- 1Unidad de Gestión Clínica Intercentros de Oncología Medica, Hospitales Universitarios Regional y Virgen de la Victoria de Málaga, Málaga, Spain
- 2Laboratorio de Biología Molecular del Centro de Investigaciones Médico-Sanitarias de Málaga (CIMES), Instituto de Investigación Biomédica de Málaga (IBIMA), Universidad de Málaga (UMA), Málaga, Spain
- 3Centro de Investigación Biomédica en Red de Oncología, CIBERONC-ISCIII, Madrid, Spain
The main obstacle for designing effective treatment approaches in breast cancer is the extensive and the characteristic heterogeneity of this tumor. The vast majority of critical genomic changes occurs during breast cancer progression, creating a significant variability within primary tumors as well as between the primary breast cancer and their metastases, a hypothesis have already demonstrated in retrospective studies (1). A clear example of this is the HER2-positive breast cancer. In these tumors, we can find all of the transcriptional subtypes of breast cancer, even the basal like or luminal A subtypes. Although the HER2-enriched is the most representative transcriptional subtype in the HER2-positive breast cancer, we can find it too in breast cancers with HER2-negative status. This intrinsic subtype shows a high expression of the HER2 and is associated with proliferation-related genes clusters, among other features. Therefore, two hypotheses can be suggested. First, the HER2 amplification can be a well-defined driver event present in all of the intrinsic subtypes, and not a subtype marker isolated. Secondly, HER2-enriched subtype can have a distinctive transcriptional landscape independent of HER2 amplification. In this review, we present an extensive revision about the last highlights and advances in clinical and genomic settings of the HER2-positive breast cancer and the HER2-enriched subtype, in an attempt to improving the knowledge of the underlying biology of both entities and to explaining the intrinsic heterogeneity of HER2-positive breast cancers.
Introduction
Breast cancer (BC) is the most common malignant tumor in women and one of the principal causes of cancer mortality in this sex, despite significant improvements obtained in the lasts decades. Conversely, male breast cancer is a rare disease with an incidence of <1% and mainly classified by immunohistochemistry as a luminal disease (2). BC is modeled by a group of heterogeneous diseases, at both an inter- and intra-tumoral level. All of them share a substantial morphological and molecular heterogeneity, what affect to his clinical behavior and therapeutic response. A crucial objective in the treatment of any cancer disease is to perform clinical decisions through a comprehensive insight of the molecular profile of the tumor to predict the probable clinical outcome of the disease individually. By the expansion of high-throughput molecular technologies, we can analyze changes in the genetic, epigenetic and proteomics contexts, so that allows improving in the comprehension of the complexity of BC biology.
One biomarker with reported heterogeneity in BC is the Human Epidermal Growth Factor Receptor 2 (HER2), a component of the EGF receptor (EGFR) family. The overexpression of this biomarker defined the HER2-positive disease. Traditionally, HER2-positive breast cancer (HER2+ BC) has been associated with a worse prognosis and inferior outcomes in survival. However, over the last years, several therapeutic advances have been improved the clinical treatment of HER2+ disease, and thus, its prognosis. After the discovery of the intrinsic subtypes through gene expression analysis, and later transcriptomic and genomic studies, there is sufficient evidence that HER2+ BC is an entity with a large heterogeneity at multiple levels (3), including cell-to-cell. There has been discrepancy about the determination of the clinical status of HER2+ over the last years, with several guidelines and updates in order to find a formal and universal consensus. In clinical practice, HER2+ tumors are categorized by immunohistochemistry (IHC) and/or by in situ hybridization (ISH) in order to tailor the different therapeutic approaches (4).
The gene expression profiling has had a large-scale impact in the progress about the knowledge of the biological heterogeneity of this tumor (5). However, in this ambit, there is a considerable variability as well, what makes it even more difficult to categorize the basis of pathological diagnosis and therapeutic approach. The principal molecular subtypes of BC have widely characterized, and within HER2+ BC the most representative intrinsic subtype is the HER2-enriched (HER2-E). However, we can find HER2+ BC with luminal A, luminal B, or even the basal-like subtype (6). The intrinsic subtype HER2-E is defined generally by a higher expression of HER2 at the RNA and protein level than other subtypes, in addition the increased expression of the tumor proliferation-related genes (6, 7). Recent studies confirm that this subtype obtains the best clinical and therapeutic results by anti-HER2 therapies, with or without chemotherapy, in both adjuvant and neoadjuvant scenarios, and regardless of the clinical status of HER2 (3). Nonetheless, no more than 50% of clinically HER2+ tumors are HER2-E, and what is more exciting, we can also find this subtype in clinically HER2-negative BC, which do not receive HER2-therapies since these drugs are not approved for the treatment of clinically HER2-negative breast tumors. Therefore, we consider it is highly important to perform an extensive revision about the latest highlights and advances in clinical outcomes and genomic features within HER2+ BC and its most representative intrinsic subtype, HER2-E, with a previous extensive revision from the state of science in which these advances are based.
Current Classification of Breast Cancer
Intertumoral heterogeneity of BC is initially illustrated with a clinical staging of the disease. The TNM staging system by the American Joint Committee on Cancer and Union for International Cancer Control (AJCC/UICC) adds information about tumor features such as size, regional lymph-node involvement or the presence of distant metastases (8). After the clinical diagnosis, the first step is the assessment of histological criteria on the primary tumor obtained by surgery and/or a core biopsy, encompassing morphology-base and immunohistochemical (IHC) analyses for testing the biomarker profile. This is a classical and non-molecular classification of BC, and sets the standard in the usual clinical practice. Classic pathological criteria, such as histological type, tumor size, grade and axillary lymph node status, are relevant for the initial prognostic evaluation (9). The expression of hormone receptors [estrogen (ER) and progesterone receptors (PR)] by IHC and the overexpression and/or amplification of HER2 by IHC and/or ISH gives additional predictive value, being elementary for guiding algorithms of treatment (9, 10), as will be discussed in the following two sections.
Histopathological Subtypes: Morphologic Heterogeneity
The histopathological classification of BC is set by the 2012 World Health Organization (WHO) (11). Most of the breast cancers are adenocarcinomas, with around 70–80% defined as invasive ductal carcinomas not otherwise specified (IDC-NOS) (11). The rest, around 25–30%, are characterized by “histological special types” such as papilar, metaplastic, cribiform, apocrine, or mucinous carcinomas, among others (11). The majority of special types is rare and differ strongly about prognosis and response to the treatments (12). The tumor grade is the other important intrinsic characteristic of tumoral heterogeneity (13, 14).
Immunohistochemistry: ER, PR, and HER2
Via the characterization of ER, PR, and HER2 status, we can divide BC in three phenotypes or entities. Hormone receptor-positive breast cancers are defined as positive by expression of ER and/or PR receptor equal to 1% or higher of invasive cancer cells (15). ER and PR receptors are expressed around 80 and 65% of breast cancers, respectively (16). Although estrogen receptor-positive tumors co-express PR in the majority of breast cancers, some cases are ER+/PR– and less frequently, ER–/PR+. The response to hormonal therapy seems to be major in breast tumors with positivity for ER and PR, with lower rates in ER+/PR– and ER–/PR+ tumors (11).
Approximately 15–20% of BC has HER2 overexpression and/or amplification, and over 50% of these co-expressing hormone receptors (13, 17). These tumors are called HER2+ BC. The remaining, with negativity for hormonal receptors and HER2, are denominated triple-negative breast cancers. A fourth protein marker, the androgen receptor (AR), is immunoexpressed in 60–80% of breast cancers, with similar proportions to prostate tumors, and specially expressed in HER2+ and triple-negative breast tumors. However, its determination is still not justified in clinical practice as there is no targeted treatment approved for this marker. Other biomarkers with heterogeneous expression include the epidermal growth factor receptor (EGFR), p53, c-myc, and proliferation markers such as Ki-67 (14, 18). Ki-67 is a nuclear protein, expressed in all phases of the cell cycle except G0, and a cellular marker of proliferation with prognostic and predictive value (16, 19).
Even so, this current and basic classification of human breast tumors presents a number of important limitations. The main one is the variability in therapeutic response and clinical outcomes, even for tumors with similar clinical and pathological features. Secondly, this classification provides limited knowledge into the biology and the molecular pathways that divide the BC in distinct subtypes and stages, stepping away from the personalized treatment paradigm.
Molecular and Genomic Classification of Breast Cancer
Expression analysis has provided an opportunity to explore comprehensive molecular profiling of BC. Differences in gene expressions patterns display basic alterations in the tumor cell biology and are associated with significant variation in terms of clinical behavior, survival (17, 20–22), and treatment outcomes (23–37). The identification of several molecular subtypes was the first insight into the molecular heterogeneity of the BC (20). Five main intrinsic subtypes have been identified based solely on gene expression patterns using DNA microarrays (20, 22): luminal A, luminal B, HER2 overexpressing or HER2-enriched (HER2-E) and basal like, with another less characterized group named normal breast-like. They are called as “intrinsic subtypes of breast cancer” and they have exposed crucial differences in several aspects. The tumor heterogeneity within hormone receptor-positive breast cancers are encompassed by the luminal A and luminal B subtypes, with better survival outcomes with respect to the non-luminal intrinsic subtypes. The luminal B breast tumor expresses hormonal receptors same as the luminal A subtype, but generally having low PR, high proliferation, high grade and worse response to hormonal therapy. At the molecular level, this subtype seems to be dramatically distinct from luminal A, at levels of gene expression, gene copy, or somatic aberrations. All of these features, confers it worse prognosis than the other luminal intrinsic subtype (5).
In 2009, Parker et al. (25) introduced a gene expression-based test named PAM50, which identifies the intrinsic molecular subtypes in four well-established transcriptional subtypes, through the expression of 50 genes in formalin-fixed paraffin embedded (FFPE) tumor tissues: luminal A, luminal B, basal-like, and HER2-enriched (25, 28, 31). The intrinsic subtypes overlap with staining of ER, PR and HER2 protein expression by IHC and complemented with ISH for testing HER2 gene amplification. However, several studies have assessed and compared the classification of breast tumors based on the PAM50 gene expression with the classification based on pathological criteria, and a low concordance rate was found in the majority of these studies (31, 34–42). For example, in a combined analysis of data from several studies including a total of 5,994 independent tumor samples, the discordance rate was found to be present in 30.72% across all patients (43). The majority of these studies performed central assessment of pathology-based biomarkers, which normally shows less discrepancies than local determination (15). Therefore, the two methods should never be considered the same to identify intrinsic biology of BC.
Nonetheless, the diverse genomic landscape of BC is not completely captured through histopathological or transcriptomic analysis. Changes in gene expression patterns are influenced by the underlying genomic structure, and we have evidence that some features of the intrinsic subtypes can be defined by copy number profiling (5, 29, 44) The development of next-generation sequencing technologies has allowed for the characterization of the mutational landscape of this disease, with the identification of novel cancer genes that found it to be recurrently mutated in BC (6, 36, 45, 46). The relevant of integration of the intrinsic subtype with genomic analysis are highlighted in one of the most complete and important molecular characterization studies that have ever been performed in BC (5). In this study, led by The Cancer Genome Atlas Project (TCGA), more than 600 primary tumors were extensively profiling at the DNA (methylation, copy-number alterations, somatic and germline mutations), RNA (i.e., miRNA sequencing and mRNA expression) and protein levels (5) (Table 1; Figure 1). After the analysis of more than 300 primary tumors, five different data-types were mixed together in a cluster of 10 clusters. The consensus clustering analysis identified four major groups of BC, which were found to be very-well summarize by the four molecular intrinsic subtypes defined by mRNA expression only (47) (Figure 2).
