- Department of Pediatrics, Memorial Sloan Kettering Cancer Center, New York, NY, United States
Glypican 3 (GPC3) is a heparan sulfate proteoglycan and cell surface oncofetal protein which is highly expressed on a variety of pediatric solid embryonal tumors including the majority of hepatoblastomas, Wilms tumors, rhabdoid tumors, certain germ cell tumor subtypes, and a minority of rhabdomyosarcomas. Via both its core protein and heparan sulfate side chains, GPC3 activates the canonical Wnt/β-catenin pathway, which is frequently overexpressed in these malignancies. Loss of function mutations in GPC3 lead to Simpson-Golabi-Behmel Syndrome, an X-linked overgrowth condition with a predisposition to GPC3-expressing cancers including hepatoblastoma and Wilms tumor. There are several immunotherapeutic approaches to targeting GPC3, including vaccines, monoclonal antibodies, antibody-drug conjugates, bispecific antibodies, cytolytic T lymphocytes, and CAR T cells. These therapies offer a potentially novel means to target these pediatric solid embryonal tumors. A key pediatric-specific consideration of GPC3-targeted immunotherapeutics is that GPC3 can be physiologically expressed in normal tissues during the first year of life, particularly in the liver and kidney. In summary, this article reviews the current evidence for targeting childhood cancers with GPC3-directed immunotherapies.
Introduction
Glypican 3 (GPC3) is an oncofetal protein which is enriched on the surface of several pediatric solid embryonal tumors. This mini review evaluates the biological role of GPC3, synthesizes the published expression data in pediatric solid embryonal tumors, and describes the current immunotherapeutic approaches to target GPC3.
Biology
Glypicans are a highly conserved family of heparan sulfate proteolgycans which are attached to the plasma membrane via a C-terminal glycosyl-phosphatidylinositol (GPI) anchor (1, 2). These surface proteins interact with growth factors to influence morphogenesis and are predominantly expressed during development (1, 2). Six glypicans (numbered 1–6) have been identified in humans and broadly are subdivided into two groups with GPC1, GPC2, GPC4, and GPC6 are the orthologs of Dally whereas GPC3 and GPC5 are the orthologs of Dlp in Drosophila melanogaster (1).
GPC3 is located on Chromosome Xq26 and encodes GPC3, also known as DGSX, GTR2-2, MXR7, OCI-5, SDYS, SGB, SGBS, and SGBS1 (2–4). During development, GPC3 is expressed in the placenta, fetal liver, fetal lung, and fetal kidney although it is absent or only minimally expressed in most adult tissues (5). This physiologic change may be mediated by suppression from DNA methylation within the GPC3 promoter region (5–7).
GPC3 consists of an N-terminal domain that includes a secretory signal peptide as well as a GPI anchored C-terminal core protein containing two heparan sulfate chains (2–4). As with other glypicans, the GPC3 core protein and heparan sulfate side chains interact with a variety of regulatory proteins important in cell growth and differentiation, including Wnt, Hedgehog, and fibroblast growth factor (FGF) (8–12). In particular, GPC3 has been shown to interact with Wnts and binds directly to Frizzled, stimulating the formation of signaling complexes between these proteins which activates the canonical Wnt/β-catenin signaling pathway (8, 10). This signaling pathway is important for normal development of the kidney and liver, and is frequently aberrantly overexpressed in pediatric embryonal tumors (3, 8, 10, 13–17).
Simpson-Golabi-Behmel Syndrome (SGBS) is an X-linked overgrowth condition similar to the more common Beckwith-Wiedemann syndrome, and is associated with renal, hepatic, skeletal, and cardiac anomalies as well as predisposition to Wilms tumor, hepatoblastoma, and neuroblastoma (2, 18). SGBS is caused by constitutional microdeletions or truncating point mutations in GPC3 which are predicted to result in a loss of function (2, 7, 18–21). Loss of GPC3 binding to insulin like growth factor 2 (IGF-2) was originally understood to cause this overgrowth phenotype but a series of subsequent papers demonstrates that this instead due, at least in part, to hyperactivation of Hedgehog signaling (20–24).
Pediatric Tumors
Pediatric malignancies derived from tissues that express GPC3 during development, such as the liver or kidney, frequently demonstrate upregulation of GPC3 which is likely important to both malignant transformation and tumorigenesis in these childhood cancers. GPC3 drives cell growth and inhibits differentiation via alterations in Wnt/β-catenin, Hedgehog, and FGF signaling which are often aberrantly expressed in pediatric embryonal tumors. In addition, alternative pathways not involved in physiologic GPC3 function, such as the Yap-Hippo pathway as has been shown in adult liver tumors, may also contribute to GPC3-mediated pediatric tumor development (25, 26). Finally, GPC3 has been reported to increase expression of the multi-drug resistance associated protein and therefore GPC3 in tumors may contribute to chemoresistance and treatment failure (27–29).
It is not fully understood how these childhood cancers are able to re-induce GPC3 expression. A study of the GPC3 promoter methylation in primary pediatric embryonal tumors revealed gain of methylation mainly in boys with Wilms tumor and loss of methylation exclusively in girls with neuroblastoma (6). Increased tumor GPC3 expression was more commonly reported in a study of women than men with hepatocellular carcinoma (HCC), the most common adult liver tumor, although this has not been reproduced in subsequent studies (5). Thus, regulation of this X-linked gene may be not only age and tissue-specific but also gender-dependent and there are likely multiple means by which GPC3 becomes aberrantly deregulated in cancer. Nevertheless, across multiple studies, the extent of immunohistochemical (IHC) expression of GPC3 is relatively consistent for any given histology of embryonal tumor (Figure 1), each of which is to be reviewed in detail below.
