- 1Cancer Care Centre, St. George Hospital, Sydney, NSW, Australia
- 2St. George and Sutherland Clinical School, Faculty of Medicine, University of New South Wales (UNSW) Sydney, Kensington, NSW, Australia
- 3Department of Urology, St. George Hospital, Sydney, NSW, Australia
- 4School of Basic Medical Sciences, Zhengzhou University, Zhengzhou, China
Radiation is a mainstay of cancer therapy. Radioresistance is a significant challenge in the treatment of locally advanced, recurrent and metastatic cancers. The mechanisms of radioresistance are complicated and still not completely understood. Exosomes are 40–150 nm vesicles released by cancer cells that contain pathogenic components, such as proteins, mRNAs, DNA fragments, non-coding RNAs, and lipids. Exosomes play a critical role in cancer progression, including cell-cell communication, tumor-stromal interactions, activation of signaling pathways, and immunomodulation. Emerging data indicate that radiation-derived exosomes increase tumor burden, decrease survival, cause radiation-induced bystander effects and promote radioresistance. In addition, radiation can change the contents of exosomes, which allows exosomes to be used as a prognostic and predictive biomarker to monitor radiation response. Therefore, understanding the roles and mechanisms of exosomes in radiation response may shed light on how exosomes play a role in radioresistance and open a new way in radiotherapy and translational medicine. In this review, we discuss recent advances in radiation-induced exosome changes in components, focus on the roles of exosome in radiation-induced bystander effect in cancer and emphasize the importance of exosomes in cancer progression and radioresistance for developing novel therapy.
Introduction
Cancer is a major health burden. Radiotherapy (RT) is widely used in more than 50% of localized cancer patients (1), and is a critical and inseparable component of comprehensive cancer treatment and care (2). In addition, RT is often combined with surgery, chemotherapy, and more recently, immunotherapy (3). Despite progress made in radiation delivery approaches and precision medicine, tumor therapeutic resistance and recurrence frequently occur in clinical settings.
Radioresistance is a complicated biological process associated with abnormal DNA damage response (DDR), apoptosis, autophagy, gene mutations, cell cycle checkpoint, and deregulated signaling pathways (4). It leads to poor prognosis in cancer patients and represents a major clinical obstacle for RT, which ultimately leads to tumor relapse and metastasis (5). Tumor microenvironment is an important factor affecting tumor progression and therapeutic response (6, 7). It was reported radioresistance is highly associated with tumor microenvironment (8, 9).
Exosomes are important components and regulators of the tumor microenvironment. Exosomes are small extracellular vesicles (40–150 nm) secreted by different cells. Exosomes are representative of the original cells and can reflect a regulated sorting mechanism (10). These vesicles are composed of proteins (receptors, transcription factors, enzymes), nucleic acids [nuclear DNA, mitochondrial DNA (mtDNA), mRNA, microRNA (miRNA), long non-coding RNA (lncRNA), and circular RNA (circRNA)], and lipids (11–14). Exosome cargos such as nucleic acid and proteins from originating tumor cells communicate with neighbor cells or recipient cells, resulting in cancer progression and recurrence (15, 16).
Stressful conditions affect exosome secretion, composition, abundance, and potential binding on recipient cells. It was reported that different physiological and environmental conditions could alter the composition of exosomes shed from cells (17). Accumulating evidence indicates an increased release of exosomes after exposure to RT and the altered contents from donor cells were more oncogenic (18–21). Several recent studies have confirmed irradiated cells were involved in radiation-related communication between cells (20, 22, 23).
Despite the progress that has been made in exosome-mediated functions in in vitro models, there are still many challenges to be faced in exosomes and radiation oncology research. Here, we review recent advances in the radiation-induced exosome changes, discuss the roles of exosome in radiation-induced bystander effect (RIBE) in cancer and emphasize the importance of exosomes in cancer radioresistance and progression for the development of novel therapeutic strategies.
Radiation-Induced Exosome Changes in Cancer Radiotherapy
Cellular stress affects the composition and abundance of exosomes, as well as their potential impact on the recipient cells. Exosomes are a major environmental factor for cellular stress, and radiation can enhance the release of exosomes and affect exosome-based intercellular communication, which has been observed in various types of normal and tumor cell lines (18, 19, 24).
An increased level of exosomal CD276 was observed in the irradiated and senescent 22RV1 prostate cancer cell line, suggesting that this marker may provide a non-invasive way to monitor the efficacy of RT for prostate cancer patients (25). It was reported that circulating Hsp72 level was increased after radiation exposure in prostate cancer mouse xenografts and clinical sample; and that the exosomes containing Hsp72 are a possible contributor, leading to pro-inflammatory cytokine production and immune modulation (26). Khan et al. found that the level of exosomal survivin was increased after proton irradiation in HeLa cells and the rate of exosome secretion was not influenced (27). These findings suggest exosomal survivin may be associated with cancer recurrence after RT and could be a potential therapeutic target for preventing cervical cancer progression.
