- Department of Morphology, Surgery and Experimental Medicine, University of Ferrara, Ferrara, Italy
Non-coding RNAs (ncRNAs) have been implicated in most cellular functions. The disruption of their function through somatic mutations, genomic imprinting, transcriptional and post-transcriptional regulation, plays an ever-increasing role in cancer development. ncRNAs, including notorious microRNAs, have been thus proposed to function as tumor suppressors or oncogenes, often in a context-dependent fashion. In parallel, ncRNAs with altered expression in cancer have been reported to exert a key role in determining drug sensitivity or restoring drug responsiveness in resistant cells. Acquisition of resistance to anti-cancer drugs is a major hindrance to effective chemotherapy and is one of the most important causes of relapse and mortality in cancer patients. For these reasons, non-coding RNAs have become recent focuses as prognostic agents and modifiers of chemo-sensitivity. This review starts with a brief outline of the role of most studied non-coding RNAs in cancer and then highlights the modulation of cancer drug resistance via known ncRNAs based mechanisms. We identified from literature 388 ncRNA-drugs interactions and analyzed them using an unsupervised approach. Essentially, we performed a network analysis of the non-coding RNAs with direct relations with cancer drugs. Within such a machine-learning framework we detected the most representative ncRNAs-drug associations and groups. We finally discussed the higher integration of the drug-ncRNA clusters with the goal of disentangling effectors from downstream effects and further clarify the involvement of ncRNAs in the cellular mechanisms underlying resistance to cancer treatments.
miRNAs and Drug Resistance in Cancer
Chemotherapy represents the primary treatment for both early and advanced tumors. However, drug resistance seriously limits the potency of conventional chemotherapeutics and novel biological agents, this constitutes a major obstacle in the treatment of cancer (1). Then, a lot of effort is aimed to identify new biomarkers, and to assess and predict the response of patients to drugs (2). Cancer drug resistance is referred as intrinsic, if tumors demonstrate to be insensitive to therapeutic agents before treatment, otherwise it is defined acquired if tumor becomes resistant during the treatment. The acquisition of resistance to several types of anticancer drugs can be due to the expression of transporters that eject drugs from cells, resulting in multidrug resistance (3). Nevertheless, several other mechanisms are involved in resistance, including insensitivity to apoptosis induced by drugs, increased repair of damaged DNA, decreased intracellular accumulation of therapeutics, and induction of mechanisms capable of drug detoxification (1). Recent data showed that other than by genetic and epigenetic changes, such as base mutations, amplifications, methylation and other post-translational modifications, drug resistance might also be due to non-coding RNA (ncRNAs) (4). The bulk of the human transcriptome, excluding the ribosomal and mitochondrial RNA, is represented by non-coding transcripts, including the most studied miRNAs and the newly discovered long non-coding RNAs (lncRNA) (5). MicroRNAs (miRNA) are small non-coding RNA molecules (18–22 nt in length) that act as negative regulators of gene expression through modulation of multiple target mRNAs, by inhibition of translation (6–9). A number of miRNA genes are located within intronic regions of genes, both coding or non-coding for proteins and can be transcriptionally regulated through their promoters (10). Other miRNAs are found either within exons, including 3′ UTRs of mRNAs, or clustered with other miRNA genes (11). Since their discovery (12, 13), the number of annotated miRNAs in the human genome has grown rapidly and they regulate a variety of cellular processes, including apoptosis (14), differentiation (15) and cell proliferation. miRNA deregulation has been demonstrated in cancer (16–19). The role of miRNAs in controlling cellular proliferation, differentiation and apoptosis, and their location at sites of translocation breakpoints or deletions (20), suggests that they might function as tumor suppressors or oncogenes (21–23). Profiles of miRNA expression differ between normal and tumor tissues, and among tumor types (18, 24–27). The association of miRNAs with cancer was first revealed in chronic lymphocytic leukemia (CLL), upon the discovery that miR-15a and miR-16-1 were frequently deleted or down-regulated (16, 28), and that their expression was inversely correlated to that of BCL2 (29). Since then, numerous studies have provided evidence for changes in microRNA expression in oncogenesis: different cancer pathways can converge to affect the same miRNAs and conversely a single miRNA can control an entire transcriptional program, affecting a lot of target genes. The deregulation of miRNAs is linked to cancer progression and clinical outcome (30), and miRNAs have been proposed as potential diagnostic markers, prognostics factors, and therapeutic targets (27, 31–33). When aberrant microRNA expression is directly involved in carcinogenesis (21), the inhibition of selectd microRNAs may have therapeutic implications. Modified antisense oligonucleotides have been designed ad-hoc and have proven effective at inhibiting microRNA function in vivo in mice (34, 35). The association of microRNA expression with cancer prognosis, therapeutic outcome and response to therapy, independently of other clinical covariates has been documented (25, 26, 36, 37), and selected miRNAs may influence cancer response to chemotherapy (38). The prognostic potential of microRNAs has been demonstrated for CLL (37), lung cancer (39), pancreatic cancer (25), and neuroblastoma (40) among others. One of the firsts observation on a possible link between miRNAs and drug resistance was reported in breast cancer (BC) suggesting that increased sensitivity of patients to anthracycline-based chemotherapy was related to deletion of chromosome 11q, a region containing MIR125B1 (41). The effect of miRNAs on chemotherapy was systematically studied by Blower et al. (42) on NCI-60, a panel of 60 cancer cell lines, used by the National Cancer Institute to screen >100,000 chemical compounds for anticancer drug sensitivity (20, 38, 42). Overall, miRNAs can mediate drug resistance through multiple pathways, including: (i) cell cycle and proliferation control, (ii) survival and/or apoptosis signaling pathways, (iii) DNA repair systems, (iv) specific drug targets, (v) adenosine triphosphate–binding cassette (ABC) transporter proteins, and/or drug metabolism, (vi) the epithelial–mesenchymal transition (EMT) process (4, 6, 43, 44). For example, miR-15b, miR-16 and miR-22 have been documented as mechanisms in chemotherapy resistance (45, 46). Cell cycle deregulation by miRNAs can induce resistance in cancer cells, as confirmed for miR-224 (47). Also, miR-24 and miR-508-5p can directly target enzymes involved in drug metabolism (48, 49). In addition to the mechanisms described above, modulation of epithelial-mesenchymal transition (EMT) can exert an effect on cancer cell resistance. Importantly, once cancer cells undergo EMT, chemo-resistance is increased and metastasis can occur (50, 51). Normal stem cells are already more resistant to drug treatment due to over-expression of drug efflux pumps and anti-apoptotic proteins (52). In this context, miR-34, miR-125b, miR-140, and miR-215 have an important role in conveying drug resistance to cancer stem cells (2). Chemotherapy can induce EMT and modulate the expression of miR-448 to promote cancer cell progression (53); conversely miR-29c or miR-224 have recently been shown to regulate the EMT process (54). miRNome dysregulation in relation to chemotherapy has been described for the most common tumor types: breast, ovarian, lung, prostate, gastric and colon cancer, squamous and hepatocellular carcinoma (HCC), cholangiocarcinoma, neuroblastoma and various types of leukemia (55). Overall, these studies highlight the complexity of adaptive/selective mechanisms in the establishment of resistance to cancer therapies.
lncRNAs and Drug Resistance in Cancer
lncRNAs have been linked to cancer progression and metastasis (56), and recently intensive research has been devoted to the molecular dissection of their roles, as well as to their diagnostic and prognostic significance (57). lncRNAs are mRNA-like transcripts 200 nt to ~100 kb in length lacking significant open reading frames. lncRNAs can be transcribed by RNA polymerase II (RNA pol II), poly-adenylated and located within nuclear or cytosolic fractions (58). lncRNAs can be divided into different categories: if overlapping with any transcript on sense or anti-sense strand lncRNAs will be classified as (i) sense or (ii) antisense respectively. When its expression is initiated along with a neighboring transcript, sense or antisense, that is proximal, (iii) bidirectional. When deriving from an intronic region, (iv) intronic or (v) intergenic if placed between two genes (53). Generally, lncRNA expression levels appear to be lower than those of protein-coding genes (54), and lncRNAs might be preferentially expressed in specific tissues (59). As to their functions, lncRNAs can regulate the expression of genes in close proximity (cis-acting regulation) or can target distant transcriptional activators or repressors (trans-acting) (53, 60). Their mechanisms of action are still diverse, and have been associated with a spectrum of biological processes, for example, epigenetics, alternative splicing, nuclear import, structural components, precursors to small RNAs and regulators of mRNA decay (60–63). Thus lncRNAs can regulate cellular functions such as chromosome dosage compensation (64), imprinting (65), cell cycle progression (66) and differentiation (67). Aberrant regulation of lncRNAs is reported in a variety of diseases, including cancer (68–71). Accumulating reports of misregulated lncRNA expression across numerous cancer types suggest that also this class of ncRNA can act in oncogenesis and tumor-suppression (72). A number of useful databases providing molecular information on lncRNAs are available (73). Loss of imprinting and redirecting chromatin remodeling complexes (74), induction of metastasis (75), depletion of miRNAs as “molecular decoy” or “miRNA sponge” (76) and direct inactivation of tumor suppressor genes (77) have been referred to specific lncRNAs. Preliminary studies commenced to report the value of ncRNAs as potential biomarkers in clinical settings (78, 79) and their roles in drug resistance (80).
A Network Analysis: The Most Central ncRNAs in Chemoresistance
In recents years, an increasing number of studies have been reported on ncRNAs, target gene modulation, and affected drug functions, pharmacogenomics or chemoresistance. With the aim to facilitate the classification of ncRNAs and drug targets, some databases have been developed, such as NRDT (81) or Pharmaco-miR (82), collecting all information about ncRNA-target gene-drugs. There are large numbers, and growing, of both ncRNAs and cancer drugs, thus the combinations between members of the two groups are very difficult to manage in a traditional review or interpretate in a database. Therefore we decided to use machine-learning systems and to study the RNA-drug interactions using a network-based approach. Basically, we took from KEGG database all approved drugs used for cancer therapy. Then, we searched in PubMed all recent studies (published from 2011 onwards) investigating ncRNAs in chemoresistance. This selection was performed by batch analysis of PubMed-NCBI (National Center for Biotechnology Information) using as major topics the drugs from KEGG, ncRNA and chemoresistance. The result of this screening was manually curated in order to avoid and remove papers with generic statements and not direct links between ncRNAs and drugs. Only the investigations that proved (by in vitro/in vivo) experiments the existence of a direct association between ncRNAs and chemoresistance were then analyzed using a machine-learning tool. We thus built a network of non-coding RNAs starting from a human-curated selection of papers and applied an ad-hoc data mining approach to dissect the network and identify the most important ncRNA/cancer drugs interactions and cliques. We obtained a fully connected network of 388 drug/ncRNA interactions (edges) and 5 unconnected pairs (Supplementary Image 1). We then went on with studying the network, which had 227 nodes: 150 miRNAs, 35 lncRNAs and 42 drugs. Three graph theory measures were considered to define the most relevant non-coding RNAs associated to therapeutics resistance: (i) degree, indicating the number of links that an ncRNA had with different nodes (here drugs) (ii) betweenness centrality, a measure of centrality in the network based on shortest paths (iii) closeness centrality, related to the distance between the ncRNA and all the other nodes in the network. Then, we ranked the nodes (drugs and ncRNAs) and edges (combinations) in the network and collected the combinations from ncRNAs with a degree >3 and a central position (closeness centrality > 0.26 and betweenness centrality >0.003) (Figure 1 and Supplementary Table 1). Finally, we performed a community structure analysis using Glay and Cytoscape (83) to identify different clusters of ncRNAs and drugs. The clusters were converted to subnetworks for convenient visualization. The visual separation of clusters was improved by overlaying the community structure on a graphic layout addressing specific topology (Figure 2).
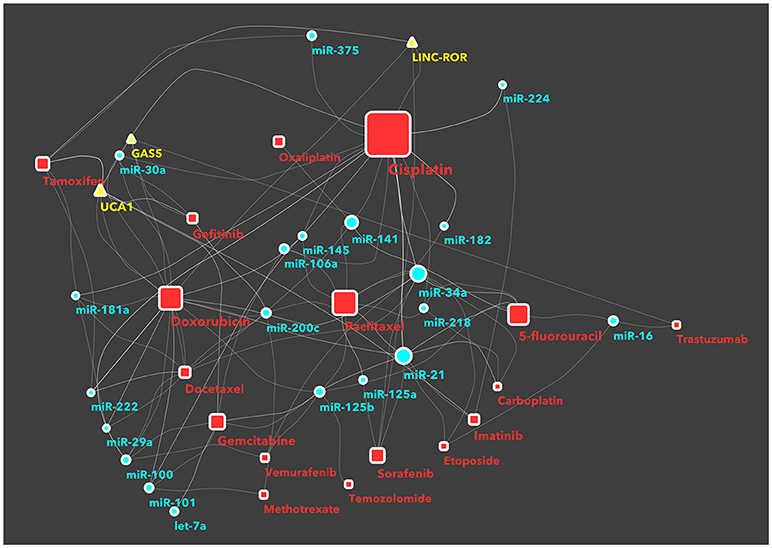
Figure 1. The network of non-coding RNAs and anti-cancer drugs. Each link between a drug and an ncRNA indicates a study in literature, investigating on the specific chemoresistance involvement of that ncRNA in cancer. The nodes (a ncRNA or a drug) shown in this figure have a degree >3, a central position in the network (expressed as betweenness centrality in the network description) >0.003, or a closer position relative to the companion drug (expressed as closeness centrality >0.26). The full network, with all nodes, is reported in supplemental information as Supplementary Image 1. Drugs are represented as red squares, miRNAs as light blue circles and lncRNAs as yellow triangles. The size of a node is proportional to its betweeenness centrality, while the size of a node name is proportional to its degree.
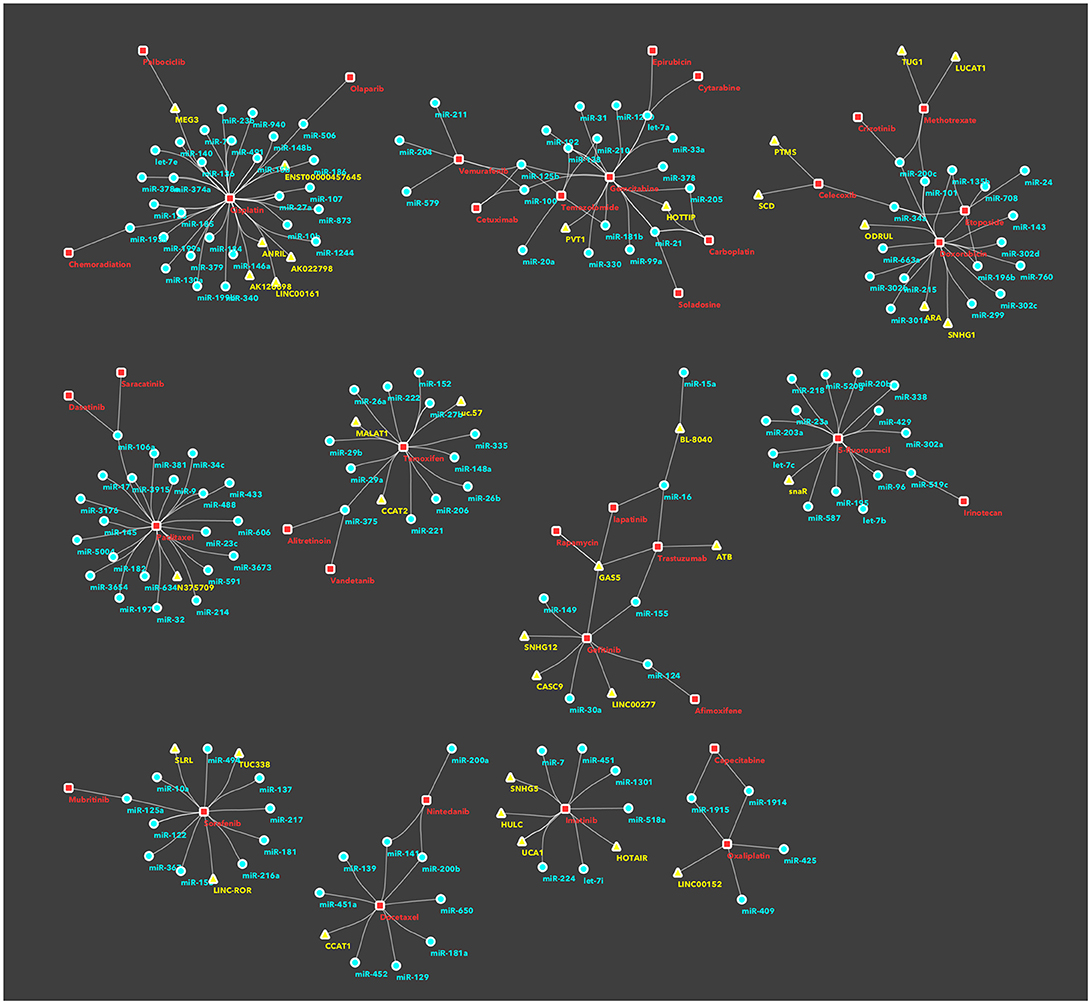
Figure 2. Subnetworks/clusters of non-coding RNAs/drugs associations, according to community analysis. This figure depicts disjoint subnetworks corresponding to the different clusters in the whole network (Supplementary Image 1) and identified using the community analysis tool in Cytoscape. This is a simplification of the involvement of ncRNAs in drug resistance, as an ncRNA, or a drug, is represented only in a single cluster/subnetwork. For sake of completeness, all interactions are described in the main text and presented in the Supplementary Table 1.
We wish to add a cautionary note to our reviewing effort. Even in the genome-wide studies (a minority among those we included in this review) for a number of conscious or unconscious reasons, scientists often end up chasing the most “popular” ncRNAs among others of “lesser pedigree.” Thus there is potentially a positive bias toward well-known ncRNAs in the overall scheme, and therefore in the final network. For this reason, we decided to keep all associations and, although the “degree” (number of associated drugs for an ncRNA) is important, we tried to avoid biased selections and included in our review all ncRNAs/pairs.
