- 1Department of Biochemistry and Genetics, La Trobe Institute for Molecular Science, La Trobe University, Melbourne, VIC, Australia
- 2Centre for Innate Immunity and Infectious Diseases, Hudson Institute of Medical Research, Melbourne, VIC, Australia
Heparanase is a β-D-endoglucuronidase that cleaves heparan sulfate, a complex glycosaminoglycan found ubiquitously throughout mammalian cells and tissues. Heparanase has been strongly associated with important pathological processes including inflammatory disease and tumor metastasis, through its ability to promote various cellular functions such as cell migration, invasion, adhesion, and cytokine release. A number of cell types express heparanase including leukocytes, cells of the vasculature as well as tumor cells. However, the relative contribution of heparanase from these different cell sources to these processes is poorly defined. It is now well-established that the immune system plays a critical role in shaping tumor progression. Intriguingly, leukocyte-derived heparanase has been shown to either assist or impede tumor progression, depending on the setting. This review covers our current knowledge of heparanase in immune regulation of tumor progression, as well as the potential applications and implications of exploiting or inhibiting heparanase in cancer therapy.
Introduction
Heparanase is the only mammalian enzyme that directly cleaves heparan sulfate side chains of heparan sulfate proteoglycans (HSPGs), key components of the extracellular matrix and basement membrane. The cleavage of heparan sulfate by heparanase regulates a number of fundamental cellular processes including cell migration (1, 2), cytokine production (3, 4), angiogenesis (5), and wound healing (6). Furthermore, heparanase has also been implicated in cell adhesion that is independent of its enzymatic activity (7, 8). The ability of heparanase to regulate these processes also makes it a key player in several pathological settings such as inflammatory disease and cancer. Heparanase contributes to various inflammatory diseases including delayed hypersensitivity, vascular injury, chronic colitis, Crohn's disease, sepsis, rheumatoid arthritis (9), atherosclerosis (10), and diabetes (11–13). Furthermore, heparanase is upregulated in response to pro-inflammatory cytokines, bacterial or viral infections, and modulates innate immune cell function. For example, in sepsis heparanase is upregulated by tumor necrosis factor-α (TNF-α) and induces shedding of the glycocalyx, thereby exposing the endothelial surface and adhesion molecules which facilitate neutrophil recruitment (14). Heparanase has also been well-characterized in cancer (15, 16), where the overexpression of heparanase often contributes to tumor progression (17, 18). The overexpression of heparanase has been detected in almost all cancer types, where it promotes metastasis (19–21), angiogenesis (19, 21, 22), and tumor proliferation (23). More recently, the role of leukocyte heparanase in tumor progression has been more closely examined, with the suggestion that it can be either pro- or anti-tumorigenic, depending on the setting.
Heparanase Expression by Leukocytes
The first documentation of heparanase expression in leukocytes was in T lymphocytes where the production of an endoglycosidase was observed in assisting their migration and penetration of the basement membrane and blood vessel entry (24). Subsequently, heparanase expression has been further characterized in T cells (25–30) as well as a number of other leukocytes including B cells (31), natural killer (NK) cells (2), monocytes (32), dendritic cells (DCs) (1, 32), macrophages (29, 30, 33–37), neutrophils (38–40), mast cells (41), and eosinophils (42). The expression of heparanase by leukocytes is inducible by various cell activatory stimuli (2, 43, 44) and has been shown to promote leukocyte migration (1, 45), cell rolling and adhesion (46, 47), the upregulation of pro-inflammatory cytokines (3), and activation of innate immune cells (34). Heparanase has also been associated with inflammatory diseases such as atherosclerosis (10) and diabetes (11–13). However, despite this progress, much remains to fully understand the role of heparanase in leukocytes and its contribution to disease. It is well-established that leukocytes are important regulators of tumor progression (48–51). An emerging area of significant clinical interest is at the intersection of heparanase, leukocytes, and cancer. We will now discuss how heparanase may regulate leukocyte function in the context of tumor progression and its relevance in cancer therapy.
Leukocyte Heparanase and Tumor Progression
Heparanase and Macrophage Activation and Infiltration Into Tumors
Tumor associated macrophages (TAMs) are often found within primary tumors and pre-metastatic sites, and their presence frequently contributes to tumor progression (52, 53). In heparanase knockout mice, macrophage infiltration into implanted Lewis lung carcinoma tumors was impaired, and tumors were smaller than in wild type animals (34). Macrophages from heparanase knockout mice also expressed lower levels of the pro-inflammatory cytokines TNF-α, interleukin-1 β (IL-1β), C-X-C motif chemokine ligand 2 (CXCL2) and IL-6 (34, 54). The opposite was observed in a model of pancreatic cancer overexpressing heparanase. Pancreatic tumor cells overexpressing heparanase were implanted into severe combined immune deficiency (SCID) mice, which lack B and T cells (55). Implanted tumors with heparanase-overexpressing pancreatic cancer cells were observed to have more infiltrating macrophages and larger tumors compared to tumors with normal heparanase expression (54) (Figure 1A). The overexpression of heparanase in these pancreatic tumors also led to increased macrophage expression of IL-6, IL-10, C-C motif chemokine ligand-2 (CCL-2), vascular endothelial growth factor (VEGF) and macrophage scavenger receptor-2 (MSR-2) (54) (Figure 1A). Indeed, TAM expression of these cytokines is an indicator of macrophage polarization to an M2 phenotype, which facilitates tumorigenesis (52, 56, 57). These findings suggest that both tumor-derived and macrophage-derived heparanase can promote the recruitment of macrophages to tumors and facilitate their entry to aid tumor progression.
