- 1Department of General Surgery, Peking Union Medical College Hospital, Chinese Academy of Medical Sciences and Peking Union Medical College, Beijing, China
- 2Peking Union Medical College, Chinese Academy of Medical Sciences and Peking Union Medical College, Beijing, China
- 3Clinical Immunology Center, Chinese Academy of Medical Sciences and Peking Union Medical College, Beijing, China
MicroRNAs (miRNAs) are a family of highly conserved, non-coding single-stranded RNAs transcribed as ~70 nucleotide precursors to an 18–22 nucleotide product (1). miRNAs can silence their homologous target genes at the post-transcriptional level, and these genes have been revealed to play an important role in tumorigenesis, invasion and metastasis (2). MicroRNA-27a (miR-27a), transcripted by miR-27a gene, has proved to implicate with many kinds of solid tumors, showing potential as a useful biomarker or drug target for clinical application. However, even though miR-27a has been reported in many cancers, the mechanism and signal pathways of miR-27 in oncogenesis, invasion, and metastasis are still obscure. Moreover, recent studies show that miR-27a pays an important role in epithelial-mesenchymal-transition, regulating tumor immune response, and chemoresistance. In this review, we summarize the current literature, demonstrate the established link between miR-27a and tumorigenesis, and focus on recently identified mechanisms. The review also aims to demonstrate the potential of miR-27a as a diagnostic and/or prognostic biomarker in solid tumors and to discuss the possibilities of targeted therapy and drug design.
Introduction
Since microRNAs (miRNAs) were discovered in Caenorhabditis elegans as important non-coding (18–22 nucleotides, nt) RNA molecules, they have attracted the attention of many researchers for their multifaceted roles in controlling cancer development by regulating target genes (3–5). miRNAs show variable distribution and expression features in different kinds of solid cancer cells, showing diverse regulatory mechanisms for deep research (6). After decades of progress, hundreds of miRNAs, beginning with line-4 miRNA to let-7 miRNA to the miRNA family, were discovered, and their functions in tumor cells have been partially explained. MicroRNA-27a, transcribed by the miR-27a gene on chromosome 19p13.13, is an important member of the miRNA family. The mature miR-27a is 22 nt long and forms one strand of the RNA duplex. With the cooperation of the RNA-induced silencing complex (RISC), miR-27a can exert different regulatory functions in different kinds of cancer. miR-27a was first implicated in breast cancer, in which high miR-27a expression increased the percentage of cells in G2/M stage, resulting in an oncogenic function (7). miR-27a was also found to be upregulated in ovarian cancer cells and prostate cancer cells and showed good potential for therapeutic applications (8, 9). In recent years, because of the heterogeneity and variability of genetic expression features of many specific oncogenes or tumor suppressors in gastrointestinal cancer, miR-27a has also been studied in gastrointestinal cancer, opening a broad area for research. For example, miR-27a is upregulated in gastric adenocarcinoma, while the suppression of miR-27a can reestablish the sensitivity of tumor cells to chemotherapeutic drugs (10, 11). It has been concluded that miR-27a plays an important role in oncogenesis, cell proliferation, tumor cell metabolism, and chemotherapy resistance. In recent years, with the further recognition of tumor biogenesis, miR-27a shows novel functions in regulating the tumor immune response and epithelial-mesenchymal transition (EMT) (12, 13). With further research on miRNA exosomes, miR-27a showed good potential use in the clinical therapeutic or prognostic areas (14). However, although miR-27a has been shown to have important research value, we found that studies on miR-27a in various tumors are diverse, and studies on the mechanisms and signaling pathways of miR-27a are inadequate. Clinical studies on the use of miR-27a as a diagnostic or prognostic biomarker are also needed. In this review, we summarize the recent developments in miR-27a research, analyze the biogenesis and functional features of miR-27a, focus on the key signaling pathways affected by miR-27a in cancer cells, summarize recent clinical research on miR-27a as a biomarker or therapeutic target, and provide directions for further studies.
Biogenesis of miR-27a in Solid Tumors
The canonical biogenesis of a miRNA has been defined and explained by previous research (3, 15). In cancer cells, the well-regulated miRNA biogenesis pathways are dysregulated since there is a shift in gene expression and dysregulation of the key enzymes, leading to the up/downregulation of different miRNAs in cancer cells. These pathways and key enzymes, such as Drosha, exportin 5, DICER and Argonaute 2 (AGO2), are briefly described in Figure 1 (3, 15, 17–19). Studies on miR-27 have indicated that the miR-27 family is a large family and that miR-27a is one of the products of the gene loci. There are several isomiRNAs of miR-27 formed in the mature progress derived from alternative and imprecise Drosha and DICER cleavage that have different expression levels and functions. In liver cancer, miR-27a-3p has a high expression level of isomiR in normal cells, while isomiR expression is decreased significantly in tumor cells. However, this differentiation is not observed at the homologous miR-27b-3p site, indicating that isomiR-27a may have a more complicated background in tumor cells (20). As a 22-nt long mimic, it is easy for miR-27a to cooperate with other miRs. It has been revealed that miR-27 operates together with miR-23 and miR-24 in a cooperative cluster since these miRNA gene clusters are located on chromosome 19(–) (21). Therefore, some studies have focused on the function and clinical use of the miR-23a-27a-24-2 cluster instead of miR-27a, which requires further discussion. In addition, it has been proven that herpesvirus saimiri expresses several non-coding RNAs that significantly reduce the level of miR-27 in a host cell, indicating an interruption of miRNA biogenesis (22). In gastrointestinal tumors, with the variable expression level of different key enzymes, miR-27a has been discussed in specific kinds of tumors, while miR-27a has shown different expression features in gastrointestinal tumors. In esophageal tumors, DROSHA/DGCR8 is upregulated, corresponding with the upregulation of miR-27a in tumor cells (23). In gastric cancer, DROSHA and DICER are upregulated, indicating a high level of miR-27a in the cytoplasm (24). It was also reported that DGCR8 and DICER were upregulated in colorectal carcinoma (25, 26). These studies indicate that miR-27a is highly expressed in gastrointestinal tumors. However, in hepatic cancer cells, a researcher found the downregulation of DICER, which indicates a lower level of miR-27a (27). The molecular and cellular pathways controlling miRNA biogenesis remain to be further studied. In 2019, one novel study showed that melanoma differentiation-associated gene-7/interleukin-24 (mda-7/IL-24), a multifunctional cytokine, displayed broad-spectrum anticancer activity by regulating the function of DICER. This study provides a new anticancer method in which key miRNA enzymes, such as DICER, are targeted to disrupt miRNA synthesis in cancer cells (28).
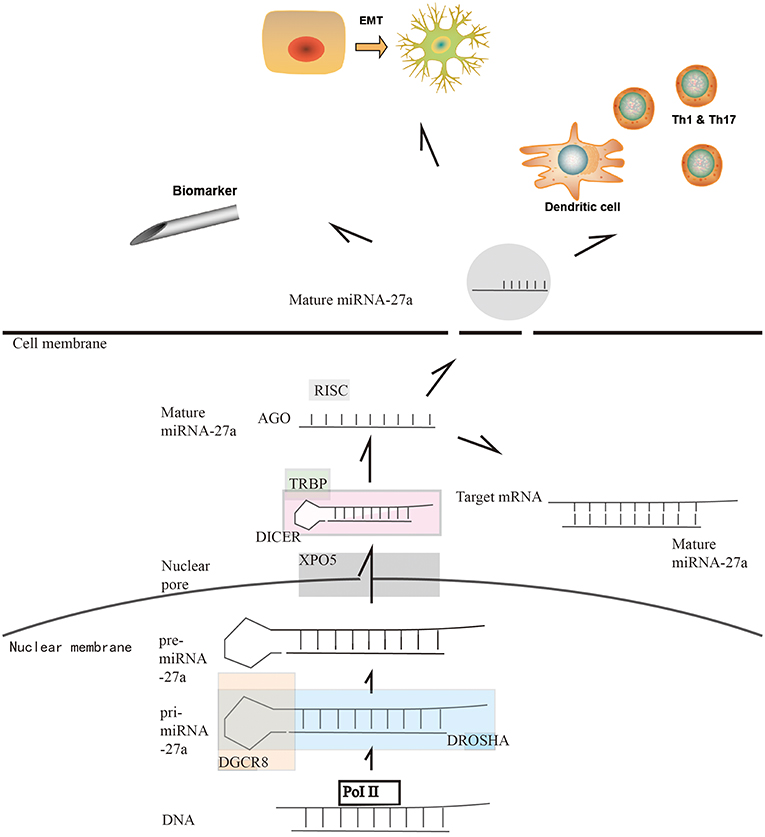
Figure 1. Overview of microRNA-27a biogenesis, highlighting key enzymes in miRNA biogenesis. miRNAs are produced in a tightly regulated pathway which is conserved across species. As a canonical biogenesis progress, the biogenesis of miR-27a begins with their transcription by RNA polymerase II (Pol II). Following RNA Pol II-mediated transcription, the first of two enzymatic cleavages produce pre-miRNAs. Drosha, a type III RNase, along with the cofactor DGCR8, binds to the primary miRNA (pri-miRNA) transcript. Two RNase domains that are present in Drosha mediate the cleavage of the 3′ and 5′ strands of pri-miRNAs to generate pre-miRNA (16). Next, the exportin 5–RAN•GTP complex (XPO 5) mediates the movement of pre-miRNAs from the nucleus into the cytosol. There, the RNase III Dicer combinding with TAR RNA binding protein (TRBP) binds to the pre-miRNAs and cleaves the terminal loop, producing a miRNA duplex. Then, incorporated into the RNA-induced silencing complex (RISC), miRNA duplex is processed by the argonaute (AGO) family of proteins to get the matured single-strand miRNA. For the function of miR-27a, on the one hand, the main function of the miRNA is that binding to selected mRNA to form a slienced mRNA duplex. On the other hand, miR-27a can form exosomes to release in to bloodstream. It is reported that miR-27a can regulate the immune system by affecting dentritic cells and macrophages. It is also reported that the exosomal miR-27a can regulate the EMT of cancer cells. Furthermore, the exosomal miR-27a in the bloodstream gives us new method of early diagnosis of cancers.
