- 1Department of Radiation Oncology, The Ohio State University Comprehensive Cancer Center, Arthur G. James Cancer Hospital and Richard J. Solove Research Institute, Columbus, OH, United States
- 2Methodist East Brook Cancer Center, Omaha, NE, United States
- 3Department of Radiation Oncology, Moffitt Cancer Center, Tampa Bay, FL, United States
Purpose: To test the hypothesis that increasing radiation dose to the thoracic marrow (TM) contributes to the development of hematologic toxicities (HT) in esophageal cancer (EC) patients receiving chemoradiation therapy (CRT).
Methods: We identified EC cases treated with curative intent CRT at our institution from 2007 to 2016. The TM was contoured as the union of the vertebral bodies (VB) from T1-L1, the ribs from T1-L1, and the sternum. The TM-mean dose and the TM volume receiving at least 5–50 Gy (V5-V50) were collected. Grade ≥ 3 HT (HT3+) was the primary endpoint. Normal tissue complication probability (NTCP) was evaluated using the Lyman-Kutcher-Burman (LKB) model. Logistic regression was used to test associations between HT3+ and dosimetric parameters. Odds ratios (OR) and 95% confidence intervals (CI) are reported with p < 0.05 considered significant. Receiver operating characteristics analysis was used to determine optimal cut points.
Results: We identified 137 EC cases, and most received concurrent carboplatin/paclitaxel (N = 83). Median radiation dose was 50.4 Gy (IQR = 50.4–50.4 Gy). The rate of HT3+ was 39.4%. Optimization of the LKB model yielded the results n = 0.70, m = 0.67, and TD50 = 20.1 Gy. The TM-V30 was most strongly associated with HT3+ and on multivariate analysis, patients with TM-V30 ≥ 14% had a 5.7-fold (95% CI 2.42–14.54, p < 0.001) increased odds of HT3+ in the entire cohort and a 4-fold (95% CI 1.54–11.11, p = 0.006) increased odds of HT3+ in the carboplatin/paclitaxel cohort compared to patients with TM-V30 < 14%. Radiation dose to the VB and rib sub-sites of the TM were also associated with HT3+, particularly VB-V40.
Conclusion: We found that increasing TM radiation dose was associated with HT3+ in EC patients treated with CRT. Radiation dose to the VB and rib sub-sites were also associated with HT3+. These findings suggest that limiting radiation dose to the TM (or its sub-sites) may be sufficient to decrease HT3+, but further prospective evaluation of these results is needed.
Introduction
Esophageal cancer is a common malignancy and leads to 16,000 deaths in the United States each year (1). Most patients with locally-advanced esophageal cancer are treated with concurrent chemoradiation (CRT) in the neoadjuvant or definitive setting (2, 3). CRT is often associated with severe acute hematologic toxicities (HT) (4, 5).
Myelosuppression is a negative prognostic factor in patients undergoing CRT for esophageal cancer (4, 6). Despite this, little is understood about the additional myelosuppressive impact of radiation to the bony structures in the thorax, such as the thoracic vertebral bodies (VB), when given with chemotherapy. Previous reports have suggested that greater radiation (RT) doses to the thoracic VB during CRT for lung cancer are associated with greater rates of HT (7, 8). In 2016, a report of 46 esophageal cancer patients receiving CRT with cisplatin and 5-fluorouracil found greater thoracic VB and rib irradiation to be associated with grade 3 leukopenia (9). Therefore, efforts to reduce radiation dose to the thoracic marrow (TM) may lead to reductions in HT. The potential benefits of reducing HT include but are not limited to: less frequent therapy interruptions or delays in care, decreased hospitalizations, decreased need for transfusions, decreased risk of infection and decreased use of antibiotics, improved quality of life, reduced costs of care, and importantly, improving cancer control/disease outcome. In the era of intensity modulated radiation therapy (IMRT), reducing the dose to the TM is potentially achievable.
In this study we aim to determine the impact of radiation dose to the TM on the development of HT. We hypothesize that increasing radiation dose to the TM contributes to the development of grade ≥ 3HT (HT3+) in esophageal cancer patients receiving CRT.
Materials and Methods
Patient Selection
We identified patients with histologically confirmed esophageal adenocarcinoma and squamous cell carcinoma treated with curative intent either neoadjuvant or definitive CRT at our institution between 2007 and 2016 in this institutional review board-approved, retrospective study. Patients were required to have complete blood count (CBC) data, which included a baseline CBC (at least 1 week prior to start of CRT) and at least 3 values during treatment. Patients who received induction chemotherapy (IC) were included in this study, provided blood counts recovered before CRT initiation. Initially, 162 patients were identified who completed radiation therapy at our institution. There were 21 patients with incomplete CBC data and 3 additional patients who did not recover blood counts after IC that were excluded from the study. One patient did not have a planning CT scan that included all the vertebral bodies contoured in our study and was thus excluded. The remaining 137 patients formed the cohort for this study.
Radiation Therapy
A free-breathing CT scan was used for patient simulation. Patients were simulated in the supine position with arms over head. A vacuum cushion bag was utilized for immobilization. The gross tumor volume (GTV) was contoured on the CT scan, encompassing the primary tumor. The gross nodal volume (GTVn) was contoured to include all identifiable nodal disease. When available, PET fusion was used to help identify areas of active disease. Expansion of the GTV and GTVn was performed to create the clinical target volume (CTV) in order to include microscopic disease, and the CTV further expanded to a planning target volume (PTV), to account for clinical set-up error. Radiation was delivered at doses of 1.8–2.0 Gy per fraction to the PTV to a dose of 50.4–59.4 Gy utilizing 3-D conformal (3DRT) or IMRT techniques.
Chemotherapy
Chemotherapy regimens included carboplatin/paclitaxel (typically carboplatin AUC 2 mg/m2/min and paclitaxel 50 mg/m2), cisplatin/5-fluorouracil (FU) (typically cisplatin 75 mg/m2 and 5-FU 1,000mg/m2), cisplatin/irinotecan (typically cisplatin 30 mg/m2 and Irinotecan 65 mg/m2), or 5FU alone (typically 5FU 300 mg/m2).
Data Collection
We retrospectively contoured the sternum, the VBs from T1-L1, and the ribs from T1-L1 for all patients (Figure 1). We defined the TM as the union of the sternum, VBs, and ribs. The TM volumes receiving 5–50 Gy (V5-V50) along with the mean TM doses were calculated from the dose volume histogram (DVH). This was done for each of the three TM sub-sites as well. CBC data was obtained from the electronic medical record. The nadirs for absolute white blood count (WBC), neutrophil count (ANC), and absolute platelet count were recorded. Hemoglobin was not included given that EC patients may present with anemia. The Common Terminology Criteria for Adverse Events version 5 was used to grade the hematologic nadir. The primary endpoint analyzed was HT3+ for WBC, ANC, and/or absolute platelet count.