Radiation also alters the molecular composition within the exosomes. In one study, it was found radiation increased levels of exosomal connective tissue growth factor (CTGF) and Insulin Like Growth Factor Binding Protein 2 (IGFBP2) proteins, both of which are important in cell migration. By transferring CTGF mRNA, exosomes from irradiated cells were found to upregulate the migration-related signaling molecules including neurotrophic tyrosine kinase receptor type 1, focal adhesion kinase, Paxillin, and proto-oncogene tyrosine-protein kinase Src in recipient cells (18). In another study, exosomes released from the irradiated head and neck squamous carcinoma cell (HNSCC) FaDu cells demonstrated a distinctive protein expression profile compared to those from non-irradiated cells (20). Interestingly, most of the proteins that are specifically overexpressed in exosomes are those involved in transcription, translocation, and cell division, indicating that exosomal cargo is reflective of radiation-induced changes in cells (20). Similarly, exosomes derived from irradiated HNSCC BHY cells were found to be associated with not only immunity but also cell adhesion and motility, the underlying molecular mechanism being enhanced AKT-signaling triggered by the exosomal proteins (28). Using a shotgun liquid chromatography–tandem mass spectrometry (LC-MS/MS) approach, Abramowicz et al. found 472 exosomal proteins that are significantly affected by ionizing radiation (IR) in HNSCC UM-SCC6 cells and identified their role in mediating the cellular response to IR (29). Zhao et al. recently identified 63 upregulated and 48 downregulated circular RNAs from the exosomes of radioresistant glioma cells compared with those in control cells. Using qRT-PCR, they found circATP8B4 from radioresistant exosomes of glioma cells may be transferred to radiation-naïve cells and promoted cell radioresistance by acting as a microRNA(miR)-766 sponge (30).
The role of exosomes in radiation has garnered increasing attention in recent years. One study screened 752 exosome-derived miRNAs of locally advanced non-small cell lung cancer (NSCLC) patients and demonstrated that increased radiation dosage reduced miR29a-3p and miR150-5p expression (31), indicating that circulating exosomal miRNAs could help predict RT toxicity. In another study evaluating the outcome of HNSCC patients treated with chemoradiation therapy (CRT), exosomes from pooled plasma samples of patients who had complete or incomplete responses to CRT were screened. They identified a distinctive expression pattern of proteins between patients who had a complete response and who did not (32). In a recent Phase I clinical trial, 18 HNSCC patients receiving a combination of cetuximab, ipilimumab and RT were serially monitored for tumor cell-derived exosomes and T cell-derived exosomes. The results suggested tumor cell-derived exosomes and T cell-derived circulating exosomes instead of immune cells were suitable for monitoring of patients' responses to oncological therapy, supporting the potential role of exosomes as a non-invasive tumor and immune cell biomarkers in cancer (33).
The exosomes in RT research is stating to move from bench to bedside. However, the main limitation is the sample numbers tested from patiensts are still low and the preliminary results obtained need to be further validated in a large cohort of patients. Another challenge is the methods used for detecting exosome contents (biomarkers) such as proteomics and next generation sequencing need to be further optimimised to obtain the maximum number of interesting pontential biomarkers for verification. Although advances in exosome-based biomarkers such as proteins and miRNAs highlight an optimistic outlook for RT, the great progress has not been achieved due to limited reports in clinical research. Future direction in this area should move on the translational research and clinical trials.
In summary, the studies so far on radiation-induced changes in exosome composition have mainly been confined to their proteome contents using in vitro cancer cell line models (Summarized in Table 1), and there is a big gap in understanding how these changes are regulated, with the hope of translating into their functional importance. Future studies in this area should focus on (1) investigating the mechanisms of how the components of exosomes from donor cells after radiation are transferred to receipt cells; (2) establishing in vivo animal models for further studying the changes of exosomal components on the effect of RT; (3) investigating whether the changes of exosomal components could be used as biomarkers to evaluate the efficacy of RT; and (4) investigating whether the changes of exosomal components could be used as useful therapeutic targets to overcome radioresistance and improve the current RT.
The Bystander Effects by Radiation-Induced Exosomes
Radiation affects not only its direct targeted cells but also non-irradiated neighbors. This is evidenced by RIBE that cells that were not exposed to radiation exhibit effects as a result of intercellular communication. RIBE can also lead to biological changes in bystander cells and tissues, including chromosomal rearrangement, genomic instability, DNA damage, gene expression alteration, and apoptosis (34).
Numerous studies have demonstrated a large and complex interconnected web of mechanisms that contribute to the generation of RIBE, including reactive oxygen species (ROS), cytokines, free radicals, immune system, and epigenetic modulators (35–37). As previously reviewed more than a decade ago, it was believed that RIBE was mediated by both “a soluble secreted factor” and the cell-to-cell gap junction (38). It was not until recently that the important role of exosomes mediating RIBE had been recognized (39). Exosomes shed by irradiated cells are putatively involved in different aspects of the systemic response to IR, including the RIBE (40–42). Jella et al. showed that exosomes released from gamma-irradiated keratinocyte HaCaT cells induced increased cell death and ROS production in non-irradiated cells (24).
Mechanistically, IR elicits a set of dysregulated proteins and nucleic acids within the cell. These effectors, such as proteins, miRNAs and mtDNAs, are packaged into exosomes during their formation, which are then released to the extracellular environment. These cargos within the radiation-targeted cell-released exosomes subsequently get access to the adjacent cells as a result of exosome migration and internalization, and prompt RIBE in the non-targeted distant cells (21, 43–45).
These components of exosomes have different functions in RIBE, such as regulation of inflammation and modulation of DDR (46).
One report demonstrated that the production and release of exosomes following radiation-induced DNA damage were regulated by the p53 pathway (47). Tian et al. demonstrated that miR-21, a well-established DDR-related miRNA, played a mediating role in bystander DNA damage since it elevated ROS levels and increased the double-strand break (DSB) marker p53-binding protein 1 (53BP1) foci in non-irradiated cells (48). The role of miR-21 in RIBE was also validated by Yin et al. and Xu et al. using different experiment models (21, 49). Exosomal miR-1246 was also found to act as a messenger and contribute to DNA damage by directly repressing the DNA Ligase 4 (LIG4) gene (22). In combination, Yentrapalli et al. found that proteins such as afamin and serpine peptidase F1 along with miRNAs including miR-204-5p, miR-92a-3p, and miR-31-5p, play an important role in inducing and regulating RIBE (50). Another group also demonstrated that exosomal proteins and RNAs could mediate short- and long-term RIBE in human MCF-7 breast cancer cells (19, 51), implying that proteins and miRNAs may work synergistically during this process.