In recent years, the distribution of miRNAs in the serum or intracellular space, including exosomes, has attracted great attention from researchers as an important feature of miRNA biogenesis because miRNAs can be used as predictive or prognostic biomarkers. Regarding miR-27a, there have been several studies on the relationship between the serum level of miR-27a and patient prognosis. miR-27a has been proven to be a predictive or prognostic biomarker in gastrointestinal tumors in recent years since miR-27a can be secreted by exosomes from cells into serum circulation in gastric cancer. It has also been reported that circulating exosomal miR-27a can be used as a novel diagnostic and prognostic biomarker in colorectal cancer and hepatitis C virus-associated hepatocellular carcinoma (HCC) (29–33). In pancreatic cancer, although there was no specific finding on exosomal miR-27a, there was an increase in miR-23 exosomes, which can be used as a biomarker (14, 34, 35). Considering the miR-23-27-242 cluster, the miR-27a exosome might be a possible research direction in pancreatic adenocarcinoma. Further functions of exosomal miR-27a, such as the regulation of cancer-associated fibroblasts, will be reviewed in the following chapter.
Roles of miR-27a in Regulating Signaling Pathways
miR-27a in Regulating AKT Signaling Pathways
microRNA-27a is involved in mechanisms related to proliferation and growth signaling pathways (18). miR-27a promotes cancer cell proliferation by repressing the expression of prohibitin, which can block E2F to interrupt the AKT or tyrosine signaling pathways (10, 11, 36). Furthermore, it has been reported that the pleckstrin homology (PH) domain and leucine-rich repeat protein phosphatase 2 (PHLPP2) are new targets of miR-27a. The miR-27a could induce suppression of PHLPP2, leading to stimulation of the AKT/GSK3β pathway (37). FOXO1 is an important transcription factor downregulated by AKT directly, while adipose tissue-secreted miR-27a promotes liver cancer by downregulating FOXO1 (38). PI3K is a novel target of miR-27a. By activating the PI3K/AKT signaling pathway, the phosphorylated survival protein AKT is strongly expressed. The upregulation of miR-27a targets PI3K, initiating apoptosis (39). Bcl-2, a key target in the PI3K/AKT and JNK pathways, is a direct target of miR-27a, and overexpression of Bcl-2 attenuates the promotion of cell damage by miR-27a (40). In addition, down-regulation of miR-27a significantly reduced the expression of cyclin D1 transcriptional activity and up-regulated the expression of p21 downstream of GSK3β (11). This evidence indicates that miR-27a regulates proliferation and growth by interacting with the AKT pathway.
miR-27a Regulates the Wnt/β-catenin Signaling Pathway
Recent studies have shown that miR-27a can directly target secreted frizzled-related protein (SFRP), a regulator of tumor suppressor proteins and Wnt signaling pathway. miR-27a is overexpressed in cancer cell lines (MGC803, BT-20, MCF-7, T-47D, and MDA-MB-231). When miR-27a is downregulated, SFRP1 is upregulated, and β-catenin, Wnt, pβ-catenin, and p-Wnt are significantly downregulated at the same time. In contrast, when transfected with miR-27a mimics, the proliferation, migration, and invasion of gastric cancer cells are remarkably increased, accompanied by a decrease in SFRP1 protein, indicating the inhibition of SFRPs and continuous activation of the Wnt/β-catenin signaling pathway (16, 41). Up-regulation of obesity-related miR-27a can also promote HCC metastasis by inhibiting SFRP1 (42). In another study, the authors found that miR-27a negatively regulates the expression of SFRP1 mRNA. HCT-116 cells transfected with miR-27a mimics show increased proliferation and invasion of colon cancer cells, while the expression of pβ-catenin is remarkably downregulated (43). In addition to the SFRP family, RARalpha-mediated transcriptional inactivation of miR-27a activates the inhibition of GSR-3β by miR-27a, which leads to cancer differentiation via the Wnt/β-catenin pathway involved in GSK-3β (44). RXRalpha is a target gene of miR-27a-3p (45). Transmembrane protein 170B (TMEM170B) is also revealed as a novel functional target of miR-27a, which promotes cytoplasmic β-catenin phosphorylation, leading to inhibition of β-catenin stabilization (46). miR-27a upregulation and the activation of PPARgamma/β-catenin signaling were verified by recent research (47). Moreover, miR-27a promotes EMT through Wnt/β-catenin signaling (12, 48). Thus, the Wnt/β-catenin signaling pathway is an important target pathway for miR-27a.
miR-27a in Regulating the Ras/MEK/ERK Signaling Pathway
Another study found that two isoforms of mature miR-27a, miR-27a-5p, and miR-27-3p, have different expression levels in gastric cancer: miR-27-3p expression is significantly higher than that of miR-27a-5p. By inhibiting B cell translocation gene 2 (BTG2), miR-27-3p can upregulate the Ras/MEK/ERK pathway and c-myc levels, promoting cancer cell proliferation (49). Novel research has shown that miR-27a promotes ERK phosphorylation by downregulation of the ERK inhibitor sprouty2 (50). It has also been proven that miR-27a regulates the p38/mitogen-activated protein kinase (MAPK) signaling pathway (51). Furthermore, miR-27a directly silences the FBXW7 gene by binding to its 3′ untranslated region (UTR), thereby reducing the expression of FBXW7 in cancer cell. Silencing of FBXW7 in turn further increases the expression of KLF5 and miR-27a, leading to the dysfunction of c-myc (52, 53). Under the regulation of c-myc gene, the expression of mature miR-23a, miR-24-2 and miR-27a is promoted, thereby subsequently decreasing the expression of SPRY2 and activating p44/42 MAPK to promote cancer cell invasion (54). Taken together, these results suggest that miR-27a also has an effect on the MAPK or RAS/MEK/ERK pathway by regulating several genes.
miR-27a in Regulating the TGF-β Signaling Pathway
miR-27a promotes tumor proliferation and invasion by inhibiting TGF-β-induced cell cycle arrest. Overexpression of miR-27a can reduce SMAD2 and SMAD4 at mRNA and protein levels, which has important tumor suppressive effects in TGF-β signaling pathway (55, 56). miR-27a can also directly target the 3′-UTR of transforming growth factor β receptor I (TGF-βRI) and downregulate TGF-β signaling (57). By repressing PPARgamma, miR-27a activates TGF-β/Smad3 signaling and contributes to the changes in the expression of connective tissue growth factor (CTGF) (58). miR-27a acts as an oncogene by silencing MAP2K4, which is an important tumor suppressor inhibiting cell proliferation and migration via the JNK/p38 signaling pathway downstream of the TGF-β/TAK1 pathway (59).
We summarize four important signaling pathways that are affected by miR-27a directly or indirectly (Figure 2). Although there is overlap between the signaling pathways, we can conclude that miR-27a has extensive effects on the mainstream signaling pathways, which brings us more confidence in understanding the function of miR-27a in tumorigenesis and converting basic medical research into clinical use.
