- 1Division of Medical Oncology & Hematology, Department of Medicine, Princess Margaret Cancer Centre, University of Toronto, Toronto, ON, Canada
- 2Experimental Therapeutics Unit, Medical Oncology Department, Hospital Clínico San Carlos, and IdISSC, Madrid, Spain
- 3Centro de Investigación del Cáncer-CSIC, Salamanca, Spain
- 4Centro de Investigación Biomédica en Red Cáncer (CIBERONC), Madrid, Spain
- 5Centro Regional de Investigaciones Biomédicas, Castilla-La Mancha University (UCLM), Albacete, Spain
Introduction: Therapeutic targeting of inhibitors of the immune response has reached the clinical setting. Inhibitors of the novel receptor LAG3, which negatively regulates T-cell activation, are under investigation. Here we explore the presence and prognostic role of LAG3 in cancer.
Methods: A systematic search of electronic databases identified publications exploring the effect of LAG3 on overall survival (OS) and (for early-stage cancers) disease-free survival (DFS). Hazard ratios (HR) were pooled in a meta-analysis using generic inverse-variance and random effect modeling. Subgroup analyses were conducted based on disease site and tumor type.
Results: Fifteen studies met the inclusion criteria. LAG3 was associated with better overall survival [HR 0.81, 95% confidence interval (CI) 0.66–0.99; P = 0.04], with subgroup analysis showing no significant differences between disease-site subgroups. The beneficial effect of LAG3 on OS was of greater magnitude in early-stage malignancies (HR 0.73, 95% CI 0.60–0.88) than in the metastatic setting (HR 1.20, 95% CI 0.70–2.05), but this difference was not statistically significant (subgroup difference p = 0.18). LAG3 did not have a significant association with DFS [HR 1.02, 95% confidence interval (CI) 0.77–1.37; P = 0.87], with subgroup analysis showing worse DFS in patients with lymphoma and improved DFS in those with breast cancer.
Conclusions: High expression of LAG3 is associated with favorable overall survival in several solid tumors. A trend toward an association in early-stage disease suggests the importance of immune surveillance in this setting.
Introduction
Inhibiting receptor downregulators of the immune response has become an attractive therapeutic approach, with successful results in some cancers (1). Inhibition of some of these receptors, including cytotoxic T-lymphocyte-associated protein-4 (CTLA-4) and programmed cell death-1 (PD-1) and its ligand PD-L1, has been shown to have anti-tumoral effects by inducing an effector response on CD8+ T cells (1–4). Compounds of this family have been approved in different indications, such as non-small cell lung (NSCLC), head and neck, bladder, and triple-negative breast cancers (5–9). In some but not all tumors, the presence of PD-L1-expression or markers associated with genomic instability such as tumor mutational burden or neo-antigen load predicts response to checkpoint inhibitors (5, 10–12).
For immune checkpoint inhibitors to be active, tumors must be in an inflamed or pre-activated state; so-called “hot tumors” (13). In this context, the presence of tumor-infiltrating lymphocytes (TILs) is necessary for adequate immune response (14, 15). Other co-inhibitory receptors have been described, including T-cell immunoglobulin and mucin-domain containing-3 (TIM-3) or the lymphocyte-activation gene-3 (LAG3) (16, 17). LAG3 is a transmembrane protein with structural homology to the CD4 co-receptor. It is found in activated CD4+ T cells, T-regulator cell, Tr1 cells, activated CD8+ T cells, natural killer cells, dendritic cells, B cells, and exhausted effector T cells (17, 18). The ligands for LAG3 include MHC class II molecules, galectin-3, liver sinusoidal endothelial cell lectin, and fibrinogen-like protein I (18, 19). Although the precise molecular mechanisms remain elusive, LAG3 negatively regulates the proliferation, activation, and effector function of T cells. To date, it is regarded as one of the multiple immune-checkpoint inhibitors that can promote immune tolerance through T-cell dysfunction and exhaustion (17, 18). Such negative effects seem to rely on the direct inhibition of CD3-mediated T-cell proliferation, cytokine production, and calcium flux (20). However, its suppressive effects are less apparent than those elicited by PD-1 or CTLA-4 in preclinical studies, suggesting the potential for a better safety profile (21, 22). Given its role as a negative regulator of T-cell activation, strategies aiming to inhibit its action are currently in clinical development (17, 23, 24). For both TIM-3 and LAG3, therapeutic antibodies are in clinical development, mainly in combination with inhibitors of PD-1 or PD-L1 (25).
The presence of TILs within the tumor is associated with a favorable outcome, demonstrating that the existence of an immune-activated state predicts an improved prognosis as well as response to immunotherapy (15, 26). In addition, the expression of PD-1 and PD-L1 is associated with a good outcome irrespective of treatment with immune checkpoint inhibitors, supporting the importance of the adaptive immune response (27–29). In this article, we aim to explore the frequency of expression and prognostic role of LAG3. We hypothesized that the expression of LAG3 would be associated with worse outcomes due to its inhibitory effects on the immune response.
Methods
Data Sources and Searches
This analysis was conducted following the Preferred Reporting Items for Systematic Reviews and Meta-analyses (PRISMA) guidelines. An electronic search of MEDLINE (host: Pubmed) from 1946 to March 30th, 2019 was performed using the search terms: “lymphocyte activation gene 3” or “LAG3” and “Cancer,” limiting results to studies in humans. Citation lists of the retrieved articles were screened manually to ensure the sensitivity of the search strategy.
