- 1Institute of Applied Biosciences, Centre for Research and Technology Hellas, Thessaloniki, Greece
- 2Hematology Department, University General Hospital of Thessaloniki AHEPA, Thessaloniki, Greece
The clonotypic B cell receptor immunoglobulin (BcR IG) plays a seminal role in B cell lymphoma development and evolution. From a clinical perspective, this view is supported by the remarkable therapeutic efficacy of BcR signaling inhibitors, even among heavily pre-treated, relapsed/refractory patients. This clinical development complements immunogenetic evidence for antigen drive in the natural history of these tumors. Indeed, BcR IG gene repertoire biases have been documented in different B cell lymphoma subtypes, alluding to selection of B cell progenitors that express particular BcR IG. Moreover, distinct entities display imprints of somatic hypermutation within the clonotypic BcR IG gene following patterns that strengthen the argument for antigen selection. Of note, at least in certain B cell lymphomas, the BcR IG genes are intraclonally diversified, likely in a context of ongoing interactions with antigen(s). Moreover, BcR IG gene repertoire profiling suggests that unique immune pathways lead to distinct B cell lymphomas through targeting cells at different stages in the B cell differentiation trajectory (e.g., germinal center B cells in follicular lymphoma, FL). Regarding the implicated antigens, although their precise nature remains to be fully elucidated, immunogenetic analysis has offered important hints by revealing similarities between the BcR IG of particular lymphomas and B cell clones with known antigenic specificity: this has paved the way to functional studies that identified relevant antigenic determinants of classes of structurally similar epitopes. Finally, in certain tumors, most notably chronic lymphocytic leukemia (CLL), immunogenetic analysis has also proven instrumental in accurate patient risk stratification since cases with differing BcR IG gene sequence features follow distinct disease courses and respond differently to particular treatment modalities. Overall, delving into the BcR IG gene sequences emerges as key to understanding B cell lymphoma pathophysiology, refining prognostication and assisting in making educated treatment choices.
Introduction
B Cell Differentation
Antigen encounter critically shapes the fate of B cells, essentially kicking off two major possible pathways: B cells can either undergo immediate proliferation and differentiation into short-lived plasma cells, or migrate to secondary lymphoid organs and enter specific structures termed germinal centers (GCs). In the former, B cells express polyreactive, low-affinity B cell receptor immunoglobulin (BcR IG) without relevant support from T cells. In the latter, B cells within the GCs are subjected to affinity maturation in a T cell-dependent manner, resulting in the generation of B cells with high-affinity BcR IG, specific for the selecting antigen. Activated B cells with high affinity BcR IG are selectively propagated and mature into two discrete B cell populations: (i) plasma cells that proliferate fast and secrete high-affinity antibodies; and, (ii) long-lived memory B cells capable of eliciting robust responses in future exposure to the original selecting antigen (1–4).
Differentiation Pathways of Mature B Cells
Memory B cells circulate in the body as resting lymphocytes until reactivation and can be distinguished from naïve B cells based on a series of distinct features. In brief, memory B cells: (i) have increased lifespan; (ii) are mainly found in areas where antigen encounter takes place; (iii) display differential expression of quiescence factors, costimulatory and anti-apoptotic molecules as well as signal transducers; (iv) are capable, upon reactivation, to proliferate and differentiate into plasma cells rapidly secreting large amounts of antibodies, thus, providing a more efficient immune response; (v) can re-enter the GC and be subjected to further affinity maturation in order to refine antigen binding specificity. At the molecular level, affinity maturation is geared by two independent yet mechanistically related processes affecting the clonotypic BcR IG i.e., somatic hypermutation (SHM) and class switch recombination (CSR) (5–7).
SHM is a highly specific mechanism introducing mostly single nucleotide changes into the rearranged variable genes of the BcR IG at a rate far exceeding mutational activity elsewhere in the genome. Mutations do not occur randomly but instead tend to cluster within the regions forming the antigen binding site, namely the complementarity-determining regions (CDRs), enriched for specific nucleotide motifs that serve as SHM hotspots. Within the GC, a single B cell progenitor generates B cells expressing different variants of its BcR IG that display distinct affinities for the given antigen. Some of the mutation combinations will result in higher affinity BcR IG and the B cells expressing these receptors will become favored for activation and proliferation (8, 9). SHM targets not only the rearranged IG genes but also some off-target genes, including BCL6, albeit with lower frequency, and even any heterologous sequence introduced downstream the IGHV gene promoter (10).
CSR is the second mechanism participating in affinity maturation within the GCs and is responsible for the change of the BcR IG isotype. There are five different isotypes, namely IgM, IgG, IgA, IgE, and IgD. Naïve B cells express IgM and/or IgD, whereas after antigen encounter B cells can express IgG, IgA, or IgE. CSR changes the constant domain of the BcR IG heavy chain without any effect on the variable domain, leading to the expression of BcR IG with high affinity for the same antigen yet different effector functions (11–13).
Both SHM and CSR depend on the activity of an enzyme called activation-induced cytidine deaminase (AID). AID deaminates a cytosine (C) turning it into uracil (U), thus causing mutations at C-G pairs. A group of low-fidelity DNA polymerases undertakes the repair of the mismatches. In SHM, the U is recognized as thymidine (T) by the repair mechanisms and the C-G pair is converted to T-adenine (A) pair through RNA-editing mechanisms. In CSR, double-stranded DNA (dsDNA) breaks are introduced at the U-G pairs. Joining and repair are targeted by different repair mechanisms leading to chromosomal recombination events (14–16).
In rare instances, memory B cells lack SHM within the BcR IG. A possible explanation for this phenomenon could be that these cells originate from early GC B cells before the actual onset of SHM (5). That said, there is also evidence that B cell activation and the subsequent generation of memory B cells can occur outside the GC environment. Indeed, a GC-independent but T cell-dependent pathway to memory B cell formation has been described, generating memory B cells with limited if any SHM. Furthermore, depending on the type of antigen, the microenvironment where antigen encounter takes place and the particular cytokine milieu, B cells may even undergo SHM and/or CSR outside the GC, although the range of available classes appears to be restricted (17, 18).
A prime example of B cells operating outside the context of GCs concerns the B cell population that resides within the marginal zone (MZ). MZ B cells provide frontline protection against blood borne pathogens and rapidly respond against encapsulated bacteria by differentiating into antigen-specific plasma cells. Additionally, they are responsible for housekeeping activities, since they recognize and remove senescent cells and other cellular debris. In order to serve these functions, MZ B cells express distinctive BcR IG where their antigen reactivity patterns are reflected in a restricted BcR IG gene repertoire. Of note, at least a fraction of MZ B cells, particularly in the spleen, carry SHM within both their BcR IG genes as well as the BCL6 gene, highlighting an active SHM mechanism. Furthermore, splenic MZ B cells share phenotypic similarities with memory B cells and display enhanced immune response potential. These similarities led to the hypothesis that splenic MZ cells are either of post-GC origin or derive from an independent differentiation pathway (19–22).
Cellular Origin of B Cell Lymphomas: Overview
Aberrations at any stage in the differentiation process of mature B cells can lead to uncontrolled proliferation and, ultimately, to the emergence of B cell non-Hodgkin lymphomas (B-NHLs) (23, 24). Antigen experienced B cells, such as GC and memory B cells are widely thought to represent progenitor cells for different types of B-NHL, most notably follicular lymphoma (FL) (25), diffuse large B cell lymphoma (DLBCL) (26, 27), and Burkitt lymphoma (BL) (28–30). A key molecular feature of these lymphomas pertains to the identification of SHM imprints within the variable domain of the clonotypic BcR IG, alluding to antigen exposure. This notion is further supported by the pronounced intraclonal diversification of the IG genes, at least in some of these tumors. One of the most notable examples is FL (31–33), where the analysis of somatic mutations led to the notion that SHM is an ongoing process continuously altering the structure of the clonotypic BcR IG under antigenic pressure.
Along the same lines, the study of the BcR IG expressed by the malignant B cells supported potential reactivity against superantigens, at least for a fraction of BL (34) and DLBCL cases. In more detail, the superantigenic binding motifs for N-acetyllactosamine-containing epitopes and Staphylococcal protein A (SpA) have been found intact in BL cases that carry BcR IGs encoded by the IGHV4-34 gene and IGHV3 subgroup genes (34), respectively. Similar findings have been reported for DLBCL cases utilizing the IGHV4-34 gene (35).
Chronic stimulation of the BcR IG by microbial antigens or autoantigens can promote the expansion and progression of malignant B cells. This is amply exemplified by gastric MALT lymphoma that is strongly associated with chronic infection by Helicobacter pylori (36). Similar links to pathogens have been identified for extranodal MZ lymphomas (ENMZL) of different tissues, such as ocular adnexa MZ lymphoma and cutaneous MZ lymphoma, which have been associated with infections by Chlamydia psitacci and Borrelia burgdorferi, respectively (37). Moreover, ENMZL of the salivary and the thyroid glands have been linked to continuous triggering by autoantigens responsible for Sjögren's syndrome and Hashimoto thyroiditis, respectively (38). Interestingly, BcR IG gene repertoire restrictions and distinctive SHM patterns characterize ENMZL as well, albeit with significantly different IG gene distributions depending on the primary site of involvement, indicating distinct antigen exposure histories (39, 40). Extensive immunogenetic profiling of MZ lymphomas and cross-comparison to other B-NHLs has also documented the existence of rare public BcR IG stereotypes shared by different entities. This finding raises the intriguing possibility that common immune mechanisms triggered by pathogens may underlie the ontogeny of diverse B lymphoproliferations likely due to targeting versatile progenitor B cells in particular microenvironments, including the GC but, perhaps, also extrafollicular sites (39).
The aforementioned examples constitute proof-of-concept about antigen-driven lymphomagenesis, while also highlighting the critical role of affinity maturation processes, particularly SHM, in B-NHL development and evolution. In these examples, the putative cell of origin can be pinpointed with some degree of certainty to a GC or post-GC cell. In the sections that follow, we will start with FL as a reference case for GC-originating lymphomas and then focus on certain other lymphoproliferative entities where the putative cell(s) of origin still remain(s) elusive. We will present the immunogenetic evidence that have assisted in gradually revealing the implicated ontogenetic processes while also acquiring an ever-increasing role in prognostication and clinical decision-making at large.
Follicular Lymphoma
Follicular lymphoma (FL), the second most common nodal lymphoma, is generally considered as a prototype of indolent lymphomas displaying a clinical course that is characterized by slow progression and high response rates to therapy (41). However, a significant fraction of patients with FL eventually develop resistant disease, while in almost half of cases the original indolent disease transforms into an aggressive subtype, such as DLBCL (42, 43).
It is widely established that FL arises in germinal centers, hence maintaining features of normal GCs. In more detail, FL cells form follicles surrounded by non-malignant antigen presenting cells (including T cells, dendritic cells and macrophages) (44); express GC surface markers such as BCL6 and CD10 (44); and, display a gene expression signature that is similar to that of centrocytes and/or centroblasts (26).