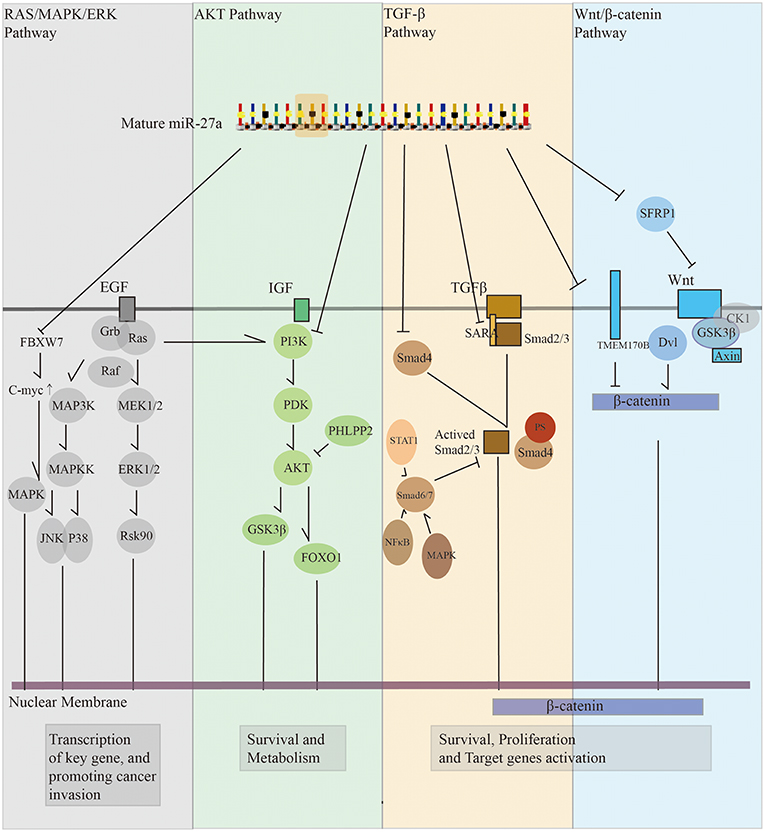
Figure 2. A brief conclusion of miR-27a in regulating cell signal pathways Panel gray, miR-27a can regulate Ras/MAPK/ERK pathway to active key gene such as c-myc. JNK and P38, which pay important role as a downstream of TGF-13 signal pathway, are also involved in Ras/MAPK cascade pathway. c-myc is also plays an important role in this progress. Panel green, miR-27a can regulate AKT pathway to keep the activation of survival signal of tumor cells. Several key enzymes in AKT pathway such as PI3K, GSK3J3, and FOXO1 are reported as a direct target of miR-27a. Panel yellow, miR-27a can decrease SMAD2 and SMAD4 mRNA and protein levels to regulate TGF-I3 signal pathway. Panel blue, by depressing TMEM170B and SFRP1, miR-27a leads to aberrant activation of Wnt/J3-catenin pathway to promote the proliferation and survival of cancer cells.
miR-27a in Promoting Tumorigenesis
miR-27a Has Different Functions in the EMT of Different Solid Tumors
EMT is an important aspect of metastasis that enables cells to migrate and populate secondary sites, and microRNAs play an important role in the EMT of tumors (60, 61). In solid tumors, such as breast cancer, gastrointestinal cancer, and ovarian cancer, transformed cells that undergo EMT lose epithelial features and acquire mesenchymal features for invasion and migration (62). EMT is performed by the so-called EMT-activated transcription factor (EMT-TF), mainly the SNAIL1, TWIST, and ZEB families (63). A recent study showed that EMT-TF can be dynamically degraded by the atypical ubiquitin E3 ligase complex Skp1-Pam-Fbxo45 (SPFFbxo45) via the ubiquitin-proteasome system (UPS). Fbxo45 recognizes EMT-TF by Zeb2 through its SPRY domain is a key step in this process, while miR-27a can directly down-regulate the expression of Fbxo45, preventing EMT-TF degradation and ensuring EMT (64). Several key signaling pathways that are highly regulated by miR-27a as mentioned before, such as the Wnt/β-catenin pathway and GSK3β, have been proven to participate in the regulation of EMT (43, 45, 48, 65). In a novel study, the mRNA expression level of miR-27a, which activates the Wnt/β-catenin signaling pathway through the inhibition of FOXO1, was significantly higher in ovarian cancer tissues and in in vitro ovarian cancer cell lines (12).
Moreover, another novel study found that AP-2β, an important transcription factor and a tumor suppressor, is inhibited by miR-27a in HCC. AP-2β modulates the levels of EMT markers through Slug and Snail. miR-27a reverses the tumor suppressive role of AP-2β by binding to the AP-2β 3′-UTR (66). Thus, a miR-27a/AP-2β/Slug/EMT regulatory axis may be a potential target for evaluating treatment and prognosis (66). miR-27a also shows heterogenic functions in different types of cells. In HCC, miR-27a-3p downregulation contributes to a higher level of Twist-1 and Bcl-2 expression, causing vasculogenic mimicry and metastasis by downregulating VE-cadherin expression, indicating the inhibition of EMT and early metastasis (67–69). miR-27a-3p may also have an antitumor function in the development of HCC by targeting dual specificity phosphatase 16 (DUSP16) to decrease the viability and migration of tumor cells (70). F-box and WD repeat domain containing 7 (FBXW7), which notably suppresses EMT and migratory activity, is a downstream target gene of miR-27a. miR-27a can inhibit FBXW7, leading to the dysregulation of downstream genes and the activation of EMT (71).
miR-27a Plays an Important Role in Regulating Cancer Stem Cells (CSCs) and the Tumor Microenvironment (TME)
In recent years, the functions and regulatory mechanisms of CSCs have attracted considerable attention. There is some evidence that CSCs are dynamic in many cancer types, while EMT-TFs, such as TWIST1 and ZEB1, play an important role in regulating CSC properties (72–74). The interconversion of CSCs and non-CSCs was also considered as a relatively common phenomenon (75). Recent studies have shown that, suggesting that the tumor endothelium may be derived from CSC (76). In breast cancer stem-like cells (BCSLCs), up-regulation of miR-27a and promotion of angiogenesis were observed after treatment of BCSLC with vascular endothelial growth factor (VEGF) (77). In a non-small cell lung cancer (NSCLC) stem-like cell line (H1650 CD133(+) CD34(–) cells), researchers have found that miR-27a has higher expression in H1650 CSCs and regulates cancer development in H1650 cells (78). In contrast, another study showed that the downregulation of miR-27a enhances the stem-like properties of small cell lung cancer (SCLC) cells in vitro (79).
The TME is described as a combination of the stroma, extracellular matrix elements, and immune cells that have an important role in cancer invasion, migration, and metastasis (80, 81). Carcinoma-associated fibroblasts (CAFs) are a highly enriched cell population in the TME that plays an important role in cancer invasion (82). A novel study showed that exosomal miR-27a in gastric cancer can induce the transformation of fibroblasts into CAFs. Moreover, CAFs overexpressing miR-27a could increase the malignant behavior of gastric cancer cells (29). Several studies have shown that miR-27a is overexpressed in liver stellate cells, influencing fat accumulation and cell proliferation, leading to hepatic fibrosis (83). It was also proven that miR-27a can induce liver fibrosis and be used as a diagnostic biomarker in advanced liver fibrosis and cirrhosis with hepatitis viruses B and C (84).
miR-27a also regulates the immune system to avoid the immune destruction of cancer cells. First, miR-27a can regulate T cell immunity. By accumulating TGF-β, miR-27a is upregulated and then activates the downstream NF-kappaB and MAPK pathways that influence the production of proinflammatory cytokines, leading to the decreased dendritic cell-mediated differentiation of Th1 and Th17 cells, which are important in the tumor immune response (13). Likewise, TGF-β upregulates the expression of the cluster in CD8(+) T cells (85). Second, miR-27a regulates macrophages to promote cancer progression. For example, the miR-23a/27a/24-2 cluster is significantly decreased in the tumor-associated macrophages (TAMs) of breast cancer patients while macrophages overexpressing the miR-23a/27a/24-2 cluster inhibit tumor growth (86). miR-27a also regulates the inflammatory response of macrophages by targeting IL-10 (87). In addition, the miR-23a/27a/24-2 microRNA cluster inhibits B cell development, which indicates a suppression in B cell immunity (88). Taken together, these and other studies suggest that miR-27 plays an important role in regulating CSCs, activating CAFs, and suppressing the immune system to avoid the immune destruction of cancer cells.
miR-27a and Therapy Resistance
Chemoresistance is frequently observed in most cancers, such as lung cancer and gastric cancer (89, 90). miR-27a has been reported to be associated with chemotherapy resistance in several cancers, while the mechanism of miR-27a in chemoresistance, despite several years of research, remains unclear (91). Multidrug resistance gene-1 (MDR1) is a well-studied gene in chemoresistance. The expression levels of MDR1 mRNA and P-glycoprotein (P-GP) and homeodomain-interacting protein kinase-2 (HIPK2) proteins are upregulated after upregulating the miR-27a level in cancer cells, while the sensitivity to paclitaxel is decreased in cancer cells transfected with miR-27a mimics (8, 92). It was also confirmed that the increase in FZD7 by miR-27a promotes the expression of MDR1/P-GP and β-catenin, leading to chemotherapy resistance (93). In gastric cancer, the downregulation of miR-27a concomitant with higher levels of MDR1, HIF1A, and HIPK2 genes indicates chemoresistance (94). In addition, researchers found increased expression of miR-27a in tumor tissues sampled from lung adenocarcinoma patients treated with cisplatin-based chemotherapy, which correlates with low RKIP expression, leading to resistance to cisplatin and a poor prognosis (95). miR-27a also weakens the effect of cisplatin by targeting RKIP in liver cancer cells (96).
One recent study found that a high level of microRNA-27a induces resistance to TNF-related apoptosis-inducing ligand (TRAIL), while the knockdown of microRNA-27a resensitizes colorectal CSCs to TRAIL-induced cell death (97). miR-27a also induces chemoresistance to tamoxifen in human breast cancer cell lines (98). A novel study found that cells show decreased expression of ERalpha and miR-27a. The overexpression of miR-27a increases the level of ERalpha, increasing the sensitivity of tamoxifen-resistant cancer cells to selective estrogen receptor modulator treatments and resensitizing the cells to tamoxifen (99).
