STAT-related or cancer genes have been described. The complexity on the genetic background indicates that LGLL can be the result of different mechanisms, emphasizing the heterogeneity of the disease, even if a highly frequent recurrent genetic event has not been demonstrated. Being the most common and specific for this disorder, STAT3 mutation currently remains the genetic marker suggestive of LGLL, whereas STAT5b gene is frequently described mutated also in many other hematologic diseases beyond LGLL.
Molecular analysis of STAT3 and STAT5b is unfortunately not so widely available nowadays, but the evidence that STAT mutations have a clinical impact supports the inclusion of this test in the LGLL diagnostic work-up. Moreover, the evaluation of STAT mutations is suggested in combination with LGL immunophenotype data to perform an appropriate classification of indolent, symptomatic and aggressive LGLL patients. STAT3 mutation is indicative of symptomatic disease and reduced patient survival; its evaluation is preferentially suggested for patients affected by CD8 T-LGLL, Tγδ LGLL, and CLPD-NK, particularly when clonal expansion is sustained by CD16+/CD56– LGLs. STAT5b mutation has a controversial significance depending on the immunophenotype of the mutated clone. In fact, it is regarded as the signature of an aggressive clinical course with a poor prognosis in CD8+ TLGLL, characterized by CD56+/CD16–/CD57– LGLs, and aggressive NK cell leukemia, while it is devoid of negative prognostic significance in CD4+ T-LGLL and Tγδ LGLL. Unfortunately, the key factors addressing these two different clinical courses are currently unknown.
STAT3 and STAT5b mutations have been included in the 2017 WHO classification of LGLL with the indication that STAT5b mutation is associated with a more aggressive disease (12). Now this statement needs to be updated (i) highlighting the correlation between STAT3 mutation, symptomatic disease and short patient survival and (ii) adding the issue of discovery of STAT5b genetic lesions also to indolent CD4+ T-LGLL and Tγδ LGLL. Moreover, in terms of aggressive LGLL harboring STAT5b mutation, the current WHO classification recognizes only ANKL, but does not yet recognize the T-related variants as a separate entity. Considering all these findings, the introduction of STAT mutation screening as diagnostic tool, together with a correct immunophenotypic analysis, is encouraged for an accurate characterization of LGLL patients.
Author Contributions
AT wrote the manuscript. AT, GB, and GC discussed the topics of the manuscript. CV and VG contributed to check the data contained in the manuscript. GS and RZ reviewed, edited, and approved the final version of the manuscript.
Funding
This study was supported by Associazione Italiana per la Ricerca sul Cancro to GS (AIRC, IG-20216).
Conflict of Interest
The authors declare that the research was conducted in the absence of any commercial or financial relationships that could be construed as a potential conflict of interest.
References
1. Zambello R, Semenzato G. Large granular lymphocyte disorders: new etiopathogenetic clues as a rationale for innovative therapeutic approaches. Haematologica. (2009) 94:1341–5. doi: 10.3324/haematol.2009.012161
2. Lamy T, Moignet A, Loughran TP Jr. LGL leukemia: from pathogenesis to treatment. Blood. (2017) 129:1082–94. doi: 10.1182/blood-2016-08-692590
3. Barila G, Calabretto G, Teramo A, Vicenzetto C, Gasparini VR, Semenzato G, et al. T cell large granular lymphocyte leukemia and chronic NK lymphocytosis. Best Pract Res Clin Haematol. (2019) 32:207–16. doi: 10.1016/j.beha.2019.06.006
4. Gattazzo C, Teramo A, Passeri F, De March E, Carraro S, Trimarco V, et al. Detection of monoclonal T populations in patients with KIR-restricted chronic lymphoproliferative disorder of NK cells. Haematologica. (2014) 99:1826–33. doi: 10.3324/haematol.2014.105726