Study Selection
Eligibility criteria for studies included: (i) studies of humans (adults and children); (ii) patients with hematological or solid tumors; (iii) reporting of a hazard ratio (HR) for overall survival (OS) and/or disease-free survival (DFS; defined as the length of time from primary treatment of an early-stage cancer to death or any signs or symptoms of recurrent cancer) or survival curves allowing estimation of the HR for OS or DFS; (iv) availability as a full-text publication; (v) clinical trials, cohort, or case-control studies; and (vi) English language publication. Case reports, conference abstracts, and letters to editors were excluded. The titles identified by the initial search were evaluated, and potentially relevant publications were retrieved in full. Two authors (JFA and PP) reviewed the full articles independently for eligibility. Disagreements were resolved by consensus.
Data Extraction
The following data were collected from the included studies using a predesigned abstraction form: name of first author, year of publication, journal, number of patients included in analysis, primary malignancy, protein expression, methods used for the evaluation of LAG3, and cut-off used for defining LAG3 intensity. Data extraction was performed by one author (RS). The outcome of interest was OS in patients both with and without LAG3-expression as defined by the individual studies. The HR for OS was extracted whenever available. In cases where the HR was not reported, it was estimated from survival curves for OS using the methods described by Parmar et al. (30). We applied a hierarchal approach to the collection of HRs, preferring those reported from multivariable analyses to univariable HR, and preferring both over HRs estimated from survival plots.
Data Synthesis and Statistical Analysis
The extracted data were pooled using RevMan 5.3 analysis software (Cochrane Collaboration, Copenhagen, Denmark). Estimates for HRs were pooled and weighted by generic inverse variance and computed by random effect modeling. Statistical heterogeneity was assessed using the Cochran's Q and I2 statistics. Subgroup analyses were performed for different disease sites. Differences between the subgroups were assessed using methods described by Deeks et al. (31). Sensitivity analyses were performed, excluding studies in pediatric tumors and when LAG3-expression was evaluated using ELISA rather than immunohistochemistry (IHC) or next-generation sequencing (NGS). NGS sub-analysis was done between genetic variants A/G and G/G, with A/A as the referent group. A post-hoc exploratory analysis was performed to evaluate associations between the expression of LAG3 and other immune markers, including tumor mutational burden (TMB) and neoantigen burden. All of the statistical tests were two-sided, and statistical significance was defined as p < 0.05. No correction was applied for multiple statistical testing.
Results
Fifteen retrospective studies comprising 6,306 patients were identified (Figure 1) (32–46). The characteristics of the included studies are listed in Table 1. LAG3 was reported as positive in 1,868 patients (31%). Fourteen studies explored the prognostic influence of LAG3 in adults, and one study was performed in a pediatric population. Two studies did not report OS outcomes, and five studies did not report DFS. The eligible studies explore outcomes in patients with breast, ovarian, gastric, lymphoma, NSCLC, colorectal, and renal cancers as well as pediatric neuroblastoma. Only two studies reported the use of neoadjuvant therapy, which was based on taxane/platinum combinations for breast cancer patients and 5-fluorouracil ± bevacizumab for colorectal cancer patients (32, 34). The eligible studies either did not deliver neoadjuvant therapy (n = 4) or excluded patients receiving pre-operative systemic therapy (n = 7) (36, 38, 40–42, 44, 46). Only two patients in the cohort of neuroblastoma were exposed to adjuvant, IL-2-based, immunotherapy (37). As for the rest of the studies, data on the treatment regimens was either not reported or details were incomplete, thereby not allowing additional investigation.
Overall Survival
Data for the association between LAG3 and OS were reported in 13 studies. LAG3 was associated with better overall survival [HR 0.81, 95% confidence interval (CI) 0.66–0.99; P = 0.04, Figure 2A]. Heterogeneity was statistically significant (Cochran Q P < 0.001, I2 = 64%). Subgroup analysis showed that there were no significant differences between disease-site subgroups (Subgroup difference P = 0.24, Figure 2B). There was no significant difference between testing for LAG3 using IHC or DNA extraction (HR 0.79, 95% CI 0.58–1.07 vs. HR 0.91, 95% CI 0.73–1.14; subgroup difference P = 0.45). There was also no significant difference between genetic variants A/G and G/G relative to the A/A control group (subgroup difference P = 0.83). The beneficial effect of LAG3 on OS was of greater magnitude in early-stage malignancies (HR 0.73, 95% CI 0.60–0.88) than in the metastatic setting (HR 1.20, 95% CI 0.70–2.05), but this difference did not meet the statistical significance requirement (subgroup difference p = 0.18). Subgroup analysis showed that there was a greater magnitude of favorable prognosis with LAG3 expression in terms of OS when HRs were extracted rather than estimated (calculated HR 0.60, 95% CI 0.40–0.91, extracted HR 0.92, 95% CI 0.75–1.12). This difference approached but did not reach statistical significance (p for difference = 0.07).
