- 1Laboratory of Endocrinology and Reproductive Biology, Hospital Clínico Universidad de Chile, Santiago, Chile
- 2Departamento de Obstetricia y Ginecología, Facultad de Medicina, Universidad de Chile, Santiago, Chile
Angiogenesis, or generation of new blood vessels from other pre-existing, is a key process to maintain the supply of nutrients and oxygen in tissues. Unfortunately, this process is exacerbated in pathologies such as retinopathies and cancers with high angiogenesis as ovarian cancer. Angiogenesis is regulated by multiple systems including growth factors and neurotrophins. One of the most studied angiogenic growth factors is the vascular endothelial growth factor (VEGF), which is overexpressed in several cancers. It has been recently described that neurotrophins could regulate angiogenesis through direct and indirect mechanisms. Neurotrophins are a family of proteins that include nerve growth factor (NGF), brain-derived growth factor (BDNF), and neurotrophins 3 and 4/5 (NT 3, NT 4/5). These molecules and their high affinity receptors (TRKs) regulate the development, maintenance, and plasticity of the nervous system. Furthermore, it was recently described that they display essential functions in non-neuronal tissues, such as reproductive organs among others. Studies have shown that several types of cancer overexpress neurotrophins such as NGF and BDNF, which might contribute to tumor progression and angiogenesis. Besides, in recent years the FDA has approved the use of pharmacologic inhibitors of pan-TRK receptors in patients with TRKs fusion-positive cancers. In this review, we discuss the mechanisms by which neurotrophins stimulate tumor progression and angiogenesis, with emphasis on gynecological cancers.
Introduction: Angiogenesis in Gynecological Malignancies
Gynecological neoplasms belong to a group of malignances that include ovarian, cervical, uterine, fallopian tubes, vulvar, vaginal cancer and gestational trophoblastic neoplasms. The following sections of this review will be focused on the first two types, which are the most frequent (1). Gynecological neoplasms are characterized by exacerbated angiogenesis (which is defined as the generation of new blood vessels from pre-existing ones) and vascular endothelial growth factor (VEGF) is the most widely studied angiogenic factor in the context of cancer. VEGF is secreted by most tumor cells, mainly in response to hypoxia and low nutrient concentrations (2), and promotes angiogenesis through its receptors expressed in endothelial cells. This antecedent has been crucial for the development of new drugs as bevacizumab, a humanized monoclonal antibody directed against human VEGF. Unfortunately, this drug has shown modest results (3), because ovarian and uterine cells may overexpress other molecules that can act as angiogenic factors, such as neurotrophins (NTs) and their receptors (4–7).
NTs are a group of molecules widely present in the central and peripheral nervous system. They have a key role in developmental neurobiology, by regulating neuronal survival, differentiation, neurites growth, and synthesis of neurotransmitters (8). NTs not only display key roles in neuronal tissues, but also in several non-neuronal tissues, such as mammary glands (9, 10) and gynecological organs (11–13). During the neoplastic processes, NTs and their receptors are overexpressed by tumoral cells, promoting progression and angiogenesis in several cancer models. For instance, the expression of NTs predicts poor survival rates in breast and ovarian cancer patients (14–16) and NTs have been proposed as potential therapeutic targets in these neoplasms (4, 17, 18).
Angiogenesis is a key process to supply nutrients and oxygen to tumor cells, as well as a way for cells to leave or enter to the circulation (19). In fact, tumors that have a high microvascular density could be more aggressive and generate distant metastasis (20). The term angiogenesis was first used by the British surgeon John Hunter in 1787; however, the study of vascular morphology in animal and human tumors began only in the first half of twentieth century (21).
Endothelial cells, a baseline membrane and pericytes are the minimal components of vasculature. Endothelial cells form a barrier that controls the trans-endothelial flux of soluble components and most cell types (22). During angiogenesis, there are several important steps: a detection of humoral paracrine signals or angiogenic factors, resulting in the sprouting of endothelial cells, followed by an orchestrated increase of endothelial cell proliferation, migration, and differentiation (23). Activation of endothelial cells is accompanied by pericytes detachment, proliferation, and migration into the vessel interstitium to envelop the surface of the vascular tube. In addition, fibroblasts and endothelial cells build and remodel the new extracellular matrix (23, 24). All of these changes are necessary to generate new capillary vessels.
Tumor Angiogenesis
Tumor growth has two phases: an avascular stage (when tumors are constrained at diameters of 1–2 mm) and a posterior vascular stage (25), in which tumor cells need to secrete soluble factors to promote an increase of angiogenesis and continued growing (26).
In the normal vasculature, endothelial cells are stable; rarely they sprout or divide and they are associated to mural cells (pericytes) in a basal membrane. However, in the case of the tumor vasculature several chromosomal abnormalities arise (27–29), as well as variations of size and thickness, irregular shape, and big trans-cellular holes and fenestrae (30, 31). These characteristics produce a decrease of blood flow and drug delivery, and increase the interstitial fluid pressure, the extravasation of blood components and the intravasation of tumor cells (30, 32). Particularly in gynecologic neoplasms, angiogenesis plays a key role, since the ovary and uterus cyclically regulate the angiogenesis during the ovarian cycle involving blood vessel growth and regression, with a fine regulation (33–35). Therefore, angiogenesis is undoubtedly crucial in gynecological cancers, but this process is uncontrolled. Given that angiogenesis is a complex process that involves different cell types, in vivo experiments constitute the ideal condition to evaluate it. Some examples of in vivo assays are: the chick embryo chorioallantoic membrane (CAM) assay (36), zebrafish embryo assay (37, 38), corneal micropocket assay (39, 40), and matrigel plug assays (41). Moreover, there are some experimental approaches in vitro to evaluate the angiogenic potential of cells, which may have some advantages, such as the reproducibility and low cost to perform these assays (42). However, it is considered that in vitro assays evaluate vasculogenesis or de novo formation of vasculature-like structures and usually involve only endothelial cells and extracellular matrix. Examples of this are tubular formation assays in matrigel (43, 44) and the recently developed microfluidic cell culture systems (45). Nevertheless, in vitro assays are widely used, because they are a cheap and reproducible method to evaluate the angiogenic potential (46).
VEGF: Classical Angiogenic Factor in Cancer
There are many known angiogenic factors, among which VEGF is the most widely studied in the context of cancer. VEGF genes include VEGF-A to VEGF-E and another related gen, placental growth factor (PLGF) (47–50). VEGF-A (from now referred as VEGF) has the most important effect in the formation of blood vessels during development or in pathological conditions as cancer (51). At the same time, VEGF undergoes alternative exon splicing (52, 53), leading to several transcripts that include VEGF121, VEGF145, VEGF165, VEGF189, and VEGF206, which give origin to VEGF peptides of 121, 145, 165, 189 and 206 amino acids, respectively (54). Besides, VEGF121 is totally secreted and VEGF165 is partially secreted from cells (55, 56). In ovarian, endometrial and cervical cancers, VEGF121 and VEGF165 are the most dominantly expressed (57–60).
Role of Neurotrophins in Gynecological Cancer Angiogenesis: NGF/TRKA and BDNF/TRKB
Neurotrophins and Its Functions in Reproductive Tissues
NTs belong to a family of homodimeric polypeptide growth factors that promote neuronal survival and differentiation, and display important functions in non-neuronal cells (13, 61). Members of the NTs family include nerve growth factor (NGF) that was first described by Dr. Levi-Montalcini in 1956 (62), brain derived neurotrophic factor BDNF, neurotrophin-3 (NT-3), and neurotrophin-4/5 (NT-4/5) (63). Among them, NGF and BDNF are the most important NTs studied in the context of reproduction and cancer. NTs bind with different affinity to Tropomyosin kinase (TRK) receptors and produce the dimerization and transphosphorylation of its tyrosine kinase domains, activating PI3K/AKT, MAPK/ERK, and PLCγ/PKC signaling pathways (64). NGF binds with high affinity to TRKA receptor, while BDNF binds preferentially to TRKB receptor (PMID: 1649702, PMID: 2927393), as shown in Figure 1.