Here we commence with describing the ncRNAs that are most prominent in relation to chemoresistance, as detailed in (Figure 1).
miR-21 has the highest scores (degree, betweenness centrality and closeness centrality) as it was associated with several drugs. The MIR21 gene is located at17q23.2, a region frequently amplified in several tumors (84, 85). Its overexpression has been observed in most cancer types and modulates the resistance toward apoptosis-inducing drugs (86–91). Down-regulation of miR-21 sensitizes cancer cells in vitro to different chemotherapeutics, including cisplatin, etoposide and doxorubicin (92–94). On the other hand, some drugs can induce alterations in miR-21 levels: e.g. soladosine can inhibit lung cancer cell invasion through miR-21 down-regulation, via PI3K/Akt signaling pathway (95). Interestingly, exogenous Epstein Barr virus modulates the PI3K/Akt pathway through LMP1, thus enhancing miR-21 expression and contributing to cisplatin reduced response in nasopharyngeal carcinoma (96). Moreover, miR-21 delivered by exosomes augmented malignancy in recipient cells and conferred paclitaxel resistance to ovarian cancer cells (97). There was also a report for enhancement of anticancer activity when Cao et al. reported that miR-21 induction sensitized gastrointestinal tumor cells to imatinib (98).
miR-34a was reported to be downstream of p53 and to function as a tumor suppressor (99). It is down-modulated in colorectal cancer (CRC) (100). In 5-Fluorouracil (5-FU)-resistant colon cancer cells ectopic expression of miR-34a inhibited cell growth and attenuated the resistance to 5-FU through down-regulation of SIRT1 and E2F3 (101), inhibition of LDHA (102) and of c-Kit, thus reducing stem cell factor (SCF)-induced migration/invasion (103). Yang et al. demonstrated that miR-34 targets BCL2 and sensitizes HCC cells to sorafenib (104). In osteosarcoma cell lines miR-34a has been tested in combination with celecoxib: the treatment showed decreased cell viability, migration and invasion through regulation of the Notch1/ROCK1-PTEN-Akt-GSK-3β axis (105). Moreover, miR-34 could enhance the therapeutic efficacy of paclitaxel in resistant prostate cancer (106). Its overexpression enhanced cisplatin sensitivity, as confirmed in gastric cancer, by targeting MET (107) and in lung cancer, through the p53/MICN axis (108). Conversely, Pu et al. found that miR-34a overexpression in osteosarcoma promoted resistance to several drugs (doxorubicin, etoposide, carboplatin, cisplatin), via repression of AGTR1 (109).
The lncRNA Urothelial Cancer-Associated 1 UCA1 gene is located at 19p13.12 (110). Different transcriptional isoforms have been reported, UCA1 (1.4 kb), UCA1a (2.2 kb) and CUDR (2.7 kb), generated by alternative splicing and poly-adenylated. UCA1 is the most abundant isoform in various malignant tumors such as bladder cancer, BC and HCC (110–113). UCA1 could promote drug resistance by directly binding to miR-204, miR-18a and miR-16 (114). UCA1 emerged as a competitive endogenous RNA (ceRNA) of multi-drug resistance associated protein 1 (MDR1), inducing resistance to imatinib in CLL cells by sequestering miR-16 (115). Overexpression of UCA1 up-regulated MDR1, resulting in imatinib resistance, whereas its silencing had the opposite effect (116). In bladder cancer, UCA1 enhanced chemoresistance to cisplatin by regulating Wnt signaling (117) and to cisplatin/gemcitabine through modulation of miR-195a (118). Recent studies reported that UCA1 regulates tamoxifen resistance in BC (119). Liu et al. demonstrated that the knockdown of this lncRNA could revert resistant phenotype and increase tamoxifen sensitivity through inhibition of the Wnt/β-Catenin pathway, thus further confirming the oncogenic role of UCA1 in BC (120). Moreover, UCA1 was shown to be released in exosomes by tamoxifen resistant BC cells and increased tamoxifen resistance in ER-positive recipient cells (121).
The members of miR-125 family (miR-125a, miR-125b-1 and miR-125b-2) play an important role in tumorigenesis and are potential biomarkers for cancer diagnosis, treatment and prognosis in clinical settings (122). MIR125A gene is on chromosome 19, while two separate loci on chromosomes 11 and 21 harbor MIR125B1 and MIR125B2, respectively (123). miR-125b expression has been found negatively correlated with 5-Fluorouracil resistance in HCC (124), while resistance to pharmacological treatments with gentamicin, cetuximab, doxorubicin and temozolomide by miR-125b still remains controversial (88, 125–127). miR-125b regulates the resistance to paclitaxel in colon cancer cells, in association with miR-125a (128). Recent data strongly supports a relevant role for miR-125b in conferring taxol resistance in BC, via suppression of pro-apoptotic BCL2 antagonist killer 1 (Bak1) (129). In contrast, in chondrosarcoma, overexpression of miR-125 enhanced the sensitivity to doxorubicin by directly targeting ERBB2-mediated glucose metabolism (130). miR-125a overexpression increased the response to paclitaxel in cervical cancer, through STAT3 down-modulation (131). Sorafenib treatment in HCC showed restoration of mir125 levels by sirtuin-7 and p21/p27 signaling blockage inhibiting cell cycle progression (132). In AML cells, via mubritinib, miR125a inhibited the ERBB pathway and cell cycle proliferation and progression, suggesting that miR-125a increased the sensitivity to the drug (133).
The MIR100 gene is at 11q24. Deregulation of miR-100 has been reported in drug resistance; however, miR-100 expression can be either over-expressed or under-expressed in diverse cancers (134). In ovarian cancer, miR-100 targets mTOR therefore reverting the cell's chemoresistance toward cisplatin (135) and chondrosarcoma (136). In pancreatic cancer, miR-100 mimics inhibit proliferation and increase sensitivity to cisplatin by targeting FGFR3 (137). Recently, it has been shown that down-modulation of miR-100 could increase β-tubulin class V expression, promoting tumor cells proliferation, with implications for paclitaxel resistance (138). Also, miR-100 reduced ATM levels in a human glioma cell line (M059J) and could sensitize tumor cells to ionizing radiation (139). In vitro, miR-100 also induced the differentiation of BC stem cells expressing a functional ER (140). Furthermore, in CRC cells miR-100, together with miR-125b, negatively regulated Wnt/β-catenin signaling, and restored responsiveness to cetuximab (125). On the other hand, in mutant p53 pancreatic carcinoma, miR-100 up-regulation was related to gemcitabine resistance (88). In accordance, the exosomes-mediated intercellular transfer of miR-100, from drug resistant BC cells, could lead to resistance in sensitive cells (141).
miR-200c acts as a tumor suppressor, and could inhibit the initiating steps of metastasis; a negative correlation with ZEB factors has been reported, suggesting that this miRNA-mediated regulatory pathway influences EMT (142–147), potentially modulating drug resistance in advanced tumors. miR-200c reverses resistance of lung cancer cells, both to chemotherapeutics, like methotrexate (148), and to targeted drugs, like crizotinib (149) and gefitinib (146, 150). In breast and renal cancers, miR-200c could be involved in resistance or re-sensitization to microtubule-targeting drug (151–153).
miR-141 is another member of the miR-200 family, also involved in EMT, invasion, migration and drug resistance (154). miR-141 overexpression contributes to acquired chemoresistance, for both in vitro and in vivo models. The initiation factor 4E (EIF4E) mRNA is a target of miR-141, that is involved in drug-induced apoptosis, conferring resistance to docetaxel-sensitive BC cells (155). miR-141 regulates cisplatin sensitivity in non-small lung cancer cells via PDCP4 inhibition and its inhibition increases cisplatin-induced apoptosis (156). In oesophageal squamous cell carcinoma, miR-141 was highly overexpressed in 5-Fluorouracil and oxaliplatin resistant cells and contributed to acquired chemo-resistance via PTEN (157). Moreover, in HCC cells, miR-141 was shown to confer resistance to 5-Fluorouracil through the inhibition of KEAP1, thereby reactivating the NRF2-dependent antioxidant pathway (158). Li et al. discovered that miR-141 together with other miRNAs like miR-16 contribute to prostate cancer chemoresistance via an exosome network (159).
Two homologous microRNAs, miR-221 and miR-222, are generally considered having an oncogenic activity (160). The expression of miR-221 and miR-222 is highly up-regulated in HER2/neu-positive human BCs resistant to endocrine therapy, compared with HER2/neu-negative tissue samples (161); also, in BC patients miR-222 is elevated in chemoresistant tissues after surgery, compared with the pre-neoadjuvant samples (162). miR-221/222 reduce the protein level of the cell cycle inhibitor p27Kip1, conferring tamoxifen (161) and doxorubicin resistance (162). Also, secreted miR-221/222 could serve as signaling molecules and mediate communication of tamoxifen resistance (163). Aberrant expression of miR-222 is tightly related to poor overall survival (164) and affect oncogenic signaling pathways associated with resistance to different drugs (165). miR-222 also mediated BC cells resistance to adriamycin via PTEN/Akt/FOXO1 (164). Furthermore, the exosome mediated release of miR-222, miR-100 and miR-30a contributes to the same effect on docetaxel and doxorubicin: loss of responsiveness in BC cells (141). In oesophageal and prostate cancers, miR-221 could modulate 5-Fluoruracil resistance via the Wnt/β-catenin-EMT pathway (166) or RB1 (167), respectively.
miR-101 (168, 169) has a relevant role in autophagy. Targeting the autophagy process is a promising therapeutic strategy to improve chemotherapy efficiency. In BC cells miR-101 inhibits basal autophagy, as well as etoposide- and rapamycin-induced autophagy, thus sensitizing cancer cells to 4-hydroxytamoxifen (4-OHT)-mediated cell death (170). In HCC, miR-101 sensitizes cell lines to cisplatin-induced apoptosis by targeting Mcl-1 (171). Likewise, miR-101 inhibits autophagy and enhances chemo-sensitivity to doxorubicin of osteosarcoma cells in vitro (172). In pancreatic cancer, miR-101 up-regulation reverts gemcitabine resistance by inhibiting the expression of ribonucleotide reductase M1 (RRM1) (173). Moreover, recent studies demonstrate that miR-101 interacts with lncRNA MALAT1 in regulatory networks that modulate cisplatin and temozolomide resistance, in lung cancer (174) and glioblastoma (80), respectively.
The miR-15/16 gene cluster in chromosome 13 (13q14) is deleted or down-regulated in some cancer types (21). This somatic alteration was reported to occur early in cancer development and could represent a target for intervention (21). miR-16 expression is affected by several drugs: in gastric cancer cell lines etoposide and 5-Fluorouracil could increase the levels of miR-16, both in vitro and in vivo, and the up-regulation of miR-16 is modulated by p38 MAPK signaling pathway (175). In BC, lapatinib and trastuzumab are reported to regulate miR-16 via PI3K/Akt (176). Noteworthy, the altered expression of both miR-15a/16-1, due to the CXCR4 inhibitor BL-8040 induced the apoptosis of AML blasts by down-regulating ERK, BCL2, MCL1 and cyclin-D1 (177).
The lncRNA GAS5, originating from the Growth Arrest-Specific 5 gene, is down-regulated in multiple cancers. GAS5 inhibits proliferation and promotes apoptosis, thus playing a tumor suppressor role (178). Several studies confirmed GAS5 as an mTOR effector, and its expression was directly correlated with chemoresistance. Thus, enhancing GAS5 expression may improve the effectiveness of rapalogues, as confirmed both in prostate tumor cells and in mantle cell lymphoma cells (179, 180); also, the down-modulation of GAS5 caused resistance to trastuzumab in BC (181). In lung adenocarcinoma cells resistant to EGFR inhibitors, GAS5 enhance gefitinib-induced cell death, via down-regulation of IGF1R (182). Lastly, in bladder transitional cell carcinoma GAS5 inhibited malignant proliferation and chemotherapy resistance to doxorubicin, partly acting via BCL2 (183).
miR-106a, a member of the miR-17 family, is associated with poor prognosis, invasion and metastasis (184). In ovarian cancer (OV), miR-106a inhibited cell survival and cisplatin resistance, through downregulation of MCL1 (185); conversely expression of miR-106a was higher in cisplatin-resistant OV. miR-106a may be involved in the modulation of cisplatin-induced apoptosis by regulating PDCD4 (186). In non-small cell lung cancer, miR-106a also confers cisplatin resistance, by targeting adenosine triphosphatase-binding cassette A1, an ABC transporter (187). Otherwise, by targeting autophagy, miR-106a enhances sensitivity of lung cancer cells to SRC inhibitors, including saracatinib and dasatinib, expliciting once more the context-dependent function of miRNAs (188). Further, dysregulation of miR-106a conferred resistance to paclitaxel in OV; its modulation resensitized resistant cells by targeting BCL10, caspase-7, and ZEB1 (189). Down-modulation of miR-106a was reported in gentamicin resistant hepatoma, participating to EMT via the PDGF-D/miR-106a/Twist1 pathway; notably, in HCC patients, miR-106a and Twist1 were associated with PDGF-D expression (190).
miR-375 is involved in a positive feedback loop with ER in BC (191) and its re-expression is sufficient to sensitize tamoxifen-resistant cells. Furthermore, miR-375 partly reversed the EMT process: metadherin (MTDH) was identified as a direct target of miR-375 and tamoxifen-treated patients with higher MTDH had a higher risk of relapse (192). Another miR-375 target is HOXB3; miR-375 inhibited cancer stem cells (CSCs) phenotype and tamoxifen resistance by regulating CSCs, through degradation of HOXB3 (193). Epigenetically down-regulated miR-375 in HER2-positive BC could induce trastuzumab resistance by targeting IGF1R (194). 9-cis retinoic acid (Alitretinoin) modulated the expression of miR-375 in BC depending on ER status: thus, miR-375 was inhibited in ERα-positive cells while highly induced in ERα-negative cells (195). The deregulation of miR-375 was also observed in other malignancies: in medullary thyroid carcinomas (MTC) miR-375 was the strongest up-regulated miRNA (196). Vandetanib is a tyrosine kinase inhibitor for the treatment of patients with recurrent or metastatic MTC that are unresectable, and/or symptomatic (197). Interestingly, miR-375 over-expression associated with SEC23A down-regulation could improve the efficacy of vandetanib (196). Thus, the expression levels of miR-375 and SEC23A pointed to vandetanib sensitivity and could be evaluated as predictive indicators for efficacy of vandetanib in MTC. Analogously, up-regulation of miR-375 increased the cisplatin-sensitivity of gastric cancer cells by regulating ERBB2 and phospho-Akt (198).
A role in chemoresistance modulation has emerged for putative tumor-suppressor miR-145 (199). miR-145 targeting of MDR1 helps to restore drug efficacy in resistant cells and in vivo models of bladder cancer and BC (200, 201). Moreover miR-145 confirmed its role in reducing chemoresistance also with paclitaxel (202) and doxorubicin (203), possibly via regulation of EMT.
miR-218 has a physiological role in neuron development and its loss of expression is involved in neurodegeneration (204). In BC, it acts as a risk factor in ductal carcinoma in situ (DCIS) (205). In association with platinum compounds, miR-218 and miR-205 inhibit tumorigenesis and overcome chemoresistance in lung cancer (206). In prostate cancer, miR-218 up-regulation inhibited tumor growth and increased chemo-sensitivity to cisplatin, by negatively regulating BCAT1 (207). Furthermore miR-218 mediated autophagy and was associated with positive response to paclitaxel in resistant endometrial carcinoma (208). It also promoted apoptosis and caused cell cycle arrest in CRC by targeting BIRC5, thus possibly enhancing first-line 5-FU treatment. Also, miR-218 through targeting the enzyme thymidylate synthase (TS), enhanced 5-FU cytotoxicity in CRC cells (209).
The let-7 family members are down-regulated in lung (210), gastric (211), colon cancer (212) and in Burkitt's lymphoma (213). Loss of let-7 was associated with the shortened post-operative survival of patients with lung cancer (210). The altered expression of let-7a could increase chemoresistance to epirubicin (214) and cytarabine (215). Furthermore, let-7a expression has demonstrated to influence chemoresistance, due to maintained treatment with gemcitabine, in pancreatic cancer patients (216, 217). Several studies have reported that let-7a acts as a tumor suppressor in renal cell carcinoma (RCC), by targeting c-Myc (218). let-7b and let-7e are down-regulated in glioblastoma and ovarian cancer, respectively and promote resistance to cisplatin by acting on the same target Cyclin D1 (219, 220). Reduced levels of both let-7b and let-7c could determine the intrinsic chemoresistance to 5-FU in RCC, possibly via AKT2 (221). Clinically, 5-FU-based chemotherapy is considered moderately effective in RCC due to rare response and severe toxicity (222); transfection of let-7b or let-7c potentiated the efficacy of 5-FU in vitro at tolerable concentrations. Moreover, let-7c up-regulation contributed to sensitize lung cancer cells with acquired cisplatin resistance, by involving ABCC2 and Bcl-XL (223). Interestingly, a combination of miR-224 and let-7i, reduced imatinib resistance in CML, probably through targeting the ST3GAL IV sialyltransferase (224).
miR-30a was found to act as an oncosuppressor, but could also promote tumor progression in several types of cancer (225). The same dual activity was described for drug resistance. In ovarian and lung carcinoma miR-30a interacted with cellular receptors (EDNRA and EGFR) and played an important role in overcoming the acquired resistance (226, 227), also via exosomes (141). miR-181a is down-regulated in glioma and lung cancer, while its up-regulation is involved in metastasis and invasion in breast and oral squamous carcinomas (228). Prostate cancer patients undergoing maintained treatment with taxane develop resistance to the therapy. Recently, Armstrong et al. discovered that miR-181a overexpression contributes to docetaxel and cabazitaxel resistance in prostate cancer cells (229). The role of miR-181a in cisplatin resistance is apparently dual: in cervical squamous cancer, it could induce chemoresistance, partly by down-regulating PRKCD (230), while it could reverse cisplatin resistance in tongue squamous cell carcinoma, acting through Twist1 (231).
miR-182 is overexpressed in a broad range of tumor types. Clinical studies associated miR-182 with increased aggressiveness and poor survival (232). miR-182 was also found to have a role in chemoresistance. Acting as a negative regulator of PDCD4, it determined a reduction of sensitivity to cisplatin and paclitaxel in OV (233) and to cisplatin in lung cancer (234). Further, in HCC miR-182 was directly correlated in vitro and in vivo with cisplatin resistance, possibly by regulating TP53 (235).
In inflammatory bowel disease (IBD) and in cancer, miR-224 has an important function. By targeting p21, it participated in cell cycle regulation at the G1/S checkpoint (236). miR-224 could induce resistance to cisplatin in lung and ovarian cancer cell lines (47, 237). In contrast, miR-224 promoted cisplatin sensitivity in osteosarcoma resistant cells by targeting Rac1 (238). miR-224 was related with CRC progression and the response to 5-fluorouracil through KRAS-dependent and -independent mechanisms (239).