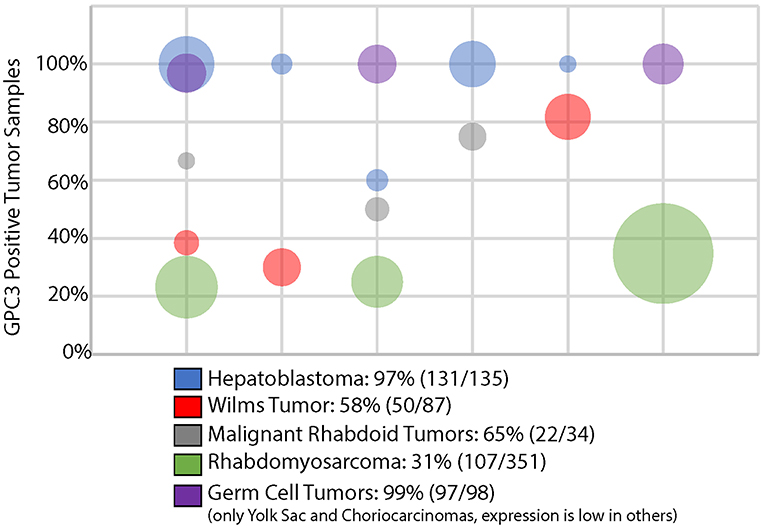
Figure 1. GPC3 immunohistochemistry in pediatric solid embryonal tumors. Bubble area is proportionate to the number of tumors evaluated in a particular study.
Hepatoblastoma
There are a variety of studies that demonstrate that GPC3 is nearly universally expressed on most hepatoblastomas although may be absent in less typical subtypes (e.g., teratoid) or portions of hepatoblastoma with mesenchymal differentiation (30–36). GPC3 was the second most highly transcriptionally overexpressed gene in a study of 48 hepatoblastoma tumors compared to normal liver (37). Although highly expressed, multiple studies have found that soluble GPC3 is an inferior serum biomarker of hepatoblastoma response compared with alpha fetoprotein, the current standard of care (37, 38). Combining the results from 5 studies evaluating GPC3 expression via IHC in hepatoblastoma found that 131/135 (97%) cases demonstrate GPC3 expression, as shown in Figure 1 (31–35).
Germ Cell Tumors
Several studies of extragonadal germ cell tumors demonstrate that yolk sac tumors and choriocarcionomas virtually always express GPC3 via IHC (Figure 1) (35, 39–41). In fact, GPC3 expression has been used to distinguish ovarian germ cell tumors from ovarian carcinomas (39). Other germ cell tumors, such as teratomas, embryonal carcinomas, and germinomas rarely express GPC3 (40, 41).
Wilms Tumors
Elevated transcriptional and proteomic expression of GPC3 is evident in a significant portion of Wilms tumors, as compared with adult kidney tumors and normal kidney tissue (36, 42, 43). Combining the results from 3 studies evaluating GPC3 expression in Wilms tumor revealed that 50/87 (58%) exhibit GPC3 expression, as shown in Figure 1 (34, 35, 43). In addition to constitutional mutations seen in patients with SGBS, somatic tumor mutations in GPC3 have even been identified in some cases of Wilms tumors (44).
Rhabdoid Tumors
A series of 3 studies of extracranial malignant rhabdoid tumors demonstrate that 22/34 (65%) of these rare and highly aggressive tumors express GPC3 (34, 45, 46). Interestingly, other extrarenal INI1 negative solid tumors except for undifferentiated sarcomas rarely express GPC3 (45). Given the challenging diagnostic overlap between some of these INI1 negative tumors, particularly extrarenal malignant rhabdoid tumor and epithelioid sarcoma, GPC3 may not only be a reasonable therapeutic target but may be helpful in improving diagnostic accuracy (45).
Rhabdomyosarcomas
A significant minority of rhabdomyosarcomas express GPC3, specifically 107/351 (31%) cases were positive in 3 different studies including both embryonal and alveolar subtypes (11, 34, 35, 47). Other pediatric sarcomas often do not express GPC3 (11, 47). Of all the glypicans, GPC3 exhibits the greatest homology with glypican 5 (GPC5), which is located on 13q32, a region of frequent genomic amplification in rhabdomyosarcomas and specifically associated with the PAX7-FOXO1 fusion (2, 11). Specifically in rhabdomyosarcomas, GPC5 has been specifically shown to potently activate Hedgehog signaling, which may be a result of its increased numbers of highly sulfated glycosaminoglan side chains compared with GPC3 (11, 48).
Neuroblastomas
There is mixed evidence regarding the role of GPC3 in neuroblastoma, with some studies showing increased expression in patients with 4S disease but most revealing absent expression of GPC3 in nearly all cases (34, 35, 42, 49, 50). The related glypican 2 (GPC2), however, has been shown to be an oncoprotein and immunotherapeutic target in high risk neuroblastoma (51, 52).
Treatments
The development of GPC3-directed targeted therapies was stimulated by research into HCC where GPC3 was not only present, but also noted to be a prognostic biomarker in adults (4, 5, 12, 53, 54). These therapies have included vaccines, monoclonal antibodies, antibody-drug conjugates, bispecific antibodies, cytolytic T lymphocytes (CTL), and chimeric antigen receptors, which are described in more detail below.