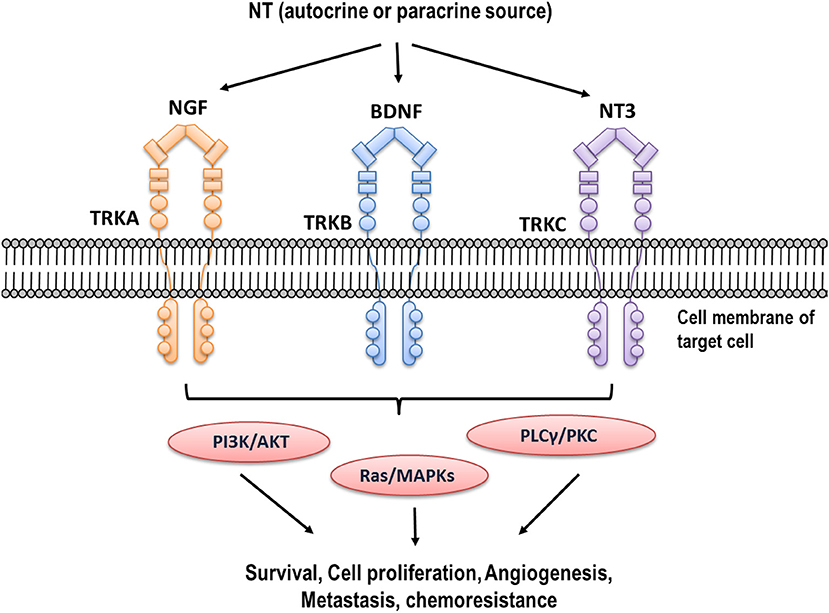
Figure 1. Neurotrophins and their high affinity receptors. Different NTs (NGF, BDNF, NT3) are expressed in high concentration in tumor cells. They bind to their high affinity receptors (TRKA, TRKB, and TRKC, respectively), producing trans-phosphorylation of tyrosine residues of intracellular domain and activating different signaling pathways such as PI3K/AKT, MAPK/ERK, and PLCγ/PKC.
Both NGF/TRKA and BDNF/TRKB are expressed in reproductive tissues as the ovary and uterus (13, 65). These NTs are involved in the control of early follicular growth and ovarian function (66–70). NGF increases cell proliferation of granulosa and thecal cells and promotes the expression of Follicle Stimulating Hormone (FSH) receptor in rat and human granulosa cells (68, 71, 72), while BDNF/TRKB are required for the growth of newly formed follicles and are involved in the maturation of human oocytes and their developmental competence after fertilization (70, 73, 74). In addition, BDNF levels in follicular fluid (75) and plasma (76) have been studied as possible predictors of in vitro fertilization outcome. BDNF and NGF seem to have a positive correlation with oocyte maturation and pre-implantation and with embryonic development in various mammalian species, including humans (73, 77–80).
On the other hand, NGF expression is present in epithelial and stromal cells in the rabbit uterus (81), as well as in human uterus (82), but its expression is lower than in the ovary (13). In addition, NGF expression seems to be necessary to ensure maternal tolerance in healthy pregnancies in mice, but an excess of NGF results in fetal rejection due to exacerbated inflammation (83). BDNF levels in menstrual blood are higher than in peripheral blood, and this factor is also present in the endometrium in both follicular and luteal phases (65). Furthermore, BDNF levels in menstrual blood of fertile women are higher than in anovulatory women (65). All these findings show that NGF and BDNF play a key role in the homeostasis and function of tissues in the context of female reproduction.
Roles of Neurotrophins as Direct and Indirect Angiogenic Factors
One of the first evidence of the angiogenic role of NGF comes from the expression of TRKA receptors in human umbilical vein endothelial cells (HUVEC): when using a VEGF-neutralizing antibody, NGF-induced HUVEC proliferation was not observed (84). In another work, NGF from different biological sources (mouse, viper and cobra) was tested in a CAM assay (85), and an increased rate of angiogenesis in a dose-dependent fashion and comparable with recombinant VEGF effects was described. Additionally, one study performed in matrigel plugs in immune-deficient mice shows that NGF strongly increases invasion, cord formation and the monolayer permeability of endothelial cells (86). Furthermore, a recent work shows that NGF increases cell proliferation, migration and differentiation of the human endothelial cell line EA.hy926 in a dose-dependent manner (87). In fact, Figure 2 shows that NGF increases inter-cellular contact structures (junctions) and polygonal structures (meshes) of EA.hy926 cells, evaluated by Image J Angiogenesis Analyzer (88). Additionally, it has been reported that NGF increases the angiogenic score of EA.hy926 cells, the effect being several times lower compared with VEGF (87).
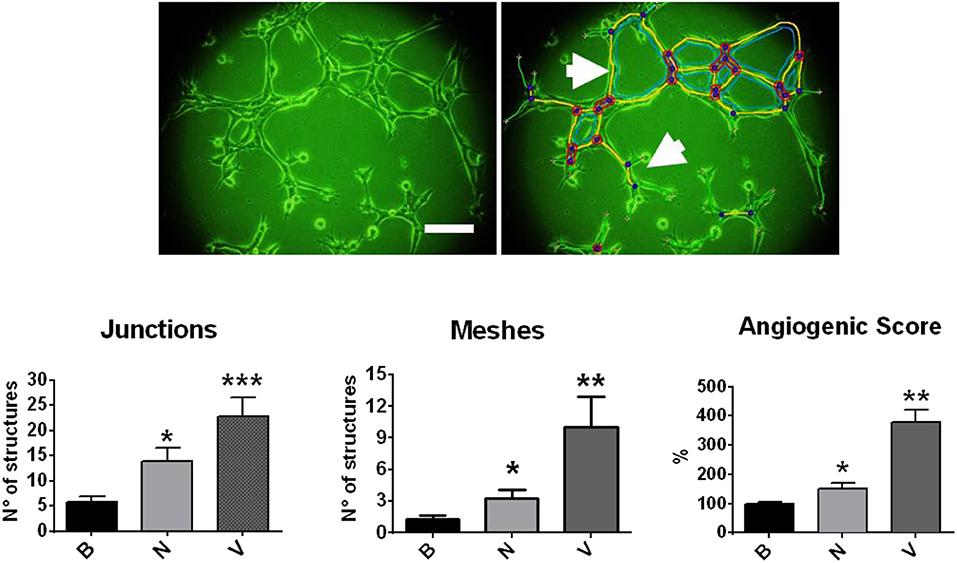
Figure 2. Effect of NGF and VEGF in a tubular formation assay in matrigel with EA.hy926 cells. Cells were disposed in matrigel and stimulated with NGF (N) and VEGF (V). Upper picture: photography of EA.hy926 cells (4 h of assay), which was analyzed by Image J Angiogenesis Analyzer. Bar charts were obtained from multicellular junctions and polygonal structures or meshes, as the arrows indicate. *p < 0.05; **p < 0.01; ***p < 0.001, according to Kruskal Wallis test. Figure obtained from Supplementary Material of Garrido et al. (87) (permission has been obtained).
In a comparable way, BDNF displays direct angiogenic effects in other types of tissues. For example, in a model of BDNF null mice, the survival of endothelial cells in intra-myocardial arteries and capillaries in the early postnatal period is impaired (89). Additionally, BDNF increases angiogenic tube formation of the endothelial cells in HUVEC (90). Besides, the overexpression of BDNF in a mouse endothelial cell line promotes endothelial cell proliferation, migration, invasion and survival (91). This evidence indicates that BDNF/TRKB exhibits a direct role in the angiogenic process and can partially explain that the anti-angiogenic therapy with Bevacizumab (neutralizing antibody against VEGF) is not optimal in the cancer context.
On the other hand, both NTs (NGF and BDNF) have an indirect angiogenic role, mediated by VEGF modulation in different cellular models. It is described that NGF and BDNF induce VEGF expression in MAPK/ERK 2-dependent pathways in granulosa cells (92) and osteoblasts (93), respectively. Besides, NGF promotes VEGF expression in neuronal superior cervical ganglia (94), while BDNF increases VEGF expression in human chondrosarcoma (95) and neuroblastoma cells (96). Another key point is that plasmatic levels of VEGF are lower in deficient BDNF animals compared to wild type animals (97). All these antecedents indicate that NTs not only act directly in vascular cells, but also affect several cell types by increasing VEGF expression and therefore their angiogenesis potential.