Finally, miR-29 family members are miRNAs that can play different roles in cancer (240). For example, they can contribute in BC to the acquisition of doxorubicin resistance by inhibition of PTEN/AKT/GSK3β (241). Conversely, miR-29b exerts a tumor suppressor activity in tamoxifen-resistant BC cells (242).
The lncRNA Regulator Of Reprogramming LINC-ROR is involved in the regulation of the pluripotent stem cells reprogramming. Its expression suppresses the induction of p53 after DNA damage and is associated with tumor progression, EMT and metastasis (243). LINC-ROR is significantly up-regulated in BC, resulting in chemotherapy tolerance and enhanced invasiveness (244). In tamoxifen-resistant BC cell lines, down-regulated LINC-ROR could inhibit EMT and enhance the sensitivity to tamoxifen by increasing miR-205 (245). A relevant study on cancer tissues from BC patients demonstrated that inhibition of LINC-ROR reversed resistance to tamoxifen by inducing autophagy (246). Moreover, LINC-ROR could mediate for sorafenib chemosensitivity in HCC, through the realease of extracellular vesicles (247).
Drugs/Non-coding RNAs Subnetworks
Non-coding RNAs can regulate several protein targets or molecular pathways that lead or inhibit drug resistance according to tumor type, stage and class of drug (248). Above we discussed the ncRNAs with the most prominent roles in the literature as measured using network statistics. There are though many ncRNAs which have been described only in association to one or few more drugs: for these rare ncRNA/drug combinations we performed a clustering analysis of the whole network and identified less than a dozen of groups. The ncRNA/drug combinations are described below as subnetworks and are visualized in (Figure 2). The Supplementary Table 2 details the effects of ncRNAs on chemoresistance.
Subnetwork 1: Gefitinib, Afimoxifene, Rapamycin, Trastuzumab, Lapatinib, BL-8040
Gefitinib is a selective inhibitor of the Epidermal Growth Factor (EGFR) protein. It is used to treat solid tumors, as non small cell lung cancer (NSCLC). It acts by inhibiting the anti-apoptotic Ras signaling cascade (249). Recent studies confirmed also that the loss of regulation of ncRNAs is involved in chemoresistant acquisition (250, 251). The GAS5 lncRNA is implicated in chemoresistance modulation of several different drugs included into this subnetwork (179–182). Another interesting lncRNA present in this group is the Small Nucleolar RNA Host Gene 12 (SNHG12), that plays an oncogenic role in various cancers (252). Moreover, SNHG12 overexpression is implicated in multidrug resistance (included gefitinib resistance), by sponging miR-181a and thus activating the MAPK/Slug pathway (253). This confirms also the involvement of miR-181a in the regulation of chemoresistance. miR-16 has been previously described, and in cancers it may regulate the response to trastuzumab and lapatinib. This miRNA plays an important role in inhibiting cell proliferation and potentiting drug effects (176). Furthermore, in leukemia miR-16 in combination with miR-15 interacts with new phase II drug (177). miR-124 has a role in neuronal differentiation (254) and may modulate resistance to gefitinib and afimoxifene: miR-124 down-regulation could reverse afimoxifene induced autophagy in BC through regulation of Beclin-1 protein (255), while in lung cancer miR-124 depletion plays a role in gefitinib resistance by regulating SNAI2 and STAT3 expressions (256). A prolonged treatment with Gefitinib dramatically reduced the expression of miR-155 and miR-200c. The depletion of these miRNAs may contribute to the decrease in the sensitivity to gefitinib (150). Intriguingly, trastuzumab positively regulates miR-155 and as a consequence, this micro RNA negatively regulates ErbB2 and the malignant cell transformation of breast epithelial cells (257).
Subnetwork 2: Cisplatin, Olaparib, Palbociclib, Chemoradiation
Cisplatin is a platinum compound classified as alkylating like agent that interferes with DNA replication and is used to treat several solid malignancies (258). The efficacy of cisplatin in cancer therapies is limited by the acquired resistance, that can lead to therapeutic failure and tumor recurrence (259). It was demonstrated that cisplatin-resistant cancer cells present an altered expression pattern of ncRNAs (260–281). Among them, miR-451 is known to exert a critical role in the pathogenesis and the development of several types of cancers, including CRC, glioblastoma and NSCLC. miR-451 is located on chromosome 17q11.2, in close proximity of ERBB2 (17q12) (282). miR-451 enhances cisplatin sensitivity in lung cancer cells through regulation of Mcl-1 (283); furthermore, it is involved in the resistance to imatinib in CML patients (284). Another ncRNA present in this network is miR-20a, a member of miR-17 family, which has an oncogenic role and is involved in leukemia and CRC (285). Moreover, Zhou et al. established that miR-20a expression in glioma cells was negatively correlated to Temozolomide sensitivity by targeting DNA methyltransferase (DNMT1) (286). In gastric cancer, miR-20a negatively regulates cylindromatosis (CYLD) expression, thus inducing cisplatin resistance (287). miR-15b had a dual role in oral tongue squamous cell carcinoma (TSCC) and lung adenocarcinoma; through the regulation of TRIM14 it was implicated in the reversion of cisplatin resistance in TSCC (288), while it decreased sensitivity to cisplatin by targeting PEBP4 in lung adenocarcinoma (289). Similarly, Chen et al. discovered the involvement of miR-136 as a tumor suppressor, which targeted E2F1 gene and reversed cisplatin resistance in glioma cells (290). On the contrary, in ovarian cancer miR-136 might induce chemoresistance through the inhibition of apoptosis, while promoting the repair of cisplatin-induced DNA damage (291). miR-27 has a well-defined role: in lung adenocarcinoma cells it contributed to cisplatin resistance by suppressing Raf Kinase Inhibitory Protein RKIP (292). Strikingly, in esophageal cancer miR-27 was associated with the transformation of normal fibroblasts to cancer-associated fibroblasts (293). The same ncRNA could have a role in the sensibilization to different drugs: e.g., miR-506-3p, which is up-regulated in ovarian cancer, has an important function in sensitizing cancer cells to both olaparib and cisplatin (294). Another interesting example is miR-193a-3p that can contribute to the inhibition of chemoradiation and of cisplatin resistance through PSEN1 and p73, respectively in esophageal tumor (295) and osteosarcoma (296). These findings confirmed an oncosuppressor activity for miR-193a-3p (297). miR-199 also may act as either a potential tumor suppressor or oncogene depending on cellular context (298). Consequently, epigenetic silencing of miR-199b-5p may contribute to raise cisplatin resistance via loss of control in cell cycle regulation (299) or miR-199a-3p may enhance cisplatin sensitization by downregulating TFAM (300). Interesting situations emerged when comparing miRNAs from the same family: i.e. down regulation of both let-7 members (let-7b and let-7e) controlled cisplatin resistance through down-modulation of cyclin D1 (219, 220). lncRNAs are an eterogeneous class of non coding RNAs and several studies demonstrated that their dysregulation could affect chemoresistance modulation as much as miRNAs (301–303). Maternally expressed 3 (MEG3) lncRNA that acts as a growth suppressor in tumor cells and selectively regulates p53 target (304), does not have a defined role in chemotherapy. Nevertheless, its up regulation seems to enhance cisplatin resistance in lung cancer (305). Meanwhile, palbociclib can determine the increment of MEG3 expression in a dose dependent manner, yielding to an increase anticancer outcome (306). Controversely, lncRNAs might also modify drug responsiveness exerting a miRNAs sponge activity acting as ceRNAs. Wang et al. demonstrated that downregulation of ANRIL lncRNA enhanced cisplatin citotoxicity via let-7a in nasopharyngeal carcinoma (307). These findings further confirm the role of let-7 family as inhibitors of chemoresistance.
Subnetwork 3: Paclitaxel, Saracatinib, Dasatinib
Subnetwork 3 incorporates several non-coding RNAs related with paclitaxel. This antineoplastic drug is a taxol derivative that blocks cell cycle progression by targeting beta-tubulin. Paclitaxel causes inhibition of mitosis and triggers the apoptotic process or the reversion of cell cycle. Paclitaxel is used to treat a number of solid cancers that include lung, ovarian, breast and pancreatic tumors (308). A number of studies produced evidence that loss of non-coding RNAs regulation can modify chemoresistance to taxol (202, 309–313). miR-182 is often up-regulated in cancers; it can enhance cell proliferation, invasion and it plays an important role in drug resistance. Two different studies found that miR-182 overexpression, by negatively regulating programmed cell death 4 (PDCD4), was involved in chemoresistance exacerbation of lung and ovarian cancers to cisplatin and paclitaxel, respectively (233, 234). Qin et al. demonstrated also that miR-182 expression increases cisplatin resistance of HCC cell by targeting TP53INP1 (tumor protein 53-induced nuclear protein 1) (235). miR-214, through targeting activating protein 2 (AP-2), contributes to regulate molecular processes in melanoma (314). Despite its role, miR-214 function in chemoresistance is still not clear: it could enhance sensitivity to cisplatin in esophageal cancer (315), or promote paclitaxel and carboplatin resistance in ovarian cancer (89). miR-9 may influence cell growth, cell cycle and it is often downregulated in cancer (316). miR-9 down-regulation is one of the key mechanisms accounting for paclitaxel resistance in ovarian carcinoma (317); while high expression of miR-9 in CD133+ glioblastoma cells activates MDR1 gene and imparts Temozolomide (TMZ) resistance (318). miR-17-5p isan oncogenic miRNA, member of the miR-17~92 cluster, which plays an important role in the control of cell cycle progression (319). Despite its oncogenic role, miR-17-5p can promote paclitaxel-induced apoptosis by increasing p53 expression in BC cells (320). The same ncRNA may also influence resistance to different drugs. It is the case of miR-106a that can enhance paclitaxel resistance through apoptosis inhibition (189) or promote sensitivity of lung cancer cells to Saracatinib and Dasatinib (188). In addition, the secretion of miRNA in exosomes is involved in paclitaxel resistance of prostate cancer (159).
Subnetwork 4: Sorafenib, Mubritinib
To treat HCC in advanced status the multikinase inhibitor Sorafenib is the only validated therapy, but tumor response rates to this drug are quite low (321). Several miRNAs, including miR-137 (322), miR-367-3p (323), and miR-125a (131, 133) or lncRNA such as LINC-ROR (247) are involved in the regulation of HCC-Sorafenib treatment efficacy. Tang et al. demonstrated that the simultaneous silencing of miR-21, miR-153, miR-216a, miR-217, miR-494, and miR-10a-5p overcome sorafenib resistance in vitro and in vivo models of HCC (324). Azumi et al. found also that up-regulation of miR-181a increased sorafenib resistance, by blocking a MAPK signaling factor (RASSF1) in HCC cells (325). miR-122 is highly expressed in the liver, where it has been implicated as a regulator of fatty-acid metabolism. This ncRNA was significantly reduced in sorafenib-resistant HCC cells. Xu et al. demonstrated that miR-122 restoration increases sensitivity to sorafenib and induces apoptosis by repressing IGF1R (326). miR-122 is also involved in the control of arginine transport by targeting the solute carrier family 7 (SLC7). Arginine is the substrate for nitric oxide (NO) synthetase and as a result, loss of miR-122 in HCC cells causes an increment of intracellular NO and resistance to sorafenib (327). Moreover, knock-down of TUC338 lncRNA increased expression of RASAL1 protein in HCC, inhibited tumor growth and sensitized cells to sorafenib (328). Sorafenib is also used in the treatment of renal carcinoma (RCC), where SRLR (sorafenib resistance-associated lncRNA in RCC) was found up-regulated in sorafenib-resistant RCCs and contributed to sorafenib tolerance (329).
Subnetwork 5: Docetaxel, Nintedanib
Docetaxel is a drug that promotes cell apoptosis after its interaction with beta-Tubulin metabolism and Bcl-2 phosphorylation. It is used to treat late-stage and metastatic BC, head and neck cancer, stomach cancer, prostate cancer and NSCLC (330). This subnetwork underlines the role of miR-129, a miRNA with tumor suppressor activity in several cancers (331). Lu et al. confirmed the role of this miRNA also in reducing drug resistance: miR-129 in gastric cancer cells reverses cisplatin-resistance through inhibition of P-gp expression (332). Nevertheless, another study demonstrated that miR-129 overexpression may be implicated in BC and docetaxel resistance, mainly through CP110 inhibition (333). Up-regulation of miR-141 and miR-181a (155, 229) also could contribute to docetaxel resistance, while down-regulation of miR-29a and miR-451 inhibited this process (334). Similarly to miR-200c, miR-200b has also a role in drug response: loss of miR-200b regulated autophagy in lung adenocarcinoma and was associated with resistance to docetaxel (335). Nintedanib inhibited VEGFR and consequently angiogenesis (336, 337). Nintedanib is also capable of reverting the resistance to gefitinib promoted by miR-200b and miR-141 (338). Dongqin et al. found that miR-451 down-regulation induced c-Myc expression, an event related to docetaxel-resistance (339). The role of miR-139 in cancer is still not clear (340), but by targeting NOTCH1, it could mediate cell sensitivity to docetaxel and 5-FU, respectively in breast (341) and CRC (342). Chen et. al. reported that miR-30a was related with docetaxel resistance in BC by horizontal exosomes transfer (141). Aberrant expression of CCAT1 lncRNA had a sponging effect on miR let-7c and, as a consequence, promoted chemoresistance to docetaxel in lung adenocarcinoma (343). This last evidence is intriguing, since it is also reported that let-7c up-regulation inhibited chemoresistance to 5-Fluorouracil in renal carcinoma (221) and sensitized resistant lung carcinoma cell (A549) to cisplatin (223).
Subnetwork 6: Gemcitabine, Temozolomide, Cetuximab, Carboplatin, Cytarabine, Epirubicin, Soladosine, Vemurafenib
Gemcitabine is a synthetic nucleoside analog used to treat various carcinomas and several investigations confirm that ncRNAs can modulate gemcitabine action (344, 345). Cao et al. demonstrated that miR-192 regulated gemcitabine and cisplatin resistance in lung adenocarcinoma through modulation of apoptosis (346). miR-192 together with miR-215, was found to be a positive regulator of TP53 (347). In glioblastoma miR-138 is involved in cell death mechanisms that promote chemoresistance to temozolomide (348), an alkylating drug similar to gemcitabine, that enhances cell apoptosis of tumor cells. Moreover miR-138 aberrant expression can provide a basis for novel gemcitabine chemoresistance markers in bladder and pancreatic ductal carcinoma (88, 349). Furthermore, miR-138 was implicated in the pathogenesis of chronic myeloid leukemia and its clinical response to imatinib (350). Cetuximab is a monoclonal antibody with a mechanism of action different from gemcitabine, however, miR-100 over-expression may promote chemoresistance against both treatments (88, 125). Depending on the cellular context both up-regulation and down-regulation of an ncRNA could lead to chemoresistance. In our review, the role of miR-205, which regulates EMT (351), emerged as one of such cases. It is apparent that miR-205 upregulation causes inhibition of chemoresistance to gemcitabine in pancreatic cancer (352), but Zarogoulidis et al. demonstrated that miR-205 and miR-218 were associated with carboplatin resistance in lung cancer (206). miR-181b over-expression increased gemcitabine resistance (353), whereas miR-181b was involved in temozolomide sensitivity in glioma by targeting MEK1 (354). Lee et al. found that hypoxia-induced miR-210 (355) could potentially reverse temozolomide resistance in glioblastoma (356). Another investigation discovered that miR-210, in association with miR-21, miR-99a, miR-100, miR-125b, and miR-138 may serve as biomarkers of gemcitabine resistance in pancreatic cancer (88). In this subnetwork we find also some important miRNAs that were previous described: miR-21-5p (91) miR-125b-5p (89, 125, 127), and let-7a (214–217). The HOTTIP lncRNA (HOXA transcript at the distal tip) can promote cancer progression and gemcitabine resistance in pancreatic cancer (357). Finally the overexpression of BC200 lncRNA has a role in the induction of cell death by carboplatin in ovarian cancer (358). Furthemore, miR-204 is highly induced by vemurafenib in resistant melanoma cells and tissues, as much as miR-211 (359). Although belonging to the same family, the expression of miR-204 is high in amelanotic melanoma cells, and acts as an effector of vemurafenib's anti-motility activity. Conversely, miR-211 which is induced in melanotic melanoma cells, mediates and potentiates the increase in pigmentation due to vemurafenib; this adaptive response de facto limits its efficacy (360). miR-204 inhibits the migration/invasion of melanoma cells with a potency similar to that of miR-211 and, more importantly, it acts in the cellular contexts in which miR-211 is absent (359). Fattore et al. demonstrated that miR-579-3p is strongly downregulated in melanoma and loss of BRAF and MDM2 regulation leads to chemoresistance to targeted therapy (361).
Subetwork 7: Oxaliplatin, Capecitabine
Oxaliplatin is used for the treatment of CRC and has been compared with other platinum compounds used for advanced cancers, such as cisplatin and carboplatin. Oxaliplatin in combination with capecitabine (XELOX) is a first-line treatment of CRC, hile for CRC in advanced stages is common to use oxaliplatin in combination with 5-FU (FOLFOX) (362). Several studies demonstrated that miRNAs modulate the chemoresistance to these drugs. In particular, Hu et al. found that circulating miR-1914-3p and miR-1915-3p are down-regulated in patients with chemoresistant CRC. Consequently, up-regulation of these miRNAs in vivo, could partially restore CRC cells sensitivity to XELOX treatment (363). Furthermore, miR-425-5p ihnibition reversed oxaliplatin resistance both in HTC116-resistant cells lines and xenograft models by modulating the expression of PDCD10 (364). Tan et al. observed a negative correlation between miR-409-3p and resistance to Oxaliplatin in CRC resistant cells (365). Moreover, as a putative miRNAs modulator, also long intergenic noncoding RNA (LINC00152), can be involved in chemosensitivity of Oxaliplatin in CRC. LINC00152 increases the chemosensitivity becoming an endogenous RNA competitor for miR-193a-3p and ErbB receptor tyrosine kinase 4 (ERBB4) (366).
