- 1Department of Surgical Oncology, The First Hospital of China Medical University, Shenyang, China
- 2Department of Breast Surgery, Shengjing Hospital of China Medical University, Shenyang, China
- 3Department of Otorhinolaryngology, The First Hospital of China Medical University, Shenyang, China
Bcl2-associated athanogene (BAG)2 as a co-chaperone has been demonstrated to be involved in tumor growth and metastasis, but its biological function in gastric cancer remains unknown. Here, we reported that BAG2 was highly expressed in gastric cancer cell lines and tissues, indicating poor prognosis. High expression of BAG2 was significantly associated with T stage and differentiation level of gastric cancer (P < 0.001). Functional experiments revealed that BAG2 knockdown in gastric cancer cells inhibited the proliferation, invasion and migration of cells through AKT/mTOR and extracellular regulated kinase (ERK) pathways. Proteomic analysis identified that BAG2 may be involved in the regulation of mitogen-activated protein kinase (MAPK) pathway. In addition, immunoprecipitation showed that BAG2 could bind to ERK1/2. Luciferase reporter assay and Western blot verified that BAG2 was down-regulated by miR186. Taken together, our findings may reveal the basic function of BAG2 and uncover a potential therapeutic target for gastric cancer.
Introduction
Gastric cancer is a highly aggressive malignancy that is currently the third cause of cancer death (1). Although the overall survival (OS) rate of gastric cancer has improved in recent years, there are still many patients with recurrence and metastasis (2). To improve the prognosis of gastric cancer patients, it is important to identify predictive biomarkers and potential therapeutic targets to develop more effective treatment strategies. BAG2 (Bcl-2-associated athanogene 2) is a protein identified by two-hybrid with heat shock protein 70 (Hsp70) as bait (3, 4), which contains at least three domains, BAG domain, BNB (Brand New Bag) domain, and amino terminal domain (5). Functioning as a co-chaperone, BAG2 interacts with the carboxyterminal region of Hsp70/C-terminal interacting protein, and then regulates the biological activity of molecular chaperones, including ubiquitin proteasome system (3, 6–8).
The roles of BAG2 in cancer are not well-studied. It has been reported that high expression of BAG2 can induce p21-dependent aging and subsequent carcinogenic stagnation (9). It also has been shown that BAG2 can promote apoptosis in thyroid cancer in response to proteasome inhibitors (10). However, in recent years, further studies have shown that BAG2 plays a pivotal role as an oncogene. BAG2 is reported as highly expressed in tumors, including colorectal cancer, breast cancer, and head and neck squamous cell carcinoma (11). BAG2 was also highly expressed in triple negative breast cancer and associated with cancer metastasis (12). Currently, the role of BAG2 in gastric cancer remains elusive.
Materials and Methods
Tissue Samples and Cell Culture
Gastric tissue samples were obtained from the patients in the First Hospital of China Medical University. Human gastric cancer cell line MKN45 were accessed from American Type Culture Collection (ATCC, USA). GES-1, MGC803, SGC7901, and HGC27 cell lines were purchased from the Cell Bank of typical Culture Preservation Committee of Chinese Academy of Sciences (China). Except that MGC803 cells were cultured in high glucose DMEM medium containing 10% FBS (Sciencell), the other cell lines were cultured in RPMI 1640 medium supplemented with 10% FBS (Sciencell) in a humidified atmosphere of 5% CO2 at 37°C.
BAG2-Specific siRNAs and miR186 Mimics Transfection
BAG2-specific siRNAs and miR186 mimics were transfected into the HGC27 cell line using X-tremeGENE siRNA Transfection Reagent (Roche). Briefly, HGC27 cells were cultured in 6-well plates overnight and when the cells reached 70% of confluency, the cells were transfected with miR186 mimics, BAG2-specific siRNAs (1# siBAG2, 5′-GGGAAGAACUCUCACCGUUTT-3′; 2# siBAG2, 5′-GGGAAAUGCCAAGAGUCAUTT-3′; 3# siBAG2, 5′-GCUGAAAGCAGAUUCAAUUTT-3′) or control siRNA (5′-UUCUCCGAACGUGUCACGUTT -3′).
BAG2-shRNAs Transfection
HGC27 cells (5 × 105 cells/well) were cultured overnight and transduced with lentivirus for expressing control shRNA (5′-TTCTCCGAACGTGTCACGT-3′) or BAG2-specific shRNA (5′-GATCAGAAGTTTCAATCCATA-3′). The cells were treated with 5 μg/ml of puromycin to generate stably BAG2 knockdown HGC27/shBAG2 cells or negative control HGC27/NC cells. The efficacy of BAG2 knockdown was verified by Western blot.
Western Blot
The different groups of cells were lyzed in RIPA lysis buffer containing PMSF, Protease/Phosphatase Inhibitor Cocktail which came from Cell Signaling Technology (CST, 5872). After being centrifuged, the concentrations of total proteins were determined. Individual cell lysate samples (20 μg/lane) were separated by SDS-PAGE on 6-12% gels and transferred onto polyvinylidene difluoride (PVDF) membranes (Millipore). The membranes were blocked with 5% BSA in TBST and incubated overnight at 4°C with primary antibodies. The antibodies included human BAG2 (Thermofisher, PA5-30922), AKT (4691), mTOR (2972), ERK1/2 (4695), p38 (9212), and GAPDH (5174) from CST; E-cadherin(ab15148), N-cadherin (ab18203), MMP9 (ab38898), and Snail (ab180714) from Abcam; Vimentin (wl00742) from Wanleibio (China). The bound antibodies were detected with horseradish peroxidase (HRP)-conjugated secondary antibodies (at 1:10000 dilution, CST, 7074). The immunoblotting signals were visualized using the enhanced chemiluminescent reagents. The relative levels of individual target proteins to the control GAPDH were determined by the densitometric analysis using the ImageJ software.
Immunohistochemistry (IHC)
Paraffin-embedded tissue sections from gastric cancer patients were used for immunohistochemistry of BAG2. After dewaxing and hydration, sections were incubated with 3% H2O2 to block endogenous enzymes before incubation with primary antibody of BAG2 (Thermofisher, PA5-72897, dilution of 1:1000) at 4°C overnight. the sections were incubated with the secondary antibody (MaxVision HRP-polymer anti-mouse/rabbit IHC Kit, 5002, Maixin). The immunostaining was visualized using diaminobenzidine. At each step, sections were rinsed several times with PBS. BAG2 expression was classified semi-quantitatively as follows: 0, no staining; 1, partial staining; 2, mild to moderate circumferential staining; and 3, strong staining. A score of 0 or 1 was considered low expression of BAG2. A score of 2 or 3 was considered high expression of BAG2.
Cell Counting Kit 8 (CCK8) Assay
The cell viability was determined by CCK8 assay (Dojindo, Japan). Cells were transfected with BAG2-siRNA or negative control siRNA in 6-well plates for 24 h. Then these cells were transferred into 96-well plates and incubated for different time periods. Then, new medium with 10% CCK8 solution was added into each well and incubated for 2 h. The cell viability was analyzed at 450 nm.
Transwell Assay
The impact of BAG2 knockdown on the invasion and migration of gastric cancer cells was determined by transwell assay. For invasion assay, membranes of the top chambers were coated with Matrigel (BD) and pre-hydrated in serum-free medium (13). Briefly, control or HGC27/siBAG2 cells (2 × 104 cells/well) were loaded on the upper chamber of 24-well transwell plates (8-μm pore size, Corning) in FBS-free medium. The bottom chambers were filled with complete medium. After 24h, cells on the upper surface of the membrane were removed using cotton swabs and migrated cells on the bottom surface were stained with 0.1% crystal violet. The numbers of migrated cells in five randomly selected fields were counted under a phase contrast microscope in a blinded manner.