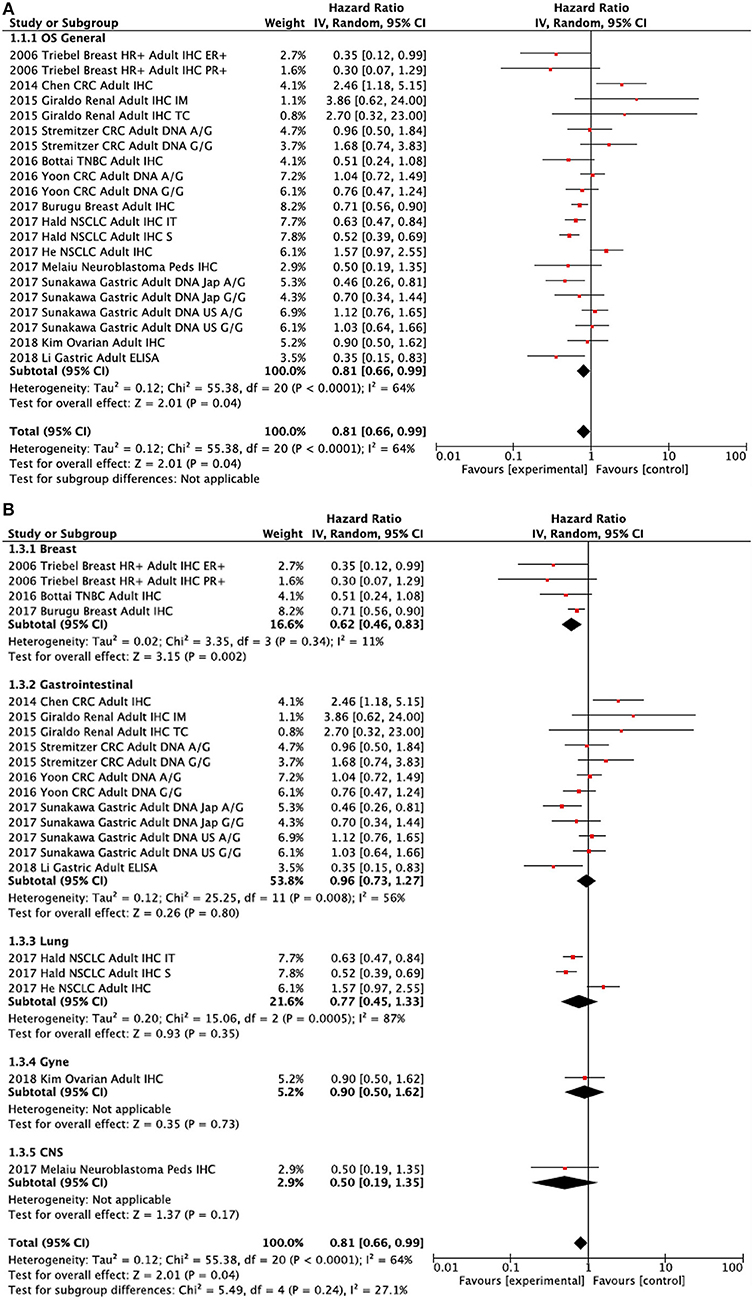
Figure 2. Forest plots showing hazard ratios for overall survival: LAG3 overall (A) and by subgroups based on disease site (B). Hazard ratios for each study are represented by squares: the size of the square represents the weight of the study in the meta-analysis; the horizontal line passing through the square represents the 95% confidence interval. All statistical tests were two-sided. The diamonds represent the estimated pooled effect. Test for overall effect based on z-test. All P-values are two-sided. CI, confidence interval; OR, odds ratio. (A) LAG3 OS Overall. (B) LAG3 OS by disease site.
Disease-Free Survival
Data for the association between LAG3 and disease-free survival (DFS) were reported in 10 out of 15 studies that included early-stage cancers. LAG3 did not exhibit a significant association with DFS [HR 1.02, 95% confidence interval (CI) 0.77–1.37; P = 0.87, Figure 3A]. Heterogeneity was statistically significant (Cochran Q P < 0.001, I2 = 70%). There was an association with worse DFS for lymphoma (HR 4.16, 95% CI 1.77–9.78, p = 0.001) and, to a lesser extent, NSCLC (HR 1.48, 95% CI 0.98–2.23, Figure 3B). Improved DFS was noted in the breast cancer group (HR 0.64, 95% CI 0.42–0.98). Excluding lymphoma and breast cancer, there was no significant difference between the remaining tumors (Subgroup difference P = 0.57), but heterogeneity remained statistically significant (Cochran Q P = 0.006, I2 = 63%). There was a modest and non-significant association between LAG3 and improved DFS in early-stage malignancies (HR 0.82, 95% CI 0.62–1.08) compared to the metastatic setting (HR 0.91, 95% CI 0.64–1.31), but this difference did not meet statistical significance requirements (subgroup difference p = 0.17). Again, subgroup analysis showed that there was a borderline significant difference in the prognostic value of LAG3 expression in terms of DFS based on whether HRs were extracted or estimated (calculated HR 1.12, 95% CI 0.46–2.72, extracted HR 0.96, 95% CI 0.72–1.27, p = 0.07).