Subnetwork 8: 5-Fluorouracil, Irinotecan
5-Fluorouracil (5-FU) is a widely used therapeutic agent for treating a range of cancers, including advanced CRC (367), liver and BCs. It interferes with DNA replication by interrupting the synthesis of pyrimidine thymidine and thereby leading to cell cycle arrest or cell death (368, 369). In the 5-FU metabolic pathway, the enzymes dihydropyrimidine dehydrogenase, thymidylate synthase, thymidine phosphorylase and methylenetetrahydrofolate reductase are important to determine resistance (370). miRNAs are altered in CRC (26) and targeting tumor-associated genes (23, 371–373). Moreover, miRNAs are promising tumor biomarkers for CRC screening (27) and are also responsible for 5-fluorouracil drug resistance (374). In particular miR-587 (369), miR-195 (375), miR-149 (376), miR-203 (377), miR-129 (378), and miR-218 (209) are involved in 5-FU response. While miR-20b (379) and miR-519c (380) influence 5-FU and Irinotecan (only miR-519c) resistance in CRC. Another interesting miRNA is miR-302a, belonging to the miR-302-367 cluster, which includes miR-302b, miR-302c, miR-302a, miR-302d, and miR-367. This cluster was first identified in human embryonic stem cells (hESCs) and human embryonic carcinoma cells (hECCs) and it has been reported to help maintaining stemness and reprogramming somatic cells into induced pluripotent stem cells (381). Recently, in vitro models have pinpointed its role in chemoresistance: miR-302a exerts its function through inhibition of IGF1R and of downstream Akt signaling: events associated with enhanced 5-FU-induced cell death in colon cancer cells (370). The up-regulation of miR-96 has been reported in several cancers (382, 383) and conversely low expression levels of miR-96 have been associated with poor clinical outcomes in CRC patients (384). miR-96 modulated 5-FU sensitivity in CRC cells by promoting apoptosis through reduction of the anti-apoptotic regulator XIAP and the p53 stability regulator UBE2N (ubiquitin-conjugating enzyme E2N) (385). miR-23a antisense enhanced 5-fluorouracil chemosensitivity in CRC cells, by acting on the APAF1/Caspase-9 apoptotic pathway (386), while miR-23a over-expression provided 5-FU resistance in a subtype of CRC (387). Like let-7c, also present in this subnetwork, let-7b resulted important for development of 5-FU chemoresistance in RCC (221). miR-34a also plays a role in resistance to 5-FU and to vemurafenib (102, 103, 388). The expression profile of lncRNAs was investigated in 5-FU-resistant colon cancer cell lines and snaR was confirmed to be downregulated (389); this loss increases cell viability after 5-FU treatment, suggesting that this lncRNA has a potential role as a negative regulator in drug response (390). miR-204 is significantly attenuated in CRC (391) and has a relevant function in this cancer as tumor-suppressive miRNA, through direct targeting of HMGA2. The miR-204/HMGA2 axis notably modulated cell proliferation and positively influenced CRC sensitivity to 5-FU (392).
Subnetwork 9: Doxorubicin, Methotrexate, Etoposide, Crizotinib, Celecoxib
Most of non-coding RNAs disregulations related to doxorubicin, methotrexate and etoposide play a role in chemoresistance exacerbation or inhibition. They are involved in several pathways that regulate cell growth, autophagy, apoptosis and cell proliferation (393–401) or miR-34a (105), lnc-SCD and lnc-PTMS (402) modulates the effects of celecoxib. Both doxorubicin and etoposide block DNA replication by topoisomerase II inhibition: thus causing errors in DNA synthesis and promoting apoptosis in cancer cells. They are often used to treat cancers including breast, bladder, ovarian, prostate and leukemia (403). The human miR-135a is encoded by two genes localized on chromosomes 3 and 12. It may have contradictory effects promoting or repressing cell migration and invasion in colon, melanoma, breast and prostate cancer cell lines (404). This subnetwork shows a relation between miR-135 and miR-196b; upregulation of these two miRNAs is reflected in ABCB1 increment. This pattern conferred resistance to genotoxic agents like etoposide and doxorubicin in leukemia cancer cells (405), an interesting result that confirms the pro-oncogenic role of miR-196b. Its over-expression has been reported in different types of leukemia (406), in the maintenance of stem cell properties and chemoresistance in CRC (407), and in castrate-resistant prostate cancer (408). Novel insights in improving the effectiveness of chemotherapy emerged with miR-708, miR-101-3p, and miR-29b. Their regulation could enhance chemosensivity of drug targeted genes involved in responses like autophagy or apoptosis (172, 409, 410). miR-29b is generally the most highly expressed ncRNA in the miR-29 family. Up-regulation of miR-29b is common in the majority of human cancers where it affects tumor progression (411). miR-29b increases etoposide and paclitaxel induced toxicity in ovarian cancer, this effect being linked to Mcl-1 (410, 412). Very interesting was the case of the miR-200 family members that include miR-200c. The expression of this miRNA was inversely correlated with the chemoresistance to antioneoplastic drugs like Doxorubicin, Crizotinib and Methotrexate. miR-200c improved drug sensivity targeting TrkB and Bmi1 in BCs (151), ZEB1, and EZH2 in lung cancer cells (148, 149). Furthermore, Ham et al. found that overexpression of LUCAT1 lncRNA promotes methotrexate resistance through miR-200c (413). A very interesting loop, if considered that miR-200c up regulation contributes to restore methotrexate sensivity. The identification of ncRNA effects on cancer drugs could promote the development of novel approaches. For example, Xu et al. found that co-delivery of miR-101 and doxorubicin suppressed malignant properties of HCC (414). The role of miR-215, as well as that of his homologous miR-192 (subnetwork 6), in cancer is ambiguous. These two miRNAs exert cell growth and migration-promoting effects in gastric cancer (415) and are positive regulators of p53, playing an important role in multiple myeloma (347). Furthermore, a recent study has confirmed that miR-215 overexpression leads to the development of doxorubicin resistance in HCC and is also associated with bad prognosis in HCC patients harboring mutated p53 (416). In another case, Doxorubicin was shown to affect the subcellular localization of lncRNAs and to enhance their functional effects. For example, Shen et al. discovered that SNGH1 was retained in the nucleus as a consequence to doxorubicin treatment, in turn leading to accumulation of p53 in the nucleus and to the enhancement of p53-dependent apoptosis (417).
Subnetwork 10: Tamoxifen, Vandetanib, Alitretinoin
Tamoxifen, a selective modulator of estrogen receptor, is an effective first-line endocrine therapy that significantly improved relapse overall and relapse-free survival for many ER+ and endocrine-responsive patients. However, a significant proportion of the advanced ER+ BC patients do not respond (418). Recurrence occurs in approximately 40% of patients (419). As pinpointed in this subnetwork, seven miRNAs could sensitize cells to tamoxifen and might serve as potential therapeutic approaches for overcoming tamoxifen-resistance in BC: miR-27b, miR-375, miR-148a, miR-152, miR-206, miR-26a, miR-26b. Conversely, only three miRNAs confered tamoxifen resistance: miR-221, miR-222, miR-335. Lastly, aberrant expression of lncRNAs has also been linked to cancer progression and metastasis (56, 420). In the complex network of ER signaling, lncRNAs are emerging as critical determinants of hormone action. As opposed to miRNAs, high expression of lncRNAs, namely LINC-ROR (248, 249), MALAT1, CCAT2, was often associated with tamoxifen treatment failure in BC: their knock-down improved tamoxifen responsiveness in BC cells while uc.57 lncRNA promoted drug sensitivity. miR-27b had a different expression pattern between tamoxifen-sensitive vs. -resistant BC cell lines (421). In particular, miR-27b was found to be down-regulated in breast tumor tissues from tamoxifen-resistant patients (422) and high levels of miR-27b correlated with poor prognosis in BC (423, 424). CSC generation and EMT are essential events in tumor cell invasion and metastasis, both present in resistance to tamoxifen (425, 426). Of note, miRNAs have been associated with EMT and resistance to standard therapies. A direct target of miR-27b in modulating drug resistance and EMT is HMGB3 (427), an oncogene that can modulate drug resistance, proliferation and metastasis (428). Notably, while tamoxifen repressed miR-27b expression, estrogen induced miR-27b in BC cells (422). We already illustrated above miR-375, that can modulate the sensitivity/resistance of drug treatments in different cancers, including BC (193) and MTC (196). At the same time an anticancer treatment like alitretinoin may exert a regulatory action on miR-375 expression in BC cells (195). miR-148a and miR-152 reduced tamoxifen resistance in ER+BC via direct down-regulating the activated leukocyte cell adhesion molecule (ALCAM) (429). miR-206 was elevated in ER+BC cell lines (161) and its knock-down induced resistance to tamoxifen, while its overexpression reduced it by regulating G1/S-related proteins (430). miR-26a/b levels were lower in tamoxifen-resistant ER+BC and the inactivation of miR-26a/b decreased tamoxifen responsiveness of cancer cells (431). Additionally, miR-26 was found to be frequently downregulated in HCC and correlated with poor survival. miR-26b significantly suppressed the NF-κB signaling and dramatically enhanced chemo-sensitivity of HCC to doxorubicin by targeting TAK1 and TAB3, two positive regulators of NF-κB pathway (432). Subnetwork 10 also includes miR-221 and miR-222. These two miRNAs have a bivalent role in drug resistance across different cancer types. In this subnetwork miR-221/221 were found to enhance tamoxifen resistance (161, 163, 433). miR-335, promoted estrogen signaling, resulting in increased potency of tamoxifen. Additionally, tumor cells with acquired tamoxifen resistance did not show miR-335 nor ESR1 expression (434). The Metastasis associated in lung adenocarcinoma transcript 1 lncRNA (MALAT1), is over-expressed in several human malignancies, including ER+BC (435). High MALAT1 levels were also associated with tamoxifen treatment failure by regulating the transcription and splicing of ESR1, thus affecting ER signaling (436). Accordingly, MALAT1 may serve as an oncogenic lncRNA in pancreatic cancer, by promoting EMT, decreasing chemosensitivity to anticancer drugs and accelerating tumor angiogenesis (437). The CCAT2 lncRNA is overexpressed in BC, with the highest expression in lymph node negative patients. However, its expression levels are informative solely for a subgroup of patients, namely for lymph node positive patients that received adjuvant 5-fluorouracil, cyclophosphamide and methotrexate chemotherapy: high levels of CCAT2 suggested that patients would not benefit from CMF (438). Tamoxifen-resistant cells present a higher level of CCAT2 compared with sensitive cell, and knockdown of CCAT2 improved their response to tamoxifen (420). The levels of transcribed ultraconserved region uc.57 are lower in BC tissues than in precancerous breast tissues. uc.57 overexpression down-modulated BCL11A and reduced tamoxifen resistance in BC cells MCF7R by inhibiting the PI3K/AKT and MAPK signaling pathways (439).
Subnetwork 11: Imatinib
Imatinib (IM) is a 2-phenyl-amino-pyrimidine, an ATP-competitive tyrosine kinase inhibitor (TKI) and one of the most potent inhibitors of ABL1. Imatinib was approved for clinical treatment of CML but the problem of drug resistance encouraged the development of new TKI generations (440). Various ncRNAs have been associated with imatinib in CML, either as enhancers or inhibitors. HOX Antisense Intergenic RNA (HOTAIR) is located in the antisense strand of the HOXC gene locus, flanked by HOXC11 and HOXC12 (441). HOTAIR expression levels correlated with metastasis in BC and its loss was linked to decrease in invasion potential (442). HOTAIR lncRNA was up-regulated in CML patients with high levels of MDR1. Moreover, the knockdown of HOTAIR led to down-regulation of MDR1 resulting in higher sensitivity to imatinib; an involvement of HOTAIR in the PI3K/Akt pathway was also proposed (443). HULC is located at 6p24.3 and its transcript is a ~500 nt long, spliced and poly-adenylated lncRNA that localizes to the cytoplasm (444). The impact of HULC in hematologic malignancies is not clear yet, but it could act as a sponge for miRNA-372 in acute lymphoblastic leukemia (445). Moreover, HULC is involved in K562 cells survival and its silencing leads to increased apoptosis in CML cells by up-regulating PI3K/AKT signaling and c-Myc (446). Colorectal cancer, gastric cancer and melanoma show aberrant expression of SNHG5 (447, 448). SNHG5 lncRNA promotes imatinb resistance in CML and, although the mechanism may be complex, it seems to act as a competing endogenous RNA for miR-205-5p (449). UCA1 lncRNA located at 19p13.12, has an important role in drug resistance (114, 116). let-7i cooperates with miR-224 to revert imatinib resistance in CML (224). miR-1301 is involved in human cancers but shows an ambiguous behavior (450, 451). It can target the Ran GTPase Activating Protein 1 (RanGAP1) mRNA, as demonstrated by inverse correlation in CML patients: the RanGAP1 protein down-regulation or an increased miR-1301 are beneficial for the sensitivity to imatinib (452). miR-7 acts as an inhibitor in hepatocellular and pancreatic carcinomas (453, 454) possibly regulating the PI3K/AKT pathway, which is also downstream of BCR-ABL (455). In fact, over-expression of miR-7 in K562 cells, exhibit a significant inhibition of proliferation and increase of apoptosis via inhibition of BCR-ABL/PI3K/AKT signaling. Another report showed that miR-7 could work in synergy with imatinib to sensitize K562 (456). As the last ncRNA in this subnetwork, miR-518a is down-regulated in imatinib-resistant gastrointestinal stromal tumor (GIST) and PIK3C2A was identified as the relevant specific target (457).
Non-connected RNA and Drug Nodes
Few ncRNA and drug combinations are not connected to the main network (and also obviously not to the subnetworks). One of these drugs is dactilosib, an imidazoquinoline derivative under phase II trial that works as dual inhibitor of PI3K and mTOR. It might improve conventional drug treatments and overcome some intrinsic adverse reactions of rapamycin and its derivates (458). Deng et al. studied dactilosib in AML and discovered that it caused up-regulation of miR-1-3p and consequent down-regulation of its targets involved in apoptosis, migration and multidrugs resistance. Moreover inhibition of miR-1-3p could interfere with dactilosib anti-proliferation effects (459).
In several human cancers miR-144 and miR-451 were identified as tumor suppressor ncRNAs (460). In terms of chemoresistance, miR-144 reversed 5-FU and imatinib resistance respectively in HCC (461) and leukemia (462). In addition, miR-144 might promote cisplatin sensitivity in prostate cancer (463) and in thyroid carcinoma (464). Whereas miR-144-3p contributed to sunitinib resistance in RCC by targeting ARID1A, a cancer gene involved in chromatin remodeling (465). Reduction of ARID1A expression could also serve as a predictive biomarker for trastuzumab resistance in BC (466). Although breast and ovarian cancer have comparable levels of HER2/ErbB2 expression patterns, pertuzumab treatment is more effective in BC. Wuerkenbieke et al. investigated this effect and found miR-150 knockdown in ovarian cancer; this might contribute to enhance pertuzumab resistance (467). ncRNAs can also be related to side-effects occurring upon cancer treatment: vascular events are a serious problem in CML patients treated with tyrosine kinase inhibitors like nilotinib. Recent findings suggest that nilotinib decreases levels of miR-3121-3p resulting in higher levels of IL-1β and adhesion molecules in vascular endothelial cells. (468). miR-132-5p expression, via CYP1A2 modulation, could reduce flutamide-induced hepatic cell toxicity (469). Finally, in a matrix in vitro screen of several miRNAs and drugs in BC, miR-126 augmented the potency of CDK4/6 or PIK3CA inhibitors in MCF7 (Luminal) and MDA-MB-453 (HER2+) cell lines (470).
Author Contributions
SV contributed to design and critical revision. FC, LM, and FB performed collection and curation of data. FC assembled data and wrote the article. LM and FB contributed to network analysis. CA contributed to network analysis and writing. All authors read and approved the final manuscript.
Conflict of Interest Statement
The authors declare that the research was conducted in the absence of any commercial or financial relationships that could be construed as a potential conflict of interest.
Acknowledgments
SV is supported by Associazione Italiana Ricerca sul Cancro AIRC (IG 17063), and Italian PRIN MIUR 2010. We thank Dr. Lucia Oton Gonzalez for language revision.
Supplementary Material
The Supplementary Material for this article can be found online at: https://www.frontiersin.org/articles/10.3389/fonc.2018.00327/full#supplementary-material
Supplementary Image 1. The complete ncRNA/drug network. The figure illustrates the connected network of all drug/ncRNA interactions (edges) and 5 unconnected pairs. The network represents a human curated selection of papers listed in PubMed to identify the most important ncRNAs/cancer drugs interactions and cliques.
Supplementary Table 1. ncRNA/drug network indices. The Excel file contains the measures for all nodes (drugs/ncRNAs) in the network.
Supplementary Table 2. ncRNA/drug interaction effect on chemoresistance. This table shows, for each subnetwork, the non-coding RNAs that influenced chemoresistance, or drug sensivity, as reported in our review. Red represents inhibition, and green enhancement, of the relative process. We indicated all drugs in the subnetworks and the related ncRNAs behaviors. Keys: ↑ ncRNA up-regulation, ↓ ncRNA down-regulation.
References
1. Broxterman HJ, Gotink KJ, Verheul HMW. Understanding the causes of multidrug resistance in cancer: a comparison of doxorubicin and sunitinib. Drug Resist Updat (2009) 12:114–26. doi: 10.1016/j.drup.2009.07.001
2. Giovannetti E, Erozenci A, Smit J, Danesi R, Peters GJ. Molecular mechanisms underlying the role of microRNAs (miRNAs) in anticancer drug resistance and implications for clinical practice. Crit Rev Oncol Hematol. (2012) 81:103–22. doi: 10.1016/j.critrevonc.2011.03.010
3. Gottesman MM, Fojo T, Bates SE. Multidrug resistance in cancer: role of ATP-dependent transporters. Nat Rev Cancer (2002) 2:48–58. doi: 10.1038/nrc706
4. Fojo T. Multiple paths to a drug resistance phenotype: mutations, translocations, deletions and amplification of coding genes or promoter regions, epigenetic changes and microRNAs. Drug Resist Updat (2007) 10:59–67. doi: 10.1016/j.drup.2007.02.002
5. Kapranov P, St Laurent G, Raz T, Ozsolak F, Reynolds CP, Sorensen PHB, et al. The majority of total nuclear-encoded non-ribosomal RNA in a human cell is “dark matter” un-annotated RNA. BMC Biol. (2010) 8:149. doi: 10.1186/1741-7007-8-149
6. Bartel DP. MicroRNAs: genomics, biogenesis, mechanism, and function. Cell (2004) 116:281–97. doi: 10.1016/S0092-8674(04)00045-5
7. He L, Hannon GJ. MicroRNAs: small RNAs with a big role in gene regulation. Nat Rev Genet. (2004) 5:522–31. doi: 10.1038/nrg1379
8. Bartel DP. MicroRNAs: target recognition and regulatory functions. Cell (2009) 136:215–33. doi: 10.1016/j.cell.2009.01.002
9. Shukla GC, Singh J, Barik S. MicroRNAs: processing, maturation, target recognition and regulatory functions. Mol Cell Pharmacol. (2011) 3:83–92.