EdU Cell Proliferation Assay
Cell proliferation was determined using the kFluor555-EdU cell proliferation kit (Keygentec, China). Cells were cultured in 6-well plates at 2 × 104 cells/well overnight and incubated with EdU (10 μM) for 2 h. Followed washing cells with PBS, cells were incubated with 4% paraformaldehyde for fixation. Then 2 mg/mL glycine was added for 5 min, washed with 3% BSA in PBS, then added 0.5% tritonX-100 for 20 min. Click-iT reaction mixture were prepared following the manufacturer's protocol. Samples were observed with a fluorescence microscope immediately after DNA was stained with Hoechst33342.
Annexin V-FITC/PI Cell Apoptosis Assay
Cells were digested with trypsin and washed with PBS. After adjusting the cell concentration to 2 × 105 cell/tube, cells were resuspended in 195 μL of binding buffer and each sample was added with 5 μL of Annexin V-FITC (BD), incubated at room temperature for 15 min in the dark. Followed washing with binding buffer, propidium iodide (PI) was added in each sample and incubated for 5 min. Then, samples were analyzed by flow cytometry.
Colony Formation Assay
HGC27/NC and HGC/shBAG2 cells were cultured in 6-well plates at a density of 2,000 cells/well overnight. Subsequently, cells were washed with PBS and supplied with fresh medium with serum every 3 days. After 12 days, the colonies were stained using 0.1% crystal violet, and photographed under a microscope (Nikon, Japan). The experiment was performed in triplicate.
Quantitative RT-PCR
The expression of miR186 and BAG2 mRNA in gastric cancer cell lines were performed by a real-time PCR system (PrimeScript™ RT reagent Kit, Takara). Briefly, total RNA was isolated from HGC27 cells using TRIzol reagent (Invitrogen, USA) according to the manufacture's instruction. The primers were synthesized by Taihegene (China). Expression of miR186 was normalized to U6 (endogenous control for miRNA) and BAG2 mRNA was normalized to Actin (endogenous control), and data was processed by the 2−(ΔΔCt) method.
Luciferase Reporter Assay
HGC27 cells were prepared in 24-well plates at a density of 5 × 104 cells/well overnight before transfection. Plasmid pmirGLO (Genechem, China) containing the BAG2 wild type (WT) and three kinds of BAG2 mutant type (Mut1, Mut2, and Mut1+2) were employed in the luciferase reporter assay. MiR186 mimics were transfected into the cells using X-tremeGENE HP DNA Transfection Reagent (Roche). After incubated for 48 h, luciferase activity was measured by a Dual-luciferase Reporter Assay System (Promega, USA) according to manufacturer's protocol.
Immunoprecipitation
HGC27 cells were harvested and lysed in cold RIPA lysis buffer containing protease inhibitors, followed by centrifuging. The cell lysates (50 μg/tube) were incubated with anti-BAG2, anti-ERK1/2 or control isotype IgG (2 μg) with gentle agitation at 4°C overnight. Subsequently, the reactive mixtures in individual tubes were added with 70 μl of protein G with TBST and the bound proteins were eluted with 2 × SDS loading buffer. The eluted proteins were subjected to SDS-PAGE and immunoblotting with anti-BAG2 and anti-ERK1/2, respectively.
Isobaric Tags for Relative and Absolute Quantitation (iTRAQ) Technology
The protein was extracted from HGC27/NC and HGC27/shBAG2 cells. After the concentration is determined by BCA kit, the protein was processed of disulfide bond cleavage and reductive alkylation of proteins with DTT and iodoacetamide, and then the protein was hydrolyzed by trypsin. Labeled with iTRAQ labeling reagent, the peptide was mixed in the same amount, and the high PH reverse pre-separation was carried out. The pre-separated components were analyzed by low PH nano-HPLC-MS/MS (Orbitrap Fusion), and the data acquisition mode was data-dependent acquisition mode. The proteins were analyzed by GO (Gene Ontology) and KEGG, and the differentially expressed proteins (DEPs) between groups was analyzed at the same time.
Statistical Analysis
Data are expressed as the mean ± SD. The difference among the groups was analyzed by one-way ANOVA and the difference between two groups was analyzed by Student's T-test. The χ2 test or linear by linear association was used to assess the correlation between BAG2 expression and clinicopathological features. The disease-free survival (DFS) of each group of patients was estimated by the Kaplan-Meier method and analyzed by Log-rank test. All statistical analyses were performed using the SPSS version 23.0 software. A P < 0.05 was considered statistically significant.
Results
High Expression of BAG2 in Gastric Carcinoma Results in Poor Prognosis
Oncomine database was used to query the differentially expressed genes (DEGs) between cancer tissues and para-cancerous gastric mucosa of patients with gastric cancer. We found that the expression of BAG2 in gastric cancer was significantly higher than that in normal gastric mucosa (P < 0.01; Figure 1A), and the expression of BAG2 in diffuse gastric cancer was higher than that in intestinal adenocarcinoma (Figure 1B). Meanwhile, we analyzed BAG2 in immortal cell lines, tissues, and tumor pathology in Human Protein Atlas database. It was revealed that BAG2 was highly expressed in abdominal tumor cell lines, including CACO-2, CAPAN-2, and Hep G2 (Figure 1C). However, in normal gastrointestinal tissues, BAG2 mRNA was at a low level and BAG2 protein was not often detected (Figure 1D). As for tumor pathology, the prognosis of BAG2 was analyzed by RNA samples from 354 cases of gastric cancer in The Cancer Genome Atlas. It was disclosed that the high expression of BAG2 in gastric cancer is associated with poor prognosis (the cutoff value of FPKM was 4.6, Log-rank P = 0.006) (Figure 1E).