Vaccines
From 2007 to 2009, a nonrandomized, open-label, phase I clinical trial with dose escalation of an HLA-A*24:02–restricted GPC3298−306 peptide vaccine enrolled 33 Japanese adults with advanced HCC (UMIN 000001395) (12, 55). The vaccine elicited a GPC3-specific CTL response in 30 patients, notably with 1 partial response and 19 with stable disease 2 months after initiation of treatment (12, 55). Following this an open label, single arm, phase II study was performed in advanced HCC patients in Japan using the HLA-A*24:02–restricted GPC3298−306 or HLA-A2-restricted GPC3144−152 peptide vaccine (UMIN 000002614) (12, 56). Although this study did not reach its primary endpoint, the 1 and 2 year event free survival was lower for the patients who underwent surgery alone as compared with those who received surgery plus vaccination (12, 56). This was statistically significant in a subgroup analysis of patients with GPC3 positive HCC (12, 56). These HLA-A*24:02 HLA-A2 GPC3-directed peptide vaccines were also used to treat Japanese patients with chemoresistant ovarian clear cell carcinoma (OCCC) (UMIN 000003696) (12, 57). This vaccine elicited a GPC3-specific CTL response in 15 of 24 patients who had peripheral blood mononuclear cells collected 3 times or more and 3 patients demonstrated a partial response (12, 57). Finally, a pediatric phase I study using these GPC3 directed vaccines was conducted in Japan and was found to be safe with a 2 month disease control rate of 66% (UMIN 000006357) (12, 58). A GPC3-specific CTL response was identified in 39% of patients in this study, the majority of whom were in remission and were diagnosed with hepatoblastoma (12, 58). To date, this is the only completed GPC3-directed immunotherapeutic clinical trial in pediatrics, as shown in Table 1.
Monoclonal Antibodies
Codrituzumab (RO5137382; RG7686; GC33) is a recombinant humanized antibody targeting GPC3 which interacts with CD16/FcγRIIIa on natural killer (NK) cells to cause antibody-dependent cytotoxicity in a GPC3-dependent manner (59–63). This drug has been studied in a series of 4 clinical trials in adults with HCC. For the first-in-man study in the US, 20 patients with advanced HCC were enrolled on a dose-escalation study of codrituzumab and a maximum tolerated dose was not reached as there were no dose limiting toxicities up to the highest planned dose level of 20 mg/kg weekly (61). Time to progression was statistically significantly higher in those HCC patients with higher GPC3 expression (61). A subsequent Japanese phase I study in advanced HCC patients revealed that 7/13 (54%) patients had stable disease, 3 of whom had prolonged (>5 month) disease stabilization (JapicCTI-101255) (62). In a phase Ib study in combination with sorafenib (NCT00976170), codrituzumab was not found to provide clinical benefit although this study demonstrated that 124I radiolabeled codrituzumab was useful to monitor antibody uptake in the tumor and persistence of GPC3 expression after treatment (60). In a randomized placebo controlled phase II study (NCT01507168), codrituzumab similarly did not show a clinical benefit in advanced HCC patients, however combined elevation of tumor GPC3 and CD16/FcγRIIIa on NK cells correlated with survival (63–65). In HCC, expression of GPC3 has been shown to be a poor prognostic feature (66). Thus, even in these highest risk HCC patients with GPC3 expression, codrituzumab may provide clinical benefit, although monotherapy alone appears to be inadequate for HCC. Given the effectiveness of checkpoint inhibition with HCC, a combination of codrituzumab with the PD-L1 inhibitor atezolizumab is currently being evaluated in a Japanese phase I study of adult HCC patients (JapicCTI-163325). To date, codrituzumab is the only GPC3-directed immunotherapy to have a completed a clinical trial in the United States, as shown in Table 1.
The Ho Lab at the National Cancer Institute (Washington, DC, USA) has generated several additional GPC3-directed antibodies which have been extensively studied preclinically, including HN3, YP7, and HS20. HN3 is a GPC3-directed antibody that recognizes a cryptic Wnt binding domain and causes cell cycle arrest in HCC models via inactivation of Yap signaling (67). YP7 is another high affinity monoclonal antibody directed to the cell surface bound GPC3 and exhibited significant growth inhibition in HCC xenografts (68). HS20 is a human monoclonal antibody that recognizes the interaction site between the C-terminal GPC3 core fragment and heparan sulfate side chains in order to disrupt their interactions with Wnt (13, 69). This antibody was found to be an effective inhibitor of Wnt/β-catenin signaling in vitro, effectively inhibited HCC xenograft growth in vivo, and further was shown to impair cell migration and motility (13, 70).
Antibody-Drug Conjugates
Since GPC3 is efficiently internalized, it also is a good candidate for conjugation of antibodies to toxins (71). As a result, HN3 and YP7 were conjugated to the Pseudomonas endotoxin A and shown to be effective at reducing growth of xenografts in vivo, although notably the HN3-based drug conjugate, which is able to interfere with Wnt/β-catenin signaling, was more effective preclinically (71). There was significant in vivo toxicity so key immunogenic epitopes were removed from this antibody-drug conjugate, termed HN3-mPE24, in order to make it clinically viable (72).
Bispecific Antibodies
ERY974 is a bispecific antibody which targets both GPC3 (it was notably generated from codrituzumab) and CD3 and demonstrates in vivo antitumor efficacy against several GPC3 positive tumors (73). Intriguingly, ERY974 was effective even against tumors with nonimmunogenic features, by causing inflammation in the local tumor microenvironment (73). This is an important observation as even tumors which are not traditionally understood to be immunologically targetable on the basis of increased neoantigen expression could potentially be treated using this approach. More recently, Sano and colleagues presented results of a follow-up study which demonstrated synergy between ERY974 with Paclitaxel and Cisplatin (74). Given that Cisplatin is already an effective treatment modality for the majority of the GPC3 expressing pediatric solid embryonal tumors, this represents a promising opportunity for future combination studies. As shown in Table 1, an adult multicenter international phase I clinical trial of ERY974 is currently open in the United States and Europe (NCT02748837) and has planned expansion cohorts for stomach, esophageal, and other GPC3-expressing cancers.