10. Baskerville S, Bartel DP. Microarray profiling of microRNAs reveals frequent coexpression with neighboring miRNAs and host genes. RNA (2005) 11:241–7. doi: 10.1261/rna.7240905
11. Bentwich I, Avniel A, Karov Y, Aharonov R, Gilad S, Barad O, et al. Identification of hundreds of conserved and nonconserved human microRNAs. Nat Genet. (2005) 37:766–70. doi: 10.1038/ng1590
12. Lee RC, Feinbaum RL, Ambros V. The C. elegans heterochronic gene lin-4 encodes small RNAs with antisense complementarity to lin-14. Cell (1993) 75:843–54.
13. Wightman B, Ha I, Ruvkun G. Posttranscriptional regulation of the heterochronic gene lin-14 by lin-4 mediates temporal pattern formation in C. elegans. Cell (1993) 75:855–62.
14. Brennecke J, Hipfner DR, Stark A, Russell RB, Cohen SM. bantam encodes a developmentally regulated microRNA that controls cell proliferation and regulates the proapoptotic gene hid in Drosophila. Cell (2003) 113:25–36. doi: 10.1016/S0092-8674(03)00231-9
15. Chen C-Z, Li L, Lodish HF, Bartel DP. MicroRNAs modulate hematopoietic lineage differentiation. Science (2004) 303:83–6. doi: 10.1126/science.1091903
16. Calin GA, Dumitru CD, Shimizu M, Bichi R, Zupo S, Noch E, et al. Frequent deletions and down-regulation of micro- RNA genes miR15 and miR16 at 13q14 in chronic lymphocytic leukemia. Proc Natl Acad Sci USA. (2002) 99:15524–9. doi: 10.1073/pnas.242606799
17. Esquela-Kerscher A, Slack FJ. Oncomirs - microRNAs with a role in cancer. Nat Rev Cancer (2006) 6:259–69. doi: 10.1038/nrc1840
18. Calin GA, Croce CM. MicroRNA signatures in human cancers. Nat Rev Cancer (2006) 6:857–66. doi: 10.1038/nrc1997
19. Kumar MS, Lu J, Mercer KL, Golub TR, Jacks T. Impaired microRNA processing enhances cellular transformation and tumorigenesis. Nat Genet. (2007) 39:673–7. doi: 10.1038/ng2003
20. Gaur A, Jewell DA, Liang Y, Ridzon D, Moore JH, Chen C, et al. Characterization of microRNA expression levels and their biological correlates in human cancer cell lines. Cancer Res. (2007) 67:2456–68. doi: 10.1158/0008-5472.CAN-06-2698
21. Croce CM. Causes and consequences of microRNA dysregulation in cancer. Nat Rev Genet. (2009) 10:704–14. doi: 10.1038/nrg2634
22. Iorio MV, Croce CM. MicroRNAs in cancer: small molecules with a huge impact. J Clin Oncol. (2009) 27:5848–56. doi: 10.1200/JCO.2009.24.0317
23. Valeri N, Braconi C, Gasparini P, Murgia C, Lampis A, Paulus-Hock V, et al. MicroRNA-135b promotes cancer progression by acting as a downstream effector of oncogenic pathways in colon cancer. Cancer Cell (2014) 25:469–83. doi: 10.1016/j.ccr.2014.03.006
24. Volinia S, Calin GA, Liu C-G, Ambs S, Cimmino A, Petrocca F, et al. A microRNA expression signature of human solid tumors defines cancer gene targets. Proc Natl Acad Sci USA. (2006) 103:2257–61. doi: 10.1073/pnas.0510565103
25. Bloomston M, Frankel WL, Petrocca F, Volinia S, Alder H, Hagan JP, et al. MicroRNA expression patterns to differentiate pancreatic adenocarcinoma from normal pancreas and chronic pancreatitis. JAMA (2007) 297:1901–8. doi: 10.1001/jama.297.17.1901
26. Schetter AJ, Leung SY, Sohn JJ, Zanetti KA, Bowman ED, Yanaihara N, et al. MicroRNA expression profiles associated with prognosis and therapeutic outcome in colon adenocarcinoma. JAMA (2008) 299:425–36. doi: 10.1001/jama.299.4.425
27. Ng EKO, Chong WWS, Jin H, Lam EKY, Shin VY, Yu J, et al. Differential expression of microRNAs in plasma of patients with colorectal cancer: a potential marker for colorectal cancer screening. Gut (2009) 58:1375–81. doi: 10.1136/gut.2008.167817
28. Calin GA, Liu C-G, Sevignani C, Ferracin M, Felli N, Dumitru CD, et al. MicroRNA profiling reveals distinct signatures in B cell chronic lymphocytic leukemias. Proc Natl Acad Sci USA. (2004) 101:11755–60. doi: 10.1073/pnas.0404432101
29. Cimmino A, Calin GA, Fabbri M, Iorio MV, Ferracin M, Shimizu M, et al. miR-15 and miR-16 induce apoptosis by targeting BCL2. Proc Natl Acad Sci USA. (2005) 102:13944–9. doi: 10.1073/pnas.0506654102
30. Valeri N, Croce CM, Fabbri M. Pathogenetic and clinical relevance of microRNAs in colorectal cancer. Cancer Genomics Proteomics (2009) 6:195–204.
31. Schepeler T, Reinert JT, Ostenfeld MS, Christensen LL, Silahtaroglu AN, Dyrskjøt L, et al. Diagnostic and prognostic microRNAs in stage II colon cancer. Cancer Res. (2008) 68:6416–24. doi: 10.1158/0008-5472.CAN-07-6110
32. Huang Z, Huang D, Ni S, Peng Z, Sheng W, Du X. Plasma microRNAs are promising novel biomarkers for early detection of colorectal cancer. Int J Cancer (2010) 127:118–26. doi: 10.1002/ijc.25007
33. Corsini LR, Bronte G, Terrasi M, Amodeo V, Fanale D, Fiorentino E, et al. The role of microRNAs in cancer: diagnostic and prognostic biomarkers and targets of therapies. Expert Opin Ther Targets (2012) 16(Suppl. 2):S103–9. doi: 10.1517/14728222.2011.650632
34. Krützfeldt J, Rajewsky N, Braich R, Rajeev KG, Tuschl T, Manoharan M, et al. Silencing of microRNAs in vivo with “antagomirs.” Nature (2005) 438:685–9. doi: 10.1038/nature04303
35. Wurdinger T, Costa FF. Molecular therapy in the microRNA era. Pharmacogenomics J. (2007) 7:297–304. doi: 10.1038/sj.tpj.6500429
36. Lu J, Getz G, Miska EA, Alvarez-Saavedra E, Lamb J, Peck D, et al. MicroRNA expression profiles classify human cancers. Nature (2005) 435:834–8. doi: 10.1038/nature03702
37. Calin GA, Ferracin M, Cimmino A, Di Leva G, Shimizu M, Wojcik SE, et al. A MicroRNA signature associated with prognosis and progression in chronic lymphocytic leukemia. N Engl J Med. (2005) 353:1793–801. doi: 10.1056/NEJMoa050995
38. Blower PE, Chung J-H, Verducci JS, Lin S, Park J-K, Dai Z, et al. MicroRNAs modulate the chemosensitivity of tumor cells. Mol Cancer Ther. (2008) 7:1–9. doi: 10.1158/1535-7163.MCT-07-0573
39. Yanaihara N, Caplen N, Bowman E, Seike M, Kumamoto K, Yi M, et al. Unique microRNA molecular profiles in lung cancer diagnosis and prognosis. Cancer Cell (2006) 9:189–98. doi: 10.1016/j.ccr.2006.01.025
40. Chen Y, Stallings RL. Differential patterns of microRNA expression in neuroblastoma are correlated with prognosis, differentiation, and apoptosis. Cancer Res. (2007) 67:976–83. doi: 10.1158/0008-5472.CAN-06-3667
41. Climent J, Dimitrow P, Fridlyand J, Palacios J, Siebert R, Albertson DG, et al. Deletion of chromosome 11q predicts response to anthracycline-based chemotherapy in early breast cancer. Cancer Res. (2007) 67:818–26. doi: 10.1158/0008-5472.CAN-06-3307
42. Blower PE, Verducci JS, Lin S, Zhou J, Chung J-H, Dai Z, et al. MicroRNA expression profiles for the NCI-60 cancer cell panel. Mol Cancer Ther. (2007) 6:1483–91. doi: 10.1158/1535-7163.MCT-07-0009
43. Erson AE, Petty EM. MicroRNAs in development and disease. Clin Genet. (2008) 74:296–306. doi: 10.1111/j.1399-0004.2008.01076.x
44. Gottesman MM, Lavi O, Hall MD, Gillet J-P. Toward a better understanding of the complexity of cancer drug resistance. Annu Rev Pharmacol Toxicol. (2016) 56:85–102. doi: 10.1146/annurev-pharmtox-010715-103111
45. Xia L, Zhang D, Du R, Pan Y, Zhao L, Sun S, et al. miR-15b and miR-16 modulate multidrug resistance by targeting BCL2 in human gastric cancer cells. Int J Cancer (2008) 123:372–379. doi: 10.1002/ijc.23501
46. Zhang H, Tang J, Li C, Kong J, Wang J, Wu Y, et al. MiR-22 regulates 5-FU sensitivity by inhibiting autophagy and promoting apoptosis in colorectal cancer cells. Cancer Lett. (2015) 356:781–90. doi: 10.1016/j.canlet.2014.10.029
47. Wang H, Zhu L-J, Yang Y-C, Wang Z-X, Wang R. MiR-224 promotes the chemoresistance of human lung adenocarcinoma cells to cisplatin via regulating G1/S transition and apoptosis by targeting p21(WAF1/CIP1). Br J Cancer (2014) 111:339–54. doi: 10.1038/bjc.2014.157
48. Mishra PJ, Humeniuk R, Mishra PJ, Longo-Sorbello GSA, Banerjee D, Bertino JR. A miR-24 microRNA binding-site polymorphism in dihydrofolate reductase gene leads to methotrexate resistance. Proc Natl Acad Sci USA. (2007) 104:13513–8. doi: 10.1073/pnas.0706217104
49. Shang Y, Zhang Z, Liu Z, Feng B, Ren G, Li K, et al. miR-508-5p regulates multidrug resistance of gastric cancer by targeting ABCB1 and ZNRD1. Oncogene (2014) 33:3267–76. doi: 10.1038/onc.2013.297
50. Puisieux A, Brabletz T, Caramel J. Oncogenic roles of EMT-inducing transcription factors. Nat Cell Biol. (2014) 16:488–94. doi: 10.1038/ncb2976
51. Rhodes LV, Martin EC, Segar HC, Miller DFB, Buechlein A, Rusch DB, et al. Dual regulation by microRNA-200b-3p and microRNA-200b-5p in the inhibition of epithelial-to-mesenchymal transition in triple-negative breast cancer. Oncotarget (2015) 6:16638–52. doi: 10.18632/oncotarget.3184
52. Reya T, Morrison SJ, Clarke MF, Weissman IL. Stem cells, cancer, and cancer stem cells. Nature (2001) 414:105–11. doi: 10.1038/35102167
53. Ponting CP, Oliver PL, Reik W. Evolution and functions of long noncoding RNAs. Cell (2009) 136:629–41. doi: 10.1016/j.cell.2009.02.006
54. Guttman M, Garber M, Levin JZ, Donaghey J, Robinson J, Adiconis X, et al. Ab initio reconstruction of cell type-specific transcriptomes in mouse reveals the conserved multi-exonic structure of lincRNAs. Nat Biotechnol. (2010) 28:503–10. doi: 10.1038/nbt.1633
55. Ma J, Dong C, Ji C. MicroRNA and drug resistance. Cancer Gene Ther. (2010) 17:523–31. doi: 10.1038/cgt.2010.18
56. Huarte M. The emerging role of lncRNAs in cancer. Nat Med. (2015) 21:1253–61. doi: 10.1038/nm.3981
57. Schmitt AM, Chang HY. Long noncoding RNAs in cancer pathways. Cancer Cell (2016) 29:452–63. doi: 10.1016/j.ccell.2016.03.010
58. Batista PJ, Chang HY. Long noncoding RNAs: cellular address codes in development and disease. Cell (2013) 152:1298–307. doi: 10.1016/j.cell.2013.02.012
59. Mercer TR, Dinger ME, Sunkin SM, Mehler MF, Mattick JS. Specific expression of long noncoding RNAs in the mouse brain. Proc Natl Acad Sci USA. (2008) 105:716–21. doi: 10.1073/pnas.0706729105
60. Goodrich JA, Kugel JF. Non-coding-RNA regulators of RNA polymerase II transcription. Nat Rev Mol Cell Biol. (2006) 7:612–6. doi: 10.1038/nrm1946
61. Ørom UA, Derrien T, Beringer M, Gumireddy K, Gardini A, Bussotti G, et al. Long noncoding RNAs with enhancer-like function in human cells. Cell (2010) 143:46–58. doi: 10.1016/j.cell.2010.09.001
62. Chen L-L, Carmichael GG. Decoding the function of nuclear long non-coding RNAs. Curr Opin Cell Biol. (2010) 22:357–64. doi: 10.1016/j.ceb.2010.03.003
63. Lipovich L, Johnson R, Lin C-Y. MacroRNA underdogs in a microRNA world: evolutionary, regulatory, and biomedical significance of mammalian long non-protein-coding RNA. Biochim Biophys Acta (2010) 1799:597–615. doi: 10.1016/j.bbagrm.2010.10.001
64. Zhao J, Sun BK, Erwin JA, Song J-J, Lee JT. Polycomb proteins targeted by a short repeat RNA to the mouse X chromosome. Science (2008) 322:750–6. doi: 10.1126/science.1163045
65. Wang C, Wang L, Ding Y, Lu X, Zhang G, Yang J, et al. LncRNA Structural characteristics in epigenetic regulation. Int J Mol Sci. (2017) 18:E2659. doi: 10.3390/ijms18122659
66. Hung T, Wang Y, Lin MF, Koegel AK, Kotake Y, Grant GD, et al. Extensive and coordinated transcription of noncoding RNAs within cell-cycle promoters. Nat Genet. (2011) 43:621–9. doi: 10.1038/ng.848
67. Ulitsky I, Shkumatava A, Jan CH, Sive H, Bartel DP. Conserved function of lincRNAs in vertebrate embryonic development despite rapid sequence evolution. Cell (2011) 147:1537–50. doi: 10.1016/j.cell.2011.11.055
68. Perez DS, Hoage TR, Pritchett JR, Ducharme-Smith AL, Halling ML, Ganapathiraju SC, et al. Long, abundantly expressed non-coding transcripts are altered in cancer. Hum Mol Genet. (2008) 17:642–55. doi: 10.1093/hmg/ddm336
69. Taft RJ, Pang KC, Mercer TR, Dinger M, Mattick JS. Non-coding RNAs: regulators of disease. J Pathol. (2010) 220:126–39. doi: 10.1002/path.2638
70. Silva JM, Perez DS, Pritchett JR, Halling ML, Tang H, Smith DI. Identification of long stress-induced non-coding transcripts that have altered expression in cancer. Genomics (2010) 95:355–62. doi: 10.1016/j.ygeno.2010.02.009
71. Gibb EA, Brown CJ, Lam WL. The functional role of long non-coding RNA in human carcinomas. Mol Cancer (2011) 10:38. doi: 10.1186/1476-4598-10-38
72. Huarte M, Rinn JL. Large non-coding RNAs: missing links in cancer? Hum Mol Genet. (2010) 19:R152–161. doi: 10.1093/hmg/ddq353
73. Dinger ME, Pang KC, Mercer TR, Crowe ML, Grimmond SM, Mattick JS. NRED: a database of long noncoding RNA expression. Nucleic Acids Res. (2009) 37:D122–126. doi: 10.1093/nar/gkn617
74. Wan L-B, Bartolomei MS. Regulation of imprinting in clusters: noncoding RNAs versus insulators. Adv Genet. (2008) 61:207–23. doi: 10.1016/S0065-2660(07)00007-7
75. Yang L, Tang Y, Xiong F, He Y, Wei F, Zhang S, et al. LncRNAs regulate cancer metastasis via binding to functional proteins. Oncotarget (2018) 9:1426–43. doi: 10.18632/oncotarget.22840
76. Ebert MS, Neilson JR, Sharp PA. MicroRNA sponges: competitive inhibitors of small RNAs in mammalian cells. Nat Methods (2007) 4:721–6. doi: 10.1038/nmeth1079
77. Yu W, Gius D, Onyango P, Muldoon-Jacobs K, Karp J, Feinberg AP, et al. Epigenetic silencing of tumour suppressor gene p15 by its antisense RNA. Nature (2008) 451:202–6. doi: 10.1038/nature06468
78. Garzon R, Volinia S, Papaioannou D, Nicolet D, Kohlschmidt J, Yan PS, et al. Expression and prognostic impact of lncRNAs in acute myeloid leukemia. Proc Natl Acad Sci USA. (2014) 111:18679–84. doi: 10.1073/pnas.1422050112
79. Zhou M, Guo M, He D, Wang X, Cui Y, Yang H, et al. A potential signature of eight long non-coding RNAs predicts survival in patients with non-small cell lung cancer. J Transl Med. (2015) 13:231. doi: 10.1186/s12967-015-0556-3
80. Cai T, Liu Y, Xiao J. Long noncoding RNA MALAT1 knockdown reverses chemoresistance to temozolomide via promoting microRNA-101 in glioblastoma. Cancer Med. (2018) 7:1404–15.doi: 10.1002/cam4.1384
81. Chen X, Sun Y-Z, Zhang D-H, Li J-Q, Yan G-Y, An J-Y, et al. NRDTD: a database for clinically or experimentally supported non-coding RNAs and drug targets associations. Database (Oxford) (2017) 2017:bax057. doi: 10.1093/database/bax057
82. Rukov JL, Wilentzik R, Jaffe I, Vinther J, Shomron N. Pharmaco-miR: linking microRNAs and drug effects. Brief Bioinform. (2014) 15:648–59. doi: 10.1093/bib/bbs082
83. Su G, Kuchinsky A, Morris JH, States DJ, Meng F. GLay: community structure analysis of biological networks. Bioinformatics (2010) 26:3135–7. doi: 10.1093/bioinformatics/btq596
84. Iorio MV, Visone R, Di Leva G, Donati V, Petrocca F, Casalini P, et al. MicroRNA signatures in human ovarian cancer. Cancer Res. (2007) 67:8699–707. doi: 10.1158/0008-5472.CAN-07-1936
85. Krichevsky AM, Gabriely G. miR-21: a small multi-faceted RNA. J Cell Mol Med. (2009) 13:39–53. doi: 10.1111/j.1582-4934.2008.00556.x
86. Pink RC, Samuel P, Massa D, Caley DP, Brooks SA, Carter DRF. The passenger strand, miR-21-3p, plays a role in mediating cisplatin resistance in ovarian cancer cells. Gynecol Oncol. (2015) 137:143–51. doi: 10.1016/j.ygyno.2014.12.042
87. Giunti L, da Ros M, Vinci S, Gelmini S, Iorio AL, Buccoliero AM, et al. Anti-miR21 oligonucleotide enhances chemosensitivity of T98G cell line to doxorubicin by inducing apoptosis. Am J Cancer Res. (2015) 5:231–42.