5. Semenzato G, Pandolfi F, Chisesi T, De Rossi G, Pizzolo G, Zambello R, et al. The lymphoproliferative disease of granular lymphocytes. A heterogeneous disorder ranging from indolent to aggressive conditions. Cancer. (1987) 60:2971–8. doi: 10.1002/1097-0142(19871215)60:12<2971::AID-CNCR2820601220>3.0.CO;2-O
6. Moignet A, Lamy T. Latest advances in the diagnosis and treatment of large granular lymphocytic leukemia. Am Soc Clin Oncol Educ Book. (2018) 38:616–25. doi: 10.1200/EDBK_200689
7. Loughran TP Jr, Zickl L, Olson TL, Wang V, Zhang D, Rajala HL, et al. Immunosuppressive therapy of LGL leukemia: prospective multicenter phase II study by the Eastern Cooperative Oncology Group (E5998). Leukemia. (2015) 29:886–94. doi: 10.1038/leu.2014.298
8. Zhang R, Shah MV, Yang J, Nyland SB, Liu X, Yun JK, et al. Network model of survival signaling in large granular lymphocyte leukemia. Proc Natl Acad Sci USA. (2008) 105:16308–13. doi: 10.1073/pnas.0806447105
9. Zambello R, Teramo A, Barila G, Gattazzo C, Semenzato G. Activating KIRs in chronic lymphoproliferative disorder of NK cells: protection from viruses and disease induction? Front Immunol. (2014) 5:72. doi: 10.3389/fimmu.2014.00072
10. Epling-Burnette PK, Liu JH, Catlett-Falcone R, Turkson J, Oshiro M, Kothapalli R, et al. Inhibition of STAT3 signaling leads to apoptosis of leukemic large granular lymphocytes and decreased Mcl-1 expression. J Clin Invest. (2001) 107:351–62. doi: 10.1172/JCI9940
11. Yu H, Jove R. The STATs of cancer–new molecular targets come of age. Nat Rev Cancer. (2004) 4:97–105. doi: 10.1038/nrc1275
12. Matutes E. The 2017 WHO update on mature T- and natural killer (NK) cell neoplasms. Int J Lab Hematol. (2018) 40(Suppl. 1):97–103. doi: 10.1111/ijlh.12817
13. Koskela HL, Eldfors S, Ellonen P, van Adrichem AJ, Kuusanmaki H, Andersson EI, et al. Somatic STAT3 mutations in large granular lymphocytic leukemia. N Engl J Med. (2012) 366:1905–13. doi: 10.1056/NEJMoa1114885
14. Teramo A, Barila G, Calabretto G, Ercolin C, Lamy T, Moignet A, et al. STAT3 mutation impacts biological and clinical features of T-LGL leukemia. Oncotarget. (2017) 8:61876–89. doi: 10.18632/oncotarget.18711
15. Barila G, Teramo A, Calabretto G, Vicenzetto C, Gasparini VR, Pavan L, et al. Stat3 mutations impact on overall survival in large granular lymphocyte leukemia: a single-center experience of 205 patients. Leukemia. (2019). doi: 10.1038/s41375-019-0644-0. [Epub ahead of print].
16. Ohgami RS, Ma L, Merker JD, Martinez B, Zehnder JL, Arber DA. STAT3 mutations are frequent in CD30+ T-cell lymphomas and T-cell large granular lymphocytic leukemia. Leukemia. (2013) 27:2244–7. doi: 10.1038/leu.2013.104
17. Jerez A, Clemente MJ, Makishima H, Koskela H, Leblanc F, Peng Ng K, et al. STAT3 mutations unify the pathogenesis of chronic lymphoproliferative disorders of NK cells and T-cell large granular lymphocyte leukemia. Blood. (2012) 120:3048–57. doi: 10.1182/blood-2012-06-435297
18. Fasan A, Kern W, Grossmann V, Haferlach C, Haferlach T, Schnittger S. STAT3 mutations are highly specific for large granular lymphocytic leukemia. Leukemia. (2013) 27:1598–600. doi: 10.1038/leu.2012.350
19. Kristensen T, Larsen M, Rewes A, Frederiksen H, Thomassen M, Moller MB. Clinical relevance of sensitive and quantitative STAT3 mutation analysis using next-generation sequencing in T-cell large granular lymphocytic leukemia. J Mol Diagn. (2014) 16:382–92. doi: 10.1016/j.jmoldx.2014.02.005
20. Clemente MJ, Przychodzen B, Jerez A, Dienes BE, Afable MG, Husseinzadeh H, et al. Deep sequencing of the T-cell receptor repertoire in CD8+ T-large granular lymphocyte leukemia identifies signature landscapes. Blood. (2013) 122:4077–85. doi: 10.1182/blood-2013-05-506386
21. Ishida F, Matsuda K, Sekiguchi N, Makishima H, Taira C, Momose K, et al. STAT3 gene mutations and their association with pure red cell aplasia in large granular lymphocyte leukemia. Cancer Sci. (2014) 105:342–6. doi: 10.1111/cas.12341