Moreover, exosomal miRNAs are able to travel remotely to influence cellular functions and regulate the niche-host reaction in targeted or non-targeted cells. It is worth mentioning that the role of a miRNA as either a positive or negative regulator in the RIBE varies from cell type to cell type. Interestingly, cells affected by RIBE and their progeny also showed the ability to secrete exosomes, and this cascade could potentially lead to a delayed RIBE-related inflammatory response (19). We have summarized exosome-mediated RIBE studies in cancer RT in Table 2. The mechanistic diagram demonstrating effects of radiation-inducible exosomal miRNAs and proteins in mediating RIBE is shown in Figure 1.
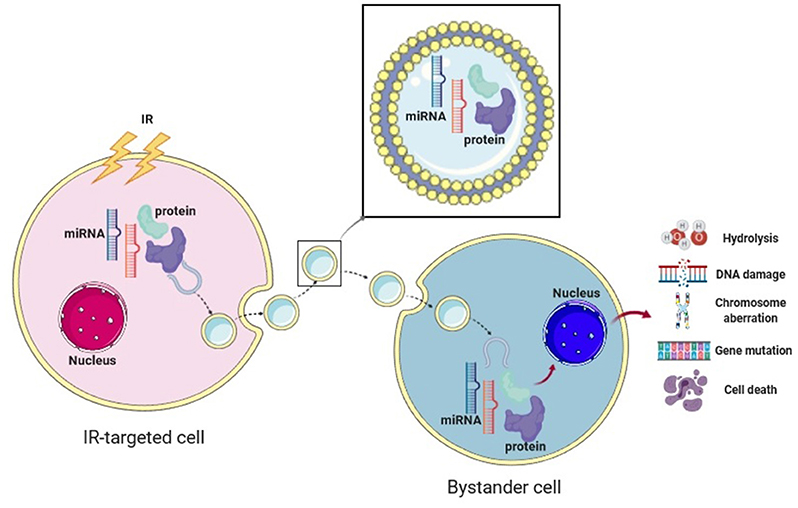
Figure 1. The bystander effect of exosomes in cancer radiotherapy. The mechanistic diagram displaying the effect of radiation-induced exosomal proteins and microRNAs in mediating cellular and molecular changes in RIBE. Created with BioRender.
In the therapeutic arena, on the one hand, RIBE is harmful to normal tissues, but on the other hand may be beneficial to induce non-irradiated cancer cell death during the treatment. RIBEs have critical implications in cancer RT. As direct effects of RT and RIBE are mechanistically distinctive and therefore it is important to develop different types of drugs to specifically target each mechanism, such as novel radiosensitisers to upregulate RIBE to kill more adjacent tumor cells; or adjuvant inhibitors to minimize the RIBE-induced systemic toxicity after RT.
Moreover, although in vitro studies bring promise for using exosomes and their cargo to regulate DDR and RIBE, it remains unclear whether these findings can be translated into the clinical setting. In future studies, the mechanisms of RIBE need to be deeply investigated, and in vivo animal models and clinical samples should be applied.
Exosomes in Cancer Progression After Radiotherapy
RT itself may increase the motility of surviving cancer cells, evidenced in glioblastoma (GBM), lung cancer and HNSCC, thus facilitating the spread of the tumor to local and distant sites (53–55). Radiation-induced exosomes have recently found to be an accomplice in promoting tumor cell motility and assisting in the pre-metastatic niche formation, the effectors again being the exosomal cargo incorporated by the recipient cells. Arscott et al. showed that radiation-derived exosomes enhanced U87MG GBM cell migration in co-culture (18). Using a wound healing assay, Mutschelknaus et al. found a pro-migratory role of exosomes in boosting the migratory capacity of BHY and FaDu HNSCC cells, in a dose-dependent and AKT-dependent manner (28). Apart from cell motility, angiogenesis also plays a crucial role in RT and tumor metastasis. Zheng et al. recently demonstrated that in lung cancer, the exosome-induced pro-angiogenesis effect was enhanced when the A549 and H1299 lung cancer cells were exposed to IR, and the miR23-mediated phosphatase and tensin homolog (PTEN) downregulation played an important role in this process (56). These findings indicate that radiation-induced exosomes function as a driver of cancer progression and metastasis during RT, and may represent a putative target to improve RT strategies.
The recent striking responses to immune-checkpoint inhibitors in the treatment of melanoma and solid tumors are paradigm-shifting and stirring up much research interest in the combination of immune-checkpoint inhibitors with RT. There is a close association between radiation response and immunity (57). RT plays either an immune-suppressive role (due to the sensitivity of leukocytes) or an immune-stimulatory role, evidenced by enhancing several antigen processing and presentation pathways (58, 59). Conventionally, the immune stimulation is believed to be T cell-mediated, but it is not until recently have we found that T cell-derived exosomes also plays a role in promoting esophageal cancer metastasis, via activation of epithelial-mesenchymal transition (EMT), β-catenin, and NF-κB/SNAIL pathways (60). This study provides a rationale for targeting exosomes during the synergy between RT and immunotherapy, but there are still quite a few unsolved riddles in this synergy, such as the sequencing, dose, and fractionation. Clearer mechanistic understandings of RT's immune-stimulatory role and further investigations on exosomes' functions in immune modulation are needed to expand this research field.
