- 1Research Group of Gastrointestinal Diseases, The Second Affiliated Hospital of Zhengzhou University, Zhengzhou, China
- 2Faculty of Health Science, Nord University, Levanger, Norway
In recent years, several newly identified T helper (TH) cell subsets, such as TH9, TH17, and TH22 cells, and their respective cytokine products, IL-9, IL-17, and IL-22, have been reported to play critical roles in the development of chronic inflammation in the colorectum. Since chronic inflammation is a potent driving force for the development of human colorectal cancer (CRC), the contributions of TH9/IL-9, TH17/IL-17, and TH22/IL-22 in the pathogenesis of CRC have recently become an increasingly popular area of scientific investigation. Extensive laboratory and clinical evidence suggests a positive relationship between these new TH subsets and the growth and formation of CRC, whereas, administration of IL-9, IL-17, and IL-22 signaling inhibitors can significantly alter the formation of colorectal chronic inflammation or CRC lesions in animal models, suggesting that blocking these cytokine signals might represent promising immunotherapeutic strategies. This review summarizes recent findings and currently available data for understanding the vital role and therapeutic significance of TH9/IL-9, TH17/IL-17, and TH22/IL-22 in the development of colorectal tumorigenesis.
Introduction
Colorectal cancer (CRC) is one of the leading causes of high cancer-associated mortality worldwide. According to the colorectal adenoma-carcinoma sequence theory, most CRCs develop from pre-existing adenomatous lesions through a multistep process with the accumulation of genetic, molecular and histological changes (1, 2). This process is significantly influenced by host immunity (3–5). T-helper (TH) 1 immunity is believed to be the most important host immune mechanism for keeping pre-neoplastic/neoplastic cells in check (6–8). To escape host immune surveillance, however, pre-neoplastic adenoma cells can develop diverse strategies to suppress antitumor immunity.
Studies in recent years have suggested that diverse TH subsets play a crucial role in the processing and maintenance of chronic inflammation (9) and tumorigenesis (10), in which their cytokine products play an essential role that modulates the development of the inflammatory microenvironment provoked by tumorigenesis and affect tumor initiation/progression (3, 11, 12). Therefore, there is great interest in studying the role of TH subsets and their cytokine products in CRC initiation/progression. Indeed, many reports have observed notably altered immune cell densities and cytokine profiles during CRC development (13–21), in which significantly increased CD4 and CD8-positive T lymphocyte populations are observed (22–24), along with decreased expression levels of TH1 cytokines and increased expression levels of TH2 cytokines in patients with CRC (7, 8, 25, 26). Recent studies have also revealed that altered cytokine profiles are correlated with disease stage and prognosis in patients with CRC (25, 27–29). Furthermore, both in vivo and in vitro studies have demonstrated that blocking cytokine signaling, as seen in interleukin (IL)-6 and IL-17 studies, significantly suppresses cell growth, proliferation, progression, and metastasis in CRC (21, 30). Therefore, ample evidence suggests that cytokines are not only key contributors to the pathogenesis of CRC (18, 20, 31–34) but also potential immunotherapeutic targets for the treatment and prevention of CRC (35, 36).
Recently, several newly identified TH subsets, for example, TH9, TH17, and TH22, have been reported to be associated with the development of chronic inflammation and carcinogenesis. The cytokine products of these subsets, IL-9, IL-17, and IL-22, respectively, are potent proinflammatory mediators and greatly contribute to the initiation and maintenance of colorectal inflammatory disorders (9, 37–39). Emerging evidence suggests that these cytokines are also involved in the development and progression of CRC, as administration of cytokine inhibitors significantly suppressed the development of colorectal inflammation and subsequent CRC occurrence in mice. Therefore, an improved understanding of the immunopathogenesis of TH subsets and their cytokine products will be helpful for designing novel translational/targeted approaches to CRC (40).
In view of the importance and increasing data about TH9, TH17, and TH22 cells and their associated cytokines in the initiation and development of CRC, here, we review recent findings, with an emphasis on the function of TH9/IL-9, TH17/IL-17, and TH22/IL-22 in the pathogenesis of CRCs, and discuss the therapeutic significance of these main cytokines in the prophylaxis of CRC.
Tumor-Infiltrating Lymphocytes (TILs) and Chromosomal Instability in Patients With CRC
TILs in colorectal adenoma/CRC have been intensively studied. The results suggest that significantly increased densities of TILs are observed in both the tumor stroma and adenoma/CRC epithelium (41, 42), and have clinical significance in predicting prognosis and chemotherapeutic outcomes in patients with CRC (43–46). Phenotypic analysis revealed that these TILs are mostly CD4-positive and CD8-positive cells. CD4-positive TILs are predominantly TH cells localized within the tumor stroma, and CD8-positive TILs are cytotoxic T lymphocytes (CTLs) that infiltrate both the tumor stroma and adenoma/CRC epithelium (23, 47–50). In addition, clinical molecular analysis revealed that ~85% of CRCs may present with chromosomal instability, while 15% display DNA mismatch repair (MMR) (51, 52). Therefore, another issue worthy of consideration is the influence of DNA microsatellite instability (MSI) on the presentation pattern of lymphocyte infiltration in CRC. Indeed, Boissière-Michot et al. (53) have reported that CRCs with MSI exhibited increased densities of CD3-positive, CD8-positive, CD45RO-positive, and T-bet-positive TILs compared with those of microsatellite stable (MSS) CRCs, whereas, the density of FoxP3-positive regulatory T cells (Tregs) was not significantly different between the two groups (53). Lee et al. examined TILs in CRCs with MSI and found that CD8-positive TILs, but not CD4-positive TILs, were significantly increased in both tumor cell niches and the tumor stroma in CRCs with MSI (54). Similarly, Matsutani et al. (23) recently showed that the density of CD8-positive TILs is associated with MMR status in CRC and intratumor CD8-positive TILs in the invading margins in randomly selected fields, and tends to be higher in MMR-deficient patients than in MMR-proficient patients (23). More recently, several studies have shown that chromosomal instability patterns significantly influence the infiltration of CD8-positive lymphocytes, survival, and therapeutic response in patients with CRC (55–57).
Collectively, these results demonstrate that chromosomal instability status in patients with CRC may significantly influence TIL infiltration.
The Role of TH9/IL-9, TH17/IL-17, and TH22/IL-22 in the Development of CRC
TH9/IL-9
TH9, a novel subset of TH cells that primarily produce IL-9 (58), has recently been shown to be involved in the pathogenesis of inflammatory bowel disease (IBD) (59, 60). The production of IL-9 by TH cells is stimulated by IL-4, IL-21, and transforming growth factor (TGF)-β and inhibited by interferon (IFN)-γ (61). Transcriptional studies have revealed that the production of IL-9 by TH9 cells is regulated by PU.1 (an ETS family transcription factor) (62), and there is ample evidence to suggest that the TH9 cytokine IL-9 could be a strong proinflammatory factor in the induction of experimental colitis (38, 63–65). An animal study showed that TH9 cells labeled with PU.1 were markedly increased in the inflamed mucosa (lamina propria) of dextran sulfate sodium (DSS)-induced colitis mice (66). In human ulcerative colitis (UC) specimens, we found that increased populations of PU.1-positive TH9 cells were predominantly located in the lamina propria, whereas, intraepithelial TH9 cells were present at very low densities and were only occasionally observed (66). However, it is worth noting that PU.1 is not a lineage-specific transcription factor for IL-9 induction in TH9 cells, as it can also be expressed in other subsets under certain circumstances. For example, it has been reported that PU.1 is expressed in B cells (67). In addition to PU.1, ETV5, another ETS family member, is an essential transcriptional factor for TH9 cells, as deficiency of both ETV5 and PU.1 in T cells results in markedly reduced induction of IL-9 (68). Therefore, a range of transcription factors might be involved in the differentiation of TH9 cells (69). Furthermore, a number of clinical studies have revealed that serum levels of IL-9 in patients with UC or Crohn's disease (CD) are higher than those in controls, and increased levels of IL-9 are significantly associated with inflammatory activity in the bowel in patients with UC or CD (37, 70, 71). Tian et al. showed that the involvement of IL-9 in the pathogenesis of UC occurs through activation of the Stat3/SOCS3 signaling pathway (72). These data support the notion that the activation of the TH9 subset and its cytokine product, IL-9, participates in the regulation of inflammatory processes in the bowel. Since chronic inflammation, as seen in IBD, may predispose patients to CRC development, the role of the proinflammatory cytokine IL-9 in the development of CRC has begun to attract increasing attention. Indeed, published studies have explored the role of IL-9 in different types of cancers, and both antitumor and protumor effects have been reported (73–75). Rivera Vargas et al. demonstrated that autophagy controls IL-9 secretion by TH9 cells and that selective autophagy targets PU.1 for degradation in CD4 T cells, resulting in the negative regulation of TH9 homeostasis and antitumor immunity (73, 76). Two clinical studies performed in southern China reported that expression levels of IL-9, in both the plasma and CRC tissues, are lower in patients with CRC compared to levels in healthy controls and are significantly associated with TNM staging. CRC patients with advanced stages have reduced levels of IL-9 compared to those with early stages (77, 78). Liu et al. demonstrated that IL-9 induces the conversion of Tregs to TH9 cells, subsequently inhibiting tumor growth in the CT26 colon cell line (79). Wang et al. reported a similar finding in Chinese patients with CRC. They found that the expression levels of IL-9 protein and messenger RNA were lower than those in the controls and correlated with TNM staging, Dukes staging, lymph node metastasis, and good prognosis. In vivo, IL-9 overexpression significantly inhibited tumor growth and resulted in a longer survival time in a mouse subcutaneous allograft model (80). Moreover, an ectopically expressed membrane-bound form of IL-9 induces an immunostimulatory effect that suppresses the growth of CT26 colon cancer cells (81). These studies seem to suggest that TH9 cells have an antitumor effect that largely depends on their ability to secrete IL-9 and may influence tumor growth by enhancing immune responses (73).