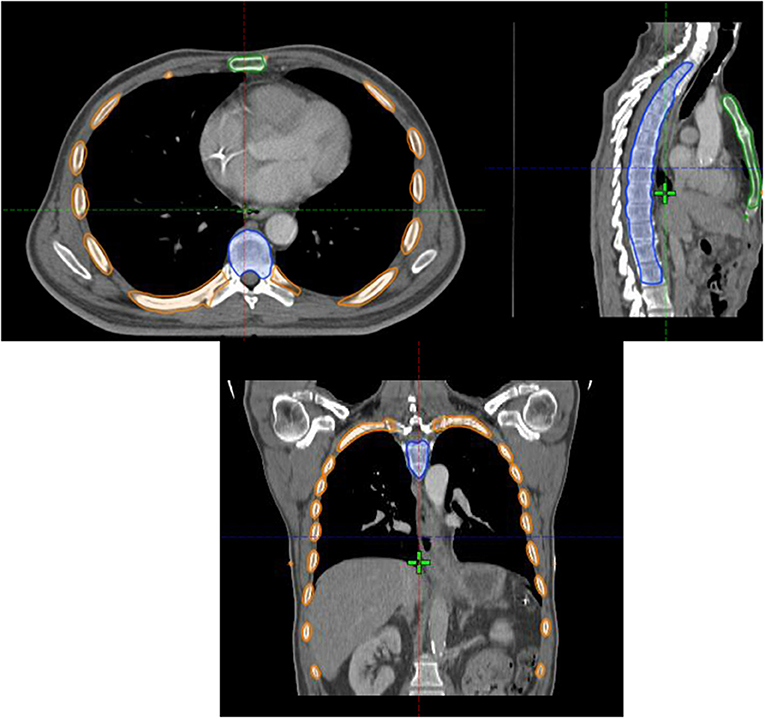
Figure 1. Axial (top left), sagittal (top right) and coronal (bottom) images of the thoracic marrow structure comprised of the sternum (green contour), ribs (orange contour), and vertebral bodies from T1-L1 (blue contour).
Statistics
Normal tissue complication probability (NTCP) was evaluated with a simplified Lyman-Kutcher-Burman (LKB) model for HT3+ using the TM as the organ-at-risk (OAR), as previously described (7, 10, 11). Maximum likelihood estimations (MLE) were used to determine optimal values for the three parameters defined by the LKB model; specifically, n, the volume parameter; m, the slope parameter; and TD50, the uniform OAR dose which results in a 50% complication risk.
Differences in baseline dosimetric parameters between the IMRT and 3DRT groups were evaluated by the Wilcoxon test. Univariate and multivariate analyses were performed using logistic regression to determine the correlation of TM V5-V50 and TM mean dose with HT3+. This was done for each of the 3 sub-sites as well. All clinical and dosimetric parameters with p ≤ 0.10 on univariate analysis were included in the multivariate model, with each dosimetric parameter being evaluated independently. Receiver operating characteristic (ROC) analysis was used to determine the optimal cut-point for dosimetric parameters. MATLAB version 9.0 (The MathWorks, Natick MA) and R version 3.3.1 (R Foundation for Statistical Computing, Vienna) optimization routines were used to maximize likelihood NTCP parameters. All other statistical analyses were performed using SAS version 9.4 (SAS Institute, Cary, NC).
Results
Summary of Patient Characteristics and TM Dosimetric Parameters
Patient, tumor, and radiation treatment characteristics are described in Table 1. The cohort included 137 patients that were mostly male (84%), median age 61 years (Interquartile range [IQR], 55–67), and predominantly with clinical stage II (34%) or stage III (54%) disease. Over 80% of tumors were located in the lower thoracic (N = 66) or gastroesophageal junction (N = 49). Most patients received concurrent carboplatin/paclitaxel (N = 83) while the rest received platinum/5FU (N = 28), irinotecan/platinum (N = 22), or 5FU alone (N = 4). A total of 43 (32%) patients received induction chemotherapy. Median RT dose was 50.4 Gy (IQR = 50.4–50.4 Gy), and the majority of patients were treated with 3DCRT (63.5%). Most patients underwent surgery after neoadjuvant CRT (60.6%).
Summary statistics for the TM mean dose and TM V5-V50 are summarized in Table 2. The TM mean dose had a median value of 13.0 Gy (IQR, 11.1 Gy−15.3 Gy). Patients treated with IMRT (N = 50) had significantly higher TM V5 (60.5% vs. 52.4%, p = 0.001) and TM V10 (46.0% vs. 39.7%, p = 0.001) compared to patients treated with 3DRT. Based on doses to the sub-sites, these differences in V5 and V10 between IMRT and 3DRT patients seem to be driven by dose to the ribs, as shown in Table 2. The TM V30 had a trend toward lower values in the IMRT vs. 3DRT patients (13.4% vs. 17.8%, p = 0.07). There were no other significant differences in TM dosimetric parameters between IMRT and 3DRT patients.
Clinical Hematologic Toxicities
Overall grade 3+ hematologic toxicity was 39.4% and overall grade 2+ toxicity was 73.0% (Table 3). The median time to grade 3+ toxicity was 28 days (IQR = 21–32 days). Incidence of Grade 3+ toxicity varied by systemic therapy regimen and was 48.2% (40 of 83 patients), 40.9% (9 of 22 patients), and 17.9% (5 of 28 patients) for those who were treated with carboplatin/paclitaxel, irinotecan-based chemotherapy, and platinum/5FU-based chemotherapy, respectively.
In total, 10 patients had chemotherapy dose reduction [1 for neutropenic fever, 1 for nausea requiring percutaneous endoscopic gastrostomy tube (PEG) placement, 1 for low ANC, and 1 for thrush, mucositis, nausea/vomiting, and weight loss, 1 for nausea, 1 for mucositis, 1 for poor performance status at start of treatment, 2 for age, 1 for diarrhea]. Also, 20 patients were hospitalized during treatment for various reasons including: dehydration, dysphagia, failure to thrive, nausea, vomiting, pneumonia, fever, and neutropenia. Chemotherapy interruption due to hematologic toxicity was seen in 17 patients whereas chemotherapy discontinuation due to hematologic toxicity was required in 4 patients.
LKB NTCP Modeling
Constrained optimization (0 < n ≤ 1, with m and TD50 unrestricted) of the LKB model for HT3+ for the entire cohort resulted in MLE values of n = 0.70, m = 0.67, and TD50 = 20.1 Gy (Figure 2A). In terms of the sub-sites, the resulting values of n, m, and TD50 were 0.09, 0.24, and 46.3 Gy for the VB; 0.60, 0.56, and 14.4 Gy for the ribs; and 2, 1.81, and 24.5 Gy for the sternum.