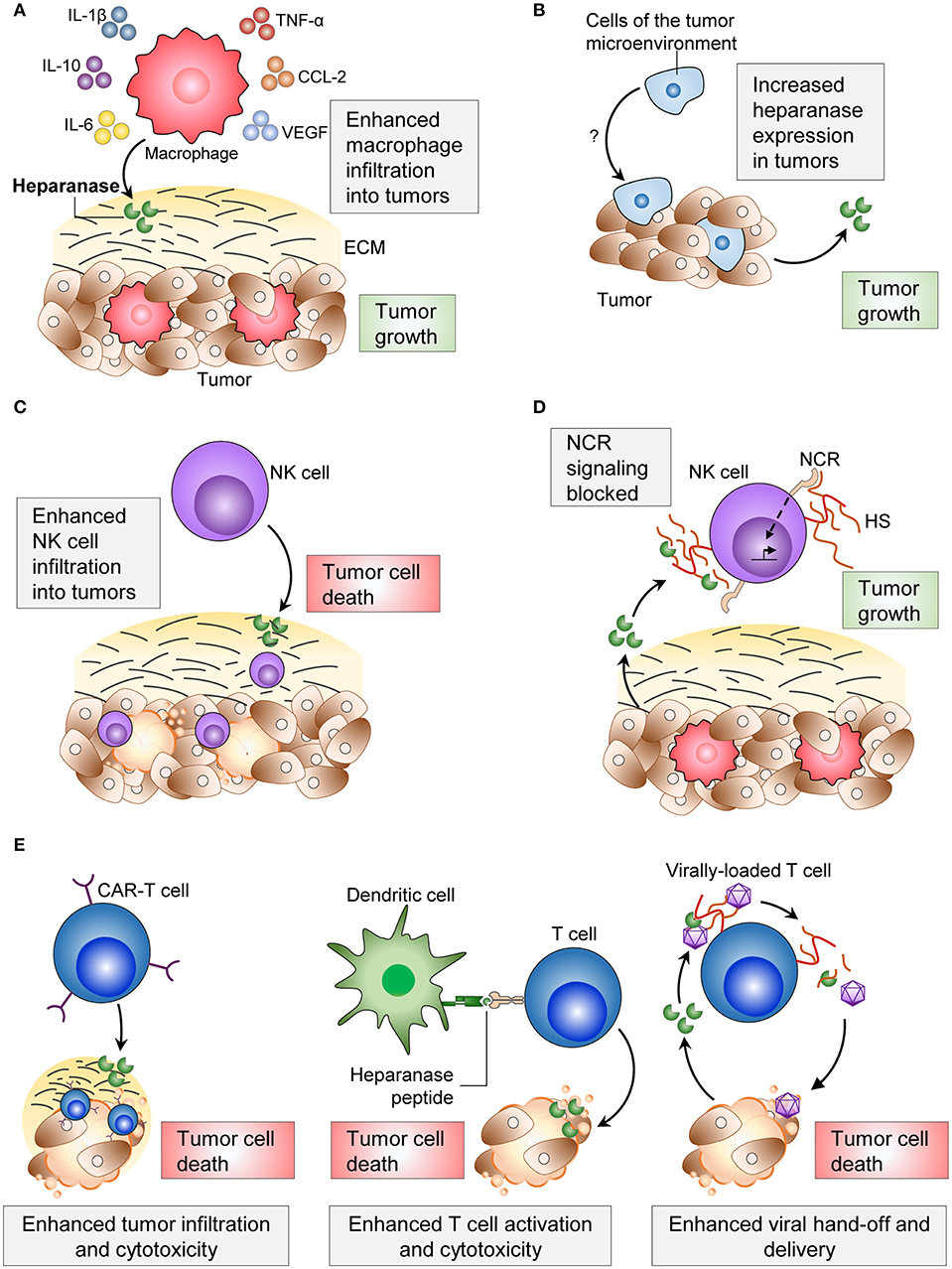
Figure 1. Effects of heparanase on immune cells and the consequences on tumor progression. (A) Heparanase from macrophages and from tumor cells increase macrophage infiltration into tumors, cytokine secretion and phagocytic ability. (B) Cells of the tumor microenvironment increase tumor cell-heparanase and increase tumor cell proliferation. (C) Heparanase enhances NK cell infiltration into tumors and consequent tumor cell clearance. (D) Tumor cell-heparanase can block NCR signaling and consequent activation of NK cells. (E) Applications of immune cell-heparanase include use in CAR T cells, dendritic cell vaccines, and viral delivery of anti-tumorigenic agents. ECM, extracellular matrix; NCR, natural killer cytotoxicity receptor; HS, heparan sulfate; CAR-T, chimeric antigen receptor-T.
During inflammation and inflammation-associated tumorigenesis, the source of heparanase is often the epithelium (58). This was identified in patients with inflammatory bowel disease (IBD) (59), and in an IBD model, epithelial cell-heparanase was found to drive the over-activation of macrophages, inflammation, and ultimately tumorigenesis (60). In this model of IBD, heparanase-overexpressing mice were also observed to have more macrophages in the colon when compared to wild type animals (60). This overexpression of heparanase in the epithelium has been characterized in other models of inflammation, including pancreatitis (61) and Barrett's epithelium in the esophagus (62). However, it remains to be explored whether epithelial cell-heparanase in these settings also influences immune cell activation. Furthermore, another study found that recombinant heparanase added to colorectal cancer cell lines could increase mRNA expression and release of monocyte chemoattractant protein-1 (MCP-1) (63), supporting the notion that heparanase may help to generate a chemokine gradient to recruit macrophages to sites of inflammation.
Together, these findings show that heparanase from the tumor cells, macrophages, and epithelial cells can promote tumorigenesis. However, not all tumor cells overexpress heparanase. Weissmann et al. found that Raji lymphoma cells expressed low levels of heparanase in vitro, but when implanted into mice, exhibited increased heparanase activity (22) (Figure 1B). This may have been a result of the tumor cells upregulating heparanase in response to stimuli from the tumor microenvironment, which could include soluble factors such as TNF-α and IL-1β (35, 43), or heparanase may have originated from other cell types within the tumor microenvironment (e.g., macrophages). Regardless of the source of heparanase, its inhibition with the heparanase neutralizing antibody Ab 1453 in these tumors was sufficient to reduce tumor growth (22). Again, the source of heparanase in this example is unclear, but this study supports the idea that tumor cells can utilize heparanase from the tumor microenvironment with similar outcomes on tumor progression.
Tumor Cells Modulate Heparanase Expression in Lymphocytes
Tumor cells can influence leukocyte function via direct cell-cell interaction (64), or through secreted factors (65). A study by Theodoro et al. found that lymphocytes from peripheral blood mononuclear cells (PBMCs) of breast cancer patients displayed higher heparanase expression than lymphocytes from healthy patients (66). The study also found that heparanase expression was higher in lymphocytes from patients with metastases, and that heparanase expression in circulating lymphocytes was reduced following surgical resection of tumors (66). Breast tumor cells when co-cultured with lymphocytes from healthy donors were shown to induce heparanase expression by the lymphocytes. Furthermore, these experiments suggested that the breast tumor cells induced the lymphocytes to produce soluble factors that were responsible for upregulating heparanase expression (66). It was proposed that by increasing expression of heparanase in tumor-infiltrating lymphocytes, the tumor would have the ability to alter gene expression of many other neoplastic and non-neoplastic cells (66). The impact of these high-heparanase expressing lymphocytes was not tested. However, since these patients had higher instances of metastasis, it suggests that these lymphocytes may be preparing to seek out tumor cells for clearance, given that heparanase is often upregulated upon T cell activation (24, 25, 27, 28).
Heparanase and NK Cell-Mediated Clearance of Tumors
NK cells efficiently kill tumor cells of many origins, and their presence within tumors often correlates with improved survival (67). We recently reported that mice deficient in NK cell-heparanase exhibited reduced NK cell tumor infiltration, resulting in impaired clearance of B16F10 melanoma tumors and metastases (2) (Figure 1C). Furthermore, immune checkpoint inhibitors targeting the programmed death ligand-1 (PDL-1) and cytotoxic T-lymphocyte-associated antigen 4 (CTLA-4) axes were less effective in the absence of NK cell-heparanase (2). These data suggest that using heparanase inhibitors concomitantly with checkpoint inhibitors would be ineffective. To our knowledge, this is the first description of a tumor suppressive role for heparanase.
Heparanase Blocks NK Cell Activation
The role of heparanase in NK cell function does not appear to be simply in cell migration and invasion, but may also regulate NK cell activation and cytotoxicity.
Heparan sulfate on the plasma membrane of NK cells can act in a -cis manner as a co-ligand for the NK cell cytotoxicity receptor (NCR) (68). However, surface heparan sulfate must compete with soluble or -trans heparan sulfate for NCR binding, which dampens NK cell activation. By cleaving heparan sulfate on the surface of NK cells, heparanase secreted by tumors can increase levels of soluble heparan sulfate, and consequently inhibit NK cell activity and cytotoxicity against tumor cells (69) (Figure 1D). It appears that low levels (69) or high levels (68) of -cis heparan sulfate-NCR interaction dampens NK cell activation, and that maintaining optimal levels of membrane-bound heparan sulfate is important for optimizing NK cell activation.