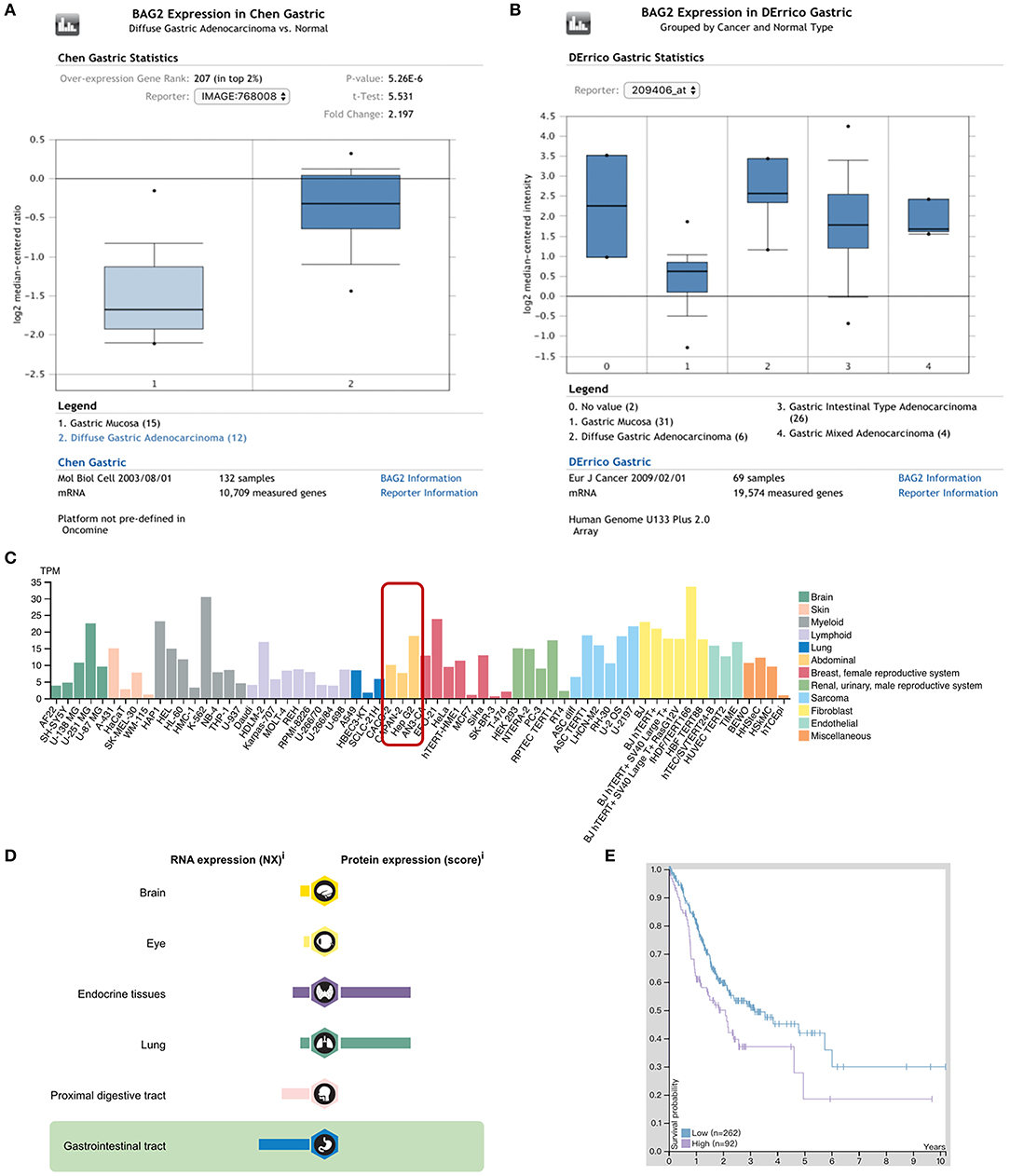
Figure 1. Expression of BAG2 in gastric carcinoma in Oncomine and Human ProteinAtlas databases. (A) Expression of BAG2 in gastric carcinoma is higher than normal gastric mucosa (Oncomine); (B) Expression of BAG2 in different types of gastric carcinoma (Oncomine); (C) BAG2 is highly expressed in various abnormal tumor cell lines (Human Protein Atlas); (D) Distribution and proportion of BAG2 mRNA and protein expression in various tissues of human body, including gastrointestinal tract (Human Protein Atlas); (E) Prognostic analysis of BAG2 in gastric cancer showed that the high expression of BAG2 suggested a poor prognosis (The purple line represents high expression of BAG2, and the blue line represents low expression of BAG2. When the FPKM cutoff value of BAG2 is 4.6, the survival time has significant statistical significance (Human Protein Atlas).
BAG2 Knockdown Inhibits Proliferation, Promotes Apoptosis, and Inhibits Invasion and Migration of Gastric Cancer Cells
To verify the above-mentioned results, we tested the expression of BAG2 in normal gastric mucosa cell line GES1 and gastric cancer cell lines with different differentiations, such as HGC27 (undifferentiated), MKN45 (poorly differentiated), MGC803 (poorly differentiated), and SGC7901 (moderately differentiated). Results showed that the expression of BAG2 in GES1 was significantly lower than that in various gastric cancer cell lines (Figure 2A). Next, we used the BAG2-specific siRNA to knock down BAG2 expression in HGC27 cells, and BAG2 knockdown on the biological behavior of gastric cancer cells was observed. Firstly, we assessed the knockdown efficiency of three different BAG2-siRNA, and observed the knockdown efficiency of all three siRNAs was more than 90%, we then randomly selected BAG2-siRNA (2#) to carry out the follow-up functional experiments (Figure 2B).
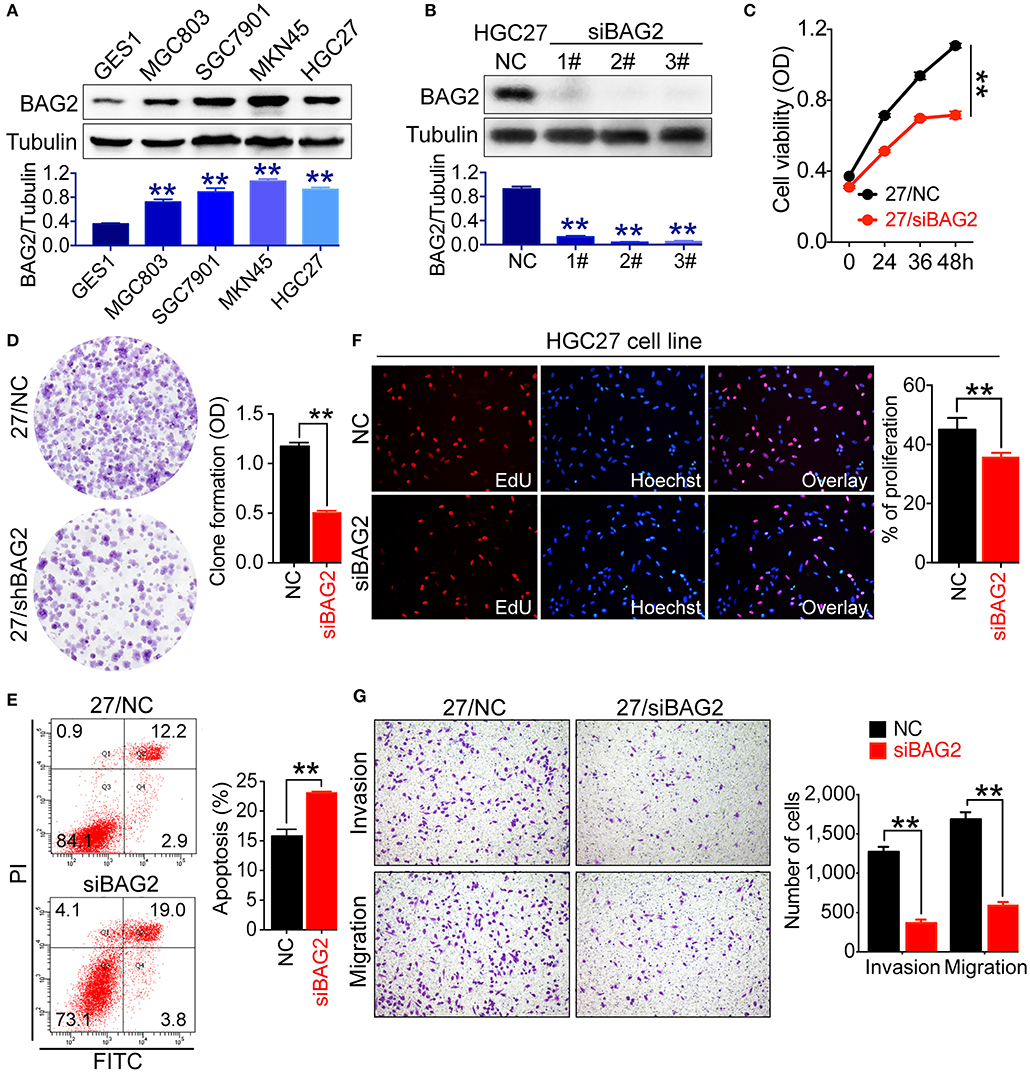
Figure 2. Biological function of BAG2 in gastric cancer cells. (A) Expression of BAG2 in GES1 and several gastric cancer cell lines (MGC803, SGC7901, MKN45, HGC27) showed that BAG2 expressed lowest in GES1 cell line; (B) Interference efficiency of BAG2-specific siRNAs; (C–G) Effect of BAG2 knockdown on cell viability (C), colony formation and cell apoptosis (D), cell proliferation (F), invasion and migration (G) of gastric cancer cells. **P < 0.01. 27/NC stands for HGC27/NC and 27/siBAG2 stands for HGC27/siBAG2.