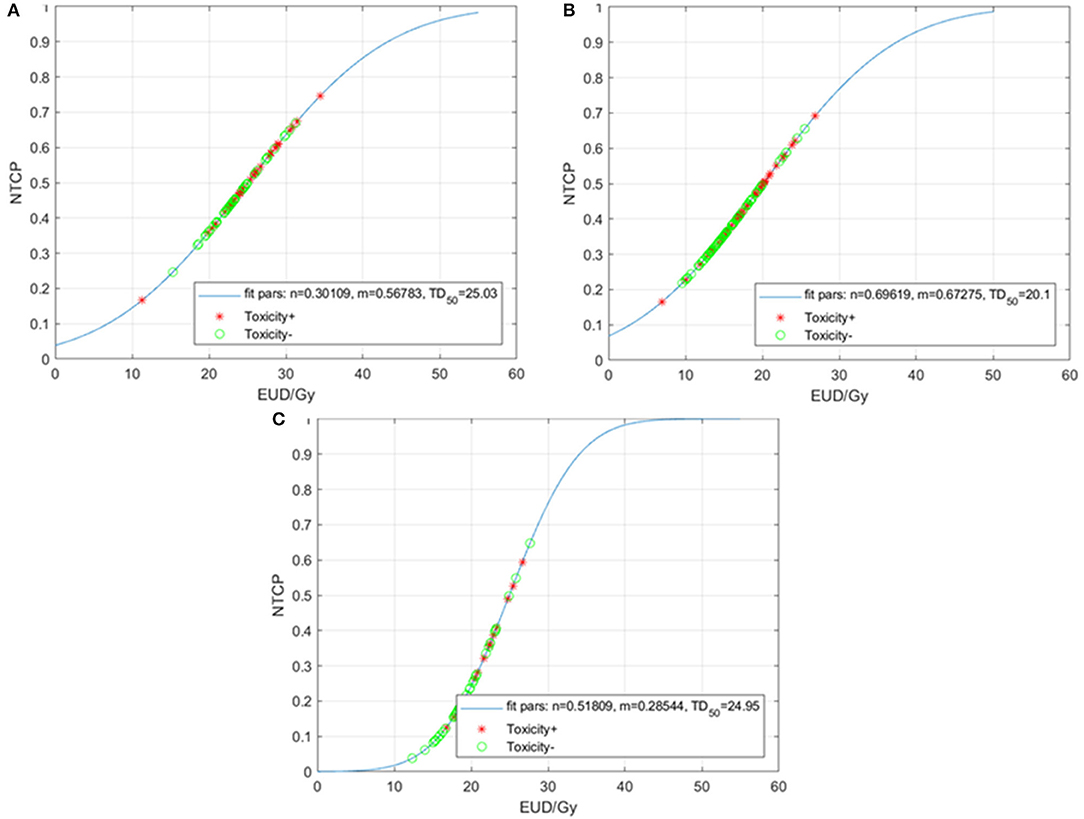
Figure 2. Normal tissue complication probability (NTCP) for acute grade ≥3 hematologic toxicities (HT3+) per the Lyman-Kutcher Burman model for all patients (A), patients treated with carboplatin/paclitaxel (B), and those treated with other systemic therapy regimens (C). Patients that experienced HT3+ during chemoradiation are represented by red asterisks; patients without HT3+ are represented by green circles. EUD, equivalent uniform dose.
Given the heterogeneity of the chemotherapy regimens, we performed separate analyses for the patients treated with carboplatin/paclitaxel (N = 83) and for those treated with other regimens (N = 54). The LKB model for HT3+ in the carboplatin/paclitaxel group generated MLE values of n = 0.30, m = 0.57, and TD50 = 25.0 Gy (Figure 2B) compared to n = 0.51, m = 0.28, and TD50 = 25.0 Gy (Figure 2C).
Last, we performed a further exploratory analysis where n was fixed at the value n = 1, as this forces the model to treat the TM as a parallel (rather than serial) OAR. Figure 3 summarizes the results. For the entire cohort, the resulting MLE values are m = 0.80, and TD50 = 17.3 Gy (Figure 3A). When separated by type of chemotherapy, the m and TD50 values are 1.12 and 14.0 Gy for the carboplatin/paclitaxel group (Figure 3B) compared to 0.42 and 19.6 Gy for patients treated with other regimens (Figure 3C).
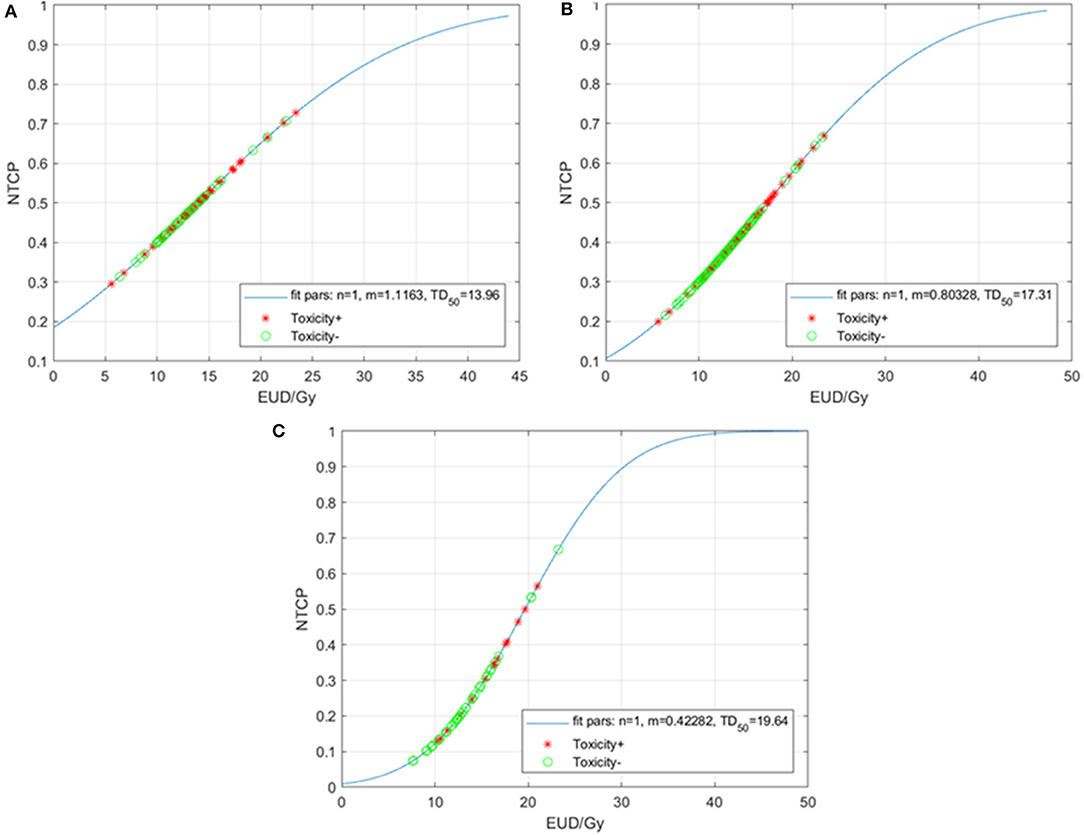
Figure 3. Normal tissue complication probability (NTCP) for acute grade ≥3 hematologic toxicities (HT3+) per the Lyman-Kutcher Burman model with n fixed (n = 1) for all patients (A), patients treated with carboplatin/paclitaxel (B), and those treated with other systemic therapy regimens (C). Patients that experienced HT3+ during chemoradiation are represented by red asterisks; patients without HT3+ are represented by green circles. EUD, equivalent uniform dose.
Predictors of Hematologic Toxicities: Total Marrow
On univariate analysis, patient age (OR = 1.04, p = 0.028), male gender (OR = 0.38, p = 0.044), and systemic therapy regimen (carboplatin/paclitaxel vs. other, OR = 2.66, p = 0.010) were factors significantly associated with the odds of HT3+, while body mass index (BMI), IMRT (vs. 3DRT), and use of induction chemotherapy were not (Table 4). In terms of dosimetric predictors, increasing TM mean dose (per Gy) was associated with significantly higher rates of HT3+ (OR = 1.13, 95% CI 1.02–1.26, p = 0.021). In addition, each 5% increase in TM-V30 was associated with higher rates of HT3+ (OR = 1.31, 95% CI 1.05–1.65, p = 0.019) with similar results seen for TM-V50 (OR = 3.10, 95% CI 1.13–9.12, p = 0.032). The other TM dosimetric parameters (V5, V10, V20, and V40) were borderline (p < 0.10) associated with higher rates of HT3+.