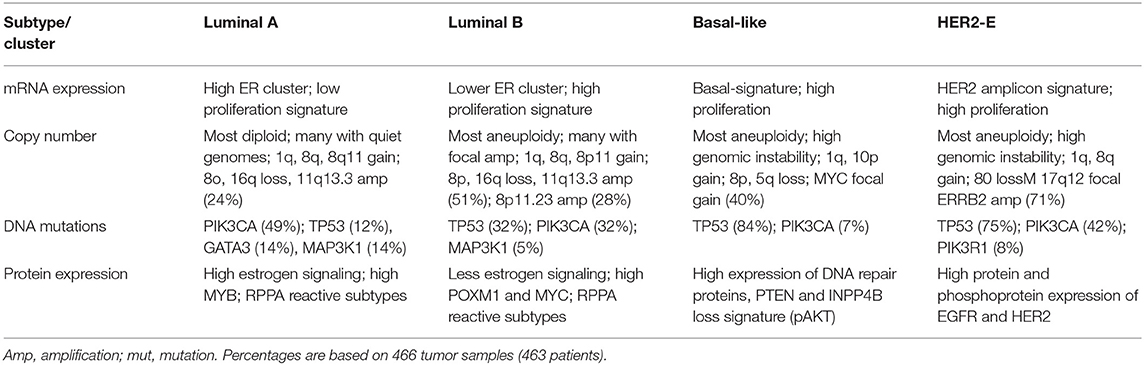
Table 1. Main data about mRNA expression, copy number, DNA mutations and protein expression in the breast cancer tissue samples analyzed in the TGCA project (5).
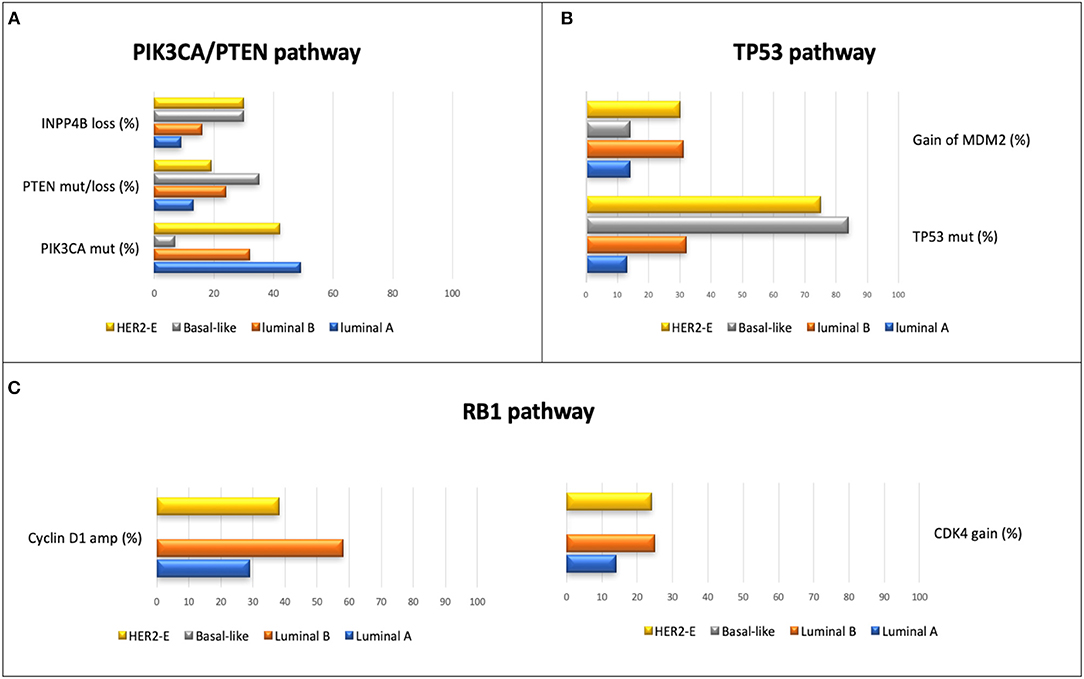
Figure 1. Principal alterations in most representative pathways in BC, according the intrinsic profiling, analyzed in the TGCA project (5); percentages are based on 466 tumor samples (463 patients). (A) Principal alterations in TP53 pathway. (B) Principal alterations in PIK3CA/PTEN pathway. (C) principal alterations in RB1 pathway. Within the basal-like intrinsic subtype, the main alterations found in this pathway were RB1 mut/loss (20%) and amplification of Cyclin E1 (9%). The expression degree of CDKN2C and RB1 was low and high, respectively, in the luminal A subtype, unlike what was reported in tumors with basal-like subtype.
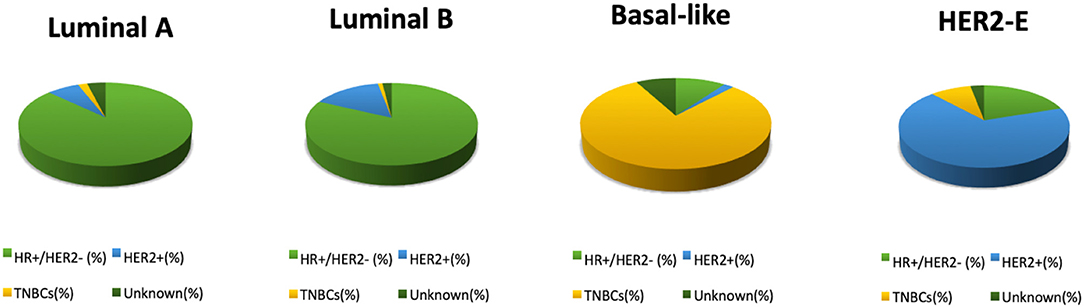
Figure 2. Distribution of PAM50 intrinsic subtypes within each IHC subtype of the breast cancers analyzed in the TGCA project (5); percentages are based on 466 tumor samples (463 patients).
Thus, all breast cancers show significant genetic diversity. Inherited variants, represented by the single-nucleotide polymorphisms (SNPs) and copy number variants (CNVs), can have an impact in a germline genetic landscape of the individual and inducing the cancer development. The single-nucleotide variants (mutations) and copy number aberrations (CNAs) are genomic changes at somatic level, thus variations acquired that contribute to the initiation and the dissemination of sporadic breast tumors (48). In a recent study, the authors integrated analysis of both, genomic and transcriptomic data, in 2,000 breast tumors as part of the METABRIC consortium (36) dataset, and proposed an alternative molecular classification (48) (Table 2). Germline variants and somatic alterations were found to be linked with changes in gene expressions, and the CNAs reported the greatest variability. Clustering analysis of joint copy number and gene expression data from the cis-associated gene reported 10 new molecular subgroups or integrative clusters with the capacity of dividing the main intrinsic subtypes into independent groups. Each integrative clusters are characterized by distinct CNAs, gene expression changes, clinical characteristics and different survival outcomes (48). This extensive heterogeneity, as a result of different cell-of-origins and molecular variations, makes that the response of patients to treatments remained variable and difficult to predict.
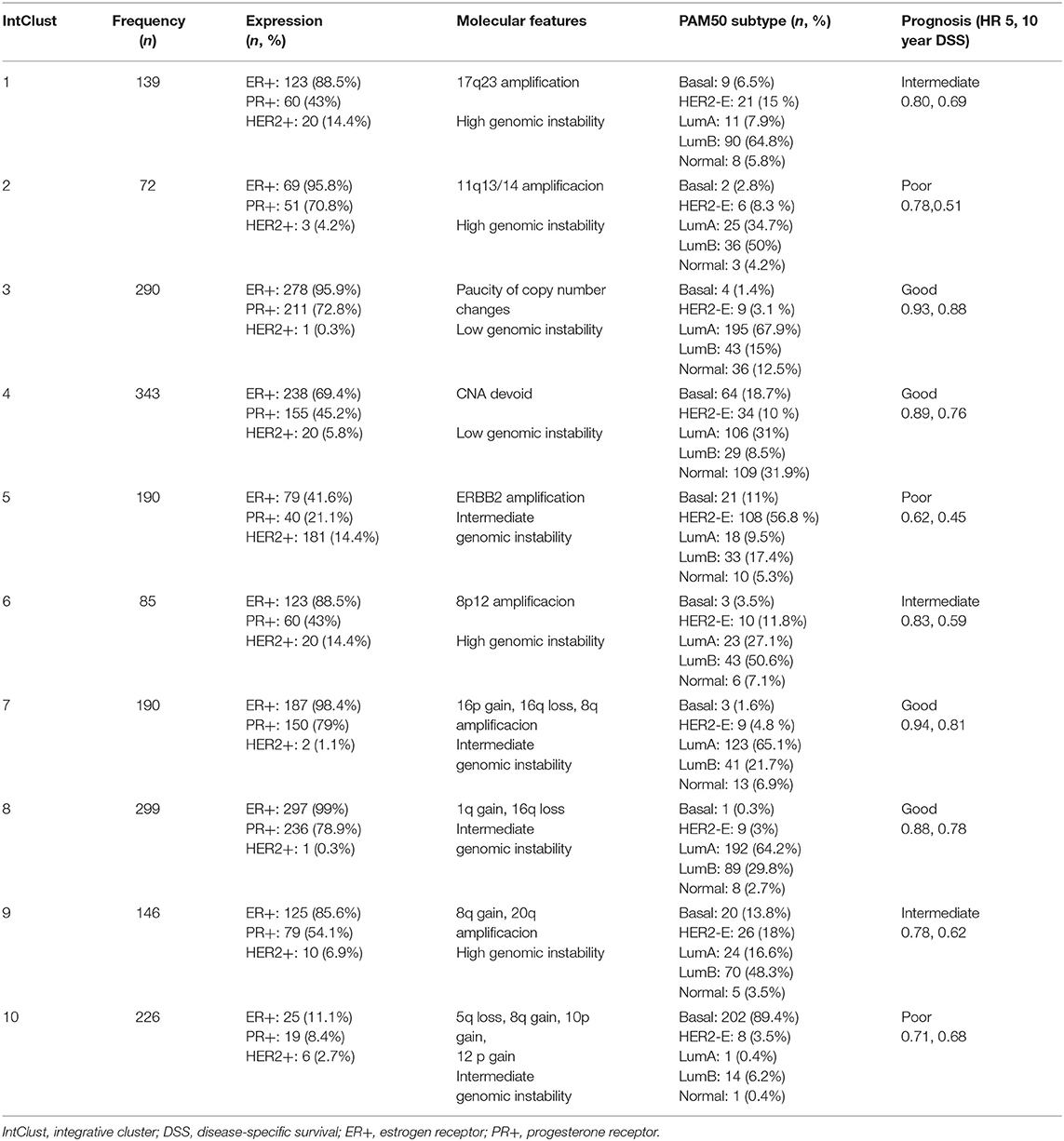
Table 2. Main features of the integrative clusters (48) in 2,000 breast tumors samples.
HER2-positive Breast Cancer and HER2-enriched Subtype
A clear example of complex heterogeneity, inter- and intratumoral, is the HER2+ BC. ERBB2/HER2 is an oncogene coding for a tyrosine kinase receptor that activates oncogenic pathways related with increase proliferation, angiogenesis and invasiveness, resulting in an highly aggressive neoplasm with poor outcomes that others BC (49, 50). The ERRBB2/HER2 gene is located in chromosomal region 17q12-21 and its amplification occurs in around 15–20% of breast cancers (10). Overexpression of the protein kinase receptor enables patients with HER2+ BC to benefit from antibody-based and anti-kinase based therapies that target this receptor, either with a combination of these targeted therapies and chemotherapy, or through dual anti-HER2 therapy without chemotherapy (51–69). This therapeutic approach, has completely changed the prognosis of HER2+ tumors.
So far, the HER2+ BC has been considered as a simple entity. Although the HER2 receptor itself has a dominant role, and the efficacy of the anti-HER2 agents support it, it is increasing the evidence that HER2 is a phenotype with one of the most extensive and specific heterogeneity (4–6, 70). HER2+ breast cancers vary clearly in their genome variations, gene expression programs, cell-of-origin and cell plasticity, what impact in their microenvironment, prognosis and therapeutic outcomes.
Immunohistochemistry Criteria: Past, Present, and Future
The HER2 status assessment was establishment by The American Society of Clinical Oncology and the College of American Pathologists (ASCO/CAP), with the publication of guidelines with recommendations for testing the level of HER2 protein overexpression by IHC and the HER2 gene amplification determined by ISH, both on FFPE breast tumor tissues. The first ASCO/CAP guideline was published in 2007 (71), and updated in 2013 (72, 73) and 2018 (4) (Table 3). In the last update, the experts refined some controversial criteria of the older guidelines and tried to systematize the testing algorithm for the unusual categories of HER2 ISH results (4) (Table 4). The results of these tests are graded semi-quantitatively as either 0 (negative), 1+ (negative), 2+ (equivocal) or 3+ (positive) by IHC, and classify as amplification (positive, Group 5), equivocal (Group 2,3,4) or negative (Group 1) by ISH. In all of these guidelines, when the HER2 status is negative by IHC and/or ISH, is not indicated the confirmation by an alternate assay. In contrast, the HER2 equivocal cases, by either HER2 IHC or HER2 ISH assays, must be analyzed with an secondary HER2 testing method, or on different tissue blocks with the same testing approach (4, 72). The answer about which of the two methods (IHC or ISH) is better for evaluating the HER2 status, continues to be unknown. Also, with the two latest updates, an important problem was added respecting the 2007 ASCO/CAP guidelines: more HER2 equivocal cases are diagnosed which an increase in reflex HER2 testing (74).