Role of NGF/TRKA in the Ovarian Cancer Angiogenesis
Ovarian Cancer is the most lethal gynecological malignancy in developed countries (98–100). It is characterized by non-specific symptoms and therefore is diagnosed at later stages, resulting in poor survival rates (101, 102). Approximately 80% of them are Epithelial Ovarian Cancer (EOC) (101) which is characterized by its high extent of angiogenesis that facilitates rapid tumor growth and dissemination (103). NGF and its high affinity receptor TRKA are found in very low levels or are absent in normal ovarian surface epithelium, whereas they are highly expressed in EOC (60). Another study shows that significantly higher levels of NGF, total TRKA, and phospho-TRKA (active receptor) are present in poorly differentiated EOC vs. normal ovary (4). In addition, NGF/TRKA stimulates cellular proliferation of EOC cells, by the activation of MAPK/ERK and AKT pathways, increasing Bcl2/Bax ratio and c-Myc (104), indicating the importance of NGF/ TRKA in EOC progression and suggesting that they could be considered as a potential tumor markers. As previously shown, several studies performed in in vitro and ex vivo models support the direct angiogenic role of NGF in EOC (105). It is relevant to point out that the TRKA receptor is present in endothelial cells from EOC biopsies (4), supporting the idea that the endothelium can respond to NGF stimulation.
On the other hand, an indirect angiogenic role of NGF has been described through the modulation of VEGF expression in EOC. In fact, in EOC explants, NGF increases in a dose-dependent manner the mRNA of VEGF121, VEGF165, and VEGF189 (60). Equivalent results were obtained in in vitro models, where NGF increases VEGF expression and protein levels in the culture supernatants of the EOC cell line (4).
Role of BDNF/TRKB in the Ovarian Cancer Angiogenesis
It has been reported that TRKB displays a key role in ovarian development, which gives proliferative signaling in granulosa cells during the beginning of mammalian ovary development (70). Increased TRKB levels can promote the increase of cell proliferation, invasion and angiogenesis, suppression of anoikis and decreased chemotherapy response and apoptosis in different cancer cell lines, including ovarian cancer cells (5, 106–112). Observational studies show that high TRKB expression in ovarian cancer is correlated with poor survival in ovarian cancer patients (5), and that TRKB is overexpressed in metastatic lesions compared with the corresponding primary lesions (113). In addition, BDNF treatment enhances cell invasion and migration of ovarian cell lines and TRKB-silenced cells increase the percentage of apoptotic cells (5). This evidence indicates that BDNF/TRKB may contribute to ovarian cancer progression.
In agreement with other authors, our group has found that TRKB receptor is present in stroma and in transformed epithelia of human ovary. The active TRKB receptor is upregulated in serous adenocarcinomas and its immunodetection is almost absent in the epithelia from functional ovaries or ovarian serous adenomas (114)
Interestingly, in ovarian cell lines, the silencing of TRKB receptor reduces VEGFR-2 mRNA by 70% (5), which suggests that BDNF could regulate the expression of VEGF receptors. In addition, a positive correlation between TRKB expression and lymph vessel density has been described in ovarian cancer (113). These results are consistent with other studies, in which BDNF promotes VEGF-C-dependent lymphangiogenesis in chondrosarcoma cells (95) and TRKB expression is associated with the expression of VEGF-C and VEGF-D in oral squamous cell carcinoma (115). These findings suggest that BDNF could be implicated in ovarian cancer progression and modulate angiogenesis and/or lymphangiogenesis by the increase of different VEGF isoforms.
Role of NTs in Cervical Cancer and Uterine Pathologies
Cervical cancer is the fourth most frequent cancer in women. Approximately 90% of deaths from cervical cancer occur in low-income and middle-income countries, in which strategies of prevention, early diagnosis, effective screening, and treatment programs are less common (116).
In the context of cervical cancer, BDNF/TRKB are perhaps the best studied NTs. It has been described that BDNF and TRKB expression are significantly higher in cervical cancer tissues than in normal tissues and that their presence is higher in advanced stages of this neoplasm (6, 7). In addition, BDNF levels are positively associated with lymph node metastasis (7) in cervical cancer patients. In cervical cancer cell lines, BDNF/TRKB increases cell proliferation (7, 117), apparently involving ERK and AKT signaling pathways (118). TRKB downregulation in cervical cancer cells suppress the activation of epithelial mesenchymal transition (EMT) by downregulation of N-cadherin and vimentin, among other proteins, and strongly diminishes cell proliferation, migration and invasion (117, 118).
Considering that the activation of ERK signaling pathway by BDNF/TRKB was associated with an increase of VEGF expression in osteoblasts (93), and given that TRKB can activate PI3K and ERK signaling pathways which regulate VEGF expression in several models (119–121), it is plausible that the VEGF expression could be increased by TRKB in cervical cancer, similarly to ovarian cancer.
There is no direct evidence that overexpression of NTs and its receptors are involved in the physiopathology of endometrial cancer. However, antecedents suggest that NTs could contribute to this pathology, since their expression increase in endometriosis (122–124), a condition that has been associated with higher risk of ovarian and endometrial cancer (125–127). The endometriosis is an estrogen-dependent inflammatory disease, characterized by the presence of endometrial-like tissue outside the uterine cavity (128). An important characteristic of this pathology is that angiogenesis is deregulated. In endometriosis, the VEGF expression is increased and promotes the spreading of new blood vessels at the endometriotic lesions and surroundings, which contributes to the survival of lesions (129). A recent study has shown that drospirenone, a drug used for endometriosis treatment, significantly decreases inflammatory cytokines and NGF expression, as well as VEGF expression in human endometriotic stromal cells (130). Similarly, Ginsenoside (a ginseng-derivate extract) decreases both VEGF and BDNF in rat endometriotic implants (131). These antecedents suggest that NTs could contribute not only to the pelvic chronic pain typical of endometriosis, but also to pathological angiogenesis, probably by the increase of VEGF levels.
Pharmacologic Inhibitors of Neurotrophin Receptors
Since the TRK receptors (TRKA, TRKB, and TRKC) are implicated in the progression of different kind of neoplasms, several drugs have been developed to target tumors that overexpress TRK receptors or present chromosomal rearrangements of TRK genes. For instance, in 2018, the Food and Drug Administration (FDA) approved Larotrectinib (Vitrakvi) for treatment of adult and pediatric patients with solid tumors that have TRK gene fusions (132). This was based in promissory results of 3 clinical trials (NCT02122913, NCT02637687, and NCT02576431) with Larotrectinib that showed an objective response rate of 75% in pediatric patients, with good tolerability and safety (133, 134). Larotrectinib is a small molecule that binds to NTs receptors, thereby preventing neurotrophin-TRK interaction and TRK activation, which results in the induction of cellular apoptosis and the inhibition of cell growth (135). It is important to point out that Larotrectinib was one of the first “tissue-agnostic drug” approved by FDA, concept that refers to a substance to treat cancer based on genetic and molecular features of tumor cells, regardless of the cancer type or origin (136).
Additionally, Entrectinib (Rozlytrek), a potent and selective ATP-competitive inhibitor, was approved by the FDA in 2019 for adults and pediatric patients above 12 years old with solid tumors (as ovarian cancer) that have a TRK fusion without a known acquired resistance mutation (137). The first results of phase I/II studies show promising results: for example, an objective response rate of 57.4% was obtained in 54 adults with advanced or metastatic TRK fusion-positive solid tumors (138). Unfortunately, some patients have reported resistance to TRK inhibition with this drug considered as first generation of TRK inhibitors (139), probably due to mutations in TRK domain (140, 141). To improve this aspect, a next-generation TRK-targeted agent is under study. For example, Loxo-195 is a recently developed drug, which phase 1/2 of the study started in 2017 in patients with TRK-positive solid tumors and TRK fusion-positive cancers (clinical trials NCT03215511 and NCT03206931). This drug could become an alternative treatment for tumors with acquired resistance to first-generation TRK-targeted agents (142). VMD-928 is another specific TRK inhibitor which is under phase 1 of the study since 2018 for treatment of advanced adult solid tumors or lymphoma (NCT03556228).
Because TRK overexpression is present in gynecological cancers, and particularly TRK fusion has been described in cervical and uterine cancer (143, 144), the use of TRK inhibitors could be beneficial in these kinds of neoplasms. However, it is necessary to continue the studies to determine their effectiveness in gynecological cancers.