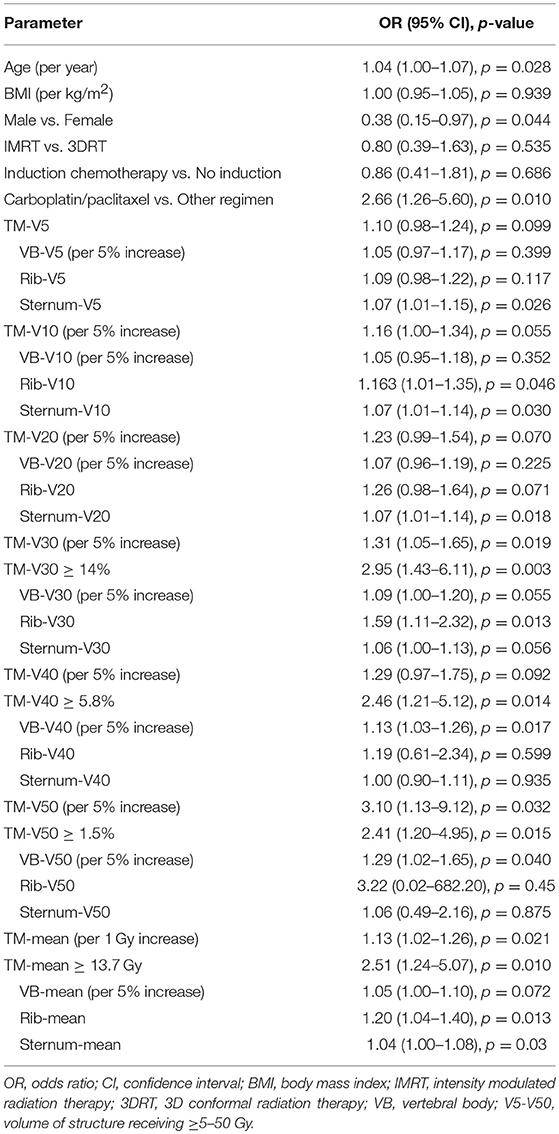
Table 4. Univariate logistic regression analysis for factors associated with grade ≥ 3 hematologic toxicity in the entire cohort.
We performed multivariate analyses that included age, gender, and type of systemic therapy regimen along with either TM-mean (model 1), TM-V5 (model 2), TM-V10 (model 3), TM-V20 (model 4), TM-V30 (model 5), TM-V40 (model 6), and TM-V50 (model 7) with results summarized in Table 5. With the exception of TM-V5 and TM-V10, in all models, the adjusted ORs for the dosimetric variable, age, and systemic therapy were significantly associated with HT3+ while the OR for gender was not. For example, in the model that includes TM-V30, the adjusted ORs for TM-V30, systemic therapy regimen (carboplatin/paclitaxel vs. other), age, and gender (male vs. female) were: 1.50 (1.16–1.99, p = 0.003), 4.05 (1.76–9.99, p = 0.002), 1.04 (1.01–1.08, p = 0.028), and 0.49 (0.17–1.37, p = 0.175).
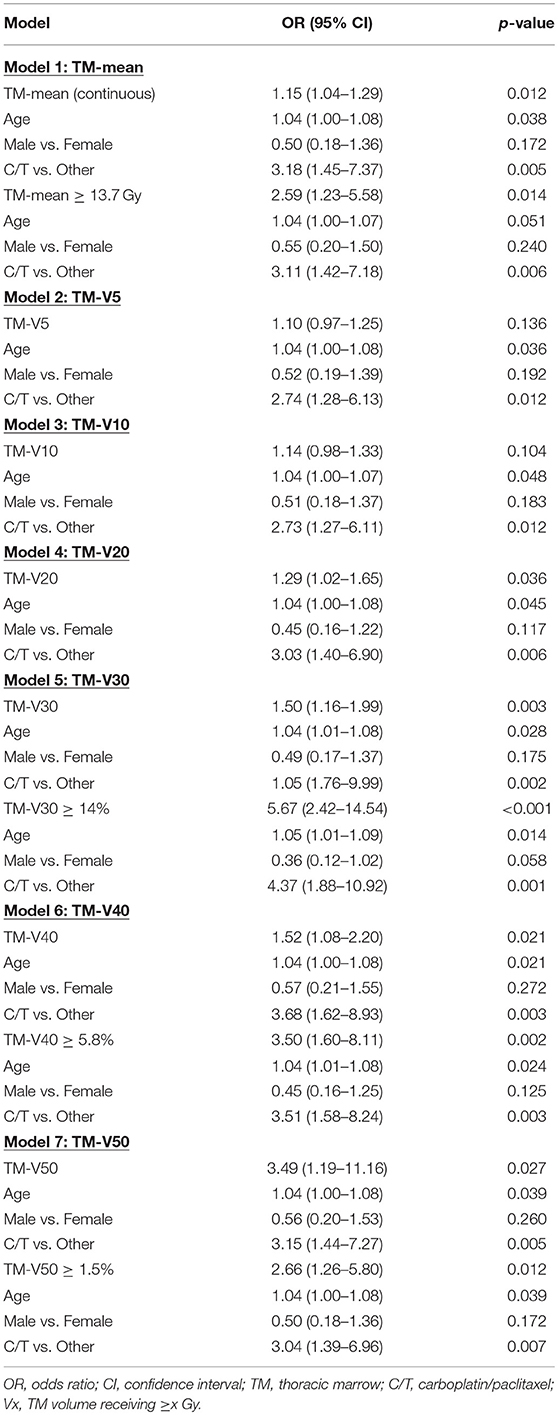
Table 5. Multivariate logistic regression analysis for factors associated with grade ≥ 3 hematologic toxicity in the entire cohort.
Given the heterogeneity in systemic therapy regimens, we performed an analysis in the patients that received carboplatin/paclitaxel, which represents the largest group (N = 83). Table 6 shows that the only variables significantly associated with HT3+ on univariate analysis were age (OR = 1.05, 95% CI 1.01–1.10, p = 0.014) and TM-V50 (OR = 4.78, 95% CI 1.16–23.50, p = 0.039) while TM-V30 was borderline associated with HT3+ (p = 0.066). On multivariate analysis, that included age and the dosimetric parameter, Table 7 shows that the only dosimetric variable significantly associated with HT3+ was TM-V50 (OR = 4.78, 95% CI 1.08–25.61, p = 0.049).