Despite these advances, further investigation is required to fully understand the role of heparanase in leukocyte function during cancer progression. Given the ability of heparanase to modulate pro-inflammatory cytokine levels (3, 60), and activate and recruit tumor-promoting leukocytes (34, 54), it is likely that heparanase plays a greater role in modulating the immune system and immune suppression during cancer progression.
Exploiting Heparanase in Cancer Immunotherapy
Despite the complex pro/anti-tumorigenic axis of heparanase, exploiting heparanase has shown promise in leukocyte-based anti-cancer therapies.
Heparanase in CAR-T Cell Therapy of Solid Tumors
Chimeric antigen receptor (CAR)-T cell therapy utilizes engineered recombinant receptors expressed on T cells containing an antigen-recognition domain of a monoclonal antibody and a T cell-activating domain (70). These CARs enable T cells to specifically and efficiently recognize tumor cells and maximize T cell function. Whilst this therapy has shown promise in many hematological malignancies (71, 72), it is relatively ineffective against solid tumors, partly attributed to the low penetration of CAR-T cells into the tumor (70). To address this, Caruana et al. overexpressed heparanase in human CAR-T cells, and found this to assist CAR-T cell infiltration into neuroblastoma patient-xenograft tumors and enhance anti-tumor activity (73) (Figure 1E). This strategy of using heparanase to increase the penetration of CAR-T cells into tumors shows promise to increase efficacy of the therapy.
Heparanase in DC Vaccines
Heparanase overexpression has been documented across the majority of tumor types, including solid tumors (74–77) and hematological tumors (22, 78). Thus, heparanase represents a potential tumor associated antigen (TAA) that could be exploited across multiple cancer types. Dendritic cell vaccines are a novel approach to selectively target tumor cells overexpressing TAA. Engineered dendritic cells overexpressing TAA can generate antigen-specific T cells that have increased cytotoxicity against tumor cells (79–81).
Heparanase-specific and reactive CD8+ T cells were identified in the bone marrow of a sample of breast cancer patients, and were functionally reactive to heparanase-overexpressing tumor cells (82). The overexpression of heparanase in DCs isolated from PBMCs was shown to enhance the activation of T cells from matching donors, and consequent cytotoxicity against target gastric cancer cells (83). This finding also held true in an animal model, where murine DCs were pulsed with murine heparanase peptides and injected into mice. This vaccine could induce cytotoxic T lymphocytes (CTLs) in mice specific to H-2kb-expressing mouse tumor cell lines (B16, LLC, and EL-4) (84). In addition, administering heparanase peptide-pulsed DCs after injecting B16 tumor cells could slow tumor growth (84). Furthermore, the immunogenicity and efficacy of these peptides was increased when generated in the branched multiple antigenic peptide conformation (85, 86).
The heparanase peptide has also been tested as a TAA in a prophylactic vaccine. Priming mice in vivo with human heparanase peptides (Hpa525, Hpa277, and Hpa405) generated CTLs that specifically targeted human tumor cell lines presenting heparanase on either HLA-A*0201 (87) or HLA-A2 (88) (Figure 1E). Injecting heparanase-pulsed DCs into mice before administering B16 tumor cells was shown to protect animals from tumor growth (84).
These data suggest that heparanase could be a robust tumor antigen, as when targeted, shows both reduction and protection against tumor growth in animal and human systems. However, targeting heparanase via a vaccine approach will rely on tumors maintaining heparanase expression to allow T cell recognition of heparanase-positive tumor cells. Regardless, the selective pressure from these vaccines on tumor cells to downregulate heparanase expression would still be advantageous in blocking tumor progression.
Heparanase in Viral-Therapeutic Delivery
The delivery of gene therapy as a cancer treatment requires specific targeting to tumor cells. A promising approach to target therapies toward tumors is through viral particles via attachment to T cells (89). An important step in the delivery of these therapies is the release or “hand off” (transfer of viral particles from T cells to target tumor cells), when T cells release their viral cargo at the tumor site (89). Heparanase present in the tumor microenvironment, either from malignant cells or activated T cells, was shown to promote viral “hand off” for the successful delivery of anti-tumor molecules to the tumor cells (89) (Figure 1E). Another study using viral gene therapy to treat a murine model of malignant plural mesothelioma found that co-infection with a heparanase-expressing adenovirus vector could enhance efficacy of virotherapy and penetrance into tumors (90), a previous limitation of this therapy. This approach showed a reduction in tumor weight, and an increase in overall survival of animals inoculated with mesothelioma. This is likely a result of heparanase increasing ECM breakdown, as heparanase was shown to enable viral particles to penetrate deeper into tumor spheroids (90).
The robust expression of heparanase across multiple cancer types and cell types makes it a useful target to manipulate and utilize its anti-cancer properties. All of these therapies described will rely on maintained heparanase expression for efficacy. As we will describe, heparanase inhibitors currently used to reduce tumor burden may not always have favorable outcomes on tumor progression, especially for patients undergoing the therapies described above.
Heparanase Inhibitors and Leukocyte Function
Our understanding of the relationship between heparanase and leukocytes during tumor progression remains limited. Similarly, much is still unknown about how heparanase inhibitors affect the anti-tumor immune response, despite their current use in clinical trials against a range of cancers (91–94). A number of heparanase inhibitors in anticancer therapy have been recently reviewed (95–97). These include the heparan sulfate mimetic Roneparstat (SST0001), 2-O-,3-O-desulfated heparin (ODSH, also known as CX-01), Necuparanib (M402), PG545 (a heparan sulfate mimetic conjugated to a lipophilic cholestanol aglycone moiety, also known as Pixatimod) (21), and PI-88 (a heparan sulfate mimetic, also known as Muparfostat) (98).
Preclinical animal models show PG545 can reduce tumor and metastatic burden in several tumor models, including breast, prostate (21), liver (21), lung (21), colon (21), ovarian (94), head, and neck cancers (21, 99, 100), melanoma (21, 101), pancreatic cancer (102, 103), and colon cancer (104). Interestingly, the mechanism by which PG545 exerts its anti-tumor properties has been shown to be multifactorial. In addition to directly blocking heparanase activity (105), PG545 has been shown to reduce heparanase expression, possibly by inhibiting VEGF and FGF2 signaling (99). PG545 has also been shown to inhibit macrophage infiltration into pancreatic tumors (102), activate NK cells via DCs (106), and activate lymphocytes (100), as part of its anti-tumor activity. A phase I clinical trial against a range of advanced solid tumors showed that PG545 stimulated the innate immune response, resulting in an at least a two-fold increase of circulating plasmacytoid DCs and NK cells in majority of patients (93). It is perhaps predominantly through this mechanism of leukocyte activation that this inhibitor exerts its anti-cancer activity, rather than direct tumor cell-heparanase inhibition. Given its modest effects as a monotherapy (93), PG545 will most likely be used in combination with chemotherapy to treat advanced cancers for maximum efficacy.
Other clinically relevant heparanase inhibitors such as Roneparstat and ODSH also display immunomodulatory effects. Roneparstat, in development for the treatment of multiple myeloma, has been observed to effect macrophage polarization by inhibiting the expression of M1 related genes in LPS-stimulated U937 macrophages (107). In a mouse model of ischemia/reperfusion injury, inhibition of heparanase with Roneparstat reduced the number of infiltrating M1 macrophages in the kidney, resulting in lower levels of pro-inflammatory cytokines (107). ODSH is another heparanase inhibitor (108) which blocks multiple steps of inflammation. As described for heparin, ODSH reduces leukocyte rolling, adhesion, and accumulation (109, 110). ODSH has also been shown to inhibit neutrophil elastase and inflammation in a mouse model of neutrophil elastase-induced airway inflammation (111) and in the sputum of cystic fibrosis patients (112). In addition, ODSH also inhibits the accumulation of neutrophils in the airway after Pseudomonas aeruginosa infection (113) and protects against platelet factor 4-induced thrombocytopenia in chemotherapy and radiotherapy-treated animals by acting on megakaryocyte proliferation. Finally, ODSH inhibits high-mobility group box 1 (HMGB1) release from macrophages (111, 113, 114), a potent proinflammatory cytokine, and inhibits P-selectin-mediated macrophage adhesion (115).