Most patients with FL (around 85%) carry the t(14;18) (q32;q21) chromosomal translocation, which constitutes a hallmark of this lymphoma (45). In specific, this translocation involves the bcl-2 gene (B cell leukemia/lymphoma 2) and the IgH (immunoglobulin heavy chain) gene locus, leading to the overexpression of the BCL2 protein that prevents cells from undergoing apoptosis. The increased frequency of t(14;18) in FL together with its presence at diagnosis support its consideration as the initial oncogenetic hit during the development of FL (41).
In regard to the timing of the t(14;18) in the natural history of FL, it was initially accepted that it takes place early in B cell development, during the initial phase of the V(D)J recombination process that involves the rearrangement between a IGHD and a IGHJ gene. However, the analysis of BCL2-IGH junctions (46, 47) provided evidence that the translocation event can also occur at a more advanced stage of the V(D)J recombination process, when an IGHV gene recombines with the assembled IGHD-IGHJ gene complex.
An alternate scenario (41) involved naive B cells carrying the t(14;18). These, previously unselected cells, would exit the bone marrow and move to secondary lymphoid tissues, where they would undergo the GC reaction. The constitutive expression of BCL2, not normally expressed in the GC microenvironemnt, could offer a selective advantage to these cells by protecting them from apoptosis (48). Finally, FL progenitor cells would acquire secondary genetic lesions due to activation induced cytidine deaminase (AID) activity (49) and transform to FL cells. This scenario of FL ontogenesis was challenged by the observation that cells similar to FL progenitor cells can be detected in the blood of healthy individuals who did not develop FL (50–52). Moreover, recent studies showed that most of the FL progenitor cells have probably undergone some kind of selection since they were not naive B cells, but rather IgD+CD27+ (or IgM+CD27+) memory cells that are GC-experienced (53, 54). Similar to FL cells, these FL-like cells in healthy individuals frequently show evidence of class-switch recombination of the translocated IGH allele, whereas the functional allele encodes a surface IgD (or IgM). The role of these FL-like cells in the pathogenesis of FL remains unknown.
Nonetheless, FL progenitor cells do exist and have a far more complicated genetic background than previously thought, as shown in 2 case reports where both the donor and the recipient developed FL after allogeneic hematopoietic cell transplantation (55, 56). In the most recent study (56), the t(14;18) was detected in the donor sample along with 14 of the 15 gene somatic mutations that were present in the tumors from both the donor and the recipient, yet at a much lower frequency.
Our understanding of the origin of FL is further complicated by an entity called FL in situ, which has been proposed as a true precursor lymphoma state (57, 58). However, this entity needs to be further studied in order to gain better insight into its natural history and relation, if any, to early stage FL.
Supraphysiological N-Glycosylation of the Clonotypic Immunoglobulin and Cellular Activation in Follicular Lymphoma
Immunogenetic studies in FL reported an unbiased IGHV gene repertoire. In the vast majority of FL cases, the rearranged IGHV genes carry imprints of SHM, with most mutations located within the CDRs (59–61). The analysis of SHM patterns in FL also revealed pronounced intraclonal diversification, indicating that the lymphoma cells further diversify their IG genes through ongoing SHM (32, 62). IG light chain genes also display imprints of SHM, albeit to a lesser degree than their partner heavy chains (63) (Table 1).
A striking feature of the BcR IG in FL concerns the unusually high incidence of novel N-glycosylation sites (Asn-X-Ser/Thr) introduced by SHM mostly in the CDRs of the heavy chains and less frequently in the light chains (64). BcR IG are known to be variably glycosylated, most commonly within the constant region of the molecule. However, the variable region may also be glycosylated and this modification is known to affect the process of antigen recognition and binding (65). In FL, these novel N-glycosylation sites are present in essentially all cases at diagnosis (64, 66) indicating that they are introduced at early stages of disease ontogeny. These modifications were not frequently present in normal B cells, suggesting their potential relevance for FL pathogenesis (64, 67).
Analysis of the added glycans in the BcR IG from FL revealed that they concern oligomannoses in the variable regions but complex sugars in the constant regions (68). These glycans interact with mannose-specific lectins, especially with dendritic cell-specific intercellular adhesion molecule-3-grabbing non-integrin (DC-SIGN) expressed by dendritic cells, macrophages and lymphatic endothelial cells. Lectin binding to FL triggers persistent activating signals leading to intracellular Ca2+ increase, sustained phosphorylation of the SYK, AKT, PLCγ2, and ERK1/2 kinases downstream the BcR, and increased expression of cMYC (69–71). This finding suggests that interactions between FL cells and the tumor microenvironment affect and promote tumor progression.
Another immunogenetic feature that could likely impact on binding to lectins concerns the IG isotype, however the published evidence remains controversial. In a recent study (70), activation by DC-SIGN occurred in FL cases of either the IgM or the IgG isotype, whereas in another study (71) only IgM+ FL cells could respond to lectin binding. IgG+ FL cases carry BcR IG that are more commonly auto-reactive compared to those expressed by IgM+ FL cases (72). Hence, arguably, although IgG+ FLs contain inserted N-glycosylation sites as in the case of IgM+ FLs, lectin-mediated BcR IG triggering has a greater effect on non-auto-reactive FLs (IgM+). That said, additional studies are required in order to fully elucidate the role, if any, of lectin-induced BcR IG activation in the natural history of IgG+ FL.
Chronic Lymphocytic Leukemia
Chronic lymphocytic leukemia (CLL) is a chronic B cell malignancy, representing 30–40% of all adult leukemias (73). It is a disease of aged individuals with unknown etiology and variable clinical course, ranging from very indolent to rather aggressive, intricately linked to and likely reflecting the underlying biological diversity. Indeed, CLL is characterized by a complex biological landscape. The combined effect of cell-intrinsic aberrations and microenvironmental triggering underlies the characteristic resistance to apoptosis, while also promoting cell proliferation, ultimately driving disease progression (74, 75).
CLL Clones Express a Restricted B Cell Receptor Immunoglobulin Gene Repertoire
Immunogenetic analysis has been at the forefront of CLL research for more than two decades, offering robust evidence that the clonotypic BcR IG engages in specific recognition of and selection by (auto)antigen. This process likely shapes clonal behavior and eventual clinical outcome. It all started in the 1990s when studies reported restricted usage of certain IGHV genes (IGHV1-69, IGHV3-7, IGHV4-34) by CLL cells and, in parallel, documented the existence of SHM patterns consistent with antigen selection in a substantial fraction of cases (76, 77). These findings were subsequently confirmed in larger cohorts strongly implying the selection of CLL progenitor cells by a restricted set of antigens (78, 79).
A turning point in CLL research was the demonstration that roughly 50% of cases utilizing the IGHV3-21 gene displayed highly similar heavy variable CDR3 (VH CDR3). These CLL cases also expressed quasi-identical light chains encoded by the IGLV3-21 gene (80). Clearly at odds with serendipity, this remarkable restriction argued for antigenic pressure leading to the selection of particular features of the clonotypic BcR IG. Soon thereafter, it became apparent that antigen binding site restrictions was a feature of the CLL BcR IG repertoire beyond IGHV3-21 expressing cases: indeed, a sizeable fraction of unrelated CLL patients were assigned to subsets characterized by highly similar, “stereotyped” VH CDR3 sequences. On these grounds, it was reasonably suggested that BcR IGs belonging to the same stereotyped subset were selected by a restricted range of antigenic epitopes (81–88).
Delving deep into this phenomenon in increasingly populated CLL cohorts (89, 90) revealed that BcR IG stereotypes collectively accounted for almost one-third of the BcR IG repertoire in CLL and could be classified into a large number of subsets, ranging in size from only a pair to hundreds of cases (“major” subsets) (Table 1). Inevitably, it became relevant to address the issue of whether stereotyped BcR IGs were exclusive to CLL or could also be found in other B-NHLs and/or other, non-malignant entities. Cross-entity comparisons identified only a few shared homologous VH CDR3, thus revealing that the majority of stereotyped BcR IGs are “CLL-biased.” The few BcR IG sequence matches concerned sequences from autoreactive B cell clones, as well as sequences from diverse B cell lymphoproliferative disorders directly or indirectly linked to infections by certain pathogens (e.g., the hepatitis C virus in the case of CLL stereotyped subset #13) (91). These observations supported the notion that, occasionally, common progenitors may give rise to distinct pathologies. Although the implicated mechanisms remain obscure, this observation underscores the versatility of B cells while also questioning the relevance of “straightforward” one-to-one matching of normal B cell subpopulations and B-NHLs.
Immunogenetics and the Ontogeny of CLL
The presence of SHM within the clonotypic IGHV genes of the malignant clones dichotomize CLL into two broad categories with postulated distinct origin: (i) cases with few or no SHM (unmutated CLL, U-CLL) purportedly originating from B cells at a point of differentiation prior to the accumulation of high levels of SHM in the context of a GC reaction (naïve B cells?); and, (ii) cases with a heavy SHM load (mutated CLL, M-CLL) that could reasonably be thought to derive from B cells antigen-selected in a classic T cell-dependent manner within the GC microenvironment (92). However, despite appearing reasonable, this orderly “binary” theory does not exhaust all potential options and scenarios. One such option could be that at least a fraction of CLL clones might derive from extrafollicular B cells. This concept is grounded on the fact that low-affinity BcR IG with few or no traces of SHM can arise in a T cell-independent manner in the MZ. A similar immunogenetic profile has been reported for memory B cells generated through proliferative expansions early after immunization in a T cell-dependent but GC-independent manner (17) (Figure 1).
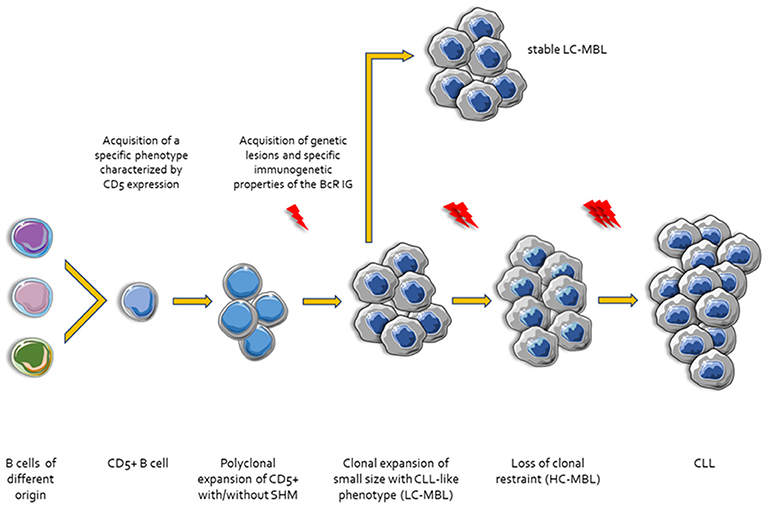
Figure 1. Ontogenetic scenarios for CLL. Different types of B cells stimulated by microenvironmental triggers acquire a specific phenotype characterized by the expression of CD5. Then, CD5+ B cells undergo clonal expansion with or without SHM and CSR. In this step, these small size clonal expansions may acquire genetic lesions display specific CLL-like immunogenetic characteristics and are referred to as Low Count-Monoclonal B-cell Lymphocytosis (LC-MBL). After acquisition of additional genetic and epigenetic changes and/or molecular characteristics of the BcR IG the clones lose the clonal restraint (High Count-MBL, HC-MBL). Finally, HC-MBL cases progress to overt CLL.