In contrast to the above findings, other studies have described a protumor effect of IL-9. We have more recently demonstrated that a population of IL-9-positive cells and protein expression levels of IL-9 are greatly increased in mouse colitis-associated cancer (CAC) tissues, compared to those of control mouse tissues (82). Tian et al. examined the expression levels of IL-9 in 12 pairs of CAC tissues and adjacent non-tumor tissues by immunohistochemistry, and found that the expression of IL-9 in CAC tissues was markedly higher than that in adjacent tissues (83). In vivo, this study showed that IL-9 overexpression in RKO and Caco-2 colon cancer cell lines results in an increasing proliferation rate that occurs through upregulating expression of c-Myc and cyclin D1 (83). Furthermore, a study from Poland demonstrated that serum levels of IL-9 in 104 CRC patients were significantly higher than those in controls (84), in contrast to reports from southern China (77, 78). Hoelzinger et al. recently used IL-9-deficient mice to investigate the effects of IL-9 in multiple models of breast and colon cancer development. These authors found that eliminating endogenous IL-9 enabled sensitization of host T cells to tumors, leading to early rejection without the requirement for vaccines or immunomodulatory therapies. Notably, IL-9-deficient mice acquired immunologic memory, which actively protected them from residual disease and tumor re-challenge, an effect that is linked to the activation of CD8-positive T cells (74).
Collectively, the current literature has revealed that TH9/IL-9 exhibit both antitumor and protumor effects (Figure 1). These controversial results could be due to the distinct experimental models, techniques and tumorigenesis conditions used in different studies. Moreover, contradictory data may also stem from differential effects of IL-9 on immune (antitumor) vs. cancer (protumor) cells. Therefore, the effect of TH9/IL-9 in the development of CRC is still an issue of debate, and much work remains to be done.
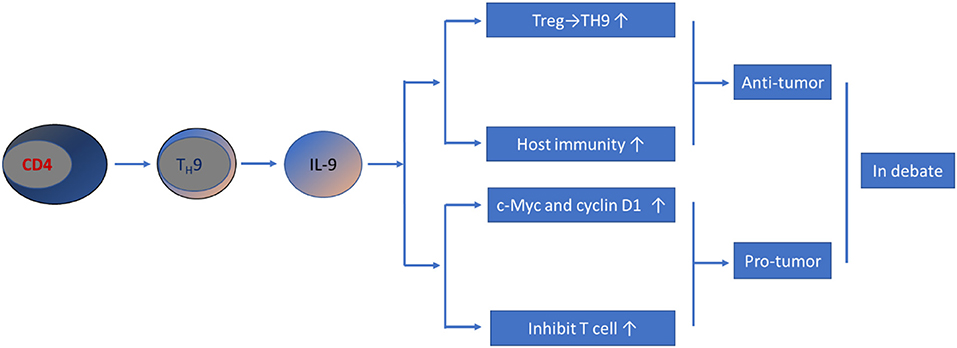
Figure 1. Schematic representation of TH9/IL-9's role in CRC pathogenesis. Current data have yielded controversial results; both pro-tumor and anti-tumor effect have been reported.
TH17/IL-17
The IL-17-expressing TH cell subset (known as TH17 cells) is another TH cell subset related to colorectal inflammation and tumorigenesis (39). Studies suggest that the differentiation of TH17 cells is stimulated by a set of upstream cytokines, including IL-1β, IL-6, IL-21, IL-23, and TGF-β, and the main cytokine products of this cell type are IL-17A and IL-17F (39). It should be mentioned that the intestine is a site of origin for TH17 cells, and whether they function as non-pathogenic TH17 cells promoting homeostasis or pathogenic TH17 cells involved in infection and autoimmunity is dependent on the upstream stimulator. TGF-β stimulation skews the differentiation of TH17 cells to a non-pathogenic phenotype that promotes homeostasis, and IL-23 accelerates the differentiation of TH17 cells toward a pathogenic phenotype that is involved infection and autoimmunity. Some previous studies have shown an antitumor effect of IL-17. For instance, Kryczek et al. reported that subcutaneous injection of the MC38 murine colon cancer cell line into IL-17-deficient mice resulted in faster growth and increased lung metastasis foci compared to that of wildtype control mice (85). Previous studies have demonstrated that the antitumor effect of IL-17 in various cancer types is related to enhanced recruitment and activity of lymphocytes, natural killer (NK) cells, and DCs into the tumor site and production of the antitumor cytokine IFN-γ (86–89). Lin et al. showed that CRC patients with higher IL-17 levels tend to have a better prognosis than those with lower IL-17 levels (90). However, the majority of published studies suggest that TH17/IL-17 has profound effects on triggering and amplifying the inflammatory process and tumorigenesis in the colorectum (21, 91, 92). Considerable evidence suggests that increased expression profiles of the TH17 cytokine product, IL-17A, are associated with the production of the proangiogenic factor vascular endothelial growth factor (VEGF) and poor prognosis in patients with CRC (93). The profile of circulating and intra-tumoral TH17 cells in patients with colon cancer, with respect to tumor staging, was analyzed. The results revealed that the percentage of TH17 cells in both the peripheral blood and cancer tissues, assessed by multicolor flow cytometry, was significantly increased in cancer patients compared to that of healthy controls or adjacent non-tumor tissues, respectively. In addition, the percentage of TH17 cells in both the peripheral blood and cancer tissues was associated with disease stage, and it was higher in patients with advanced stages than in those with early stages (94). Furthermore, functional studies identified that the type A receptor (IL-17RA) is a critical mediator of IL-17 in promoting colorectal tumorigenesis (95). We have, for the first time, examined IL-17A dynamics along the human colorectal adenoma-carcinoma spectrum (31) and have found that the expression of IL-17A, at both the mRNA and protein levels, was significantly increased in the adenoma stage and persisted to the CRC stage. Since the differentiation of TH17 cells is stimulated by a set of cytokines, IL-1β and IL-6, which are important for early stages, and IL-23, which is important for late stages of development and the expansion of TH17 cells, we examined these stimulating factors. The data showed that TH17 differentiation-stimulating factors IL-1β, IL-6, and IL-23, but not TGF-β, were significantly increased in a similar expression pattern as IL-17 along the colorectal adenoma-carcinoma sequence, suggesting activated TH17 differentiation and elevated IL-17 production throughout this sequence. Interestingly, we found that increased IL-17 expression levels was associated with an increased dysplasia grading score in adenomas, which is one of the most important histological parameters in assessing the neoplastic progression of an adenoma toward a CRC. Analysis of IL-17A gene variants revealed that heterozygous rs10484879 was associated with increased disease risk, while the rs3748067 genotype was associated with a reduced risk of CRC (96). Samiei et al. revealed that the IL-17A197AA genotype was significantly associated with an increased CRC risk in Malaysian patients, compared with those with the GG and AG genotypes (97). These data suggest that IL-17A increases susceptibility to CRC. More recently, the contribution of IL-17 to chemotherapeutic agent resistance in CRC has been investigated. Cisplatin-based anticancer therapy is an important chemotherapeutic strategy for the treatment of CRC. Sharp et al. reported that elevated systemic levels of the TH17 cytokines, IL-17F and IL-23, were associated with stage IV colon cancer (98). Furthermore, the effect of IL-17 on the induction of chemoresistance in CRC is mediated through targeting phosphorylated protein kinase B (p-Akt), the apoptosis regulators Bax and Bcl-2 and the serine/threonine-protein kinase mTOR (99).
Despite general advances in diagnosis and treatment, the long-term survival of CRC remains low, except when early curative surgery is performed. The prognostic significance of TH17/IL-17 in human CRC has been evaluated. Le Gouvello et al. have shown that increased expression of IL-17 in CRC tumor tissues is associated with proficient MMR status, indicating poor prognosis in patients with CRC (100). Other studies have also confirmed that increased levels of IL-17 are associated with aggressive features and poor prognosis in CRC (93, 101). Moreover, a clinical study revealed that CRC patients with high expression of the TH17 cluster and a high density of IL-17-positive cells in situ may have a shorter disease-free survival time and early relapse after primary CRC tumor surgical resection (24). Since systemic metastasis has often already occurred at diagnosis, approximately half of CRC patients ultimately die due to relapse and metastasis after resection of primary tumors (102). Therefore, recent studies have explored the influence of TH17/IL-17 on CRC metastasis. The promoting effect of IL-17 on colorectal tumorigenesis has been reported in mouse CRC models (103). The data showed that the development of CRC is facilitated via the activation of TH17 cells, whereas, blocking IL-17A signaling significantly suppressed hyperplastic and neoplastic lesion formation (103), suggesting that activation of TH17/IL-17 plays an important role in the formation of premalignant lesions and the establishment of CRC lesions. Thus, targeting TH17/IL-17 signaling may represent a promising approach for future CRC immunotherapy (104).
In addition to IL-17A, IL-17F has been shown to be another potential factor involved in the development of CRC. Tong et al. have shown a protective effect of IL-17F in the development of CRC. They found decreased tumor growth of IL-17F-transfected HCT116 cells compared to that of mock transfectants when transplanted into nude mice. However, increased colonic tumor numbers and tumor areas were detected in IL-17F-deficient mice compared to those of wildtype mice after colon cancer induction (105). Furthermore, Nemati et al. found that the IL-17F T7488 allele is associated with a decreased risk of CRC and tumor progression, whereas, the AG genotype of the IL-17A G197A SNP is associated with an increased risk of CRC (106). However, Chae and Bothwell reported that IL-17F deficiency significantly inhibits spontaneous intestinal tumorigenesis in the small intestine of Apc(Min/+) mice, probably through decreased IL-1β, Cox-2, and IL-17 receptor C (IL-17RC) expression and decreased infiltration of immune cells in the lamina propria (107). The role of IL-22 in the pathogenesis of CRC is also somewhat controversial (see TH22/IL-22 section). In addition, TH17 cells under certain circumstances can coproduce IL-9, IFN-γ, or IL-10. The function of these cytokines in the development of CRC might be significantly different. Finally, some TH17 cytokines can be made by many TH subsets, and TH17 differentiation-stimulating factors may also regulate other TH subsets under certain conditions. This makes the cytokine network in human tumors very complex.