In addition to targeting CDC-27, miR-27a and its downregulation also confer increased radioresistance in triple-negative breast cancer (TNBC) cells (100). As mentioned before, the miR-27a-induced transformation of cancer-associated fibroblasts will decrease drug transportation and efficacy by the TME (101).
miR-27a in Clinical Applications
miR-27a as a Biomarker
Since miR-27a is closely related to oncogenesis, many studies have been eager to translate it into clinical applications as a biomarker to help with diagnosis and treatment. miR-27a, specifically exosome miR-27a, has been used as a biomarker for diagnostic and prognostic applications in several solid tumors such as gastric cancer, colorectal cancer, and breast cancer (Table 1).
miR-27a as a Biomarker in the Diagnosis and Prognosis of Gastric Cancer
In gastric cancer, there are several studies showing that miR-27a can be a good candidate as a biomarker for diagnosis and prognosis (30, 103, 105, 110, 111). From 164 gastric cancer patients and 127 normal people, miR-27a was identified to have a markedly higher confidence interval (0.879) than those of carcinoembryonic antigen (CEA) (0.503) and carbohydrate antigen 19-9 (CA19-9) (0.600), indicating that miR-27a can serve as a biomarker for gastric cancer diagnosis (102). In a recent study, the miR-27a expression levels were found to be significantly higher in patients with gastric cancer compared to the validation plasma cohort (30). Moreover, the serum miR-27a level could be a predictive marker for lymph node metastasis in gastric cancer (103). miR-27a can also be a prognostic biomarker in gastric cancer, and the significantly upregulated expression of miR-27a highly correlates with a poor prognosis in gastric cancer patients (112). Exosomal miR-27a can be a predictor of the tumor response to treatment or chemotherapy resistance. In a novel study, the serum level of exosomal miR-27a was detected in 74 gastric cancer patients who received neoadjuvant chemotherapy by qRT-PCR, and the expression of miR-27a in the serum of gastric cancer patients decreased significantly after neoadjuvant chemotherapy. Gastric cancer patients who had a higher level of miR-27a tended to have tumor invasion and migration, indicating poor efficacy and prognosis after neoadjuvant chemotherapy (110). In another study, patients with upregulated miR-27a expression had significantly worse overall survival (OS) than patients with lower miR-27a expression (P = 0.024), while a higher miR-27a expression level indicated resistance to fluoropyrimidine-based chemotherapy (105).
miR-27a as a Biomarker in the Diagnosis and Prognosis of Colorectal Cancer
In colorectal cancer, recent studies have shown good potential for miR-27a as a biomarker for diagnosis and prognosis. Serum samples from 427 colon cancer patients and 276 healthy donors were included in a recent study. The results showed that miR-27a-3p has a sensitivity of 89% and a specificity of 81% in distinguishing colon cancer patients from healthy donors, indicating circulating microRNAs as a highly sensitive, non-invasive early detection method for colorectal cancer (106). Another study in 2018, which included 369 peripheral blood samples, also showed that exosomal miR-27a in the plasma may act as a non-invasive biomarker for early diagnosis. More importantly, this research provided the first evidence that patients with colorectal cancer with high circulating exosomal miR-27a expression had a poor prognosis, which made up for the previous research gap (32).
miR-27a as a Biomarker in Other Solid Cancers
Similar to gastric cancer and colorectal cancer, miR-27 shows wide potential for clinical applications in many solid cancers. miR-27a has been well studied in breast cancer as a biomarker for diagnosis and prognosis for breast cancer or TNBC (107, 113–115). The role of miRNAs in resistance to treatment for breast cancer is also one of the core issues discussed at present (116). miR-27a also shows potential in the diagnosis/prognosis of prostate cancer and the identification of metastasis after radical prostatectomy (108, 117). In HCC, compared to that of a single AFP marker, the combination of miR-125b/miR-27a/AFP had a higher sensitivity and specificity for the diagnosis of early-stage HCC (109). Several studies have suggested the potential use of serum miR-27a as a biomarker for HCC diagnosis; however, further studies are needed (33, 118). In conclusion, miR-27a has broad prospects in clinical applications as a biomarker for early diagnosis, prognosis, tumor stage, and the evaluation of chemotherapy efficacy or metastasis.
miR-27a as a Potential Target for Drug Design
Although many basic studies have revealed that miR-27a may be a promising drug design target, few drugs that target miR-27 have been developed and examined in clinical trials. In 2011, one study found that ethyl 2-((2,3-bis(nitrooxy)propyl) disulfanyl)benzoate, known as GT-094, a nitric oxide-releasing non-steroidal anti-inflammatory drug (NO-NSAID), decreased Sp1, Sp3, and Sp4 expression in colon cancer cells by downregulating miR-27a and inducing ZBTB10, revealing an anticancer function of this compound (119). Moreover, by downregulating miR-27a expression, curcumin and boswellic acid can induce chemoprevention in colon cancer, which provides new ideas for cancer prevention (120). Arsenic trioxide, a widely used anticancer drug in leukemia, was found to suppress cell growth and migration via the inhibition of miR-27a in breast cancer cells, providing a novel antitumor mechanism in miR-27a for the treatment of breast cancer (121). In addition, one novel study showed that liraglutide inhibited the proliferation and promoted the apoptosis of MCF-7 breast cancer cell lines through the downregulation of microRNA-27a expression (122). These studies provide a new method of drug design and a novel use of traditional drugs.
Discussion
With an in-depth understanding of cancer, several studies on the mechanism of tumorigenesis have been revealed. miRNAs, as important components that regulate the progression, invasion, and metastasis of cancer cells, should not be disregarded for their remarkable applications in the diagnosis and prognosis of cancer. We conclude that miR-27a plays an important role in oncogenesis, cell proliferation, tumor cell metabolism, and chemotherapy resistance. miR-27a also shows novel functions in regulating the tumor immune response, CAFs, and EMT. Previous studies on these basic medical mechanisms of miR-27 have been reviewed (123, 124). In this review, especially, we analyzed the recent application of miR-27a in several solid tumors, such as gastric cancer, breast cancer, and colorectal cancer, as a biomarker for diagnostic and prognostic applications. However, there is still much work to do in the future on the other solid tumors such as lung cancer and pancreatic cancer, which have insufficient evidence in clinical diagnostic and prognostic applications of miR-27a. We found that there's little research of miR-27a in clinical study on pancreatic cancer or lung cancer while there's a possibility of linkage cause miR-27a has a profound influence in signaling pathways of these cancers. Further research directions could be toward these areas. What's more, though increasing the effectiveness of diagnosis and/or prognosis, the shortcoming of miR-27a still exists. Limited by the popularity of instrumentation and experimental technology, we failed to find the current clinical trials based on miR-27a as a biomarker compared to traditional biomarkers such as carbohydrate antigen. MicroRNA also has a significant heterogeneity of expression level, leading to more obstacle in clinical using, for example, hypoxia can infect the expression level of microRNA in different parts of the tumor, leading to a different examine result, which may bring difficulties in diagnosis (18, 125, 126). In reality, biopsy samples tend to probe one specific area and do not provide more details into the dynamics of miRNA expression in solid tumors, which brings further problems in the application. Nevertheless, using microRNAs as a therapeutic or diagnostic method has progressed from bench to bedside, with some successful phase I and/or phase II trials in cancer (NCT01829971, NCT02369198). Furthermore, more economical and effective methods of microRNA capture give us precise identification of the miRNA targetsome such as miR-CLIP seq. The mature database of microRNA brings us convenience in researching the potential pathways. We are looking forward to more clinical trials of miR-27a put into practice. We are also expecting further research on drug design based on miR-27a since there are emerging molecular closing related to miR-27a expression come out.
Author Contributions
JZ was the first author of this review. ZC contributed equally to this review. All authors read and approved the final manuscript.
Funding
This study was supported by grants from the National Natural Science Foundation of China (No. 81772639 and No. 81802475); the Natural Science Foundation of Beijing (No. 7192157); the CAMS Innovation Fund for Medical Sciences (CIFMS) (No. 2016-I2M-1-001); the Nonprofit Central Research Institute Fund of Chinese Academy of Medical Sciences (No. 2018PT32014 and No. 2018PT32002); the China Postdoctoral Science Foundation (No. 198831); the CAMS Innovation Fund for Medical Students (No. 2017-1002-1-16); and the PUMC Youth Fund and the Fundamental Research Funds for the Central Universities (No. 2017320027).
Conflict of Interest Statement
The authors declare that the research was conducted in the absence of any commercial or financial relationships that could be construed as a potential conflict of interest.
Acknowledgments
I'd like to thank all those who have contributions in the course of my writing this review. Firstly, I'd like to show my sincere gratitude to my supervisor, YZ who has inspired me on my start of writing review, and rechecks the basic concepts of my review. Secondly, I'm appreciated that ZC offered me sufficient information and pointed out correct directions on time. At last, I'd like to thank those leaders, teachers of the department of general surgery, Peking Union Medical College Hospital, and my families who have encouraged me and given me confidence.
Abbreviations
RISC, RNA-induced silencing complex; EMT, epithelial-mesenchymal transition; AGO2, Argonaute 2; XPO5, exportin 5; PHLPP2, pleckstrin homology domain and leucine-rich repeat protein phosphatase 2; FOXO1, Forkhead box protein O1; SFRP, secreted frizzled-related protein; HCC, hepatocellular carcinoma; BTG2, B cell translocation gene 2; FBXW7, F-Box and WD Repeat Domain Containing 7 gene; TGF-β, tumor growth factor beta; TMEM170B, Transmembrane protein 170B; PPAR, peroxisome proliferator activated-receptors; DUSP16, dual specificity phosphatase 16; UPS, ubiquitin-proteasome system; BCSLCs, breast cancer stem-like cells; NSCLC, non-small cell lung cancer; CAFs, carcinoma-associated fibroblasts; FZD7, Frizzled Class Receptor 7; TNBC, triple-negative breast cancer.