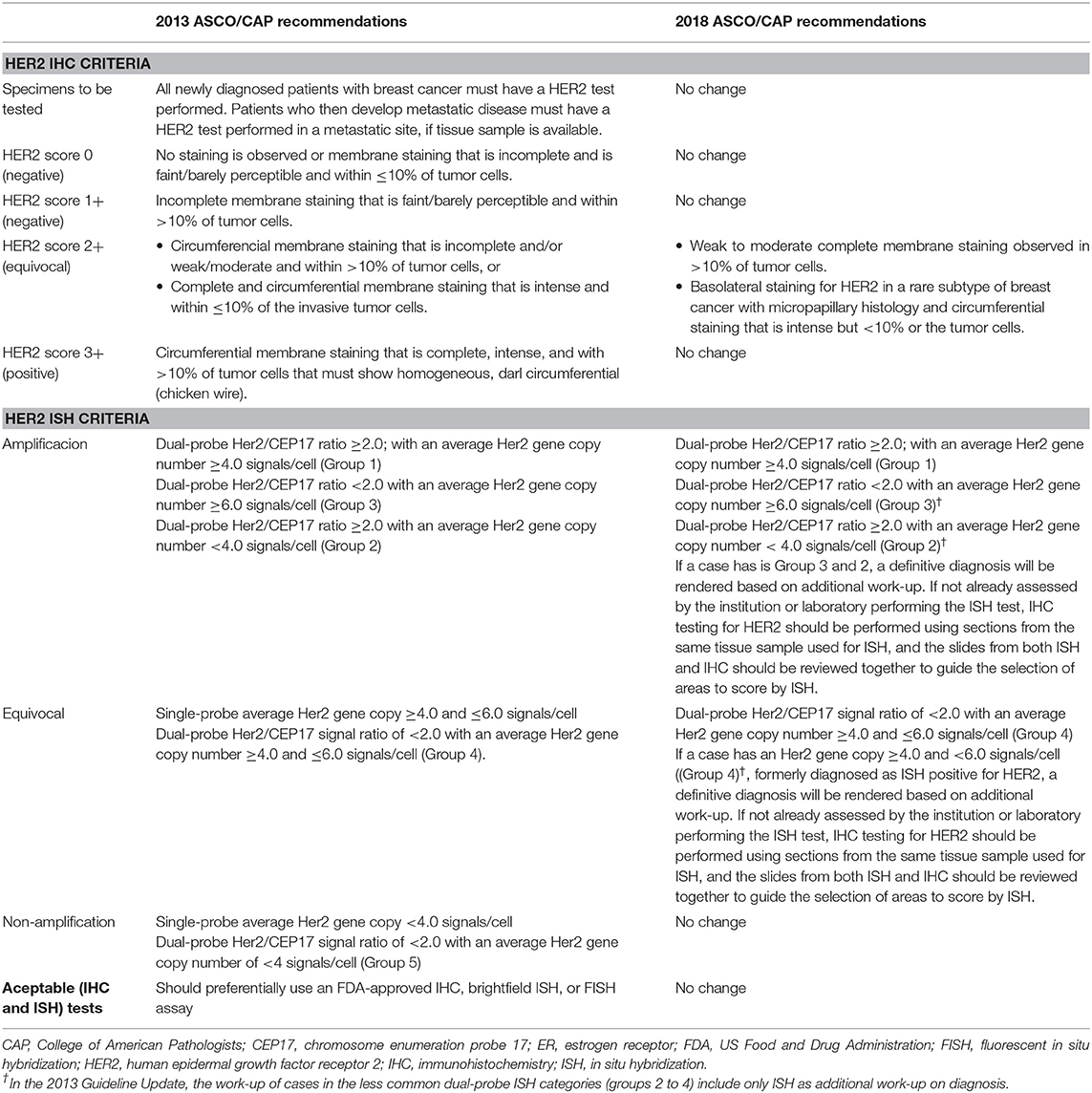
Table 3. 2018 ASCO/CAP summary recommendations [original recommendations and focused update recommendations (4)].
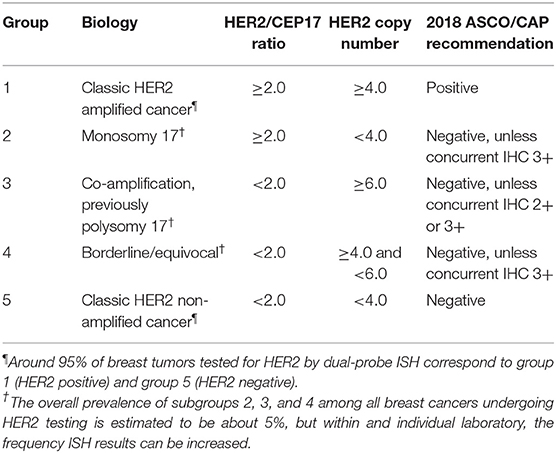
Table 4. Summary of test result scenarios and recommended final HER2 status (4).
The concordance between HER2 gene status and HER2 protein expression is generally high, even though discordance between IHC and ISH assay is not uncommon. Both methods detect biological different targets, HER2 protein and HER2 gene expression, respectively, and each assay has its own advantages and disadvantages. The main discordant results are caused by tumor heterogeneity (4, 75–79) focusing mainly in HER2-equivocal cases (4, 73, 76), being a critical factor in the accurate HER2 status evaluation. The ASCO/CAP 2013 guidelines defined heterogeneity as findings of between 5 and 50% of total cells with HER2/CEN17 ratio >2.0 or >6 Her2 signals/cells (72), and the ASCO/CAP 2018 update such as the presence of any aggregated population of amplified cells comprising >10% of the tumor cells on the slide (4). In the low-grade HER2 amplification cases (defined as HER2/CEN17 ratio between 2 and 4) a significant HER2 genetic heterogeneity is detected more frequently than breast cancers with a high-grade HER2 amplification (defined as HER2/CEN17 ratio ≥4.0) and HER2 protein overexpression (defined by IHC 3+) (48, 80). Thus, the evaluation of HER2 through IHC staining and gene amplification, can be remarkably heterogeneous and this could affect the selection of patients, the therapeutic response and the disease-free survival (DFS) rates (76, 81). With an incidence among the studies of 5–40% of HER2 intratumoral heterogeneity (ITH), it cannot be ignored.
HER2 IHC and HER2 ISH tests are employed to select patients for HER2-targeted therapy, and each assay have their advantages and weakness. With the object of improving the assessment of the individual HER2 ITH in tumor samples, Nitta and colleagues, elaborated and validated a protocol in FFPE xenograft tumor tissue sections and in FFPE BC tissue-microarray (TMA) slides, that allows simultaneous brightfield-microscopy detection of HER2 protein and HER2 gene expression, called first tricolor HER2 gene-protein assay (GPA) (82). This test exposed the heterogeneity of HER2 protein expression in different BC cells populations (82). A recent study with this assay reported relevant and clinical implications of this intra-heterogeneity (83). Through the combined assessment of HER2 gene amplification and HER2 protein status, five patterns were established. Three of them (type 3 to 5) were defined as a heterogeneous HER2 status and if the tumor case presented any of these types, it related to have ITH. Type 1 (homogeneous HER2 gene amplification and HER2 protein overexpression in all tumor cells) and type 2 (homogeneously amplified HER2 gene tumor cells, but without HER2 protein overexpression) were defined as homogenous HER2 status. The type 1 and type 2 were previously reported as “micro-heterogeneity” (42, 84, 85), what can only be detected by GPA. In the final analyses, the HER2 ITH was an independent factor associated with incomplete pathological response to anti-HER2 neoadjuvant chemotherapy in a cohort of 64 patients (83). Thus, a histopathological-level, a test that allows the recognition of discordance between HER2 gene amplification and protein expression simultaneously, could improve the clinical selection of patients for anti-HER2 therapies, due to a better accuracy of the HER2 IHT in the HER2+ BC.
Molecular Portraits
HER2+ BC has been historically divided in two distinct diseases based on the expression of hormonal receptors, while the gene expression analyses have proved that HER2+ BC is constituted of all the main intrinsic subtypes. In the HR+/HER2+ BC, two intrinsic subtypes are predominantly isolated: Luminal B and HER2-E (43). Within HR–/HER2+ tumors, around 50–88% have the HER2-E subtype, followed by other poor prognostic subtypes such as the luminal B or the basal-like subtype (41). The HER2-E subtype is defined by high expression of HER2-related and proliferation-related genes of the 17q amplicon (e.g., ERBB2/HER2 and GRB7), an average expression of luminal-related genes (e.g., ESR1, FGFR4, FOXA1, and PGR) and proteins, and by low or missing expression of basal-related genes and proteins (e.g., cytokeratins 5 and 6, OFXC19) (1, 5). At the DNA level, these tumors are characterized by the greatest number of mutations across the genome. About 70–75% and 40% of HER2-E tumors are TP53 and PIK3CA mutated, respectively (5, 6, 44) (Figure 2). Thus, any HER2+ BC can be included in the HER2-E, basal-like, or luminal molecular subtypes, and this affect significantly to their biological behavior and therapeutic outcomes. Conversely, the HER2-E subtype seems to capture some, but not all clinically HER2+ tumors, while HER2-E tumors can be identified within HER2-negative breast tumors, both in hormone receptor-positive or negative profiling (5, 6, 37, 44).
The concept of intrinsic subtypes has provided large insights into the heterogeneity of HER2+ disease. Prat et al. performed an analysis with data of TCGA (5) and METABRIC studies (36) with the purpose to evaluated how molecular subtypes and clinical HER2 status (defined by 2007 ASCO/CAP guidelines and/or DNA copy-number data) overlapped (44). HER2+ BC had a higher frequency of HER2-E subtype (47 vs. 7.1% in HER2-negative tumors), with a lower frequency of luminal A (10.7 vs. 39%) and basal-like subtypes (14.1 vs. 23.4%). Conversely, the ratio of HER2+ BC was 64.6% in HER2-E vs. 20, 14.4, and 7.3% in luminal B, basal-like and luminal A subtypes, respectively (44). Among HER2+ and HER2-negative BC, <5% genes were found to be expressed differently within each molecular subtype, and respect to the subtype, the genes significant up-regulated in HER2+ breast cancers, were found enriched for genes located in the 17q12 and 17q21 DNA amplicons. The HER2 gene expression and the expression of other 17q12 amplicon genes, were significantly upper in HER2+ tumors with HER2-E and basal-like intrinsic subtypes. Finally, after a clustering analysis of a METABRIC dataset of the most variable genes across the four subtypes, the results revealed that overall profile of them is largely maintained regardless of the clinical HER2 status, except for the HER2-E subtype (44). Thus, it seems that of gene expression the HER2+ BC of a given subtype is practically indistinguishable from a HER2-negative tumor with the identical subtype, except for the higher expression of genes in or close to the HER2 amplicon on 17q in the HER2+ tumors.
In the study about the ten integrative clusters previously described (48), ERBB2 amplified cancers joined in the integrative Cluster 5 (IntClust), unlike the classification of the intrinsic subtypes of Perou et al. (20), or with the analyses of Prat et al. (44). Several publications, have been compared the prognostic value of the 10 integrative clusters classification in front of the intrinsic subtypes, and the authors concluded that they do not confer supplementary information apart from the provided by the intrinsic subtype (44).