Cytolytic T Lymphocytes
During the aforementioned peptide vaccination clinical trials, as well as a clinical study of HCC patients (UMIN 000005093), multiple peptide specific CTL clones were generated from peripheral blood and tumor tissue (12). These third party T cells are actively being developed for adoptive immune cell treatment of GPC3-positive tumors, as has been effectively utilized in the treatment of EBV associated post-transplantation lymphomas (12, 75, 76).
Chimeric Antigen Receptors
The Heczey Lab at Baylor College of Medicine (Houston, TX, United States) has generated several GPC3-targeted chimeric antigen receptor (CAR) constructs (77). Notably all of these GPC3/CARs rendered T cells highly cytotoxic to GPC3-positive HCC, hepatoblastoma, and malignant rhabdoid tumor cell lines in vitro as well as HCC and malignant rhabdoid tumors in vivo (77). The GPC3 directed CAR with the 4-1BB Zeta chain was the most effective at inducing T cell expansion and proliferation (77). As a result, two clinical trials are currently in development, GLYCAR T cells (NCT02905188) for adults with HCC and GAP T cells (NCT02932956) for children aged 1–21 with GPC3 positive liver tumors (Table 1).
Challenges
Although GPC3 is expressed in a wide variety of pediatric solid tumors, it is also expressed physiologically in infants, predominantly in the liver and kidney, with detectable serum levels during the first year of life (35). Thus, GPC3 targeted therapies could cause significant toxicity not seen in adults thus far due to persistent physiologic expression of GPC3 in the liver and kidney. If indeed clinical trials in pediatrics reveal immunogenic targeting of normal tissues, strategies to limit toxicity will need to be employed, such as limiting age to children >1 year of age as is being done in the GAP T cell study (NCT02932956). Given the generalized expression of GPC3 in the fetus and placenta, GPC3 based immunotherapies are likely to be teratogenic. Care must be made when counseling and treating women of childbearing age with GPC3-based immunotherapies. Finally, immunotherapies targeting these cancers need to be designed such that they preferntially target the core C-terminal GPC3 protein, its heparan sulfate side chains, or their interactome rather than the soluble N-terminal GPC3. In fact, soluble GPC3 expression may be useful as a biomarker of response to GPC3 therapies.
Conclusions
The heparan sulfate proteoglycan GPC3 is an attractive target for drug development as it is highly upregulated in HCC and several pediatric solid embryonal tumors and is responsible for driving key growth and developmental pathways which are currently not effectively targeted using our existing therapies (2, 12, 78). At this point, there is very limited clinical experience with GPC3-directed immunotherapeutics in pediatric oncology: A GPC3-directed vaccine study was conducted in Japan for children with solid tumors expressing GPC3 (UMIN 000006357) and in December 2018, the GAP CAR T cell study (NCT02932956) opened for children and young adults with GPC3-expressing liver tumors (12, 58). Vaccines, monoclonal antibodies, antibody-drug conjugates, bispecific antibodies, CTLs, and CAR T cell based therapies are all emerging treatment options which may provide enhanced ability to target GPC3 in pediatric solid embryonal tumors. As ongoing clinical trials in adults demonstrate which of these GPC3-based modalities are safe and beneficial, it is imperative that we rigorously evaluate the role of these potentially life-saving therapies in children and adolescents with GPC3-driven tumors.
Author Contributions
MO generated the initial draft of the manuscript. All authors reviewed the manuscript for content and accuracy and are responsible for its content.
Funding
This review was sponsored by Cannonball Kids' Cancer. MO is supported by the National Cancer Institute of the National Institutes of Health under Award Number K12CA184746. All authors are also supported by the MSK Cancer Center Support Grant/Core Grant P30 CA008748.
Conflict of Interest Statement
The authors declare that the research was conducted in the absence of any commercial or financial relationships that could be construed as a potential conflict of interest.
Abbreviations
CTL, Cytolytic T Lymphocytes; FGF, Fibroblast growth factor; GPC, Glypican; GPI, Glycosyl-phosphatidylinositol; HCC, Hepatocellular carcinoma; IGF, Insulin-like growth factor; IHC, Immunohistochemistry; NK, Natural killer; OCCC, Ovarian clear cell carcinomas; SGBS, Simpson-Golabi-Behmel Syndrome.
References
1. Iozzo RV, Schaefer L. Proteoglycan form and function: a comprehensive nomenclature of proteoglycans. Matrix Biol. (2015) 42:11–55. doi: 10.1016/j.matbio.2015.02.003
2. Filmus J. Glypicans in growth control and cancer. Glycobiology (2001) 11:19R–23R. doi: 10.1093/glycob/11.3.19R
3. Ho M, Kim H. Glypican-3: a new target for cancer immunotherapy. Eur J Cancer (2011) 47:333–8. doi: 10.1016/j.ejca.2010.10.024
4. Hoseini SS, Cheung NV. Immunotherapy of hepatocellular carcinoma using chimeric antigen receptors and bispecific antibodies. Cancer Lett. (2017) 399:44–52. doi: 10.1016/j.canlet.2017.04.013
5. Hsu HC, Cheng W, Lai PL. Cloning and expression of a developmentally regulated transcript MXR7 in hepatocellular carcinoma: biological significance and temporospatial distribution. Cancer Res. (1997) 57:5179–84.