In summary, current evidence has shown that IL-17 promotes metastasis in CRC. Possible mechanisms by which this occurs include the following: (1) IL-17 stimulates the activation of metastasis-related genes, such as tumor necrosis factor-α (TNF-α), nuclear factor kappa B (NF-κB), CC chemokine receptor 6 (CCR6), matrix metalloproteinase (MMP)-2, MMP-7, MMP-9, and MMP-13 (108–111). (2) IL-17 potentially enhances angiogenesis by increasing the production of VEGF (93, 104). Further studies revealed that IL-17 does not directly stimulate the growth of vascular endothelial cells but strongly induces the migration and cord formation of endothelial cells (92, 112). (3) IL-17 is capable of remodeling the CRC stroma (113). (4) IL-17 promotes the production of myeloid-derived suppressor cells that create a favorable milieu for CRC development and progression (114–117). Therefore, the involvement of TH17/IL-17 in the pathogenesis of CRC occurs through a mixed pathway (Figure 2).
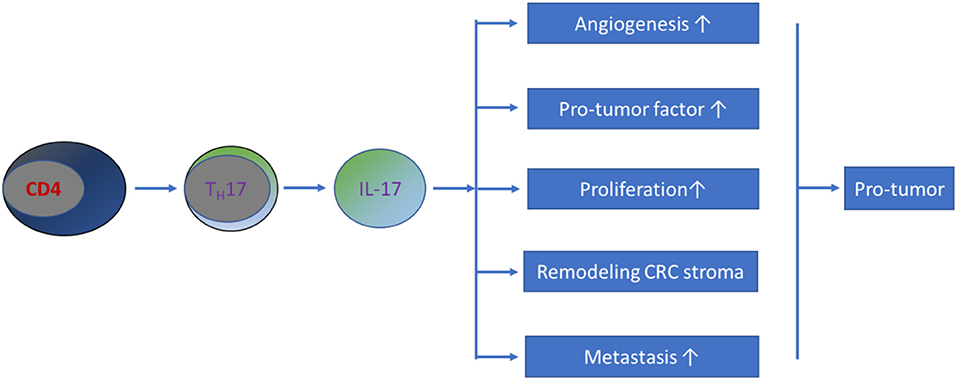
Figure 2. Schematic representation of TH17/IL-17's role in CRC pathogenesis. Most current studies have demonstrated that TH17/IL-17 exhibits a pro-tumor effect on the development of CRC through a mixture of mechanisms.
TH22/IL-22
TH22 cells compose another novel T cell subset. RORγt acts as a positive transcription factor and T-bet acts as a negative transcription factor for TH22 cell differentiation (118). In addition, AhR has also been considered a transcription factor for IL-22 produced by TH22 cells (119). TH22 cells produce cytokines, such as IL-22, IL-26, and IL-33. Although it is not clear whether TH22 cells are a separate subset or derived from TH17 cells, given that there is still debate about whether IL-22 is also a TH17 cytokine, many observations obtained over the past few years have indicated that numerous factors, including IL-1β, IL-6, IL-21, and IL-23, may stimulate the production of IL-22 in activated TH22 cells (118, 119). The role of IL-22 in modulating the progression of inflammation, as seen in IBD, has been primarily studied (120, 121). However, these results are somewhat controversial. One study reported that UC patients exhibited significantly reduced numbers of IL-22-positive cells in actively inflamed tissues, compared with the numbers in both non-inflamed tissues and normal tissues from healthy controls (9). Another study conducted in patients with CD reported that IL-22 protects the intestinal barrier by enhancing intestinal epithelial cell migration in CD (122), which was supported by the findings that anti-TNF-α therapy in patients with CD results in increased IL-22 production by CD4-positive T cells and enhanced repair of damaged mucosa (123). Sugimoto et al. reported that IL-22 gene delivery elicited rapid attenuation of local intestinal inflammation by enhancing Stat3 activation within colonic epithelial cells in mice (124). However, other studies have shown the opposite relationship between TH22/IL-22 and chronic inflammation in the colorectum. Analysis of IL-22 levels and circulating TH22 cells in UC showed that UC patients had higher levels of IL-22 and TH22 cells than those of healthy controls, and both features were associated with disease severity (125, 126). In addition, IL-22 antibody administration significantly reduced colorectal inflammation in DSS-induced colitis mice compared with that of control mice (127). Therefore, current data from most studies suggest that TH22/IL-22 might participate in the development and modulation of colorectal inflammation.
Regarding the role of TH22/IL-22 in cancers, evidence from early studies revealed that IL-22 promotes the growth of tumor cells in many types of cancers, including lung adenocarcinoma and hepatocellular carcinoma (128, 129). In patients with gastric cancer, dysregulation of circulating TH22 cell frequency and IL-22 expression levels have been demonstrated, and were associated with advanced tumor features and patient survival (130, 131). Regarding the role of TH22/IL-22 in CRC, in vitro studies have shown that IL-22 enhances nitric oxide synthase expression in colon cancer cells, significantly contributing to conversion to nitrites, which are associated with colonic inflammation and carcinogenesis (132). Subsequent studies have shown that IL-22 has a direct proliferative effect on colonic epithelial cells (133). Sun et al. revealed that coculture of single cells, isolated from colon cancer tissues with recombinant IL-22 for 24 h, resulted in a significantly increased proliferation rate, which could be blocked by an anti-IL-22 antibody (134). Furthermore, IL-22 potentially stimulates intestinal epithelial cells to secrete IL-10, a main contributor to the formation of an immunosuppressive milieu in CRC (135). IL-22 has also consistently been shown to be protumor in CRC animal models. The results obtained from both in vivo and in vitro models illustrated that IL-22 is associated with CRC progression through activating the Stat3 pathway (133, 134, 136, 137). Various studies in recent years have attempted to clarify the promoting effect of IL-22 in the pathogenesis of CRC. The results showed that BALB/c nude mice transplanted subcutaneously with RKO colon cancer cells tended to have faster tumor growth after intraperitoneal injection of IL-22 every other day, compared to that of control mice (136). Recently, Wang et al. revealed that IL-22 is associated with the development of dysplasia in a murine model of CAC (138). By using IL-22BP-deficient mice, Huber et al. showed that the soluble receptor of IL-22, IL-22 binding protein (IL-22BP), has a crucial role in controlling tumorigenesis and epithelial cell proliferation in the mouse colon (133). In support of the hypothesis that TH22 is involved in the development of CRC, Doulabi et al. reported that increased populations of intratumoral TH17, TH22, and CD4-positive T cells, coproducing IL-17/IL-22 in patients with colon cancer, were observed in tumor tissues (94). This result is in contrast with the results of a previous study that reported markedly reduced percentages of TH22 cells and IL-22 levels in CRC patients, compared with those of healthy controls that were negatively associated with the pathological stages of CRC (139). Another clinical study demonstrated that the percentage of TH22 cells in the CD4-positive T cell subset was significantly higher in CRC tissues, compared to that of para-tumor tissues, as determined by flow cytometry in patients with CRC (136). In addition, IL-22 genetic polymorphisms have been shown to be a risk factor for colon cancer (140), and elevated serum IL-22 levels correlate with chemoresistance in patients with CRC (141). Furthermore, both IL-22 and its functional transmembrane receptor (IL-22R1) have been found to be significantly expressed in CRC epithelial and tumor stromal cells (142). Taken together with the above findings, most current data suggest a promoting effect of TH22/IL-22 on the development of CRC (see Figure 3), making it an attractive target for anticancer therapy.
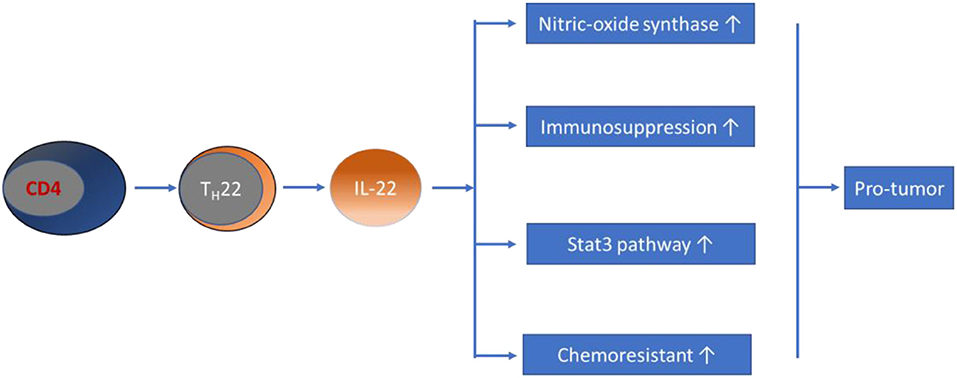
Figure 3. Schematic representation of TH22/IL-22's role in CRC pathogenesis. Evidence obtained from most studies has suggested that TH22/IL-22 promotes the development of CRC through diverse pathways.
Potential Therapeutic Significance of TH9/IL-9, TH17/IL-17, and TH22/IL-22 in CRC
Because of the current lack of effective immunotherapies for the treatment of CRC, the search for novel immunotherapeutic targets has become critically important (36, 143). Strong evidence now suggests that chronic inflammation is a potent driving force for tumor development, in which diverse TH cells and their cytokine products play essential roles (144, 145). It is therefore hypothesized that TH cells and their cytokine network may represent potential therapeutic targets for the treatment of human cancer (14, 146–151). Immunotherapy against various cancer types, including CRC, has been well-reviewed (146, 152). These outstanding reviews have indicated that current immunotherapy strategies, including anti-cytokine antibodies, immune adjuvants, and vaccines, immune checkpoint inhibitors and immune cell-based therapies, can potentially enhance host antitumor immune function and prolong patient survival time in human cancers (152, 153).