88. Dhayat SA, Mardin WA, Seggewiß J, Ströse AJ, Matuszcak C, Hummel R, et al. MicroRNA profiling implies new markers of gemcitabine chemoresistance in mutant p53 pancreatic ductal adenocarcinoma. PLoS ONE (2015) 10:e0143755. doi: 10.1371/journal.pone.0143755
89. Frederick PJ, Green HN, Huang JS, Egger ME, Frieboes HB, Grizzle WE, et al. Chemoresistance in ovarian cancer linked to expression of microRNAs. Biotech Histochem. (2013) 88:403–9. doi: 10.3109/10520295.2013.788736
90. Wei X, Wang W, Wang L, Zhang Y, Zhang X, Chen M, et al. MicroRNA-21 induces 5-fluorouracil resistance in human pancreatic cancer cells by regulating PTEN and PDCD4. Cancer Med. (2016) 5:693–702. doi: 10.1002/cam4.626
91. Wu Z-H, Tao Z-H, Zhang J, Li T, Ni C, Xie J, et al. MiRNA-21 induces epithelial to mesenchymal transition and gemcitabine resistance via the PTEN/AKT pathway in breast cancer. Tumour Biol. (2016) 37:7245–54. doi: 10.1007/s13277-015-4604-7
92. Yang Z, Fang S, Di Y, Ying W, Tan Y, Gu W. Modulation of NF-κB/miR-21/PTEN pathway sensitizes non-small cell lung cancer to cisplatin. PLoS ONE (2015) 10:e0121547. doi: 10.1371/journal.pone.0121547
93. Vanas V, Haigl B, Stockhammer V, Sutterlüty-Fall H. MicroRNA-21 increases proliferation and cisplatin sensitivity of osteosarcoma-derived cells. PLoS ONE (2016) 11:e0161023. doi: 10.1371/journal.pone.0161023
94. Seca H, Lima RT, Lopes-Rodrigues V, Guimaraes JE, Almeida GM, Vasconcelos MH. Targeting miR-21 induces autophagy and chemosensitivity of leukemia cells. Curr Drug Targets (2013) 14:1135–43. doi: 10.2174/13894501113149990185
95. Shen K-H, Hung J-H, Chang C-W, Weng Y-T, Wu M-J, Chen P-S. Solasodine inhibits invasion of human lung cancer cell through downregulation of miR-21 and MMPs expression. Chem Biol Interact. (2017) 268:129–35. doi: 10.1016/j.cbi.2017.03.005
96. Yang G-D, Huang T-J, Peng L-X, Yang C-F, Liu R-Y, Huang H-B, et al. Epstein-Barr Virus_Encoded LMP1 upregulates microRNA-21 to promote the resistance of nasopharyngeal carcinoma cells to cisplatin-induced apoptosis by suppressing PDCD4 and Fas-L. PLoS ONE (2013) 8:e78355. doi: 10.1371/journal.pone.0078355
97. Au Yeung CL, Co N-N, Tsuruga T, Yeung T-L, Kwan S-Y, Leung CS, et al. Exosomal transfer of stroma-derived miR21 confers paclitaxel resistance in ovarian cancer cells through targeting APAF1. Nat Commun. (2016) 7:11150. doi: 10.1038/ncomms11150
98. Cao C-L, Niu H-J, Kang S-P, Cong C-L, Kang S-R. miRNA-21 sensitizes gastrointestinal stromal tumors (GISTs) cells to Imatinib via targeting B-cell lymphoma 2 (Bcl-2). Eur Rev Med Pharmacol Sci. (2016) 20:3574–81.
99. Welch C, Chen Y, Stallings RL. MicroRNA-34a functions as a potential tumor suppressor by inducing apoptosis in neuroblastoma cells. Oncogene (2007) 26:5017–22. doi: 10.1038/sj.onc.1210293
100. Akao Y, Nakagawa Y, Hirata I, Iio A, Itoh T, Kojima K, et al. Role of anti-oncomirs miR-143 and−145 in human colorectal tumors. Cancer Gene Ther (2010) 17:398–408. doi: 10.1038/cgt.2009.88
101. Akao Y, Noguchi S, Iio A, Kojima K, Takagi T, Naoe T. Dysregulation of microRNA-34a expression causes drug-resistance to 5-FU in human colon cancer DLD-1 cells. Cancer Lett. (2011) 300:197–204. doi: 10.1016/j.canlet.2010.10.006
102. Li X, Zhao H, Zhou X, Song L. Inhibition of lactate dehydrogenase A by microRNA-34a resensitizes colon cancer cells to 5-fluorouracil. Mol Med Rep. (2015) 11:577–82. doi: 10.3892/mmr.2014.2726
103. Siemens H, Jackstadt R, Kaller M, Hermeking H. Repression of c-Kit by p53 is mediated by miR-34 and is associated with reduced chemoresistance, migration and stemness. Oncotarget (2013) 4:1399–415. doi: 10.18632/oncotarget.1202
104. Yang F, Li Q, Gong Z, Zhou L, You N, Wang S, et al. MicroRNA-34a targets Bcl-2 and sensitizes human hepatocellular carcinoma cells to sorafenib treatment. Technol Cancer Res Treat. (2014) 13:77–86. doi: 10.7785/tcrt.2012.500364
105. Chen X, Peng D, Shen Y, Liu B, Zhou H, Tao H, et al. The potential combinational effect of miR-34a with celecoxib in osteosarcoma. Anticancer Drugs (2017) 28:888–97. doi: 10.1097/CAD.0000000000000530
106. Wen D, Peng Y, Lin F, Singh RK, Mahato RI. Micellar delivery of miR-34a modulator rubone and paclitaxel in resistant prostate cancer. Cancer Res. (2017) 77:3244–54. doi: 10.1158/0008-5472.CAN-16-2355
107. Zhang Z, Kong Y, Yang W, Ma F, Zhang Y, Ji S, et al. Upregulation of microRNA-34a enhances the DDP sensitivity of gastric cancer cells by modulating proliferation and apoptosis via targeting MET. Oncol Rep. (2016) 36:2391–7. doi: 10.3892/or.2016.5016
108. Song C, Lu P, Sun G, Yang L, Wang Z, Wang Z. miR-34a sensitizes lung cancer cells to cisplatin via p53/miR-34a/MYCN axis. Biochem Biophys Res Commun. (2017) 482:22–7. doi: 10.1016/j.bbrc.2016.11.037
109. Pu Y, Zhao F, Li Y, Cui M, Wang H, Meng X, et al. The miR-34a-5p promotes the multi-chemoresistance of osteosarcoma via repression of the AGTR1 gene. BMC Cancer (2017) 17:45. doi: 10.1186/s12885-016-3002-x
110. Wang X-S, Zhang Z, Wang H-C, Cai J-L, Xu Q-W, Li M-Q, et al. Rapid identification of UCA1 as a very sensitive and specific unique marker for human bladder carcinoma. Clin Cancer Res. (2006) 12:4851–8. doi: 10.1158/1078-0432.CCR-06-0134
111. Wang F, Li X, Xie X, Zhao L, Chen W. UCA1, a non-protein-coding RNA up-regulated in bladder carcinoma and embryo, influencing cell growth and promoting invasion. FEBS Lett (2008) 582:1919–27. doi: 10.1016/j.febslet.2008.05.012
112. Wang F, Ying H-Q, He B-S, Pan Y-Q, Deng Q-W, Sun H-L, et al. Upregulated lncRNA-UCA1 contributes to progression of hepatocellular carcinoma through inhibition of miR-216b and activation of FGFR1/ERK signaling pathway. Oncotarget (2015) 6:7899–917. doi: 10.18632/oncotarget.3219
113. Huang J, Zhou N, Watabe K, Lu Z, Wu F, Xu M, et al. Long non-coding RNA UCA1 promotes breast tumor growth by suppression of p27 (Kip1). Cell Death Dis. (2014) 5:e1008. doi: 10.1038/cddis.2013.541
114. Wang H, Guan Z, He K, Qian J, Cao J, Teng L. LncRNA UCA1 in anti-cancer drug resistance. Oncotarget (2017) 8:64638–50. doi: 10.18632/oncotarget.18344
115. Wang KC, Chang HY. Molecular mechanisms of long noncoding RNAs. Mol Cell. (2011) 43:904–14. doi: 10.1016/j.molcel.2011.08.018
116. Xiao Y, Jiao C, Lin Y, Chen M, Zhang J, Wang J, et al. lncRNA UCA1 contributes to imatinib resistance by acting as a ceRNA against miR-16 in chronic myeloid leukemia cells. DNA Cell Biol. (2017) 36:18–25. doi: 10.1089/dna.2016.3533
117. Fan Y, Shen B, Tan M, Mu X, Qin Y, Zhang F, et al. Long non-coding RNA UCA1 increases chemoresistance of bladder cancer cells by regulating Wnt signaling. FEBS J (2014) 281:1750–8. doi: 10.1111/febs.12737
118. Pan J, Li X, Wu W, Xue M, Hou H, Zhai W, et al. Long non-coding RNA UCA1 promotes cisplatin/gemcitabine resistance through CREB modulating miR-196a-5p in bladder cancer cells. Cancer Lett. (2016) 382:64–76. doi: 10.1016/j.canlet.2016.08.015
119. Li X, Wu Y, Liu A, Tang X. Long non-coding RNA UCA1 enhances tamoxifen resistance in breast cancer cells through a miR-18a-HIF1α feedback regulatory loop. Tumour Biol. (2016) 37:14733–14743. doi: 10.1007/s13277-016-5348-8
120. Liu H, Wang G, Yang L, Qu J, Yang Z, Zhou X. Knockdown of Long non-coding RNA UCA1 increases the tamoxifen sensitivity of breast cancer cells through inhibition of Wnt/β-catenin pathway. PLoS ONE (2016) 11:e0168406. doi: 10.1371/journal.pone.0168406
121. Xu C-G, Yang M-F, Ren Y-Q, Wu C-H, Wang L-Q. Exosomes mediated transfer of lncRNA UCA1 results in increased tamoxifen resistance in breast cancer cells. Eur Rev Med Pharmacol Sci. (2016) 20:4362–4368.
122. Yin H, Sun Y, Wang X, Park J, Zhang Y, Li M, et al. Progress on the relationship between miR-125 family and tumorigenesis. Exp Cell Res. (2015) 339:252–60. doi: 10.1016/j.yexcr.2015.09.015
123. Sun Y-M, Lin K-Y, Chen Y-Q. Diverse functions of miR-125 family in different cell contexts. J Hematol Oncol. (2013) 6:6. doi: 10.1186/1756-8722-6-6
124. Jiang J-X, Gao S, Pan Y-Z, Yu C, Sun C-Y. Overexpression of microRNA-125b sensitizes human hepatocellular carcinoma cells to 5-fluorouracil through inhibition of glycolysis by targeting hexokinase II. Mol Med Rep. (2014) 10:995–1002. doi: 10.3892/mmr.2014.2271
125. Lu Y, Zhao X, Liu Q, Li C, Graves-Deal R, Cao Z, et al. lncRNA MIR100HG-derived miR-100 and miR-125b mediate cetuximab resistance via Wnt/β-catenin signaling. Nat Med. (2017) 23:1331–41. doi: 10.1038/nm.4424
126. Iida K, Fukushi J-I, Matsumoto Y, Oda Y, Takahashi Y, Fujiwara T, et al. miR-125b develops chemoresistance in Ewing sarcoma/primitive neuroectodermal tumor. Cancer Cell Int. (2013) 13:21. doi: 10.1186/1475-2867-13-21
127. Haemmig S, Baumgartner U, Glück A, Zbinden S, Tschan MP, Kappeler A, et al. miR-125b controls apoptosis and temozolomide resistance by targeting TNFAIP3 and NKIRAS2 in glioblastomas. Cell Death Dis. (2014) 5:e1279. doi: 10.1038/cddis.2014.245
128. Chen J, Chen Y, Chen Z. MiR-125a/b regulates the activation of cancer stem cells in paclitaxel-resistant colon cancer. Cancer Invest. (2013) 31:17–23. doi: 10.3109/07357907.2012.743557
129. Zhou M, Liu Z, Zhao Y, Ding Y, Liu H, Xi Y, et al. MicroRNA-125b confers the resistance of breast cancer cells to paclitaxel through suppression of pro-apoptotic Bcl-2 antagonist killer 1 (Bak1) expression. J Biol Chem. (2010) 285:21496–507. doi: 10.1074/jbc.M109.083337
130. Tang X, Zheng W, Ding M, Guo K, Yuan F, Feng H, et al. miR-125b acts as a tumor suppressor in chondrosarcoma cells by the sensitization to doxorubicin through direct targeting the ErbB2-regulated glucose metabolism. Drug Des Devel Ther. (2016) 10:571–83. doi: 10.2147/DDDT.S90530
131. Fan Z, Cui H, Yu H, Ji Q, Kang L, Han B, et al. MiR-125a promotes paclitaxel sensitivity in cervical cancer through altering STAT3 expression. Oncogenesis (2016) 5:e197. doi: 10.1038/oncsis.2016.1
132. Potenza N, Mosca N, Zappavigna S, Castiello F, Panella M, Ferri C, et al. MicroRNA-125a-5p Is a downstream effector of sorafenib in its antiproliferative activity toward human hepatocellular carcinoma cells. J Cell Physiol. (2017) 232:1907–13. doi: 10.1002/jcp.25744
133. Ufkin ML, Peterson S, Yang X, Driscoll H, Duarte C, Sathyanarayana P. miR-125a regulates cell cycle, proliferation, and apoptosis by targeting the ErbB pathway in acute myeloid leukemia. Leuk Res. (2014) 38:402–10. doi: 10.1016/j.leukres.2013.12.021
134. Li C, Gao Y, Zhang K, Chen J, Han S, Feng B, et al. Multiple roles of MicroRNA-100 in human cancer and its therapeutic potential. Cell Physiol Biochem. (2015) 37:2143–59. doi: 10.1159/000438572
135. Guo P, Xiong X, Zhang S, Peng D. miR-100 resensitizes resistant epithelial ovarian cancer to cisplatin. Oncol Rep. (2016) 36:3552–8. doi: 10.3892/or.2016.5140
136. Zhu Z, Wang C-P, Zhang Y-F, Nie L. MicroRNA-100 resensitizes resistant chondrosarcoma cells to cisplatin through direct targeting of mTOR. Asian Pac J Cancer Prev. (2014) 15:917–923.
137. Li Z, Li X, Yu C, Wang M, Peng F, Xiao J, et al. MicroRNA-100 regulates pancreatic cancer cells growth and sensitivity to chemotherapy through targeting FGFR3. Tumour Biol. (2014) 35:11751–9. doi: 10.1007/s13277-014-2271-8
138. Lobert S, Jefferson B, Morris K. Regulation of β-tubulin isotypes by micro-RNA 100 in MCF7 breast cancer cells. Cytoskeleton (Hoboken) (2011) 68:355–362. doi: 10.1002/cm.20517
139. Ng WL, Yan D, Zhang X, Mo Y-Y, Wang Y. Over-expression of miR-100 is responsible for the low-expression of ATM in the human glioma cell line: M059J. DNA Repair (Amst) (2010) 9:1170–5. doi: 10.1016/j.dnarep.2010.08.007
140. Petrelli A, Carollo R, Cargnelutti M, Iovino F, Callari M, Cimino D, et al. By promoting cell differentiation, miR-100 sensitizes basal-like breast cancer stem cells to hormonal therapy. Oncotarget (2015) 6:2315–30. doi: 10.18632/oncotarget.2962
141. Chen W, Liu X, Lv M, Chen L, Zhao J, Zhong S, et al. Exosomes from drug-resistant breast cancer cells transmit chemoresistance by a horizontal transfer of microRNAs. PLoS ONE (2014) 9:e95240. doi: 10.1371/journal.pone.0095240
142. Korpal M, Kang Y. The emerging role of miR-200 family of microRNAs in epithelial-mesenchymal transition and cancer metastasis. RNA Biol. (2008) 5:115–119.