Interestingly, CLL monoclonal antibodies (mAbs), especially those with no or limited SHM, display similarities with natural antibodies present in the blood circulation of healthy individuals that recognize non-protein antigenic epitopes common in pathogenic and commensal organisms as well as cellular debris (19, 20). In fact, similar to natural antibodies, recombinant mAbs from U-CLL clones were found to exhibit polyreactivity and low-affinity binding against apoptotic cells and bacteria (93–100). On the other hand, recombinant mAbs from M-CLL clones recognized a more restricted set of antigenic epitopes, yet with higher affinity. Relevant to mention, when mutated mAbs were reverted back to their germline configuration they acquired reactivity against a more extended range of antigens, thus indicating that both U-CLL and M-CLL could derive from autoreactive progenitor B cells (96).
In mice, natural antibodies originate from B-1 cells, a self-renewing CD5+ B cell population with a distinctive, highly restricted BcR IG gene repertoire. The existence of a distinct B-1 lineage with unique properties and role in humans is still contested (101, 102). That notwithstanding, the immunogenetic analogy between B-1 cells and stereotyped CLL clones, especially those belonging to U-CLL, cannot be overlooked, prompting speculations that such clones could originate from a (still elusive) population of evolutionarily conserved B cells functionally intermediate between innate and adaptive immune cells. Put differently, BcR IG stereotypy may reflect origin from a particular cell population characterized by inherent invariance that is responsible for highly conserved, “housekeeping” immune functions (90).
An alternative approach to tracing the cellular origin of CLL was to assess AID expression and functionality. Pioneer studies showed that AID was expressed at higher levels and was more active in vivo in U-CLL compared to M-CLL (103, 104). More extensive studies demonstrated that, in both CLL mutational subgroups, AID was fully functional in only a small fraction of cells that had recently divided or were actively dividing (105). Moreover, it was reported that ex vivo exposure of CLL cells, from both U-CLL and M-CLL, to stimuli mimicking T cell triggering resulted in upregulated expression of AID (105). These intriguing findings challenged a naïve origin for U-CLL, instead supporting that the properties of the corresponding unmutated or minimally mutated BcR IG were optimal for clonal vigor, hence functionally selected.
Additional in vitro studies focusing on the signaling capacity of the BcR IG showed differential signaling capacities in U-CLL and M-CLL (106–109). The observed differences in the ability to signal through the BcR IG can be attributed to either differences in the nature and strength of antigenic stimulation or the different cellular origin of U-CLL and M-CLL. In specific, mutated BcR IGs were more often associated with decreased signaling capacity due to desensitization, ultimately leading to anergy. In contrast, U-CLL cases were shown to express more competent BcR IG indicating that antigenic stimulation can promote the survival and growth of the leukemic cells possibly explaining disease aggressiveness (110–112).
Besides classic antigen-driven stimulation, cell-autonomous signaling triggered by BcR IG self-association has been proposed as an alternative mode of activation exclusive for CLL amongst all studied mature B-NHLs. This signaling mode was shown to promote Ca2+ influx and nuclear factor-κB target gene transcription without the implication of exogenous antigen (113). Cell-autonomous signaling was also shown to play a role in leukemia development in the EμTCL1 mouse model that represents an established animal model of aggressive CLL (114).
Recently, we investigated the mechanism of cell-autonomous signaling in cases belonging to stereotyped subsets #2 and #4 that represent paradigmatic examples of aggressive and indolent disease, respectively. We documented BcR IG self-association in both subsets, albeit with different, subset-specific patterns of interactions and resultant cell activation status (115). These findings are not only relevant to CLL biology but also offer a novel perspective to BcR antagonism as an potential therapeutic strategy for CLL.
Immunogenetics in Clinical Decision-Making
In 1999 two independent studies reported that the SHM status of the clonotypic rearranged IGHV genes has important prognostic implications since U-CLL patients generally experience a significantly more aggressive disease course compared to M-CLL patients (116, 117). In the ensuing years, IGHV gene SHM status emerged as one of the most robust prognostic markers, independent of clinical stage or other biomarkers. More importantly, it remains stable overtime, thus contrasting other well-established prognostic/predictive markers, including genomic aberrations, which are influenced by or reflect disease evolution (118).
Thanks to the evidence amassed over the last 20 years, it is now widely held that the SHM status of the rearranged IGHV gene represents a cornerstone for the development of biologically-grounded prognostic schemes enabling accurate risk stratification in CLL. This notion is supported, amongst others, by the asymmetric distribution of cell-intrinsic aberrations with important prognostic/predictive value (e.g., cytogenetic aberrations and gene mutations) between U-CLL vs. M-CLL (119–121). Characteristic examples concern the adverse-prognostic del(17p) and del(11q) that are significantly enriched in the former, thus contrasting MYD88 mutations which predominate by far in the latter. Interestingly, even more striking associations between immunogenetic characteristics and oncogenetic aberrations have been reported in CLL subsets. For instance, stereotyped subset #2 exhibits a 45–50% incidence of SF3B1 mutations as opposed to only 5–10% in generic CLL cohorts (122, 123); moreover, stereotyped subset #8 (IGHV4-39/IGKV1(D)-39), the CLL subgroup with the highest risk for Richter's transformation amongst all CLL (124), exhibits a ~60% incidence of trisomy 12 as opposed to only ~15% in generic CLL cohorts (120). Subsequently, similar observations have been made for other B-NHL as well e.g., significant enrichment of (i) TNFAIP3 mutations in ocular adnexa lymphoma cases expressing IGHV4-34 BcR IG (125, 126); (ii) KLF2 mutations in splenic marginal zone lymphoma cases expressing IGHV1-2*04 BcR IG (127–129). These findings indicate that a distinctive signaling capacity shaped by particular BcR IG may favor the acquisition and/or selection of certain ongogenetic hits by as yet undetermined mechanisms.
Returning to prognostication, another point to consider concerns the differing relative significance of cell-intrinsic aberrations in U-CLL vs. M-CLL. Hence, trisomy 12 appears to be associated with a rather favorable outcome when present in U-CLL whereas the opposite holds true for M-CLL. A similar observation has been made for TP53 aberrations [del(17p) and/or TP53 mutations], where M-CLL patients showed significantly longer time-to-first-tratment (TTFT) and overall survival (OS) compared to U-CLL patients carrying the same genomic aberrations IG (130, 131). Prompted by these observations, more recently, we followed a compartmentalized approach in order to study the prognosis of CLL patients divided into M-CLL and U-CLL and identied distinct factors contributing to disease prognostication in each subgroup (132). In detail, whereas TP53 aberrations were associated with inferior outcome in both M-CLL and U-CLL, trisomy 12 and/or stereotyped subset #2 membership constituted adverse prognosticators for the former whereas SF3B1 mutations and del(11q) had the most significant negative impact amongst the latter (132) (Figure 2).
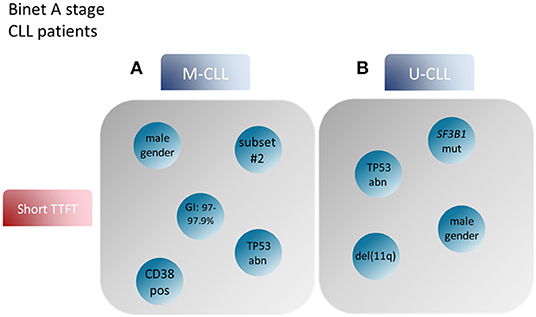
Figure 2. Prognostic factors for CLL patient groups based on the mutational status of the BcR IG. Modified after Baliakas et al. (132). (A) Prognostic factors such as male gender, mutational status around the 2% cutoff, TP53abn, +12 and assignment to stereotyped subset #2 correlated with short TTFT in M-CLL. (B) On the other hand, male gender, TP53abn, SF3B1 mutations and del(11q) in U-CLL patients were associated with similar, short TTFT (99). BcR IG, B cell receptor immunoglobulin; TTFT, time to first treatment; TP53abn, del(17p) and/or TP53 mutations; +12, trisomy 12; M-CLL, CLL with mutated IGHV genes; U-CLL, CLL with unmutated IGHV genes.
With hindsight, the 1999 publications from the Chiorazzi and Stevenson groups on IGHV gene SHM status and prognostication in CLL represent a true landmark in cancer research due to linking a “physiological” mark of immune maturation with disease outcome (116, 117). The immediate, direct effect was to spur an intense study of IG genes: thus, a research activity reserved for specialized labs became a test for routine diagnostic labs. This led to the amassment of IG gene sequences from thousands of patients, a true treasure trove of information with not only prognostic but also biological relevance. The late, indirect effect was a major contribution toward a paradigm change in CLL treatment brought about by the advent of BcR signaling inhibitors (BCRi) i.e., ibrutinib, a BTK inhibitor, and idelalisib, a PI3Kδ inhibitor (133, 134).
Additional evidence strengthening the clinical importance of immunogenetics in CLL came about more recently from studies showing that IGHV gene SHM status was also strongly associated with the clinical response to chemoimmunotherapy (more particularly the fludarabine cyclophosphamide rituximab regimen, FCR). In more detail, the duration of 1st complete remission (CR) was significantly shorter in U-CLL compared to M-CLL, highlighting that theurapeutic interventions should be tailored to the SHM status (135). Of note, such effect was not seen with ibrutinib as 1st line treatment, where this agent proved equally effective across both U-CLL and M-CLL (133). On this evidence, the 2018 iwCLL guidelines explicitly state that this biomarker should be assessed for all CLL patients in both general practice and clinical trials (136).
Is everything settled when it comes to immunogenetics and clinical decision making? For a number of reasons, the answer appears to be no. First, the distinction into U-CLL vs. M-CLL relies on the use of a 98% cut-off value of identity between the clonotypic rearranged gene and its closest germline counterpart. Although this cut-off still holds, caution is warranted for M-CLL cases with IGHV germline identity close to the 98% value (i.e., 97–97.9%) for which the term “IG-borderline” was coined. These cases do not appear to represent a homogeneous group with intermediate prognosis but rather a mix of indolent and aggressive cases, clearly indicating the need for further study (137). Second, ample evidence now exists that subgroups of patients within each mutational group (i.e., M-CLL and U-CLL) may deviate from the expected “norm” for that group: the most compelling case concerns stereotyped subsets.