As the above findings indicated that TH9 and its cytokine product IL-9 are associated with the development of chronic inflammation in the colorectum, additional studies further analyzed the therapeutic effect of blocking IL-9 signaling in a colitis mouse model (66). We were able to show that blocking IL-9 signaling by intraperitoneal administration of IL-9 antibody rapidly ameliorates colorectal inflammation in DSS-induced colitis mice (66). However, the role of TH9/IL-9 in the development of CRC remains unclear. To the best of our knowledge, studies examining the therapeutic effect of blocking IL-9 signaling in the development of CRC remains to be investigated. Furthermore, several studies have looked at the therapeutic effect of blocking IL-17A in the treatment and prevention of CRC (92, 110–112). Numasaki et al. have shown that IL-17 promotes tumor cell growth in vivo by enhancing angiogenesis in mice with MCA205/IL-17 tumors, while angiogenic activity was markedly suppressed by a neutralizing IL-17 monoclonal antibody (112). We previously demonstrated that administration of an IL-17A antibody strongly suppresses the development of DSS/DMH-induced CRC in mice (20). These findings have highlighted a key role of IL-17 in CRC development. Currently, secukinumab, ixekizumab, and brodalumab, which are biological agents that target IL-17 signaling, are being studied and have successfully passed phase III clinical trials for rheumatoid arthritis and psoriatic arthritis. These antibodies may represent new options for potential CRC treatments; therefore, it would be interesting to evaluate the therapeutic effect of these antibodies alone or combined with chemotherapy in treating relapsed or metastatic CRCs (104). Since the TH22-related cytokine IL-22 has been reported to promote tumorigenesis in distinct cancer entities, including lung, ovarian, breast, gastric, pancreatic, and colon cancers, the IL-22-IL-22R1 pathway has become an attractive target for anticancer therapy (154–156). An examination of the role of IL-22 in lung adenocarcinoma has shown that IL-22 in K-ras-driven lung cancer mediates tumor proliferation and remodeling of the tumor microenvironment in a mouse model (129). Since a previous study demonstrated that IL-22 has a crucial role in controlling tumorigenesis and epithelial cell proliferation in the colon (133), IL-22 may represent an attractive therapeutic target. Sun et al. examined the effect of IL-22 on the proliferation of single cells from colon cancer tissues by coculture with or without recombinant IL-22 for 24 h. The results showed that recombinant IL-22 stimulates colon cell proliferation, while anti-IL-22 blocks this effect (134). Another study tested the efficacy of a novel IL-22BP-based colon cancer gene therapy strategy (157). By delivering the IL-22BP gene with a newly developed non-viral gene vector, DOTAP-modified mPEG-PCL micelles (DMP), the inhibitory efficacy of the DMP/IL-22BP complex on mouse colon cancer was examined via intraperitoneal administration. These results showed that the DMP/IL-22BP complex blocked IL-22 signaling and exhibited a significant inhibitory effect on cancer cell growth both in vitro and in vivo. These anticancer mechanisms may be related to induction of apoptosis, enhanced infiltration of lymphocytes in tumor tissues and inhibition of angiogenesis. The results suggest that blocking IL-22 signaling may represent a promising strategy for CRC cancer gene therapy (157). Administration of a specific antibody is one of the simplest and most efficient methods to block cytokine signaling, and one study demonstrated that antibody-based targeted delivery against IL-22 in DSS-induced colitis mice resulted in more rapid recovery from clinical symptoms and improved colon morphology compared to controls (127). Currently, the delivery of IL-22 antibodies in a CRC animal model has not been tested, and evaluation of therapeutic efficacy remains unknown.
Concluding Remarks
Current findings suggest a pivotal role of the TH subsets, TH17 and TH22, in the pathogenesis of CRC. In this context, their cytokine products have been identified as key contributors in CRC tumorigenesis through several pathways, such as stimulating proliferation, enhancing angiogenesis, recruiting immune suppressor cells, and remodeling tumor stroma. Therefore, strategies aimed at suppressing these cytokine signals as a new immunotherapeutic option for the treatment and prevention of CRC are of key interest. Regarding the role of TH9 and its cytokine product IL-9 in the pathogenesis of CRC, it is rather diverse as it can have a protumor function as well as antitumor characteristics. Therefore, the contribution of TH9/IL-9 in CRC development remains an issue of debate. It is hoped that further studies will eventually elucidate the precise mechanisms and molecular characterization of signaling pathways involved in TH subset cytokine actions in CRC, which will in turn help to improve immunotherapeutic approaches.
Author Contributions
The author confirms being the sole contributor of this work and has approved it for publication.
Funding
This project was supported by the Innovation Scientists and Technicians Troop Construction Projects of Henan Province (CXTD20150009), the Nature and Science Foundation of Henan Province (Program No. 182300410326), and the Medical Research Program, Northern Norway Regional Health Authority (Program No. SFP-44-04).
Conflict of Interest
The author declares that the research was conducted in the absence of any commercial or financial relationships that could be construed as a potential conflict of interest.
Abbreviations
CAC, colitis associated cancer; CCR, CC chemokine receptor; CD, Crohn's disease; CRC, colorectal cancer; DMH, 1,2-dimethylhydrazine; DMP, the DOTAP modified mPEG-PCL micelles; DSS, dextran sulfate sodium; IBD, inflammatory bowel disease; IFN-γ, interferon γ; IL, interleukin; IL-17RA, IL-17 type A receptor; IL-22BP, IL-22 binding protein; MMP, matrix metalloproteinase; MMR, mismatch repair; MSI, microsatellite instable; NF-κB, nuclear factor kappa B; p-Akt, phosphorylated-protein kinase B; TGF-β, transforming growth factor β; TH, T helper; TIL, tumor-infiltrating lymphocyte; TNF-α, tumor necrosis factor α; Treg, regulatory T cell; UC, ulcerative colitis; VEGF, vascular endothelial growth factor.
References
1. Fearon ER, Vogelstein B. A genetic model for colorectal tumorigenesis. Cell. (1990) 61:759–67. doi: 10.1016/0092-8674(90)90186-I
2. Cui G, Xu G, Zhu L, Pang Z, Zheng W, Li Z, et al. Temporal and spatial changes of cells positive for stem-like markers in different compartments and stages of human colorectal adenoma-carcinoma sequence. Oncotarget. (2017) 8:45311–22. doi: 10.18632/oncotarget.17330
3. Shi Y, Li Z, Zheng W, Liu X, Sun C, Laugsand JB, et al. Changes of immunocytic phenotypes and functions from human colorectal adenomatous stage to cancerous stage: update. Immunobiology. (2015) 220:1186–96. doi: 10.1016/j.imbio.2015.06.003
4. Croci DO, Zacarias Fluck MF, Rico MJ, Matar P, Rabinovich GA, Scharovsky OG. Dynamic cross-talk between tumor and immune cells in orchestrating the immunosuppressive network at the tumor microenvironment. Cancer Immunol Immunother. (2007) 56:1687–700. doi: 10.1007/s00262-007-0343-y
5. McLean MH, Murray GI, Stewart KN, Norrie G, Mayer C, Hold GL, et al. The inflammatory microenvironment in colorectal neoplasia. PLoS ONE. (2011) 6:e15366. doi: 10.1371/journal.pone.0015366
6. Berghella AM, Pellegrini P, Del Beato T, Marini M, Tomei E, Adorno D, et al. The significance of an increase in soluble interleukin-2 receptor level in colorectal cancer and its biological regulating role in the physiological switching of the immune response cytokine network from TH1 to TH2 and back. Cancer Immunol Immunother. (1998) 45:241–9. doi: 10.1007/s002620050439