Exosomes in Radioresistance
Despite the recent advances in RT, many cancer patients, especially the locally advanced ones, failed radiation treatment (radioresistance), leading to a local recurrence or even distant metastasis. As previously reviewed (4), radioresistance can arise either from genetic or phenotypic changes within the tumor or as a result of the tumor stromal and microenvironment protecting the tumor against IR. Exosomes are one of the key components of the tumor microenvironment and increasing evidence suggests that they play a significant role in facilitating the development of radioresistance.
The key players in exosome-mediated radiosensitivity are found to be exosomal non-coding RNAs, proteins, and the crosstalk with survival and apoptotic pathways. Mutschelknaus et al. recently demonstrated that exosomes derived from irradiated HNSCC cells transmitted pro-survival signals to recipient cells via exosome cargos (52). In breast cancer, RNAs within exosomes were found to regulate radioresistance via an antiviral STAT1/NOTCH3 pathway (61). Radiation-induced exosomal miR-208a increased the proliferation and radioresistance via targeting p21 with activation of the AKT/mTOR pathway in lung cancer (62). In GBM, Mrowczynski et al. recently discovered that exosomes could enhance cell survival to radiation exposure by increasing levels of oncogenic miRNAs, mRNAs and pro-survival pathway proteins and at the same time decreasing levels of tumor-suppressive miRNAs and mRNAs (23). Recently, other exosomal non-coding RNAs were also found to be involved in the promotion of radioresistance, such as long non-coding RNA AHIF in glioblastoma (63), and circATP8B4 in glioma (30).
While exosomes were reported to play an important role in the promotion of cancer radioresistance, other reports were controversial. Wang et al. recently reported that autocrine secretions enhance the radioresistance of H460 NSCLC cell line in an exosome-independent manner and that these secretions mainly affect the DNA repair process (64). In another study, it was shown that exosomes derived form mesenchymal stem cells (MSCs), combined with RT, enhance RT-induced cell death in tumor and metastatic tumor foci in a melanoma mouse model. The finding provides a rationale to use MSCs-derived exosomes as an adjuvant to support and complement RT (65). These data indicate the role of exosomes in cancer radioresistance is complicated and could be affected by many factors such as tumor type, tumor microenvironment, experimental methods or different combinations of therapies.
Cancer is highly heterogeneous and includes a small subset of cells that possess the capacity of self-renewal and differentiation, referred to as cancer stem cells (CSCs) (66). CSCs are inherently more resistant to radiation than ordinary cancer cells, and more likely to survive after being exposed to RT. On one hand, these surviving CSCs may release exosomes to transfer resistant or refractory phenotypes to recipient cells, limiting the treatment efficacy. It was found that lncRNA H19 in exosomes derived from CSCs induced angiogenesis in hepatocellular carcinoma (67). Exosomes released from prostate and breast CSCs were also capable to induce autophagy (68), which has been shown to modulate sensitivity of cancer to RT (69). On the other, CSCs can also be eliminated via being reprogrammed into non-tumorigenic cells, using exosomes derived from adipose-derived stem cells (70). It not only re-justifies that the CSCs have to be eradicated during cancer therapy, but also preludes the usage of exosomes with modified surface or cargos to target CSCs.
In summary, all the data indicate that radiation-derived exosomes play important roles in cancer radioresistance through re-programmed cargos and intercellular communication. mRNAs, non-coding RNAs and signaling pathway proteins are closely related in exosome-associated radioresistance. CSC-associated exosomes are also a potential player in radioresistance and should be deeply investigated in the future study. The potential mechanisms of exosomes in radioresistance are shown in Figure 2.
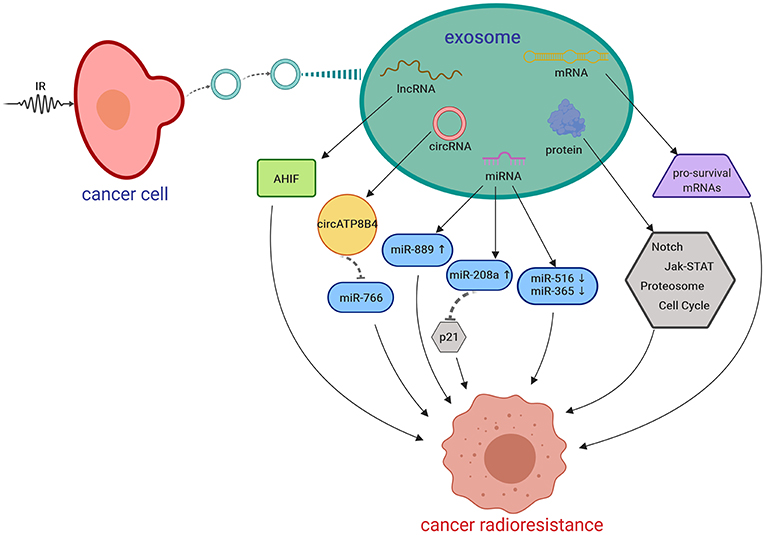
Figure 2. Putative mechanisms of IR-induced exosomes in promoting cancer radioresistance. AHIF, a natural antisense transcript; circRNA, circular RNA; IR, irradiation; lncRNA, long non-coding RNA; miRNA, microRNA; mRNA, messenger RNA. Created with BioRender.
Conclusions
Exploiting the biological functions of exosomes is intriguing, as they provide a snapshot of the entire tumor, transfer molecules intercellularly and can be used as a therapeutic target. In order to achieve these goals, isolation of exosomes should be standardized and optimized.