Then we analyzed cell viability and proliferation of BAG2 knockdown gastric cancer cells using Cell Counting Kit-8 (CCK8) and colony formation assay, respectively. Knockdown of BAG2 significantly inhibited the cell viability and proliferation of HGC27 cells (Figures 2C,D). Using EdU reagent and Annexin V-FITC/PI cell apoptosis kit, we found that the proliferation ability of gastric cancer cells was markedly inhibited, and the apoptotic cells were significantly increased in BAG2 knockdown cells (Figures 2E,F). In addition, we also examined the influences of BAG2 on the invasion and migration of gastric cancer cells by Transwell chamber. The results showed that knockdown of BAG2 could notably inhibit the invasion and migration of gastric cancer cells (Figure 2G).
BAG2-Related Differential Expressed Proteins by iTRAQ Technology
Followed by transduction with lentiviral shRNA for BAG2 to stably reduce its expression within the 10th generation (Figure 3A), we utilized iTRAQ technology for further bioinformatics analysis. It was found that BAG2 was involved in a number of cellular processes, including metabolic processes, biological regulation, cellular component organization or biogenesis, immune system processes, reproductive processes, biological adhesion, rhythm process, etc. (Figure 3C).
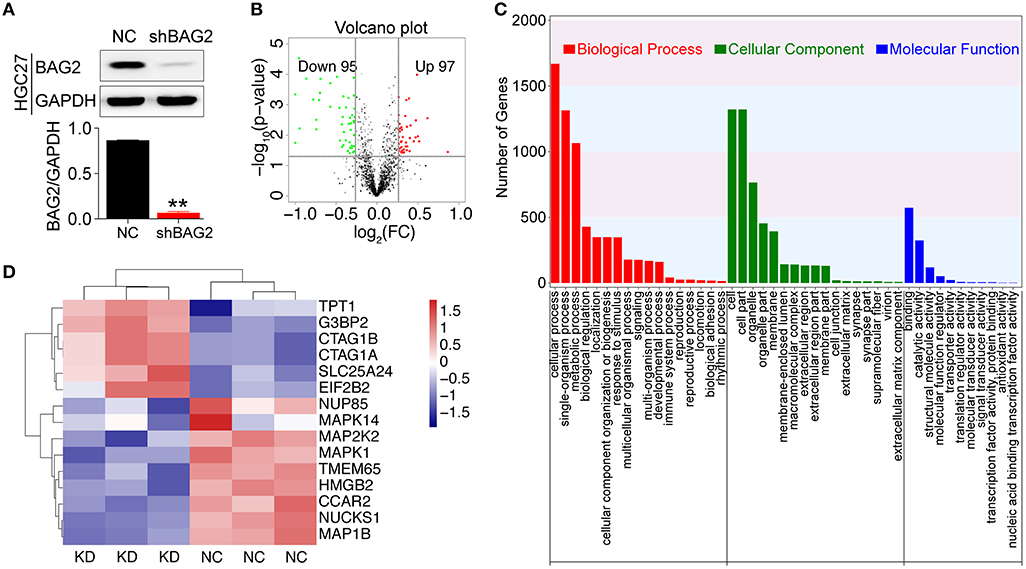
Figure 3. Proteomic analysis after knockdown BAG2. (A) Efficiency verification of shRNA for BAG2 knockdown in the HGC27 cell line (** P < 0.01); (B) Volcanic map of DEPs showed 97 up-regulated and 95 down-regulated (when the difference multiple was more than 1.2 times or <1/1.2 times. The p-value was <0.05 by t-test, which was regarded as DEPs); (C) iTRAQ proteomic analysis of biological processes (BP), cell component (CC) and molecular function (MF) in which BAG2 may be involved; (D) Clustering map of partial BAG2-related DEPs with statistical differences.
Moreover, the transcript differences between HGC27/NC and HGC27/shBAG2 (KD) were analyzed. A total of 196 DEPs were identified, and compared with NC group, 97 proteins were up-regulated, while 95 proteins were down-regulated (Figure 3B). Among them, the DEPs involved in the occurrence and development of cancer are detailed in the protein cluster diagram, including MAPK14, MAPK1 and CCAR2 (Figure 3D).
BAG2 Regulates the Proliferation of Gastric Cancer Cells Through AKT/mTOR and ERK Pathway and Affects the Migration Through EMT Process
The results of DEPs showed that BAG2 was regulated by mitogen-activated protein kinase (MAPK) and other signaling pathways, and previous cell functional experiments showed that BAG2 knockdown could attenuate the proliferation of gastric cancer cells. Therefore, we further detected the changes of MAPK signaling pathway after knockdown of BAG2. We found that the expression of p38 and p42/44 (ERK1/2) in MAPK signaling pathway were significantly down-regulated, and AKT, mTOR were also notably down-regulated (Figure 4A). In addition, immunoprecipitation suggested that BAG2 could bind to ERK1/2, thereby promoting the progression of gastric cancer (Figure 4B).
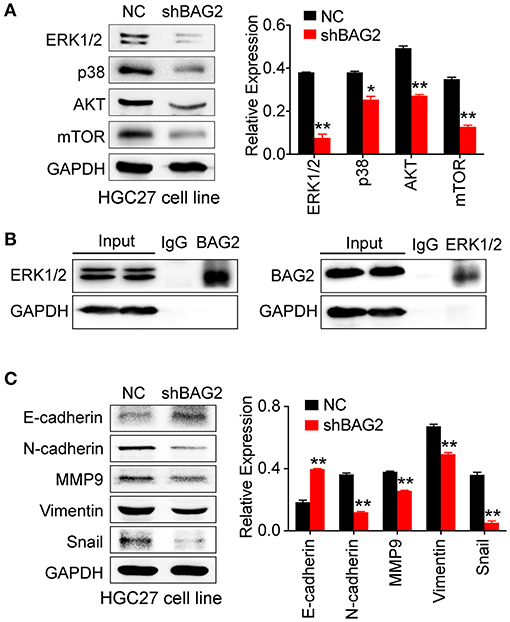
Figure 4. Effects of BAG2 knockdown on AKT/mTOR pathway, ERK pathway, and EMT process. (A) BAG2 knockdown significantly inhibits the expression of AKT, mTOR, p38, ERK1/2; (B) Immunoprecipitation result showed that BAG2 could bind to ERK1/2; (C) BAG2 knockdown significantly up-regulated E-cadherin and inhibited the expression of N-cadherin, MMP9, Vimentin and Snail, which were related to EMT process. *P < 0.05; **P < 0.01.
Our data showed that BAG2 enhanced cell invasion and migration, which may be related to epithelial-mesenchymal transformation (EMT). Therefore, we detected the expression of E-cadherin, N-cadherin, Vimentin, MMP9, and Snail in EMT in the absence of BAG2, and found out that the expression of other EMT-related proteins decreased in varying degrees except E-cadherin (Figure 4C).
Overexpression of miR186 Can Partially Inhibit the Expression of BAG2
Protein expression is typically regulated by miRNAs. To analyze specific binding between miRNA sequence and BAG2-3′UTR, we searched several miRNA target prediction related databases, including starBase v2.0 (http://starbase.sysu.edu.cn/), miRanda (http://www.microrna.org/), PicTar (http://pictar.mdc-berlin.de/) and TargetScant (http://www.targetscan.org/), we finally determined that miR186 might specifically bind to BAG2-3′UTR. Moreover, in DIANA website, where multiple interaction sites between miR186 and BAG2 may be found, we determined the first two sites for further verification (Binding sequence showed in Figure 5A).