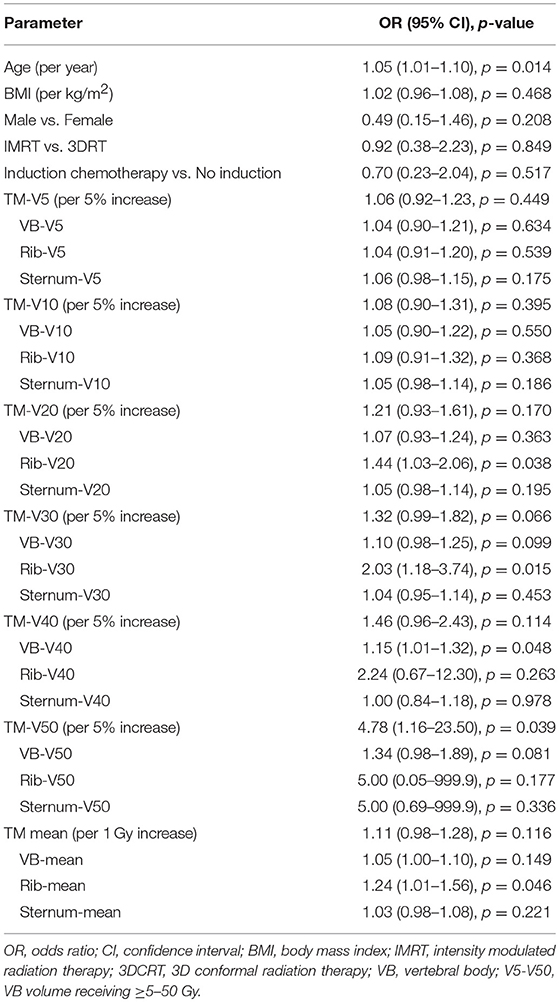
Table 6. Univariate logistic regression analysis for factors associated with grade ≥ 3 hematologic toxicity in patients treated with carboplatin/paclitaxel (N = 83).
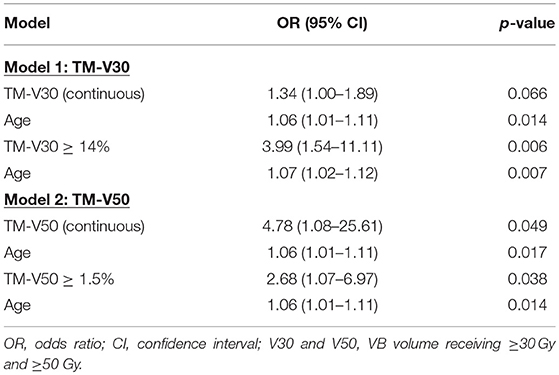
Table 7. Multivariate logistic regression analysis for factors associated with grade ≥ 3 hematologic toxicity in patients treated with carboplatin/paclitaxel (N = 83).
We further set out to identify the optimal cut-points for the dosimetric variables most strongly associated with HT3+ (TM-mean, TM-V30, TM-V40, and TM-V50) using ROC analysis. Based on the ROC analysis, the optimal cut-points and area under the curve (AUC) for these parameters were 13.7 Gy with AUC = 0.631 (TM-mean), 14% with AUC = 0.629 (TM-V30), 5.8% with AUC = 0.600 (TM-V40), and 1.5% with AUC = 0.628 (TM-V50) (Figure 4). Tables 5, 7 summarize the univariate and multivariate results of these parameters using the respective cut-points in the entire cohort and the carboplatin/paclitaxel cohort. For example, patients with TM-V50 ≥ 1.5% had a 2.66-fold increase (95% CI 1.26–5.80, p = 0.012) in the odds of HT3+ for the entire cohort and a 2.68-fold increase (95% CI 1.07–6.97, p = 0.038) in the carboplatin/paclitaxel cohort after adjusting for confounders.
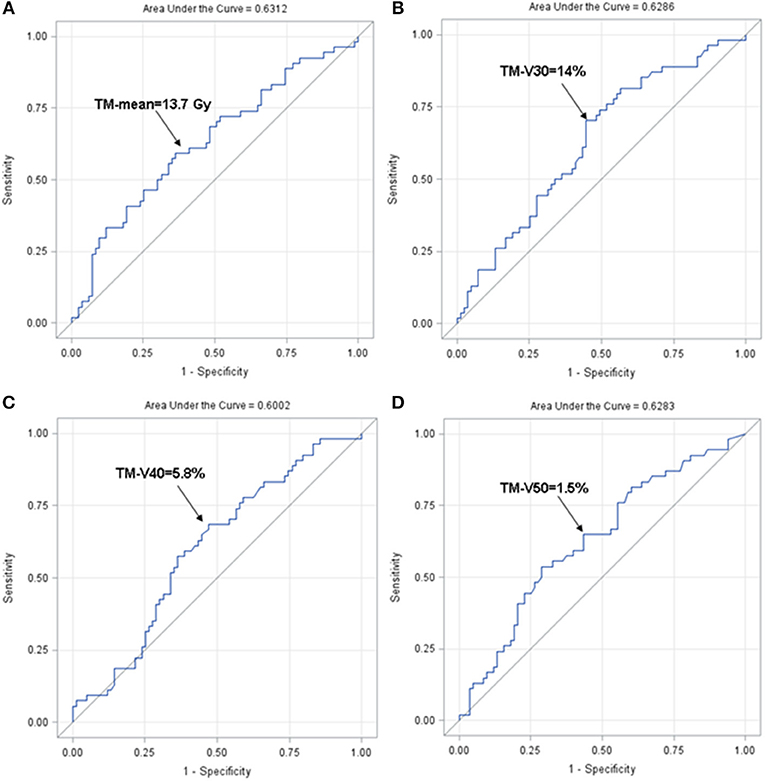
Figure 4. Receiver operating characteristics curve for TM-mean (A), TM-V30 (B), TM-V40 (C), and TM-V50 (D). ROC, receiver operating characteristic.
Predictors of Hematologic Toxicities: Total Marrow Sub-Sites
Next, we performed univariate analyses of each TM sub-site in both the entire cohort and carboplatin/paclitaxel cohorts with results summarized in Tables 4, 6. While several sternum dosimetric parameters were associated with HT3+ in the entire cohort on univariate analysis (Table 4), none were associated or borderline-associated with HT3+ in the carboplatin/paclitaxel cohort (Table 6). Therefore, we focus the rest of this section on the VB and ribs dosimetric parameters.
On multivariate analysis, the VB-mean dose (OR = 1.06, p = 0.032), VB-V30 (OR = 1.15, p = 0.010), VB-V40 (OR = 1.19, p = 0.002) and VB-V50 (OR = 1.32, p = 0.013) were associated with higher rates of HT3+ in the entire cohort (Table 8) while only VB-V40 (OR = 1.16, p = 0.044) remained significant in the carboplatin/paclitaxel cohort (Table 9). The optimal cut-point for VB-V40 was 29% resulting in odds-ratios of 3.58 (95% CI 1.60–8.04, p = 0.002) and 3.55 (95% CI 1.36–9.26, p = 0.010) for patients with VB-V40 ≥ 29% vs. VB-V40 < 29% in the entire cohort and carboplatin/paclitaxel cohorts, respectively.