Conclusions
NGF/TRKA and BDNF/TRKB are the main NTs studied in the context of cancer. These NTs and their receptors are over-expressed in gynecological neoplasms, such as ovarian and cervical cancers, in which they promote the progression of these diseases. Furthermore, NTs are involved in uterine pathologies such as endometriosis, which suggests that they could contribute to endometrial cancer progression, however this has not been elucidated yet. NTs are indirect angiogenic factors, acting through the induction of VEGF expression in ovarian cancer cells; besides, it is possible that NTs could display the same effect in other cancer cells such as cervical and endometrial. In addition, NTs exhibit a direct angiogenic role, mainly studied in endothelial cells that express NTs receptors, and respond by increasing endothelial cell proliferation, migration and differentiation. Moreover, NTs increases angiogenesis both in in vitro and in vivo models. Consequently, NTs and their receptors may be considered as important angiogenic factors, mostly in the context of anti-angiogenic therapy against VEGF, where overexpression of NTs could increase the angiogenesis independent of VEGF levels and contribute to therapy failure. Since NTs and TRK receptors are drivers of a wide variety of adult and pediatric cancers as gynecological neoplasms, the FDA has recently approved pan-TRK inhibitors for the treatment of TRK fusion-positive solid tumors. Because TRK fusion has been described in several gynecological cancers, the recently developed TRK inhibitors emerge as a new therapeutic approach for the treatment in this subtype of neoplasms. Given that angiogenesis is a key feature in gynecological neoplasms, and NTs acts as direct and indirect angiogenic factors, it may be relevant to study whether TRK inhibitors could improve the efficacy of anti-angiogenic drugs as bevacizumab, which was not elucidated yet.
Author Contributions
MG, IT, and CR: conceptualization. MG: writing original draft. MV and CR: writing, review, and editing.
Funding
National Fund for Scientific and Technological Development (FONDECYT) #1160139.
Conflict of Interest Statement
The authors declare that the research was conducted in the absence of any commercial or financial relationships that could be construed as a potential conflict of interest.
References
1. World Cancer Research Fund International. Worldwide Cancer Data. (2019). Availableonline at: https://www.wcrf.org/dietandcancer/cancer-trends/worldwide-cancer-data (accessed June 13, 2019).
2. Shweiki D, Itin A, Soffer D, Keshet E. Vascular endothelial growth factor induced by hypoxia may mediate hypoxia-initiated angiogenesis. Nature. (1992) 359:843–5. doi: 10.1038/359843a0
3. Zand B, Coleman RL, Sood AK. Targeting angiogenesis in gynecologic cancers. Hematol Oncol Clin North Am. (2012) 26:543–63. doi: 10.1016/j.hoc.2012.01.009
4. Tapia V, Gabler F, Munoz M, Yazigi R, Paredes A, Selman A, et al. Tyrosine kinase A receptor (trkA): a potential marker in epithelial ovarian cancer. Gynecol Oncol. (2011) 121:13–23. doi: 10.1016/j.ygyno.2010.12.341
5. Au CW, Siu MK, Liao X, Wong ES, Ngan HY, Tam KF, et al. Tyrosine kinase B receptor and BDNF expression in ovarian cancers - Effect on cell migration, angiogenesis and clinical outcome. Cancer Lett. (2009) 281:151–61. doi: 10.1016/j.canlet.2009.02.025
6. Moon A, Won KY, Lee JY, Kang I, Lee SK, Lee J. Expression of BDNF, TrkB, and p53 in early-stage squamous cell carcinoma of the uterine cervix. Pathology. (2011) 43:453–8. doi: 10.1097/PAT.0b013e3283484a3a
7. Yuan Y, Ye HQ, Ren QC. Proliferative role of BDNF/TrkB signaling is associated with anoikis resistance in cervical cancer. Oncol Rep. (2018) 40:621–34. doi: 10.3892/or.2018.6515
8. Snider WD. Functions of the neurotrophins during nervous system development: what the knockouts are teaching us. Cell. (1994) 77:627–38. doi: 10.1016/0092-8674(94)90048-5
9. Ballard O, Morrow AL. Human milk composition: nutrients and bioactive factors. Pediatr Clin North Am. (2013) 60:49–74. doi: 10.1016/j.pcl.2012.10.002
10. Colitti M. Expression of NGF, BDNF and their high-affinity receptors in ovine mammary glands during development and lactation. Histochem Cell Biol. (2015) 144:559–70. doi: 10.1007/s00418-015-1360-0
11. Dissen GA, Hirshfield AN, Malamed S, Ojeda SR. Expression of neurotrophins and their receptors in the mammalian ovary is developmentally regulated: changes at the time of folliculogenesis. Endocrinology. (1995) 136:4681–92. doi: 10.1210/endo.136.10.7664689
12. Wessels JM, Wu L, Leyland NA, Wang H, Foster WG. The brain-uterus connection: brain derived neurotrophic factor (BDNF) and its receptor (Ntrk2) are conserved in the mammalian uterus. PLoS ONE. (2014) 9:e94036. doi: 10.1371/journal.pone.0094036
13. Camerino C, Conte E, Cannone M, Caloiero R, Fonzino A, Tricarico D. Nerve growth factor, brain-derived neurotrophic factor and osteocalcin gene relationship in energy regulation, bone homeostasis and reproductive organs analyzed by mRNA quantitative evaluation and linear correlation analysis. Front Physiol. (2016) 7:456. doi: 10.3389/fphys.2016.00456
14. Noh SJ, Bae JS, Jamiyandorj U, Park HS, Kwon KS, Jung SH, et al. Expression of nerve growth factor and heme oxygenase-1 predict poor survival of breast carcinoma patients. BMC Cancer. (2013) 13:516. doi: 10.1186/1471-2407-13-516
15. Tsai YF, Tseng LM, Hsu CY, Yang MH, Chiu JH, Shyr YM. Brain-derived neurotrophic factor (BDNF) -TrKB signaling modulates cancer-endothelial cells interaction and affects the outcomes of triple negative breast cancer. PLoS ONE. (2017) 12:e0178173. doi: 10.1371/journal.pone.0178173
16. Yu X, Liu Z, Hou R, Nie Y, Chen R. Nerve growth factor and its receptors on onset and diagnosis of ovarian cancer. Oncol Lett. (2017) 14:2864–8. doi: 10.3892/ol.2017.6527
17. Adriaenssens E, Vanhecke E, Saule P, Mougel A, Page A, Romon R, et al. Nerve growth factor is a potential therapeutic target in breast cancer. Cancer Res. (2008) 68:346–51. doi: 10.1158/0008-5472.CAN-07-1183
18. Saleh Gargari S, Taheri M, Kholghi Oskooei V, Omrani MD, Ghafouri-Fard S. Transcription levels of nicotinamide nucleotide transhydrogenase and its antisense in breast cancer samples. Cell J. (2019) 21:331–6. doi: 10.22074/cellj.2019.6238