6. Boily G, Saikali Z, Sinnett D. Methylation analysis of the glypican 3 gene in embryonal tumours. Br J Cancer (2004) 90:1606–11. doi: 10.1038/sj.bjc.6601716
7. Huber R, Hansen RS, Strazzullo M, Pengue G, Mazzarella R, D'Urso M, et al. DNA methylation in transcriptional repression of two differentially expressed X-linked genes, GPC3 and SYBL1. Proc Natl Acad Sci USA. (1999) 96:616–21.
8. Capurro MI, Xiang YY, Lobe C, Filmus J. Glypican-3 promotes the growth of hepatocellular carcinoma by stimulating canonical Wnt signaling. Cancer Res. (2005) 65:6245–54. doi: 10.1158/0008-5472.CAN-04-4244
9. Song HH, Shi W, Xiang YY, Filmus J. The loss of glypican-3 induces alterations in Wnt signaling. J Biol Chem. (2005) 280:2116–25. doi: 10.1074/jbc.M410090200
10. Capurro M, Martin T, Shi W, Filmus J. Glypican-3 binds to Frizzled and plays a direct role in the stimulation of canonical Wnt signaling. J Cell Sci. (2014) 127(Pt 7):1565–75. doi: 10.1242/jcs.140871
11. Cassinelli G, Zaffaroni N, Lanzi C. The heparanase/heparan sulfate proteoglycan axis: a potential new therapeutic target in sarcomas. Cancer Lett. (2016) 382:245–54. doi: 10.1016/j.canlet.2016.09.004
12. Shimizu Y, Suzuki T, Yoshikawa T, Tsuchiya N, Sawada Y, Endo I, et al. Cancer immunotherapy-targeted glypican-3 or neoantigens. Cancer Sci. (2018) 109:531–41. doi: 10.1111/cas.13485
13. Gao W, Kim H, Feng M, Phung Y, Xavier CP, Rubin JS, et al. Inactivation of Wnt signaling by a human antibody that recognizes the heparan sulfate chains of glypican-3 for liver cancer therapy. Hepatology (2014) 60:576–87. doi: 10.1002/hep.26996
14. Nejak-Bowen K, Monga SPS. Wnt/β-catenin signaling in hepatic organogenesis. Organogenesis (2008) 4:92–9. doi: 10.4161/org.4.2.5855
15. Behari J. The Wnt/beta-catenin signaling pathway in liver biology and disease. Exp Rev Gastroenterol Hepatol. (2010) 4:745–56. doi: 10.1586/egh.10.74
16. Pulkkinen K, Murugan S, Vainio S. Wnt signaling in kidney development and disease. Organogenesis (2008) 4:55–9. doi: 10.4161/org.4.2.5849
17. De Cat B, Muyldermans SY, Coomans C, Degeest G, Vanderschueren B, Creemers J, et al. Processing by proprotein convertases is required for glypican-3 modulation of cell survival, Wnt signaling, and gastrulation movements. J Cell Biol. (2003) 163:625–35. doi: 10.1083/jcb.200302152
18. Tenorio J, Arias P, Martínez-Glez V, Santos F, García-Miñaur S, Nevado J, et al. Simpson-Golabi-Behmel syndrome types I and II. Orphanet J Rare Dis. (2014) 9:138. doi: 10.1186/s13023-014-0138-0
19. Gonzalez AD, Kaya M, Shi W, Song H, Testa JR, Penn LZ, et al. OCI-5/GPC3, a glypican encoded by a gene that is mutated in the simpson-golabi-behmel overgrowth syndrome, induces apoptosis in a cell line–specific manner. J Cell Biol. (1998) 141:1407–14. doi: 10.1083/jcb.141.6.1407
20. Pilia G, Hughes-Benzie RM, MacKenzie A, Baybayan P, Chen EY, Huber R, et al. Mutations in GPC3, a glypican gene, cause the Simpson-Golabi-Behmel overgrowth syndrome. Nat Genet. (1996) 12:241. doi: 10.1038/ng0396-241
21. Vuillaume ML, Moizard MP, Rossignol S, Cottereau E, Vonwill S, Alessandri JL, et al. Mutation update for the GPC3 gene involved in Simpson-Golabi-Behmel syndrome and review of the literature. Hum Mutat. (2018) 39:790–805. doi: 10.1002/humu.23612
22. Capurro MI, Xu P, Shi W, Li F, Jia A, Filmus J. Glypican-3 inhibits Hedgehog signaling during development by competing with patched for Hedgehog binding. Dev Cell (2008) 14:700–11. doi: 10.1016/j.devcel.2008.03.006
23. Cano-Gauci DF, Song HH, Yang H, McKerlie C, Choo B, Shi W, et al. Glypican-3–deficient mice exhibit developmental overgrowth and some of the abnormalities typical of simpson-golabi-behmel syndrome. J Cell Biol. (1999) 146:255–64. doi: 10.1083/jcb.146.1.255
24. Cheng W, Tseng C-J, Lin TTC, Cheng I, Pan H-W, Hsu H-C, et al. Glypican-3-mediated oncogenesis involves the Insulin-like growth factor-signaling pathway. Carcinogenesis (2008) 29:1319–26. doi: 10.1093/carcin/bgn091
25. Miao HL, Pan ZJ, Lei CJ, Wen JY, Li MY, Liu ZK, et al. Knockdown of GPC3 inhibits the proliferation of Huh7 hepatocellular carcinoma cells through down-regulation of YAP. J Cell Biochem. (2013) 114:625–31. doi: 10.1002/jcb.24404
26. Reis H, Bertram S, Pott L, Canbay A, Gallinat A, Baba HA. Markers of hippo-pathway activity in tumor forming liver lesions. Pathol Oncol Res. (2017) 23:33–9. doi: 10.1007/s12253-016-0079-0
27. Lage H, Dietel M. Cloning and characterization of human cDNAs encoding a protein with high homology to rat intestinal development protein OCI-5. Gene (1997) 188:151–6. doi: 10.1016/S0378-1119(96)00689-0
28. Lage H, Dietel M, Froschle G, Reymann A. Expression of the novel mitoxantrone resistance associated gene MXR7 in colorectal malignancies. Int J Clin Pharmacol Ther. (1998) 36:58–60.