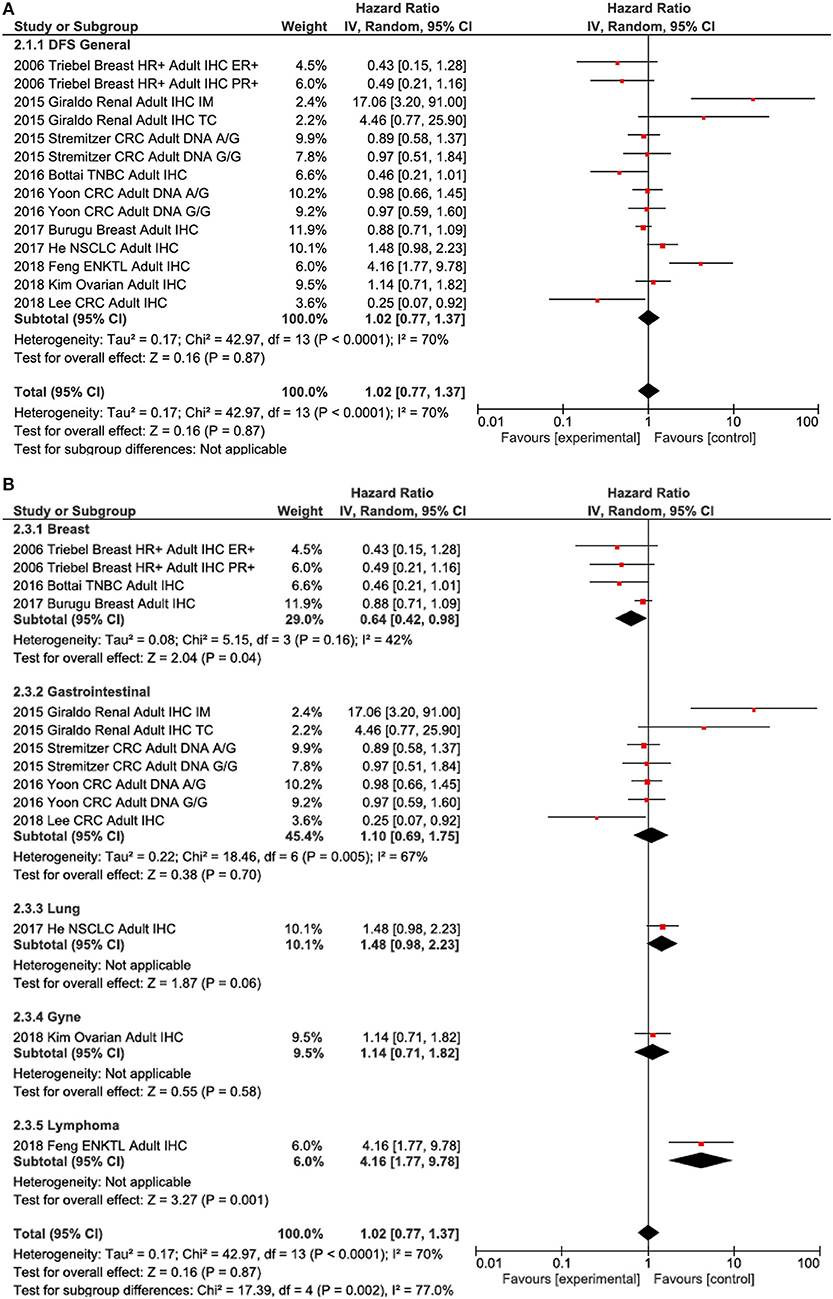
Figure 3. Forest plots showing hazard ratios for disease-free survival (DFS): LAG3 overall (A) and by subgroups based on disease site (B). Hazard ratios for each study are represented by squares: the size of the square represents the weight of the study in the meta-analysis; the horizontal line passing through the square represents the 95% confidence interval. All statistical tests were two-sided. The diamonds represent the estimated pooled effect. Test for overall effect based on z-test. All P-values are two-sided. CI, confidence interval; OR, odds ratio. (A) LAG3 DFS overall. (B) LAG3 DFS by disease site.
Correlation of LAG3 With Other Immunological Markers
The analysis of potential associations between LAG3 expression in TILs and other biomarkers in our work was hindered by the small number of studies providing combined data on PD-1, PD-L1, CD8, TIM3, and TMB. In a cohort of breast cancer patients, PD-L1, PD-1, and CD8 were positive in 53, 61, and 26% of LAG3+ TILs, respectively. In one study, concurrent infiltration of LAG3+ and CD8+ TILs in ER- breast cancer patients was associated with significantly longer DFS (HR 0.49, 95% 0.32–0.74) (32). Of note, LAG3+/PD-L1+ TILs have been identified in 15% of patients with triple-negative breast cancer (45). In an NSCLC cohort, PD-L1 and PD-1 were expressed in 47 and 70% of LAG3+ TILs, respectively. Up to 31% of NSCLC with LAG3+ TILs also expressed PD-L1 and, of note, the group of tumors without expression of either PD-L1- or LAG3- TILs showed longer relapse-free survival (2.09 years) than the group with PD-L1+ tumor cells and LAG3+ TILs (0.67 years) (46). Furthermore, significant positive correlations have been reported in renal cell carcinoma samples between LAG3 and CD8 and PD-1, indicating not only the coexistence of biomarkers but proportional variations (41). Finally, LAG3 and TIM3 appeared to be co-expressed in up to 71% of cases of extranodal nasal NK/T cell lymphoma (33). There were no data included on TMB or neoantigen burden.
Discussion
In the present article, we describe the frequency of expression and prognostic value of LAG3 in several tumors. We find the presence of LAG3 to be associated with better OS. In the included studies, the effect was consistent in different tumor types. While, on average, no effect on DFS was observed, there was significant heterogeneity in effect between different disease sites. Of note as an interesting finding is the marked association in early-stage disease, which suggests an immunologic role in minimal residual disease.
LAG3 is a co-inhibitory receptor that represses the effector response of cytotoxic T cells (23, 24). In this context, several therapies are currently in clinical development to inhibit its activation, therefore facilitating an immune response (23). Synergy has been reported with the combination of LAG3 and PD-1 inhibition in murine models of melanoma, colorectal cancer, liver cancer, and fibrosarcoma, achieving responses in tumors largely resistant to single-agent immunotherapy (47). Also, CTLA-4 inhibition has been found to elicit an increase in the frequency of LAG3+ TILs in melanoma patients (48). These findings, together with a better safety profile, underscore the clinical interest of targeting LAG3 either alone or in combination with other immune checkpoint inhibitors. It has been demonstrated that concomitant treatment with LAG3 inhibitors with anti-PD-1 or PD-L1 can produce an enhanced effect, and, in this context, ongoing clinical studies are evaluating these combinations (2).
No difference was found between the methods used for the analysis of LAG3 expression or the different genetic variants defining expression. This suggests the sensitivity of the observed effect. Significant heterogeneity was observed among different tumors, and this may be a reflection of the heterogeneity observed in the immune response and in the presence of TILs in the different tumor types (49). This mirrors the discrepancy between tumors in the objective responses observed when checkpoint inhibitors are administered and the fact that response is not associated consistently with the expression of PD1 or PD-L1 in all tumor types (50).