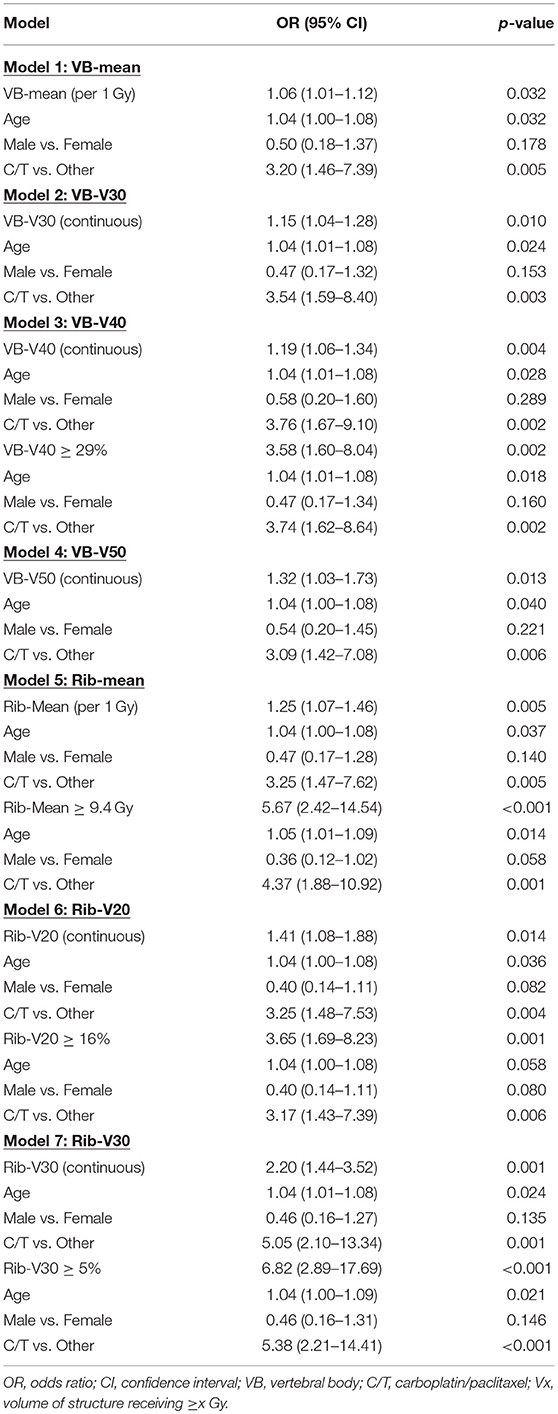
Table 8. Multivariate logistic regression analysis of factors associated with grade ≥ 3 hematologic toxicities using the VB and rib sub-sites only in the entire cohort.
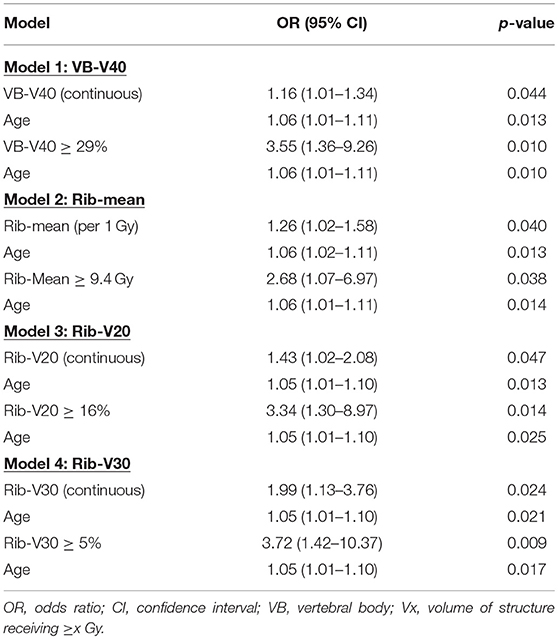
Table 9. Multivariate logistic regression analysis of factors associated with grade ≥ 3 hematologic toxicities using the VB and rib sub-sites only in the carboplatin/paclitaxel cohort.
Similarly, multivariate analysis revealed that rib-mean (OR = 1.25, p = 0.005), rib-V20 (OR = 1.41, p = 0.014), and rib-V30 (OR = 2.20, p = 0.001) were significantly associated with HT3+ in the entire cohort (Table 8). Those 3 variables (rib-mean, rib-V20, and rib-V30) remained significantly associated with HT3+ in the carboplatin/taxol cohort (Table 9).
The rib-mean dose (OR = 1.20, p = 0.013), rib-V10 (OR = 1.16, p = 0.046), and rib-V30 (OR = 1.59, p = 0.013) were associated with higher rates of HT3+ in the entire cohort while rib-V20 (OR = 1.26, p = 0.071) was borderline associated with HT3+. In the carboplatin/paclitaxel cohort, rib-mean (OR = 1.24, p = 0.046), rib-V20 (OR = 1.43, p = 0.038), and rib-V30 (OR = 2.03, p = 0.015) were significantly associated with HT3+. The optimal cut-points for rib-mean, rib-V20, and rib-V30 were 9.4 Gy, 16 and 5%, respectively.
We performed an exploratory direct comparison of the significant VB and rib parameters. Table 10 demonstrates the multivariate analyses incorporating the optimal cutpoints for VB-V40 and rib-mean, rib-V20, or rib-V30 for the entire cohort and the carboplatin/paclitaxel cohort. For the entire cohort, the rib dosimetric parameter slightly out-performed (smaller p-values) the VB-V40 while the VB-V40 generally outperformed the rib parameters in the carboplatin/taxol cohort.
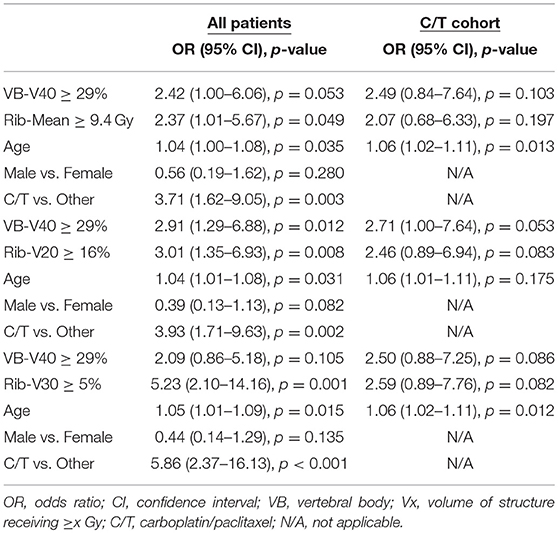
Table 10. Multivariate analyses including the VB and rib dosimetric parameters in the entire cohort and carboplatin/paclitaxel cohorts.
Discussion
In this study, we found that increasing TM radiation dose is associated with the development of acute HT3+ in esophageal cancer patients treated with CRT. In our NTCP analysis for the entire cohort, we found that the n value in the LKB model was close but not exactly equal to 1 (n = 0.70) and in the carboplatin/paclitaxel cohort, our results demonstrated that n = 0.30. These findings imply that the TM structure is not exactly a parallel organ in the way we defined it for this study. Nonetheless, TM-mean dose as well as many of the other dosimetric parameters had a strong association with the development of HT3+.
The observation that the LKB model resulted in a value of n = 0.70 for the entire cohort and n = 0.30 for the carboplatin/paclitaxel cohort was unexpected. A previous study of patients receiving thoracic CRT for lung cancer used the thoracic VBs as a surrogate for bone marrow found that the n = 1 when the LKB model was applied to the data set (7). In that study, VB was defined as the T1-T10 vertebral bodies, which was slightly different than the VB definition in this study, where we included T1-L1 (given that distal esophageal and GE junction tumors would result in more radiation exposure to the lower thoracic vertebrae, as well as L1). We did see that incorporating the ribs and sternum into the structure TM increased the n to closer to 1. In addition, previous studies of pelvic bone marrow (lumbosacral spine beginning from the top of L5, the ilium, and the low pelvic bones) in patients receiving CRT for anal/gynecologic malignancies have also found a value of n = 1 on LKB modeling (10, 12, 13). A value of n = 1 makes physiologic sense as the interpretation is that the marrow is composed of functional subunits, similar to organs such as the lung and liver, and that it is the mean radiation dose to the marrow that is the driver of toxicity. In our study, particularly in the more uniform group of patients treated with carboplatin/paclitaxel, the mean TM dose was not strongly associated with development of HT3+, which at least is consistent with the LKB model, in which n = 0.30. The reason for the discrepant n value we found could be due to a multitude of factors including an insufficient sample size to detect the mean dose as a significant predictor of HT3+, an incorrect definition of the TM structure, and the variability in chemotherapy regimens. We tried to account for some of these factors with subgroup analyses (e.g., the carboplatin/paclitaxel group only), but ultimately, validation of these results in a separate and ideally prospectively followed cohort would be needed.