29. Wichert A, Stege A, Midorikawa Y, Holm PS, Lage H. Glypican-3 is involved in cellular protection against mitoxantrone in gastric carcinoma cells. Oncogene (2004) 23:945–55. doi: 10.1038/sj.onc.1207237
30. Woodfield SE, Shi Y, Patel RH, Jin J, Major A, Sarabia SF, et al. A novel cell line based orthotopic xenograft mouse model that recapitulates human hepatoblastoma. Sci Rep. (2017) 7:17751. doi: 10.1038/s41598-017-17665-8
31. Zhou S, Parham DM, Yung E, Pattengale P, Wang L. Quantification of glypican 3, beta-catenin and claudin-1 protein expression in hepatoblastoma and paediatric hepatocellular carcinoma by colour deconvolution. Histopathology (2015) 67:905–13. doi: 10.1111/his.12730
32. Zynger DL, Gupta A, Luan C, Chou PM, Yang GY, Yang XJ. Expression of glypican 3 in hepatoblastoma: an immunohistochemical study of 65 cases. Hum Pathol. (2008) 39:224–30. doi: 10.1016/j.humpath.2007.06.006
33. Yamauchi N, Watanabe A, Hishinuma M, Ohashi K-i, Midorikawa Y, Morishita Y, et al. The glypican 3 oncofetal protein is a promising diagnostic marker for hepatocellular carcinoma. Modern Pathol. (2005) 18:1591. doi: 10.1038/modpathol.3800436
34. Chan ES, Pawel BR, Corao DA, Venneti S, Russo P, Santi M, et al. Immunohistochemical expression of glypican-3 in pediatric tumors: an analysis of 414 cases. Pediatr Dev Pathol. (2013) 16:272–7. doi: 10.2350/12-06-1216-OA.1
35. Kinoshita Y, Tanaka S, Souzaki R, Miyoshi K, Kohashi K, Oda Y, et al. Glypican 3 expression in pediatric malignant solid tumors. Eur J Pediatr Surg. (2015) 25:138–44. doi: 10.1055/s-0034-1393961
36. Toretsky JA, Zitomersky NL, Eskenazi AE, Voigt RW, Strauch ED, Sun CC, et al. Glypican-3 expression in Wilms tumor and hepatoblastoma. J Pediatr Hematol Oncol. (2001) 23:496–9. doi: 10.1097/00043426-200111000-00006
37. Sumazin P, Chen Y, Trevino LR, Sarabia SF, Hampton OA, Patel K, et al. Genomic analysis of hepatoblastoma identifies distinct molecular and prognostic subgroups. Hepatology (2017) 65:104–21. doi: 10.1002/hep.28888
38. Zhou S, O'Gorman MRG, Yang F, Andresen K, Wang L. Glypican 3 as a serum marker for hepatoblastoma. Sci Rep. (2017) 7:45932. doi: 10.1038/srep45932
39. Esheba GE, Pate LL, Longacre TA. Oncofetal protein glypican-3 Distinguishes Yolk Sac tumor from clear cell carcinoma of the ovary. Am J Surg Pathol. (2008) 32:600–7. doi: 10.1097/PAS.0b013e31815a565a
40. Zynger DL, Everton MJ, Dimov ND, Chou PM, Yang XJ. Expression of glypican 3 in ovarian and extragonadal germ cell tumors. Am J Clin Pathol. (2008) 130:224–30. doi: 10.1309/8DN7DQRDFB4QNH3N
41. Zynger DL, Dimov ND, Luan C, Tean Teh B, Yang XJ. Glypican 3: a novel marker in testicular germ cell tumors. Am J Surg Pathol. (2006) 30:1570–5. doi: 10.1097/01.pas.0000213322.89670.48
42. Saikali Z, Sinnett D. Expression of glypican 3 (GPC3) in embryonal tumors. Int J Cancer (2000) 89:418–22. doi: 10.1002/1097-0215(20000920)89:5<418::AID-IJC4>3.0.CO;2-I
43. Tretiakova M, Zynger DL, Luan C, Andeen NK, Finn LS, Kocherginsky M, et al. Glypican 3 overexpression in primary and metastatic Wilms tumors. Virchows Archiv. (2015) 466:67–76. doi: 10.1007/s00428-014-1669-4
44. White GRM, Kelsey AM, Varley JM, Birch JM. Somatic glypican 3 (GPC3) mutations in Wilms' tumour. Br J Cancer (2002) 86:1920–2. doi: 10.1038/sj.bjc.6600417
45. Kohashi K, Nakatsura T, Kinoshita Y, Yamamoto H, Yamada Y, Tajiri T, et al. Glypican 3 expression in tumors with loss of SMARCB1/INI1 protein expression. Hum Pathol. (2013) 44:526–33. doi: 10.1016/j.humpath.2012.06.014
46. Venneti S, Le P, Martinez D, Xie SX, Sullivan LM, Rorke-Adams LB, et al. Malignant rhabdoid tumors express stem cell factors, which relate to the expression of EZH2 and Id proteins. Am J Surg Pathol. (2011) 35:1463–72. doi: 10.1097/PAS.0b013e318224d2cd
47. Thway K, Selfe J, Missiaglia E, Fisher C, Shipley J. Glypican-3 is expressed in rhabdomyosarcomas but not adult spindle cell and pleomorphic sarcomas. J Clin Pathol. (2011) 64:587–91. doi: 10.1136/jclinpath-2011-200071
48. Li F, Shi W, Capurro M, Filmus J. Glypican-5 stimulates rhabdomyosarcoma cell proliferation by activating Hedgehog signaling. J Cell Biol. (2011) 192:691–704. doi: 10.1083/jcb.201008087
49. Knelson EH, Gaviglio AL, Nee JC, Starr MD, Nixon AB, Marcus SG, et al. Stromal heparan sulfate differentiates neuroblasts to suppress neuroblastoma growth. J Clin Invest. (2014) 124:3016–31. doi: 10.1172/JCI74270
50. Al-Saraireh YM, Haddadin WJ, Alboaisa NS, Youssef AM, Alsbou MS, Al-Shuneigat JM, et al. Glypican-3 expression in primary and metastatic neuroblastoma. JJBS (2016) 9:261–67.