19. Carmeliet P, Jain RK. Angiogenesis in cancer and other diseases. Nature. (2000) 407:249–57. doi: 10.1038/35025220
20. Weidner N. Intratumor microvessel density as a prognostic factor in cancer. Am J Pathol. (1995) 147:9–19.
21. Folkman J. History of angiogenesis. In: Figg WD, Folkman J. Angiogenesis. New York, NY: Springer (2008). p. 18–31.
22. Zuazo-Gaztelu I, Casanovas O. Unraveling the role of angiogenesis in cancer ecosystems. Front Oncol. (2018) 8:248. doi: 10.3389/fonc.2018.00248
24. Bielenberg DR, Zetter BR. The contribution of angiogenesis to the process of metastasis. Cancer J. (2015) 21:267–73. doi: 10.1097/PPO.0000000000000138
25. Knighton D, Ausprunk D, Tapper D, Folkman J. Avascular and vascular phases of tumour growth in the chick embryo. Br J Cancer. (1977) 35:347–56. doi: 10.1038/bjc.1977.49
26. Bussolino F, Albini A, Camussi G, Presta M, Viglietto G, Ziche M, et al. Role of soluble mediators in angiogenesis. Eur J Cancer. (1996) 32A:2401–12. doi: 10.1016/S0959-8049(96)00390-5
27. Hida K, Hida Y, Amin DN, Flint AF, Panigrahy D, Morton CC, et al. Tumor-associated endothelial cells with cytogenetic abnormalities. Cancer Res. (2004) 64:8249–55. doi: 10.1158/0008-5472.CAN-04-1567
28. Streubel B, Chott A, Huber D, Exner M, Jager U, Wagner O, et al. Lymphoma-specific genetic aberrations in microvascular endothelial cells in B-cell lymphomas. N Engl J Med. (2004) 351:250–9. doi: 10.1056/NEJMoa033153
29. Akino T, Hida K, Hida Y, Tsuchiya K, Freedman D, Muraki C, et al. Cytogenetic abnormalities of tumor-associated endothelial cells in human malignant tumors. Am J Pathol. (2009) 175:2657–67. doi: 10.2353/ajpath.2009.090202
30. Hashizume H, Baluk P, Morikawa S, McLean JW, Thurston G, Roberge S, et al. Openings between defective endothelial cells explain tumor vessel leakiness. Am J Pathol. (2000) 156:1363–80. doi: 10.1016/S0002-9440(10)65006-7
31. Dudley AC. Tumor endothelial cells. Cold Spring Harb Perspect Med. (2012) 2:a006536. doi: 10.1101/cshperspect.a006536
32. Padera TP, Stoll BR, Tooredman JB, Capen D, di Tomaso E, Jain RK. Pathology: cancer cells compress intratumour vessels. Nature. (2004) 427:695. doi: 10.1038/427695a
33. Augustin HG, Braun K, Telemenakis I, Modlich U, Kuhn W. Ovarian angiogenesis. Phenotypic characterization of endothelial cells in a physiological model of blood vessel growth and regression. Am J Pathol. (1995) 147:339–51.
34. Modlich U, Kaup FJ, Augustin HG. Cyclic angiogenesis and blood vessel regression in the ovary: blood vessel regression during luteolysis involves endothelial cell detachment and vessel occlusion. Lab Invest. (1996) 74:771–80.
35. Fraser HM, Lunn SF. Regulation and manipulation of angiogenesis in the primate corpus luteum. Reproduction. (2001) 121:355–62. doi: 10.1530/rep.0.1210355
36. Ribatti D. The chick embryo chorioallantoic membrane (CAM) assay. Reprod Toxicol. (2016) 70:97–101. doi: 10.1016/j.reprotox.2016.11.004
37. Schuermann A, Helker CS, Herzog W. Angiogenesis in zebrafish. Semin Cell Dev Biol. (2014) 31:106–14. doi: 10.1016/j.semcdb.2014.04.037
38. Chavez MN, Aedo G, Fierro FA, Allende ML, Egana JT. Zebrafish as an emerging model organism to study angiogenesis in development and regeneration. Front Physiol. (2016) 7:56. doi: 10.3389/fphys.2016.00056
39. Rogers MS, Birsner AE, D'Amato RJ. The mouse cornea micropocket angiogenesis assay. Nat Protoc. (2007) 2:2545–50. doi: 10.1038/nprot.2007.368
40. Nakao S, Hafezi-Moghadam A. The corneal micropocket assay: a model of angiogenesis and lymphangiogenesis. Methods Mol Biol. (2016) 1430:311–6. doi: 10.1007/978-1-4939-3628-1_21
41. Akhtar N, Dickerson EB, Auerbach R. The sponge/Matrigel angiogenesis assay. Angiogenesis. (2002) 5:75–80. doi: 10.1023/A:1021507031486
42. Arnaoutova I, Kleinman HK. In vitro angiogenesis: endothelial cell tube formation on gelled basement membrane extract. Nat Protoc. (2010) 5:628–35. doi: 10.1038/nprot.2010.6
43. Ponce ML. Tube formation: an in vitro Matrigel angiogenesis assay. Methods Mol Biol. (2009) 467:183–8. doi: 10.1007/978-1-59745-241-0_10
44. Khoo CP, Micklem K, Watt SM. A comparison of methods for quantifying angiogenesis in the Matrigel assay in vitro. Tissue Eng Part C Methods. (2011) 17:895–906. doi: 10.1089/ten.tec.2011.0150
45. Young EW. Advances in microfluidic cell culture systems for studying angiogenesis. J Lab Autom. (2013) 18:427–36. doi: 10.1177/2211068213495206
46. Simons M, Alitalo K, Annex BH, Augustin HG, Beam C, Berk BC, et al. State-of-the-art methods for evaluation of angiogenesis and tissue vascularization: a scientific statement from the American Heart Association. Circ Res. (2015) 116:e99–132. doi: 10.1161/RES.0000000000000054
47. Li X, Eriksson U. Novel VEGF family members: VEGF-B, VEGF-C and VEGF-D. Int J Biochem Cell Biol. (2001) 33:421–6. doi: 10.1016/S1357-2725(01)00027-9
48. Hoeben A, Landuyt B, Highley MS, Wildiers H, Van Oosterom AT, De Bruijn EA. Vascular endothelial growth factor and angiogenesis. Pharmacol Rev. (2004) 56:549–80. doi: 10.1124/pr.56.4.3
49. Lal N, Puri K, Rodrigues B. Vascular endothelial growth factor B and its signaling. Front Cardiovasc Med. (2018) 5:39. doi: 10.3389/fcvm.2018.00039
50. Rauniyar K, Jha SK, Jeltsch M. Biology of vascular endothelial growth factor C in the morphogenesis of lymphatic vessels. Front Bioeng Biotechnol. (2018) 6:7. doi: 10.3389/fbioe.2018.00007
51. Holmes DI, Zachary I. The vascular endothelial growth factor (VEGF) family: angiogenic factors in health and disease. Genome Biol. (2005) 6:209. doi: 10.1186/gb-2005-6-2-209
52. Akiri G, Nahari D, Finkelstein Y, Le SY, Elroy-Stein O, Levi BZ. Regulation of vascular endothelial growth factor (VEGF) expression is mediated by internal initiation of translation and alternative initiation of transcription. Oncogene. (1998) 17:227–36. doi: 10.1038/sj.onc.1202019
53. Peach CJ, Mignone VW, Arruda MA, Alcobia DC, Hill SJ, Kilpatrick LE, et al. Molecular pharmacology of VEGF-A isoforms: binding and signalling at VEGFR2. Int J Mol Sci. (2018) 19:E1264. doi: 10.3390/ijms19041264
54. Tischer E, Mitchell R, Hartman T, Silva M, Gospodarowicz D, Fiddes JC, et al. The human gene for vascular endothelial growth factor. Multiple protein forms are encoded through alternative exon splicing. J Biol Chem. (1991) 266:11947–54.
55. Ferrara N, Houck KA, Jakeman LB, Winer J, Leung DW. The vascular endothelial growth factor family of polypeptides. J Cell Biochem. (1991) 47:211–8. doi: 10.1002/jcb.240470305
56. Guzman-Hernandez ML, Potter G, Egervari K, Kiss JZ, Balla T. Secretion of VEGF-165 has unique characteristics, including shedding from the plasma membrane. Mol Biol Cell. (2014) 25:1061–72. doi: 10.1091/mbc.e13-07-0418
57. Fujimoto J, Ichigo S, Hirose R, Sakaguchi H, Tamaya T. Expressions of vascular endothelial growth factor (VEGF) and its mRNA in uterine endometrial cancers. Cancer Lett. (1998) 134:15–22. doi: 10.1016/S0304-3835(98)00232-8
58. Bermont L, Lamielle F, Fauconnet S, Esumi H, Weisz A, Adessi GL. Regulation of vascular endothelial growth factor expression by insulin-like growth factor-I in endometrial adenocarcinoma cells. Int J Cancer. (2000) 85:117–23. doi: 10.1002/(SICI)1097-0215(20000101)85:1<117::AID-IJC21>3.0.CO;2-X
59. Stimpfl M, Tong D, Fasching B, Schuster E, Obermair A, Leodolter S, et al. Vascular endothelial growth factor splice variants and their prognostic value in breast and ovarian cancer. Clin Cancer Res. (2002) 8:2253–9.