More work needs to be done to define and understand the effects of heparanase inhibitors on cells of the immune system. Heparanase inhibitors have been used as anti-inflammatory agents, and have been shown to impair lymphocyte trafficking (116, 117) and leukocyte function (46, 47, 61, 118). It is possible that in some tumor settings, heparanase inhibitors may inhibit leukocyte function, and consequently tip the balance away from tumor clearance and in favor of tumor progression. Heparanase inhibitors may be effective against tumors in which leukocyte-heparanase aids tumor progression, such as colorectal and pancreatic carcinoma (52), but perhaps less effective against other solid tumors which have little heparanase expression in the tumor microenvironment. Choosing the appropriate anti-cancer therapy will lie in finding the balance in particular cancer settings between inhibiting pro-tumorigenic heparanase and promoting its anti-tumorigenic effects.
Conclusions
This review describes how leukocyte-heparanase can be a double-edged sword in tumor progression; it can enhance tumor immune surveillance and tumor cell clearance, but also promote tumor survival and growth. We also discuss the potential of using heparanase in leukocyte therapies against tumors, and the effects of heparanase inhibitors on tumor progression and immunity.
We are just beginning to understand the influence of heparanase on a pro/anti-tumor immune response, and there are still many questions to answer. How do the pro/anti-tumorigenic effects of heparanase differ across different cancer types? Does the tumorigenic effect of heparanase change during cancer progression? And how does the expression or role of heparanase change during treatment regimens? Answering these questions may help guide the appropriate use of heparanase inhibitors, and the use of heparanase-assisted therapies for the treatment of cancer.
Author Contributions
All authors listed have made a substantial, direct and intellectual contribution to the work, and approved it for publication.
Funding
This research was supported by project grants from the National Health and Medical Research Council of Australia (APP471424) and the Association for International Cancer Research (#08-0215).
Conflict of Interest Statement
The authors declare that the research was conducted in the absence of any commercial or financial relationships that could be construed as a potential conflict of interest.
References
1. Poon IKH, Goodall KJ, Phipps S, Chow JDY, Pagler EB, Andrews DM, et al. Mice deficient in heparanase exhibit impaired dendritic cell migration and reduced airway inflammation. Eur J Immunol. (2014) 44:1016–30. doi: 10.1002/eji.201343645
2. Putz EM, Mayfosh AJ, Kos K, Barkauskas DS, Nakamura K, Town L, et al. NK cell heparanase controls tumor invasion and immune surveillance. J Clin Invest. (2017) 127:2777–88. doi: 10.1172/JCI92958
3. Goodall KJ, Poon IKH, Phipps S, Hulett MD. Soluble heparan sulfate fragments generated by heparanase trigger the release of pro-inflammatory cytokines through TLR-4. PLoS ONE. (2014) 9:e109596. doi: 10.1371/journal.pone.0109596
4. Bitan M, Weiss L, Reibstein I, Zeira M, Fellig Y, Slavin S, et al. Heparanase upregulates Th2 cytokines, ameliorating experimental autoimmune encephalitis. Mol Immunol. (2010) 47:1890–8. doi: 10.1016/j.molimm.2010.03.014
5. Elkin Mi, Ilan N, Ishai-Michaeli R, Friedmann Y, Papo O, Pecher I, et al. Heparanase as mediator of angiogenesis: mode of action. FASEB J. (2001) 15:1661–3. doi: 10.1096/fj.00-0895fje
6. Zcharia E, Zilka R, Yaar A, Yacoby-Zeevi O, Zetser A, Metzger S, et al. Heparanase accelerates wound angiogenesis and wound healing in mouse and rat models. FASEB J. (2005) 19:211–21. doi: 10.1096/fj.04-1970com
7. Goldshmidt O, Zcharia E, Cohen M, Aingorn H, Cohen I, Nadav L, et al. Heparanase mediates cell adhesion independent of its enzymatic activity. FASEB J. (2003) 17:1015–25. doi: 10.1096/fj.02-0773com
8. Levy-Adam F, Feld S, Suss-Toby E, Vlodavsky I, Ilan N. Heparanase facilitates cell adhesion and spreading by clustering of cell surface heparan sulfate proteoglycans. PLoS ONE. (2008) 3:e2319. doi: 10.1371/journal.pone.0002319
9. Goldberg R, Meirovitz A, Hirshoren N, Bulvik R, Binder A, Rubinstein AM, et al. Versatile role of heparanase in inflammation. Matrix Biol. (2013) 32:234–40. doi: 10.1016/j.matbio.2013.02.008
10. Vlodavsky I, Blich M, Li JP, Sanderson RD, Ilan N. Involvement of heparanase in atherosclerosis and other vessel wall pathologies. Matrix Biol. (2013) 32:241–51. doi: 10.1016/j.matbio.2013.03.002
11. Parish CR, Freeman C, Ziolkowski AF, He YQ, Sutcliffe EL, Zafar A, et al. Unexpected new roles for heparanase in Type 1 diabetes and immune gene regulation. Matrix Biol. (2013) 32:228–33. doi: 10.1016/j.matbio.2013.02.007
12. Wang F, Wan A, Rodrigues B. The function of heparanase in diabetes and its complications. Can J Diabetes. (2013) 37:332–8. doi: 10.1016/j.jcjd.2013.05.008
13. Simeonovic CJ, Ziolkowski AF, Wu Z, Choong FJ, Freeman C, Parish CR. Heparanase and autoimmune diabetes. Front Immunol. (2013) 4:1–7. doi: 10.3389/fimmu.2013.00471
14. Schmidt EP, Yang Y, Janssen WJ, Gandjeva A, Perez MJ, Barthel L, et al. The pulmonary endothelial glycocalyx regulates neutrophil adhesion and lung injury during experimental sepsis. Nat Med. (2012) 18:1217–23. doi: 10.1038/nm.2843
15. Matos LL, Suarez ER, Theodoro TR, Trufelli DC, Melo CM, Garcia LF, et al. The profile of heparanase expression distinguishes differentiated thyroid carcinoma from benign neoplasms. PLoS ONE. (2015) 10:e0141139. doi: 10.1371/journal.pone.0141139
16. Tang B, Xie R, Qin Y, Xiao Y, Yong X, Zheng L, et al. Human telomerase reverse transcriptase (hTERT) promotes gastric cancer invasion through cooperating with c-Myc to upregulate heparanase expression. Oncotarget. (2015) 7:11364–79. doi: 10.18632/oncotarget.6575
17. Vlodavsky I, Singh P, Boyango I, Gutter-Kapon L, Elkin M, Sanderson RD, et al. Heparanase: from basic research to therapeutic applications in cancer and inflammation. Drug Resist Updat. (2016) 29:54–75. doi: 10.1016/j.drup.2016.10.001
18. Arvatz G, Weissmann M, Ilan N, Vlodavsky I. Heparanase and cancer progression: new directions, new promises. Hum Vaccines Immunother. (2016) 12:2253–6. doi: 10.1080/21645515.2016.1171442
19. Goldshmidt O, Zcharia E, Abramovitch R, Metzger S, Aingorn H, Friedmann Y, et al. Cell surface expression and secretion of heparanase markedly promote tumor angiogenesis and metastasis. Proc Natl Acad Sci USA. (2002) 99:10031–6. doi: 10.1073/pnas.152070599
20. Edovitsky E, Elkin M, Zcharia E, Peretz T, Vlodavsky I. Heparanase gene silencing, tumor invasiveness, angiogenesis, and metastasis. J Natl Cancer Inst. (2004) 96:1219–30. doi: 10.1093/jnci/djh230
21. Dredge K, Hammond E, Handley P, Gonda TJ, Smith MT, Vincent C, et al. PG545, a dual heparanase and angiogenesis inhibitor, induces potent anti-tumour and anti-metastatic efficacy in preclinical models. Br J Cancer. (2011) 104:635–42. doi: 10.1038/bjc.2011.11
22. Weissmann M, Arvatz G, Horowitz N, Feld S, Naroditsky I, Zhang Y, et al. Heparanase-neutralizing antibodies attenuate lymphoma tumor growth and metastasis. Proc Natl Acad Sci USA. (2016) 113:704–9. doi: 10.1073/pnas.1519453113
23. Rubinfeld H, Cohen-Kaplan V, Nass D, Ilan N, Meisel S, Cohen ZR, et al. Heparanase is highly expressed and regulates proliferation in GH-secreting pituitary tumor cells. Endocrinology. (2011) 152:4562–70. doi: 10.1210/en.2011-0273
24. Naparstek Y, Cohen IR, Fuks Z, Vlodavsky I. Activated T lymphocytes produce a matrix-degrading heparan sulphate endoglycosidase. Nature. (1984) 310:241–4.