7. Cui G, Goll R, Olsen T, Steigen SE, Husebekk A, Vonen B, et al. Reduced expression of microenvironmental Th1 cytokines accompanies adenomas-carcinomas sequence of colorectum. Cancer Immunol Immunother. (2007) 56:985–95. doi: 10.1007/s00262-006-0259-y
8. Pellegrini P, Berghella AM, Del Beato T, Cicia S, Adorno D, Casciani CU. Disregulation in TH1 and TH2 subsets of CD4+ T cells in peripheral blood of colorectal cancer patients and involvement in cancer establishment and progression. Cancer Immunol Immunother. (1996) 42:1–8. doi: 10.1007/s002620050244
9. Leung JM, Davenport M, Wolff MJ, Wiens KE, Abidi WM, Poles MA, et al. IL-22-producing CD4+ cells are depleted in actively inflamed colitis tissue. Mucosal Immunol. (2014) 7:124–33. doi: 10.1038/mi.2013.31
10. Pages F, Galon J, Dieu-Nosjean MC, Tartour E, Sautes-Fridman C, Fridman WH. Immune infiltration in human tumors: a prognostic factor that should not be ignored. Oncogene. (2010) 29:1093–102. doi: 10.1038/onc.2009.416
11. Gerlach K, Weigmann B. The dichotomous function of interleukin-9 in cancer diseases. J Mol Med. (2019) 97:1377–83. doi: 10.1007/s00109-019-01826-5
12. Fantini MC, Pallone F. Cytokines: from gut inflammation to colorectal cancer. Curr Drug Targets. (2008) 9:375–80. doi: 10.2174/138945008784221206
13. Csiszar A, Szentes T, Haraszti B, Balazs A, Petranyi GG, Pocsik E. The pattern of cytokine gene expression in human colorectal carcinoma. Pathol Oncol Res. (2004) 10:109–16. doi: 10.1007/BF02893465
14. Mager LF, Wasmer MH, Rau TT, Krebs P. Cytokine-induced modulation of colorectal cancer. Front Oncol. (2016) 6:96. doi: 10.3389/fonc.2016.00096
15. Yuan A, Steigen SE, Goll R, Vonen B, Husbekk A, Cui G, et al. Dendritic cell infiltration pattern along the colorectal adenoma-carcinoma sequence. Apmis. (2008) 116:445–56. doi: 10.1111/j.1600-0463.2008.00879.x
16. Cui G, Yuan A, Goll R, Vonen B, Florholmen J. Dynamic changes of interleukin-8 network along the colorectal adenoma-carcinoma sequence. Cancer Immunol Immunother. (2009) 58:1897–905. doi: 10.1007/s00262-009-0702-y
17. Yuan A, Chen JJ, Yao PL, Yang PC. The role of interleukin-8 in cancer cells and microenvironment interaction. Front Biosci. (2005) 10:853–65. doi: 10.2741/1579
18. Cui G, Yuan A, Sun Z, Zheng W, Pang Z. IL-1beta/IL-6 network in the tumor microenvironment of human colorectal cancer. Pathol Res Pract. (2018) 214:986–92. doi: 10.1016/j.prp.2018.05.011
19. Cui G, Yang H, Zhao J, Yuan A, Florholmen J. Elevated proinflammatory cytokine IL-17A in the adjacent tissues along the adenoma-carcinoma sequence. Pathol Oncol Res. (2015) 21:139–46. doi: 10.1007/s12253-014-9799-1
20. Qi H, Yang H, Xu G, Ren J, Hua W, Shi Y, et al. Therapeutic efficacy of IL-17A antibody injection in preventing the development of colitis associated carcinogenesis in mice. Immunobiology. (2015) 220:54–9. doi: 10.1016/j.imbio.2014.09.002
21. Shi Y, Lin H, Cui J, Qi H, Florholmen J, Liu Z, et al. The role of interleukin-17A in colorectal tumorigenesis. Cancer Biother Radiopharm. (2013) 28:429–32. doi: 10.1089/cbr.2012.1396
22. Trajkovski G, Ognjenovic L, Jota G, Hadzi-Manchev D, Trajkovska V, Volcevski G, et al. Tumour lymphocytic infiltration, its structure and influence in colorectal cancer progression. Open Access Maced J Med Sci. (2018) 6:1003–9. doi: 10.3889/oamjms.2018.279
23. Matsutani S, Shibutani M, Maeda K, Nagahara H, Fukuoka T, Iseki Y, et al. Verification of the methodology for evaluating tumor-infiltrating lymphocytes in colorectal cancer. Oncotarget. (2018) 9:15180–97. doi: 10.18632/oncotarget.24612
24. Tosolini M, Kirilovsky A, Mlecnik B, Fredriksen T, Mauger S, Bindea G, et al. Clinical impact of different classes of infiltrating T cytotoxic and helper cells (Th1, th2, treg, th17) in patients with colorectal cancer. Cancer Res. (2011) 71:1263–71. doi: 10.1158/0008-5472.CAN-10-2907
25. Contasta I, Berghella AM, Pellegrini P, Adorno D. Passage from normal mucosa to adenoma and colon cancer: alteration of normal sCD30 mechanisms regulating TH1/TH2 cell functions. Cancer Biother Radiopharm. (2003) 18:549–57. doi: 10.1089/108497803322287628
26. Cui G, Florholmen J. Polarization of cytokine profile from Th1 into Th2 along colorectal adenoma-carcinoma sequence: implications for the biotherapeutic target? Inflamm Allergy Drug Targets. (2008) 7:94–7. doi: 10.2174/187152808785107589
27. Piancatelli D, Romano P, Sebastiani P, Adorno D, Casciani CU. Local expression of cytokines in human colorectal carcinoma: evidence of specific interleukin-6 gene expression. J Immunother. (1999) 22:25–32. doi: 10.1097/00002371-199901000-00004
28. Berghella AM, Pellegrini P, Piancatelli D, Maccarone D, Del Beato T, Giubilei D, et al. Progression mechanisms in colon cancer: soluble interleukin-2 (IL-2) receptor, IL-2 plus anti-CD3 proliferative response and tumour stage correlations. Cancer Immunol Immunother. (1994) 38:160–6. doi: 10.1007/BF01525636
29. Kaminska J, Nowacki MP, Kowalska M, Rysinska A, Chwalinski M, Fuksiewicz M, et al. Clinical significance of serum cytokine measurements in untreated colorectal cancer patients: soluble tumor necrosis factor receptor type I–an independent prognostic factor. Tumour Biol. (2005) 26:186–94. doi: 10.1159/000086951
30. Waldner MJ, Foersch S, Neurath MF. Interleukin-6–a key regulator of colorectal cancer development. Int J Biol Sci. (2012) 8:1248–53. doi: 10.7150/ijbs.4614
31. Cui G, Yuan A, Goll R, Florholmen J. IL-17A in the tumor microenvironment of the human colorectal adenoma-carcinoma sequence. Scand J Gastroenterol. (2012) 47:1304–12. doi: 10.3109/00365521.2012.725089
32. Cui G, Yuan A, Pang Z, Zheng W, Li Z, Goll R. Contribution of IL-33 to the pathogenesis of colorectal cancer. Front Oncol. (2018) 8:561. doi: 10.3389/fonc.2018.00561
33. Cui G, Yuan A, Zhu L, Florholmen J, Goll R. Increased expression of interleukin-21 along colorectal adenoma-carcinoma sequence and its predicating significance in patients with sporadic colorectal cancer. Clin Immunol. (2017) 183:266–72. doi: 10.1016/j.clim.2017.09.003
34. Cui J, Xu G, Liu J, Pang Z, Florholmen J, Cui G. The expression of non-mast histamine in tumor associated microvessels in human colorectal cancers. Pathol Oncol Res. (2013) 19:311–6. doi: 10.1007/s12253-012-9584-y
35. Cui G, Shi Y, Cui J, Tang F, Florholmen J. Immune microenvironmental shift along human colorectal adenoma-carcinoma sequence: is it relevant to tumor development, biomarkers and biotherapeutic targets? Scand J Gastroenterol. (2012) 47:367–77. doi: 10.3109/00365521.2011.648950
36. Wang K, Grivennikov SI, Karin M. Implications of anti-cytokine therapy in colorectal cancer and autoimmune diseases. Ann Rheum Dis. (2013) 72(Suppl. 2):ii100–3. doi: 10.1136/annrheumdis-2012-202201
37. Defendenti C, Sarzi-Puttini P, Saibeni S, Bollani S, Bruno S, Almasio PL, et al. Significance of serum Il-9 levels in inflammatory bowel disease. Int J Immunopathol Pharmacol. (2015) 28:569–75. doi: 10.1177/0394632015600535
38. Gerlach K, Hwang Y, Nikolaev A, Atreya R, Dornhoff H, Steiner S, et al. TH9 cells that express the transcription factor PU.1 drive T cell-mediated colitis via IL-9 receptor signaling in intestinal epithelial cells. Nat Immunol. (2014) 15:676–86. doi: 10.1038/ni.2920
39. Korn T, Bettelli E, Oukka M, Kuchroo VK. IL-17 and Th17 Cells. Annu Rev Immunol. (2009) 27:485–517. doi: 10.1146/annurev.immunol.021908.132710
40. Schwartz SA, Hernandez A, Mark Evers B. The role of NF-kappaB/IkappaB proteins in cancer: implications for novel treatment strategies. Surg Oncol. (1999) 8:143–53. doi: 10.1016/S0960-7404(00)00012-8
41. Cui G, Yuan A, Vonen B, Florholmen J. Progressive cellular response in the lamina propria of the colorectal adenoma-carcinoma sequence. Histopathology. (2009) 54:550–60. doi: 10.1111/j.1365-2559.2009.03273.x
42. Banner BF, Sonmez-Alpan E, Yousem SA. An immunophenotypic study of the inflammatory cell populations in colon adenomas and carcinomas. Mod Pathol. (1993) 6:295–301.