Another interesting component of the NTCP modeling occurred when the n value was defined as 1, and optimization of the LKB model was done for only the TD50 and m values. Here we see the impact of chemotherapy on the LKB model. In patients treated with carboplatin/paclitaxel, the TD50 = 14.0 Gy and the slope parameter m = 1.16, which results in a shallow, slowly rising NTCP curve. For patients treated with other chemotherapy regimens, TD50 = 19.6 Gy and m = 0.42 (steep, faster rise). Consistent with the results of the LKB models, significant differences in acute HT3+ were seen in patients depending on the concurrent chemotherapy regimen. On multivariate analysis, treatment with carboplatin/paclitaxel, the most common regimen at our institution, was the variable most strongly associated with development of HT3+. These findings lead to the hypothesis that it may be more important to keep radiation dose to the TM at a minimum for patients treated with carboplatin/paclitaxel as opposed to other regimens.
To date, only one other study has examined the impact of radiation dose to bony structures in the thorax in patients receiving CRT for EC (9). This study included 41 patients all treated with cisplatin/5FU and IMRT to a dose of 41.2–43.2 Gy to a large field with a simultaneous integrated boost to the gross disease to 46–48 Gy. The VB was defined as T1-T12 and radiation to the ribs, sternum, scapula and clavicle were also assessed. The endpoints analyzed included grade ≥ 3 WBC or grade ≥ 2 ANC. The authors found that higher radiation dose to the VB (mean dose and V5-V30) and rib (mean dose and V5-V30) were associated with higher rates of grade 3 WBC toxicity, and that the VB dose had the strongest association with hematologic toxicity. The study suggested constraining the VB mean dose <35.9 Gy, V20 < 70%, and V10 < 77% for patients receiving concurrent cisplatin/5FU. Irradiation of the VBs has also been studied in the setting of lung cancer. Barney et al. suggested constraining the VB to mean dose ≤23 Gy, V5 ≤ 65%, V10 ≤ 60%, and V20 ≤ 50% may decrease acute HT in patients receiving CRT for NSCLC (7). Deek et al. also noted the relationship between VB radiation and leukopenia, recommending a mean VB doses of ≤ 23.9 Gy, V20 ≤ 56.0%, and V30 ≤ 52.1% (8). While the radiation therapy techniques and doses, chemotherapy regimens, and endpoints used in these studies vary from each other and the current study, the important message is that radiation therapy to the bony marrow in the thorax (particularly the VB and ribs) contributes to HT.
Contouring the TM in the thorax is tedious. There are significant heterogeneities in Hounsfield Units in the cortex and the marrow of bony structures, which poses challenges for auto-contouring features in treatment planning software. This is particularly true for each individual rib as it courses from the costovertebral junction to the costo-sternal junction. Thus, we were interested in analyzing whether any of the other sub-sites (especially the sternum or VB) alone could serve as a surrogate for the TM as a dose-limiting structure in an effort to reduce hematologic toxicities. While none of the sternum dosimetric parameters were associated with HT3+ in the carboplatin/paclitaxel cohort, there were VB dosimetric parameters associated with HT3+ in this group as well as the entire cohort, particularly VB-V40. Of course, the rib mean dose, rib-V20 and rib-V40 were also associated with HT3+. In direct comparison of VB-V40 and rib dosimetric parameters, the rib parameters appeared to be slightly more important on multivariate analysis in the entire cohort, but not in the more uniform cohort of patients that received carboplatin/paclitaxel. Given the significant amount of time it currently takes to contour the ribs, prospectively investigating whether limiting the VB-V40 (or other VB parameters) is appealing. If validated, the more pragmatic approach of contouring VBs would have a greater impact on saving time in busy, routine clinical practices.
This study is subject to numerous limitations. First and foremost, this is a retrospective analysis from a single institution and all of the results can be viewed as only hypothesis-generating. However, these data could serve as a solid starting point for a prospective study, as a secondary analysis of currently ongoing or recently completed cooperative group clinical trials involving chemoradiation in esophageal cancer patients, or as baseline data to be externally validated in an independent data set. In addition, our definition of one of the TM sub-sites, the VB structure (T1-L1) is different from that of Lee et al. (T1-T12) which is also different from the lung cancer studies (T1-T10). We considered these differences at the study outset but felt strongly that the VB structure should include L1 due to the frequent radiation exposure of L1 in patients receiving CRT for lower thoracic or gastroesophageal junction tumors, which comprised 84% of our patient population. Ideally, the structure-at-risk should be defined as the entire bone marrow for each patient, but this approach is neither practical nor possible in routine practice. Lastly, the bony structures contoured on CT imaging are used as a surrogate for active bone marrow, but associations between radiation dose to the TM and HT3+ may be stronger if functional imaging techniques were used to define active bone marrow as has been done in pelvic malignancies (14–17).
Nonetheless, these data provide a starting point for future prospective evaluation. Currently, we have a single arm phase II trial under development for esophageal cancer patients undergoing chemoradiation with IMRT and weekly carboplatin/paclitaxel. We will assess the feasibility of constraining the VB-V40 < 29% while not affecting our lung or heart dosimetric parameters. The study will be powered to reduce the estimated HT3+ toxicity rate from 50% to <35%. If this trial meets its primary endpoint, then a randomized trial of IMRT vs. 3DCRT in this patient population is warranted.
In conclusion, we found that increasing TM radiation dose was associated with HT3+ in patients with esophageal cancer treated with CRT. Our results showed that radiation dose to sub-sites of the TM, particularly, the ribs and the VB, is also associated with HT3+. In particular, VB-V40 > 29% was associated with a >3.5-fold increased rate of HT3+ in all patients and in the group treated with carboplatin/paclitaxel. From a practical standpoint, limiting dose to the VB (as opposed to the TM or the ribs) may be sufficient to limit HT3+, but further prospective evaluation of these results, as described above, is needed.
Data Availability
The datasets generated for this study are available on request to the corresponding author.
Ethics Statement
This study was carried out in accordance with the recommendations of The Ohio State University Institutional Review Board. This was a retrospective study and is therefore exempt from obtaining written informed consent from subjects. The retrospective protocol was approved by the Institutional Review Board of The Ohio State University.
Author Contributions
DF and JB wrote the manuscript. DF, CB, and JA extracted the data and created the database. AA, JB, and DF performed the statistical analyses. All authors reviewed the data analysis, study conclusions, contributed to the manuscript revision, read, and approved the submitted version.
Conflict of Interest Statement
The authors declare that the research was conducted in the absence of any commercial or financial relationships that could be construed as a potential conflict of interest.