143. Zhou X, Men X, Zhao R, Han J, Fan Z, Wang Y, et al. miR-200c inhibits TGF-β-induced-EMT to restore trastuzumab sensitivity by targeting ZEB1 and ZEB2 in gastric cancer. Cancer Gene Ther. (2018) doi: 10.1038/s41417-017-0005-y
144. Jiang T, Dong P, Li L, Ma X, Xu P, Zhu H, et al. MicroRNA-200c regulates cisplatin resistance by targeting ZEB2 in human gastric cancer cells. Oncol Rep. (2017) 38:151–8. doi: 10.3892/or.2017.5659
145. Sato H, Shien K, Tomida S, Okayasu K, Suzawa K, Hashida S, et al. Targeting the miR-200c/LIN28B axis in acquired EGFR-TKI resistance non-small cell lung cancer cells harboring EMT features. Sci Rep. (2017) 7:40847. doi: 10.1038/srep40847
146. Zhou G, Zhang F, Guo Y, Huang J, Xie Y, Yue S, et al. miR-200c enhances sensitivity of drug-resistant non-small cell lung cancer to gefitinib by suppression of PI3K/Akt signaling pathway and inhibites cell migration via targeting ZEB1. Biomed Pharmacother. (2017) 85:113–9. doi: 10.1016/j.biopha.2016.11.100
147. Duran GE, Wang YC, Moisan F, Francisco EB, Sikic BI. Decreased levels of baseline and drug-induced tubulin polymerisation are hallmarks of resistance to taxanes in ovarian cancer cells and are associated with epithelial-to-mesenchymal transition. Br J Cancer (2017) 116:1318–28. doi: 10.1038/bjc.2017.102
148. Shan W, Zhang X, Li M, Deng F, Zhang J. Over expression of miR-200c suppresses invasion and restores methotrexate sensitivity in lung cancer A549 cells. Gene (2016) 593:265–71. doi: 10.1016/j.gene.2016.07.038
149. Gao H-X, Yan L, Li C, Zhao L-M, Liu W. miR-200c regulates crizotinib-resistant ALK-positive lung cancer cells by reversing epithelial-mesenchymal transition via targeting ZEB1. Mol Med Rep. (2016) 14:4135–43. doi: 10.3892/mmr.2016.5770
150. Narita M, Shimura E, Nagasawa A, Aiuchi T, Suda Y, Hamada Y, et al. Chronic treatment of non-small-cell lung cancer cells with gefitinib leads to an epigenetic loss of epithelial properties associated with reductions in microRNA-155 and−200c. PLoS ONE (2017) 12:e0172115. doi: 10.1371/journal.pone.0172115
151. Kopp F, Oak PS, Wagner E, Roidl A. miR-200c sensitizes breast cancer cells to doxorubicin treatment by decreasing TrkB and Bmi1 expression. PLoS ONE (2012) 7:e50469. doi: 10.1371/journal.pone.0050469
152. Liu J, Meng T, Yuan M, Wen L, Cheng B, Liu N, et al. MicroRNA-200c delivered by solid lipid nanoparticles enhances the effect of paclitaxel on breast cancer stem cell. Int J Nanomed. (2016) 11:6713–25. doi: 10.2147/IJN.S111647
153. Chang I, Mitsui Y, Fukuhara S, Gill A, Wong DK, Yamamura S, et al. Loss of miR-200c up-regulates CYP1B1 and confers docetaxel resistance in renal cell carcinoma. Oncotarget (2015) 6:7774–87. doi: 10.18632/oncotarget.3484
154. Gao Y, Feng B, Han S, Zhang K, Chen J, Li C, et al. The Roles of MicroRNA-141 in Human cancers: from diagnosis to treatment. Cell Physiol Biochem. (2016) 38:427–48. doi: 10.1159/000438641
155. Yao Y-S, Qiu W-S, Yao R-Y, Zhang Q, Zhuang L-K, Zhou F, et al. miR-141 confers docetaxel chemoresistance of breast cancer cells via regulation of EIF4E expression. Oncol Rep. (2015) 33:2504–12. doi: 10.3892/or.2015.3866
156. Fu W-F, Chen W-B, Dai L, Yang G-P, Jiang Z-Y, Pan L, et al. Inhibition of miR-141 reverses cisplatin resistance in non-small cell lung cancer cells via upregulation of programmed cell death protein 4. Eur Rev Med Pharmacol Sci. (2016) 20:2565–72.
157. Jin Y-Y, Chen Q-J, Xu K, Ren H-T, Bao X, Ma Y-N, et al. Involvement of microRNA-141-3p in 5-fluorouracil and oxaliplatin chemo-resistance in esophageal cancer cells via regulation of PTEN. Mol Cell Biochem. (2016) 422:161–70. doi: 10.1007/s11010-016-2816-9
158. Shi L, Wu L, Chen Z, Yang J, Chen X, Yu F, et al. MiR-141 Activates Nrf2-dependent antioxidant pathway via down-regulating the expression of Keap1 conferring the resistance of hepatocellular carcinoma cells to 5-fluorouracil. Cell Physiol Biochem. (2015) 35:2333–48. doi: 10.1159/000374036
159. Li J, Yang X, Guan H, Mizokami A, Keller ET, Xu X, et al. Exosome-derived microRNAs contribute to prostate cancer chemoresistance. Int J Oncol. (2016) 49:838–46. doi: 10.3892/ijo.2016.3560
160. Garofalo M, Quintavalle C, Romano G, Croce CM, Condorelli G. miR221/222 in cancer: their role in tumor progression and response to therapy. Curr Mol Med. (2012) 12:27–33. doi: 10.2174/156652412798376170
161. Miller TE, Ghoshal K, Ramaswamy B, Roy S, Datta J, Shapiro CL, et al. MicroRNA-221/222 confers tamoxifen resistance in breast cancer by targeting p27Kip1. J Biol Chem. (2008) 283:29897–903. doi: 10.1074/jbc.M804612200
162. Wang D-D, Yang S-J, Chen X, Shen H-Y, Luo L-J, Zhang X-H, et al. miR-222 induces Adriamycin resistance in breast cancer through PTEN/Akt/p27kip1 pathway. Tumour Biol. (2016) 37:15315–24. doi: 10.1007/s13277-016-5341-2
163. Wei Y, Lai X, Yu S, Chen S, Ma Y, Zhang Y, et al. Exosomal miR-221/222 enhances tamoxifen resistance in recipient ER-positive breast cancer cells. Breast Cancer Res Treat. (2014) 147:423–31. doi: 10.1007/s10549-014-3037-0
164. Shen H, Wang D, Li L, Yang S, Chen X, Zhou S, et al. MiR-222 promotes drug-resistance of breast cancer cells to adriamycin via modulation of PTEN/Akt/FOXO1 pathway. Gene (2017) 596:110–8. doi: 10.1016/j.gene.2016.10.016
165. Rao X, Di Leva G, Li M, Fang F, Devlin C, Hartman-Frey C, et al. MicroRNA-221/222 confers breast cancer fulvestrant resistance by regulating multiple signaling pathways. Oncogene (2011) 30:1082–97. doi: 10.1038/onc.2010.487
166. Wang Y, Zhao Y, Herbst A, Kalinski T, Qin J, Wang X, et al. miR-221 mediates chemoresistance of esophageal adenocarcinoma by direct targeting of DKK2 expression. Ann Surg. (2016) 264:804–14. doi: 10.1097/SLA.0000000000001928
167. Zhao L, Zou D, Wei X, Wang L, Zhang Y, Liu S, et al. MiRNA-221-3p desensitizes pancreatic cancer cells to 5-fluorouracil by targeting RB1. Tumour Biol. (2016) 39:16053–63. doi: 10.1007/s13277-016-5445-8
168. Varambally S, Cao Q, Mani R-S, Shankar S, Wang X, Ateeq B, et al. Genomic loss of microRNA-101 leads to overexpression of histone methyltransferase EZH2 in cancer. Science (2008) 322:1695–9. doi: 10.1126/science.1165395
169. Su H, Yang J-R, Xu T, Huang J, Xu L, Yuan Y, et al. MicroRNA-101, down-regulated in hepatocellular carcinoma, promotes apoptosis and suppresses tumorigenicity. Cancer Res. (2009) 69:1135–42. doi: 10.1158/0008-5472.CAN-08-2886
170. Frankel LB, Wen J, Lees M, Høyer-Hansen M, Farkas T, Krogh A, et al. microRNA-101 is a potent inhibitor of autophagy. EMBO J. (2011) 30:4628–41. doi: 10.1038/emboj.2011.331
171. Xu Y, An Y, Wang Y, Zhang C, Zhang H, Huang C, et al. miR-101 inhibits autophagy and enhances cisplatin-induced apoptosis in hepatocellular carcinoma cells. Oncol Rep. (2013) 29:2019–24. doi: 10.3892/or.2013.2338
172. Chang Z, Huo L, Li K, Wu Y, Hu Z. Blocked autophagy by miR-101 enhances osteosarcoma cell chemosensitivity in vitro. ScientificWorldJournal (2014) 2014:794756. doi: 10.1155/2014/794756
173. Fan P, Liu L, Yin Y, Zhao Z, Zhang Y, Amponsah PS, et al. MicroRNA-101-3p reverses gemcitabine resistance by inhibition of ribonucleotide reductase M1 in pancreatic cancer. Cancer Lett. (2016) 373:130–7. doi: 10.1016/j.canlet.2016.01.038
174. Wang H, Wang L, Zhang G, Lu C, Chu H, Yang R, et al. MALAT1/miR-101-3p/MCL1 axis mediates cisplatin resistance in lung cancer. Oncotarget (2018) 9:7501–12. doi: 10.18632/oncotarget.23483
175. Wang F, Song X, Li X, Xin J, Wang S, Yang W, et al. Noninvasive visualization of microRNA-16 in the chemoresistance of gastric cancer using a dual reporter gene imaging system. PLoS ONE (2013) 8:e61792. doi: 10.1371/journal.pone.0061792
176. Venturutti L, Cordo Russo RI, Rivas MA, Mercogliano MF, Izzo F, Oakley RH, Pereyra MG, De Martino M, Proietti CJ, Yankilevich P, et al. MiR-16 mediates trastuzumab and lapatinib response in ErbB-2-positive breast and gastric cancer via its novel targets CCNJ and FUBP1. Oncogene (2016) 35:6189–6202. doi: 10.1038/onc.2016.151
177. Abraham M, Klein S, Bulvik B, Wald H, Weiss ID, Olam D, et al. The CXCR4 inhibitor BL-8040 induces the apoptosis of AML blasts by downregulating ERK, BCL-2, MCL-1 and cyclin-D1 via altered miR-15a/16-1 expression. Leukemia (2017) 31:2336–46. doi: 10.1038/leu.2017.82
178. Pickard MR, Williams GT. Molecular and cellular mechanisms of action of tumour suppressor GAS5 LncRNA. Genes (Basel) (2015) 6:484–99. doi: 10.3390/genes6030484
179. Yacqub-Usman K, Pickard MR, Williams GT. Reciprocal regulation of GAS5 lncRNA levels and mTOR inhibitor action in prostate cancer cells. Prostate (2015) 75:693–705. doi: 10.1002/pros.22952
180. Mourtada-Maarabouni M, Williams GT. Role of GAS5 noncoding RNA in mediating the effects of rapamycin and its analogues on mantle cell lymphoma cells. Clin Lymphoma Myeloma Leuk. (2014) 14:468–73. doi: 10.1016/j.clml.2014.02.011
181. Li W, Zhai L, Wang H, Liu C, Zhang J, Chen W, et al. Downregulation of LncRNA GAS5 causes trastuzumab resistance in breast cancer. Oncotarget (2016) 7:27778–86. doi: 10.18632/oncotarget.8413
182. Dong S, Qu X, Li W, Zhong X, Li P, Yang S, et al. The long non-coding RNA, GAS5, enhances gefitinib-induced cell death in innate EGFR tyrosine kinase inhibitor-resistant lung adenocarcinoma cells with wide-type EGFR via downregulation of the IGF-1R expression. J Hematol Oncol. (2015) 8:43. doi: 10.1186/s13045-015-0140-6
183. Zhang H, Guo Y, Song Y, Shang C. Long noncoding RNA GAS5 inhibits malignant proliferation and chemotherapy resistance to doxorubicin in bladder transitional cell carcinoma. Cancer Chemother Pharmacol. (2017) 79:49–55. doi: 10.1007/s00280-016-3194-4
184. Pan Y-J, Zhuang Y, Zheng J-N, Pei D-S. MiR-106a: promising biomarker for cancer. Bioorg Med Chem Lett. (2016) 26:5373–7. doi: 10.1016/j.bmcl.2016.10.042
185. Rao Y, Shi H, Ji M, Chen C. MiR-106a targets Mcl-1 to suppress cisplatin resistance of ovarian cancer A2780 cells. J Huazhong Univ Sci Technol Med Sci. (2013) 33:567–72. doi: 10.1007/s11596-013-1160-5
186. Li H, Xu H, Shen H, Li H. microRNA-106a modulates cisplatin sensitivity by targeting PDCD4 in human ovarian cancer cells. Oncol Lett. (2014) 7:183–88. doi: 10.3892/ol.2013.1644
187. Ma Y, Li X, Cheng S, Wei W, Li Y. MicroRNA-106a confers cisplatin resistance in non-small cell lung cancer A549 cells by targeting adenosine triphosphatase-binding cassette A1. Mol Med Rep. (2015) 11:625–32. doi: 10.3892/mmr.2014.2688
188. Rothschild SI, Gautschi O, Batliner J, Gugger M, Fey MF, Tschan MP. MicroRNA-106a targets autophagy and enhances sensitivity of lung cancer cells to Src inhibitors. Lung Cancer. (2017) 107:73–83. doi: 10.1016/j.lungcan.2016.06.004
189. Huh JH, Kim TH, Kim K, Song J-A, Jung YJ, Jeong J-Y, et al. Dysregulation of miR-106a and miR-591 confers paclitaxel resistance to ovarian cancer. Br J Cancer (2013) 109:452–61. doi: 10.1038/bjc.2013.305
190. Wang R, Li Y, Hou Y, Yang Q, Chen S, Wang X, et al. The PDGF-D/miR-106a/Twist1 pathway orchestrates epithelial-mesenchymal transition in gemcitabine resistance hepatoma cells. Oncotarget (2015) 6:7000–10. doi: 10.18632/oncotarget.3193
191. de Souza Rocha Simonini P, Breiling A, Gupta N, Malekpour M, Youns M, Omranipour R, et al. Epigenetically deregulated microRNA-375 is involved in a positive feedback loop with estrogen receptor alpha in breast cancer cells. Cancer Res. (2010) 70:9175–84. doi: 10.1158/0008-5472.CAN-10-1318
192. Ward A, Balwierz A, Zhang JD, Küblbeck M, Pawitan Y, Hielscher T, et al. Re-expression of microRNA-375 reverses both tamoxifen resistance and accompanying EMT-like properties in breast cancer. Oncogene (2013) 32:1173–82. doi: 10.1038/onc.2012.128
193. Fu H, Fu L, Xie C, Zuo W-S, Liu Y-S, et al. miR-375 inhibits cancer stem cell phenotype and tamoxifen resistance by degrading HOXB3 in human ER-positive breast cancer. Oncol Rep. (2017) 37:1093–9. doi: 10.3892/or.2017.5360
194. Ye X-M, Zhu H-Y, Bai W-D, Wang T, Wang L, Chen Y, et al. Epigenetic silencing of miR-375 induces trastuzumab resistance in HER2-positive breast cancer by targeting IGF1R. BMC Cancer (2014) 14:134. doi: 10.1186/1471-2407-14-134
195. Perri M, Caroleo MC, Liu N, Gallelli L, De Sarro G, Kagechika H, et al. 9-cis Retinoic acid modulates myotrophin expression and its miR in physiological and pathophysiological cell models. Exp Cell Res. (2017) 354:25–30. doi: 10.1016/j.yexcr.2017.03.022
196. Lassalle S, Zangari J, Popa A, Ilie M, Hofman V, Long E, et al. MicroRNA-375/SEC23A as biomarkers of the in vitro efficacy of vandetanib. Oncotarget (2016) 7:30461–78. doi: 10.18632/oncotarget.8458
197. Perri F, Pezzullo L, Chiofalo MG, Lastoria S, Di Gennaro F, Scarpati GDV, et al. Targeted therapy: a new hope for thyroid carcinomas. Crit Rev Oncol Hematol (2015) 94:55–63. doi: 10.1016/j.critrevonc.2014.10.012
198. Zhou N, Qu Y, Xu C, Tang Y. Upregulation of microRNA-375 increases the cisplatin-sensitivity of human gastric cancer cells by regulating ERBB2. Exp Ther Med. (2016) 11:625–30. doi: 10.3892/etm.2015.2920
199. Sachdeva M, Zhu S, Wu F, Wu H, Walia V, Kumar S, et al. p53 represses c-Myc through induction of the tumor suppressor miR-145. Proc Natl Acad Sci USA. (2009) 106:3207–12. doi: 10.1073/pnas.0808042106
200. Zhan M, Zhao X, Wang H, Chen W, Xu S, Wang W, et al. miR-145 sensitizes gallbladder cancer to cisplatin by regulating multidrug resistance associated protein 1. Tumour Biol. (2016) 37:10553–62. doi: 10.1007/s13277-016-4957-6
201. Gao M, Miao L, Liu M, Li C, Yu C, Yan H, et al. miR-145 sensitizes breast cancer to doxorubicin by targeting multidrug resistance-associated protein-1. Oncotarget (2016) 7:59714–26. doi: 10.18632/oncotarget.10845
202. Zhu X, Li Y, Xie C, Yin X, Liu Y, Cao Y, et al. miR-145 sensitizes ovarian cancer cells to paclitaxel by targeting Sp1 and Cdk6. Int J Cancer (2014) 135:1286–96. doi: 10.1002/ijc.28774
203. Ju B-L, Chen Y-B, Zhang W-Y, Yu C-H, Zhu D-Q, Jin J. miR-145 regulates chemoresistance in hepatocellular carcinoma via epithelial mesenchymal transition. Cell Mol Biol (Noisy-le-grand) (2015) 61:12–16.
204. Amin ND, Bai G, Klug JR, Bonanomi D, Pankratz MT, Gifford WD, et al. Loss of motoneuron-specific microRNA-218 causes systemic neuromuscular failure. Science (2015) 350:1525–29. doi: 10.1126/science.aad2509
205. Volinia S, Bertagnolo V, Grassilli S, Brugnoli F, Manfrini M, Galasso M, et al. Levels of miR-126 and miR-218 are elevated in ductal carcinoma in situ (DCIS) and inhibit malignant potential of DCIS derived cells. Oncotarget (2018) 9:23543–53. doi: 10.18632/oncotarget.25261
206. Zarogoulidis P, Petanidis S, Kioseoglou E, Domvri K, Anestakis D, et al. MiR-205 and miR-218 expression is associated with carboplatin chemoresistance and regulation of apoptosis via Mcl-1 and Survivin in lung cancer cells. Cell Signal. (2015) 27:1576–88. doi: 10.1016/j.cellsig.2015.04.009
207. Zhu W, Shao Y, Peng Y. MicroRNA-218 inhibits tumor growth and increases chemosensitivity to CDDP treatment by targeting BCAT1 in prostate cancer. Mol Carcinog (2017) 56:1570–77. doi: 10.1002/mc.22612
208. Ran X, Yang J, Liu C, Zhou P, Xiao L, Zhang K. MiR-218 inhibits HMGB1-mediated autophagy in endometrial carcinoma cells during chemotherapy. Int J Clin Exp Pathol. (2015) 8:6617–26.