43. Wallace K, Lewin DN, Sun S, Spiceland CM, Rockey DC, Alekseyenko AV, et al. Tumor-infiltrating lymphocytes and colorectal cancer survival in African American and Caucasian patients. Cancer Epidemiol Biomarkers Prev. (2018) 27:755–61. doi: 10.1158/1055-9965.EPI-17-0870
44. Xie QK, He WZ, Hu WM, Yang L, Jiang C, Kong PF, et al. Tumor-infiltrating lymphocyte as a prognostic biomarker in stage IV colorectal cancer should take into account the metastatic status and operation modality. Cancer Manag Res. (2018) 10:1365–75. doi: 10.2147/CMAR.S162147
45. Shibutani M, Maeda K, Nagahara H, Fukuoka T, Iseki Y, Matsutani S, et al. Tumor-infiltrating lymphocytes predict the chemotherapeutic outcomes in patients with stage IV colorectal cancer. In Vivo. (2018) 32:151–8. doi: 10.21873/invivo.11218
46. Zhao Y, Ge X, He J, Cheng Y, Wang Z, Wang J, et al. The prognostic value of tumor-infiltrating lymphocytes in colorectal cancer differs by anatomical subsite: a systematic review and meta-analysis. World J Surg Oncol. (2019) 17:85. doi: 10.1186/s12957-019-1621-9
47. Dock J, Ramirez CM, Hultin L, Hausner MA, Hultin P, Elliott J, et al. Distinct aging profiles of CD8+ T cells in blood versus gastrointestinal mucosal compartments. PLoS ONE. (2017) 12:e0182498. doi: 10.1371/journal.pone.0182498
48. Preza GC, Yang OO, Elliott J, Anton PA, Ochoa MT. T lymphocyte density and distribution in human colorectal mucosa, and inefficiency of current cell isolation protocols. PLoS ONE. (2015) 10:e0122723. doi: 10.1371/journal.pone.0122723
49. Liu F, Hu X, Zimmerman M, Waller JL, Wu P, Hayes-Jordan A, et al. TNFalpha cooperates with IFN-gamma to repress Bcl-xL expression to sensitize metastatic colon carcinoma cells to TRAIL-mediated apoptosis. PLoS ONE. (2011) 6:e16241. doi: 10.1371/journal.pone.0016241
50. Koch M, Beckhove P, Op den Winkel J, Autenrieth D, Wagner P, Nummer D, et al. Tumor infiltrating T lymphocytes in colorectal cancer: tumor-selective activation and cytotoxic activity in situ. Ann Surg. (2006) 244:986–92. Discussion 92–3. doi: 10.1097/01.sla.0000247058.43243.7b
51. Ogino S, Goel A. Molecular classification and correlates in colorectal cancer. J Mol Diagn. (2008) 10:13–27. doi: 10.2353/jmoldx.2008.070082
52. Jass JR. Classification of colorectal cancer based on correlation of clinical, morphological and molecular features. Histopathology. (2007) 50:113–30. doi: 10.1111/j.1365-2559.2006.02549.x
53. Boissiere-Michot F, Lazennec G, Frugier H, Jarlier M, Roca L, Duffour J, et al. Characterization of an adaptive immune response in microsatellite-instable colorectal cancer. Oncoimmunology. (2014) 3:e29256. doi: 10.4161/onci.29256
54. Lee SY, Miyai K, Han HS, Hwang DY, Seong MK, Chung H, et al. Microsatellite instability, EMAST, and morphology associations with T cell infiltration in colorectal neoplasia. Dig Dis Sci. (2012) 57:72–8. doi: 10.1007/s10620-011-1825-5
55. Le DT, Durham JN, Smith KN, Wang H, Bartlett BR, Aulakh LK, et al. Mismatch repair deficiency predicts response of solid tumors to PD-1 blockade. Science. (2017) 357:409–13. doi: 10.1126/science.aan6733
56. Asaoka Y, Ijichi H, Koike K. PD-1 blockade in tumors with mismatch-repair deficiency. N Engl J Med. (2015) 373:1979. doi: 10.1056/NEJMc1510353
57. Smyth EC, Wotherspoon A, Peckitt C, Gonzalez D, Hulkki-Wilson S, Eltahir Z, et al. Mismatch repair deficiency, microsatellite instability, and survival: an exploratory analysis of the Medical Research Council Adjuvant Gastric Infusional Chemotherapy (MAGIC) trial. JAMA Oncol. (2017) 3:1197–203. doi: 10.1001/jamaoncol.2016.6762
58. Goswami R, Kaplan MH. A brief history of IL-9. J Immunol. (2011) 186:3283–8. doi: 10.4049/jimmunol.1003049
59. Vyas SP, Goswami R. A decade of Th9 cells: role of Th9 cells in inflammatory bowel disease. Front Immunol. (2018) 9:1139. doi: 10.3389/fimmu.2018.01139
60. Matusiewicz K, Iwanczak B, Matusiewicz M. Th9 lymphocytes and functions of interleukin 9 with the focus on IBD pathology. Adv Med Sci. (2018) 63:278–84. doi: 10.1016/j.advms.2018.03.002
61. Kaplan MH. Th9 cells: differentiation and disease. Immunol Rev. (2013) 252:104–15. doi: 10.1111/imr.12028
62. Chang HC, Sehra S, Goswami R, Yao W, Yu Q, Stritesky GL, et al. The transcription factor PU.1 is required for the development of IL-9-producing T cells and allergic inflammation. Nat Immunol. (2010) 11:527–34. doi: 10.1038/ni.1867
63. Gerlach K, McKenzie AN, Neurath MF, Weigmann B. IL-9 regulates intestinal barrier function in experimental T cell-mediated colitis. Tissue Barriers. (2015) 3:e983777. doi: 10.4161/21688370.2014.983777
64. Song EM, Jung SA, Lee JS, Kim SE, Shim KN, Jung HK, et al. [Benzoxazole derivative B-98 ameliorates dextran sulfate sodium-induced acute murine colitis and the change of T cell profiles in acute murine colitis model]. Korean J Gastroenterol. (2013) 62:33–41. doi: 10.4166/kjg.2013.62.1.33
65. Veldhoen M, Uyttenhove C, van Snick J, Helmby H, Westendorf A, Buer J, et al. Transforming growth factor-beta 'reprograms' the differentiation of T helper 2 cells and promotes an interleukin 9-producing subset. Nat Immunol. (2008) 9:1341–6. doi: 10.1038/ni.1659
66. Yuan A, Yang H, Qi H, Cui J, Hua W, Li C, et al. IL-9 antibody injection suppresses the inflammation in colitis mice. Biochem Biophys Res Commun. (2015) 468:921–6. doi: 10.1016/j.bbrc.2015.11.057
67. Wang H, Jain S, Li P, Lin JX, Oh J, Qi C, et al. Transcription factors IRF8 and PU.1 are required for follicular B cell development and BCL6-driven germinal center responses. Proc Natl Acad Sci USA. (2019) 116:9511–20. doi: 10.1073/pnas.1901258116
68. Koh B, Hufford MM, Pham D, Olson MR, Wu T, Jabeen R, et al. The ETS family transcription factors Etv5 and PU.1 function in parallel to promote Th9 cell development. J Immunol. (2016) 197:2465–72. doi: 10.4049/jimmunol.1502383
69. Malik S, Awasthi A. Transcriptional control of Th9 cells: role of Foxo1 in Interleukin-9 induction. Front Immunol. (2018) 9:995. doi: 10.3389/fimmu.2018.00995
70. Matusiewicz M, Neubauer K, Bednarz-Misa I, Gorska S, Krzystek-Korpacka M. Systemic interleukin-9 in inflammatory bowel disease: association with mucosal healing in ulcerative colitis. World J Gastroenterol. (2017) 23:4039–46. doi: 10.3748/wjg.v23.i22.4039
71. Dong-dong Q, Shi-lu J, Bao-zhen L, Jian Z, Chun-xia X. The expression and significance of interleukin-9, interleukin-6 and tumor necrosis factor-α in colonic mucosa of patients with ulcerative colitis. J Int Transl Med. (2016) 4:172–8. doi: 10.11910/2227-6394.2016.04.03.05
72. Tian L, Li Y, Zhang J, Chang R, Li J, Huo L. IL-9 promotes the pathogenesis of ulcerative colitis through STAT3/SOCS3 signaling. Biosci Rep. (2018) 38:BSR20181521. doi: 10.1042/BSR20181521
73. Rivera Vargas T, Humblin E, Vegran F, Ghiringhelli F, Apetoh L. TH9 cells in anti-tumor immunity. Semin Immunopathol. (2017) 39:39–46. doi: 10.1007/s00281-016-0599-4
74. Hoelzinger DB, Dominguez AL, Cohen PA, Gendler SJ. Inhibition of adaptive immunity by IL9 can be disrupted to achieve rapid T-cell sensitization and rejection of progressive tumor challenges. Cancer Res. (2014) 74:6845–55. doi: 10.1158/0008-5472.CAN-14-0836
75. Lu Y, Wang Q, Xue G, Bi E, Ma X, Wang A, et al. Th9 cells represent a unique subset of CD4(+) T cells endowed with the ability to eradicate advanced tumors. Cancer Cell. (2018) 33:1048–60.e7. doi: 10.1016/j.ccell.2018.05.004
76. Rivera Vargas T, Cai Z, Shen Y, Dosset M, Benoit-Lizon I, Martin T, et al. Selective degradation of PU.1 during autophagy represses the differentiation and antitumour activity of TH9 cells. Nat Commun. (2017) 8:559. doi: 10.1038/s41467-017-00468-w
77. Huang Y, Cao Y, Zhang S, Gao F. Association between low expression levels of interleukin-9 and colon cancer progression. Exp Ther Med. (2015) 10:942–6. doi: 10.3892/etm.2015.2588
78. Wang J, Dong X, Zhu X, Zhao H, Mao D, Zhao X. [Expression of interleukin-9 in colon cancer tissues and its clinical significance]. Nan Fang Yi Ke Da Xue Xue Bao. (2018) 38:943–8. doi: 10.3969/j.issn.1673-4254.2018.08.07
79. Liu JQ, Li XY, Yu HQ, Yang G, Liu ZQ, Geng XR, et al. Tumor-specific Th2 responses inhibit growth of CT26 colon-cancer cells in mice via converting intratumor regulatory T cells to Th9 cells. Sci Rep. (2015) 5:10665. doi: 10.1038/srep10665
80. Wang J, Sun M, Zhao H, Huang Y, Li D, Mao D, et al. IL-9 exerts antitumor effects in colon cancer and transforms the tumor microenvironment in vivo. Technol Cancer Res Treat. (2019) 18:1533033819857737. doi: 10.1177/1533033819857737
81. Do Thi VA, Park SM, Lee H, Kim YS. Ectopically expressed membrane-bound form of IL-9 exerts immune-stimulatory effect on CT26 colon carcinoma cells. Immune Netw. (2018) 18:e12. doi: 10.4110/in.2018.18.e12
82. Sun Z, Zhang Y, Xu G, Zhu L, Zhang X, Cui G. Dynamic changes of IL-9 in development of colitis associated colorectal cancer in mice. J Zhengzhou Univ. (2018) 53:622–5. doi: 10.13705/j.issn.1671-6825.2018.03.006
83. Tian L, Li Y, Chang R, Zhang P, Zhang J, Huo L. Lentiviral vector-mediated IL-9 overexpression stimulates cell proliferation by targeting c-myc and cyclin D1 in colitis-associated cancer. Oncol Lett. (2019) 17:175–82. doi: 10.3892/ol.2018.9567
84. Krzystek-Korpacka M, Zawadzki M, Kapturkiewicz B, Lewandowska P, Bednarz-Misa I, Gorska S, et al. Subsite heterogeneity in the profiles of circulating cytokines in colorectal cancer. Cytokine. (2018) 110:435–41. doi: 10.1016/j.cyto.2018.05.015