References
1. Ma YC, Chen J. MIR27A (microRNA 27a). Atlas Genet Cytogenet Oncol Haematol. (2012) 16:813–6. doi: 10.4267/2042/48229
2. Yang C, Tabatabaei SN, Ruan X, Hardy P. The dual regulatory role of MiR-181a in breast cancer. Cell Physiol Biochem. (2017) 44:843–56. doi: 10.1159/000485351
3. Bartel DP. MicroRNAs: genomics, biogenesis, mechanism, and function. Cell. (2004) 116:281–97. doi: 10.1016/S0092-8674(04)00045-5
4. Spizzo R, Nicoloso MS, Croce CM, Calin GA. SnapShot: MicroRNAs in cancer. Cell. (2009) 137:586–586 e1. doi: 10.1016/j.cell.2009.04.040
5. Esteller M. Non-coding RNAs in human disease. Nat Rev Genet. (2011) 12:861–74. doi: 10.1038/nrg3074
6. Shin VY, Chu KM. MiRNA as potential biomarkers and therapeutic targets for gastric cancer. World J Gastroenterol. (2014) 20:10432–9. doi: 10.3748/wjg.v20.i30.10432
7. Mertens-Talcott SU, Chintharlapalli S, Li X, Safe S. The oncogenic microRNA-27a targets genes that regulate specificity protein transcription factors and the G2-M checkpoint in MDA-MB-231 breast cancer cells. Cancer Res. (2007) 67:11001–11. doi: 10.1158/0008-5472.CAN-07-2416
8. Li Z, Hu S, Wang J, Cai J, Xiao L, Yu L, et al. MiR-27a modulates MDR1/P-glycoprotein expression by targeting HIPK2 in human ovarian cancer cells. Gynecol Oncol. (2010) 119:125–30. doi: 10.1016/j.ygyno.2010.06.004
9. Fletcher CE, Dart DA, Sita-Lumsden A, Cheng H, Rennie PS, Bevan CL. Androgen-regulated processing of the oncomir miR-27a, which targets Prohibitin in prostate cancer. Hum Mol Genet. (2012) 21:3112–27. doi: 10.1093/hmg/dds139
10. Liu T, Tang H, Lang Y, Liu M, Li X. MicroRNA-27a functions as an oncogene in gastric adenocarcinoma by targeting prohibitin. Cancer Lett. (2009) 273:233–42. doi: 10.1016/j.canlet.2008.08.003
11. Zhao X, Yang L, Hu J. Down-regulation of miR-27a might inhibit proliferation and drug resistance of gastric cancer cells. J Exp Clin Cancer Res. (2011) 30:55. doi: 10.1186/1756-9966-30-55
12. Zhang LY, Chen Y, Jia J, Zhu X, He Y, Wu LM. MiR-27a promotes EMT in ovarian cancer through active Wnt/-catenin signalling by targeting FOXO1. Cancer Biomark. (2019) 24:31–42. doi: 10.3233/CBM-181229
13. Min S, Li L, Zhang M, Zhang Y, Liang X, Xie Y, et al. TGF-beta-associated miR-27a inhibits dendritic cell-mediated differentiation of Th1 and Th17 cells by TAB3, p38 MAPK, MAP2K4 and MAP2K7. Genes Immun. (2012) 13:621–31. doi: 10.1038/gene.2012.45
14. Sun Z, Shi K, Yang S, Liu J, Zhou Q, Wang G, et al. Effect of exosomal miRNA on cancer biology and clinical applications. Mol Cancer. (2018) 17:147. doi: 10.1186/s12943-018-0897-7
15. Rupaimoole R, Slack FJ. MicroRNA therapeutics: towards a new era for the management of cancer and other diseases. Nat Rev Drug Discov. (2017) 16:203–22. doi: 10.1038/nrd.2016.246
16. Wu F, Li J, Guo N, Wang XH, Liao YQ. MiRNA-27a promotes the proliferation and invasion of human gastric cancer MGC803 cells by targeting SFRP1 via Wnt/beta-catenin signaling pathway. Am J Cancer Res. (2017) 7:405–16.
17. Ha M, Kim VN. Regulation of microRNA biogenesis. Nat Rev Mol Cell Biol. (2014) 15:509–24. doi: 10.1038/nrm3838
18. Rupaimoole R, Calin GA, Lopez-Berestein G, Sood AK. miRNA deregulation in cancer cells and the tumor microenvironment. Cancer Discov. (2016) 6:235–46. doi: 10.1158/2159-8290.CD-15-0893
19. Lin S, Gregory RI. MicroRNA biogenesis pathways in cancer. Nat Rev Cancer. (2015) 15:321–33. doi: 10.1038/nrc3932
20. Ma M, Yin Z, Zhong H, Liang T, Guo L. Analysis of the expression, function, and evolution of miR-27 isoforms and their responses in metabolic processes. Genomics. (2018). doi: 10.1016/j.ygeno.2018.08.004. [Epub ahead of print].
21. Liang T, Yu J, Liu C, Guo L. An exploration of evolution, maturation, expression and function relationships in mir-23 approximately 27 approximately 24 cluster. PLoS ONE. (2014) 9:e106223. doi: 10.1371/journal.pone.0106223
22. Cazalla D, Yario T, Steitz JA. Down-regulation of a host microRNA by a Herpesvirus saimiri noncoding RNA. Science. (2010) 328:1563–6. doi: 10.1126/science.1187197
23. Sugito N, Ishiguro H, Kuwabara Y, Kimura M, Mitsui A, Kurehara H, et al. RNASEN regulates cell proliferation and affects survival in esophageal cancer patients. Clin Cancer Res. (2006) 12:7322–8. doi: 10.1158/1078-0432.CCR-06-0515
24. Tchernitsa O, Kasajima A, Schäfer R, Kuban RJ, Ungethüm U, Györffy B, et al. Systematic evaluation of the miRNA-ome and its downstream effects on mRNA expression identifies gastric cancer progression. J Pathol. (2010) 222:310–9. doi: 10.1002/path.2759
25. Kim B, Lee JH, Park JW, Kwon TK, Baek SK, Hwang I, et al. An essential microRNA maturing microprocessor complex component DGCR8 is up-regulated in colorectal carcinomas. Clin Exp Med. (2014) 14:331–6. doi: 10.1007/s10238-013-0243-8
26. Faber C, Horst D, Hlubek F, Kirchner T. Overexpression of Dicer predicts poor survival in colorectal cancer. Eur J Cancer. (2011) 47:1414–9. doi: 10.1016/j.ejca.2011.01.006
27. Wu JF, Shen W, Liu NZ, Zeng GL, Yang M, Zuo GQ, et al. Down-regulation of Dicer in hepatocellular carcinoma. Med Oncol. (2011) 28:804–9. doi: 10.1007/s12032-010-9520-5
28. Pradhan AK, Bhoopathi P, Talukdar S, Scheunemann D, Sarkar D, Cavenee WK, et al. MDA-7/IL-24 regulates the miRNA processing enzyme DICER through downregulation of MITF. Proc Natl Acad Sci USA. (2019) 116:5687–92. doi: 10.1073/pnas.1819869116
29. Wang J, Guan X, Zhang Y, Ge S, Zhang L, Li H, et al. Exosomal miR-27a derived from gastric cancer cells regulates the transformation of fibroblasts into cancer-associated fibroblasts. Cell Physiol Biochem. (2018) 49:869–83. doi: 10.1159/000493218
30. Park JL, Kim M, Song KS, Kim SY, Kim YS. Cell-free miR-27a, a potential diagnostic and prognostic biomarker for gastric cancer. Genomics Inform. (2015) 13:70–5. doi: 10.5808/GI.2015.13.3.70
31. Park JL, Kim M, Song KS, Kim SY, Kim YS. ERRATUM: acknowledgments correction. Cell-free miR-27a, a potential diagnostic and prognostic biomarker for gastric cancer. Genomics Inform. (2015) 13:156. doi: 10.5808/GI.2015.13.4.156
32. Liu X, Pan B, Sun L, Chen X, Zeng K, Hu X, et al. Circulating exosomal miR-27a and miR-130a act as novel diagnostic and prognostic biomarkers of colorectal cancer. Cancer Epidemiol Biomarkers Prev. (2018) 27:746–54. doi: 10.1158/1055-9965.EPI-18-0067
33. Rashad NM, El-Shal AS, Shalaby SM, Mohamed SY. Serum miRNA-27a and miRNA-18b as potential predictive biomarkers of hepatitis C virus-associated hepatocellular carcinoma. Mol Cell Biochem. (2018) 447:125–36. doi: 10.1007/s11010-018-3298-8
34. Grossi I, Salvi A, Baiocchi G, Portolani N, De Petro G. Functional role of microRNA-23b-3p in cancer biology. Microrna. (2018) 7:156–66. doi: 10.2174/2211536607666180629155025
35. Wang WS, Liu LX, Li GP, Chen Y, Li CY, Jin DY, et al. Combined serum CA19–9 and miR-27a-3p in peripheral blood mononuclear cells to diagnose pancreatic cancer. Cancer Prev Res. (2013) 6:331–8. doi: 10.1158/1940-6207.CAPR-12-0307
36. Wang S, Nath N, Adlam M, Chellappan S. Prohibitin, a potential tumor suppressor, interacts with RB and regulates E2F function. Oncogene. (1999) 18:3501–10. doi: 10.1038/sj.onc.1202684