Indeed, the study of major CLL stereotyped subsets has revealed that similarities between cases in a given subset extend beyond immunogenetic and other biological characteristics of the malignant clones (genomic aberrations, epigenomic status, BcR IG 3D conformation and signaling capacity) to disease course and outcome (108, 115, 120, 138–141). A most characteristic example concerns stereotyped subsets #2 (IGHV3-21/IGLV3-21 BcR IG) and #4 (IGHV4-34/IGKV2-30 BcR IG). Though both concern mostly M-CLL, the former displays a very aggressive clinical course similar to that of patients with TP53 aberrations, despite rarely carrying such lesions, thus sharply contrasting subset #4 that has emerged as the prototype of indolent CLL (120). Therefore, BcR IG stereotypy may assist meaningfully in refining prognostication in CLL beyond the binary M-CLL vs. U-CLL distinction, although more evidence is essential before integrating this information into clinical decision making, a view also shared by the recent iwCLL guidelines.
Mantle Cell Lymphoma
Mantle cell lymphoma (MCL) is an aggressive lymphoma representing 5–10% of all B-NHL. In sharp contrast to the remarkably heterogeneous profile of genomic aberrations in CLL, MCL is characterized by the almost ubiquitous presence of the t(11;14) (q13;q32) chromosomal translocation underlying the formation of the IGH/CCND1 fusion gene and, ultimately, leading to cyclin D1 overexpression, a true pathologic hallmark of MCL (142, 143).
Traditionally, MCL has been associated with a rather aggressive clinical course. However, MCL patients with specific clinical characteristics, such as those with non-nodal disease presentation, tend to follow a more indolent course of the disease, characterized by prolonged TTFT and PFS as well as excellent OS (144). Until recently, the reason for this divergent clinical behavior, especially in view of the quite consistent genomic landscape, has remained elusive.
Immunogenetics in MCL: An Indelible Imprint of Antigen Selection
Immunogenetic studies from the early 2000s reported overexpression of certain IGHV genes in MCL, alluding to functional selection (145–150). However, due to the small sample size of these studies, definitive conclusions could not be drawn until almost a decade later when the analysis of a large MCL series (>800 cases) provided compelling evidence implicating antigen involvement in disease ontogeny (151). In more detail, just four IGHV genes, namely IGHV3-21, IGHV4-34, IGHV1-8, and IGHV3-23, collectively accounted for almost 50% of the total repertoire. SHM was present in the clonotypic rearranged IGHV genes of ~70% of MCL cases with patterns indicative of a post-GC derivation; moreover, in analogy to CLL, asymmetries were noted regarding IGHV gene usage in subgroups of MCL with distinct SHM status. Last but not least, stereotyped BcR IG were documented in MCL based on the presence of specific gene associations and shared amino acid motifs within the VH CDR3 region. Stereotyped subsets in MCL represented almost 10% of all cases, the vast majority utilizing either the IGHV3-21 or the IGHV4-34 gene (Table 1).
Not paradoxically, comparisons were made to CLL, especially regarding BcR IG stereotypes and SHM patterns, revealing disease-biased profiles. Furthermore, stereotyped BcR IG utilizing the same IGHV gene (e.g., IGHV3-21 or IGHV4-34) clearly differed in MCL vs. CLL, hence could be safely considered as disease-specific (151). Furthermore, several recurrent SHM present in rearrangements of a given IGHV gene were found to be “MCL-biased” since they were either under-represented or completely absent in other B cell malignancies. Overall, these findings constituted a unique profile arguing compellingly for antigen selection in MCL ontogeny (151).
Prompted by the CLL case, many studies have investigated whether IGHV gene SHM status may serve as a prognosticator also in MCL, however the obtained results were not always consistent (146–149). Still, a tendency of MCL patients with a heavier SHM load to experience a more indolent disease was evident. These patients presented more frequently with early-stage disease, absence of bone marrow infiltration and non-nodal disease and, moreover, were characterized by lower relapse rates and longer relapse-free survival (149, 152) (Table 1).
How Many Ontogenetic Pathways to MCL?
Considering the evidence presented above, one could draw a plausible ontogenetic scenario for MCL whereby cases with unmutated BcR IG might derive from a pre-GC naïve B cell, whereas those with mutated BcR IG might derive from an antigen-experienced, post-GC B cell (142). In line with this, SOX11-positive, BcR IG-unmutated clones had a gene expression profile (GEP) similar to that of naïve B cells, whereas the GEP of SOX11-negative, BcR IG-mutated patients was close to that of memory B cells (152).
However, other evidence questions this scenario. First, BcR IG stereotypes, a telltale sign of selection, were found even in cases lacking any SHM within the clonotypic IGHV genes (151). Second, almost all MCL cases express AID, with significantly higher levels amongst those with unmutated BcR IG (153), whereas a fraction of cases exhibit ongoing CSR in vivo (154). Third, MCL clones, regardless their SHM status, respond favorably to the BTK inhibitor ibrutinib (155). Hence, alternative ontogenetic scenarios can be envisioned (156), including, amongst others, a normal B cell subpopulation intermediate between naïve and GC cells, with a low impact of SHM, and an IgM+IgD+CD27−CD23−CD5+CD10− phenotype (157); B cells maturing in GC-independent but T cell-dependent pathways; B cells participating in early phases of GC reactions, prior to class switching etc. (Figure 3).
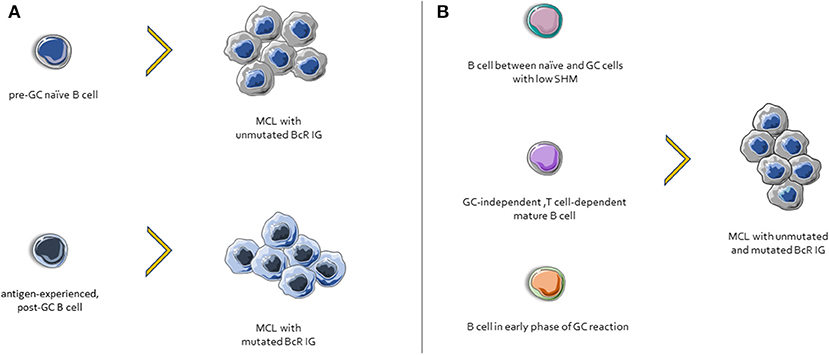
Figure 3. Possible scenarios for MCL ontogenesis. (A) Cases with unmutated BcR IG derive from naïve B cells, whereas those with mutated BcR IG might derive from an antigen-experienced, post-GC B cells. (B) Other possible MCL progenitor cells include: (i) a normal B cell subpopulation between naïve and GC cells, characterized by few SHMs, (ii) cells that differentiated in a GC-independent but T cell-dependent microenvironment, and (iii) B cells from early phases of GC reactions.
Amidst all this uncertainty, one thing is certain: naivety does not become MCL!
Concluding Remarks
B cell maturation is a highly complex process where even small derogations can pave the way to the development of malignancy. Critical for the neoplastic transformation of mature B cells is the communication with the tumor microenvironment, more specifically, antigenic stimulation. The key role of antigens in the onset and evolution of mature B cell lymphomas has been corroborated by ever-growing evidence from different, complementary research fields. Immunogenetics has been at the forefront of such endeavors and is reasonably anticipated to hold its leading role in the years to come.
Author Contributions
KG, AA, LZ-I, and ES wrote the manuscript. MP, AC, and KS supervised and wrote the manuscript.
Funding
This work was supported by COSMIC, a Marie Curie European Training Network funded from the European Union's Horizon 2020 research and innovation programme under grant agreement no. 765158; NOVEL, an ERANET Transcan-2 project; and, CLLon, funded by the Hellenic Foundation for Research and Innovation and the General Secretariat for Research and Technology of Greece (CLLon grant agreement no. 336).
Conflict of Interest
The authors declare that the research was conducted in the absence of any commercial or financial relationships that could be construed as a potential conflict of interest.