22. Qiu ZY, Fan L, Wang L, Qiao C, Wu YJ, Zhou JF, et al. STAT3 mutations are frequent in Tcell large granular lymphocytic leukemia with pure red cell aplasia. J Hematol Oncol. (2013) 6:82. doi: 10.1186/1756-8722-6-82
23. Rajala HL, Olson T, Clemente MJ, Lagstrom S, Ellonen P, Lundan T, et al. The analysis of clonal diversity and therapy responses using STAT3 mutations as a molecular marker in large granular lymphocytic leukemia. Haematologica. (2015) 100:91–9. doi: 10.3324/haematol.2014.113142
24. Johansson P, Bergmann A, Rahmann S, Wohlers I, Scholtysik R, Przekopowitz M, et al. Recurrent alterations of TNFAIP3 (A20) in T-cell large granular lymphocytic leukemia. Int J Cancer. (2016) 138:121–4. doi: 10.1002/ijc.29697
25. Sanikommu SR, Clemente MJ, Chomczynski P, Afable MG II, Jerez A, Thota S, et al. Clinical features and treatment outcomes in large granular lymphocytic leukemia (LGLL). Leuk Lymphoma. (2018) 59:416–22. doi: 10.1080/10428194.2017.1339880
26. Shi M, He R, Feldman AL, Viswanatha DS, Jevremovic D, Chen D, et al. STAT3 mutation and its clinical and histopathologic correlation in T-cell large granular lymphocytic leukemia. Hum Pathol. (2018) 73:74–81. doi: 10.1016/j.humpath.2017.12.014
27. Andersson E, Kuusanmaki H, Bortoluzzi S, Lagstrom S, Parsons A, Rajala H, et al. Activating somatic mutations outside the SH2-domain of STAT3 in LGL leukemia. Leukemia. (2016) 30:1204–8. doi: 10.1038/leu.2015.263
28. Rajala HL, Eldfors S, Kuusanmaki H, van Adrichem AJ, Olson T, Lagstrom S, et al. Discovery of somatic STAT5b mutations in large granular lymphocytic leukemia. Blood. (2013) 121:4541–50. doi: 10.1182/blood-2012-12-474577
29. Andersson EI, Tanahashi T, Sekiguchi N, Gasparini VR, Bortoluzzi S, Kawakami T, et al. High incidence of activating STAT5B mutations in CD4-positive T-cell large granular lymphocyte leukemia. Blood. (2016) 128:2465–8. doi: 10.1182/blood-2016-06-724856
30. Bilori B, Thota S, Clemente MJ, Patel B, Jerez A, Afable Ii M, et al. Tofacitinib as a novel salvage therapy for refractory T-cell large granular lymphocytic leukemia. Leukemia. (2015) 29:24279. doi: 10.1038/leu.2015.280
31. Haapaniemi EM, Kaustio M, Rajala HL, van Adrichem AJ, Kainulainen L, Glumoff V, et al. Autoimmunity, hypogammaglobulinemia, lymphoproliferation, and mycobacterial disease in patients with activating mutations in STAT3. Blood. (2015) 125:639–48. doi: 10.1182/blood-2014-04-570101
32. Morgan EA, Lee MN, DeAngelo DJ, Steensma DP, Stone RM, Kuo FC, et al. Systematic STAT3 sequencing in patients with unexplained cytopenias identifies unsuspected large granular lymphocytic leukemia. Blood Adv. (2017) 1:1786–9. doi: 10.1182/bloodadvances.2017011197
33. Andersson EI, Rajala HL, Eldfors S, Ellonen P, Olson T, Jerez A, et al. Novel somatic mutations in large granular lymphocytic leukemia affecting the STAT-pathway and T-cell activation. Blood Cancer J. (2013) 3:e168. doi: 10.1038/bcj.2013.65
34. Andersson EI, Coppe A, Bortoluzzi S. A guilt-by-association mutation network in LGL leukemia. Oncotarget. (2017) 8:93299–300. doi: 10.18632/oncotarget.21699
35. Raess PW, Cascio MJ, Fan G, Press R, Druker BJ, Brewer D, et al. Concurrent STAT3, DNMT3A, and TET2 mutations in T-LGL leukemia with molecularly distinct clonal hematopoiesis of indeterminate potential. Am J Hematol. (2017) 92:E6–8. doi: 10.1002/ajh.24586
36. Coppe A, Andersson EI, Binatti A, Gasparini VR, Bortoluzzi S, Clemente M, et al. Genomic landscape characterization of large granular lymphocyte leukemia with a systems genetics approach. Leukemia. (2017) 31:1243–6. doi: 10.1038/leu.2017.49
37. Zambello R, Teramo A, Gattazzo C, Semenzato G. Are T-LGL leukemia and NK-chronic lymphoproliferative disorder really two distinct diseases? Transl Med UniSa. (2014) 8:4–11.