An interesting finding is the association of LAG3 with a better outcome in the early-stage disease. It is well-established that the immune system plays a central role in avoiding long-term relapses in early-stage tumors (51). Although this effect is mediated by mechanisms that are not well-described, the presence of an active immune state contributes to the maintenance of cells in a quiescent state (51). In our study, we unexpectedly identified a better outcome with LAG3 in early-stage tumors. We are not aware of prior data reporting this association.
An association between the expression of immune inhibitory molecules such as PD-L1 and CTLA-4 and improved tumor outcomes has been described previously (29, 52, 53). Such an association seems paradoxical, since PD-L1, CTLA-4, and LAG3 elicit immunosuppressive responses and facilitate tumor escape. However, the upregulation of these molecules may initiate a negative feedback mechanism that creates an active immune environment in an inflamed tumor, which leads to an improved prognosis. Indeed, these markers usually overlap with CD8, which reflects an active host immunity and has established prognostic value (54). The expression of LAG3 was associated significantly with expression of PD-L1 and CD8 in the only two studies reporting such data (32, 41). More research is required to confirm these results.
The early stages of disease have been linked to a more intact host immunity and also to reduced tumor clonal heterogeneity. There is growing evidence that neoantigen heterogeneity may negatively influence immune surveillance and prognosis (55). Whether early-stage cancers have a more intact immune response due to a reduced pool of clonal neoantigens to target is a hypothesis that warrants investigation.
Contrary to our findings, the expression of other immune inhibitory receptors such as TIM3 has been associated with a worse prognosis (56), although no analysis based on early- or advanced-stage disease was performed. The results with LAG3 are more consistent with data observed with PD1 and PD-L1, which demonstrates a clear favorable prognosis when these markers are expressed (27–29).
The association between LAG3+ and other immunological biomarkers could not be addressed systematically in our work because of data scarcity. However, an association with biomarker aggregation is suggested by some studies in breast, NSCLC, and renal cancer patients (32, 41, 46). LAG3 and PD-1 have been found to be co-expressed consistently on both CD4+ and CD8+ TILs in several murine cancer models. Additionally, the co-inhibition of LAG3 and PD-1 elicits improved anti-tumor CD8+ T-cell responses (47). Additional data are needed to determine the impact on survival of aggregated biomarker expression in TILs and/or tumor cells. Similarly, an association has been described recently between tumors with LAG3+ TILs and a higher TMB based on data from the Cancer Genome Atlas (57). Unfortunately, none of the studies included in the current analysis reported data on TMB. While heterogenous and immature, this body of work suggests a functional interplay between immune markers and TMB. This warrants further research, especially regarding the potential for this interplay to provide predictive or prognostic value.
This study has limitations. This is a retrospective analysis of published articles. Therefore, it is susceptible to publication bias and also relies on summary data, not individual patient data. Furthermore, for some included studies (6 of 15), we estimated HR from survival plots, as it was not reported in the individual articles. Subgroup analysis showed that there was no significant difference in the prognostic value of LAG3 expression based on whether HRs were extracted or estimated. Finally, only one cohort reported data on hematologic malignancy and one on a pediatric population, so additional data on the prognostic value of LAG3 expression in hematological and pediatric malignancies is warranted.
In conclusion, we report the prognostic role of LAG3 in several tumors, suggesting that high expression is associated with a favorable outcome, particularly in terms of OS. The trend toward an association with outcome in early-stage disease supports the importance of immune surveillance in the setting of minimal residual disease.
Author Contributions
AO: concept and design. JF-A, PP, and RS: collection and assembly of data. EA and RS: data analysis and interpretation. All authors: manuscript writing and final approval of manuscript.
Conflict of Interest
EA reports personal fees from Genentech/Roche, personal fees from Apobiologix, personal fees from Myriad Genetics, personal fees from Agendia, outside the submitted work. AO reports personal fees from Entrechem, Servier, and Daiichi-Sankyo outside the submitted work. AP reports personal fees from Daiichi-Sankyo outside the submitted work. PP-S reports personal fees from Merck and MSD outside the submitted work.
The remaining authors declare that the research was conducted in the absence of any commercial or financial relationships that could be construed as a potential conflict of interest.