References
1. Monroe JG, Dorshkind K. Fate decisions regulating bone marrow and peripheral B lymphocyte development. Adv Immunol. (2007) 95:1–50. doi: 10.1016/S0065-2776(07)95001-4
2. Zhang Y, Garcia-Ibanez L, Toellner KM. Regulation of germinal center B-cell differentiation. Immunol Rev. (2016) 270:8–19. doi: 10.1111/imr.12396
3. Hardy RR, Hayakawa K. B cell development pathways. Annu Rev Immunol. (2001) 19:595–621. doi: 10.1146/annurev.immunol.19.1.595
4. Casola S. Control of peripheral B-cell development. Curr Opin Immunol. (2007) 19:143–9. doi: 10.1016/j.coi.2007.02.010
5. Seifert M, Kuppers R. Human memory B cells. Leukemia. (2016) 30:2283–92. doi: 10.1038/leu.2016.226
6. Kurosaki T, Aiba Y, Kometani K, Moriyama S, Takahashi Y. Unique properties of memory B cells of different isotypes. Immunol Rev. (2010) 237:104–16. doi: 10.1111/j.1600-065X.2010.00939.x
7. Tangye SG, Tarlinton DM. Memory B cells: effectors of long-lived immune responses. Eur J Immunol. (2009) 39:2065–75. doi: 10.1002/eji.200939531
8. Maul RW, Gearhart PJ. Controlling somatic hypermutation in immunoglobulin variable and switch regions. Immunol Res. (2010) 47:113–22. doi: 10.1007/s12026-009-8142-5
9. Peled JU, Kuang FL, Iglesias-Ussel MD, Roa S, Kalis SL, Goodman MF, et al. The biochemistry of somatic hypermutation. Annu Rev Immunol. (2008) 26:481–511. doi: 10.1146/annurev.immunol.26.021607.090236
10. Migliazza A, Martinotti S, Chen W, Fusco C, Ye BH, Knowles DM, et al. Frequent somatic hypermutation of the 5' noncoding region of the BCL6 gene in B-cell lymphoma. Proc Natl Acad Sci USA. (1995) 92:12520–4. doi: 10.1073/pnas.92.26.12520
11. Xu Z, Zan H, Pone EJ, Mai T, Casali P. Immunoglobulin class-switch DNA recombination: induction, targeting and beyond. Nat Rev Immunol. (2012) 12:517–31. doi: 10.1038/nri3216
12. Stavnezer J, Schrader CE. IgH chain class switch recombination: mechanism and regulation. J Immunol. (2014) 193:5370–8. doi: 10.4049/jimmunol.1401849
13. Stavnezer J, Guikema JE, Schrader CE. Mechanism and regulation of class switch recombination. Annu Rev Immunol. (2008) 26:261–92. doi: 10.1146/annurev.immunol.26.021607.090248
14. Pavri R, Nussenzweig MC. AID targeting in antibody diversity. Adv Immunol. (2011) 110:1–26. doi: 10.1016/B978-0-12-387663-8.00005-3
15. Cogne M. Activation-induced deaminase in B lymphocyte maturation and beyond. Biomed J. (2013) 36:259–68. doi: 10.4103/2319-4170.113191
16. Gazumyan A, Bothmer A, Klein IA, Nussenzweig MC, McBride KM. Activation-induced cytidine deaminase in antibody diversification and chromosome translocation. Adv Cancer Res. (2012) 113:167–90. doi: 10.1016/B978-0-12-394280-7.00005-1
17. Takemori T, Kaji T, Takahashi Y, Shimoda M, Rajewsky K. Generation of memory B cells inside and outside germinal centers. Eur J Immunol. (2014) 44:1258–64. doi: 10.1002/eji.201343716
18. Seifert M, Kuppers R. Molecular footprints of a germinal center derivation of human IgM+(IgD+)CD27+ B cells and the dynamics of memory B cell generation. J Exp Med. (2009) 206:2659–69. doi: 10.1084/jem.20091087
19. Weill JC, Weller S, Reynaud CA. Human marginal zone B cells. Annu Rev Immunol. (2009) 27:267–85. doi: 10.1146/annurev.immunol.021908.132607
20. Cerutti A, Cols M, Puga I. Marginal zone B cells: virtues of innate-like antibody-producing lymphocytes. Nat Rev Immunol. (2013) 13:118–32. doi: 10.1038/nri3383
21. Martin F, Kearney JF. Marginal-zone B cells. Nat Rev Immunol. (2002) 2:323–35. doi: 10.1038/nri799
22. Pillai S, Cariappa A, Moran ST. Marginal zone B cells. Ann Rev Immun. (2005) 23:161–96. doi: 10.1146/annurev.immunol.23.021704.115728
23. Dunn-Walters D, Thiede C, Alpen B, Spencer J. Somatic hypermutation and B-cell lymphoma. Philos Transact R Soc Lond Ser B Biol Sci. (2001) 356:73–82. doi: 10.1098/rstb.2000.0751
24. Klein U, Dalla-Favera R. Germinal centres: role in B-cell physiology and malignancy. Nat Rev Immunol. (2008) 8:22–33. doi: 10.1038/nri2217
25. Stamatopoulos K, Kosmas C, Belessi C, Stavroyianni N, Kyriazopoulos P, Papadaki T. Molecular insights into the immunopathogenesis of follicular lymphoma. Immunol Today. (2000) 21:298–305. doi: 10.1016/S0167-5699(00)01650-9
26. Alizadeh AA, Eisen MB, Davis RE, Ma C, Lossos IS, Rosenwald A, et al. Distinct types of diffuse large B-cell lymphoma identified by gene expression profiling. Nature. (2000) 403:503–11. doi: 10.1038/35000501
27. Lossos IS, Alizadeh AA, Eisen MB, Chan WC, Brown PO, Botstein D, et al. Ongoing immunoglobulin somatic mutation in germinal center B cell-like but not in activated B cell-like diffuse large cell lymphomas. Proc Natl Acad Sci USA. (2000) 97:10209–13. doi: 10.1073/pnas.180316097
28. Amato T, Abate F, Piccaluga P, Iacono M, Fallerini C, Renieri A, et al. Clonality analysis of immunoglobulin gene rearrangement by next-generation sequencing in endemic burkitt lymphoma suggests antigen drive activation of BCR as opposed to sporadic burkitt lymphoma. Am J Clin Pathol. (2016) 145:116–27. doi: 10.1093/ajcp/aqv011
29. Chapman CJ, Wright D, Stevenson FK. Insight into Burkitt's lymphoma from immunoglobulin variable region gene analysis. Leukemia Lymphoma. (1998) 30:257–67. doi: 10.3109/10428199809057539
30. Klein U, Klein G, Ehlin-Henriksson B, Rajewsky K, Kuppers R. Burkitt's lymphoma is a malignancy of mature B cells expressing somatically mutated V region genes. Mol Med. (1995) 1:495–505. doi: 10.1007/BF03401587
31. Levy S, Mendel E, Kon S, Avnur Z, Levy R. Mutational hot spots in Ig V region genes of human follicular lymphomas. J Exp Med. (1988) 168:475–89. doi: 10.1084/jem.168.2.475
32. Zelenetz AD, Chen TT, Levy R. Clonal expansion in follicular lymphoma occurs subsequent to antigenic selection. J Exp Med. (1992) 176:1137–48. doi: 10.1084/jem.176.4.1137
33. Zhu D, Hawkins RE, Hamblin TJ, Stevenson FK. Clonal history of a human follicular lymphoma as revealed in the immunoglobulin variable region genes. Br J Haematol. (1994) 86:505–12. doi: 10.1111/j.1365-2141.1994.tb04780.x
34. Baptista MJ, Calpe E, Fernandez E, Colomo L, Cardesa-Salzmann TM, Abrisqueta P, et al. Analysis of the IGHV region in Burkitt's lymphomas supports a germinal center origin and a role for superantigens in lymphomagenesis. Leukemia Res. (2014) 38:509–15. doi: 10.1016/j.leukres.2014.01.001
35. Young RM, Wu T, Schmitz R, Dawood M, Xiao W, Phelan JD, et al. Survival of human lymphoma cells requires B-cell receptor engagement by self-antigens. Proc Natl Acad Sci USA. (2015) 112:13447–54. doi: 10.1073/pnas.1514944112
36. Bertoni F, Coiffier B, Salles G, Stathis A, Traverse-Glehen A, Thieblemont C, et al. MALT lymphomas: pathogenesis can drive treatment. Oncology. (2011) 25:1134–42, 47.
37. Ferreri AJ, Govi S, Ponzoni M. Marginal zone lymphomas and infectious agents. Semin Cancer Biol. (2013) 23:431–40. doi: 10.1016/j.semcancer.2013.09.004
38. Bende RJ, van Maldegem F, van Noesel CJ. Chronic inflammatory disease, lymphoid tissue neogenesis and extranodal marginal zone B-cell lymphomas. Haematologica. (2009) 94:1109–23. doi: 10.3324/haematol.2009.005983
39. Xochelli A, Bikos V, Polychronidou E, Galigalidou C, Agathangelidis A, Charlotte F, et al. Disease-biased and shared characteristics of the immunoglobulin gene repertoires in marginal zone B cell lymphoproliferations. J Pathol. (2019). 247:416–21. doi: 10.1002/path.5209
40. Dagklis A, Ponzoni M, Govi S, Cangi MG, Pasini E, Charlotte F, et al. Immunoglobulin gene repertoire in ocular adnexal lymphomas: hints on the nature of the antigenic stimulation. Leukemia. (2012) 26:814–21. doi: 10.1038/leu.2011.276
41. Kridel R, Sehn LH, Gascoyne RD. Pathogenesis of follicular lymphoma. J Clin Investig. (2012) 122:3424–31. doi: 10.1172/JCI63186
42. Bastion Y, Sebban C, Berger F, Felman P, Salles G, Dumontet C, et al. Incidence, predictive factors, and outcome of lymphoma transformation in follicular lymphoma patients. J Clin Oncol. (1997) 15:1587–94. doi: 10.1200/JCO.1997.15.4.1587
43. Montoto S, Davies AJ, Matthews J, Calaminici M, Norton AJ, Amess J, et al. Risk and clinical implications of transformation of follicular lymphoma to diffuse large B-cell lymphoma. J Clin Oncol. (2007) 25:2426–33. doi: 10.1200/JCO.2006.09.3260
44. Sabattini E, Bacci F, Sagramoso C, Pileri SA. WHO classification of tumours of haematopoietic and lymphoid tissues in 2008: an overview. Pathologica. (2010) 102:83–7.
45. Weiss LM, Warnke RA, Sklar J, Cleary ML. Molecular analysis of the t(14;18) chromosomal translocation in malignant lymphomas. N Engl J Med. (1987) 317:1185–9. doi: 10.1056/NEJM198711053171904
46. Stamatopoulos K, Kosmas C, Belessi C, Papadaki T, Afendaki S, Anagnostou D, et al. t(14;18) chromosomal translocation in follicular lymphoma: an event occurring with almost equal frequency both at the D to J(H) and at later stages in the rearrangement process of the immunoglobulin heavy chain gene locus. Br J Haematol. (1997) 99:866–72. doi: 10.1046/j.1365-2141.1997.4853290.x
47. Johnson PWM, Price CGA, Smith T, Cotter FE, Meerabux J, Rohatiner AZS, et al. Detection of cells bearing the T(1418) translocation following myeloablative treatment and autologous bone-marrow transplantation for follicular lymphoma. J Clin Oncol. (1994) 12:798–805. doi: 10.1200/JCO.1994.12.4.798
48. Roulland S, Faroudi M, Mamessier E, Sungalee S, Salles G, Nadel B. Early steps of follicular lymphoma pathogenesis. Adv Immunol. (2011) 111:1–46. doi: 10.1016/B978-0-12-385991-4.00001-5
49. Pasqualucci L, Bhagat G, Jankovic M, Compagno M, Smith P, Muramatsu M, et al. AID is required for germinal center-derived lymphomagenesis. Nat Genet. (2008) 40:108–12. doi: 10.1038/ng.2007.35