25. de Mestre AM, Staykova MA, Hornby JR, Willenborg DO, Hulett MD. Expression of the heparan sulfate-degrading enzyme heparanase is induced in infiltrating CD4+ T cells in experimental autoimmune encephalomyelitis and regulated at the level of transcription by early growth response gene1. J Leukoc Biol. (2007) 82:1289–300. doi: 10.1189/jlb.0507315
26. de Mestre AM, Soe-Htwe T, Sutcliffe EL, Rao S, Pagler EB, Hornby JR, et al. Regulation of mouse Heparanase gene expression in T lymphocytes and tumor cells. Immunol Cell Biol. (2007) 85:205–14. doi: 10.1038/sj.icb.7100022
27. Fridman R, Lider O, Naparstek Y, Fuks Z, Vlodavsky I, Cohen IR. Soluble antigen induces T lymphocytes to secrete an endoglycosidase that degrades the heparan sulfate moiety of subendothelial extracellular matrix. J Cell Physiol. (1987) 130:85–92. doi: 10.1002/jcp.1041300113
28. Stoler-Barak L, Petrovich E, Aychek T, Gurevich I, Tal O, Hatzav M, et al. Heparanase of murine effector lymphocytes and neutrophils is not required for their diapedesis into sites of inflammation. FASEB J. (2015) 29:2010–21. doi: 10.1096/fj.14-265447
29. Santos TCF, dos Gomes AM, Paschoal ME, Stelling MP, Rumjanek VM, Junior A, et al. Heparanase expression and localization in different types of human lung cancer. Biochim Biophys Acta. (2014) 1840:2599–608. doi: 10.1016/j.bbagen.2014.04.010
30. Savion N, Vlodavsky I, Fuks Z. Interaction of T lymphocytes and macrophages with cultured vascular endothelial cells: attachment, invasion, and subsequent degradation of the subendothelial extracellular matrix. J Cell Physiol. (1984) 118:169–78. doi: 10.1002/jcp.1041180209
31. Laskov R, Michaeli RI, Sharir H, Yefenof E, Vlodavsky I. Production of heparanase by normal and neoplastic murine B-lymphocytes. Int J Cancer. (1991) 47:92–8. doi: 10.1002/ijc.2910470117
32. Benhamron S, Nechushtan H, Verbovetski I, Krispin A, Abboud-Jarrous G, Zcharia E, et al. Translocation of active heparanase to cell surface regulates degradation of extracellular matrix heparan sulfate upon transmigration of mature monocyte-derived dendritic cells. J Immunol. (2006) 176:6417–24. doi: 10.4049/jimmunol.176.11.6417
33. Savion N, Disatnik MH, Nevo Z. Murine macrophage heparanase: inhibition and comparison with metastatic tumor cells. J Cell Physiol. (1987) 130:77–84. doi: 10.1002/jcp.1041300112
34. Gutter-Kapon L, Alishekevitz D, Shaked Y, Li J-P, Aronheim A, Ilan N, et al. Heparanase is required for activation and function of macrophages. Proc Natl Acad Sci USA. (2016) 113:E7808–17. doi: 10.1073/pnas.1611380113
35. Secchi MF, Crescenzi M, Masola V, Russo FP, Floreani A, Onisto M. Heparanase and macrophage interplay in the onset of liver fibrosis. Sci Rep. (2017) 7:14956. doi: 10.1038/s41598-017-14946-0
36. Tao C, Wang W, Zhou P, Zhou X, Zhang Q, Liu B. Molecular characterization, expression profiles of the porcine SDC2 and HSPG2 genes and their association with hematologic parameters. Mol Biol Rep. (2013) 40:2549–56. doi: 10.1007/s11033-012-2340-2
37. Graham LD, Underwood PA. Comparison of the heparanase enzymes from mouse melanoma cells, mouse macrophages, and human platelets. Biochem Mol Biol Int. (1996) 39:563–71.
38. Matzner Y, Bar-Ner M, Yahalom J, Ishai-Michaeli R, Fuks Z, Vlodavsky I. Degradation of heparan sulfate in the subendothelial extracellular matrix by a readily released heparanase from human neutrophils. Possible role in invasion through basement membranes. J Clin Invest. (1985) 76:1306–13. doi: 10.1172/JCI112104
39. Komatsu N, Waki M, Sue M, Tokuda C, Kasaoka T, Nakajima M, et al. Heparanase expression in B16 melanoma cells and peripheral blood neutrophils before and after extravasation detected by novel anti-mouse heparanase monoclonal antibodies. J Immunol Methods. (2008) 331:82–93. doi: 10.1016/j.jim.2007.11.014
40. Bartlett MR, Underwood PA, Parish CR. Comparative analysis of the ability of leucocytes, endothelial cells and platelets to degrade the subendothelial basement membrane: evidence for cytokine dependence and detection of a novel sulfatase. Immunol Cell Biol. (1995) 73:113–24. doi: 10.1038/icb.1995.19
41. Bashkin P, Razin E, Eldor A, Vlodavsky I. Degranulating mast cells secrete an endoglycosidase that degrades heparan sulfate in subendothelial extracellular matrix. Blood. (1990) 75:2204–12.