37. Ding L, Zhang S, Xu M, Zhang R, Sui P, Yang Q. MicroRNA-27a contributes to the malignant behavior of gastric cancer cells by directly targeting PH domain and leucine-rich repeat protein phosphatase 2. J Exp Clin Cancer Res. (2017) 36:45. doi: 10.1186/s13046-017-0516-2
38. Sun B, Li J, Shao D, Pan Y, Chen Y, Li S, et al. Adipose tissue-secreted miR-27a promotes liver cancer by targeting FOXO1 in obese individuals. Onco Targets Ther. (2015) 8:735–44. doi: 10.2147/OTT.S80945
39. Liu G, Cao P, Chen H, Yuan W, Wang J, Tang X. MiR-27a regulates apoptosis in nucleus pulposus cells by targeting PI3K. PLoS ONE. (2013) 8:e75251. doi: 10.1371/journal.pone.0075251
40. Liu X, Meng J TanshinoneIIA ameliorates lipopolysaccharide-induced inflammatory response in bronchial epithelium cell line BEAS-2B by down-regulating miR-27a. Biomed Pharmacother. (2018) 104:158–64. doi: 10.1016/j.biopha.2018.05.021
41. Kong LY, Xue M, Zhang QC, Su CF. In vivo and in vitro effects of microRNA-27a on proliferation, migration and invasion of breast cancer cells through targeting of SFRP1 gene via Wnt/beta-catenin signaling pathway. Oncotarget. (2017) 8:15507–19. doi: 10.18632/oncotarget.14662
42. Chen Y, Zhang F, Zhao Y, He K, Zheng X, Pan Y, et al. Obesity-associated miR-27a upregulation promotes hepatocellular carcinoma metastasis through suppressing SFRP1. Onco Targets Ther. (2018) 11:3281–92. doi: 10.2147/OTT.S162978
43. Ba S, Xuan Y, Long ZW, Chen HY, Zheng SS. MicroRNA-27a promotes the proliferation and invasiveness of colon cancer cells by targeting SFRP1 through the Wnt/beta-catenin signaling pathway. Cell Physiol Biochem. (2017) 42:1920–33. doi: 10.1159/000479610
44. Chen S, Sun YY, Zhang ZX, Li YH, Xu ZM, Fu WN. Transcriptional suppression of microRNA-27a contributes to laryngeal cancer differentiation via GSK-3beta-involved Wnt/beta-catenin pathway. Oncotarget. (2017) 8:14708–18. doi: 10.18632/oncotarget.14769
45. Liang J, Tang J, Shi H, Li H, Zhen T, Duan J, et al. miR-27a-3p targeting RXRalpha promotes colorectal cancer progression by activating Wnt/beta-catenin pathway. Oncotarget. (2017) 8:82991–3008. doi: 10.18632/oncotarget.19635
46. Li M, Han Y, Zhou H, Li X, Lin C, Zhang E, et al. Transmembrane protein 170B is a novel breast tumorigenesis suppressor gene that inhibits the Wnt/beta-catenin pathway. Cell Death Dis. (2018) 9:91. doi: 10.1038/s41419-017-0128-y
47. Zhou Z, Wan J, Hou X, Geng J, Li X, Bai X. MicroRNA-27a promotes podocyte injury via PPARgamma-mediated beta-catenin activation in diabetic nephropathy. Cell Death Dis. (2017) 8:e2658. doi: 10.1038/cddis.2017.74
48. Qiao B, He BX, Cai JH, Tao Q, King-Yin Lam A. MicroRNA-27a-3p modulates the wnt/beta-catenin signaling pathway to promote epithelial-mesenchymal transition in oral squamous carcinoma stem cells by targeting SFRP1. Sci Rep. (2017) 7:44688. doi: 10.1038/srep44688
49. Zhou L, Liang X, Zhang L, Yang L, Nagao N, Wu H, et al. MiR-27a-3p functions as an oncogene in gastric cancer by targeting BTG2. Oncotarget. (2016) 7:51943–54. doi: 10.18632/oncotarget.10460
50. Saha B, Bruneau JC, Kodys K, Szabo G. Alcohol-induced miR-27a regulates differentiation and M2 macrophage polarization of normal human monocytes. J Immunol. (2015) 194:3079–87. doi: 10.4049/jimmunol.1402190
51. Cao Z, Chen L. Inhibition of miR-27a suppresses the inflammatory response via the p38/MAPK pathway in intervertebral disc cells. Exp Ther Med. (2017) 14:4572–8. doi: 10.3892/etm.2017.5053
52. King B, Trimarchi T, Reavie L, Xu L, Mullenders J, Ntziachristos P, et al. The ubiquitin ligase FBXW7 modulates leukemia-initiating cell activity by regulating MYC stability. Cell. (2013) 153:1552–66. doi: 10.1016/j.cell.2013.05.041
53. Liu Z, Liu X, Liu S, Cao Q. Cholesterol promotes the migration and invasion of renal carcinoma cells by regulating the KLF5/miR-27a/FBXW7 pathway. Biochem Biophys Res Commun. (2018) 502:69–75. doi: 10.1016/j.bbrc.2018.05.122
54. Li X, Liu X, Xu W, Zhou P, Gao P, Jiang S, et al. c-MYC-regulated miR-23a/24–2/27a cluster promotes mammary carcinoma cell invasion and hepatic metastasis by targeting Sprouty2. J Biol Chem. (2013) 288:18121–33. doi: 10.1074/jbc.M113.478560
55. Chae DK, Ban E, Yoo YS, Kim EE, Baik JH, Song EJ. MIR-27a regulates the TGF-beta signaling pathway by targeting SMAD2 and SMAD4 in lung cancer. Mol Carcinog. (2017) 56:1992–8. doi: 10.1002/mc.22655
56. Xu Q, Tong JL, Zhang CP, Xiao Q, Lin XL, Xiao XY. miR-27a induced by colon cancer cells in HLECs promotes lymphangiogenesis by targeting SMAD4. PLoS ONE. (2017) 12:e0186718. doi: 10.1371/journal.pone.0186718
57. Fang F, Huang B, Sun S, Xiao M, Guo J, Yi X, et al. miR-27a inhibits cervical adenocarcinoma progression by downregulating the TGF-betaRI signaling pathway. Cell Death Dis. (2018) 9:395. doi: 10.1038/s41419-018-0431-2
58. Hou X, Tian J, Geng J, Li X, Tang X, Zhang J, et al. MicroRNA-27a promotes renal tubulointerstitial fibrosis via suppressing PPARgamma pathway in diabetic nephropathy. Oncotarget. (2016) 7:47760–76. doi: 10.18632/oncotarget.10283
59. Pan W, Wang H, Jianwei R, Ye Z. MicroRNA-27a promotes proliferation, migration and invasion by targeting MAP2K4 in human osteosarcoma cells. Cell Physiol Biochem. (2014) 33:402–12. doi: 10.1159/000356679
60. Exposito-Villen A, E Aránega A, Franco D Functional role of non-coding rnas during epithelial-to-mesenchymal transition. Noncoding RNA. (2018) 4:E14. doi: 10.3390/ncrna4020014
61. Gulei D, Magdo L, Jurj A, Raduly L, Cojocneanu-Petric R, Moldovan A, et al. The silent healer: miR-205–5p up-regulation inhibits epithelial to mesenchymal transition in colon cancer cells by indirectly up-regulating E-cadherin expression. Cell Death Dis. (2018) 9:66. doi: 10.1038/s41419-017-0102-8
62. Braicu C, Gulei D, Cojocneanu R, Raduly L, Jurj A, Knutsen E, et al. miR-181a/b therapy in lung cancer: reality or myth? Mol Oncol. (2019) 13:9–25. doi: 10.1002/1878-0261.12420
63. Brabletz T, Kalluri R, Nieto MA, Weinberg RA. EMT in cancer. Nat Rev Cancer. (2018) 18:128–34. doi: 10.1038/nrc.2017.118
64. Xu M, Zhu C, Zhao X, Chen C, Zhang H, Yuan H, et al. Atypical ubiquitin E3 ligase complex Skp1-Pam-Fbxo45 controls the core epithelial-to-mesenchymal transition-inducing transcription factors. Oncotarget. (2015) 6:979–94. doi: 10.18632/oncotarget.2825
65. McCubrey JA, Fitzgerald TL, Yang LV, Lertpiriyapong K, Steelman LS, Abrams SL, et al. Roles of GSK-3 and microRNAs on epithelial mesenchymal transition and cancer stem cells. Oncotarget. (2017) 8:14221–50. doi: 10.18632/oncotarget.13991
66. Yang L, Qiu J, Xiao Y, Hu X, Liu Q, Chen L, et al. AP-2beta inhibits hepatocellular carcinoma invasion and metastasis through Slug and Snail to suppress epithelial-mesenchymal transition. Theranostics. (2018) 8:3707–21. doi: 10.7150/thno.25166
67. Zhao N, Sun BC, Zhao XL, Wang Y, Meng J, Che N, et al. Role of Bcl-2 and its associated miRNAs in vasculogenic mimicry of hepatocellular carcinoma. Int J Clin Exp Pathol. (2015) 8:15759–68.