The TCGA dataset study also offers the opportunity to examine additional characteristics of the intrinsic subtype based on HER2 status (5). Through the analysis of protein expression, miRNA, DNA methylation and gene expression, slight molecular differences between HER2+ and HER2-negative tumors within each subtype were detected. The vast majority of proteins up-regulated in HER2+ BC derived again from genes located in the 17qDNA region. After the publication of the TCGA study, the last and distinctive study with a similar approach was published in July 2016 (6). The complex molecular heterogeneity within HER2+ disease was highlighted and explained for the first time by whole-sequencing genome (WGS) and transcriptome sequencing data from HER2+ BC samples (6). The authors selected a total of 289 HER2+ breast cancers with FFPE tissues identified within the French PHRE/SIGNAL programs (86, 87). An overall of 99 selected tumors were analyzed for genome-wide expression portraits, out of which 64 tumors and matched normal DNA were subjected to WGS. On the basis of gene-expression data in an unsupervised hierarchical cluster analysis, four groups were defined with specific genomic alterations (somatic mutations, copy-number changes, and structural alterations). Groups A and B encompassed most HR-positive tumors, and groups C and D mostly contained HR-negative tumors. Using the PAM50 assay to identify the intrinsic subtypes, the tumors were mainly luminal B (A and B groups) and HER2E (in C and D groups), with only a marginal number of luminal A and basal tumors (6). These groups displayed specific genomic alterations too. All samples in group D and none in group A displayed mutations in TP53, while only one sample in group D harbored a mutation in PIK3CA, with equal distribution of such mutations in the other groups. A similar gradient, was also observed in terms of genomic and cell of origin transcriptomic signatures (6, 88). Group D showed more genomic instability and a progenitor luminal signature. In contrast, group A was more stable and showed a typical mature luminal signature (88). These observations are concordant with the cell-of-origin scheme (88–91), in which the intra-tumoral heterogeneity reflects the developmental stage of the epithelial mammary cells. Thus, multiple phenotypes can emerge from one cell-of-origin depending on the initiating genetic event (91).
Thanks to WGS data the authors obtained information about the amplification process itself and about how and maybe when it is arising. The process was consistent with a breakage-fusion-bridge (BFB) folding mechanism, supported by the sequence of copy numbers and the orientation of clipped reads (88, 92). However, the present of long distance and inter-chromosomal rearrangements supported that the amplification is a complex phenomenon, probably comprising multiple amplicons on the same or different chromosomes and several interlaced mechanisms (88). All of this suggests that HER2 amplification, although probably strongly selected, is an embedded event that is superimposed on the standard time course of the breast carcinogenesis (88).
Another relevant article recently published, with genomic and transcriptome analysis too, concluded in a similar theory: HER2 could be defined as a pan-cancer phenomenon (93). The authors explored genomics data (RNA sequencing, expression and copy number changes) across three cohorts of patients [TGCA (5), METABRIC (36) consortium and the USO1062 phase III trial population (94)], with more than 3,000 breast tumors samples analyzed. PAM50 was employed for classifying the intrinsic subtypes. Their results were similar to the previously described: (i) the concordance between HER2 amplification and HER2-E subtype was really poorly (only 47% of HER2 amplify tumors presented this intrinsic subtype); (ii) it was find no evidence for cooperating copy number drivers with HER2 outside chromosome 17, and finally (iii) after the transcriptional profiling of the HER2-E subtype, the authors reported that HER2+ tumors are hormonally driven, either by ER in hormone receptor-positive and HER2-E BC, or by AR in hormone receptor-negative and HER2-E BC (93).
Clinical Implications
Trastuzumab was approved in 2001 for metastatic BC patients after the results reported by Slamon et al. (51), in a randomized clinical trial. In adjuvant setting, data from five randomized trials showed a significant improvement in DFS in women with early HER2+ BC after adjuvant treatment with an anti-HER2 antibody called trastuzumab. Latest updates confirmed a benefit sustained over time, resulting finally in a significant improvement in overall survival (OS). In the same way, the treatment with anti-HER2 therapy plus chemotherapy, improved the outcomes in OS in patients with metastatic disease, with numerous randomized clinical trials of anti-HER2 therapies published. To date, the level of expression of HR and HER2 status continue guiding the algorithm of treatment for the HER2+ BC in the clinical practice. Other pathological variables (tumor size, nodal status) provided independent prognostic information. However, if we take into consideration the intrinsic subtype that characterized the tumor, the impact of the clinical and pathological features its decreases considerably.
After the first clinical trial of a HER2-targeted therapy for BC (51), improving strategies to select patients candidate for these therapies has become a critical element to the successful development of anti-HER2 drugs. To date, this selection remaining based on the degree of HER2 positivity in the tumor, by IHC and/or ISH scores (50–53). None biomarker beyond HER2 itself has demonstrated clinical utility across the majority of randomized clinical trials published. Further, although patients with HER2+ disease obtain the greatest benefit from anti-HER2 treatment, the response is greatly heterogeneous, and a substantial proportion of patients present primary or secondary resistance.
The relationship between the grade of HER2 amplification or protein overexpression and the measure of benefit from the different anti-HER2 therapies, has been largely assessed in both early and metastatic disease studies. Available evidence supports a higher probability of success to these therapies in tumors with an increased HER2 protein expression or greater HER2 mRNA levels, although lower HER2 expression or mRNA levels have been associated with clinical benefit too (95–101). Several studies in the neoadjuvant context, have showed an association between rates of pathological complete response (pCR) and a higher HER2 amplification, increased HER2 mRNA levels or HER2 protein overexpression (99–101). In adjuvant studies, such association not impacted either DFS or OS. What's more, centralized laboratory analysis of HER2 testing in the NSABP-B31 (102) and NCCTG N9831 (103) adjuvant trastuzumab trials found a treatment benefit in women with HER2-negative tumors.
Respect to the expression of HR, in the neoadjuvant setting different trials has exhibited heterogeneous response rates after neoadjuvant with chemotherapy and anti-HER2 therapy between hormone receptor-positive and receptor-negative tumors, that is not limited to trastuzumab (10). Achieving a pCR seems to have a significant impact in patient outcomes, with the strongest correlation found in HER2+ BC without expression of hormonal receptors. However, the greatest benefit from anti-HER2 drugs in hormone receptor-negative breast cancers, has not been found in the 3-large adjuvant clinical trials evaluating 1-year of trastuzumab vs. placebo, and both groups seem to obtain similar benefits (103).
Another example that confirm the clinical impact of the HER2 heterogeneity is a phase II study led by the Danna-Farber Cancer Institute and recently presented at ASCO 2019 (104). In this clinical trial, the patients received neoadjuvant treatment with 6 cycles of T-DM1 plus pertuzumab. The authors assessment the heterogeneity in basal time (by baseline ultrasound-guide core biopsies from two distinct areas of each tumor), and this entity was defined as at least one of the six areas with either (1) HER2 positivity by ISH in more than 5% and <50% of tumor cells, or (2) a tumoral area with negative result for HER2. Among the 164 patients included, the heterogeneity in HER2 was identify in 10% of evaluable cases without any pCR among cases classified as heterogeneous, being the Residual Cancer Burden (RCB) III the pathological response more frequent in these patients. Secondary analysis also demonstrated a significant relation between pCR (or RCB-0) and HER2 3+ vs. HER2 2+ by IHC. The association between heterogeneity and pCR remained significant when adjusted by hormone receptor status and HER2 IHC measurement (104). These findings, as well as those previously described by Nitta et al. (83), confirm that the ITH is a distinct entity, more diverse than we could expect with the classic pathological evaluation. The heterogeneity in HER2+ BC exist and the treatment of these patients only with anti-HER2 therapies can be insufficient. This entity may need treated with chemotherapy plus anti-HER2 drugs and with novel treatment approaches.
Sensitivity to Anti-HER2 Based Chemotherapy
The impact of the intrinsic subtyping has been researched retrospectively, either trials evaluating anti-HER2 based chemotherapy in the neoadjuvant [i.e., NeoALTTO (105), CALGB-40601 (84), NOAH (42), CHER-LOB (85) and BERENICE (106)] and adjuvant [i.e., NSABP-B31 (107) and N9831 (108)] settings. Again, in all of these analyses the impact of the HR status, HER2 amplification or the HER2 expression at the protein or mRNA levels, fall into a second or third level such as predictive biomarkers with respect to the intrinsic subtype. In the neoadjuvant setting, when HER2+ BC were clasifficated by PAM50 molecular assay, HER2-E subtype was associated with a higher pCR rate (exceeding 50% in all trials) and DFS rates compared to non-HER2-E subtypes, following either trastuzumab plus chemotherapy treatment (42, 84, 85) or with dual HER2 blockade without chemotherapy.
Sensitivity to Dual HER2 Blockade-Only
Nowadays, an area with great interest for the oncologist community is to identify what patients might be treated with a regimen based on dual HER2 blockade without chemotherapy. It has been presented results of several neoadjuvant studies, which submit that a subgroup of patients with HER2+ BC are especially sensitive to the dual HER2 blockade, achieves pCR rates around 70%, so that could potentially be treated without chemotherapy (109).
The HER2-E breast tumors are driven by HER2/EGFR signaling, such as it showed, through a silico and omyc analyses, in the TCGA breast cancer project (5). So, this intrinsic subtype should benefit the most from anti-HER2 dual-blockade. The benefit achieved in HER-negative BC with HER2-E intrinsic subtype can be explained because these tumors preserve the higher expression of EGFR, with independence of expression degree of hormonal receptors (7). However, the greater response rate in the HER2-E subtype in previous studies could not distinguish anti-HER2 sensitivity vs. cytotoxic therapy-sensitivity. HER2-E subtype could be a predictor itself of anti-HER2 therapy benefit, and this theory should be validated in future randomized trials. If this happened, this intrinsic subtype could help to select a group of patients with HER2+ BC that might be cured with anti-HER2 drugs without chemotherapy, or patients with metastatic disease that can be treated with less intensive treatment, such as dual HER2 blockade-only.
Immune Infiltration
The tumor-infiltrating lymphocytes (TILs) are white bloodstream cells that migrate toward the tumor. In this heterogeneous group of cells, we have found several types of white cells, including T cells, B cells, and even Natural-Killer (NK) cells, although the T cells are the most representative. Overall, TILs comprising the majority of mononuclear immune infiltrates from the innate and adaptive immune response, with rates that depending of tumor type and stage. An important feature of these cells is that their functions changes dynamically, throughout tumor progression and in response to oncology treatments, being able to acquire dramatically opposite functions. The TILs represent pre-existing anti-tumor immunity, with prognostic relevance and predictive value in BC, especially for HER2+ and triple negative breast cancers (110, 111), although the BC has not classically been considered as an immunogenic neoplasm. In contrast to mucosal tissues, normal breast tissue contain limited aggregates of immune cells (112).
In HER2+ BC patients, the TILS are linked to favorable long-term prognosis and survival outcomes, both on early (110, 113–115) and metastatic disease (116). Within HER2+ BC, non-luminal subtypes have the highest levels of TILs, especially the HER2-E intrinsic subtype (7, 117). This has been associated with higher rates of pCR and better survival outcomes following chemotherapy and anti-HER2 neoadjuvant treatment (118), and with response to immunotherapy, as suggest the results from the PANACEA phase IB/II trial (119).
However, in multivariable models adjusted for PAM50 subtypes, TILs seems lost their significant association with better outcomes, due that the intrinsic subtype profiling appears encompasses the information provided by TILs (7). Thus, if immunotherapy aspires to obtain relevance in the treatment of BC, future trials should explore theses new therapies according to the intrinsic subtype, especially in the HER2+ BC.
Therapeutic Resistance
Different resistance mechanisms to anti-HER2 therapy have been described, which mostly favoring the reactivation of the HER2 pathway or its downstream signaling (109, 120). Most of the therapeutic failures in the treatment of HER2+ BC come from acquired resistance by sub-clones of cells that are highly selected by the therapeutic pressure. The real prevalence and clinical impact of these mechanisms remain largely unclear, majority of them involve genetic or epigenetic aberrations, and have been mainly described in relation to single HER2 blockade (120). Therefore, these mechanisms should clearly be reviewed, because antiHER2 combinations could select different alterations respect to single HER2 blockade.