42. Temkin V, Aingorn H, Puxeddu I, Goldshmidt O, Zcharia E, Gleich GJ, et al. Eosinophil major basic protein: first identified natural heparanase-inhibiting protein. J Allergy Clin Immunol. (2004) 113:703–9. doi: 10.1016/j.jaci.2003.11.038
43. Chen G, Wang D, Vikramadithyan R, Yagyu H, Saxena U, Pillarisetti S, et al. Inflammatory cytokines and fatty acids regulate endothelial cell heparanase expression. Biochemistry. (2004) 43:4971–7. doi: 10.1021/bi0356552
44. de Mestre AM, Khachigian LM, Santiago FS, Staykova MA, Hulett MD. Regulation of inducible heparanase gene transcription in activated T cells by early growth response 1. J Biol Chem. (2003) 278:50377–85. doi: 10.1074/jbc.M310154200
45. Digre A, Singh K, Åbrink M, Reijmers RM, Sandler S, Vlodavsky I, et al. Overexpression of heparanase enhances T lymphocyte activities and intensifies the inflammatory response in a model of murine rheumatoid arthritis. Sci Rep. (2017) 7:1–12. doi: 10.1038/srep46229
46. Changyaleket B, Chong ZZ, Dull RO, Nanegrungsunk D, Xu H. Heparanase promotes neuroinflammatory response during subarachnoid hemorrhage in rats. J Neuroinflammation. (2017) 14:1–9. doi: 10.1186/s12974-017-0912-8
47. Lever R, Rose MJ, McKenzie EA, Page CP. Heparanase induces inflammatory cell recruitment in vivo by promoting adhesion to vascular endothelium. AJP Cell Physiol. (2014) 306:C1184–90. doi: 10.1152/ajpcell.00269.2013
48. Smith HA, Kang Y. The metastasis-promoting roles of tumor-associated immune cells. J Mol Med. (2013) 91:411–29. doi: 10.1007/s00109-013-1021-5
49. Bremnes RM, Al-Shibli K, Donnem T, Sirera R, Al-Saad S, Andersen S, et al. The role of tumor-infiltrating immune cells and chronic inflammation at the tumor site on cancer development, progression, and prognosis: emphasis on non-small cell lung cancer. J Thorac Oncol. (2011) 6:824–33. doi: 10.1097/JTO.0b013e3182037b76
50. Jochems C, Schlom J. Tumor-infiltrating immune cells and prognosis: the potential link between conventional cancer therapy and immunity. Exp Biol Med. (2011) 236:567–79. doi: 10.1258/ebm.2011.011007
51. Thorsson V, Gibbs DL, Brown SD, Wolf D, Bortone DS, Ou Yang T-H, et al. The immune landscape of cancer. Immunity. (2018) 48:812–30. doi: 10.1016/j.immuni.2018.03.023
52. Nielsen SR, Schmid MC. Macrophages as key drivers of cancer progression and metastasis. Mediat Inflamm. (2017) 2017:9624760. doi: 10.1155/2017/9624760
53. Noy R, Pollard JW. Tumor-associated macrophages: from mechanisms to therapy. Immunity. (2014) 41:49–61. doi: 10.1016/j.immuni.2014.06.010
54. Hermano E, Meirovitz A, Meir K, Nussbaum G, Appelbaum L, Peretz T, et al. Macrophage polarization in pancreatic carcinoma: role of heparanase enzyme. J Natl Cancer Inst. (2014) 106:1–10. doi: 10.1093/jnci/dju332
55. Bosma MJ, Carroll AM. The SCID mouse mutant: definition, characterization, and potential uses. Annu Rev Immunol. (1991) 9:323–50. doi: 10.1146/annurev.iy.09.040191.001543
56. Weagel E, Smith C, Liu PG, Robison R, O'Neill K. Macrophage polarization and its role in cancer. J Clin Cell Immunol. (2015) 6:4–11. doi: 10.4172/2155-9899.1000338
57. Duque GA, Descoteaux A. Macrophage cytokines: involvement in immunity and infectious diseases. Front Immunol. (2014) 5:1–12. doi: 10.3389/fimmu.2014.00491
58. Meirovitz A, Goldberg R, Binder A, Rubinstein AM, Hermano E, Elkin M. Heparanase in inflammation and inflammation-associated cancer. FEBS J. (2013) 280:2307–19. doi: 10.1111/febs.12184
59. Waterman M, Ben-Izhak O, Eliakim R, Groisman G, Vlodavsky I, Ilan N. Heparanase upregulation by colonic epithelium in inflammatory bowel disease. Mod Pathol. (2007) 20:8–14. doi: 10.1038/modpathol.3800710
60. Lerner I, Hermano E, Zcharia E, Rodkin D, Bulvik R, Doviner V, et al. Heparanase powers a chronic inflammatory circuit that promotes colitis-associated tumorigenesis in mice. J Clin Invest. (2011) 121:1709–21. doi: 10.1172/JCI43792
61. Khamaysi I, Singh P, Nasser S, Awad H, Chowers Y, Sabo E, et al. The role of heparanase in the pathogenesis of acute pancreatitis: a potential therapeutic target. Sci Rep. (2017) 7:715. doi: 10.1038/s41598-017-00715-6
62. Brun R, Naroditsky I, Waterman M, Ben-Izhak O, Groisman G, Ilan N, et al. Heparanase expression by Barrett's epithelium and during esophageal carcinoma progression. Mod Pathol. (2009) 22:1548–54. doi: 10.1038/modpathol.2009.115
63. Tsunekawa N, Higashi N, Kogane Y, Waki M, Shida H, Nishimura Y, et al. Heparanase augments inflammatory chemokine production from colorectal carcinoma cell lines. Biochem Biophys Res Commun. (2015) 543:1–6. doi: 10.1016/j.bbrc.2015.12.074
64. Liu Y, Zeng G. Cancer and innate immune system interactions. J Immunother. (2012) 35:299–308. doi: 10.1097/CJI.0b013e3182518e83
65. Kano A. Tumor cell secretion of soluble factor(s) for specific immunosuppression. Sci Rep. (2015) 5:1–8. doi: 10.1038/srep08913
66. Theodoro TR, de Matos LL, Sant Anna AVL, Fonseca FLA, Semedo P, Martins LC, et al. Heparanase expression in circulating lymphocytes of breast cancer patients depends on the presence of the primary tumor and/or systemic metastasis. Neoplasia. (2007) 9:504–10. doi: 10.1593/neo.07241
67. Larsen SK, Gao Y, Basse PH. NK cells in the tumor microenvironment. Crit Rev Oncog. (2014) 19:91–105. doi: 10.1016/j.biotechadv.2011.08.021.Secreted
68. Brusilovsky M, Radinsky O, Cohen L, Yossef R, Shemesh A, Braiman A, et al. Regulation of natural cytotoxicity receptors by heparan sulfate proteoglycans in -cis: a lesson from NKp44. Eur J Immunol. (2015) 45:1180–91. doi: 10.1002/eji.201445177
69. Mayes K, Elsayed Z, Alhazmi A, Waters M, Alkhatib SG, Roberts M, et al. BPTF inhibits NK cell activity and the abundance of natural cytotoxicity receptor co-ligands. Oncotarget. (2017) 8:64344–57. doi: 10.18632/oncotarget.17834
70. Di S, Li Z. Treatment of solid tumors with chimeric antigen receptor-engineered T cells: current status and future prospects. Sci China Life Sci. (2016) 59:360–9. doi: 10.1007/s11427-016-5025-6
71. Kalos M, Levine BL, Porter DL, Katz S, Grupp SA, Bagg A, et al. T cells with chimeric antigen receptors have potent antitumor effects and can establish memory in patients with advanced leukemia. Sci. Transl. Med. (2011) 3:95ra73. doi: 10.1126/scitranslmed.3002842