References
1. Siegel RL, Miller KD, Jemal A. Cancer statistics, 2018. CA Cancer J Clin. (2018) 68:7–30. doi: 10.3322/caac.21442
2. Cooper JS, Guo MD, Herskovic A, Macdonald JS, Martenson JA Jr, Al-Sarraf M, et al. Chemoradiotherapy of locally advanced esophageal cancer: long-term follow-up of a prospective randomized trial (RTOG 85-01). Radiation Therapy Oncology Group. JAMA. (1999) 281:1623–7. doi: 10.1001/jama.281.17.1623
3. van Hagen P, Hulshof MC, van Lanschot JJ, Steyerberg EW, van Berge Henegouwen MI, Wijnhoven BP, et al. Preoperative chemoradiotherapy for esophageal or junctional cancer. N Engl J Med. (2012) 366:2074–84. doi: 10.1056/NEJMoa1112088
4. Ohira M, Kubo N, Yamashita Y, Sakurai K, Toyokawa T, Tanaka H, et al. Impact of chemoradiation-induced myelosuppression on prognosis of patients with locally advanced esophageal cancer after chemoradiotherapy followed by esophagectomy. Anticancer Res. (2015) 35:4889–95.
5. Tepper J, Krasna MJ, Niedzwiecki D, Hollis D, Reed CE, Goldberg R, et al. Phase III trial of trimodality therapy with cisplatin, fluorouracil, radiotherapy, and surgery compared with surgery alone for esophageal cancer: CALGB 9781. J Clin Oncol. (2008) 26:1086–92. doi: 10.1200/JCO.2007.12.9593
6. Miyoshi N, Yano M, Takachi K, Kishi K, Noura S, Eguchi H, et al. Myelotoxicity of preoperative chemoradiotherapy is a significant determinant of poor prognosis in patients with T4 esophageal cancer. J Surg Oncol. (2009) 99:302–6. doi: 10.1002/jso.21235
7. Barney CL, Scoville N, Allan E, Ayan A, DiCostanzo D, Haglund KE, et al. Radiation dose to the thoracic vertebral bodies is associated with acute hematologic toxicities in patients receiving concurrent chemoradiation for lung cancer: results of a single-center retrospective analysis. Int J Radiat Oncol Biol Phys. (2018) 100:748–55. doi: 10.1016/j.ijrobp.2017.11.025
8. Deek MP, Benenati B, Kim S, Chen T, Ahmed I, Zou W, et al. Thoracic vertebral body irradiation contributes to acute hematologic toxicity during chemoradiation therapy for non-small cell lung cancer. Int J Radiat Oncol Biol Phys. (2016) 94:147–54. doi: 10.1016/j.ijrobp.2015.09.022
9. Lee J, Lin JB, Sun FJ, Lu KW, Lee CH, Chen YJ, et al. Dosimetric predictors of acute haematological toxicity in oesophageal cancer patients treated with neoadjuvant chemoradiotherapy. Br J Radiol. (2016) 89:20160350. doi: 10.1259/bjr.20160350
10. Bazan JG, Luxton G, Mok EC, Koong AC, Chang DT. Normal tissue complication probability modeling of acute hematologic toxicity in patients treated with intensity-modulated radiation therapy for squamous cell carcinoma of the anal canal. Int J Radiat Oncol Biol Phys. (2012) 84:700–6. doi: 10.1016/j.ijrobp.2011.12.072
11. Luxton G, Keall PJ, King CR. A new formula for normal tissue complication probability (NTCP) as a function of equivalent uniform dose (EUD). Phys Med Biol. (2008) 53:23–36. doi: 10.1088/0031-9155/53/1/002
12. Bazan JG, Luxton G, Kozak MM, Anderson EM, Hancock SL, Kapp DS, et al. Impact of chemotherapy on normal tissue complication probability models of acute hematologic toxicity in patients receiving pelvic intensity modulated radiation therapy. Int J Radiat Oncol Biol Phys. (2013) 87:983–91. doi: 10.1016/j.ijrobp.2013.09.017
13. Cheng JC, Bazan JG, Wu JK, Koong AC, Chang DT. Lumbosacral spine and marrow cavity modeling of acute hematologic toxicity in patients treated with intensity modulated radiation therapy for squamous cell carcinoma of the anal canal. Pract Radiat Oncol. (2014) 4:198–206. doi: 10.1016/j.prro.2013.07.011
14. Li N, Noticewala SS, Williamson CW, Shen H, Sirak I, Tarnawski R, et al. Feasibility of atlas-based active bone marrow sparing intensity modulated radiation therapy for cervical cancer. Radiother Oncol. (2017) 123:325–30. doi: 10.1016/j.radonc.2017.02.017
15. Liang Y, Bydder M, Yashar CM, Rose BS, Cornell M, Hoh CK, et al. Prospective study of functional bone marrow-sparing intensity modulated radiation therapy with concurrent chemotherapy for pelvic malignancies. Int J Radiat Oncol Biol Phys. (2013) 85:406–14. doi: 10.1016/j.ijrobp.2012.04.044
16. Mell LK, Kochanski JD, Roeske JC, Haslam JJ, Mehta N, Yamada SD, et al. Dosimetric predictors of acute hematologic toxicity in cervical cancer patients treated with concurrent cisplatin and intensity-modulated pelvic radiotherapy. Int J Radiat Oncol Biol Phys. (2006) 66:1356–65. doi: 10.1016/j.ijrobp.2006.03.018
17. Mell LK, Sirak I, Wei L, Tarnawski R, Mahantshetty U, Yashar CM, et al. Bone marrow-sparing intensity modulated radiation therapy with concurrent cisplatin for stage IB-IVA cervical cancer: an international multicenter phase II clinical trial (INTERTECC-2). Int J Radiat Oncol Biol Phys. (2017) 97:536–45. doi: 10.1016/j.ijrobp.2016.11.027
Keywords: hematologic toxicity, radiation dose, bone marrow, esophageal cancer, IMRT, 3DCRT, NTCP
Citation: Fabian D, Ayan A, DiCostanzo D, Barney CL, Aljabban J, Diaz DA, Miller ED, Wuthrick E, Williams TM and Bazan JG (2019) Increasing Radiation Dose to the Thoracic Marrow Is Associated With Acute Hematologic Toxicities in Patients Receiving Chemoradiation for Esophageal Cancer. Front. Oncol. 9:147. doi: 10.3389/fonc.2019.00147
Received: 19 October 2018; Accepted: 21 February 2019;
Published: 15 March 2019.
Edited by:
Youssef Zeidan, American University of Beirut, LebanonReviewed by:
Ima Paydar, Hospital of the University of Pennsylvania, United StatesMichael Chuong, Baptist Health South Florida, United States
Todd A. Aguilera, University of Texas Southwestern Medical Center, United States
Copyright © 2019 Fabian, Ayan, DiCostanzo, Barney, Aljabban, Diaz, Miller, Wuthrick, Williams and Bazan. This is an open-access article distributed under the terms of the Creative Commons Attribution License (CC BY). The use, distribution or reproduction in other forums is permitted, provided the original author(s) and the copyright owner(s) are credited and that the original publication in this journal is cited, in accordance with accepted academic practice. No use, distribution or reproduction is permitted which does not comply with these terms.
*Correspondence: Jose G. Bazan, jose.bazan2@osumc.edu