Among the main mechanisms described we have (1) an incomplete blockade of the HER2 receptor with the activation of compensatory mechanisms by the HER2 receptors family; (2) the activation of alternative receptor tyrosine kinases (RTKs) or other membrane receptors outside of the HER2 family [such as insulin-like grow factor 1 receptor (IGF-1R), AXL Receptor Tyrosine Kinase (AXL) or MET (121)] and (3) the alterations in downstream signaling pathways, especially in the PI3K/AKT/mTOR axis. The hyperactivation of PI3K/AKT/mTOR pathway is the best characterized and seems to be the alteration most important to initiate and perpetuate the resistance to anti-HER2 therapies in HER2+ tumors with any degree of the hormone receptor expression (120). Activating mutations in PIK3CA (122) or reduced levels of tumor suppressor genes (mutations or loss of PTEN, and loss of INPP4-B, among others) are the main molecular alterations than maintain this hyperactivation. The role of targeting these pathways has been evaluated in numerous randomized clinical trials. Among these trials, we have the BOLERO-1 and BOLERO-3, both evaluating the role of everolimus, an mTOR inhibitor, in combination with trastuzumab plus paclitaxel as first-line treatment (BOLERO-1) (63) or in combination with trastuzumab and vinorelbine in trastuzumab-resistant advanced HER2-positive BC (BOLERO 3) (123). The results of them were disappointing and the increase in toxicity very significant. The most relevant data of both studies comes from the combined biomarker analyses that reported an improvement in PFS for patients that harboring PIK3CA mutations or PTEN loss and were treated with everolimus (124). Current efforts have focused on evaluating the activity, in combination with anti-HER2 treatment, of pan-PI3K and alpha-specific PI3K inhibitors. Until now the alpha-specific PI3K inhibitors are the drugs with the most promising therapeutic results and less incidence of serious toxicities respects the pan-PI3K inhibitors (125). These drugs, such as alpelisib (BYL719) (126), target the PI3K-alfa protein, the most frequently altered PI3K isoform in solid tumors and breast cancers, encoded by the PIK3CA gene and with a prominent role in PI3K signaling.
Another relevant mechanism recently proposed is related to the activity of the cyclinD1-cyclin-dependent kinase 4/6 (CDK 4/6) axis. Their enhanced activation can be driven by cyclin D1/CDK4 overexpression or CD4 mutations, causing resistance to hormonal treatment in hormone receptor-positive breast cancers (127). We already have preclinical evidence (128, 129) and from controlled trials (130) about the role of Cyclin D1-CDK 4/6 axis in the anti-HER2 resistance. Using transgenic mouse models, Goel et al. (128) showed that the suppression of CDK4 activity reduces TSC2 (tuberin) phosphorylation, with a partial suppression of mTORC1 and, hence p70-S6K activity, which relieve feedback inhibition of EGFR family kinases rendering cells more sensitive to the effects of EGFR/HER2 inhibitors and overcome acquired resistance to anti-HER2 treatment. The chemotherapy-free trastuzumab-pertuzumab-palbociclib-fulvestrant combination tested in neoadjuvant setting, has recently exhibited promising activity in terms of reduction of ki67 and rate of pCR for breast tumors with positivity of HER2+ and hormonal receptors (130). So, the combination of CKD4/6 and HER2 inhibitors could be a valid option to chemotherapy-containing regimens, at least in a subgroup of patients.
So, hypothetically, the vast majority of resistance mechanisms described could be targeted by drugs that are already available, such as inhibitors of ER, cyclins, mTOR or FGFR1 (109, 120). However, the potential therapeutic advantage of combining these drugs with standard anti-HER2 therapy should be weighed against the potential risk of serious toxicities. Moreover, as a result of intra- and inter-tumoral heterogeneity, different mechanisms can co-exist in a same patient, keeping the potential possibility to contemporaneously target all resistant tumor clones. The HER2-E is the second intrinsic subtype, after the luminal A, with greater percentage of aberrations in PI3K/AKT/mTOR axis (by PI3KCA mutations/loss of PTEN) and alterations in RB1 pathway (by Cyclin D1 amplification and/or CDK4 gain). So, if both axis are implicated in the resistance of anti-HER2 treatment, this intrinsic subtype could be the most appropriated to design future clinical trials that testing the role of targeting all pathways simultaneously and to prevent of development of acquired resistances, independently of pathological evaluation of HER2, which does not seem to adequately measure the ITH, an entity already established as other potential resistance mechanism.
Conclusion
To date, amplification and/or overexpression of HER2 remains the only biomarker regarding treatment decisions with anti-HER2 drugs, but it is insufficient itself to clarify the heterogeneous therapeutic outcomes. The complex heterogeneity of the HER2+ BC is a critical aspect, as it has been described at multiple levels: intra-tumoral, at gene expression, transcriptomic and genomic levels. The HER2+ BC do not represent a subtype itself, but are instead dispersed along the whole breast cancer spectrum, from hormone receptor-positive luminal to hormone receptor-negative basal phenotype, with genome variations accordingly to these phenotypes and incidentally defined by a specific gene amplification. Perhaps, combining phenotypic (i.e., gene expression groups) and mechanistic (i.e., co-amplifications) characteristics, may improve the actual classification of HER2+ BC, with the identification of more homogeneous subgroups and improving the knowledge of the genetic mechanisms implicated in the heterogeneity of this disease. This could lead to rational therapeutic strategies, exploring additional pathways and genes co-amplified with ERBB2, especially relevant for patients who show an initial weak response or that exhibit treatment resistance, patients with a particularly poor prognosis.
Although HER2 amplification is traditionally associated with HER2-E transcriptional subtype, these are substantially distinct. HER2 amplification seems an oncogenic driver present in all subtypes in place of a biomarker itself of an intrinsic subtype, and its strong enrichment in the HER2-E subtype has masked the nature of this entity. Taking into consideration only the intrinsic subtype, any prognostic value attributable to clinical and pathological variables such as the degree, ER/PR or HER2 status by IHC and/or ISH, disappears, as happens with the amplification of HER2 isolated taken as a predictive factor itself.
We already have data of efficacy for anti-HER2 therapy in patients with HER2-negative tumors, with a considerable proportion of patients with HER2+ breast cancers not achieving such clinical benefit. Overall, the evidence so far suggests that all BC with HER2-E intrinsic subtype benefit from anti-HER2 treatment. Although much remains to be done, with the data available and presented in this review, it seems that the HER2-E intrinsic subtype would be a more appropriate biomarker to assess the real benefit of anti-HER2 treatment in all phenotypes of BC.
Respecting the actual HER2+ BC therapeutic setting, the most recent studies try to improve the results of patients adding new anti-HER2 drugs, still without a selection by molecular features, thus, achieving a discrete therapeutic benefit in the most of these trials, and increasing toxicity and costs. The intrinsic molecular subtyping of BC fairly has extended our knowledge about the behavior of this tumor and should have an established place in the clinical practice. After this revision, we would like to conclude that the HER2-E subtype should be established itself as the best predictor of prognosis and clinical outcomes of the BC with this intrinsic subtype, what would allow for the extension of the use of anti-HER2 drugs for HER2-negative tumors and to improve the selection of patients with HER2+ BC for combination of anti-HER2 therapies.
Author Contributions
AG-O: authorship and complete writing of the manuscript. AS-M and AR: general review of the manuscript and contribution of bibliography. MC: help with the interpretation of molecular data. MP: contribution and bibliographical guidance and has helped too with the interpretation of molecular data. NE: contribution and bibliographical guidance. EC: general review, partial correction of the manuscript, and contribution of bibliography. All authors read and approved the final manuscript.
Conflict of Interest
The authors declare that the research was conducted in the absence of any commercial or financial relationships that could be construed as a potential conflict of interest.
References
1. Cejalvo JM, de Dueñas EM, Galván P, García-Recio S, Gasión OB, Paré L, et al. Intrinsic subtypes and gene expression profiles in primary and metastatic breast cancer. Cancer Res. (2017) 77:2213–21. doi: 10.1158/0008-5472.CAN-16-2717
2. Sánchez-Muñoz A, Vicioso L, Santonja A, Álvarez M, Plata-Fernández Y, Miramón J, et al. Male breast cancer: correlation between immunohistochemical subtyping and PAM50 intrinsic subtypes, and the subsequent clinical outcomes. Mod Pathol. (2018) 31:299–306. doi: 10.1038/modpathol.2017.129
3. Harbeck N. Insights into biology of luminal HER2 vs. enriched HER2 subtypes: therapeutic implications. Breast. (2015) 24:S44–8. doi: 10.1016/j.breast.2015.07.011
4. Wolff AC, Hammond MEH, Allison KH, Harvey BE, Mangu PB, Bartlett JMS, et al. Human epidermal growth factor receptor 2 testing in breast cancer: American Society of Clinical Oncology/College of American Pathologists Clinical Practice Guideline Focused Update. J Clin Oncol. (2018) 36:2105–22. doi: 10.1200/JCO.2018.77.8738
5. Cancer Genome Atlas Network. Comprehensive molecular portraits of human breast tumours. Nature. (2012) 490:61–70. doi: 10.1038/nature11412
6. Ferrari A, Vincent-Salomon A, Pivot X, Sertier A-S, Thomas E, Tonon L, et al. A whole-genome sequence and transcriptome perspective on HER2-positive breast cancers. Nat Commun. (2016) 7:12222. doi: 10.1038/ncomms12222
7. Cejalvo JM, Pascual T, Fernández-Martínez A, Brasó-Maristany F, Gomis RR, Perou CM, et al. Clinical implications of the non-luminal intrinsic subtypes in hormone receptor-positive breast cancer. Cancer Treat Rev. (2018) 67:63–70. doi: 10.1016/j.ctrv.2018.04.015
8. Giuliano AE, Edge SB, Hortobagyi GN. Eighth edition of the AJCC cancer staging manual: breast cancer. Ann Surg Oncol. (2018) 25:1783–5. doi: 10.1245/s10434-018-6486-6
9. Elston CW, Ellis IO, Pinder SE. Pathological prognostic factors in breast cancer. Crit Rev Oncol Hematol. (1999) 31:209–23. doi: 10.1016/S1040-8428(99)00034-7
10. Loibl S, Gianni L. HER2-positive breast cancer. Lancet. (2017) 389:2415–29. doi: 10.1016/S0140-6736(16)32417-5
11. Lakhani SR, Ellis IO, Schnitt SJ, Tan PH, van de Vijver MJ. WHO Classification of Tumours of the Breast. Available online at: http://publications.iarc.fr/Book-And-Report-Series/Who-Iarc-Classification-Of-Tumours/WHO-Classification-Of-Tumours-Of-The-Breast-2012 (accessed March 14, 2019).