68. Zhao N, Sun BC, Zhao XL, Wang Y, Sun HZ, Dong XY, et al. Changes in microRNAs associated with Twist-1 and Bcl-2 overexpression identify signaling pathways. Exp Mol Pathol. (2015) 99:524–32. doi: 10.1016/j.yexmp.2015.08.018
69. Zhao N, Sun H, Sun B, Zhu D, Zhao X, Wang Y, et al. miR-27a-3p suppresses tumor metastasis and VM by down-regulating VE-cadherin expression and inhibiting EMT: an essential role for Twist-1 in HCC. Sci Rep. (2016) 6:23091. doi: 10.1038/srep23091
70. Li JM, Zhou J, Xu Z, Huang HJ, Chen MJ, Ji JS. MicroRNA-27a-3p inhibits cell viability and migration through down-regulating DUSP16 in hepatocellular carcinoma. J Cell Biochem. (2018) 119:5143–52. doi: 10.1002/jcb.26526
71. Jiang G, Shi W, Fang H, Zhang X. miR27a promotes human breast cancer cell migration by inducing EMT in a FBXW7dependent manner. Mol Med Rep. (2018) 18:5417–26. doi: 10.3892/mmr.2018.9587
72. Batlle E, Clevers H. Cancer stem cells revisited. Nat Med. (2017) 23:1124–34. doi: 10.1038/nm.4409
73. Chaffer CL, Marjanovic ND, Lee T, Bell G, Kleer CG, Reinhardt F, et al. Poised chromatin at the ZEB1 promoter enables breast cancer cell plasticity and enhances tumorigenicity. Cell. (2013) 154:61–74. doi: 10.1016/j.cell.2013.06.005
74. Beck B, Lapouge G, Rorive S, Drogat B, Desaedelaere K, Delafaille S, et al. Different levels of Twist1 regulate skin tumor initiation, stemness, and progression. Cell Stem Cell. (2015) 16:67–79. doi: 10.1016/j.stem.2014.12.002
75. Singh AK, Arya RK, Maheshwari S, Singh A, Meena S, Pandey P, et al. Tumor heterogeneity and cancer stem cell paradigm: updates in concept, controversies and clinical relevance. Int J Cancer. (2015) 136:1991–2000. doi: 10.1002/ijc.28804
76. Jayaraman A, Kumar P, Marin S, de Atauri P, Mateo F, M Thomson T, et al. Untargeted metabolomics reveals distinct metabolic reprogramming in endothelial cells co-cultured with CSC and non-CSC prostate cancer cell subpopulations. PLoS ONE. (2018) 13:e0192175. doi: 10.1371/journal.pone.0192175
77. Tang W, Yu F, Yao H, Cui X, Jiao Y, Lin L, et al. miR-27a regulates endothelial differentiation of breast cancer stem like cells. Oncogene. (2014) 33:2629–38. doi: 10.1038/onc.2013.214
78. Luo W, Zhang D, Ma S, Wang C, Zhang Q, Wang H, et al. miR-27a is highly expressed in H1650 cancer stem cells and regulates proliferation, migration, and invasion. J Cancer Res Ther. (2018) 14(Suppl.):S1004-11. doi: 10.4103/0973-1482.199450
79. Miao Y, Li J, Qiu X, Li Y, Wang Z, Luan Y. miR-27a regulates the self renewal of the H446 small cell lung cancer cell line in vitro. Oncol Rep. (2013) 29:161–8. doi: 10.3892/or.2012.2095
80. Quail DF, Joyce JA. Microenvironmental regulation of tumor progression and metastasis. Nat Med. (2013) 19:1423–37. doi: 10.1038/nm.3394
81. Wang M, Zhao J, Zhang L, Wei F, Lian Y, Wu Y, et al. Role of tumor microenvironment in tumorigenesis. J Cancer. (2017) 8:761–73. doi: 10.7150/jca.17648
82. Kalluri R, Zeisberg M. Fibroblasts in cancer. Nat Rev Cancer. (2006) 6:392. doi: 10.1038/nrc1877
83. Ji J, Zhang J, Huang G, Qian J, Wang X, Mei S. Over-expressed microRNA-27a and 27b influence fat accumulation and cell proliferation during rat hepatic stellate cell activation. FEBS Lett. (2009) 583:759–66. doi: 10.1016/j.febslet.2009.01.034
84. Zhang H, Yan XL, Guo XX, Shi MJ, Lu YY, Zhou QM, et al. MiR-27a as a predictor for the activation of hepatic stellate cells and hepatitis B virus-induced liver cirrhosis. Oncotarget. (2018) 9:1075–90. doi: 10.18632/oncotarget.23262
85. Chandran PA, Keller A, Weinmann L, Seida AA, Braun M, Andreev K, et al. The TGF-beta-inducible miR-23a cluster attenuates IFN-gamma levels and antigen-specific cytotoxicity in human CD8(+) T cells. J Leukoc Biol. (2014) 96:633–45. doi: 10.1189/jlb.3A0114-025R
86. Ma S, Liu M, Xu Z, Li Y, Guo H, Ge Y, et al. A double feedback loop mediated by microRNA-23a/27a/24–2 regulates M1 versus M2 macrophage polarization and thus regulates cancer progression. Oncotarget. (2016) 7:13502–19. doi: 10.18632/oncotarget.6284
87. Xie N, Cui H, Banerjee S, Tan Z, Salomao R, Fu M, et al. miR-27a regulates inflammatory response of macrophages by targeting IL-10. J Immunol. (2014) 193:327–34. doi: 10.4049/jimmunol.1400203
88. Kong KY, Owens KS, Rogers JH, Mullenix J, Velu CS, Grimes HL, et al. MIR-23A microRNA cluster inhibits B-cell development. Exp Hematol. (2010) 38:629–40 e1. doi: 10.1016/j.exphem.2010.04.004
89. Shanker M, Willcutts D, Roth JA, Ramesh R. Drug resistance in lung cancer. Lung Cancer (Auckl). (2010) 1:23–36. doi: 10.2147/LCTT.S6861
90. Jia Y, Li Z, Cheng X, Wu X, Pang F, Shi J, et al. Depletion of death-associated protein-3 induces chemoresistance in gastric cancer cells through the beta-catenin/LGR5/Bcl-2 axis. J Investig Med. (2019) 67:856–61. doi: 10.1136/jim-2018-000934
91. Gao P, Wei JM, Li PY, Zhang CJ, Jian WC, Zhang YH, et al. Screening of deoxyribozyme with high reversal efficiency against multidrug resistance in breast carcinoma cells. J Cell Mol Med. (2011) 15:2130–8. doi: 10.1111/j.1582-4934.2010.01240.x
92. Zhang H, Li M, Han Y, Hong L, Gong T, Sun L, et al. Down-regulation of miR-27a might reverse multidrug resistance of esophageal squamous cell carcinoma. Dig Dis Sci. (2010) 55:2545–51. doi: 10.1007/s10620-009-1051-6
93. Chen Z, Ma T, Huang C, Zhang L, Lv X, Xu T, et al. MiR-27a modulates the MDR1/P-glycoprotein expression by inhibiting FZD7/beta-catenin pathway in hepatocellular carcinoma cells. Cell Signal. (2013) 25:2693–701. doi: 10.1016/j.cellsig.2013.08.032
94. Danza K, Silvestris N, Simone G, Signorile M, Saragoni L, Brunetti O, et al. Role of miR-27a, miR-181a and miR-20b in gastric cancer hypoxia-induced chemoresistance. Cancer Biol Ther. (2016) 17:400–6. doi: 10.1080/15384047.2016.1139244
95. Li J, Wang Y, Song Y, Fu Z, Yu W. miR-27a regulates cisplatin resistance and metastasis by targeting RKIP in human lung adenocarcinoma cells. Mol Cancer. (2014) 13:193. doi: 10.1186/1476-4598-13-193
96. Li W, Yu ZX, Ma BF. The increase of miR-27a affects the role of cisplatin on proliferation and migration capacities of liver cancer cells. Eur Rev Med Pharmacol Sci. (2018) 22:5490–8. doi: 10.26355/eurrev_201809_15809
97. Zhang R, Xu J, Zhao J, Bai J. Knockdown of miR-27a sensitizes colorectal cancer stem cells to TRAIL by promoting the formation of Apaf-1-caspase-9 complex. Oncotarget. (2017) 8:45213–23. doi: 10.18632/oncotarget.16779
98. Ye P, Fang C, Zeng H, Shi Y, Pan Z, An N, et al. Differential microRNA expression profiles in tamoxifen-resistant human breast cancer cell lines induced by two methods. Oncol Lett. (2018) 15:3532–9. doi: 10.3892/ol.2018.7768
99. Ljepoja B, García-Roman J, Sommer AK, Wagner E, Roidl A. MiRNA-27a sensitizes breast cancer cells to treatment with Selective Estrogen Receptor Modulators. Breast. (2019) 43:31–8. doi: 10.1016/j.breast.2018.10.007
100. Ren YQ, Fu F, Han J. MiR-27a modulates radiosensitivity of triple-negative breast cancer (TNBC) cells by targeting CDC27. Med Sci Monit. (2015) 21:1297–303. doi: 10.12659/MSM.893974