51. Bosse KR, Raman P, Zhu Z, Lane M, Martinez D, Heitzeneder S, et al. Identification of GPC2 as an oncoprotein and candidate immunotherapeutic target in high-risk neuroblastoma. Cancer Cell (2017) 32:295–309.e12. doi: 10.1016/j.ccell.2017.08.003
52. Li N, Fu H, Hewitt SM, Dimitrov DS, Ho M. Therapeutically targeting glypican-2 via single-domain antibody-based chimeric antigen receptors and immunotoxins in neuroblastoma. Proc Natl Acad Sci USA. (2017) 114:E6623–31. doi: 10.1073/pnas.1706055114
53. Nakatsura T, Yoshitake Y, Senju S, Monji M, Komori H, Motomura Y, et al. Glypican-3, overexpressed specifically in human hepatocellular carcinoma, is a novel tumor marker. Biochem Biophys Res Commun. (2003) 306:16–25. doi: 10.1016/S0006-291X(03)00908-2
54. Okabe H, Satoh S, Kato T, Kitahara O, Yanagawa R, Yamaoka Y, et al. Genome-wide analysis of gene expression in human hepatocellular carcinomas using cDNA microarray: identification of genes involved in viral carcinogenesis and tumor progression. Cancer Res. (2001) 61:2129–37.
55. Sawada Y, Yoshikawa T, Nobuoka D, Shirakawa H, Kuronuma T, Motomura Y, et al. Phase I trial of a glypican-3-derived peptide vaccine for advanced hepatocellular carcinoma: immunologic evidence and potential for improving overall survival. Clin Cancer Res. (2012) 18:3686–96. doi: 10.1158/1078-0432.CCR-11-3044
56. Sawada Y, Yoshikawa T, Ofuji K, Yoshimura M, Tsuchiya N, Takahashi M, et al. Phase II study of the GPC3-derived peptide vaccine as an adjuvant therapy for hepatocellular carcinoma patients. Oncoimmunology (2016) 5:e1129483. doi: 10.1080/2162402X.2015.1129483
57. Suzuki S, Sakata J, Utsumi F, Sekiya R, Kajiyama H, Shibata K, et al. Efficacy of glypican-3-derived peptide vaccine therapy on the survival of patients with refractory ovarian clear cell carcinoma. Oncoimmunology (2016) 5:e1238542. doi: 10.1080/2162402X.2016.1238542
58. Tsuchiya N, Hosono A, Yoshikawa T, Shoda K, Nosaka K, Shimomura M, et al. Phase I study of glypican-3-derived peptide vaccine therapy for patients with refractory pediatric solid tumors. Oncoimmunology (2018) 7:e1377872. doi: 10.1080/2162402X.2017.1377872
59. Ishiguro T, Sugimoto M, Kinoshita Y, Miyazaki Y, Nakano K, Tsunoda H, et al. Anti-glypican 3 antibody as a potential antitumor agent for human liver cancer. Cancer Res. (2008) 68:9832–8. doi: 10.1158/0008-5472.CAN-08-1973
60. Abou-Alfa GK, Yen CJ, Hsu CH, O'Donoghue J, Beylergil V, Ruan S, et al. Phase Ib study of codrituzumab in combination with sorafenib in patients with non-curable advanced hepatocellular carcinoma (HCC). Cancer Chemother Pharmacol. (2017) 79:421–9. doi: 10.1007/s00280-017-3241-9
61. Zhu AX, Gold PJ, El-Khoueiry AB, Abrams TA, Morikawa H, Ohishi N, et al. First-in-man phase I study of GC33, a novel recombinant humanized antibody against glypican-3, in patients with advanced hepatocellular carcinoma. Clin Cancer Res. (2013) 19:920–8. doi: 10.1158/1078-0432.CCR-12-2616
62. Ikeda M, Ohkawa S, Okusaka T, Mitsunaga S, Kobayashi S, Morizane C, et al. Japanese phase I study of GC33, a humanized antibody against glypican-3 for advanced hepatocellular carcinoma. Cancer Sci. (2014) 105:455–62. doi: 10.1111/cas.12368
63. Abou-Alfa GK, Puig O, Daniele B, Kudo M, Merle P, Park JW, et al. Randomized phase II placebo controlled study of codrituzumab in previously treated patients with advanced hepatocellular carcinoma. J Hepatol. (2016) 65:289–95. doi: 10.1016/j.jhep.2016.04.004
64. Chen G, Chen YC, Reis B, Belousov A, Jukofsky L, Rossin C, et al. Combining expression of GPC3 in tumors and CD16 on NK cells from peripheral blood to identify patients responding to codrituzumab. Oncotarget (2018) 9:10436–44. doi: 10.18632/oncotarget.23830
65. Nakamura M, Xu C, Diack C, Ohishi N, Lee RM, Iida S, et al. Time-to-event modelling of effect of codrituzumab on overall survival in patients with hepatocellular carcinoma. Br J Clin Pharmacol. (2018) 84:944–51. doi: 10.1111/bcp.13530
66. Zhang J, Zhang M, Ma H, Song X, He L, Ye X, et al. Overexpression of glypican-3 is a predictor of poor prognosis in hepatocellular carcinoma: an updated meta-analysis. Medicine (2018) 97:e11130. doi: 10.1097/MD.0000000000011130