72. Davila ML, Riviere I, Wang X, Bartido S, Park J, Curran K, et al. Efficacy and toxicity management of 19-28z CAR T cell therapy in B cell acute lymphoblastic leukemia. Sci Transl Med. (2014) 6:224ra25. doi: 10.1126/scitranslmed.3008226
73. Caruana I, Savoldo B, Hoyos V, Weber G, Liu H, Kim ES, et al. Heparanase promotes tumor infiltration and antitumor activity of CAR-redirected T lymphocytes. Nat Med. (2015) 21:1–8. doi: 10.1038/nm.3833
74. Kundu S, Xiong A, Spyrou A, Wicher G, Marinescu VD, Edqvist P-HD, et al. Heparanase promotes glioma progression and is inversely correlated with patient survival. Mol Cancer Res. (2016) 14:1243–53. doi: 10.1158/1541-7786.MCR-16-0223
75. Hammond E, Khurana A, Shridhar V, Dredge K. The role of heparanase and sulfatases in the modification of heparan sulfate proteoglycans within the tumor microenvironment and opportunities for novel cancer therapeutics. Front Oncol. (2014) 4:195. doi: 10.3389/fonc.2014.00195
76. Ilan N, Elkin M, Vlodavsky I. Regulation, function and clinical significance of heparanase in cancer metastasis and angiogenesis. IJBCB. (2006) 38:2018–39. doi: 10.1016/j.biocel.2006.06.004
77. Cohen-Kaplan V, Jrbashyan J, Yanir Y, Naroditsky I, Ben-Izhak O, Ilan N, et al. Heparanase induces signal transducer and activator of transcription (STAT) protein phosphorylation. J Biol Chem. (2012) 287:6668–78. doi: 10.1074/jbc.M111.271346
78. Ishai-Michaeli R, Eldor A, Vlodavsky I. Heparanase activity expressed by platelets, neutrophils, and lymphoma cells releases active fibroblast growth factor from extracellular matrix. Cell Regul. (1990) 1:833–42. doi: 10.1017/CBO9781107415324.004
79. Timmerman JM, Levy R. Dendritic cell vaccines for cancer immunotherapy. Annu Rev Med. (1999) 50:507–29. doi: 10.1146/annurev.med.50.1.507
80. Bol KF, Schreibelt G, Gerritsen WR, De Vries IJM, Figdor CG. Dendritic cell-based immunotherapy: state of the art and beyond. Clin Cancer Res. (2016) 22:1897–906. doi: 10.1158/1078-0432.CCR-15-1399
81. Boudreau JE, Bonehill A, Thielemans K, Wan Y. Engineering dendritic cells to enhance cancer immunotherapy. Mol Ther. (2011) 19:841–53. doi: 10.1038/mt.2011.57
82. Sommerfeldt N, Beckhove P, Ge Y, Schütz F, Choi C, Bucur M, et al. Heparanase: a new metastasis-associated antigen recognized in breast cancer patients by spontaneously induced memory T lymphocytes. Cancer Res. (2006) 66:7716–23. doi: 10.1158/0008-5472.CAN-05-2363
83. Cai YG, Fang DC, Chen L, Tang XD, Chen T, Yu ST, et al. Dendritic cells reconstituted with a human heparanase gene induce potent cytotoxic T-cell responses against gastric tumor cells in vitro. Tumor Biol. (2007) 28:238–46. doi: 10.1159/000107584
84. Tang XD, Wan Y, Chen L, Chen T, Yu ST, Xiong Z, et al. H-2Kb-restricted CTL epitopes from mouse heparanase elicit an antitumor immune response in vivo. Cancer Res. (2008) 68:1529–37. doi: 10.1158/0008-5472.CAN-07-5965
85. Tang X-D, Wang G-Z, Guo J, Lu M-H, Li C-Z, Li N, et al. Multiple antigenic peptides based on H-2Kb-restricted CTL epitopes from murine heparanase induce a potent antitumor immune response in vivo. Mol Cancer Ther. (2012) 11:1183–92. doi: 10.1158/1535-7163.MCT-11-0607
86. Wang G-Z, Tang X-D, Lu M-H, Gao J-H, Liang G-P, Li N, et al. Multiple antigenic peptides of human heparanase elicit a much more potent immune response against tumors. Cancer Prev Res. (2011) 4:1285–95. doi: 10.1158/1940-6207.CAPR-11-0083
87. Tang X-D, Liang G-P, Li C, Wan Y, Chen T, Chen L, et al. Cytotoxic T lymphocyte epitopes from human heparanase can elicit a potent anti-tumor immune response in mice. Cancer Immunol Immunother. (2010) 59:1041–7. doi: 10.1007/s00262-010-0829-x
88. Chen T, Tang X-D, Wan Y, Chen L, Yu S-T, Xiong Z, et al. HLA-A2-restricted cytotoxic T lymphocyte epitopes from human heparanase as novel targets for broad-spectrum tumor immunotherapy. Neoplasia. (2008) 10:977–86. doi: 10.1593/neo.08576
89. Cole C, Qiao J, Kottke T, Diaz RM, Ahmed A, Sanchez-Perez L, et al. Tumor-targeted, systemic delivery of therapeutic viral vectors using hitchhiking on antigen-specific T cells. Nat Med. (2005) 11:1073–81. doi: 10.1038/nm1297
90. Watanabe Y, Kojima T, Kagawa S, Uno F, Hashimoto Y, Kyo S, et al. A novel translational approach for human malignant pleural mesothelioma: heparanase-assisted dual virotherapy. Oncogene. (2010) 29:1145–54. doi: 10.1038/onc.2009.415
91. Liu C-J, Lee P-H, Lin D-Y, Wu C-C, Jeng L-B, Lin P-W, et al. Heparanase inhibitor PI-88 as adjuvant therapy for hepatocellular carcinoma after curative resection: a randomized phase II trial for safety and optimal dosage. J Hepatol. (2009) 50:958–68. doi: 10.1016/j.jhep.2008.12.023
92. Liu CJ, Chang J, Lee PH, Lin DY, Wu CC, Jeng L, et al. Adjuvant heparanase inhibitor PI-88 therapy for hepatocellular carcinoma recurrence. World J Gastroenterol. (2014) 20:11381–93. doi: 10.3748/wjg.v20.i32.11384
93. Dredge K, Brennan TV, Hammond E, Lickliter JD, Lin L, Bampton D, et al. A Phase I study of the novel immunomodulatory agent PG545 (pixatimod) in subjects with advanced solid tumours. Br J Cancer. (2018) 118:1035–41. doi: 10.1038/s41416-018-0006-0
94. Winterhoff B, Freyer L, Hammond E, Giri S, Mondal S, Roy D, et al. PG545 enhances anti-cancer activity of chemotherapy in ovarian models and increases surrogate biomarkers such as VEGF in preclinical and clinical plasma samples. Eur J Cancer. (2015) 51:879–92. doi: 10.1016/j.ejca.2015.02.007
95. Heyman B, Yang Y. Mechanisms of heparanase inhibitors in cancer therapy. Exp Hematol. (2016) 44:1002–12. doi: 10.1016/j.exphem.2016.08.006
96. Lanzi C, Zaffaroni N, Cassinelli G. Targeting heparan sulfate proteoglycans and their modifying enzymes to enhance anticancer chemotherapy efficacy and overcome drug resistance. Curr Med Chem. (2017) 24:2860–86. doi: 10.2174/0929867324666170216114248
97. Lanzi C, Cassinelli G. Heparan sulfate mimetics in cancer therapy: the challenge to define structural determinants and the relevance of targets for optimal activity. Molecules. (2018) 23:2915. doi: 10.3390/molecules23112915
98. Khachigian LM, Parish CR. Phosphomannopentaose sulfate (PI-88): heparan sulfate mimetic with clinical potential in multiple vascular pathologies. Cardiovasc Drug Rev. (2004) 22:1–6. doi: 10.1111/j.1527-3466.2004.tb00127.x