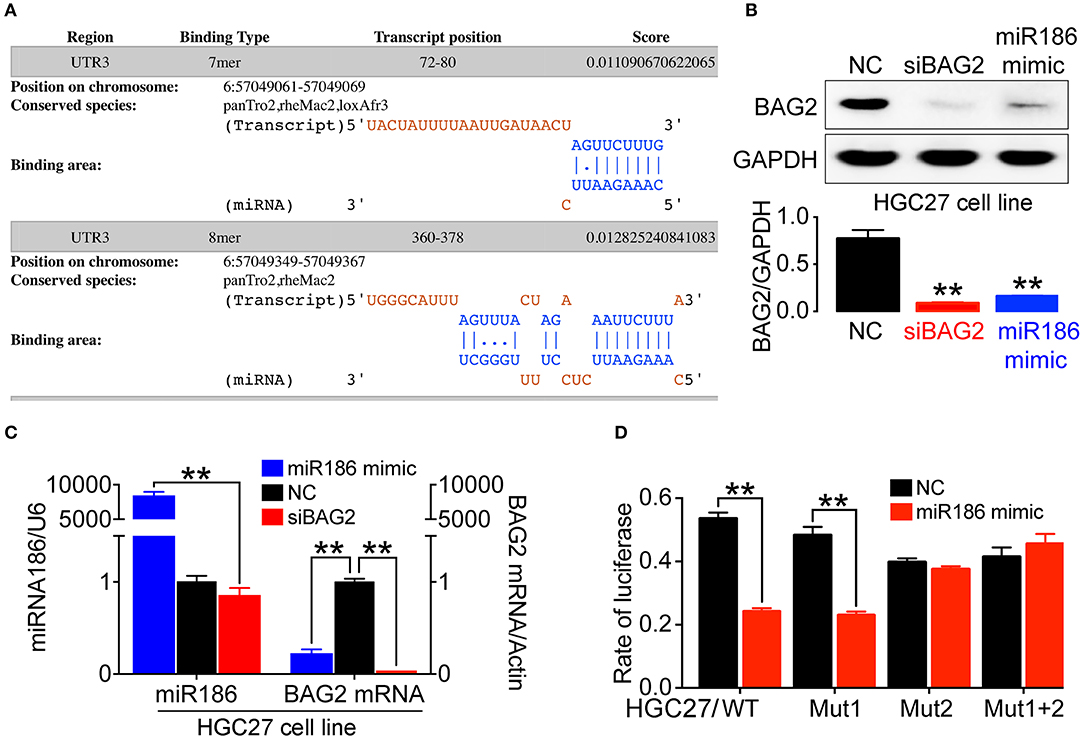
Figure 5. The expression of BAG2 was regulated by miR186. (A) Prediction of two high score binding sites between miR186 and BAG2-3′UTR on DIANA website; (B) Overexpression of miR186 inhibits expression of BAG2 protein; (C) Overexpression of miR186 inhibited BAG2 mRNA; (D) The effect of miR186 on the activity of luciferase reporter containing BAG2-3′UTR-WT, BAG2-3′UTR-Mut1, BAG2-3′UTR-Mut2 or BAG2-3′UTR-Mut1+2 was tested by luciferase reporter gene (**P < 0.01).
Therefore, by using Western blot and quantitative reverse transcription polymerase chain reaction (RT-qPCR), we verified that overexpression of miR186 (miR186 mimic) can inhibit the expression of BAG2 by degrading BAG2 mRNA (Figures 5B,C). In addition, through the Dual-luciferase Reporter Assay, we showed that miR186 could bind to BAG2-3′UTR through the second binding site (Figure 5D). Taken together, our data indicated that BAG2 is a direct target gene of miR186.
Clinical Significance of BAG2 in Gastric Cancer
In order to further confirm the clinical significance of BAG2 in gastric cancer, we specifically analyzed the prognostic significance and biological behavior of BAG2 in gastric cancer through KM-plotter database, in which the results showed that high expression of BAG2 indicated poor prognosis of gastric cancer (All, P = 0.064; GSE62254, P < 0.01; GSE15459, P < 0.01; GSE51101, P < 0.05; GSE14210, P = 0.064; GSE29272, P = 0.22 and GSE22377, P = 0.37) (Figure 6A). To verify the results of the above-mentioned bioinformatics analysis, we obtained the specimens of cancer tissue and para-cancerous gastric mucosa from 189 patients with gastric cancer. Immunohistochemistry was undertaken to confirm the localization of BAG2 protein, and it showed BAG2 mainly existed in cytoplasm (Figure 6B). Statistical results revealed that the BAG2 was low expressed in 96.3% (182/189) of normal gastric mucosa and high expressed in 48.1% (91/189) of cancer tissue (P < 0.01) (Figure 6B). Statistical analysis showed that the expression of BAG2 protein was significantly correlated with T stage and differentiation degree (P < 0.001). But there was no significant correlation between gender, N stage, M stage and clinical stages (Table 1).
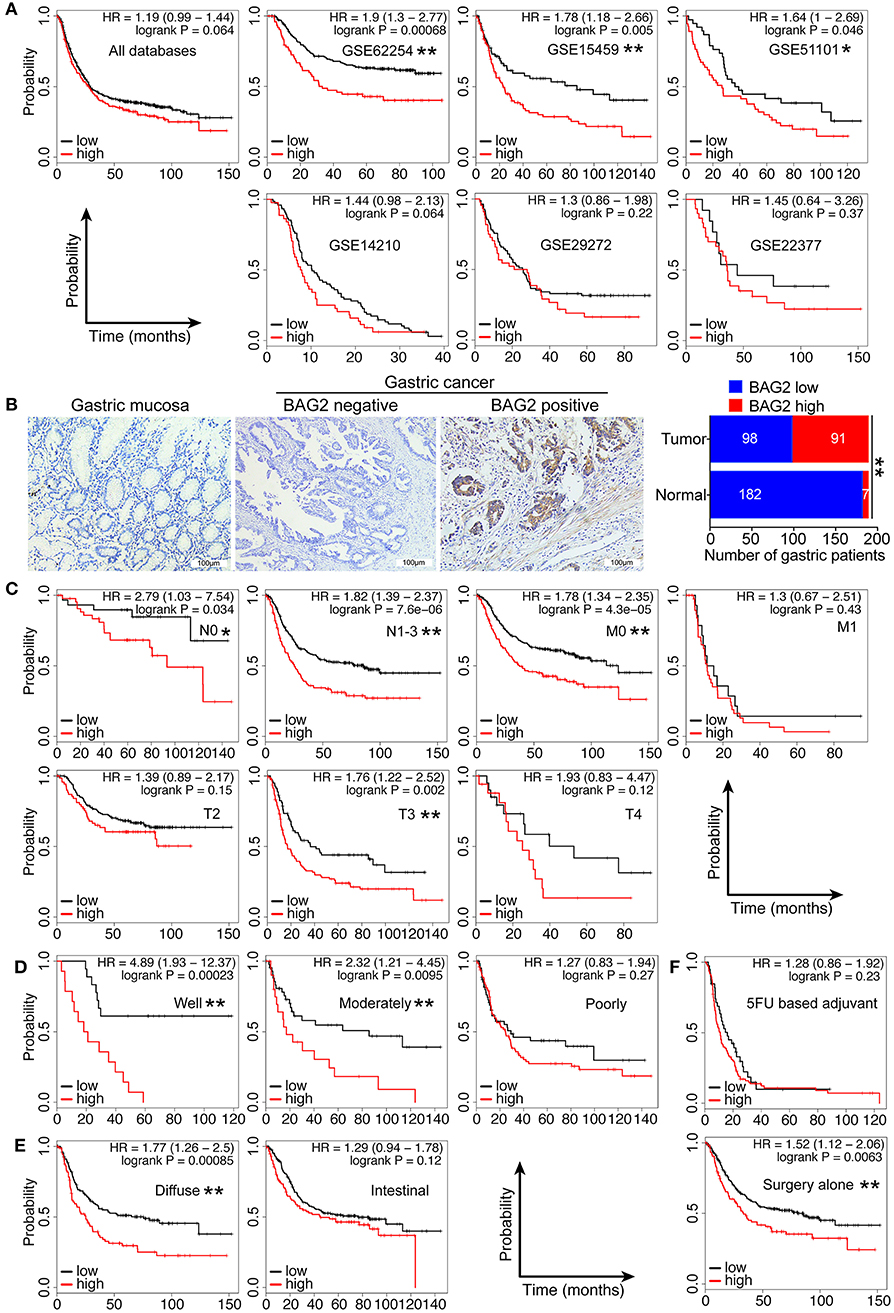
Figure 6. Clinical significance of high expression of BAG2 in gastric carcinoma. (A) Gastric cancer databases from KM-plotter shows high expression of BAG2 mRNA in gastric cancer suggests poor prognosis; (B) Typical immunohistochemical staining of BAG2 in para-cancerous and cancer tissue of patients with gastric cancer (400× magnification); (C–F) Prognostic analysis of BAG2 mRNA in different subgroups of gastric cancer database from KM-plotter, including T, N, M stage, differentiation degree, pathological type and treatment (*P < 0.05; ** P < 0.01).