References
1. Ribas A, Wolchok JD. Cancer immunotherapy using checkpoint blockade. Science. (2018) 359:1350–5. doi: 10.1126/science.aar4060
2. Keenan TE, Burke KP, Van Allen EM. Genomic correlates of response to immune checkpoint blockade. Nat Med. (2019) 25:389–402. doi: 10.1038/s41591-019-0382-x
3. Walunas TL, Lenschow DJ, Bakker CY, Linsley PS, Freeman GJ, Green JM, et al. CTLA-4 can function as a negative regulator of T cell activation. Immunity. (1994) 1:405–13. doi: 10.1016/1074-7613(94)90071-X
4. Iwai Y, Ishida M, Tanaka Y, Okazaki T, Honjo T, Minato N. Involvement of PD-L1 on tumor cells in the escape from host immune system and tumor immunotherapy by PD-L1 blockade. Proc Natl Acad Sci USA. (2002) 99:12293–7. doi: 10.1073/pnas.192461099
5. Herbst RS, Baas P, Kim DW, Felip E, Pérez-Gracia JL, Han JY, et al. Pembrolizumab versus docetaxel for previously treated, PD-L1-positive, advanced non-small-cell lung cancer (KEYNOTE-010): a randomised controlled trial. Lancet. (2016) 387:1540–50. doi: 10.1016/S0140-6736(15)01281-7
6. Garon EB, Rizvi NA, Hui R, Leighl N, Balmanoukian AS, Eder JP, et al. Pembrolizumab for the treatment of non-small-cell lung cancer. N Engl J Med. (2015) 372:2018–28. doi: 10.1056/NEJMoa1501824
7. Rini BI, Powles T, Atkins MB, Escudier B, McDermott DF, Suarez C, et al. Atezolizumab plus bevacizumab versus sunitinib in patients with previously untreated metastatic renal cell carcinoma (IMmotion151): a multicentre, open-label, phase 3, randomised controlled trial. Lancet. (2019) 393:2404–15. doi: 10.1016/S0140-6736(19)30723-8
8. Balar AV, Galsky MD, Rosenberg JE, Powles T, Petrylak DP, Bellmunt J, et al. Atezolizumab as first-line treatment in cisplatin-ineligible patients with locally advanced and metastatic urothelial carcinoma: a single-arm, multicentre, phase 2 trial. Lancet. (2017) 389:67–76. doi: 10.1016/S0140-6736(16)32455-2
9. Schmid P, Adams S, Rugo HS, Schneeweiss A, Barrios CH, Iwata H, et al. Atezolizumab and nab-paclitaxel in advanced triple-negative breast cancer. N Engl J Med. (2018) 379:2108–21. doi: 10.1056/NEJMoa1809615
10. Rizvi NA, Hellmann MD, Snyder A, Kvistborg P, Makarov V, Havel JJ, et al. Cancer immunology. Mutational landscape determines sensitivity to PD-1 blockade in non-small cell lung cancer. Science. (2015) 348:124–8. doi: 10.1126/science.aaa1348
11. Miao D, Margolis CA, Vokes NI, Liu D, Taylor-Weiner A, Wankowicz SM, et al. Genomic correlates of response to immune checkpoint blockade in microsatellite-stable solid tumors. Nat Genet. (2018) 50:1271–81. doi: 10.1038/s41588-018-0200-2
12. Shukla SA, Bachireddy P, Schilling B, Galonska C, Zhan Q, Bango C, et al. Cancer-germline antigen expression discriminates clinical outcome to CTLA-4 blockade. Cell. (2018) 173:624–33.e628. doi: 10.1016/j.cell.2018.03.026
13. Prendergast GC, Mondal A, Dey S, Laury-Kleintop LD, Muller AJ. Inflammatory reprogramming with IDO1 inhibitors: turning immunologically unresponsive 'cold' tumors 'hot'. Trends Cancer. (2018) 4:38–58. doi: 10.1016/j.trecan.2017.11.005
14. Salgado R, Denkert C, Demaria S, Sirtaine N, Klauschen F, Pruneri G, et al. Harmonization of the evaluation of tumor infiltrating lymphocytes (TILs) in breast cancer: recommendations by an international TILs-working group 2014. Ann Oncol. (2015) 26:259–71. doi: 10.1093/annonc/mdu450
15. Adams S, Gray RJ, Demaria S, Goldstein L, Perez EA, Shulman LN, et al. Prognostic value of tumor-infiltrating lymphocytes in triple-negative breast cancers from two phase III randomized adjuvant breast cancer trials: ECOG 2197 and ECOG 1199. J Clin Oncol. (2014) 32:2959–66. doi: 10.1200/JCO.2013.55.0491
16. Freeman GJ, Casasnovas JM, Umetsu DT, DeKruyff RH. TIM genes: a family of cell surface phosphatidylserine receptors that regulate innate and adaptive immunity. Immunol Rev. (2010) 235:172–89. doi: 10.1111/j.0105-2896.2010.00903.x
17. He Y, Rivard CJ, Rozeboom L, Yu H, Ellison K, Kowalewski A, et al. Lymphocyte-activation gene-3, an important immune checkpoint in cancer. Cancer Sci. (2016) 107:1193–7. doi: 10.1111/cas.12986
18. Anderson AC, Joller N, Kuchroo VK. Lag-3, Tim-3, and TIGIT: Co-inhibitory receptors with specialized functions in immune regulation. Immunity. (2016) 44:989–1004. doi: 10.1016/j.immuni.2016.05.001
19. Wang J, Sanmamed MF, Datar I, Su TT, Ji L, Sun J, et al. Fibrinogen-like protein 1 is a major immune inhibitory ligand of LAG-3. Cell. (2019) 176:334–47.e12. doi: 10.1016/j.cell.2018.11.010
20. Hannier S, Tournier M, Bismuth G, Triebel F. CD3/TCR complex-associated lymphocyte activation gene-3 molecules inhibit CD3/TCR signaling. J Immunol Baltim Md. (1998) 161:4058–65.