60. Campos X, Munoz Y, Selman A, Yazigi R, Moyano L, Weinstein-Oppenheimer C, et al. Nerve growth factor and its high-affinity receptor trkA participate in the control of vascular endothelial growth factor expression in epithelial ovarian cancer. Gynecol Oncol. (2007) 104:168–75. doi: 10.1016/j.ygyno.2006.07.007
61. Skaper SD. The biology of neurotrophins, signalling pathways, and functional peptide mimetics of neurotrophins and their receptors. CNS Neurol Disord Drug Targets. (2008) 7:46–62. doi: 10.2174/187152708783885174
62. Cohen S, Levi-Montalcini R. A nerve growth-stimulating factor isolated from snake venom. Proc Natl Acad Sci USA. (1956) 42:571–4. doi: 10.1073/pnas.42.9.571
63. Hallbook F. Evolution of the vertebrate neurotrophin and Trk receptor gene families. Curr Opin Neurobiol. (1999) 9:616–21. doi: 10.1016/S0959-4388(99)00011-2
64. Reichardt LF. Neurotrophin-regulated signalling pathways. Philos Trans R Soc Lond B Biol Sci. (2006) 361:1545–64. doi: 10.1098/rstb.2006.1894
65. Russo N, Russo M, Daino D, Freschi L, Fiore L, Merlini S, et al. Evaluation of brain-derived neurotrophic factor in menstrual blood and its identification in human endometrium. Gynecol Endocrinol. (2012) 28:492–5. doi: 10.3109/09513590.2011.633667
66. Dissen GA, Mayerhofer A, Ojeda SR. Participation of nerve growth factor in the regulation of ovarian function. Zygote. (1996) 4:309–12. doi: 10.1017/S0967199400003300
67. Mayerhofer A, Dissen GA, Parrott JA, Hill DF, Mayerhofer D, Garfield RE, et al. Involvement of nerve growth factor in the ovulatory cascade: trkA receptor activation inhibits gap junctional communication between thecal cells. Endocrinology. (1996) 137:5662–70. doi: 10.1210/endo.137.12.8940397
68. Dissen GA, Romero C, Hirshfield AN, Ojeda SR. Nerve growth factor is required for early follicular development in the mammalian ovary. Endocrinology. (2001) 142:2078–86. doi: 10.1210/endo.142.5.8126
69. Spears N, Molinek MD, Robinson LL, Fulton N, Cameron H, Shimoda K, et al. The role of neurotrophin receptors in female germ-cell survival in mouse and human. Development. (2003) 130:5481–91. doi: 10.1242/dev.00707
70. Paredes A, Romero C, Dissen GA, DeChiara TM, Reichardt L, Cornea A, et al. TrkB receptors are required for follicular growth and oocyte survival in the mammalian ovary. Dev Biol. (2004) 267:430–49. doi: 10.1016/j.ydbio.2003.12.001
71. Romero C, Paredes A, Dissen GA, Ojeda SR. Nerve growth factor induces the expression of functional FSH receptors in newly formed follicles of the rat ovary. Endocrinology. (2002) 143:1485–94. doi: 10.1210/endo.143.4.8711
72. Salas C, Julio-Pieper M, Valladares M, Pommer R, Vega M, Mastronardi C, et al. Nerve growth factor-dependent activation of trkA receptors in the human ovary results in synthesis of follicle-stimulating hormone receptors and estrogen secretion. J Clin Endocrinol Metab. (2006) 91:2396–403. doi: 10.1210/jc.2005-1925
73. Kawamura K, Kawamura N, Mulders SM, Sollewijn Gelpke MD, Hsueh AJ. Ovarian brain-derived neurotrophic factor (BDNF) promotes the development of oocytes into preimplantation embryos. Proc Natl Acad Sci USA. (2005) 102:9206–11. doi: 10.1073/pnas.0502442102
74. Yu Y, Yan J, Li M, Yan L, Zhao Y, Lian Y, et al. Effects of combined epidermal growth factor, brain-derived neurotrophic factor and insulin-like growth factor-1 on human oocyte maturation and early fertilized and cloned embryo development. Hum Reprod. (2012) 27:2146–59. doi: 10.1093/humrep/des099
75. Wang X, Sun Z, Zhen J, Yu Q. Brain-derived neurotrophic factor from follicular fluid is positively associated with rate of mature ooocytes collected and cleavage rate in intracytoplasmic sperm injection patients. J Assist Reprod Genet. (2011) 28:1053–8. doi: 10.1007/s10815-011-9635-4
76. Monteleone P, Artini PG, Simi G, Cela V, Casarosa E, Begliuomini S, et al. Brain derived neurotrophic factor circulating levels in patients undergoing IVF. J Assist Reprod Genet. (2007) 24:477–80. doi: 10.1007/s10815-007-9169-y
77. Seifer DB, Feng B, Shelden RM, Chen S, Dreyfus CF. Brain-derived neurotrophic factor: a novel human ovarian follicular protein. J Clin Endocrinol Metab. (2002) 87:655–9. doi: 10.1210/jcem.87.2.8213
78. Kawamura K, Kawamura N, Fukuda J, Kumagai J, Hsueh AJ, Tanaka T. Regulation of preimplantation embryo development by brain-derived neurotrophic factor. Dev Biol. (2007) 311:147–58. doi: 10.1016/j.ydbio.2007.08.026
79. Linher-Melville K, Li J. The roles of glial cell line-derived neurotrophic factor, brain-derived neurotrophic factor and nerve growth factor during the final stage of folliculogenesis: a focus on oocyte maturation. Reproduction. (2013) 145:R43–54. doi: 10.1530/REP-12-0219
80. Wang DH, Ren J, Zhou CJ, Han Z, Wang L, Liang CG. Supplementation with CTGF, SDF1, NGF, and HGF promotes ovine in vitro oocyte maturation and early embryo development. Domest Anim Endocrinol. (2018) 65:38–48. doi: 10.1016/j.domaniend.2018.05.003
81. Maranesi M, Parillo F, Leonardi L, Rebollar PG, Alonso B, Petrucci L, et al. Expression of nerve growth factor and its receptors in the uterus of rabbits: functional involvement in prostaglandin synthesis. Domest Anim Endocrinol. (2016) 56:20–8. doi: 10.1016/j.domaniend.2016.02.001
82. The Human Protein Atlas. NGF. (2019). Available online at: https://www.proteinatlas.org/ENSG00000134259-NGF/tissue (accessed June 13, 2019).
83. Frank P, Barrientos G, Tirado-Gonzalez I, Cohen M, Moschansky P, Peters EM, et al. Balanced levels of nerve growth factor are required for normal pregnancy progression. Reproduction. (2014) 148:179–89. doi: 10.1530/REP-14-0112
84. Cantarella G, Lempereur L, Presta M, Ribatti D, Lombardo G, Lazarovici P, et al. Nerve growth factor-endothelial cell interaction leads to angiogenesis in vitro and in vivo. FASEB J. (2002) 16:1307–9. doi: 10.1096/fj.01-1000fje
85. Lazarovici P, Gazit A, Staniszewska I, Marcinkiewicz C, Lelkes PI. Nerve growth factor (NGF) promotes angiogenesis in the quail chorioallantoic membrane. Endothelium. (2006) 13:51–9. doi: 10.1080/10623320600669053
86. Romon R, Adriaenssens E, Lagadec C, Germain E, Hondermarck H, Le Bourhis X. Nerve growth factor promotes breast cancer angiogenesis by activating multiple pathways. Mol Cancer. (2010) 9:157. doi: 10.1186/1476-4598-9-157
87. Garrido MP, Vera C, Vega M, Quest AFG, Romero C. Metformin prevents nerve growth factor-dependent proliferative and proangiogenic effects in epithelial ovarian cancer cells and endothelial cells. Ther Adv Med Oncol. (2018) 10:1758835918770984. doi: 10.1177/1758835918770984
88. Aranda E, Owen GI. A semi-quantitative assay to screen for angiogenic compounds and compounds with angiogenic potential using the EA.hy926 endothelial cell line. Biol Res. (2009) 42:377–89. doi: 10.4067/S0716-97602009000300012
89. Donovan MJ, Lin MI, Wiegn P, Ringstedt T, Kraemer R, Hahn R, et al. Brain derived neurotrophic factor is an endothelial cell survival factor required for intramyocardial vessel stabilization. Development. (2000) 127:4531–40.