Exosomes in radiation research is a new and developing area. Radiation affects not only the production of exosomes but also the composition within, which makes exosomes an ideal prognostic and/or predictive biomarker to monitor the radiation response.
The radiation-altered exosomal cargos can be taken up by recipient cells, thus exerting various biological functions to impact radiosensitivity, and it is also worthwhile to note that this impact can be the results of synergistic or opposing effects of different exosomes for the sake of their heterogeneity. Due to the unique physical and biological features of exosomes, using functional exosomes to target CSCs and facilitate immunotherapy, is a promising avenue to be explored in RT, since exosomes are more stable, endogenous, and can be easily engineered or labeled.
There are still many challenges existing for exosome study. The future development of methodologies for exosome isolation and purification should be universal, precise, and suitable for clinical settings. Our experience is the combination of two or more approaches is a better choice for exosome isolation. In addition, the optimal sample source should be determined based on the cancer types and the preservation conditions of samples should be standardized. Furthermore, specific biomarkers of tumor exosomes should be screened and exploited for investigating the mechanisms of radioresistance.
Currently, knowledge of exosomes in cancer RT is in its infancy and mostly limited in in vitro studies. Further in vivo and clinical studies are warranted. Increasing knowledge of the biology of exosome and its cargo, along with standardized methods for exosome isolation and characterization will greatly contribute to a better understanding of mechanisms of exosome-mediated RT response and a good harnessing of exosomes as a therapeutic target, which might ultimately lead to the development of novel treatment strategies.
Author Contributions
JN, JB, DM, MK, PG, and YL reviewed the literature, developed the structures, wrote the review, and approved the final manuscript.
Funding
This work is mainly supported by the St. George Hospital Cancer Research Trust Fund and Prostate & Breast Cancer Foundation.
Conflict of Interest Statement
The authors declare that the research was conducted in the absence of any commercial or financial relationships that could be construed as a potential conflict of interest.
References
1. Barton MB, Jacob S, Shafiq J, Wong K, Thompson SR, Hanna TP, et al. Estimating the demand for radiotherapy from the evidence: a review of changes from 2003 to 2012. Radiother Oncol. (2014) 112:140–4. doi: 10.1016/j.radonc.2014.03.024
2. Atun R, Jaffray DA, Barton MB, Bray F, Baumann M, Vikram B, et al. Expanding global access to radiotherapy. Lancet Oncol. (2015) 16:1153–86. doi: 10.1016/S1470-2045(15)00222-3
3. Yaromina A, Krause M, Baumann M. Individualization of cancer treatment from radiotherapy perspective. Mol Oncol. (2012) 6:211–21. doi: 10.1016/j.molonc.2012.01.007
4. Ni J, Bucci J, Chang L, Malouf D, Graham P, Li Y. Targeting MicroRNAs in prostate cancer radiotherapy. Theranostics. (2017) 7:3243–59. doi: 10.7150/thno.19934
5. Rycaj K, Tang DG. Cancer stem cells and radioresistance. Int J Radiat Biol. (2014) 90:615–21. doi: 10.3109/09553002.2014.892227
6. Swartz MA, Iida N, Roberts EW, Sangaletti S, Wong MH, Yull FE, et al. Tumor microenvironment complexity: emerging roles in cancer therapy. Cancer Res. (2012) 72:2473–80. doi: 10.1158/0008-5472.CAN-12-0122
7. Son B, Lee S, Youn H, Kim E, Kim W, Youn B. The role of tumor microenvironment in therapeutic resistance. Oncotarget. (2016) 8:3933–45. doi: 10.18632/oncotarget.13907
8. Barker HE, Paget JTE, Khan AA, Harrington KJ. The tumour microenvironment after radiotherapy: mechanisms of resistance and recurrence. Nat Rev Cancer. (2015) 15:409–25. doi: 10.1038/nrc3958
9. Chan R, Sethi P, Jyoti A, McGarry R, Upreti M. Investigating the radioresistant properties of lung cancer stem cells in the context of the tumor microenvironment. Radiat Res. (2016) 185:169–81. doi: 10.1667/RR14285.1
10. Valadi H, Ekström K, Bossios A, Sjöstrand M, Lee JJ, Lötvall JO. Exosome-mediated transfer of mRNAs and microRNAs is a novel mechanism of genetic exchange between cells. Nat Cell Biol. (2007) 9:654–9. doi: 10.1038/ncb1596
11. Mathivanan S, Ji H, Simpson RJ. Exosomes: extracellular organelles important in intercellular communication. J Proteomics. (2010) 73:1907–20. doi: 10.1016/j.jprot.2010.06.006
12. D'Asti E, Garnier D, Lee TH, Montermini L, Meehan B, Rak J. Oncogenic extracellular vesicles in brain tumor progression. Front physiol. (2012) 3:294. doi: 10.3389/fphys.2012.00294