67. Feng M, Gao W, Wang R, Chen W, Man YG, Figg WD, et al. Therapeutically targeting glypican-3 via a conformation-specific single-domain antibody in hepatocellular carcinoma. Proc Natl Acad Sci USA. (2013) 110:E1083–91. doi: 10.1073/pnas.1217868110
68. Phung Y, Gao W, Man YG, Nagata S, Ho M. High-affinity monoclonal antibodies to cell surface tumor antigen glypican-3 generated through a combination of peptide immunization and flow cytometry screening. mAbs (2012) 4:592–9. doi: 10.4161/mabs.20933
69. Wands JR, Kim M. WNT/beta-catenin signaling and hepatocellular carcinoma. Hepatology (2014) 60:452–4. doi: 10.1002/hep.27081
70. Gao W, Kim H, Ho M. Human monoclonal antibody targeting the heparan sulfate chains of glypican-3 inhibits HGF-mediated migration and motility of hepatocellular carcinoma cells. PLoS ONE (2015) 10:e0137664. doi: 10.1371/journal.pone.0137664
71. Gao W, Tang Z, Zhang YF, Feng M, Qian M, Dimitrov DS, et al. Immunotoxin targeting glypican-3 regresses liver cancer via dual inhibition of Wnt signalling and protein synthesis. Nat Commun. (2015) 6:6536. doi: 10.1038/ncomms7536
72. Wang C, Gao W, Feng M, Pastan I, Ho M. Construction of an immunotoxin, HN3-mPE24, targeting glypican-3 for liver cancer therapy. Oncotarget (2017) 8:32450–60. doi: 10.18632/oncotarget.10592
73. Ishiguro T, Sano Y, Komatsu SI, Kamata-Sakurai M, Kaneko A, Kinoshita Y, et al. An anti-glypican 3/CD3 bispecific T cell-redirecting antibody for treatment of solid tumors. Sci Transl Med. (2017) 9:eaal4291. doi: 10.1126/scitranslmed.aal4291
74. Sano Y, Azuma Y, Tsunenari T, Kinoshita Y, Kayukawa Y, Mutoh H, et al. Abstract 3653: combining ERY974, a novel T cell-redirecting bispecific antibody targeting glypican-3, with chemotherapy profoundly improved antitumor efficacy over its monotherapy in xenograft model. Cancer Res. (2017) 77(13 Suppl.):3653. doi: 10.1158/1538-7445.AM2017-3653
75. Barker JN, Doubrovina E, Sauter C, Jaroscak JJ, Perales MA, Doubrovin M, et al. Successful treatment of EBV-associated posttransplantation lymphoma after cord blood transplantation using third-party EBV-specific cytotoxic T lymphocytes. Blood (2010) 116:5045–9. doi: 10.1182/blood-2010-04-281873
76. Doubrovina E, Oflaz-Sozmen B, Prockop SE, Kernan NA, Abramson S, Teruya-Feldstein J, et al. Adoptive immunotherapy with unselected or EBV-specific T cells for biopsy-proven EBV+ lymphomas after allogeneic hematopoietic cell transplantation. Blood (2012) 119:2644–56. doi: 10.1182/blood-2011-08-371971
77. Li W, Guo L, Rathi P, Marinova E, Gao X, Wu MF, et al. Redirecting T cells to Glypican-3 with 4-1BB zeta chimeric antigen receptors results in Th1 polarization and potent antitumor activity. Hum Gene Therapy (2017) 28:437–48. doi: 10.1089/hum.2016.025
Keywords: glypican 3, hepatoblastoma (HB), germ cell tumors (GCT), Wilms tumor (WT), rhabdoid tumor, rhabdomyosarcoma, neuroblastoma, immunotherapy
Citation: Ortiz MV, Roberts SS, Glade Bender J, Shukla N and Wexler LH (2019) Immunotherapeutic Targeting of GPC3 in Pediatric Solid Embryonal Tumors. Front. Oncol. 9:108. doi: 10.3389/fonc.2019.00108
Received: 04 December 2018; Accepted: 05 February 2019;
Published: 26 February 2019.
Edited by:
Giuliana Cassinelli, Istituto Nazionale dei Tumori (IRCCS), ItalyReviewed by:
Jorge Filmus, Sunnybrook Research Institute (SRI), CanadaMin Hee Kang, Texas Tech University Health Sciences Center, United States
Copyright © 2019 Ortiz, Roberts, Glade Bender, Shukla and Wexler. This is an open-access article distributed under the terms of the Creative Commons Attribution License (CC BY). The use, distribution or reproduction in other forums is permitted, provided the original author(s) and the copyright owner(s) are credited and that the original publication in this journal is cited, in accordance with accepted academic practice. No use, distribution or reproduction is permitted which does not comply with these terms.
*Correspondence: Michael V. Ortiz, b3J0aXptMkBtc2tjYy5vcmc=