50. Limpens J, Stad R, Vos C, de Vlaam C, de Jong D, van Ommen GJ, et al. Lymphoma-associated translocation t(14;18) in blood B cells of normal individuals. Blood. (1995) 85:2528–36. doi: 10.1182/blood.V85.9.2528.bloodjournal8592528
51. Dolken G, Illerhaus G, Hirt C, Mertelsmann R. BCL-2/JH rearrangements in circulating B cells of healthy blood donors and patients with nonmalignant diseases. J Clin Oncol. (1996) 14:1333–44. doi: 10.1200/JCO.1996.14.4.1333
52. Schuler F, Dolken L, Hirt C, Kiefer T, Berg T, Fusch G, et al. Prevalence and frequency of circulating t(14;18)-MBR translocation carrying cells in healthy individuals. Int J Cancer. (2009) 124:958–63. doi: 10.1002/ijc.23958
53. Hirt C, Dolken G, Janz S, Rabkin CS. Distribution of t(14;18)-positive, putative lymphoma precursor cells among B-cell subsets in healthy individuals. Br J Haematol. (2007) 138:349–53. doi: 10.1111/j.1365-2141.2007.06671.x
54. Roulland S, Navarro J, Grenot P, Milili M, Agopian J, Montpellier B, et al. Follicular lymphoma-like B-cells in healthy individuals: a novel intermediate step in early lymphomagenesis. Blood. (2006) 108:247a-a. doi: 10.1182/blood.V108.11.821.821
55. Carlotti E, Wrench D, Matthews J, Iqbal S, Davies A, Norton A, et al. Transformation of follicular lymphoma to diffuse large B-cell lymphoma may occur by divergent evolution from a common progenitor cell or by direct evolution from the follicular lymphoma clone. Blood. (2009) 113:3553–7. doi: 10.1182/blood-2008-08-174839
56. Weigert O, Kopp N, Lane AA, Yoda A, Dahlberg SE, Neuberg D, et al. Molecular ontogeny of donor-derived follicular lymphomas occurring after hematopoietic cell transplantation. Cancer Discov. (2012) 2:47–55. doi: 10.1158/2159-8290.CD-11-0208
57. Jegalian AG, Eberle FC, Pack SD, Mirvis M, Raffeld M, Pittaluga S, et al. Follicular lymphoma in situ: clinical implications and comparisons with partial involvement by follicular lymphoma. Blood. (2011) 118:2976–84. doi: 10.1182/blood-2011-05-355255
58. Cong PJ, Raffeld M, Teruya-Feldstein J, Sorbara L, Pittaluga S, Jaffe ES. In situ localization of follicular lymphoma: description and analysis by laser capture microdissection. Blood. (2002) 99:3376–82. doi: 10.1182/blood.V99.9.3376
59. Noppe SM, Heirman C, Bakkus MH, Brissinck J, Schots R, Thielemans K. The genetic variability of the VH genes in follicular lymphoma: the impact of the hypermutation mechanism. Br J Haematol. (1999) 107:625–40. doi: 10.1046/j.1365-2141.1999.01732.x
60. Aarts WM, Bende RJ, Steenbergen EJ, Kluin PM, Ooms ECM, Pals ST, et al. Variable heavy chain gene analysis of follicular lymphomas: correlation between heavy chain isotype expression and somatic mutation load. Blood. (2000) 95:2922–9. doi: 10.1182/blood.V95.9.2922.009k38_2922_2929
61. Kosmas C, Stamatopoulos K, Papadaki T, Belessi C, Yataganas X, Anagnostou D, et al. Somatic hypermutation of immunoglobulin variable region genes: focus on follicular lymphoma and multiple myeloma. Immunol Rev. (1998) 162:281–92. doi: 10.1111/j.1600-065X.1998.tb01448.x
62. Ottensmeier CH, Thompsett AR, Zhu DL, Wilkins BS, Sweetenham JW, Stevenson FK. Analysis of V-H genes in follicular and diffuse lymphoma shows ongoing somatic mutation and multiple isotype transcripts in early disease with changes during disease progression. Blood. (1998) 91:4292–9. doi: 10.1182/blood.V91.11.4292
63. Stamatopoulos K, Kosmas C, Papadaki T, Pouliou E, Belessi C, Afendaki S, et al. Follicular lymphoma immunoglobulin kappa light chains are affected by the antigen selection process, but to a lesser degree than their partner heavy chains. Br J Haematol. (1997) 96:132–46. doi: 10.1046/j.1365-2141.1997.8492477.x
64. Zhu DL, McCarthy H, Ottensmeier CH, Johnson P, Hamblin TJ, Stevenson FK. Acquisition of potential N-glycosylation sites in the immunoglobulin variable region by somatic mutation is a distinctive feature of follicular lymphoma. Blood. (2002) 99:2562–8. doi: 10.1182/blood.V99.7.2562
65. Dunn-Walters D, Boursier L, Spencer J. Effect of somatic hypermutation on potential N-glycosylation sites in human immunoglobulin heavy chain variable regions. Mol Immunol. (2000) 37:107–13. doi: 10.1016/S0161-5890(00)00038-9
66. Zabalegui N, de Cerio ALD, Inoges S, Rodriguez-Calvillo M, Perez-Calvo J, Hernandez M, et al. Acquired potential N-glycosylation sites within the tumor-specific immunoglobulin heavy chains of B-cell malignancies. Haematologica. (2004) 89:541–6.
67. Zhu D, Ottensmeier CH, Du MQ, McCarthy H, Stevenson FK. Incidence of potential glycosylation sites in immunoglobulin variable regions distinguishes between subsets of Burkitt's lymphoma and mucosa-associated lymphoid tissue lymphoma. Br J Haematol. (2003) 120:217–22. doi: 10.1046/j.1365-2141.2003.04064.x
68. Radcliffe CM, Arnold JN, Suter DM, Wormald MR, Harvey DJ, Royle L, et al. Human follicular lymphoma cells contain oligomannose glycans in the antigen-binding site of the B-cell receptor. J Biol Chem. (2007) 282:7405–15. doi: 10.1074/jbc.M602690200
69. Coelho V, Krysov S, Ghaemmaghami AM, Emara M, Potter KN, Johnson P, et al. Glycosylation of surface Ig creates a functional bridge between human follicular lymphoma and microenvironmental lectins. Proc Natl Acad Sci USA. (2010) 107:18587–92. doi: 10.1073/pnas.1009388107
70. Linley A, Krysov S, Ponzoni M, Johnson PW, Packham G, Stevenson FK. Lectin binding to surface Ig variable regions provides a universal persistent activating signal for follicular lymphoma cells. Blood. (2015) 126:1902–10. doi: 10.1182/blood-2015-04-640805
71. Amin R, Mourcin F, Uhel F, Pangault C, Ruminy P, Dupre L, et al. DC-SIGN-expressing macrophages trigger activation of mannosylated IgM B-cell receptor in follicular lymphoma. Blood. (2015) 126:1911–20. doi: 10.1182/blood-2015-04-640912
72. Cha SC, Qin H, Kannan S, Rawal S, Watkins LS, Baio FE, et al. Nonstereotyped Lymphoma B Cell Receptors Recognize Vimentin as a Shared Autoantigen. J Immunol. (2013) 190:4887–98. doi: 10.4049/jimmunol.1300179
73. Chiorazzi N, Rai KR, Ferrarini M. Chronic lymphocytic leukemia. N Engl J Med. (2005) 352:804–15. doi: 10.1056/NEJMra041720
74. Fischer K, Hallek M. Optimizing frontline therapy of CLL based on clinical and biological factors. Hematol Am Soc Hematol Educ Program. (2017) 2017:338–45. doi: 10.1182/asheducation-2017.1.338
75. Hallek M. Chronic lymphocytic leukemia: 2015 Update on diagnosis, risk stratification, and treatment. Am J Hematol. (2015) 90:446–60. doi: 10.1002/ajh.23979
76. Efremov DG, Ivanovski M, Siljanovski N, Pozzato G, Cevreska L, Fais F, et al. Restricted immunoglobulin VH region repertoire in chronic lymphocytic leukemia patients with autoimmune hemolytic anemia. Blood. (1996) 87:3869–76. doi: 10.1182/blood.V87.9.3869.bloodjournal8793869
77. Hashimoto S, Dono M, Wakai M, Allen SL, Lichtman SM, Schulman P, et al. Somatic diversification and selection of immunoglobulin heavy and light chain variable region genes in IgG+ CD5+ chronic lymphocytic leukemia B cells. J Exp Med. (1995) 181:1507–17. doi: 10.1084/jem.181.4.1507
78. Fais F, Ghiotto F, Hashimoto S, Sellars B, Valetto A, Allen SL, et al. Chronic lymphocytic leukemia B cells express restricted sets of mutated and unmutated antigen receptors. J Clin Investig. (1998) 102:1515–25. doi: 10.1172/JCI3009
79. Johnson TA, Rassenti LZ, Kipps TJ. Ig VH1 genes expressed in B cell chronic lymphocytic leukemia exhibit distinctive molecular features. J Immunol. (1997) 158:235–46.
80. Tobin G, Thunberg U, Johnson A, Eriksson I, Soderberg O, Karlsson K, et al. Chronic lymphocytic leukemias utilizing the VH3–21 gene display highly restricted Vlambda2–14 gene use and homologous CDR3s: implicating recognition of a common antigen epitope. Blood. (2003) 101:4952–7. doi: 10.1182/blood-2002-11-3485
81. Bomben R, Dal Bo M, Capello D, Benedetti D, Marconi D, Zucchetto A, et al. Comprehensive characterization of IGHV3-21-expressing B-cell chronic lymphocytic leukemia: an Italian multicenter study. Blood. (2007) 109:2989–98. doi: 10.1182/blood-2006-10-051110
82. Murray F, Darzentas N, Hadzidimitriou A, Tobin G, Boudjogra M, Scielzo C, et al. Stereotyped patterns of somatic hypermutation in subsets of patients with chronic lymphocytic leukemia: implications for the role of antigen selection in leukemogenesis. Blood. (2008) 111:1524–33. doi: 10.1182/blood-2007-07-099564
83. Stamatopoulos K, Belessi C, Moreno C, Boudjograh M, Guida G, Smilevska T, et al. Over 20% of patients with chronic lymphocytic leukemia carry stereotyped receptors: Pathogenetic implications and clinical correlations. Blood. (2007) 109:259–70. doi: 10.1182/blood-2006-03-012948
84. Messmer BT, Albesiano E, Efremov DG, Ghiotto F, Allen SL, Kolitz J, et al. Multiple distinct sets of stereotyped antigen receptors indicate a role for antigen in promoting chronic lymphocytic leukemia. J Exp Med. (2004) 200:519–25. doi: 10.1084/jem.20040544
85. Tobin G, Thunberg U, Karlsson K, Murray F, Laurell A, Willander K, et al. Subsets with restricted immunoglobulin gene rearrangement features indicate a role for antigen selection in the development of chronic lymphocytic leukemia. Blood. (2004) 104:2879–85. doi: 10.1182/blood-2004-01-0132
86. Widhopf GF 2nd, Rassenti LZ, Toy TL, Gribben JG, Wierda WG, Kipps TJ. Chronic lymphocytic leukemia B cells of more than 1% of patients express virtually identical immunoglobulins. Blood. (2004) 104:2499–504. doi: 10.1182/blood-2004-03-0818
87. Stamatopoulos K, Belessi C, Hadzidimitriou A, Smilevska T, Kalagiakou E, Hatzi K, et al. Immunoglobulin light chain repertoire in chronic lymphocytic leukemia. Blood. (2005) 106:3575–83. doi: 10.1182/blood-2005-04-1511