90. Usui T, Naruo A, Okada M, Hayabe Y, Yamawaki H. Brain-derived neurotrophic factor promotes angiogenic tube formation through generation of oxidative stress in human vascular endothelial cells. Acta Physiol. (2014) 211:385–94. doi: 10.1111/apha.12249
91. Lam CT, Yang ZF, Lau CK, Tam KH, Fan ST, Poon RT. Brain-derived neurotrophic factor promotes tumorigenesis via induction of neovascularization: implication in hepatocellular carcinoma. Clin Cancer Res. (2011) 17:3123–33. doi: 10.1158/1078-0432.CCR-10-2802
92. Julio-Pieper M, Lozada P, Tapia V, Vega M, Miranda C, Vantman D, et al. Nerve growth factor induces vascular endothelial growth factor expression in granulosa cells via a trkA receptor/mitogen-activated protein kinase-extracellularly regulated kinase 2-dependent pathway. J Clin Endocrinol Metab. (2009) 94:3065–71. doi: 10.1210/jc.2009-0542
93. Zhang Z, Zhang Y, Zhou Z, Shi H, Qiu X, Xiong J, et al. BDNF regulates the expression and secretion of VEGF from osteoblasts via the TrkB/ERK1/2 signaling pathway during fracture healing. Mol Med Rep. (2017) 15:1362–7. doi: 10.3892/mmr.2017.6110
94. Calza L, Giardino L, Giuliani A, Aloe L, Levi-Montalcini R. Nerve growth factor control of neuronal expression of angiogenetic and vasoactive factors. Proc Natl Acad Sci USA. (2001) 98:4160–5. doi: 10.1073/pnas.051626998
95. Lin CY, Hung SY, Chen HT, Tsou HK, Fong YC, Wang SW, et al. Brain-derived neurotrophic factor increases vascular endothelial growth factor expression and enhances angiogenesis in human chondrosarcoma cells. Biochem Pharmacol. (2014) 91:522–33. doi: 10.1016/j.bcp.2014.08.008
96. Nakamura K, Martin KC, Jackson JK, Beppu K, Woo CW, Thiele CJ. Brain-derived neurotrophic factor activation of TrkB induces vascular endothelial growth factor expression via hypoxia-inducible factor-1alpha in neuroblastoma cells. Cancer Res. (2006) 66:4249–55. doi: 10.1158/0008-5472.CAN-05-2789
97. Halade GV, Ma Y, Ramirez TA, Zhang J, Dai Q, Hensler JG, et al. Reduced BDNF attenuates inflammation and angiogenesis to improve survival and cardiac function following myocardial infarction in mice. Am J Physiol Heart Circ Physiol. (2013) 305:H1830–42. doi: 10.1152/ajpheart.00224.2013
98. Oberaigner W, Minicozzi P, Bielska-Lasota M, Allemani C, de Angelis R, Mangone L, et al. Survival for ovarian cancer in Europe: the across-country variation did not shrink in the past decade. Acta Oncol. (2012) 51:441–53. doi: 10.3109/0284186X.2011.653437
99. Doufekas K, Olaitan A. Clinical epidemiology of epithelial ovarian cancer in the UK. Int J Womens Health. (2014) 6:537–45. doi: 10.2147/IJWH.S40894
100. Siegel RL, Miller KD, Jemal A. Cancer statistics, 2019. CA Cancer J Clin. (2019) 69:7–34. doi: 10.3322/caac.21551
101. Hennessy BT, Coleman RL, Markman M. Ovarian cancer. Lancet. (2009) 374:1371–82. doi: 10.1016/S0140-6736(09)61338-6
102. Jemal A, Bray F, Center MM, Ferlay J, Ward E, Forman D. Global cancer statistics. CA Cancer J Clin. (2011) 61:69–90. doi: 10.3322/caac.20107
103. Doubeni CA, Doubeni AR, Myers AE. Diagnosis and management of ovarian cancer. Am Fam Physician. (2016) 93:937–44.
104. Urzua U, Tapia V, Geraldo MP, Selman A, Vega M, Romero C. Nerve growth factor stimulates cellular proliferation of human epithelial ovarian cancer. Horm Metab Res. (2012) 44:656–61. doi: 10.1055/s-0032-1304617
105. Vera C, Tapia V, Vega M, Romero C. Role of nerve growth factor and its TRKA receptor in normal ovarian and epithelial ovarian cancer angiogenesis. J Ovarian Res. (2014) 7:82. doi: 10.1186/s13048-014-0082-6
106. Matsumoto K, Wada RK, Yamashiro JM, Kaplan DR, Thiele CJ. Expression of brain-derived neurotrophic factor and p145TrkB affects survival, differentiation, and invasiveness of human neuroblastoma cells. Cancer Res. (1995) 55:1798–806.
107. Dionne CA, Camoratto AM, Jani JP, Emerson E, Neff N, Vaught JL, et al. Cell cycle-independent death of prostate adenocarcinoma is induced by the trk tyrosine kinase inhibitor CEP-751 (KT6587). Clin Cancer Res. (1998) 4:1887–98.
108. Miknyoczki SJ, Lang D, Huang L, Klein-Szanto AJ, Dionne CA, Ruggeri BA. Neurotrophins and Trk receptors in human pancreatic ductal adenocarcinoma: expression patterns and effects on in vitro invasive behavior. Int J Cancer. (1999) 81:417–27. doi: 10.1002/(SICI)1097-0215(19990505)81:3<417::AID-IJC16>3.0.CO;2-6
109. Perez-Pinera P, Hernandez T, Garcia-Suarez O, de Carlos F, Germana A, Del Valle M, et al. The Trk tyrosine kinase inhibitor K252a regulates growth of lung adenocarcinomas. Mol Cell Biochem. (2007) 295:19–26. doi: 10.1007/s11010-006-9267-7
110. Yu X, Liu L, Cai B, He Y, Wan X. Suppression of anoikis by the neurotrophic receptor TrkB in human ovarian cancer. Cancer Sci. (2008) 99:543–52. doi: 10.1111/j.1349-7006.2007.00722.x
111. Cittelly DM, Dimitrova I, Howe EN, Cochrane DR, Jean A, Spoelstra NS, et al. Restoration of miR-200c to ovarian cancer reduces tumor burden and increases sensitivity to paclitaxel. Mol Cancer Ther. (2012) 11:2556–65. doi: 10.1158/1535-7163.MCT-12-0463
112. Lim WC, Kim H, Kim YJ, Park SH, Song JH, Lee KH, et al. Delphinidin inhibits BDNF-induced migration and invasion in SKOV3 ovarian cancer cells. Bioorg Med Chem Lett. (2017) 27:5337–43. doi: 10.1016/j.bmcl.2017.09.024
113. Zheng W, Dai Q, Tao P, Sun A, Wang Y, Bao L, et al. Overexpression of tyrosine kinase receptor B promotes metastasis of ovarian serous adenocarcinoma by lymphangiogenesis. Tumori. (2011) 97:756–61. doi: 10.1177/030089161109700613
114. Garrido MP, Selman A, Gabler F, Vega M, Romero C. Abstract A26: Levels of phospho-Connexin 43 and phospho-TRKB in epithelial ovarian cancer. Clin Cancer Res. (2016) 22:A26. doi: 10.1158/1557-3265.OVCA15-A26
115. Sasahira T, Ueda N, Yamamoto K, Bhawal UK, Kurihara M, Kirita T, et al. Trks are novel oncogenes involved in the induction of neovascularization, tumor progression, and nodal metastasis in oral squamous cell carcinoma. Clin Exp Metastasis. (2012) 30:165–76. doi: 10.1007/s10585-012-9525-x
116. World Health Organization. Cervical Cancer. (2019). Available online at: https://www.who.int/cancer/prevention/diagnosis-screening/cervical-cancer/en/ (accessed June 13, 2019).
117. Sun CY, Chu ZB, Huang J, Chen L, Xu J, Xu AS, et al. siRNA-mediated inhibition of endogenous brainderived neurotrophic factor gene modulates the biological behavior of HeLa cells. Oncol Rep. (2017) 37:2751–60. doi: 10.3892/or.2017.5569
118. Yuan Y, Ye HQ, Ren QC. Upregulation of the BDNF/TrKB pathway promotes epithelial-mesenchymal transition, as well as the migration and invasion of cervical cancer. Int J Oncol. (2018) 52:461–72.