21. Jha V, Workman CJ, McGaha TL, Li L, Vas J, Vignali DAA, et al. Lymphocyte activation gene-3 (LAG-3) negatively regulates environmentally-induced autoimmunity. PLoS ONE. (2014) 9:e104484. doi: 10.1371/journal.pone.0104484
22. Workman CJ, Cauley LS, Kim IJ, Blackman MA, Woodland DL, Vignali DAA. Lymphocyte activation gene-3 (CD223) regulates the size of the expanding T cell population following antigen activation in vivo. J Immunol Baltim Md. (2004) 172:5450–5. doi: 10.4049/jimmunol.172.9.5450
23. Andrews LP, Marciscano AE, Drake CG, Vignali DA. LAG3 (CD223) as a cancer immunotherapy target. Immunol Rev. (2017) 276:80–96. doi: 10.1111/imr.12519
24. Triebel F, Jitsukawa S, Baixeras E, Roman-Roman S, Genevee C, Viegas-Pequignot E, et al. LAG-3, a novel lymphocyte activation gene closely related to CD4. J Exp Med. (1990) 171:1393–405. doi: 10.1084/jem.171.5.1393
25. Smyth MJ, Ngiow SF, Ribas A, Teng MW. Combination cancer immunotherapies tailored to the tumour microenvironment. Nat Rev Clin Oncol. (2016) 13:143–58. doi: 10.1038/nrclinonc.2015.209
26. Martínez-Canales S, Cifuentes F, López De Rodas Gregorio M, Serrano-Oviedo L, Galán-Moya EM, Amir E, et al. Transcriptomic immunologic signature associated with favorable clinical outcome in basal-like breast tumors. PLoS ONE. (2017) 12:e0175128. doi: 10.1371/journal.pone.0175128
27. Van Berckelaer C, Rypens C, van Dam P, Pouillon L, Parizel M, Schats KA, et al. Infiltrating stromal immune cells in inflammatory breast cancer are associated with an improved outcome and increased PD-L1 expression. Breast Cancer Res. (2019) 21:28. doi: 10.1186/s13058-019-1108-1
28. Hou Y, Nitta H, Wei L, Banks PM, Lustberg M, Wesolowski R, et al. PD-L1 expression and CD8-positive T cells are associated with favorable survival in HER2-positive invasive breast cancer. Breast J. (2018) 24:911–9. doi: 10.1111/tbj.13112
29. Xing X, Guo J, Ding G, Li B, Dong B, Feng Q, et al. Analysis of PD1, PDL1, PDL2 expression and T cells infiltration in 1014 gastric cancer patients. Oncoimmunology. (2018) 7:e1356144. doi: 10.1080/2162402X.2017.1356144
30. Parmar MK, Torri V, Stewart L. Extracting summary statistics to perform meta-analyses of the published literature for survival endpoints. Stat Med. (1998) 17:2815–34. doi: 10.1002/(sici)1097-0258(19981230)17:24<2815::aid-sim110>3.0.co;2-8
31. Deeks JJ. Systematic reviews in health care: systematic reviews of evaluations of diagnostic and screening tests. BMJ. (2001) 323:157–62. doi: 10.1136/bmj.323.7305.157
32. Burugu S, Gao D, Leung S, Chia SK, Nielsen TO. LAG-3+ tumor infiltrating lymphocytes in breast cancer: clinical correlates and association with PD-1/PD-L1+ tumors. Ann Oncol. (2017) 28: 2977–84. doi: 10.1093/annonc/mdx557
33. Feng Y, Zhong M, Liu Y, Wang L, Tang Y. Expression of TIM-3 and LAG-3 in extranodal NK/T cell lymphoma, nasal type. Histol Histopathol. (2018) 33:307–15. doi: 10.14670/HH-11-931
34. Stremitzer S, Sunakawa Y, Zhang W, Yang D, Ning Y, Stintzing S, et al. Variations in genes involved in immune response checkpoints and association with outcomes in patients with resected colorectal liver metastases. Pharmacogenomics J. (2015) 15:521–9. doi: 10.1038/tpj.2015.14
35. Kim HS, Kim JY, Lee YJ, Kim SH, Lee JY, Nam EJ, et al. Expression of programmed cell death ligand 1 and immune checkpoint markers in residual tumors after neoadjuvant chemotherapy for advanced high-grade serous ovarian cancer. Gynecol Oncol. (2018) 151:414–21. doi: 10.1016/j.ygyno.2018.08.023
36. Yoon S, Kang BW, Park SY, Kim HJ, Park JS, Choi GS, et al. Prognostic relevance of genetic variants involved in immune checkpoints in patients with colorectal cancer. J Cancer Res Clin Oncol. (2016) 142:1775–80. doi: 10.1007/s00432-016-2196-2
37. Melaiu O, Mina M, Chierici M, Boldrini R, Jurman G, Romania P, et al. PD-L1 Is a therapeutic target of the bromodomain inhibitor JQ1 and, combined with HLA class I, a promising prognostic biomarker in neuroblastoma. Clin Cancer Res. (2017) 23:4462–72. doi: 10.1158/1078-0432.CCR-16-2601
38. Sunakawa Y, Cao S, Volz NB, Berger MD, Yang D, Parekh A, et al. Genetic variations in immunomodulatory pathways to predict survival in patients with locoregional gastric cancer. Pharmacogenomics J. (2017) 17:528–34. doi: 10.1038/tpj.2016.46
39. Triebel F, Hacene K, Pichon MF. A soluble lymphocyte activation gene-3 (sLAG-3) protein as a prognostic factor in human breast cancer expressing estrogen or progesterone receptors. Cancer Lett. (2006) 235:147–53. doi: 10.1016/j.canlet.2005.04.015
40. Chen J, Chen Z. The effect of immune microenvironment on the progression and prognosis of colorectal cancer. Med Oncol. (2014) 31:82. doi: 10.1007/s12032-014-0082-9
41. Giraldo NA, Becht E, Pagès F, Skliris G, Verkarre V, Vano Y, et al. Orchestration and prognostic significance of immune checkpoints in the microenvironment of primary and metastatic renal cell cancer. Clin Cancer Res. (2015) 21:3031–40. doi: 10.1158/1078-0432.CCR-14-2926