13. Fanale D, Taverna S, Russo A, Bazan V. Circular RNA in Exosomes. Adv Exp Med Biol. (2018) 1087:109–17. doi: 10.1007/978-981-13-1426-1_9
14. Sansone P, Savini C, Kurelac I, Chang Q, Amato LB, Strillacci A, et al. Packaging and transfer of mitochondrial DNA via exosomes regulate escape from dormancy in hormonal therapy-resistant breast cancer. Proc Natl Acad Sci USA. (2017) 114:E9066–75. doi: 10.1073/pnas.1704862114
15. Malla B, Zaugg K, Vassella E, Aebersold DM, Dal Pra A. Exosomes and exosomal microRNAs in prostate cancer radiation therapy. Int J Radiat Oncol Biol Phys. (2017) 98:982–95. doi: 10.1016/j.ijrobp.2017.03.031
16. Mathieu M, Martin-Jaular L, Lavieu G, Théry C. Specificities of secretion and uptake of exosomes and other extracellular vesicles for cell-to-cell communication. Nat Cell Biol. (2019) 21:9–17. doi: 10.1038/s41556-018-0250-9
17. Villarroya-Beltri C, Baixauli F, Gutiérrez-Vázquez C, Sánchez-Madrid F, Mittelbrunn M. Sorting it out: regulation of exosome loading. Semin Cancer Biol. (2014) 28:3–13. doi: 10.1016/j.semcancer.2014.04.009
18. Arscott WT, Tandle AT, Zhao S, Shabason JE, Gordon IK, Schlaff CD, et al. Ionizing radiation and glioblastoma exosomes: implications in tumor biology and cell migration. Transl Oncol. (2013) 6:638–48. doi: 10.1593/tlo.13640
19. Al-Mayah A, Bright S, Chapman K, Irons S, Luo P, Carter D, et al. The non-targeted effects of radiation are perpetuated by exosomes. Mutat Res. (2015) 772:38–45. doi: 10.1016/j.mrfmmm.2014.12.007
20. Jelonek K, Wojakowska A, Marczak L, Muer A, Tinhofer-Keilholz I, Lysek-Gladysinska M, et al. Ionizing radiation affects protein composition of exosomes secreted in vitro from head and neck squamous cell carcinoma. Acta Biochim Pol. (2015) 62:265–72. doi: 10.18388/abp.2015_970
21. Xu S, Wang J, Ding N, Hu W, Zhang X, Wang B, et al. Exosome-mediated microRNA transfer plays a role in radiation-induced bystander effect. RNA Biol. (2015) 12:1355–63. doi: 10.1080/15476286.2015.1100795
22. Mo L-J, Song M, Huang Q-H, Guan H, Liu X-D, Xie D-F, et al. Exosome-packaged miR-1246 contributes to bystander DNA damage by targeting LIG4. Br J Cancer. (2018) 119:492–502. doi: 10.1038/s41416-018-0192-9
23. Mrowczynski OD, Madhankumar AB, Sundstrom JM, Zhao Y, Kawasawa YI, Slagle-Webb B, et al. Exosomes impact survival to radiation exposure in cell line models of nervous system cancer. Oncotarget. (2018) 9:36083–101. doi: 10.18632/oncotarget.26300
24. Jella KK, Rani S, O'Driscoll L, McClean B, Byrne HJ, Lyng FM. Exosomes are involved in mediating radiation induced bystander signaling in human keratinocyte cells. Radiat Res. (2014) 181:138–45. doi: 10.1667/RR13337.1
25. Lehmann BD, Paine MS, Brooks AM, McCubrey JA, Renegar RH, Wang R, et al. Senescence-associated exosome release from human prostate cancer cells. Cancer Res. (2008) 68:7864–71. doi: 10.1158/0008-5472.CAN-07-6538
26. Hurwitz MD, Kaur P, Nagaraja GM, Bausero MA, Manola J, Asea A. Radiation therapy induces circulating serum Hsp72 in patients with prostate cancer. Radiother Oncol. (2010) 95:350–8. doi: 10.1016/j.radonc.2010.03.024
27. Khan S, Jutzy JM, Aspe JR, McGregor DW, Neidigh JW, Wall NR. Survivin is released from cancer cells via exosomes. Apoptosis. (2011) 16:1–12. doi: 10.1007/s10495-010-0534-4
28. Mutschelknaus L, Azimzadeh O, Heider T, Winkler K, Vetter M, Kell R, et al. Radiation alters the cargo of exosomes released from squamous head and neck cancer cells to promote migration of recipient cells. Sci Rep. (2017) 7:12423. doi: 10.1038/s41598-017-12403-6
29. Abramowicz A, Wojakowska A, Marczak L, Lysek-Gladysinska M, Smolarz M, Story MD, et al. Ionizing radiation affects the composition of the proteome of extracellular vesicles released by head-and-neck cancer cells in vitro. J Radiat Res. (2019) 60:289–97. doi: 10.1093/jrr/rrz001
30. Zhao M, Xu J, Zhong S, Liu Y, Xiao H, Geng L, et al. Expression profiles and potential functions of circular RNAs in extracellular vesicles isolated from radioresistant glioma cells. Oncol Rep. (2019) 41:1893–900. doi: 10.3892/or.2019.6972
31. Dinh T-KT, Fendler W, Chałubinska-Fendler J, Acharya SS, O'Leary C, Deraska PV, et al. Circulating miR-29a and miR-150 correlate with delivered dose during thoracic radiation therapy for non-small cell lung cancer. Radiat Oncol. (2016) 11:61. doi: 10.1186/s13014-016-0636-4
32. Rodrigues-Junior DM, Tan SS, de Souza Viana L, Carvalho AL, Lim SK, Iyer NG, et al. A preliminary investigation of circulating extracellular vesicles and biomarker discovery associated with treatment response in head and neck squamous cell carcinoma. BMC Cancer. (2019) 19:373. doi: 10.1186/s12885-019-5565-9