88. Belessi CJ, Davi FB, Stamatopoulos KE, Degano M, Andreou TM, Moreno C, et al. IGHV gene insertions and deletions in chronic lymphocytic leukemia: “CLL-biased” deletions in a subset of cases with stereotyped receptors. Eur J Immunol. (2006) 36:1963–74. doi: 10.1002/eji.200535751
89. Agathangelidis A, Darzentas N, Hadzidimitriou A, Brochet X, Murray F, Yan XJ, et al. Stereotyped B-cell receptors in one-third of chronic lymphocytic leukemia: a molecular classification with implications for targeted therapies. Blood. (2012) 119:4467–75. doi: 10.1182/blood-2011-11-393694
90. Darzentas N, Hadzidimitriou A, Murray F, Hatzi K, Josefsson P, Laoutaris N, et al. A different ontogenesis for chronic lymphocytic leukemia cases carrying stereotyped antigen receptors: molecular and computational evidence. Leukemia. (2010) 24:125–32. doi: 10.1038/leu.2009.186
91. Kostareli E, Gounari M, Janus A, Murray F, Brochet X, Giudicelli V, et al. Antigen receptor stereotypy across B-cell lymphoproliferations: the case of IGHV4-59/IGKV3-20 receptors with rheumatoid factor activity. Leukemia. (2012) 26:1127–31. doi: 10.1038/leu.2011.311
92. Chiorazzi N, Ferrarini M. Cellular origin(s) of chronic lymphocytic leukemia: cautionary notes and additional considerations and possibilities. Blood. (2011) 117:1781–91. doi: 10.1182/blood-2010-07-155663
93. Catera R, Silverman GJ, Hatzi K, Seiler T, Didier S, Zhang L, et al. Chronic lymphocytic leukemia cells recognize conserved epitopes associated with apoptosis and oxidation. Mol Med. (2008) 14:665–74. doi: 10.2119/2008-00102.Catera
94. Chu CC, Catera R, Hatzi K, Yan XJ, Zhang L, Wang XB, et al. Chronic lymphocytic leukemia antibodies with a common stereotypic rearrangement recognize nonmuscle myosin heavy chain IIA. Blood. (2008) 112:5122–9. doi: 10.1182/blood-2008-06-162024
95. Chu CC, Catera R, Zhang L, Didier S, Agagnina BM, Damle RN, et al. Many chronic lymphocytic leukemia antibodies recognize apoptotic cells with exposed nonmuscle myosin heavy chain IIA: implications for patient outcome and cell of origin. Blood. (2010) 115:3907–15. doi: 10.1182/blood-2009-09-244251
96. Herve M, Xu K, Ng YS, Wardemann H, Albesiano E, Messmer BT, et al. Unmutated and mutated chronic lymphocytic leukemias derive from self-reactive B cell precursors despite expressing different antibody reactivity. J Clin Investig. (2005) 115:1636–43. doi: 10.1172/JCI24387
97. Lanemo Myhrinder A, Hellqvist E, Sidorova E, Soderberg A, Baxendale H, Dahle C, et al. A new perspective: molecular motifs on oxidized LDL, apoptotic cells, and bacteria are targets for chronic lymphocytic leukemia antibodies. Blood. (2008) 111:3838–48. doi: 10.1182/blood-2007-11-125450
98. Seiler T, Woelfle M, Yancopoulos S, Catera R, Li W, Hatzi K, et al. Characterization of structurally defined epitopes recognized by monoclonal antibodies produced by chronic lymphocytic leukemia B cells. Blood. (2009) 114:3615–24. doi: 10.1182/blood-2009-01-197822
99. Zwick C, Fadle N, Regitz E, Kemele M, Stilgenbauer S, Buhler A, et al. Autoantigenic targets of B-cell receptors derived from chronic lymphocytic leukemias bind to and induce proliferation of leukemic cells. Blood. (2013) 121:4708–17. doi: 10.1182/blood-2012-08-447904
100. ten Hacken E, Gounari M, Back JW, Shimanovskaya E, Scarfo L, Kim E, et al. Calreticulin as a novel B-cell receptor antigen in chronic lymphocytic leukemia. Haematologica. (2017) 102:e394–6. doi: 10.3324/haematol.2017.169102
101. Hardy RR. B-1 B cell development. J Immunol. (2006) 177:2749–54. doi: 10.4049/jimmunol.177.5.2749
102. Dorshkind K, Montecino-Rodriguez E. Fetal B-cell lymphopoiesis and the emergence of B-1-cell potential. Nat Rev Immunol. (2007) 7:213–9. doi: 10.1038/nri2019
103. McCarthy H, Wierda WG, Barron LL, Cromwell CC, Wang J, Coombes KR, et al. High expression of activation-induced cytidine deaminase (AID) and splice variants is a distinctive feature of poor-prognosis chronic lymphocytic leukemia. Blood. (2003) 101:4903–8. doi: 10.1182/blood-2002-09-2906
104. Oppezzo P, Vuillier F, Vasconcelos Y, Dumas G, Magnac C, Payelle-Brogard B, et al. Chronic lymphocytic leukemia B cells expressing AID display dissociation between class switch recombination and somatic hypermutation. Blood. (2003) 101:4029–32. doi: 10.1182/blood-2002-10-3175
105. Patten PE, Chu CC, Albesiano E, Damle RN, Yan XJ, Kim D, et al. IGHV-unmutated and IGHV-mutated chronic lymphocytic leukemia cells produce activation-induced deaminase protein with a full range of biologic functions. Blood. (2012) 120:4802–11. doi: 10.1182/blood-2012-08-449744
106. Lanham S, Hamblin T, Oscier D, Ibbotson R, Stevenson F, Packham G. Differential signaling via surface IgM is associated with VH gene mutational status and CD38 expression in chronic lymphocytic leukemia. Blood. (2003) 101:1087–93. doi: 10.1182/blood-2002-06-1822
107. Zupo S, Isnardi L, Megna M, Massara R, Malavasi F, Dono M, et al. CD38 expression distinguishes two groups of B-cell chronic lymphocytic leukemias with different responses to anti-IgM antibodies and propensity to apoptosis. Blood. (1996) 88:1365–74. doi: 10.1182/blood.V88.4.1365.bloodjournal8841365
108. Ntoufa S, Vardi A, Papakonstantinou N, Anagnostopoulos A, Aleporou-Marinou V, Belessi C, et al. Distinct innate immunity pathways to activation and tolerance in subgroups of chronic lymphocytic leukemia with distinct immunoglobulin receptors. Mol Med. (2012) 18:1281–91. doi: 10.2119/molmed.2011.00480
109. Chatzouli M, Ntoufa S, Papakonstantinou N, Chartomatsidou E, Anagnostopoulos A, Kollia P, et al. Heterogeneous functional effects of concomitant B cell receptor and TLR stimulation in chronic lymphocytic leukemia with mutated versus unmutated Ig genes. J Immunol. (2014) 192:4518–24. doi: 10.4049/jimmunol.1302102
110. Apollonio B, Scielzo C, Bertilaccio MT, Ten E, Scarfo L, Ranghetti P, et al. Targeting B-cell anergy in chronic lymphocytic leukemia. Blood. (2013) 121:3879–88, S1–8. doi: 10.1182/blood-2012-12-474718
111. Mockridge CI, Potter KN, Wheatley I, Neville LA, Packham G, Stevenson FK. Reversible anergy of sIgM-mediated signaling in the two subsets of CLL defined by VH-gene mutational status. Blood. (2007) 109:4424–31. doi: 10.1182/blood-2006-11-056648
112. Muzio M, Apollonio B, Scielzo C, Frenquelli M, Vandoni I, Boussiotis V, et al. Constitutive activation of distinct BCR-signaling pathways in a subset of CLL patients: a molecular signature of anergy. Blood. (2008) 112:188–95. doi: 10.1182/blood-2007-09-111344
113. Duhren-von Minden M, Ubelhart R, Schneider D, Wossning T, Bach MP, Buchner M, et al. Chronic lymphocytic leukaemia is driven by antigen-independent cell-autonomous signalling. Nature. (2012) 489:309–12. doi: 10.1038/nature11309
114. Jimenez de Oya N, De Giovanni M, Fioravanti J, Ubelhart R, Di Lucia P, Fiocchi A, et al. Pathogen-specific B-cell receptors drive chronic lymphocytic leukemia by light-chain-dependent cross-reaction with autoantigens. EMBO Mol Med. (2017) 9:1482–90. doi: 10.15252/emmm.201707732
115. Minici C, Gounari M, Ubelhart R, Scarfo L, Duhren-von Minden M, Schneider D, et al. Distinct homotypic B-cell receptor interactions shape the outcome of chronic lymphocytic leukaemia. Nat Commun. (2017) 8:15746. doi: 10.1038/ncomms15746
116. Damle RN, Wasil T, Fais F, Ghiotto F, Valetto A, Allen SL, et al. Ig V gene mutation status and CD38 expression as novel prognostic indicators in chronic lymphocytic leukemia. Blood. (1999) 94:1840–7. doi: 10.1182/blood.V94.6.1840
117. Hamblin TJ, Davis Z, Gardiner A, Oscier DG, Stevenson FK. Unmutated Ig V(H) genes are associated with a more aggressive form of chronic lymphocytic leukemia. Blood. (1999) 94:1848–54. doi: 10.1182/blood.V94.6.1848
118. Sutton LA, Hadzidimitriou A, Baliakas P, Agathangelidis A, Langerak AW, Stilgenbauer S, et al. Immunoglobulin genes in chronic lymphocytic leukemia: key to understanding the disease and improving risk stratification. Haematologica. (2017) 102:968–71. doi: 10.3324/haematol.2017.165605
119. Rodriguez-Vicente AE, Diaz MG, Hernandez-Rivas JM. Chronic lymphocytic leukemia: a clinical and molecular heterogenous disease. Cancer Genet. (2013) 206:49–62. doi: 10.1016/j.cancergen.2013.01.003
120. Baliakas P, Hadzidimitriou A, Sutton LA, Minga E, Agathangelidis A, Nichelatti M, et al. Clinical effect of stereotyped B-cell receptor immunoglobulins in chronic lymphocytic leukaemia: a retrospective multicentre study. Lancet Haematol. (2014) 1:e74–84. doi: 10.1016/S2352-3026(14)00005-2
121. Athanasiadou A, Stamatopoulos K, Gaitatzi M, Stavroyianni N, Fassas A, Anagnostopoulos A. Recurrent cytogenetic findings in subsets of patients with chronic lymphocytic leukemia expressing IgG-switched stereotyped immunoglobulins. Haematologica. (2008) 93:473–4. doi: 10.3324/haematol.11872
122. Rossi D, Spina V, Bomben R, Rasi S, Dal-Bo M, Bruscaggin A, et al. Association between molecular lesions and specific B-cell receptor subsets in chronic lymphocytic leukemia. Blood. (2013) 121:4902–5. doi: 10.1182/blood-2013-02-486209
123. Strefford JC, Sutton LA, Baliakas P, Agathangelidis A, Malcikova J, Plevova K, et al. Distinct patterns of novel gene mutations in poor-prognostic stereotyped subsets of chronic lymphocytic leukemia: the case of SF3B1 and subset #2. Leukemia. (2013) 27:2196–9. doi: 10.1038/leu.2013.98
124. Rossi D, Spina V, Cerri M, Rasi S, Deambrogi C, De Paoli L, et al. Stereotyped B-cell receptor is an independent risk factor of chronic lymphocytic leukemia transformation to Richter syndrome. Clin Cancer Res. (2009) 15:4415–22. doi: 10.1158/1078-0432.CCR-08-3266