12. Weigelt B, Geyer FC, Reis-Filho JS. Histological types of breast cancer: how special are they? Mol Oncol. (2010) 4:192–208. doi: 10.1016/j.molonc.2010.04.004
13. Harris L, Fritsche H, Mennel R, Norton L, Ravdin P, Taube S, et al. American Society of Clinical Oncology 2007 update of recommendations for the use of tumor markers in breast cancer. J Clin Oncol. (2007) 25:5287–312. doi: 10.1200/JCO.2007.14.2364
14. Rakha EA, Reis-Filho JS, Baehner F, Dabbs DJ, Decker T, Eusebi V, et al. Breast cancer prognostic classification in the molecular era: the role of histological grade. Breast Cancer Res. (2010) 12:207. doi: 10.1186/bcr2607
15. Hammond MEH, Hayes DF, Dowsett M, Allred DC, Hagerty KL, Badve S, et al. American Society of Clinical Oncology/College of American Pathologists Guideline Recommendations for immunohistochemical testing of estrogen and progesterone receptors in breast cancer. J Clin Oncol. (2010) 134:e48–72. doi: 10.1200/JOP.777003
16. de Azambuja E, Cardoso F, de Castro G, Colozza M, Mano MS, Durbecq V, et al. Ki-67 as prognostic marker in early breast cancer: a meta-analysis of published studies involving 12,155 patients. Br J Cancer. (2007) 96:1504–13. doi: 10.1038/sj.bjc.6603756
17. Wirapati P, Sotiriou C, Kunkel S, Farmer P, Pradervand S, Haibe-Kains B, et al. Meta-analysis of gene expression profiles in breast cancer: toward a unified understanding of breast cancer subtyping and prognosis signatures. Breast Cancer Res. (2008) 10:R65. doi: 10.1186/bcr2124
18. Turashvili G, Brogi E. Tumor heterogeneity in breast cancer. Front Med. (2017) 4:227. doi: 10.3389/fmed.2017.00227
19. Yerushalmi R, Woods R, Ravdin PM, Hayes MM, Gelmon KA. Ki67 in breast cancer: prognostic and predictive potential. Lancet Oncol. (2010) 11:174–83. doi: 10.1016/S1470-2045(09)70262-1
20. Perou CM, Sørlie T, Eisen MB, van de Rijn M, Jeffrey SS, Rees CA, et al. Molecular portraits of human breast tumours. Nature. (2000) 406:747–52. doi: 10.1038/35021093
21. Sørlie T, Perou CM, Tibshirani R, Aas T, Geisler S, Johnsen H, et al. Gene expression patterns of breast carcinomas distinguish tumor subclasses with clinical implications. Proc Natl Acad Sci USA. (2001) 98:10869–74. doi: 10.1073/pnas.191367098
22. Sorlie T, Tibshirani R, Parker J, Hastie T, Marron JS, Nobel A, et al. Repeated observation of breast tumor subtypes in independent gene expression data sets. Proc Natl Acad Sci USA. (2003) 100:8418–23. doi: 10.1073/pnas.0932692100
23. Carey LA, Perou CM, Livasy CA, Dressler LG, Cowan D, Conway K, et al. Race, breast cancer subtypes, and survival in the Carolina Breast Cancer Study. JAMA. (2006) 295:2492–502. doi: 10.1001/jama.295.21.2492
24. Rouzier R, Perou CM, Symmans WF, Ibrahim N, Cristofanilli M, Anderson K, et al. Breast cancer molecular subtypes respond differently to preoperative chemotherapy. Clin Cancer Res. (2005) 11:5678–85. doi: 10.1158/1078-0432.CCR-04-2421
25. Parker JS, Mullins M, Cheang MCU, Leung S, Voduc D, Vickery T, et al. Supervised risk predictor of breast cancer based on intrinsic subtypes. J Clin Oncol. (2009) 27:1160–7. doi: 10.1200/JCO.2008.18.1370
26. Hugh J, Hanson J, Cheang MCU, Nielsen TO, Perou CM, Dumontet C, et al. Breast cancer subtypes and response to docetaxel in node-positive breast cancer: use of an immunohistochemical definition in the BCIRG 001 trial. J Clin Oncol. (2009) 27:1168–76. doi: 10.1200/JCO.2008.18.1024
27. Prat A, Parker JS, Karginova O, Fan C, Livasy C, Herschkowitz JI, et al. Phenotypic and molecular characterization of the claudin-low intrinsic subtype of breast cancer. Breast Cancer Res. (2010) 12:R68. doi: 10.1186/bcr2635
28. Nielsen TO, Parker JS, Leung S, Voduc D, Ebbert M, Vickery T, et al. A comparison of PAM50 intrinsic subtyping with immunohistochemistry and clinical prognostic factors in tamoxifen-treated estrogen receptor-positive breast cancer. Clin Cancer Res. (2010) 16:5222–32. doi: 10.1158/1078-0432.CCR-10-1282
29. Prat A, Ellis MJ, Perou CM. Practical implications of gene-expression-based assays for breast oncologists. Nat Rev Clin Oncol. (2011) 9:48–57. doi: 10.1038/nrclinonc.2011.178
30. Cheang MCU, Voduc KD, Tu D, Jiang S, Leung S K, Chia S, et al. Responsiveness of intrinsic subtypes to adjuvant anthracycline substitution in the NCIC.CTG MA.5 randomized trial. Clin Cancer Res. (2012) 18:2402–12. doi: 10.1158/1078-0432.CCR-11-2956
31. Chia SK, Bramwell VH, Tu D, Shepherd LE, Jiang S, Vickery T, et al. A 50-gene intrinsic subtype classifier for prognosis and prediction of benefit from adjuvant tamoxifen. Clin Cancer Res. (2012) 18:4465–72. doi: 10.1158/1078-0432.CCR-12-0286
32. Esserman LJ, Berry DA, Cheang MCU, Yau C, Perou CM, Carey L, et al. Chemotherapy response and recurrence-free survival in neoadjuvant breast cancer depends on biomarker profiles: results from the I-SPY 1 TRIAL (CALGB 150007/150012; ACRIN 6657). Breast Cancer Res Treat. (2012) 132:1049–62. doi: 10.1007/s10549-011-1895-2
33. Prat A, Cheang MCU, Martín M, Parker JS, Carrasco E, Caballero R, et al. Prognostic significance of progesterone receptor-positive tumor cells within immunohistochemically defined luminal A breast cancer. J Clin Oncol. (2013) 31:203–9. doi: 10.1200/JCO.2012.43.4134
34. Ellis MJ, Suman VJ, Hoog J, Lin L, Snider J, Prat A, et al. Randomized phase II neoadjuvant comparison between letrozole, anastrozole, and exemestane for postmenopausal women with estrogen receptor-rich stage 2 to 3 breast cancer: clinical and biomarker outcomes and predictive value of the baseline PAM50-based intrinsic subtype–ACOSOG Z1031. J Clin Oncol. (2011) 29:2342–9. doi: 10.1200/JCO.2010.31.6950
35. Dowsett M, Sestak I, Lopez-Knowles E, Sidhu K, Dunbier AK, Cowens JW, et al. Comparison of PAM50 risk of recurrence score with oncotype DX and IHC4 for predicting risk of distant recurrence after endocrine therapy. J Clin Oncol. (2013) 31:2783–90. doi: 10.1200/JCO.2012.46.1558
36. Curtis C, Shah SP, Chin S-F, Turashvili G, Rueda OM, Dunning MJ, et al. The genomic and transcriptomic architecture of 2,000 breast tumours reveals novel subgroups. Nature. (2012) 486:346–52. doi: 10.1038/nature10983
37. Lesurf R, Griffith OL, Griffith M, Hundal J, Trani L, Watson MA, et al. Genomic characterization of HER2-positive breast cancer and response to neoadjuvant trastuzumab and chemotherapy-results from the ACOSOG Z1041 (Alliance) trial. Ann Oncol. (2017) 28:1070–7. doi: 10.1093/annonc/mdx048
38. Bastien RRL, Rodríguez-Lescure Á, Ebbert MTW, Prat A, Munárriz B, Rowe L, et al. PAM50 breast cancer subtyping by RT-qPCR and concordance with standard clinical molecular markers. BMC Med Genomics. (2012) 5:44. doi: 10.1186/1755-8794-5-44
39. Carey LA, Berry DA, Ollila D, Harris L, Krop IE, Weckstein D, et al. Clinical and translational results of CALGB 40601: a neoadjuvant phase III trial of weekly paclitaxel and trastuzumab with or without lapatinib for HER2-positive breast cancer. JCO. (2013) 31:500. doi: 10.1200/jco.2013.31.15_suppl.500
40. Gnant M, Filipits M, Greil R, Stoeger H, Rudas M, Bago-Horvath Z, et al. Predicting distant recurrence in receptor-positive breast cancer patients with limited clinicopathological risk: using the PAM50 Risk of Recurrence score in 1478 postmenopausal patients of the ABCSG-8 trial treated with adjuvant endocrine therapy alone. Ann Oncol. (2014) 25:339–45. doi: 10.1093/annonc/mdt494
41. Prat A, Perou CM. Deconstructing the molecular portraits of breast cancer. Mol Oncol. (2011) 5:5–23. doi: 10.1016/j.molonc.2010.11.003
42. Prat A, Bianchini G, Thomas M, Belousov A, Cheang MCU, Koehler A, et al. Research-based PAM50 subtype predictor identifies higher responses and improved survival outcomes in HER2-positive breast cancer in the NOAH study. Clin Cancer Res. (2014) 20:511–21. doi: 10.1158/1078-0432.CCR-13-0239
43. Prat A, Pineda E, Adamo B, Galván P, Fernández A, Gaba L, et al. Clinical implications of the intrinsic molecular subtypes of breast cancer. Breast. (2015) 24:S26–35. doi: 10.1016/j.breast.2015.07.008
44. Prat A, Carey LA, Adamo B, Vidal M, Tabernero J, Cortés J, et al. Molecular features and survival outcomes of the intrinsic subtypes within HER2-positive breast cancer. J Natl Cancer Inst. (2014) 106: dju152. doi: 10.1093/jnci/dju152
45. Banerji S, Cibulskis K, Rangel-Escareno C, Brown KK, Carter SL, Frederick AM, et al. Sequence analysis of mutations and translocations across breast cancer subtypes. Nature. (2012) 486:405–9. doi: 10.1038/nature11154
46. Stephens PJ, Tarpey PS, Davies H, Van Loo P, Greenman C, Wedge DC, et al. The landscape of cancer genes and mutational processes in breast cancer. Nature. (2012) 486:400–4. doi: 10.1038/nature11017
47. Pascual T, Martin M, Fernández-Martínez A, Paré L, Alba E, Rodríguez-Lescure Á, et al. A pathology-based combined model to identify PAM50 non-luminal intrinsic disease in hormone receptor-positive HER2-negative breast cancer. Front Oncol. (2019) 9:303. doi: 10.3389/fonc.2019.00303
48. Dawson S-J, Rueda OM, Aparicio S, Caldas C. A new genome-driven integrated classification of breast cancer and its implications. EMBO J. (2013) 32:617–28. doi: 10.1038/emboj.2013.19
49. Baselga J. Why the epidermal growth factor receptor? The rationale for cancer therapy. Oncologist. (2002) 7(Suppl. 4):2–8. doi: 10.1634/theoncologist.7-suppl_4-2
50. Slamon DJ, Clark GM, Wong SG, Levin WJ, Ullrich A, McGuire WL. Human breast cancer: correlation of relapse and survival with amplification of the HER-2/neu oncogene. Science. (1987) 235:177–82. doi: 10.1126/science.3798106
51. Slamon DJ, Leyland-Jones B, Shak S, Fuchs H, Paton V, Bajamonde A, et al. Use of chemotherapy plus a monoclonal antibody against HER2 for metastatic breast cancer that overexpresses HER2. N Engl J Med. (2001) 344:783–92. doi: 10.1056/NEJM200103153441101
52. Paik S, Bryant J, Tan-Chiu E, Romond E, Hiller W, Park K, et al. Real-world performance of HER2 testing–National Surgical Adjuvant Breast and Bowel Project experience. J Natl Cancer Inst. (2002) 94:852–4. doi: 10.1093/jnci/94.11.852
53. Piccart-Gebhart MJ, Procter M, Leyland-Jones B, Goldhirsch A, Untch M, Smith I, et al. Trastuzumab after adjuvant chemotherapy in HER2-positive breast cancer. N Engl J Med. (2005) 353:1659–72. doi: 10.1056/NEJMoa052306
54. Burstein HJ, Harris LN, Marcom PK, Lambert-Falls R, Havlin K, Overmoyer B, et al. Trastuzumab and vinorelbine as first-line therapy for HER2-overexpressing metastatic breast cancer: multicenter phase II trial with clinical outcomes, analysis of serum tumor markers as predictive factors, and cardiac surveillance algorithm. J Clin Oncol. (2003) 21:2889–95. doi: 10.1200/JCO.2003.02.018
55. Joensuu H, Kellokumpu-Lehtinen P-L, Bono P, Alanko T, Kataja V, Asola R, et al. Adjuvant docetaxel or vinorelbine with or without trastuzumab for breast cancer. N Engl J Med. (2006) 354:809–20. doi: 10.1056/NEJMoa053028
56. Dennis S, Wolfgang E, Nicholas R, Tadeusz P, Miguel M, Michael P, et al. Adjuvant trastuzumab in HER2-positive breast cancer. N Engl J Med. (2011) 365:1273–83. doi: 10.1056/NEJMoa0910383
57. Baselga J, Cortés J, Kim S-B, Im S-A, Hegg R, Im Y-H, et al. Pertuzumab plus trastuzumab plus docetaxel for metastatic breast cancer. N Engl J Med. (2012) 366:109–19. doi: 10.1056/NEJMoa1113216
58. Cameron D, Casey M, Oliva C, Newstat B, Imwalle B, Geyer CE. Lapatinib plus capecitabine in women with HER-2-positive advanced breast cancer: final survival analysis of a phase III randomized trial. Oncologist. (2010) 15:924–34. doi: 10.1634/theoncologist.2009-0181
59. Andersson M, Lidbrink E, Bjerre K, Wist E, Enevoldsen K, Jensen AB, et al. Phase III randomized study comparing docetaxel plus trastuzumab with vinorelbine plus trastuzumab as first-line therapy of metastatic or locally advanced human epidermal growth factor receptor 2-positive breast cancer: the HERNATA study. J Clin Oncol. (2011) 29:264–71. doi: 10.1200/JCO.2010.30.8213