33. Theodoraki MN, Yerneni S, Gooding WE, Ohr J, Clump DA, Bauman JE, et al. Circulating exosomes measure responses to therapy in head and neck cancer patients treated with cetuximab, ipilimumab, and IMRT. Oncoimmunology. (2019) 8:1593805. doi: 10.1080/2162402X.2019.1593805
34. Morgan WF. Non-targeted and delayed effects of exposure to ionizing radiation: I. Radiation-induced genomic instability and bystander effects in vitro. Radiat Res. (2003) 159:567–80. doi: 10.1667/0033-7587(2003)159[0567:NADEOE]2.0.CO;2
35. Shao C, Folkard M, Prise KM. Role of TGF-beta1 and nitric oxide in the bystander response of irradiated glioma cells. Oncogene. (2008) 27:434–40. doi: 10.1038/sj.onc.1210653
36. He M, Zhao M, Shen B, Prise KM, Shao C. Radiation-induced intercellular signaling mediated by cytochrome-c via a p53-dependent pathway in hepatoma cells. Oncogene. (2010) 30:1947–55. doi: 10.1038/onc.2010.567
37. Hei TK, Zhou H, Ivanov VN, Hong M, Lieberman HB, Brenner DJ, et al. Mechanism of radiation-induced bystander effects: a unifying model. J Pharm Pharmacol. (2008) 60:943–50. doi: 10.1211/jpp.60.8.0001
38. Morgan WF, Sowa MB. Non-targeted bystander effects induced by ionizing radiation. Mutat Res. (2007) 616:159–64. doi: 10.1016/j.mrfmmm.2006.11.009
39. Jelonek K, Widlak P, Pietrowska M. The influence of ionizing radiation on exosome composition, secretion and intercellular communication. Protein Pept Lett. (2016) 23:656–63. doi: 10.2174/0929866523666160427105138
40. Albanese J, Dainiak N. Ionizing radiation alters Fas antigen ligand at the cell surface and on exfoliated plasma membrane-derived vesicles: implications for apoptosis and intercellular signaling. Radiat Res. (2000) 153:49–61. doi: 10.1667/0033-7587(2000)153[0049:IRAFAL]2.0.CO;2
41. Peinado H, Lavotshkin S, Lyden D. The secreted factors responsible for pre-metastatic niche formation: old sayings and new thoughts. Semin Cancer Biol. (2011) 21:139–46. doi: 10.1016/j.semcancer.2011.01.002
42. Record M, Subra C, Silvente-Poirot S, Poirot M. Exosomes as intercellular signalosomes and pharmacological effectors. Biochem Pharmacol. (2011) 81:1171–82. doi: 10.1016/j.bcp.2011.02.011
43. Le M, Fernandez-Palomo C, McNeill FE, Seymour CB, Rainbow AJ, Mothersill CE. Exosomes are released by bystander cells exposed to radiation-induced biophoton signals: reconciling the mechanisms mediating the bystander effect. PLoS ONE. (2017) 12:e0173685. doi: 10.1371/journal.pone.0173685
44. Ariyoshi K, Miura T, Kasai K, Fujishima Y, Nakata A, Yoshida M. Radiation-Induced bystander effect is mediated by mitochondrial DNA in exosome-like vesicles. Sci Rep. (2019) 9:9103. doi: 10.1038/s41598-019-45669-z
45. Szatmári T, Kis D, Bogdándi EN, Benedek A, Bright S, Bowler D, et al. Extracellular vesicles mediate radiation-induced systemic bystander signals in the bone marrow and spleen. Front Immunol. (2017) 8:347. doi: 10.3389/fimmu.2017.00347
46. Klammer H, Mladenov E, Li F, Iliakis G. Bystander effects as manifestation of intercellular communication of DNA damage and of the cellular oxidative status. Cancer Lett. (2015) 356:58–71. doi: 10.1016/j.canlet.2013.12.017
47. Yu X, Harris SL, Levine AJ. The regulation of exosome secretion: a novel function of the p53 protein. Cancer Res. (2006) 66:4795–801. doi: 10.1158/0008-5472.CAN-05-4579
48. Tian W, Yin X, Wang L, Wang J, Zhu W, Cao J, et al. The key role of miR-21-regulated SOD2 in the medium-mediated bystander responses in human fibroblasts induced by α-irradiated keratinocytes. Mutat Res. (2015) 780:77–85. doi: 10.1016/j.mrfmmm.2015.08.003
49. Yin X, Tian W, Wang L, Wang J, Zhang S, Cao J, et al. Radiation quality-dependence of bystander effect in unirradiated fibroblasts is associated with TGF-β1-Smad2 pathway and miR-21 in irradiated keratinocytes. Sci Rep. (2015) 5:11373. doi: 10.1038/srep11373
50. Yentrapalli R, Merl-Pham J, Azimzadeh O, Mutschelknaus L, Peters C, Hauck SM, et al. Quantitative changes in the protein and miRNA cargo of plasma exosome-like vesicles after exposure to ionizing radiation. Int J Radiat Biol. (2017) 93:569–80. doi: 10.1080/09553002.2017.1294772
51. Al-Mayah AH, Irons SL, Pink RC, Carter DR, Kadhim MA. Possible role of exosomes containing RNA in mediating nontargeted effect of ionizing radiation. Radiat Res. (2012) 177:539–45. doi: 10.1667/RR2868.1
52. Mutschelknaus L, Peters C, Winkler K, Yentrapalli R, Heider T, Atkinson MJ, et al. Exosomes derived from squamous head and neck cancer promote cell survival after ionizing radiation. PLoS ONE. (2016) 11:e0152213. doi: 10.1371/journal.pone.0152213
53. Vilalta M, Rafat M, Graves EE. Effects of radiation on metastasis and tumor cell migration. Cell Mol Life Sci. (2016) 73:2999–3007. doi: 10.1007/s00018-016-2210-5