38. Kawakami T, Sekiguchi N, Kobayashi J, Yamane T, Nishina S, Sakai H, et al. STAT3 mutations in natural killer cells are associated with cytopenia in patients with chronic lymphoproliferative disorder of natural killer cells. Int J Hematol. (2019) 109:563–71. doi: 10.1007/s12185-019-02625-x
39. Barila G, Teramo A, Calabretto G, Ercolin C, Boscaro E, Trimarco V, et al. Dominant cytotoxic NK cell subset within CLPD-NK patients identifies a more aggressive NK cell proliferation. Blood Cancer J. (2018) 8:51. doi: 10.1038/s41408-018-0088-1
40. Poullot E, Zambello R, Leblanc F, Bareau B, De March E, Roussel M, et al. Chronic natural killer lymphoproliferative disorders: characteristics of an international cohort of 70 patients. Ann Oncol. (2014) 25:2030–5. doi: 10.1093/annonc/mdu369
41. Gasparini VR, Binatti A, Teramo A, Coppe A, Vicenzetto C, Calabretto G, et al. A first highdefinition landscape of somatic mutations in chronic lymphoproliferative disorder of NK cells. In: Jan Cools AE, editor. 24th Congress of the European Hematology Association. Amsterdam: HemaSphere (2019). p. 132–3. doi: 10.1097/01.HS9.0000559660.30415.c9
42. Dufva O, Kankainen M, Kelkka T, Sekiguchi N, Awad SA, Eldfors S, et al. Aggressive natural killer-cell leukemia mutational landscape and drug profiling highlight JAK-STAT signaling as therapeutic target. Nat Commun. (2018) 9:1567. doi: 10.1038/s41467-018-03987-2
43. Kerr CM, Clemente MJ, Chomczynski PW, Przychodzen B, Nagata Y, Adema V, et al. Subclonal STAT3 mutations solidify clonal dominance. Blood Adv. (2019) 3:917–21. doi: 10.1182/bloodadvances.2018027862
44. Dutta A, Yan D, Hutchison RE, Mohi G. STAT3 mutations are not sufficient to induce large granular lymphocytic leukaemia in mice. Br J Haematol. (2018) 180:911–5. doi: 10.1111/bjh.14487
45. Mishra A, Liu S, Sams GH, Curphey DP, Santhanam R, Rush LJ, et al. Aberrant overexpression of IL-15 initiates large granular lymphocyte leukemia through chromosomal instability and DNA hypermethylation. Cancer Cell. (2012) 22:645–55. doi: 10.1016/j.ccr.2012.09.009
46. Couronne L, Scourzic L, Pilati C, Della Valle V, Duffourd Y, Solary E, et al. STAT3 mutations identified in human hematologic neoplasms induce myeloid malignancies in a mouse bone marrow transplantation model. Haematologica. (2013) 98:1748–52. doi: 10.3324/haematol.2013.085068
47. Teramo A, Gattazzo C, Passeri F, Lico A, Tasca G, Cabrelle A, et al. Intrinsic and extrinsic mechanisms contribute to maintain the JAK/STAT pathway aberrantly activated in T-type large granular lymphocyte leukemia. Blood. (2013) 121:3843–54, S1. doi: 10.1182/blood-2012-07-441378
48. Klein K, Witalisz-Siepracka A, Maurer B, Prinz D, Heller G, Leidenfrost N, et al. STAT5BN642H drives transformation of NKT cells: a novel mouse model for CD56+ T-LGL leukemia. Leukemia. (2019) 33:2336–40. doi: 10.1038/s41375-019-0471-3
49. Pham HTT, Maurer B, Prchal-Murphy M, Grausenburger R, Grundschober E, Javaheri T, et al. STAT5BN642H is a driver mutation for T cell neoplasia. J Clin Invest. (2018) 128:387–401. doi: 10.1172/JCI94509