At the same time, we employed KM-Plotter database to analyze the correlation between the high/low expression of BAG2 and the clinicopathological characteristics of patients with gastric cancer (Table 2). The results showed that high expression of BAG2 in female patients was significantly higher than that in male patients (65.5 vs. 23.6%, P < 0.01), and that in high grade T stage (T3+T4) was remarkably higher than that in low grade (T2). In N stage and M stage, the expression of BAG2 in high grade was higher than that in low grade (P < 0.01). In addition, we found that high expression of BAG2 was positively correlated with the degree of differentiation and the expression of HER2 (P < 0.01), while there was no significant difference in BAG2 expression among different pathological types (P = 0.813). Furthermore, the high expression of BAG2 in patients treated with 5FU chemotherapy was markedly higher than that in patients treated with surgery alone (74.5 vs. 27.0%, P < 0.01) (Table 2).
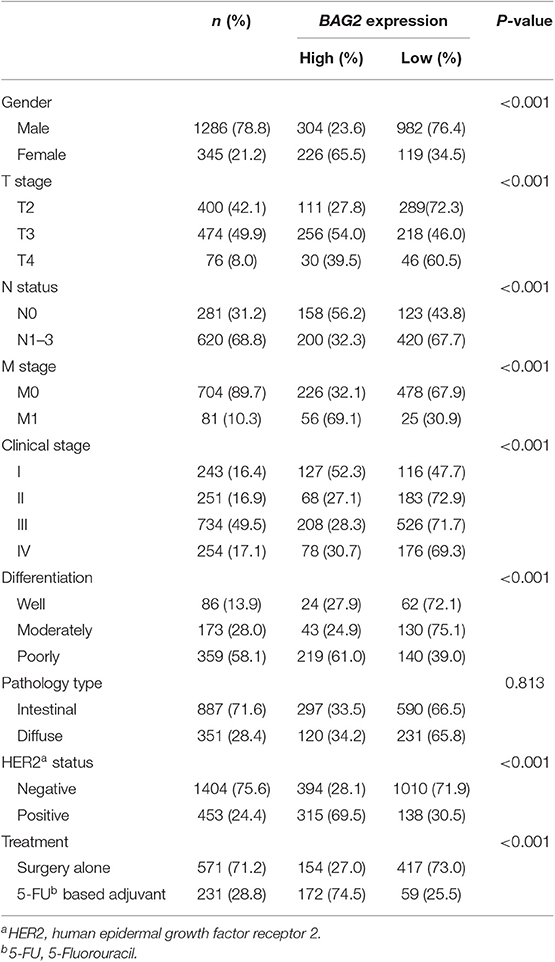
Table 2. Relationship between BAG2 expression and clinicalpathological features of gastric patients from KM-plotter.
In view of the fact that the high expression of BAG2 associates with poor prognosis in patients with gastric cancer and that is related to several clinicopathological characteristics, we further analyzed the prognosis of high/low expression of BAG2 in different clinicopathological subgroups. The findings showed that high expression of BAG2 showed different degrees of poor prognosis in different T3 stage, N0 and N1-3 stage, M0 stage with statistically significance (Figure 6C). In addition, compared with poorly differentiated carcinoma, in well-differentiated and moderately differentiated carcinoma, the high expression of BAG2 showed a significantly poor prognosis (Figure 6D). Meanwell, compared with intestinal type, the high expressed BAG2 indicated a significantly poor prognosis in the diffuse type (Figure 6E). Finally, in the 5FU chemotherapy group, the expression of BAG2 had no difference on the prognosis, but in the surgery alone group, the high expression of BAG2 was significantly correlated with the poor prognosis, which could better reflect the correlation between the expression of BAG2 and the prognosis of gastric cancer (Figure 6F).
Discussion
Numerous evidences have shown that BAG2 plays a substantial role in the pathogenesis of neurodegenerative diseases and neurotoxicity, such as Alzheimer's disease (14), Parkinson's disease (15), sevoflurane-induced neurotoxicity (16), etc. In recent years, the role of BAG2 in cancer has significantly attracted scholars' attention. High-throughput sequencing or proteomics of different types of tumors reflects the high expression of BAG2 in cancer, including ovarian cancer (17), papillary thyroid carcinoma (18), fibrosarcoma (19) and multiple myeloma (20). Yang et al. (12) found that the overexpression of BAG2 in triple-negative breast cancer was closely associated with poor clinical results, and the unique role of BAG2 was confirmed as an enzyme of cancer-promoting or anticancer genes. However, a limited number of studies have concentrated on the expression of BAG2 in gastric cancer.
In this study, we found that the expression of BAG2 in gastric cancer was significantly higher than that in normal gastric mucosa according to Oncomine and Human Protein Atlas databases. Simultaneously, we preliminarily confirmed the high expression of BAG2 in gastric cancer cell lines and its low expression in gastric mucosa cell line (GES1), which laid a foundation for further study. Previous studies have shown that BAG2 mediates important cellular responses to stress, including cell cycle arrest and apoptosis (15, 21). In our study of the biological function of BAG2 in gastric cancer cells, we found an increase in apoptotic cells, the proliferation and migration of gastric cancer cells were significantly inhibited after BAG2 knockdown. Therefore, it is important to further investigate the molecular mechanism of BAG2, playing these biological functions in gastric cancer.
Phosphatidylinositol-3-kinase/AKT/mTOR signaling pathway is considered as the main regulator of tumor (22). Abnormal mTOR signals can be observed in different types of cancer. Once overactivated, mTOR signaling promotes cell proliferation and metabolism, thereby promoting tumorigenesis and development (23). Our data confirm that BAG2 can regulate the proliferation of gastric cancer cells by activating AKT/mTOR pathway. P38 cascades are activated by various stresses or cytokines, and there are a variety of signaling pathways and a wide range of branches downstream of p38. Ueda et al. (24) found that BAG2 is directly phosphorylated by MAPKAP kinase 2 in vitro and in vivo, which is the main substrate of p38 and mediates several p38 dependent processes. Our results of protein spectrum and immunoblotting showed that BAG2 knockdown resulted in decreased levels of p38 and ERK1/2 proteins. In addition, immunoprecipitation suggests that BAG2 can bind ERK1/2, and then promote the progression of gastric cancer.
MiRNA is a class of non-coding single-stranded RNA molecules, playing a pivotal regulatory role in the process of biological development (25). MiRNAs regulate the expression of target gene at the post-transcriptional level, mainly though paired with the base of the 3′UTR of the target mRNA in a complete or incomplete complementary manner (26). Thus, miRNAs play a vital role in tumorigenesis, biological development, organogenesis, apparent regulation, and metabolism (27, 28). Previous studies have shown that miR186 targeted IGF-1R in glioma (29), and Yin Yang 1 (YY1) and cyclin dependent kinase 6 (CDK6) in prostate cancer (30). In addition, PTTG1 (31), P2X7 (32), FOXO1 (33), AKAP12 (34, 35) and Caspase-10 (36) have also been identified as direct targets of miR186 in different types of cancer in recent years. Here, we verified that overexpression of miR186 can inhibit the expression of BAG2. Through the dual-luciferase reporter assay, we found that miR186 directly interact with the second site (see Figure 5D) of BAG2-3′UTR.