101. Tanaka K, Miyata H, Sugimura K, Fukuda S, Kanemura T, Yamashita K, et al. miR-27 is associated with chemoresistance in esophageal cancer through transformation of normal fibroblasts to cancer-associated fibroblasts. Carcinogenesis. (2015) 36:894–903. doi: 10.1093/carcin/bgv067
102. Liu R, Zhang C, Hu Z, Li G, Wang C, Yang C, et al. A five-microRNA signature identified from genome-wide serum microRNA expression profiling serves as a fingerprint for gastric cancer diagnosis. Eur J Cancer. (2011) 47:784–91. doi: 10.1016/j.ejca.2010.10.025
103. Kim SY, Jeon TY, Choi CI, Kim DH, Kim DH, Kim GH, et al. Validation of circulating miRNA biomarkers for predicting lymph node metastasis in gastric cancer. J Mol Diagn. (2013) 15:661–9. doi: 10.1016/j.jmoldx.2013.04.004
104. Stenholm L, Stoehlmacher-Williams J, Al-Batran SE, Heussen N, Akin S, Pauligk C, et al. Prognostic role of microRNA polymorphisms in advanced gastric cancer: a translational study of the Arbeitsgemeinschaft Internistische Onkologie (AIO). Ann Oncol. (2013) 24:2581–8. doi: 10.1093/annonc/mdt330
105. Huang D, Wang H, Liu R, Li H, Ge S, Bai M, et al. miRNA27a is a biomarker for predicting chemosensitivity and prognosis in metastatic or recurrent gastric cancer. J Cell Biochem. (2014) 115:549–56. doi: 10.1002/jcb.24689
106. Vychytilova-Faltejskova P, Radova L, Sachlova M, Kosarova Z, Slaba K, Fabian P, et al. Serum-based microRNA signatures in early diagnosis and prognosis prediction of colon cancer. Carcinogenesis. (2016) 37:941–50. doi: 10.1093/carcin/bgw078
107. Gasparini P, Cascione L, Fassan M, Lovat F, Guler G, Balci S, et al. microRNA expression profiling identifies a four microRNA signature as a novel diagnostic and prognostic biomarker in triple negative breast cancers. Oncotarget. (2014) 5:1174–84. doi: 10.18632/oncotarget.1682
108. Nam RK, Wallis CJD, Amemiya Y, Benatar T, Seth A. Identification of a novel MicroRNA panel associated with metastasis following radical prostatectomy for prostate cancer. Anticancer Res. (2018) 38:5027–34. doi: 10.21873/anticanres.12821
109. Zuo D, Chen L, Liu X, Wang X, Xi Q, Luo Y, et al. Combination of miR-125b and miR-27a enhances sensitivity and specificity of AFP-based diagnosis of hepatocellular carcinoma. Tumour Biol. (2016) 37:6539–49. doi: 10.1007/s13277-015-4545-1
110. Xu C, Cheng H, Li N, Zhou N, Tang X. Relationship between microRNA-27a and efficacy of neoadjuvant chemotherapy in gastric cancer and mechanism in gastric cancer cell growth and metastasis. Biosci Rep. (2019) 39:BSR20181175. doi: 10.1042/BSR20181175
111. Li X, Luo F, Li Q, Xu M, Feng D, Zhang G, et al. Identification of new aberrantly expressed miRNAs in intestinal-type gastric cancer and its clinical significance. Oncol Rep. (2011) 26:1431–9. doi: 10.3892/or.2011.1437
112. Zheng Q, Chen C, Guan H, Kang W, Yu C. Prognostic role of microRNAs in human gastrointestinal cancer: a systematic review and meta-analysis. Oncotarget. (2017) 8:46611–23. doi: 10.18632/oncotarget.16679
113. Tang W, Zhu J, Su S, Wu W, Liu Q, Su F, et al. MiR-27 as a prognostic marker for breast cancer progression and patient survival. PLoS ONE. (2012) 7:e51702. doi: 10.1371/journal.pone.0051702
114. Wang B, Ma N, Wang Y. Association between the hsa-mir-27a variant and breast cancer risk: a meta-analysis. Asian Pac J Cancer Prev. (2012) 13:6207–10. doi: 10.7314/APJCP.2012.13.12.6207
115. Lü L, Mao X, Shi P, He B, Xu K, Zhang S, et al. MicroRNAs in the prognosis of triple-negative breast cancer: A systematic review and meta-analysis. Medicine. (2017) 96:e7085. doi: 10.1097/MD.0000000000007085
116. Teoh SL, Das S. The role of MicroRNAs in diagnosis, prognosis, metastasis and resistant cases in breast cancer. Curr Pharm Des. (2017) 23:1845–59. doi: 10.2174/1381612822666161027120043
117. Gao W, Hong Z, Huang H, Zhu A, Lin S, Cheng C, et al. miR-27a in serum acts as biomarker for prostate cancer detection and promotes cell proliferation by targeting Sprouty2. Oncol Lett. (2018) 16:5291–8. doi: 10.3892/ol.2018.9274
118. Tak H, Kang H, Ji E, Hong Y, Kim W, Lee EK. Potential use of TIA-1, MFF, microRNA-200a-3p, and microRNA-27 as a novel marker for hepatocellular carcinoma. Biochem Biophys Res Commun. (2018) 497:1117–22. doi: 10.1016/j.bbrc.2018.02.189
119. Pathi SS, Jutooru I, Chadalapaka G, Sreevalsan S, Anand S, Thatcher GR, et al. GT-094, a NO-NSAID, inhibits colon cancer cell growth by activation of a reactive oxygen species-microRNA-27a: ZBTB10-specificity protein pathway. Mol Cancer Res. (2011) 9:195–202. doi: 10.1158/1541-7786.MCR-10-0363
120. Toden S, Okugawa Y, Buhrmann C, Nattamai D, Anguiano E, Baldwin N, et al. Novel evidence for curcumin and boswellic acid-induced chemoprevention through regulation of miR-34a and miR-27a in colorectal cancer. Cancer Prev Res. (2015) 8:431–43. doi: 10.1158/1940-6207.CAPR-14-0354
121. Zhang S, Ma C, Pang H, Zeng F, Cheng L, Fang B, et al. Arsenic trioxide suppresses cell growth and migration via inhibition of miR-27a in breast cancer cells. Biochem Biophys Res Commun. (2016) 469:55–61. doi: 10.1016/j.bbrc.2015.11.071
122. Zhao W, Zhang X, Zhou Z, Sun B, Gu W, Liu J, et al. Liraglutide inhibits the proliferation and promotes the apoptosis of MCF-7 human breast cancer cells through downregulation of microRNA-27a expression. Mol Med Rep. (2018) 17:5202–12. doi: 10.3892/mmr.2018.8475
123. Ding L, Ni J, Yang F, Huang L, Deng H, Wu Y, et al. Promising therapeutic role of miR-27b in tumor. Tumour Biol. (2017) 39:1010428317691657. doi: 10.1177/1010428317691657
124. Li X, Xu M, Ding L, Tang J. MiR-27a: a novel biomarker and potential therapeutic target in tumors. J Cancer. (2019) 10:2836–48. doi: 10.7150/jca.31361
125. Rupaimoole R, Wu SY, Pradeep S, Ivan C, Pecot CV, Gharpure KM, et al. Hypoxia-mediated downregulation of miRNA biogenesis promotes tumour progression. Nat Commun. (2014) 5:5202. doi: 10.1038/ncomms6202
Keywords: microRNA, microRNA-27a, signaling pathways, biomarker, clinical application
Citation: Zhang J, Cao Z, Yang G, You L, Zhang T and Zhao Y (2019) MicroRNA-27a (miR-27a) in Solid Tumors: A Review Based on Mechanisms and Clinical Observations. Front. Oncol. 9:893. doi: 10.3389/fonc.2019.00893
Received: 11 July 2019; Accepted: 27 August 2019;
Published: 12 September 2019.
Edited by:
Ondrej Slaby, Brno University of Technology, CzechiaReviewed by:
Qi Zhao, Sun Yat-sen University Cancer Center (SYSUCC), ChinaJun Zhong, National Cancer Institute (NCI), United States
Copyright © 2019 Zhang, Cao, Yang, You, Zhang and Zhao. This is an open-access article distributed under the terms of the Creative Commons Attribution License (CC BY). The use, distribution or reproduction in other forums is permitted, provided the original author(s) and the copyright owner(s) are credited and that the original publication in this journal is cited, in accordance with accepted academic practice. No use, distribution or reproduction is permitted which does not comply with these terms.
*Correspondence: Jingcheng Zhang, MTg4MTEzNTEyNTBAMTYzLmNvbQ==; Taiping Zhang, dHBpbmd6aGFuZ0B5YWhvby5jb20=; Yupei Zhao, emhhbzgwMjhAMjYzLm5ldA==
†These authors have contributed equally to this work