85. Kryczek I, Wei S, Szeliga W, Vatan L, Zou W. Endogenous IL-17 contributes to reduced tumor growth and metastasis. Blood. (2009) 114:357–9. doi: 10.1182/blood-2008-09-177360
86. Yang L, Liu H, Zhang L, Hu J, Chen H, Wang L, et al. Effect of IL-17 in the development of colon cancer in mice. Oncol Lett. (2016) 12:4929–36. doi: 10.3892/ol.2016.5329
87. O'Sullivan T, Saddawi-Konefka R, Gross E, Tran M, Mayfield SP, Ikeda H, et al. Interleukin-17D mediates tumor rejection through recruitment of natural killer cells. Cell Rep. (2014) 7:989–98. doi: 10.1016/j.celrep.2014.03.073
88. Lu L, Pan K, Zheng HX, Li JJ, Qiu HJ, Zhao JJ, et al. IL-17A promotes immune cell recruitment in human esophageal cancers and the infiltrating dendritic cells represent a positive prognostic marker for patient survival. J Immunother. (2013) 36:451–8. doi: 10.1097/CJI.0b013e3182a802cf
89. Honorati MC, Neri S, Cattini L, Facchini A. IL-17 enhances the susceptibility of U-2 OS osteosarcoma cells to NK cell lysis. Clin Exp Immunol. (2003) 133:344–9. doi: 10.1046/j.1365-2249.2003.02234.x
90. Lin Y, Xu J, Su H, Zhong W, Yuan Y, Yu Z, et al. Interleukin-17 is a favorable prognostic marker for colorectal cancer. Clin Transl Oncol. (2015) 17:50–6. doi: 10.1007/s12094-014-1197-3
91. Galvez J. Role of Th17 Cells in the Pathogenesis of Human IBD. ISRN Inflamm. (2014) 2014:928461. doi: 10.1155/2014/928461
92. Razi S, Baradaran Noveiry B, Keshavarz-Fathi M, Rezaei N. IL-17 and colorectal cancer: from carcinogenesis to treatment. Cytokine. (2019) 116:7–12. doi: 10.1016/j.cyto.2018.12.021
93. Liu J, Duan Y, Cheng X, Chen X, Xie W, Long H, et al. IL-17 is associated with poor prognosis and promotes angiogenesis via stimulating VEGF production of cancer cells in colorectal carcinoma. Biochem Biophys Res Commun. (2011) 407:348–54. doi: 10.1016/j.bbrc.2011.03.021
94. Doulabi H, Rastin M, Shabahangh H, Maddah G, Abdollahi A, Nosratabadi R, et al. Analysis of Th22, Th17 and CD4(+)cells co-producing IL-17/IL-22 at different stages of human colon cancer. Biomed Pharmacother. (2018) 103:1101–6. doi: 10.1016/j.biopha.2018.04.147
95. Wang K, Kim MK, Di Caro G, Wong J, Shalapour S, Wan J, et al. Interleukin-17 receptor a signaling in transformed enterocytes promotes early colorectal tumorigenesis. Immunity. (2014) 41:1052–63. doi: 10.1016/j.immuni.2014.11.009
96. Bedoui SA, Barbirou M, Stayoussef M, Dallel M, Mokrani A, Makni L, et al. Association of interleukin-17A polymorphisms with the risk of colorectal cancer: a case-control study. Cytokine. (2018) 110:18–23. doi: 10.1016/j.cyto.2018.04.017
97. Samiei G, Yip WK, Leong PP, Jabar MF, Dusa NM, Mohtarrudin N, et al. Association between polymorphisms of interleukin-17A G197A and interleukin-17F A7488G and risk of colorectal cancer. J Cancer Res Ther. (2018) 14(Suppl.):S299–305. doi: 10.4103/0973-1482.235345
98. Sharp SP, Avram D, Stain SC, Lee EC. Local and systemic Th17 immune response associated with advanced stage colon cancer. J Surg Res. (2017) 208:180–6. doi: 10.1016/j.jss.2016.09.038
99. Sui G, Qiu Y, Yu H, Kong Q, Zhen B. Interleukin-17 promotes the development of cisplatin resistance in colorectal cancer. Oncol Lett. (2019) 17:944–50. doi: 10.3892/ol.2018.9645
100. Le Gouvello S, Bastuji-Garin S, Aloulou N, Mansour H, Chaumette MT, Berrehar F, et al. High prevalence of Foxp3 and IL17 in MMR-proficient colorectal carcinomas. Gut. (2008) 57:772–9. doi: 10.1136/gut.2007.123794
101. Schetter AJ, Nguyen GH, Bowman ED, Mathe EA, Yuen ST, Hawkes JE, et al. Association of inflammation-related and microRNA gene expression with cancer-specific mortality of colon adenocarcinoma. Clin Cancer Res. (2009) 15:5878–87. doi: 10.1158/1078-0432.CCR-09-0627
102. Cascinu S, Poli D, Zaniboni A, Lonardi S, Labianca R, Sobrero A, et al. The prognostic impact of primary tumour location in patients with stage II and stage III colon cancer receiving adjuvant therapy. A GISCAD analysis from three large randomised trials. Eur J Cancer. (2019) 111:1–7. doi: 10.1016/j.ejca.2019.01.020
103. Chae WJ, Gibson TF, Zelterman D, Hao L, Henegariu O, Bothwell AL. Ablation of IL-17A abrogates progression of spontaneous intestinal tumorigenesis. Proc Natl Acad Sci USA. (2010) 107:5540–4. doi: 10.1073/pnas.0912675107
104. Ibrahim S, Girault A, Ohresser M, Lereclus E, Paintaud G, Lecomte T, et al. Monoclonal antibodies targeting the IL-17/IL-17RA axis: an opportunity to improve the efficiency of anti-VEGF therapy in fighting metastatic colorectal cancer? Clin Colorectal Cancer. (2018) 17:e109–13. doi: 10.1016/j.clcc.2017.10.003
105. Tong Z, Yang XO, Yan H, Liu W, Niu X, Shi Y, et al. A protective role by interleukin-17F in colon tumorigenesis. PLoS ONE. (2012) 7:e34959. doi: 10.1371/journal.pone.0034959
106. Nemati K, Golmoghaddam H, Hosseini SV, Ghaderi A, Doroudchi M. Interleukin-17FT7488 allele is associated with a decreased risk of colorectal cancer and tumor progression. Gene. (2015) 561:88–94. doi: 10.1016/j.gene.2015.02.014
107. Chae WJ, Bothwell AL. IL-17F deficiency inhibits small intestinal tumorigenesis in ApcMin/+ mice. Biochem Biophys Res Commun. (2011) 414:31–6. doi: 10.1016/j.bbrc.2011.09.016
108. Chin CC, Chen CN, Kuo HC, Shi CS, Hsieh MC, Kuo YH, et al. Interleukin-17 induces CC chemokine receptor 6 expression and cell migration in colorectal cancer cells. J Cell Physiol. (2015) 230:1430–7. doi: 10.1002/jcp.24796
109. Wang Q, Feng M, Yu T, Liu X, Zhang P. Intratumoral regulatory T cells are associated with suppression of colorectal carcinoma metastasis after resection through overcoming IL-17 producing T cells. Cell Immunol. (2014) 287:100–5. doi: 10.1016/j.cellimm.2014.01.002
110. Tseng JY, Yang CY, Liang SC, Liu RS, Yang SH, Lin JK, et al. Interleukin-17A modulates circulating tumor cells in tumor draining vein of colorectal cancers and affects metastases. Clin Cancer Res. (2014) 20:2885–97. doi: 10.1158/1078-0432.CCR-13-2162
111. Xie Z, Qu Y, Leng Y, Sun W, Ma S, Wei J, et al. Human colon carcinogenesis is associated with increased interleukin-17-driven inflammatory responses. Drug Des Devel Ther. (2015) 9:1679–89. doi: 10.2147/DDDT.S79431
112. Numasaki M, Fukushi J, Ono M, Narula SK, Zavodny PJ, Kudo T, et al. Interleukin-17 promotes angiogenesis and tumor growth. Blood. (2003) 101:2620–7. doi: 10.1182/blood-2002-05-1461
113. Lotti F, Jarrar AM, Pai RK, Hitomi M, Lathia J, Mace A, et al. Chemotherapy activates cancer-associated fibroblasts to maintain colorectal cancer-initiating cells by IL-17A. J Exp Med. (2013) 210:2851–72. doi: 10.1084/jem.20131195
114. Yazawa T, Shibata M, Gonda K, Machida T, Suzuki S, Kenjo A, et al. Increased IL-17 production correlates with immunosuppression involving myeloid-derived suppressor cells and nutritional impairment in patients with various gastrointestinal cancers. Mol Clin Oncol. (2013) 1:675–9. doi: 10.3892/mco.2013.134
115. He D, Li H, Yusuf N, Elmets CA, Li J, Mountz JD, et al. IL-17 promotes tumor development through the induction of tumor promoting microenvironments at tumor sites and myeloid-derived suppressor cells. J Immunol. (2010) 184:2281–8. doi: 10.4049/jimmunol.0902574
116. Yan J, Huang J. Innate gammadeltaT17 cells convert cancer-elicited inflammation into immunosuppression through myeloid-derived suppressor cells. Oncoimmunology. (2014) 3:e953423. doi: 10.4161/21624011.2014.953423
117. Wu P, Wu D, Ni C, Ye J, Chen W, Hu G, et al. gammadeltaT17 cells promote the accumulation and expansion of myeloid-derived suppressor cells in human colorectal cancer. Immunity. (2014) 40:785–800. doi: 10.1016/j.immuni.2014.03.013
118. Plank MW, Kaiko GE, Maltby S, Weaver J, Tay HL, Shen W, et al. Th22 cells form a distinct th lineage from Th17 cells in vitro with unique transcriptional properties and Tbet-dependent Th1 plasticity. J Immunol. (2017) 198:2182–90. doi: 10.4049/jimmunol.1601480
119. Yeste A, Mascanfroni ID, Nadeau M, Burns EJ, Tukpah AM, Santiago A, et al. IL-21 induces IL-22 production in CD4+ T cells. Nat Commun. (2014) 5:3753. doi: 10.1038/ncomms4753
120. Duhen T, Geiger R, Jarrossay D, Lanzavecchia A, Sallusto F. Production of interleukin 22 but not interleukin 17 by a subset of human skin-homing memory T cells. Nat Immunol. (2009) 10:857–63. doi: 10.1038/ni.1767
121. Li LJ, Gong C, Zhao MH, Feng BS. Role of interleukin-22 in inflammatory bowel disease. World J Gastroenterol. (2014) 20:18177–88. doi: 10.3748/wjg.v20.i48.18177
122. Brand S, Beigel F, Olszak T, Zitzmann K, Eichhorst ST, Otte JM, et al. IL-22 is increased in active Crohn's disease and promotes proinflammatory gene expression and intestinal epithelial cell migration. Am J Physiol Gastrointest Liver Physiol. (2006) 290:G827–38. doi: 10.1152/ajpgi.00513.2005
123. Fang L, Pang Z, Shu W, Wu W, Sun M, Cong Y, et al. Anti-TNF therapy induces CD4+ T-cell production of IL-22 and promotes epithelial repairs in patients with Crohn's disease. Inflamm Bowel Dis. (2018) 24:1733–44. doi: 10.1093/ibd/izy126
124. Sugimoto K, Ogawa A, Mizoguchi E, Shimomura Y, Andoh A, Bhan AK, et al. IL-22 ameliorates intestinal inflammation in a mouse model of ulcerative colitis. J Clin Invest. (2008) 118:534–44. doi: 10.1172/JCI33194
125. Arj A, Razavizadeh M, Mohammadi H, Nikoueinejad H, Akbari H. The correlation between the numerical status of Th22 cells and serum level of IL-22 with severity of ulcerative colitis. Iran J Allergy Asthma Immunol. (2018) 17:78–84.