119. Trisciuoglio D, Iervolino A, Zupi G, Del Bufalo D. Involvement of PI3K and MAPK signaling in bcl-2-induced vascular endothelial growth factor expression in melanoma cells. Mol Biol Cell. (2005) 16:4153–62. doi: 10.1091/mbc.e04-12-1087
120. Karar J, Maity A. PI3K/AKT/mTOR pathway in angiogenesis. Front Mol Neurosci. (2011) 4:51. doi: 10.3389/fnmol.2011.00051
121. Chen JG, Fan HY, Wang T, Lin LY, Cai TG. Silencing KRT16 inhibits keratinocyte proliferation and VEGF secretion in psoriasis via inhibition of ERK signaling pathway. Kaohsiung J Med Sci. (2019) 35:284–96. doi: 10.1002/kjm2.12034
122. Anaf V, Simon P, El Nakadi I, Fayt I, Simonart T, Buxant F, et al. Hyperalgesia, nerve infiltration and nerve growth factor expression in deep adenomyotic nodules, peritoneal and ovarian endometriosis. Hum Reprod. (2002) 17:1895–900. doi: 10.1093/humrep/17.7.1895
123. Browne AS, Yu J, Huang RP, Francisco AM, Sidell N, Taylor RN. Proteomic identification of neurotrophins in the eutopic endometrium of women with endometriosis. Fertil Steril. (2012) 98:713–9. doi: 10.1016/j.fertnstert.2012.05.027
124. Dewanto A, Dudas J, Glueckert R, Mechsner S, Schrott-Fischer A, Wildt L, et al. Localization of TrkB and p75 receptors in peritoneal and deep infiltrating endometriosis: an immunohistochemical study. Reprod Biol Endocrinol. (2016) 14:43. doi: 10.1186/s12958-016-0178-5
125. Burghaus S, Haberle L, Schrauder MG, Heusinger K, Thiel FC, Hein A, et al. Endometriosis as a risk factor for ovarian or endometrial cancer - results of a hospital-based case-control study. BMC Cancer. (2015) 15:751. doi: 10.1186/s12885-015-1821-9
126. Demirkiran F. Is endometriosis a preneoplastic condition? Womens Health. (2015) 11:701–3. doi: 10.2217/whe.15.57
127. Yu HC, Lin CY, Chang WC, Shen BJ, Chang WP, Chuang CM. Increased association between endometriosis and endometrial cancer: a nationwide population-based retrospective cohort study. Int J Gynecol Cancer. (2015) 25:447–52. doi: 10.1097/IGC.0000000000000384
128. Kennedy S, Bergqvist A, Chapron C, D'Hooghe T, Dunselman G, Greb R, et al. ESHRE guideline for the diagnosis and treatment of endometriosis. Hum Reprod. (2005) 20:2698–704. doi: 10.1093/humrep/dei135
129. McLaren J. Vascular endothelial growth factor and endometriotic angiogenesis. Hum Reprod Update. (2000) 6:45–55. doi: 10.1093/humupd/6.1.45
130. Makabe T, Koga K, Miyashita M, Takeuchi A, Sue F, Taguchi A, et al. Drospirenone reduces inflammatory cytokines, vascular endothelial growth factor (VEGF) and nerve growth factor (NGF) expression in human endometriotic stromal cells. J Reprod Immunol. (2017) 119:44–8. doi: 10.1016/j.jri.2016.12.002
131. Qin X, Liu Y, Feng Y, Jiang J. Ginsenoside Rf alleviates dysmenorrhea and inflammation through the BDNF-TrkB-CREB pathway in a rat model of endometriosis. Food Funct. (2019) 10:244–9. doi: 10.1039/C8FO01839A
132. FDA. FDA Approves Larotrectinib for Solid Tumors with NTRK Gene Fusions. (2018). Available online at: https://www.fda.gov/drugs/fda-approves-larotrectinib-solid-tumors-ntrk-gene-fusions-0 (accessed August 25, 2018).
133. Laetsch TW, DuBois SG, Mascarenhas L, Turpin B, Federman N, Albert CM, et al. Larotrectinib for paediatric solid tumours harbouring NTRK gene fusions: phase 1 results from a multicentre, open-label, phase 1/2 study. Lancet Oncol. (2018) 19:705–14. doi: 10.1016/S1470-2045(18)30119-0
134. Hong DS, Bauer TM, Lee JJ, Dowlati A, Brose MS, Farago AF, et al. Larotrectinib in adult patients with solid tumours: a multi-centre, open-label, phase I dose-escalation study. Ann Oncol. (2019) 30:325–31. doi: 10.1093/annonc/mdy539
135. National Cancer Institute. NCI Drug Dictionary: Larotrectinib Sulfate. (2019). Available online at: https://www.cancer.gov/publications/dictionaries/cancer-drug/def/larotrectinib (accessed August 25, 2019).
136. Flaherty KT, Le DT, Lemery S. Tissue-agnostic drug development. Am Soc Clin Oncol Educ Book. (2017) 37:222–30. doi: 10.14694/EDBK_173855
137. FDA. FDA Approves Entrectinib for NTRK Solid Tumors and ROS-1 NSCLC. (2019). Available online at: https://www.fda.gov/drugs/resources-information-approved-drugs/fda-approves-entrectinib-ntrk-solid-tumors-and-ros-1-nsclc (accessed August 25, 2019).
138. Al-Salama ZT, Keam SJ. Entrectinib: first global approval. Drugs. (2019) 79:1477–83. doi: 10.1007/s40265-019-01177-y
139. Cocco E, Schram AM, Kulick A, Misale S, Won HH, Yaeger R, et al. Resistance to TRK inhibition mediated by convergent MAPK pathway activation. Nat Med. (2019) 25:1422–7. doi: 10.1038/s41591-019-0542-z
140. Estrada-Bernal A, Le AT, Tuch B, Kutateladze T, Doebele RC. Abstract C65: TRK kinase domain mutations that induce resistance to a pan-TRK inhibitor. Mol Cancer Ther. (2015) 14:C65. doi: 10.1158/1535-7163.TARG-15-C65
141. Estrada-Bernal A, Le AT, Tuch B, Kutateladze TG, Doebele RC. Abstract LB-118: Identification of TRKA and TRKB kinase domain mutations that induce resistance to a pan-TRK inhibitor. Cancer Res. (2016) 76:LB–118. doi: 10.1158/1538-7445.AM2016-LB-118
142. Hyman D, Kummar S, Farago A, Geoerger B, Mau-Sorensen M, Taylor M, et al. Abstract CT127: phase I and expanded access experience of LOXO-195 (BAY 2731954), a selective next-generation TRK inhibitor (TRKi). Cancer Res. (2019) 79:CT127. doi: 10.1158/1538-7445.AM2019-CT127
143. Chiang S, Cotzia P, Hyman DM, Drilon A, Tap WD, Zhang L, et al. NTRK fusions define a novel uterine sarcoma subtype with features of fibrosarcoma. Am J Surg Pathol. (2018) 42:791–8. doi: 10.1097/PAS.0000000000001055
Keywords: gynecological cancers, angiogenesis, VEGF, BDNF, NGF
Citation: Garrido MP, Torres I, Vega M and Romero C (2019) Angiogenesis in Gynecological Cancers: Role of Neurotrophins. Front. Oncol. 9:913. doi: 10.3389/fonc.2019.00913
Received: 21 June 2019; Accepted: 02 September 2019;
Published: 19 September 2019.
Edited by:
Laurence A. Marchat, National Polytechnic Institute, MexicoReviewed by:
Rafael Roesler, Federal University of Rio Grande Do Sul, BrazilCaroline Brunetto De Farias, Children's Cancer Institute (ICI), Brazil
Copyright © 2019 Garrido, Torres, Vega and Romero. This is an open-access article distributed under the terms of the Creative Commons Attribution License (CC BY). The use, distribution or reproduction in other forums is permitted, provided the original author(s) and the copyright owner(s) are credited and that the original publication in this journal is cited, in accordance with accepted academic practice. No use, distribution or reproduction is permitted which does not comply with these terms.
*Correspondence: Carmen Romero, Y3JvbWVybyYjeDAwMDQwO2hjdWNoLmNs