EMT is a key process in tumor progression and metastasis (37). Li et al. (38) showed that overexpression of miR186 inhibits metastasis and EMT of colorectal cancer cells. Zhao et al. (39) found that miR186 strongly inhibits cell movement and EMT by down-regulating Twist1 in prostate cancer cells. Our data showed that BAG2 knockdown might suppress intercellular EMT process by down-regulating the expression of N-cadherin, MMP9, Vimentin and Snail, thereby reducing invasion and migration of gastric cancer cells. This is consistent with the previous results of miR186 overexpression inhibiting EMT process of cancer cells (38). In conclusion, it is suggested that the process of BAG2 promoting EMT in gastric cancer cells may be partially regulated by miR186.
According to our data about EdU and apoptosis assays, the cell cycle of HGC27 was blocked, the cell proliferation decreased, and the number of apoptotic cells increased after BAG2 knockdown. In addition, it has been reported that miR186 could inhibit cell cycle by targeting cyclin D1, CDK2, and CDK6 (40), and our results support the inhibition of BAG2 expression by miR186. At the same time, in DEPs detected by iTRAQ technology, we found that cell cycle and apoptosis regulatory protein 2 (CCAR2) decreased after BAG2 knockdown, suggesting that CCAR2 may act as a downstream factor of BAG2 to form miR186-BAG2-CCAR2 axis to regulate gastric cancer cell cycle and apoptosis. However, these findings need to be further verified.
Conclusion
We demonstrated that BAG2 is a more valuable prognostic indicator of gastric cancer. The miR186-BAG2-ERK1/2 axis is involved in gastric cancer progression, which may provide a molecular basis for understanding of the pathophysiological function of BAG2, in addition to support the fact that these molecules could comprise a potential target for gastric cancer.
Data Availability Statement
The datasets analyzed during the current study are available from the corresponding author on reasonable request.
Ethics Statement
The studies involving human participants were reviewed and approved by the Ethical Committee of the First Hospital of China Medical University. The patients/participants provided their written informed consent to participate in this study.
Author Contributions
LS, GC, CL, and KL contributed conception and design of the study. LS and GC performed the experiments. AS, ZW, and HH organized the database. ZG and WL performed the statistical analysis. LS and GC wrote the first draft of the manuscript. CL and KL wrote sections of the manuscript. All authors contributed to manuscript revision, read and approved the submitted version.
Funding
This study was supported by grants from the National Natural Science Foundation of China (No. 31601142) and the Key Research and Development Program Guidance Project of Liaoning Province (No. 2017225034).
Conflict of Interest
The authors declare that the research was conducted in the absence of any commercial or financial relationships that could be construed as a potential conflict of interest.
Acknowledgments
We would like to thank Professor Guoxin Liang for his revision of this article. All authors have contributed significantly to the content of the manuscript.
References
1. Bray F, Ferlay J, Soerjomataram I, Siegel RL, Torre LA, Jemal A. Global cancer statistics 2018: GLOBOCAN estimates of incidence and mortality worldwide for 36 cancers in 185 countries. CA Cancer J Clin. (2018) 68:394–424. doi: 10.3322/caac.21492
2. Seeruttun SR, Cheung WY, Wang W, Fang C, Liu ZM, Li JQ, et al. Identification of molecular biomarkers for the diagnosis of gastric cancer and lymph-node metastasis. Gastroenterol Rep. (2019) 7:57–66. doi: 10.1093/gastro/goy023
3. Schonbuhler B, Schmitt V, Huesmann H, Kern A, Gamerdinger M, Behl C. BAG2 Interferes with CHIP-Mediated Ubiquitination of HSP72. Int J Mol Sci. (2016) 18:E69. doi: 10.3390/ijms18010069
4. Taylor IR, Dunyak BM, Komiyama T, Shao H, Ran X, Assimon VA, et al. High-throughput screen for inhibitors of protein-protein interactions in a reconstituted heat shock protein 70 (Hsp70) complex. J Biol Chem. (2018) 293:4014–25. doi: 10.1074/jbc.RA117.001575
5. Qin L, Guo J, Zheng Q, Zhang H. BAG2 structure, function and involvement in disease. Cell Mol Biol Lett. (2016) 21:18. doi: 10.1186/s11658-016-0020-2
6. Sondermann H, Scheufler C, Schneider C, Hohfeld J, Hartl FU, Moarefi I. Structure of a Bag/Hsc70 complex: convergent functional evolution of Hsp70 nucleotide exchange factors. Science. (2001) 291:1553–7. doi: 10.1126/science.1057268
7. Arndt V, Daniel C, Nastainczyk W, Alberti S, Hohfeld J. BAG-2 acts as an inhibitor of the chaperone-associated ubiquitin ligase CHIP. Mol Biol Cell. (2005) 16:5891–900. doi: 10.1091/mbc.e05-07-0660
8. Dai Q, Qian SB, Li HH, McDonough H, Borchers C, Huang D, et al. Regulation of the cytoplasmic quality control protein degradation pathway by BAG2. J Biol Chem. (2005) 280:38673–81. doi: 10.1074/jbc.M507986200
9. Zhang J, Lou X, Yang S, He S, Yang L, Liu M, et al. BAG2 is a target of the c-Myc gene and is involved in cellular senescence via the p21(CIP1) pathway. Cancer Lett. (2012) 318:34–41. doi: 10.1016/j.canlet.2011.11.033
10. Wang HQ, Zhang HY, Hao FJ, Meng X, Guan Y, Du ZX. Induction of BAG2 protein during proteasome inhibitor-induced apoptosis in thyroid carcinoma cells. Br J Pharmacol. (2008) 155:655–60. doi: 10.1038/bjp.2008.302
11. Yue X, Zhao Y, Liu J, Zhang C, Yu H, Wang J, et al. BAG2 promotes tumorigenesis through enhancing mutant p53 protein levels and function. Elife. (2015) 4. doi: 10.7554/eLife.08401
12. Yang KM, Bae E, Ahn SG, Pang K, Park Y, Park J, et al. Co-chaperone BAG2 determines the pro-oncogenic role of cathepsin B in triple-negative breast cancer cells. Cell Rep. (2017) 21:2952–64. doi: 10.1016/j.celrep.2017.11.026
13. Liu C, Sun L, Yang J, Liu T, Yang Y, Kim SM, et al. FSIP1 regulates autophagy in breast cancer. Proc Natl Acad Sci USA. (2018) 115:13075–80. doi: 10.1073/pnas.1809681115
14. Qu D, Hage A, Don-Carolis K, Huang E, Joselin A, Safarpour F, et al. BAG2 gene-mediated regulation of PINK1 protein is critical for mitochondrial translocation of PARKIN and neuronal survival. J Biol Chem. (2015) 290:30441–52. doi: 10.1074/jbc.M115.677815
15. Song Z, Xu S, Song B, Zhang Q. Bcl-2-associated athanogene 2 prevents the neurotoxicity of MPP+ via interaction with DJ-1. J Mol Neurosci. (2015) 55:798–802. doi: 10.1007/s12031-014-0481-6
16. Qi J, Jia Y, Wang W, Lu H, Wang Y, Li Z. The role of Bag2 in neurotoxicity induced by the anesthetic sevoflurane. J Cell Biochem. (2018). doi: 10.1002/jcb.28029. [Epub ahead of print].