60. Gianni L, Pienkowski T, Im Y-H, Tseng L-M, Liu M-C, Lluch A, et al. 5-year analysis of neoadjuvant pertuzumab and trastuzumab in patients with locally advanced, inflammatory, or early-stage HER2-positive breast cancer (NeoSphere): a multicentre, open-label, phase 2 randomised trial. Lancet Oncol. (2016) 17:791–800. doi: 10.1016/S1470-2045(16)00163-7
61. Rimawi MF, Mayer IA, Forero A, Nanda R, Goetz MP, Rodriguez AA, et al. Multicenter phase II study of neoadjuvant lapatinib and trastuzumab with hormonal therapy and without chemotherapy in patients with human epidermal growth factor receptor 2-overexpressing breast cancer: TBCRC 006. J Clin Oncol. (2013) 31:1726–31. doi: 10.1200/JCO.2012.44.8027
62. Perez EA, Romond EH, Suman VJ, Jeong J-H, Sledge G, Geyer CE, et al. Trastuzumab plus adjuvant chemotherapy for human epidermal growth factor receptor 2-positive breast cancer: planned joint analysis of overall survival from NSABP B-31 and NCCTG N9831. J Clin Oncol. (2014) 32:3744–52. doi: 10.1200/JCO.2014.55.5730
63. Hurvitz SA, Andre F, Jiang Z, Shao Z, Mano MS, Neciosup SP, et al. Combination of everolimus with trastuzumab plus paclitaxel as first-line treatment for patients with HER2-positive advanced breast cancer (BOLERO-1): a phase 3, randomised, double-blind, multicentre trial. Lancet Oncol. (2015) 16:816–29. doi: 10.1016/S1470-2045(15)00051-0
64. Gelmon KA, Boyle FM, Kaufman B, Huntsman DG, Manikhas A, Di Leo A, et al. Lapatinib or trastuzumab plus taxane therapy for human epidermal growth factor receptor 2-positive advanced breast cancer: final results of NCIC CTG MA.31. J Clin Oncol. (2015) 33:1574–83. doi: 10.1200/JCO.2014.56.9590
65. Verma S, Miles D, Gianni L, Krop IE, Welslau M, Baselga J, et al. Trastuzumab emtansine for HER2-positive advanced breast cancer. N Engl J Med. (2012) 367:1783–91. doi: 10.1056/NEJMoa1209124
66. Perez EA, Suman VJ, Davidson NE, Gralow JR, Kaufman PA, Visscher DW, et al. Sequential versus concurrent trastuzumab in adjuvant chemotherapy for breast cancer. J Clin Oncol. (2011) 29:4491–7. doi: 10.1200/JCO.2011.36.7045
67. Tolaney SM, Barry WT, Dang CT, Yardley DA, Moy B, Marcom PK, et al. Adjuvant paclitaxel and trastuzumab for node-negative, HER2-positive breast cancer. N Engl J Med. (2015) 372:134–41. doi: 10.1056/NEJMoa1406281
68. Prat A, Baselga J. Dual human epidermal growth factor receptor 2 (HER2) blockade and hormonal therapy for the treatment of primary HER2-positive breast cancer: one more step toward chemotherapy-free therapy. J Clin Oncol. (2013) 31:1703–6. doi: 10.1200/JCO.2012.48.4998
69. Prat A, Baselga J. The role of hormonal therapy in the management of hormonal-receptor-positive breast cancer with co-expression of HER2. Nat Clin Pract Oncol. (2008) 5:531–42. doi: 10.1038/ncponc1179
70. Montemurro F, Di Cosimo S, Arpino G. Human epidermal growth factor receptor 2 (HER2)-positive and hormone receptor-positive breast cancer: new insights into molecular interactions and clinical implications. Ann Oncol. (2013) 24:2715–24. doi: 10.1093/annonc/mdt287
71. Wolff AC, Hammond MEH, Schwartz JN, Hagerty KL, Allred DC, Cote RJ, et al. American Society of Clinical Oncology/College of American Pathologists guideline recommendations for human epidermal growth factor receptor 2 testing in breast cancer. J Clin Oncol. (2006) 25:118–45. doi: 10.1200/JCO.2006.09.2775
72. Wolff AC, Hammond MEH, Hicks DG, Dowsett M, McShane LM, Allison KH, et al. Recommendations for human epidermal growth factor receptor 2 testing in breast cancer: American Society of Clinical Oncology/College of American Pathologists clinical practice guideline update. J Clin Oncol. (2013) 31:3997–4013. doi: 10.1200/JCO.2013.50.9984
73. Bethune GC, Veldhuijzen van Zanten D, MacIntosh RF, Rayson D, Younis T, Thompson K, et al. Impact of the 2013 American Society of Clinical Oncology/College of American Pathologists guideline recommendations for human epidermal growth factor receptor 2 (HER2) testing of invasive breast carcinoma: a focus on tumours assessed as “equivocal” for HER2 gene amplification by fluorescence in-situ hybridization. Histopathology. (2015) 67:880–7. doi: 10.1111/his.12723
74. Varga Z, Noske A. Impact of modified 2013 ASCO/CAP guidelines on HER2 testing in breast cancer. One year experience. PLoS ONE. (2015) 10:e0140652. doi: 10.1371/journal.pone.0140652
75. Nitta H, Kelly BD, Allred C, Jewell S, Banks P, Dennis E, et al. The assessment of HER2 status in breast cancer: the past, the present, and the future. Pathol Int. (2016) 66:313–24. doi: 10.1111/pin.12407
76. Seol H, Lee HJ, Choi Y, Lee HE, Kim YJ, Kim JH, et al. Intratumoral heterogeneity of HER2 gene amplification in breast cancer: its clinicopathological significance. Mod Pathol. (2012) 25:938–48. doi: 10.1038/modpathol.2012.36
77. Hou Y, Nitta H, Li Z. HER2 gene protein assay is useful to determine HER2 status and evaluate HER2 heterogeneity in HER2 equivocal breast cancer. Am J Clin Pathol. (2017) 147:89–95. doi: 10.1093/ajcp/aqw211
78. Hanna WM, Rüschoff J, Bilous M, Coudry RA, Dowsett M, Osamura RY, et al. HER2 in situ hybridization in breast cancer: clinical implications of polysomy 17 and genetic heterogeneity. Mod Pathol. (2014) 27:4–18. doi: 10.1038/modpathol.2013.103
79. Yang Y-L, Fan Y, Lang R-G, Gu F, Ren M-J, Zhang X-M, et al. Genetic heterogeneity of HER2 in breast cancer: impact on HER2 testing and its clinicopathologic significance. Breast Cancer Res Treat. (2012) 134:1095–102. doi: 10.1007/s10549-012-2046-0
80. Brunelli M, Manfrin E, Martignoni G, Miller K, Remo A, Reghellin D, et al. Genotypic intratumoral heterogeneity in breast carcinoma with HER2/neu amplification: evaluation according to ASCO/CAP criteria. Am J Clin Pathol. (2009) 131:678–82. doi: 10.1309/AJCP09VUTZWZXBMJ
81. Bartlett AI, Starcyznski J, Robson T, Maclellan A, Campbell FM, van de Velde CJH, et al. Heterogeneous HER2 gene amplification: impact on patient outcome and a clinically relevant definition. Am J Clin Pathol. (2011) 136:266–74. doi: 10.1309/AJCP0EN6AQMWETZZ
82. Nitta H, Kelly BD, Padilla M, Wick N, Brunhoeber P, Bai I, et al. A gene-protein assay for human epidermal growth factor receptor 2 (HER2): brightfield tricolor visualization of HER2 protein, the HER2 gene, and chromosome 17 centromere (CEN17) in formalin-fixed, paraffin-embedded breast cancer tissue sections. Diagn Pathol. (2012) 7:60. doi: 10.1186/1746-1596-7-60
83. Hou Y, Nitta H, Wei L, Banks PM, Portier B, Parwani AV, et al. HER2 intratumoral heterogeneity is independently associated with incomplete response to anti-HER2 neoadjuvant chemotherapy in HER2-positive breast carcinoma. Breast Cancer Res Treat. (2017) 166:447–57. doi: 10.1007/s10549-017-4453-8
84. Carey LA, Berry DA, Cirrincione CT, Barry WT, Pitcher BN, Harris LN, et al. Molecular heterogeneity and response to neoadjuvant human epidermal growth factor receptor 2 targeting in CALGB 40601, a randomized phase III trial of paclitaxel plus trastuzumab with or without lapatinib. J Clin Oncol. (2016) 34:542–9. doi: 10.1200/JCO.2015.62.1268
85. Dieci MV, Prat A, Tagliafico E, Paré L, Ficarra G, Bisagni G, et al. Integrated evaluation of PAM50 subtypes and immune modulation of pCR in HER2-positive breast cancer patients treated with chemotherapy and HER2-targeted agents in the CherLOB trial. Ann Oncol. (2016) 27:1867–73. doi: 10.1093/annonc/mdw262
86. Pivot X, Romieu G, Debled M, Pierga J-Y, Kerbrat P, Bachelot T, et al. 6 months versus 12 months of adjuvant trastuzumab for patients with HER2-positive early breast cancer (PHARE): a randomised phase 3 trial. Lancet Oncol. (2013) 14:741–8. doi: 10.1016/S1470-2045(13)70225-0
87. Kramar A, Bachelot T, Madrange N, Pierga J-Y, Kerbrat P, Espié M, et al. Trastuzumab duration effects within patient prognostic subgroups in the PHARE trial. Ann Oncol. (2014) 25:1563–70. doi: 10.1093/annonc/mdu177
88. Ferrari A, Sertier A-S, Vincent-Salomon A, Pivot X, Pauporté I, Saintigny P, et al. A phenotypic and mechanistic perspective on heterogeneity of HER2-positive breast cancers. Mol Cell Oncol. (2016) 3:e1232186. doi: 10.1080/23723556.2016.1232186
89. Visvader JE, Stingl J. Mammary stem cells and the differentiation hierarchy: current status and perspectives. Genes Dev. (2014) 28:1143–58. doi: 10.1101/gad.242511.114
90. Koren S, Bentires-Alj M. Breast tumor heterogeneity: source of fitness, hurdle for therapy. Mol Cell. (2015) 60:537–46. doi: 10.1016/j.molcel.2015.10.031
91. Skibinski A, Kuperwasser C. The origin of breast tumor heterogeneity. Oncogene. (2015) 34:5309–16. doi: 10.1038/onc.2014.475
92. Greenman CD, Pleasance ED, Newman S, Yang F, Fu B, Nik-Zainal S, et al. Estimation of rearrangement phylogeny for cancer genomes. Genome Res. (2012) 22:346–61. doi: 10.1101/gr.118414.110
93. Daemen A, Manning G. HER2 is not a cancer subtype but rather a pan-cancer event and is highly enriched in AR-driven breast tumors. Breast Cancer Res. (2018) 20:8. doi: 10.1186/s13058-018-0933-y
94. Wilson TR, Yu J, Lu X, Spoerke JM, Xiao Y, O'Brien C, et al. The molecular landscape of high-risk early breast cancer: comprehensive biomarker analysis of a phase III adjuvant population. NPJ Breast Cancer. (2016) 2:16022. doi: 10.1038/npjbcancer.2016.22
95. Baselga J, Cortés J, Im S-A, Clark E, Ross G, Kiermaier A, et al. Biomarker analyses in CLEOPATRA: a phase III, placebo-controlled study of pertuzumab in human epidermal growth factor receptor 2-positive, first-line metastatic breast cancer. J Clin Oncol. (2014) 32:3753–61. doi: 10.1200/JCO.2013.54.5384
96. Baselga J, Lewis Phillips GD, Verma S, Ro J, Huober J, Guardino AE, et al. Relationship between tumor biomarkers and efficacy in EMILIA, a phase III study of trastuzumab emtansine in HER2-positive metastatic breast cancer. Clin Cancer Res. (2016) 22:3755–63. doi: 10.1158/1078-0432.CCR-15-2499
97. Kim S-B, Wildiers H, Krop IE, Smitt M, Yu R, Lysbet de Haas S, et al. Relationship between tumor biomarkers and efficacy in TH3RESA, a phase III study of trastuzumab emtansine (T-DM1) vs. treatment of physician's choice in previously treated HER2-positive advanced breast cancer. Int J Cancer. (2016) 139:2336–42. doi: 10.1002/ijc.30276
98. Schneeweiss A, Chia S, Hegg R, Tausch C, Deb R, Ratnayake J, et al. Evaluating the predictive value of biomarkers for efficacy outcomes in response to pertuzumab- and trastuzumab-based therapy: an exploratory analysis of the TRYPHAENA study. Breast Cancer Res. (2014) 16:R73. doi: 10.1186/bcr3690
99. Denkert C, Huober J, Loibl S, Prinzler J, Kronenwett R, Darb-Esfahani S, et al. HER2 and ESR1 mRNA expression levels and response to neoadjuvant trastuzumab plus chemotherapy in patients with primary breast cancer. Breast Cancer Res. (2013) 15:R11. doi: 10.1186/bcr3384
100. Scaltriti M, Nuciforo P, Bradbury I, Sperinde J, Agbor-Tarh D, Campbell C, et al. High HER2 expression correlates with response to the combination of lapatinib and trastuzumab. Clin Cancer Res. (2015) 21:569–76. doi: 10.1158/1078-0432.CCR-14-1824
101. Arnould L, Arveux P, Couturier J, Gelly-Marty M, Loustalot C, Ettore F, et al. Pathologic complete response to trastuzumab-based neoadjuvant therapy is related to the level of HER-2 amplification. Clin Cancer Res. (2007) 13:6404–9. doi: 10.1158/1078-0432.CCR-06-3022
102. Paik S, Kim C, Wolmark N. HER2 status and benefit from adjuvant trastuzumab in breast cancer. N Engl J Med. (2008) 358:1409–11. doi: 10.1056/NEJMc0801440
103. Perez EA, Press MF, Dueck AC, Jenkins RB, Kim C, Chen B, et al. Immunohistochemistry and fluorescence in situ hybridization assessment of HER2 in clinical trials of adjuvant therapy for breast cancer (NCCTG N9831, BCIRG 006, and BCIRG 005). Breast Cancer Res Treat. (2013) 138:99–108. doi: 10.1007/s10549-013-2444-y
104. Meeting Library. HER2 Heterogeneity as a Predictor of Response to Neoadjuvant T-DM1 Plus Pertuzumab: Results From a Prospective Clinical Trial. Available online at: https://meetinglibrary.asco.org/record/174997/abstract (accessed September 8, 2019).