42. Li N, Jilisihan B, Wang W, Tang Y, Keyoumu S. Soluble LAG3 acts as a potential prognostic marker of gastric cancer and its positive correlation with CD8+T cell frequency and secretion of IL-12 and INF-gamma in peripheral blood. Cancer Biomark. (2018) 23:341–51. doi: 10.3233/CBM-181278
43. Lee SJ, Jun SY, Lee IH, Kang BW, Park SY, Kim HJ, et al. CD274, LAG3, and IDO1 expressions in tumor-infiltrating immune cells as prognostic biomarker for patients with MSI-high colon cancer. J Cancer Res Clin Oncol. (2018) 144:1005–14. doi: 10.1007/s00432-018-2620-x
44. Hald SM, Rakaee M, Martinez I, Richardsen E, Al-Saad S, Paulsen EE, et al. LAG-3 in Non-small-cell lung cancer: expression in primary tumors and metastatic lymph nodes is associated with improved survival. Clin Lung Cancer. (2018) 19:249–59. e242. doi: 10.1016/j.cllc.2017.12.001
45. Bottai G, Raschioni C, Losurdo A, Di Tommaso L, Tinterri C, Torrisi R, et al. An immune stratification reveals a subset of PD-1/LAG-3 double-positive triple-negative breast cancers. Breast Cancer Res. (2016) 18:121. doi: 10.1186/s13058-016-0783-4
46. He Y, Yu H, Rozeboom L, Rivard CJ, Ellison K, Dziadziuszko R, et al. LAG-3 Protein expression in non-small cell lung cancer and its relationship with PD-1/PD-L1 and tumor-infiltrating lymphocytes. J Thorac Oncol. (2017) 12:814–23. doi: 10.1016/j.jtho.2017.01.019
47. Woo SR, Turnis ME, Goldberg MV, Bankoti J, Selby M, Nirschl CJ, et al. Immune inhibitory molecules LAG-3 and PD-1 synergistically regulate T-cell function to promote tumoral immune escape. Cancer Res. (2012) 72:917–27. doi: 10.1158/0008-5472.CAN-11-1620
48. Bjoern J, Lyngaa R, Andersen R, Hölmich LR, Hadrup SR, Donia M, et al. Influence of ipilimumab on expanded tumour derived T cells from patients with metastatic melanoma. Oncotarget. (2017) 8:27062–74. doi: 10.18632/oncotarget.16003
49. Pao W, Ooi CH, Birzele F, Ruefli-Brasse A, Cannarile MA, Reis B, et al. Tissue-specific immunoregulation: a call for better understanding of the “immunostat” in the context of cancer. Cancer Discov. (2018) 8:395–402. doi: 10.1158/2159-8290.CD-17-1320
50. Nowicki TS, Hu-Lieskovan S, Ribas A. Mechanisms of resistance to PD-1 and PD-L1 blockade. Cancer J. (2018) 24:47–53. doi: 10.1097/PPO.0000000000000303
51. Sosa MS, Bragado P, Aguirre-Ghiso JA. Mechanisms of disseminated cancer cell dormancy: an awakening field. Nat Rev Cancer. (2014) 14:611–22. doi: 10.1038/nrc3793
52. Kong P, Wang J, Song Z, Liu S, He W, Jiang C, et al. Circulating Lymphocytes, PD-L1 expression on tumor-infiltrating lymphocytes, and survival of colorectal cancer patients with different mismatch repair gene status. J Cancer. (2019) 10:1745–54. doi: 10.7150/jca.25187
53. Yu H, Yang J, Jiao S, Li Y, Zhang W, Wang J. Cytotoxic T lymphocyte antigen 4 expression in human breast cancer: implications for prognosis. Cancer Immunol. Immunother. (2015) 64:853–60. doi: 10.1007/s00262-015-1696-2
54. Fumet JD, Richard C, Ledys F, Klopfenstein Q, Joubert P, Routy B, et al. Prognostic and predictive role of CD8 and PD-L1 determination in lung tumor tissue of patients under anti-PD-1 therapy. Br J Cancer. (2018) 119:950–60. doi: 10.1038/s41416-018-0220-9
55. McGranahan N, Furness AJS, Rosenthal R, Ramskov S, Lyngaa R, Saini SK, et al. Clonal neoantigens elicit T cell immunoreactivity and sensitivity to immune checkpoint blockade. Science. (2016) 351:1463–9.
56. Zhang Y, Cai P, Liang T, Wang L, Hu L. TIM-3 is a potential prognostic marker for patients with solid tumors: a systematic review and meta-analysis. Oncotarget. (2017) 8:31705–13. doi: 10.18632/oncotarget.15954
Keywords: LAG3, cancer, meta-analysis, lymphocyte activation gene 3, disease-free survival, overall survival
Citation: Saleh RR, Peinado P, Fuentes-Antrás J, Pérez-Segura P, Pandiella A, Amir E and Ocaña A (2019) Prognostic Value of Lymphocyte-Activation Gene 3 (LAG3) in Cancer: A Meta-Analysis. Front. Oncol. 9:1040. doi: 10.3389/fonc.2019.01040
Received: 09 July 2019; Accepted: 25 September 2019;
Published: 15 October 2019.
Edited by:
Khashayarsha Khazaie, Mayo Clinic College of Medicine and Science, United StatesReviewed by:
Katerina A. Chlichlia, Democritus University of Thrace, GreeceMasoud H. Manjili, Virginia Commonwealth University, United States
Ashwin Somasundaram, University of Pittsburgh, United States
Copyright © 2019 Saleh, Peinado, Fuentes-Antrás, Pérez-Segura, Pandiella, Amir and Ocaña. This is an open-access article distributed under the terms of the Creative Commons Attribution License (CC BY). The use, distribution or reproduction in other forums is permitted, provided the original author(s) and the copyright owner(s) are credited and that the original publication in this journal is cited, in accordance with accepted academic practice. No use, distribution or reproduction is permitted which does not comply with these terms.
*Correspondence: Alberto Ocaña, alberto.ocana@salud.madrid.org; albertoo@sescam.jccm.es