50. Fehniger TA, Suzuki K, Ponnappan A, VanDeusen JB, Cooper MA, Florea SM, et al. Fatal leukemia in interleukin 15 transgenic mice follows early expansions in natural killer and memory phenotype CD8+ T cells. J Exp Med. (2001) 193:219–31. doi: 10.1084/jem.193.2.219
51. Mariotti B, Calabretto G, Rossato M, Teramo A, Castellucci M, Barila G, et al. Identification of a miR-146b-FasL axis in the development of neutropenia in T large granular lymphocyte leukemia. Haematologica. (2019). doi: 10.3324/haematol.2019.225060. [Epub ahead of print].
52. Savola P, Kelkka T, Rajala HL, Kuuliala A, Kuuliala K, Eldfors S, et al. Somatic mutations in clonally expanded cytotoxic T lymphocytes in patients with newly diagnosed rheumatoid arthritis. Nat Commun. (2017) 8:15869. doi: 10.1038/ncomms15869
53. Teramo A, Ciabatti E, Tarrini G, Petrini I, Barila G, Calabretto G, et al. Clonotype and mutational pattern in TCRγδ large granular lymphocyte leukemia. In: Malcovati L, editor. 22nd Congress of the European Hematology Association. Madrid: Haematologica (2017). p. 573.
54. Zhu F, Wang KB, Rui L. STAT3 activation and oncogenesis in lymphoma. Cancers. (2019) 12:E19. doi: 10.3390/cancers12010019
55. Orlova A, Wagner C, de Araujo ED, Bajusz D, Neubauer HA, Herling M, et al. Direct targeting options for STAT3 and STAT5 in cancer. Cancers. (2019) 11:E1930. doi: 10.3390/cancers11121930
56. Yang L, Lin S, Xu L, Lin J, Zhao C, Huang X. Novel activators and small-molecule inhibitors of STAT3 in cancer. Cytokine Growth Factor Rev. (2019) 49:10–22. doi: 10.1016/j.cytogfr.2019.10.005
Keywords: large granular lymphocyte (LGL), T-LGL leukemia (T-LGLL), chronic lymphoproliferative disease of NK cells (CLPD-NK), STAT3, STAT5b, mutation
Citation: Teramo A, Barilà G, Calabretto G, Vicenzetto C, Gasparini VR, Semenzato G and Zambello R (2020) Insights Into Genetic Landscape of Large Granular Lymphocyte Leukemia. Front. Oncol. 10:152. doi: 10.3389/fonc.2020.00152
Received: 21 November 2019; Accepted: 28 January 2020;
Published: 18 February 2020.
Edited by:
Francesco Maura, Memorial Sloan Kettering Cancer Center, United StatesReviewed by:
Bruno Fattizzo, IRCCS Ca 'Granda Foundation Maggiore Policlinico Hospital, ItalyHabibe Kurt, Brown University, United States
Valeria Ferla, IRCCS Ca 'Granda Foundation Maggiore Policlinico Hospital, Italy
Copyright © 2020 Teramo, Barilà, Calabretto, Vicenzetto, Gasparini, Semenzato and Zambello. This is an open-access article distributed under the terms of the Creative Commons Attribution License (CC BY). The use, distribution or reproduction in other forums is permitted, provided the original author(s) and the copyright owner(s) are credited and that the original publication in this journal is cited, in accordance with accepted academic practice. No use, distribution or reproduction is permitted which does not comply with these terms.
*Correspondence: Gianpietro Semenzato, g.semenzato@unipd.it