126. Yu LZ, Wang HY, Yang SP, Yuan ZP, Xu FY, Sun C, et al. Expression of interleukin-22/STAT3 signaling pathway in ulcerative colitis and related carcinogenesis. World J Gastroenterol. (2013) 19:2638–49. doi: 10.3748/wjg.v19.i17.2638
127. Bootz F, Ziffels B, Neri D. Antibody-based targeted delivery of interleukin-22 promotes rapid clinical recovery in mice with DSS-induced colitis. Inflamm Bowel Dis. (2016) 22:2098–105. doi: 10.1097/MIB.0000000000000851
128. Jiang R, Tan Z, Deng L, Chen Y, Xia Y, Gao Y, et al. Interleukin-22 promotes human hepatocellular carcinoma by activation of STAT3. Hepatology. (2011) 54:900–9. doi: 10.1002/hep.24486
129. Khosravi N, Caetano MS, Cumpian AM, Unver N, De la Garza Ramos C, Noble O, et al. IL22 Promotes Kras-mutant lung cancer by induction of a protumor immune response and protection of stemness properties. Cancer Immunol Res. (2018) 6:788–97. doi: 10.1158/2326-6066.CIR-17-0655
130. Liu T, Peng L, Yu P, Zhao Y, Shi Y, Mao X, et al. Increased circulating Th22 and Th17 cells are associated with tumor progression and patient survival in human gastric cancer. J Clin Immunol. (2012) 32:1332–9. doi: 10.1007/s10875-012-9718-8
131. Chen X, Wang Y, Wang J, Wen J, Jia X, Wang X, et al. Accumulation of T-helper 22 cells, interleukin-22 and myeloid-derived suppressor cells promotes gastric cancer progression in elderly patients. Oncol Lett. (2018) 16:253–61. doi: 10.3892/ol.2018.8612
132. Ziesche E, Bachmann M, Kleinert H, Pfeilschifter J, Muhl H. The interleukin-22/STAT3 pathway potentiates expression of inducible nitric-oxide synthase in human colon carcinoma cells. J Biol Chem. (2007) 282:16006–15. doi: 10.1074/jbc.M611040200
133. Huber S, Gagliani N, Zenewicz LA, Huber FJ, Bosurgi L, Hu B, et al. IL-22BP is regulated by the inflammasome and modulates tumorigenesis in the intestine. Nature. (2012) 491:259–63. doi: 10.1038/nature11535
134. Sun D, Lin Y, Hong J, Chen H, Nagarsheth N, Peng D, et al. Th22 cells control colon tumorigenesis through STAT3 and Polycomb Repression complex 2 signaling. Oncoimmunology. (2016) 5:e1082704. doi: 10.1080/2162402X.2015.1082704
135. Cella M, Fuchs A, Vermi W, Facchetti F, Otero K, Lennerz JK, et al. A human natural killer cell subset provides an innate source of IL-22 for mucosal immunity. Nature. (2009) 457:722–5. doi: 10.1038/nature07537
136. Huang YH, Cao YF, Jiang ZY, Zhang S, Gao F. Th22 cell accumulation is associated with colorectal cancer development. World J Gastroenterol. (2015) 21:4216–24. doi: 10.3748/wjg.v21.i14.4216
137. Jiang R, Wang H, Deng L, Hou J, Shi R, Yao M, et al. IL-22 is related to development of human colon cancer by activation of STAT3. BMC Cancer. (2013) 13:59. doi: 10.1186/1471-2407-13-59
138. Wang C, Gong G, Sheh A, Muthupalani S, Bryant EM, Puglisi DA, et al. Interleukin-22 drives nitric oxide-dependent DNA damage and dysplasia in a murine model of colitis-associated cancer. Mucosal Immunol. (2017) 10:1504–17. doi: 10.1038/mi.2017.9
139. Ling L, Zhao P, Yan G, Chen M, Zhang T, Wang L, et al. The frequency of Th17 and Th22 cells in patients with colorectal cancer at pre-operation and post-operation. Immunol Invest. (2015) 44:56–69. doi: 10.3109/08820139.2014.936445
140. Thompson CL, Plummer SJ, Tucker TC, Casey G, Li L. Interleukin-22 genetic polymorphisms and risk of colon cancer. Cancer Causes Control. (2010) 21:1165–70. doi: 10.1007/s10552-010-9542-5
141. Wu T, Cui L, Liang Z, Liu C, Liu Y, Li J. Elevated serum IL-22 levels correlate with chemoresistant condition of colorectal cancer. Clin Immunol. (2013) 147:38–9. doi: 10.1016/j.clim.2013.02.007
142. Khare V, Paul G, Movadat O, Frick A, Jambrich M, Krnjic A, et al. IL10R2 overexpression promotes IL22/STAT3 signaling in colorectal carcinogenesis. Cancer Immunol Res. (2015) 3:1227–35. doi: 10.1158/2326-6066.CIR-15-0031
143. Lim C, Savan R. The role of the IL-22/IL-22R1 axis in cancer. Cytokine Growth Factor Rev. (2014) 25:257–71. doi: 10.1016/j.cytogfr.2014.04.005
144. Bilinski C, Burleson J, Forouhar F. Inflammation associated with neoplastic colonic polyps. Ann Clin Lab Sci. (2012) 42:266–70.
145. Lasry A, Zinger A, Ben-Neriah Y. Inflammatory networks underlying colorectal cancer. Nat Immunol. (2016) 17:230–40. doi: 10.1038/ni.3384
146. Yang Y. Cancer immunotherapy: harnessing the immune system to battle cancer. J Clin Invest. (2015) 125:3335–7. doi: 10.1172/JCI83871
147. Jacobson-Brown P, Neuman MG. Colorectal polyposis and immune-based therapies. Can J Gastroenterol. (2004) 18:239–49. doi: 10.1155/2004/742713
148. Baldwin AS. Control of oncogenesis and cancer therapy resistance by the transcription factor NF-kappaB. J Clin Invest. (2001) 107:241–6. doi: 10.1172/JCI11991
149. Fridman WH, Berger A, Cugnenc PH, Tartour E. Cytokines as prognostic and therapeutic tools in human cancer. Eur Cytokine Netw. (1997) 8:321–2.
150. Wolman R, de Gara C, Isenberg D. Acute pancreatitis in systemic lupus erythematosus: report of a case unrelated to drug therapy. Ann Rheum Dis. (1988) 47:77–9. doi: 10.1136/ard.47.1.77
151. Dalerba P, Maccalli C, Casati C, Castelli C, Parmiani G. Immunology and immunotherapy of colorectal cancer. Crit Rev Oncol Hematol. (2003) 46:33–57. doi: 10.1016/S1040-8428(02)00159-2
152. Topalian SL, Wolchok JD, Chan TA, Mellman I, Palucka K, Banchereau J, et al. Immunotherapy: the path to win the war on cancer? Cell. (2015) 161:185–6. doi: 10.1016/j.cell.2015.03.045
153. Ventola CL. Cancer immunotherapy, part 1: current strategies and agents. P T. (2017) 42:375–83.
154. Markota A, Endres S, Kobold S. Targeting interleukin-22 for cancer therapy. Hum Vaccin Immunother. (2018) 14:2012–5. doi: 10.1080/21645515.2018.1461300
155. Voigt C, May P, Gottschlich A, Markota A, Wenk D, Gerlach I, et al. Cancer cells induce interleukin-22 production from memory CD4(+) T cells via interleukin-1 to promote tumor growth. Proc Natl Acad Sci USA. (2017) 114:12994–9. doi: 10.1073/pnas.1705165114
156. Wang T, Zhang Z, Xing H, Wang L, Zhang G, Yu N, et al. Elevated Th22 cells and related cytokines in patients with epithelial ovarian cancer. Medicine. (2017) 96:e8359. doi: 10.1097/MD.0000000000008359
Keywords: colorectum, tumorigenesis, cytokine, TH, therapeutic
Citation: Cui G (2019) TH9, TH17, and TH22 Cell Subsets and Their Main Cytokine Products in the Pathogenesis of Colorectal Cancer. Front. Oncol. 9:1002. doi: 10.3389/fonc.2019.01002
Received: 28 May 2019; Accepted: 18 September 2019;
Published: 04 October 2019.
Edited by:
Lionel Apetoh, Institut National de la Santé et de la Recherche Médicale (INSERM), FranceReviewed by:
Frederique Vegran, INSERM U1231 Lipides, Nutrition, Cancer (LNC), FranceAnneli Peters, Ludwig Maximilian University of Munich, Germany
Copyright © 2019 Cui. This is an open-access article distributed under the terms of the Creative Commons Attribution License (CC BY). The use, distribution or reproduction in other forums is permitted, provided the original author(s) and the copyright owner(s) are credited and that the original publication in this journal is cited, in accordance with accepted academic practice. No use, distribution or reproduction is permitted which does not comply with these terms.
*Correspondence: Guanglin Cui, guanglin.cui@nord.no