105. de Azambuja E, Holmes AP, Piccart-Gebhart M, Holmes E, Di Cosimo S, Swaby RF, et al. Lapatinib with trastuzumab for HER2-positive early breast cancer (NeoALTTO): survival outcomes of a randomised, open-label, multicentre, phase 3 trial and their association with pathological complete response. Lancet Oncol. (2014) 15:1137–46. doi: 10.1016/S1470-2045(14)70320-1
106. Swain SM, Ewer MS, Viale G, Delaloge S, Ferrero J-M, Verrill M, et al. Pertuzumab, trastuzumab, and standard anthracycline- and taxane-based chemotherapy for the neoadjuvant treatment of patients with HER2-positive localized breast cancer (BERENICE): a phase II, open-label, multicenter, multinational cardiac safety study. Ann Oncol. (2018) 29:646–53. doi: 10.1093/annonc/mdx773
107. Pogue-Geile KL, Song N, Jeong J-H, Gavin PG, Kim S-R, Blackmon NL, et al. Intrinsic subtypes, PIK3CA mutation, and the degree of benefit from adjuvant trastuzumab in the NSABP B-31 trial. J Clin Oncol. (2015) 33:1340–7. doi: 10.1200/JCO.2014.56.2439
108. Perez EA, Ballman KV, Mashadi-Hossein A, Tenner KS, Kachergus JM, Norton N, et al. Intrinsic subtype and therapeutic response among HER2-positive breaty st tumors from the NCCTG (Alliance) N9831 trial. J Natl Cancer Inst. (2017) 109:djw207. doi: 10.1093/jnci/djw207
109. Rimawi MF, Schiff R, Osborne CK. Targeting HER2 for the treatment of breast cancer. Annu Rev Med. (2015) 66:111–28. doi: 10.1146/annurev-med-042513-015127
110. Xu T, He B-S, Liu X-X, Hu X-X, Lin K, Pan Y-Q, et al. The predictive and prognostic role of stromal tumor-infiltrating lymphocytes in HER2-positive breast cancer with trastuzumab-based treatment: a meta-analysis and systematic review. J Cancer. (2017) 8:3838–48. doi: 10.7150/jca.21051
111. Ochi T, Bianchini G, Ando M, Nozaki F, Kobayashi D, Criscitiello C, et al. Predictive and prognostic value of stromal tumour-infiltrating lymphocytes before and after neoadjuvant therapy in triple negative and HER2-positive breast cancer. Eur J Cancer. (2019) 118:41–8. doi: 10.1016/j.ejca.2019.05.014
112. Degnim AC, Brahmbhatt RD, Radisky DC, Hoskin TL, Stallings-Mann M, Laudenschlager M, et al. Immune cell quantitation in normal breast tissue lobules with and without lobulitis. Breast Cancer Res Treat. (2014) 144:539–49. doi: 10.1007/s10549-014-2896-8
113. Salgado R, Denkert C, Campbell C, Savas P, Nuciforo P, Nucifero P, et al. Tumor-infiltrating lymphocytes and associations with pathological complete response and event-free survival in HER2-positive early-stage breast cancer treated with lapatinib and trastuzumab: a secondary analysis of the NeoALTTO trial. JAMA Oncol. (2015) 1:448–54. doi: 10.1001/jamaoncol.2015.0830
114. Bianchini G, Pusztai L, Pienkowski T, Im Y-H, Bianchi GV, Tseng L-M, et al. Immune modulation of pathologic complete response after neoadjuvant HER2-directed therapies in the NeoSphere trial. Ann Oncol. (2015) 26:2429–36. doi: 10.1093/annonc/mdv395
115. Solinas C, Ceppi M, Lambertini M, Scartozzi M, Buisseret L, Garaud S, et al. Tumor-infiltrating lymphocytes in patients with HER2-positive breast cancer treated with neoadjuvant chemotherapy plus trastuzumab, lapatinib or their combination: a meta-analysis of randomized controlled trials. Cancer Treat Rev. (2017) 57:8–15. doi: 10.1016/j.ctrv.2017.04.005
116. Luen SJ, Salgado R, Fox S, Savas P, Eng-Wong J, Clark E, et al. Tumour-infiltrating lymphocytes in advanced HER2-positive breast cancer treated with pertuzumab or placebo in addition to trastuzumab and docetaxel: a retrospective analysis of the CLEOPATRA study. Lancet Oncol. (2017) 18:52–62. doi: 10.1016/S1470-2045(16)30631-3
117. Nuciforo P, Pascual T, Cortés J, Llombart-Cussac A, Fasani R, Paré L, et al. A predictive model of pathologic response based on tumor cellularity and tumor-infiltrating lymphocytes (CelTIL) in HER2-positive breast cancer treated with chemo-free dual HER2 blockade. Ann Oncol. (2018) 29:170–7. doi: 10.1093/annonc/mdx647
118. Cortazar P, Zhang L, Untch M, Mehta K, Costantino JP, Wolmark N, et al. Pathological complete response and long-term clinical benefit in breast cancer: the CTNeoBC pooled analysis. Lancet. (2014) 384:164–72. doi: 10.1016/S0140-6736(13)62422-8
119. Loi S, Giobbie-Hurder A, Gombos A, Bachelot T, Hui R, Curigliano G, et al. Pembrolizumab plus trastuzumab in trastuzumab-resistant, advanced, HER2-positive breast cancer (PANACEA): a single-arm, multicentre, phase 1b−2 trial. Lancet Oncol. (2019) 20:371–82. doi: 10.1016/S1470-2045(18)30812-X
120. Vernieri C, Milano M, Brambilla M, Mennitto A, Maggi C, Cona MS, et al. Resistance mechanisms to anti-HER2 therapies in HER2-positive breast cancer: current knowledge, new research directions and therapeutic perspectives. Crit Rev Oncol Hematol. (2019) 139:53–66. doi: 10.1016/j.critrevonc.2019.05.001
121. Kennedy SP, Hastings JF, Han JZR, Croucher DR. The under-appreciated promiscuity of the epidermal growth factor receptor family. Front Cell Dev Biol. (2016) 4:88. doi: 10.3389/fcell.2016.00088
122. Bahrami A, Khazaei M, Shahidsales S, Hassanian SM, Hasanzadeh M, Maftouh M, et al. The therapeutic potential of PI3K/Akt/mTOR inhibitors in breast cancer: rational and progress. J Cell Biochem. (2018) 119:213–22. doi: 10.1002/jcb.26136
123. André F, O'Regan R, Ozguroglu M, Toi M, Xu B, Jerusalem G, et al. Everolimus for women with trastuzumab-resistant, HER2-positive, advanced breast cancer (BOLERO-3): a randomised, double-blind, placebo-controlled phase 3 trial. Lancet Oncol. (2014) 15:580–91. doi: 10.1016/S1470-2045(14)70138-X
124. André F, Hurvitz S, Fasolo A, Tseng L-M, Jerusalem G, Wilks S, et al. Molecular alterations and everolimus efficacy in human epidermal growth factor receptor 2–overexpressing metastatic breast cancers: combined exploratory biomarker analysis from BOLERO-1 and BOLERO-3. JCO. (2016) 34:2115–24. doi: 10.1200/JCO.2015.63.9161
125. Yang J, Nie J, Ma X, Wei Y, Peng Y, Wei X. Targeting PI3K in cancer: mechanisms and advances in clinical trials. Mol Cancer. (2019) 18:26. doi: 10.1186/s12943-019-0954-x
126. Phase I Study of Alpelisib (BYL-719) and Trastuzumab Emtansine (T-DM1) in HER2-Positive Metastatic Breast Cancer (MBC) After Trastuzumab and Taxane Therapy. Springer. Available online at: https://link.springer.com/article/10.1007%2Fs10549-018-4792-0 (accessed September 8, 2019).
127. Overcoming CDK4/6 inhibitor resistance. In: ER-Positive Breast Cancer in: Endocrine-Related Cancer, Vol. 26 (2019). Available online at: https://erc.bioscientifica.com/view/journals/erc/26/1/ERC-18-0317.xml (accessed September 6, 2019).
128. Goel S, Wang Q, Watt AC, Tolaney SM, Dillon DA, Li W, et al. Overcoming therapeutic resistance in HER2-positive breast cancers with CDK4/6 inhibitors. Cancer Cell. (2016) 29:255–69. doi: 10.1016/j.ccell.2016.02.006
129. Finn RS, Dering J, Conklin D, Kalous O, Cohen DJ, Desai AJ, et al. PD 0332991, a selective cyclin D kinase 4/6 inhibitor, preferentially inhibits proliferation of luminal estrogen receptor-positive human breast cancer cell lines in vitro. Breast Cancer Res. (2009) 11:R77. doi: 10.1186/bcr2419
130. Gianni L, Bisagni G, Colleoni M, Del Mastro L, Zamagni C, Mansutti M, et al. Neoadjuvant treatment with trastuzumab and pertuzumab plus palbociclib and fulvestrant in HER2-positive, ER-positive breast cancer (NA-PHER2): an exploratory, open-label, phase 2 study. Lancet Oncol. (2018) 19:249–56. doi: 10.1016/S1470-2045(18)30001-9
Keywords: breast cancer, HER2-positive, intrinsic subtype, heterogeneity, HER2-enriched, molecular
Citation: Godoy-Ortiz A, Sanchez-Muñoz A, Chica Parrado MR, Álvarez M, Ribelles N, Rueda Dominguez A and Alba E (2019) Deciphering HER2 Breast Cancer Disease: Biological and Clinical Implications. Front. Oncol. 9:1124. doi: 10.3389/fonc.2019.01124
Received: 16 June 2019; Accepted: 09 October 2019;
Published: 29 October 2019.
Edited by:
Mothaffar Rimawi, Baylor College of Medicine, United StatesReviewed by:
Howard Donninger, University of Louisville, United StatesParvin Mehdipour, Tehran University of Medical Sciences, Iran
Copyright © 2019 Godoy-Ortiz, Sanchez-Muñoz, Chica Parrado, Álvarez, Ribelles, Rueda Dominguez and Alba. This is an open-access article distributed under the terms of the Creative Commons Attribution License (CC BY). The use, distribution or reproduction in other forums is permitted, provided the original author(s) and the copyright owner(s) are credited and that the original publication in this journal is cited, in accordance with accepted academic practice. No use, distribution or reproduction is permitted which does not comply with these terms.
*Correspondence: Ana Godoy-Ortiz, anagodort@gmail.com