54. Moncharmont C, Levy A, Guy JB, Falk AT, Guilbert M, Trone JC, et al. Radiation-enhanced cell migration/invasion process: a review. Crit Rev Oncol Hematol. (2014) 92:133–42. doi: 10.1016/j.critrevonc.2014.05.006
55. Edalat L, Stegen B, Klumpp L, Haehl E, Schilbach K, Lukowski R, et al. BK K+ channel blockade inhibits radiation-induced migration/brain infiltration of glioblastoma cells. Oncotarget. (2016) 7:14259–78. doi: 10.18632/oncotarget.7423
56. Zheng Y, Liu L, Chen C, Ming P, Huang Q, Li C, et al. The extracellular vesicles secreted by lung cancer cells in radiation therapy promote endothelial cell angiogenesis by transferring miR-23a. PeerJ. (2017) 5:e3627. doi: 10.7717/peerj.3627
57. Lumniczky K, Candéias SM, Gaipl US, Frey B. Editorial: radiation and the immune system: current knowledge and future perspectives. Front Immunol. (2017) 8:1933. doi: 10.3389/fimmu.2017.01933
58. Golden EB, Pellicciotta I, Demaria S, Barcellos-Hoff MH, Formenti SC. The convergence of radiation and immunogenic cell death signaling pathways. Front Oncol. (2012) 2:88. doi: 10.3389/fonc.2012.00088
59. Buchwald ZS, Efstathiou JA. Immunotherapy and radiation - a new combined treatment approach for bladder cancer? Bladder Cancer. (2015) 1:15–27. doi: 10.3233/BLC-150014
60. Min H, Sun X, Yang X, Zhu H, Liu J, Wang Y, et al. Exosomes derived from irradiated esophageal carcinoma-infiltrating T cells promote metastasis by inducing the epithelial-mesenchymal transition in esophageal cancer cells. Pathol Oncol Res. (2018) 24:11–8. doi: 10.1007/s12253-016-0185-z
61. Boelens MC, Wu TJ, Nabet BY, Xu B, Qiu Y, Yoon T, et al. Exosome transfer from stromal to breast cancer cells regulates therapy resistance pathways. Cell. (2014) 159:499–513. doi: 10.1016/j.cell.2014.09.051
62. Tang Y, Cui Y, Li Z, Jiao Z, Zhang Y, He Y, et al. Radiation-induced miR-208a increases the proliferation and radioresistance by targeting p21 in human lung cancer cells. J Exp Clin Cancer Res. (2016) 35:7. doi: 10.1186/s13046-016-0285-3
63. Dai X, Liao K, Zhuang Z, Chen B, Zhou Z, Zhou S, et al. AHIF promotes glioblastoma progression and radioresistance via exosomes. Int J Oncol. (2019) 54:261–70. doi: 10.3892/ijo.2018.4621
64. Wang S, Gao P, Li N, Chen P, Wang J, He N, et al. Autocrine secretions enhance radioresistance in an exosome-independent manner in NSCLC cells. Int J Oncol. (2019) 54:229–38. doi: 10.3892/ijo.2018.4620
65. de Araujo Farias V, O'Valle F, Serrano-Saenz S, Anderson P, Andrés E, López-Peñalver J, et al. Exosomes derived from mesenchymal stem cells enhance radiotherapy-induced cell death in tumor and metastatic tumor foci. Mol Cancer. (2018) 17:122. doi: 10.1186/s12943-018-0867-0
66. Ni J, Cozzi P, Hao J, Duan W, Graham P, Kearsley J, et al. Cancer stem cells in prostate cancer chemoresistance. Curr Cancer Drug Targets. (2014) 14:225–40. doi: 10.2174/1568009614666140328152459
67. Conigliaro A, Costa V, Lo Dico A, Saieva L, Buccheri S, Dieli F, et al. CD90+ liver cancer cells modulate endothelial cell phenotype through the release of exosomes containing H19 lncRNA. Mol Cancer. (2015) 14:155. doi: 10.1186/s12943-015-0426-x
68. Kumar D, Gupta D, Shankar S, Srivastava RK. Biomolecular characterization of exosomes released from cancer stem cells: possible implications for biomarker and treatment of cancer. Oncotarget. (2015) 6:3280–91. doi: 10.18632/oncotarget.2462
69. Li L, Liu WL, Su L, Lu ZC, He XS. The role of autophagy in cancer radiotherapy. Curr Mol Pharmacol. (2019). doi: 10.2174/1874467212666190809154518
Keywords: cancer, exosomes, radiotherapy, bystander, radioresistance
Citation: Ni J, Bucci J, Malouf D, Knox M, Graham P and Li Y (2019) Exosomes in Cancer Radioresistance. Front. Oncol. 9:869. doi: 10.3389/fonc.2019.00869
Received: 10 July 2019; Accepted: 21 August 2019;
Published: 06 September 2019.
Edited by:
Paul N. Span, Radboud University Nijmegen Medical Centre, NetherlandsReviewed by:
James William Jacobberger, Case Western Reserve University, United StatesEvagelia C. Laiakis, Georgetown University, United States
Copyright © 2019 Ni, Bucci, Malouf, Knox, Graham and Li. This is an open-access article distributed under the terms of the Creative Commons Attribution License (CC BY). The use, distribution or reproduction in other forums is permitted, provided the original author(s) and the copyright owner(s) are credited and that the original publication in this journal is cited, in accordance with accepted academic practice. No use, distribution or reproduction is permitted which does not comply with these terms.
*Correspondence: Yong Li, y.li@unsw.edu.au