125. Agathangelidis A, Xochelli A, Stamatopoulos K. A gene is known by the company it keeps: enrichment of TNFAIP3 gene aberrations in MALT lymphomas expressing IGHV4-34 antigen receptors. J Pathol. (2017) 243:403–6. doi: 10.1002/path.4982
126. Moody S, Escudero-Ibarz L, Wang M, Clipson A, Ochoa Ruiz E, Dunn-Walters D, et al. Significant association between TNFAIP3 inactivation and biased immunoglobulin heavy chain variable region 4-34 usage in mucosa-associated lymphoid tissue lymphoma. J Pathol. (2017) 243:3–8. doi: 10.1002/path.4933
127. Parry M, Rose-Zerilli MJ, Ljungstrom V, Gibson J, Wang J, Walewska R, et al. Genetics and prognostication in splenic marginal zone lymphoma: revelations from deep sequencing. Clin Cancer Res. (2015) 21:4174–83. doi: 10.1158/1078-0432.CCR-14-2759
128. Bikos V, Darzentas N, Hadzidimitriou A, Davis Z, Hockley S, Traverse-Glehen A, et al. Over 30% of patients with splenic marginal zone lymphoma express the same immunoglobulin heavy variable gene: ontogenetic implications. Leukemia. (2012) 26:1638–46. doi: 10.1038/leu.2012.3
129. Clipson A, Wang M, de Leval L, Ashton-Key M, Wotherspoon A, Vassiliou G, et al. KLF2 mutation is the most frequent somatic change in splenic marginal zone lymphoma and identifies a subset with distinct genotype. Leukemia. (2015) 29:1177–85. doi: 10.1038/leu.2014.330
130. Rossi D, Rasi S, Spina V, Bruscaggin A, Monti S, Ciardullo C, et al. Integrated mutational and cytogenetic analysis identifies new prognostic subgroups in chronic lymphocytic leukemia. Blood. (2013) 121:1403–12. doi: 10.1182/blood-2012-09-458265
131. Baliakas P, Hadzidimitriou A, Sutton LA, Rossi D, Minga E, Villamor N, et al. Recurrent mutations refine prognosis in chronic lymphocytic leukemia. Leukemia. (2015) 29:329–36. doi: 10.1038/leu.2014.196
132. Baliakas P, Moysiadis T, Hadzidimitriou A, Xochelli A, Jeromin S, Agathangelidis A, et al. Tailored approaches grounded on immunogenetic features for refined prognostication in chronic lymphocytic leukemia. Haematologica. (2018) 104:360–69. doi: 10.3324/haematol.2018.195032
133. Byrd JC, Furman RR, Coutre SE, Burger JA, Blum KA, Coleman M, et al. Three-year follow-up of treatment-naive and previously treated patients with CLL and SLL receiving single-agent ibrutinib. Blood. (2015) 125:2497–506. doi: 10.1182/blood-2014-10-606038
134. Jones JA, Robak T, Brown JR, Awan FT, Badoux X, Coutre S, et al. Efficacy and safety of idelalisib in combination with ofatumumab for previously treated chronic lymphocytic leukaemia: an open-label, randomised phase 3 trial. Lancet Haematol. (2017) 4:e114–26. doi: 10.1016/S2352-3026(17)30019-4
135. Chai-Adisaksopha C, Brown JR. FCR achieves long-term durable remissions in patients with IGHV-mutated CLL. Blood. (2017) 130:2278–82. doi: 10.1182/blood-2017-07-731588
136. Hallek M, Cheson BD, Catovsky D, Caligaris-Cappio F, Dighiero G, Dohner H, et al. iwCLL guidelines for diagnosis, indications for treatment, response assessment, and supportive management of CLL. Blood. (2018) 131:2745–60. doi: 10.1182/blood-2017-09-806398
137. Langerak AW, Davi F, Ghia P, Hadzidimitriou A, Murray F, Potter KN, et al. Immunoglobulin sequence analysis and prognostication in CLL: guidelines from the ERIC review board for reliable interpretation of problematic cases. Leukemia. (2011) 25:979–84. doi: 10.1038/leu.2011.49
138. Gounari M, Ntoufa S, Apollonio B, Papakonstantinou N, Ponzoni M, Chu CC, et al. Excessive antigen reactivity may underlie the clinical aggressiveness of chronic lymphocytic leukemia stereotyped subset #8. Blood. (2015) 125:3580–7. doi: 10.1182/blood-2014-09-603217
139. Kanduri M, Marincevic M, Halldorsdottir AM, Mansouri L, Junevik K, Ntoufa S, et al. Distinct transcriptional control in major immunogenetic subsets of chronic lymphocytic leukemia exhibiting subset-biased global DNA methylation profiles. Epigenetics. (2012) 7:1435–42. doi: 10.4161/epi.22901
140. Papakonstantinou N, Ntoufa S, Chartomatsidou E, Papadopoulos G, Hatzigeorgiou A, Anagnostopoulos A, et al. Differential microRNA profiles and their functional implications in different immunogenetic subsets of chronic lymphocytic leukemia. Mol Med. (2013) 19:115–23. doi: 10.2119/molmed.2013.00005
141. Papakonstantinou N, Ntoufa S, Tsagiopoulou M, Moysiadis T, Bhoi S, Malousi A, et al. Integrated epigenomic and transcriptomic analysis reveals TP63 as a novel player in clinically aggressive chronic lymphocytic leukemia. Int J Cancer. (2018) 144:2695–706. doi: 10.1002/ijc.31999
142. Agathangelidis A, Hadzidimitriou A, Rosenquist R, Stamatopoulos K. Unlocking the secrets of immunoglobulin receptors in mantle cell lymphoma: implications for the origin and selection of the malignant cells. Semin Cancer Biol. (2011) 21:299–307. doi: 10.1016/j.semcancer.2011.09.009
143. Perez-Galan P, Dreyling M, Wiestner A. Mantle cell lymphoma: biology, pathogenesis, and the molecular basis of treatment in the genomic era. Blood. (2011) 117:26–38. doi: 10.1182/blood-2010-04-189977
144. Royo C, Navarro A, Clot G, Salaverria I, Gine E, Jares P, et al. Non-nodal type of mantle cell lymphoma is a specific biological and clinical subgroup of the disease. Leukemia. (2012) 26:1895–8. doi: 10.1038/leu.2012.72
145. Thorselius M, Walsh S, Eriksson I, Thunberg U, Johnson A, Backlin C, et al. Somatic hypermutation and V(H) gene usage in mantle cell lymphoma. Eur J Haematol. (2002) 68:217–24. doi: 10.1034/j.1600-0609.2002.01662.x
146. Walsh SH, Thorselius M, Johnson A, Soderberg O, Jerkeman M, Bjorck E, et al. Mutated VH genes and preferential VH3-21 use define new subsets of mantle cell lymphoma. Blood. (2003) 101:4047–54. doi: 10.1182/blood-2002-11-3479
147. Camacho FI, Algara P, Rodriguez A, Ruiz-Ballesteros E, Mollejo M, Martinez N, et al. Molecular heterogeneity in MCL defined by the use of specific VH genes and the frequency of somatic mutations. Blood. (2003) 101:4042–6. doi: 10.1182/blood-2002-11-3456
148. Kienle D, Krober A, Katzenberger T, Ott G, Leupolt E, Barth TF, et al. VH mutation status and VDJ rearrangement structure in mantle cell lymphoma: correlation with genomic aberrations, clinical characteristics, and outcome. Blood. (2003) 102:3003–9. doi: 10.1182/blood-2003-05-1383
149. Orchard J, Garand R, Davis Z, Babbage G, Sahota S, Matutes E, et al. A subset of t(11;14) lymphoma with mantle cell features displays mutated IgVH genes and includes patients with good prognosis, nonnodal disease. Blood. (2003) 101:4975–81. doi: 10.1182/blood-2002-06-1864
150. Schraders M, Oeschger S, Kluin PM, Hebeda K, Schuuring E, Groenen PJ, et al. Hypermutation in mantle cell lymphoma does not indicate a clinical or biological subentity. Mod Pathol. (2009) 22:416–25. doi: 10.1038/modpathol.2008.199
151. Hadzidimitriou A, Agathangelidis A, Darzentas N, Murray F, Delfau-Larue MH, Pedersen LB, et al. Is there a role for antigen selection in mantle cell lymphoma? Immunogenetic support from a series of 807 cases. Blood. (2011) 118:3088–95. doi: 10.1182/blood-2011-03-343434
152. Navarro A, Clot G, Royo C, Jares P, Hadzidimitriou A, Agathangelidis A, et al. Molecular subsets of mantle cell lymphoma defined by the IGHV mutational status and SOX11 expression have distinct biologic and clinical features. Cancer Res. (2012) 72:5307–16. doi: 10.1158/0008-5472.CAN-12-1615
153. Xochelli A, Sutton LA, Agathangelidis A, Stalika E, Karypidou M, Marantidou F, et al. Molecular evidence for antigen drive in the natural history of mantle cell lymphoma. Am J Pathol. (2015) 185:1740–8. doi: 10.1016/j.ajpath.2015.02.006
154. Klapper W, Szczepanowski M, Heidorn K, Muschen M, Liedtke S, Sotnikova A, et al. Immunoglobulin class-switch recombination occurs in mantle cell lymphomas. J Pathol. (2006) 209:250–7. doi: 10.1002/path.1961
155. Wang ML, Rule S, Martin P, Goy A, Auer R, Kahl BS, et al. Targeting BTK with ibrutinib in relapsed or refractory mantle-cell lymphoma. N Engl J Med. (2013) 369:507–16. doi: 10.1056/NEJMoa1306220
156. Pouliou E, Xochelli A, Kanellis G, Stalika E, Sutton LA, Navarro A, et al. Numerous ontogenetic roads to mantle cell lymphoma: immunogenetic and immunohistochemical evidence. Am J Pathol. (2017) 187:1454–8. doi: 10.1016/j.ajpath.2017.02.017
Keywords: chronic lymphocytic leukemia (CLL), mantle cell lymphoma (MCL), germinal center (GC), immunogenetics, ontogeny, patient prognosis
Citation: Gemenetzi K, Agathangelidis A, Zaragoza-Infante L, Sofou E, Papaioannou M, Chatzidimitriou A and Stamatopoulos K (2020) B Cell Receptor Immunogenetics in B Cell Lymphomas: Immunoglobulin Genes as Key to Ontogeny and Clinical Decision Making. Front. Oncol. 10:67. doi: 10.3389/fonc.2020.00067
Received: 13 October 2019; Accepted: 15 January 2020;
Published: 31 January 2020.
Edited by:
Basem M. William, The Ohio State University, United StatesReviewed by:
Davide Rossi, Istituto Oncologico Della Svizzera Italiana, SwitzerlandPier Paolo Piccaluga, University of Bologna, Italy
Copyright © 2020 Gemenetzi, Agathangelidis, Zaragoza-Infante, Sofou, Papaioannou, Chatzidimitriou and Stamatopoulos. This is an open-access article distributed under the terms of the Creative Commons Attribution License (CC BY). The use, distribution or reproduction in other forums is permitted, provided the original author(s) and the copyright owner(s) are credited and that the original publication in this journal is cited, in accordance with accepted academic practice. No use, distribution or reproduction is permitted which does not comply with these terms.
*Correspondence: Anastasia Chatzidimitriou, YWNoYXR6aWRpbWl0cmlvdUBjZXJ0aC5ncg==