17. Zhang XY, Hong SS, Zhang M, Cai QQ, Zhang MX, Xu CJ. Proteomic alterations of fibroblasts induced by ovarian cancer cells reveal potential cancer targets. Neoplasma. (2018) 65:104–12. doi: 10.4149/neo_2018_101
18. Selmansberger M, Feuchtinger A, Zurnadzhy L, Michna A, Kaiser JC, Abend M, et al. CLIP2 as radiation biomarker in papillary thyroid carcinoma. Oncogene. (2015) 34:3917–25. doi: 10.1038/onc.2014.311
19. Klopfleisch R, Meyer A, Lenze D, Hummel M, Gruber AD. Canine cutaneous peripheral nerve sheath tumours versus fibrosarcomas can be differentiated by neuroectodermal marker genes in their transcriptome. J Comp Pathol. (2013) 148:197–205. doi: 10.1016/j.jcpa.2012.06.004
20. Ge F, Zhang L, Tao SC, Kitazato K, Zhang ZP, Zhang XE, et al. Quantitative proteomic analysis of tumor reversion in multiple myeloma cells. J Proteome Res. (2011) 10:845–55. doi: 10.1021/pr100992e
21. Liang S, Song Z, Wu Y, Gao Y, Gao M, Liu F, et al. MicroRNA-27b modulates inflammatory response and apoptosis during mycobacterium tuberculosis infection. J Immunol. (2018) 200:3506–18. doi: 10.4049/jimmunol.1701448
22. Yang J, Nie J, Ma X, Wei Y, Peng Y, Wei X. Targeting PI3K in cancer: mechanisms and advances in clinical trials. Mol Cancer. (2019) 18:26. doi: 10.1186/s12943-019-0954-x
23. Tian T, Li X, Zhang J. mTOR signaling in cancer and mTOR inhibitors in solid tumor targeting therapy. Int J Mol Sci. (2019) 20:E755. doi: 10.3390/ijms20030755
24. Ueda K, Kosako H, Fukui Y, Hattori S. Proteomic identification of Bcl2-associated athanogene 2 as a novel MAPK-activated protein kinase 2 substrate. J Biol Chem. (2004) 279:41815–21. doi: 10.1074/jbc.M406049200
25. Takahashi RU, Prieto-Vila M, Hironaka A, Ochiya T. The role of extracellular vesicle microRNAs in cancer biology. Clin Chem Lab Med. (2017) 55:648–56. doi: 10.1515/cclm-2016-0708
26. Ariyoshi J, Momokawa D, Eimori N, Kobori A, Murakami A, Yamayoshi A. Development of Novel Antisense Oligonucleotides for the Functional Regulation of RNA-Induced Silencing Complex (RISC) by Promoting the Release of microRNA from RISC. Bioconjug Chem. (2015) 26:2454–60. doi: 10.1021/acs.bioconjchem.5b00501
27. Liu HS, Xiao HS. MicroRNAs as potential biomarkers for gastric cancer. World J Gastroenterol. (2014) 20:12007–17. doi: 10.3748/wjg.v20.i34.12007
28. Jamali L, Tofigh R, Tutunchi S, Panahi G, Borhani F, Akhavan S, et al. Circulating microRNAs as diagnostic and therapeutic biomarkers in gastric and esophageal cancers. J Cell Physiol. (2018) 233:8538–50. doi: 10.1002/jcp.26850
29. Jiang J, Wang W, Fang D, Jin X, Ding L, Sun X. MicroRNA186 targets IGF1R and exerts tumorsuppressing functions in glioma. Mol Med Rep. (2017) 16:7821–8. doi: 10.3892/mmr.2017.7586
30. Lu S, Wang MS, Chen PJ, Ren Q, Bai P. miRNA-186 inhibits prostate cancer cell proliferation and tumor growth by targeting YY1 and CDK6. Exp Ther Med. (2017) 13:3309–14. doi: 10.3892/etm.2017.4387
31. Xiao Q, Wei Z, Li Y, Zhou X, Chen J, Wang T, et al. miR186 functions as a tumor suppressor in osteosarcoma cells by suppressing the malignant phenotype and aerobic glycolysis. Oncol Rep. (2018) 39:2703–10. doi: 10.3892/or.2018.6394
32. Zhou L, Qi X, Potashkin JA, Abdul-Karim FW, Gorodeski GI. MicroRNAs miR-186 and miR-150 down-regulate expression of the pro-apoptotic purinergic P2X7 receptor by activation of instability sites at the 3'-untranslated region of the gene that decrease steady-state levels of the transcript. J Biol Chem. (2008) 283:28274–86. doi: 10.1074/jbc.M802663200
33. Myatt SS, Wang J, Monteiro LJ, Christian M, Ho KK, Fusi L, et al. Definition of microRNAs that repress expression of the tumor suppressor gene FOXO1 in endometrial cancer. Cancer Res. (2010) 70:367–77. doi: 10.1158/0008-5472.CAN-09-1891
34. Goeppert B, Schmezer P, Dutruel C, Oakes C, Renner M, Breinig M, et al. Down-regulation of tumor suppressor A kinase anchor protein 12 in human hepatocarcinogenesis by epigenetic mechanisms. Hepatology. (2010) 52:2023–33. doi: 10.1002/hep.23939
35. Jones DZ, Schmidt ML, Suman S, Hobbing KR, Barve SS, Gobejishvili L, et al. Micro-RNA-186–5p inhibition attenuates proliferation, anchorage independent growth and invasion in metastatic prostate cancer cells. BMC Cancer. (2018) 18:421. doi: 10.1186/s12885-018-4258-0
36. Zhang J, Du Y, Wu C, Ren X, Ti X, Shi J, et al. Curcumin promotes apoptosis in human lung adenocarcinoma cells through miR-186* signaling pathway. Oncol Rep. (2010) 24:1217–23. doi: 10.3892/or_00000975
37. Lee TK, Poon RT, Yuen AP, Ling MT, Kwok WK, Wang XH, et al. Twist overexpression correlates with hepatocellular carcinoma metastasis through induction of epithelial-mesenchymal transition. Clin Cancer Res. (2006) 12:5369–76. doi: 10.1158/1078-0432.CCR-05-2722
38. Li J, Xia L, Zhou Z, Zuo Z, Xu C, Song H, et al. MiR-186–5p upregulation inhibits proliferation, metastasis and epithelial-to-mesenchymal transition of colorectal cancer cell by targeting ZEB1. Arch Biochem Biophys. (2018) 640:53–60. doi: 10.1016/j.abb.2018.01.002
39. Zhao X, Wang Y, Deng R, Zhang H, Dou J, Yuan H, et al. miR186 suppresses prostate cancer progression by targeting Twist1. Oncotarget. (2016) 7:33136–51. doi: 10.18632/oncotarget.8887
Keywords: gastric cancer, BAG2, miR186, iTRAQ proteomics technology, therapeutic target
Citation: Sun L, Chen G, Sun A, Wang Z, Huang H, Gao Z, Liang W, Liu C and Li K (2020) BAG2 Promotes Proliferation and Metastasis of Gastric Cancer via ERK1/2 Signaling and Partially Regulated by miR186. Front. Oncol. 10:31. doi: 10.3389/fonc.2020.00031
Received: 31 May 2019; Accepted: 09 January 2020;
Published: 31 January 2020.
Edited by:
Jia Wei, Nanjing Drum Tower Hospital, ChinaReviewed by:
Angela Hague, University of Bristol, United KingdomGabriele Multhoff, Technical University of Munich, Germany
Copyright © 2020 Sun, Chen, Sun, Wang, Huang, Gao, Liang, Liu and Li. This is an open-access article distributed under the terms of the Creative Commons Attribution License (CC BY). The use, distribution or reproduction in other forums is permitted, provided the original author(s) and the copyright owner(s) are credited and that the original publication in this journal is cited, in accordance with accepted academic practice. No use, distribution or reproduction is permitted which does not comply with these terms.
*Correspondence: Kai Li, Y211X2xpa2FpQDE2My5jb20=