99. Hammond E, Brandt R, Dredge K. PG545, a heparan sulfate mimetic, reduces heparanase expression in vivo, blocks spontaneous metastases and enhances overall survival in the 4T1 breast carcinoma model. PLoS ONE. (2012) 7:e52175. doi: 10.1371/journal.pone.0052175
100. Hammond E, Haynes NM, Cullinane C, Brennan TV, Bampton D, Handley P, et al. Immunomodulatory activities of pixatimod: emerging nonclinical and clinical data, and its potential utility in combination with PD-1 inhibitors. J Immunother Cancer. (2018) 6:54. doi: 10.1186/s40425-018-0363-5
101. Dredge K, Hammond E, Davis K, Li CP, Liu L, Johnstone K, et al. The PG500 series: novel heparan sulfate mimetics as potent angiogenesis and heparanase inhibitors for cancer therapy. Invest New Drugs. (2010) 28:276–83. doi: 10.1007/s10637-009-9245-5
102. Ostapoff KT, Awasthi N, Cenik BK, Hinz S, Dredge K, Schwarz RE, et al. PG545, an angiogenesis and heparanase inhibitor, reduces primary tumor growth and metastasis in experimental pancreatic cancer. Mol Cancer Ther. (2013) 12:1190–201. doi: 10.1158/1535-7163.MCT-12-1123
103. Jung D-B, Yun M, Kim E-O, Kim J, Kim B, Jung JH, et al. The heparan sulfate mimetic PG545 interferes with Wnt/beta-catenin signaling and significantly suppresses pancreatic tumorigenesis alone and in combination with gemcitabine. Oncotarget. (2015) 6:4992–5004. doi: 10.18632/oncotarget.3214
104. Singh P, Blatt A, Feld S, Zohar Y, Saadi E, Barki-Harrington L, et al. The heparanase inhibitor PG545 attenuates colon cancer initiation and growth, associating with increased p21 expression. Neoplasia. (2017) 19:175–84. doi: 10.1016/j.neo.2016.12.001
105. Hammond E, Handley P, Dredge K, Bytheway I. Mechanisms of heparanase inhibition by the heparan sulfate mimetic PG545 and three structural analogues. FEBS Open Bio. (2013) 3:346–51. doi: 10.1016/j.fob.2013.07.007
106. Brennan TV, Lin L, Brandstadter JD, Rendell VR, Dredge K, Huang X, et al. Heparan sulfate mimetic PG545-mediated antilymphoma effects require TLR9-dependent NK cell activation. J Clin Invest. (2016) 126:207–19. doi: 10.1172/JCI76566
107. Masola V, Zaza G, Bellin G, Dall'Olmo L, Granata S, Vischini G, et al. Heparanase regulates the M1 polarization of renal macrophages and their crosstalk with renal epithelial tubular cells after ischemia/reperfusion injury. FASEB J. (2018) 32:742–56. doi: 10.1096/fj.201700597R
108. Lapierre F, Holme K, Lam L, Tressler RJ, Storm N, Wee J, et al. Chemical modifications of heparin that diminish its anticoagulant but preserve its heparanase-inhibitory, angiostatic, anti-tumor and anti-metastatic properties. Glycobiology. (1996) 6:355–66. doi: 10.1093/glycob/6.3.355
109. Nagata K, Suto Y, Cognetti J, Browne KD, Kumasaka K, Johnson VE, et al. Early low-anticoagulant desulfated heparin after traumatic brain injury. J Trauma Acute Care Surg. (2018) 84:727–35. doi: 10.1097/ta.0000000000001819
110. Smailbegovic A, Lever R, Page CP. The effects of heparin on the adhesion of human peripheral blood mononuclear cells to human stimulated umbilical vein endothelial cells. Br J Pharmacol. (2001) 134:827–36. doi: 10.1038/sj.bjp.0704321
111. Griffin KL, Fischer BM, Kummarapurugu AB, Zheng S, Kennedy TP, Rao NV, et al. A. 2-O, 3-O-desulfated heparin inhibits neutrophil elastase-induced HMGB-1 secretion and airway inflammation. Am J Respir Cell Mol Biol. (2014) 50:684–9. doi: 10.1165/rcmb.2013-0338RC
112. Kummarapurugu AB, Afosah DK, Sankaranarayanan NV, Gangji RN, Zheng S, Kennedy T, et al. Molecular principles for heparin oligosaccharide-based inhibition of neutrophil elastase in cystic fibrosis. J Biol Chem. (2018) 293:12480–90. doi: 10.1074/jbc.RA118.002644
113. Sharma L, Wu J, Patel V, Sitapara R, Rao NV, Kennedy TP, et al. Partially-desulfated heparin improves survival in Pseudomonas pneumonia by enhancing bacterial clearance and ameliorating lung injury. J Immunotoxicol. (2014) 11:260–7. doi: 10.3109/1547691X.2013.839587
114. Zheng S, Kummarapurugu AB, Afosah DK, Sankaranarayanan NV, Boothello RS, Desai UR, et al. 2-O, 3-O desulfated heparin blocks high mobility group box 1 release by inhibition of p300 acetyltransferase activity. Am J Respir Cell Mol Biol. (2017) 56:90–8. doi: 10.1165/rcmb.2016-0069OC
115. Rao NV, Argyle B, Xu X, Reynolds PR, Walenga JM, Prechel M, et al. Low anticoagulant heparin targets multiple sites of inflammation, suppresses heparin-induced thrombocytopenia, and inhibits interaction of RAGE with its ligands. Am J Physiol Physiol. (2010) 299:C97–110. doi: 10.1152/ajpcell.00009.2010
116. Lider O, Mekori YA, Miller T, Bar-Tana R, Vlodavsky I, Baharav E, et al. Inhibition of T lymphocyte heparanase by heparin prevents T cell migration and T cell-mediated immunity. Eur J Immunol. (1990) 20:493–9. doi: 10.1002/eji.1830200306
117. Vlodavsky I, Eldor A, Haimovitz-Friedman A, Matzner Y, Ishai-Michaeli R, Lider O, et al. Expression of heparanase by platelets and circulating cells of the immune system: possible involvement in diapedesis and extravasation. Invasion Metastasis. (1992) 12:112–27.
Keywords: heparanase, leukocytes, macrophages, natural killer cells, immunotherapy, tumor progression
Citation: Mayfosh AJ, Baschuk N and Hulett MD (2019) Leukocyte Heparanase: A Double-Edged Sword in Tumor Progression. Front. Oncol. 9:331. doi: 10.3389/fonc.2019.00331
Received: 26 February 2019; Accepted: 11 April 2019;
Published: 29 April 2019.
Edited by:
Giuliana Cassinelli, National Tumor Institute, ItalyReviewed by:
Mauro Sergio Pavao, Federal University of Rio de Janeiro, BrazilLukas Martin, University Hospital RWTH Aachen, Germany
Copyright © 2019 Mayfosh, Baschuk and Hulett. This is an open-access article distributed under the terms of the Creative Commons Attribution License (CC BY). The use, distribution or reproduction in other forums is permitted, provided the original author(s) and the copyright owner(s) are credited and that the original publication in this journal is cited, in accordance with accepted academic practice. No use, distribution or reproduction is permitted which does not comply with these terms.
*Correspondence: Mark D. Hulett, m.hulett@latrobe.edu.au