209. Li P-L, Zhang X, Wang L-L, Du L-T, Yang Y-M, Li J, et al. MicroRNA-218 is a prognostic indicator in colorectal cancer and enhances 5-fluorouracil-induced apoptosis by targeting BIRC5. Carcinogenesis (2015) 36:1484–93. doi: 10.1093/carcin/bgv145
210. Takamizawa J, Konishi H, Yanagisawa K, Tomida S, Osada H, Endoh H, et al. Reduced expression of the let-7 microRNAs in human lung cancers in association with shortened postoperative survival. Cancer Res. (2004) 64:3753–6. doi: 10.1158/0008-5472.CAN-04-0637
211. Zhang H-H, Wang X-J, Li G-X, Yang E, Yang N-M. Detection of let-7a microRNA by real-time PCR in gastric carcinoma. World J Gastroenterol. (2007) 13:2883–8. doi: 10.3748/wjg.v13.i20.2883
212. Akao Y, Nakagawa Y, Naoe T. let-7 microRNA functions as a potential growth suppressor in human colon cancer cells. Biol Pharm Bull. (2006) 29:903–6. doi: 10.1248/bpb.29.903
213. Sampson VB, Rong NH, Han J, Yang Q, Aris V, Soteropoulos P, et al. MicroRNA let-7a down-regulates MYC and reverts MYC-induced growth in Burkitt lymphoma cells. Cancer Res. (2007) 67:9762–70. doi: 10.1158/0008-5472.CAN-07-2462
214. Wu J, Li S, Jia W, Deng H, Chen K, Zhu L, et al. Reduced Let-7a is associated with chemoresistance in primary breast cancer. PLoS ONE (2015) 10:e0133643. doi: 10.1371/journal.pone.0133643
215. Chen Y, Jacamo R, Konopleva M, Garzon R, Croce C, Andreeff M. CXCR4 downregulation of let-7a drives chemoresistance in acute myeloid leukemia. J Clin Invest. (2013) 123:2395–407. doi: 10.1172/JCI66553
216. Xiao G, Wang X, Yu Y. CXCR4/Let-7a axis regulates metastasis and chemoresistance of pancreatic cancer cells through targeting HMGA2. Cell Physiol Biochem. (2017) 43:840–51. doi: 10.1159/000481610
217. Bhutia YD, Hung SW, Krentz M, Patel D, Lovin D, Manoharan R, et al. Differential processing of let-7a precursors influences RRM2 expression and chemosensitivity in pancreatic cancer: role of LIN-28 and SET oncoprotein. PLoS ONE (2013) 8:e53436. doi: 10.1371/journal.pone.0053436
218. Liu Y, Yin B, Zhang C, Zhou L, Fan J. Hsa-let-7a functions as a tumor suppressor in renal cell carcinoma cell lines by targeting c-myc. Biochem Biophys Res Commun. (2012) 417:371–5. doi: 10.1016/j.bbrc.2011.11.119
219. Guo Y, Yan K, Fang J, Qu Q, Zhou M, Chen F. Let-7b expression determines response to chemotherapy through the regulation of cyclin D1 in glioblastoma. J Exp Clin Cancer Res. (2013) 32:41. doi: 10.1186/1756-9966-32-41
220. Cai J, Yang C, Yang Q, Ding H, Jia J, Guo J, et al. Deregulation of let-7e in epithelial ovarian cancer promotes the development of resistance to cisplatin. Oncogenesis (2013) 2:e75. doi: 10.1038/oncsis.2013.39
221. Peng J, Mo R, Ma J, Fan J. let-7b and let-7c are determinants of intrinsic chemoresistance in renal cell carcinoma. World J Surg Oncol. (2015) 13:175. doi: 10.1186/s12957-015-0596-4
222. Ljungberg B, Cowan NC, Hanbury DC, Hora M, Kuczyk MA, Merseburger AS, et al. EAU guidelines on renal cell carcinoma: the 2010 update. Eur Urol. (2010) 58:398–406. doi: 10.1016/j.eururo.2010.06.032
223. Zhan M, Qu Q, Wang G, Zhou H. Let-7c sensitizes acquired cisplatin-resistant A549 cells by targeting ABCC2 and Bcl-XL. Pharmazie (2013) 68:955–61.
224. Zhou H, Li Y, Liu B, Shan Y, Li Y, Zhao L, et al. Downregulation of miR-224 and let-7i contribute to cell survival and chemoresistance in chronic myeloid leukemia cells by regulating ST3GAL IV expression. Gene (2017) 626:106–18. doi: 10.1016/j.gene.2017.05.030
225. Yang X, Chen Y, Chen L. The versatile role of microRNA-30a in human cancer. Cell Physiol Biochem. (2017) 41:1616–32. doi: 10.1159/000471111
226. Sestito R, Cianfrocca R, Rosanò L, Tocci P, Semprucci E, Di Castro V, et al. miR-30a inhibits endothelin A receptor and chemoresistance in ovarian carcinoma. Oncotarget (2016) 7:4009–23. doi: 10.18632/oncotarget.6546
227. Meng F, Wang F, Wang L, Wong SCC, Cho WCS, Chan LWC. MiR-30a-5p overexpression may overcome EGFR-inhibitor resistance through regulating PI3K/AKT signaling pathway in non-small cell lung cancer cell lines. Front Genet. (2016) 7:197. doi: 10.3389/fgene.2016.00197
228. Yang C, Tabatabaei SN, Ruan X, Hardy P. The dual regulatory role of MiR-181a in breast cancer. Cell Physiol Biochem. (2017) 44:843–56. doi: 10.1159/000485351
229. Armstrong CM, Liu C, Lou W, Lombard AP, Evans CP, Gao AC. MicroRNA-181a promotes docetaxel resistance in prostate cancer cells. Prostate (2017) 77:1020–8. doi: 10.1002/pros.23358
230. Chen Y, Ke G, Han D, Liang S, Yang G, Wu X. MicroRNA-181a enhances the chemoresistance of human cervical squamous cell carcinoma to cisplatin by targeting PRKCD. Exp Cell Res. (2014) 320:12–20. doi: 10.1016/j.yexcr.2013.10.014
231. Liu M, Wang J, Huang H, Hou J, Zhang B, Wang A. miR-181a-Twist1 pathway in the chemoresistance of tongue squamous cell carcinoma. Biochem Biophys Res Commun. (2013) 441:364–70. doi: 10.1016/j.bbrc.2013.10.051
232. Wei Q, Lei R, Hu G. Roles of miR-182 in sensory organ development and cancer. Thorac Cancer (2015) 6:2–9. doi: 10.1111/1759-7714.12164
233. Wang Y-Q, Guo R-D, Guo R-M, Sheng W, Yin L-R. MicroRNA-182 promotes cell growth, invasion, and chemoresistance by targeting programmed cell death 4 (PDCD4) in human ovarian carcinomas. J Cell Biochem. (2013) 114:1464–73. doi: 10.1002/jcb.24488
234. Ning F, Wang F, Li M, Yu Z-S, Hao Y, Chen S. MicroRNA-182 modulates chemosensitivity of human non-small cell lung cancer to cisplatin by targeting PDCD4. Diagn Pathol. (2014) 9:143. doi: 10.1186/1746-1596-9-143
235. Qin J, Luo M, Qian H, Chen W. Upregulated miR-182 increases drug resistance in cisplatin-treated HCC cell by regulating TP53INP1. Gene (2014) 538:342–7. doi: 10.1016/j.gene.2013.12.043
236. Olaru AV, Yamanaka S, Vazquez C, Mori Y, Cheng Y, Abraham JM, et al. MicroRNA-224 negatively regulates p21 expression during late neoplastic progression in inflammatory bowel disease. Inflamm Bowel Dis. (2013) 19:471–80. doi: 10.1097/MIB.0b013e31827e78eb
237. Zhao H, Bi T, Qu Z, Jiang J, Cui S, Wang Y. Expression of miR-224-5p is associated with the original cisplatin resistance of ovarian papillary serous carcinoma. Oncol Rep. (2014) 32:1003–12. doi: 10.3892/or.2014.3311
238. Geng S, Gu L, Ju F, Zhang H, Wang Y, Tang H, et al. MicroRNA-224 promotes the sensitivity of osteosarcoma cells to cisplatin by targeting Rac1. J Cell Mol Med. (2016) 20:1611–19. doi: 10.1111/jcmm.12852
239. Amankwatia EB, Chakravarty P, Carey FA, Weidlich S, Steele RJC, Munro AJ, et al. MicroRNA-224 is associated with colorectal cancer progression and response to 5-fluorouracil-based chemotherapy by KRAS-dependent and -independent mechanisms. Br J Cancer (2015) 112:1480–90. doi: 10.1038/bjc.2015.125
240. Jiang H, Zhang G, Wu J-H, Jiang C-P. Diverse roles of miR-29 in cancer (review). Oncol Rep. (2014) 31:1509–16. doi: 10.3892/or.2014.3036
241. Shen H, Li L, Yang S, Wang D, Zhong S, Zhao J, et al. MicroRNA-29a contributes to drug-resistance of breast cancer cells to adriamycin through PTEN/AKT/GSK3β signaling pathway. Gene (2016) 593:84–90. doi: 10.1016/j.gene.2016.08.016
242. Muluhngwi P, Krishna A, Vittitow SL, Napier JT, Richardson KM, Ellis M, et al. Tamoxifen differentially regulates miR-29b-1 and miR-29a expression depending on endocrine-sensitivity in breast cancer cells. Cancer Lett. (2017) 388:230–8. doi: 10.1016/j.canlet.2016.12.007
243. Pan Y, Li C, Chen J, Zhang K, Chu X, Wang R, et al. The emerging roles of long noncoding RNA ROR (lincRNA-ROR) and its possible mechanisms in human cancers. Cell Physiol Biochem. (2016) 40:219–29. doi: 10.1159/000452539
244. Eades G, Wolfson B, Zhang Y, Li Q, Yao Y, Zhou Q. lincRNA-RoR and miR-145 regulate invasion in triple-negative breast cancer via targeting ARF6. Mol Cancer Res. (2015) 13:330–8. doi: 10.1158/1541-7786.MCR-14-0251
245. Zhang H-Y, Liang F, Zhang J-W, Wang F, Wang L, Kang X-G. Effects of long noncoding RNA-ROR on tamoxifen resistance of breast cancer cells by regulating microRNA-205. Cancer Chemother Pharmacol. (2017) 79:327–37. doi: 10.1007/s00280-016-3208-2
246. Li Y, Jiang B, Zhu H, Qu X, Zhao L, Tan Y, et al. Inhibition of long non-coding RNA ROR reverses resistance to Tamoxifen by inducing autophagy in breast cancer. Tumour Biol. (2017) 39:1010428317705790. doi: 10.1177/1010428317705790
247. Takahashi K, Yan IK, Kogure T, Haga H, Patel T. Extracellular vesicle-mediated transfer of long non-coding RNA ROR modulates chemosensitivity in human hepatocellular cancer. FEBS Open Bio. (2014) 4:458–67. doi: 10.1016/j.fob.2014.04.007
248. Ayers D, Vandesompele J. Influence of microRNAs and long non-coding RNAs in cancer chemoresistance. Genes (Basel) (2017) 8:E95. doi: 10.3390/genes8030095
249. Sordella R, Bell DW, Haber DA, Settleman J. Gefitinib-sensitizing EGFR mutations in lung cancer activate anti-apoptotic pathways. Science (2004) 305:1163–7. doi: 10.1126/science.1101637
250. Ma P, Zhang M, Nie F, Huang Z, He J, Li W, et al. Transcriptome analysis of EGFR tyrosine kinase inhibitors resistance associated long noncoding RNA in non-small cell lung cancer. Biomed Pharmacother. (2017) 87:20–6. doi: 10.1016/j.biopha.2016.12.079
251. Flamini V, Jiang WG, Cui Y. Therapeutic role of MiR-140-5p for the treatment of non-small cell lung cancer. Anticancer Res. (2017) 37:4319–27. doi: 10.21873/anticanres.11825
252. Zhou S, Yu L, Xiong M, Dai G. LncRNA SNHG12 promotes tumorigenesis and metastasis in osteosarcoma by upregulating Notch2 by sponging miR-195-5p. Biochem Biophys Res Commun. (2018) 495:1822–32. doi: 10.1016/j.bbrc.2017.12.047
253. Wang P, Chen D, Ma H, Li Y. LncRNA SNHG12 contributes to multidrug resistance through activating the MAPK/Slug pathway by sponging miR-181a in non-small cell lung cancer. Oncotarget (2017) 8:84086–101. doi: 10.18632/oncotarget.20475
254. Hou Q, Ruan H, Gilbert J, Wang G, Ma Q, Yao W-D, Man H-Y. MicroRNA miR124 is required for the expression of homeostatic synaptic plasticity. Nat Commun. (2015) 6:10045. doi: 10.1038/ncomms10045
255. Zhang F, Wang B, Long H, Yu J, Li F, Hou H, Yang Q. Decreased miR-124-3p expression prompted breast cancer cell progression mainly by targeting beclin-1. Clin Lab. (2016) 62:1139–45.
256. Hu F-Y, Cao X-N, Xu Q-Z, Deng Y, Lai S-Y, Ma J, et al. miR-124 modulates gefitinib resistance through SNAI2 and STAT3 in non-small cell lung cancer. J Huazhong Univ Sci Technol. Med Sci. (2016) 36:839–45. doi: 10.1007/s11596-016-1672-x
257. He X-H, Zhu W, Yuan P, Jiang S, Li D, Zhang H-W, et al. miR-155 downregulates ErbB2 and suppresses ErbB2-induced malignant transformation of breast epithelial cells. Oncogene (2016) 35:6015–25. doi: 10.1038/onc.2016.132
258. Dasari S, Tchounwou PB. Cisplatin in cancer therapy: molecular mechanisms of action. Eur J Pharmacol. (2014) 740:364–78. doi: 10.1016/j.ejphar.2014.07.025
259. Stordal B, Davey M. Understanding cisplatin resistance using cellular models. IUBMB Life (2007) 59:696–9. doi: 10.1080/15216540701636287
260. Wang Y, Zhang L, Zheng X, Zhong W, Tian X, Yin B, et al. Long non-coding RNA LINC00161 sensitises osteosarcoma cells to cisplatin-induced apoptosis by regulating the miR-645-IFIT2 axis. Cancer Lett. (2016) 382:137–46. doi: 10.1016/j.canlet.2016.08.024
261. Zhang Z, Zhang L, Yin Z-Y, Fan X-L, Hu B, Wang L-Q, et al. miR-107 regulates cisplatin chemosensitivity of A549 non small cell lung cancer cell line by targeting cyclin dependent kinase 8. Int J Clin Exp Pathol. (2014) 7:7236–41.
262. Bourguignon LYW, Wong G, Shiina M. Up-regulation of histone methyltransferase, DOT1L, by matrix hyaluronan promotes MicroRNA-10 expression leading to tumor cell invasion and chemoresistance in cancer stem cells from head and neck squamous cell carcinoma. J Biol Chem. (2016) 291:10571–85. doi: 10.1074/jbc.M115.700021
263. Li W, Wang W, Ding M, Zheng X, Ma S, Wang X. MiR-1244 sensitizes the resistance of non-small cell lung cancer A549 cell to cisplatin. Cancer Cell Int. (2016) 16:30. doi: 10.1186/s12935-016-0305-6
264. Li B, Chen H, Wu N, Zhang W-J, Shang L-X. Deregulation of miR-128 in ovarian cancer promotes cisplatin resistance. Int J Gynecol Cancer (2014) 24:1381–8. doi: 10.1097/IGC.0000000000000252
265. Sui C, Meng F, Li Y, Jiang Y. miR-148b reverses cisplatin-resistance in non-small cell cancer cells via negatively regulating DNA (cytosine-5)-methyltransferase 1(DNMT1) expression. J Transl Med. (2015) 13:132. doi: 10.1186/s12967-015-0488-y
266. Tung M-C, Lin P-L, Cheng Y-W, Wu D-W, Yeh S-D, Chen C-Y, et al. Reduction of microRNA-184 by E6 oncoprotein confers cisplatin resistance in lung cancer via increasing Bcl-2. Oncotarget (2016) 7:32362–74. doi: 10.18632/oncotarget.8708
267. Pei K, Zhu J-J, Wang C-E, Xie Q-L, Guo J-Y. MicroRNA-185-5p modulates chemosensitivity of human non-small cell lung cancer to cisplatin via targeting ABCC1. Eur Rev Med Pharmacol Sci. (2016) 20:4697–704.
268. Zhu X, Shen H, Yin X, Long L, Xie C, Liu Y, et al. miR-186 regulation of Twist1 and ovarian cancer sensitivity to cisplatin. Oncogene (2016) 35:323–32. doi: 10.1038/onc.2015.84
269. Huang K, Chen J, Yang M-S, Tang Y-J, Pan F. Inhibition of Src by microRNA-23b increases the cisplatin sensitivity of chondrosarcoma cells. Cancer Biomark (2017) 18:231–9. doi: 10.3233/CBM-160102
270. Shi L, Chen Z-G, Wu L-L, Zheng J-J, Yang J-R, Chen X-F, et al. miR-340 reverses cisplatin resistance of hepatocellular carcinoma cell lines by targeting Nrf2-dependent antioxidant pathway. Asian Pac J Cancer Prev. (2014) 15:10439–44.
271. Li N, Yang L, Wang H, Yi T, Jia X, Chen C, Xu P. MiR-130a and MiR-374a function as novel regulators of cisplatin resistance in human ovarian cancer A2780 cells. PLoS ONE (2015) 10:e0128886. doi: 10.1371/journal.pone.0128886
272. Chen X, Jiang Y, Huang Z, Li D, Chen X, Cao M, et al. miRNA-378 reverses chemoresistance to cisplatin in lung adenocarcinoma cells by targeting secreted clusterin. Sci Rep. (2016) 6:19455. doi: 10.1038/srep19455
273. Hao G-J, Hao H-J, Ding Y-H, Wen H, Li X-F, Wang Q-R, et al. Suppression of EIF4G2 by miR-379 potentiates the cisplatin chemosensitivity in nonsmall cell lung cancer cells. FEBS Lett. (2017) 591:636–45. doi: 10.1002/1873-3468.12566
274. Zhang Y, Xu W, Ni P, Li A, Zhou J, Xu S. MiR-99a and MiR-491 regulate cisplatin resistance in human gastric cancer cells by targeting CAPNS1. Int J Biol Sci. (2016) 12:1437–47. doi: 10.7150/ijbs.16529
275. Zhao H, Yu X, Ding Y, Zhao J, Wang G, Wu X, et al. MiR-770-5p inhibits cisplatin chemoresistance in human ovarian cancer by targeting ERCC2. Oncotarget (2016) 7:53254–68. doi: 10.18632/oncotarget.10736
276. Chen X, Zhang Y, Shi Y, Lian H, Tu H, Han S, et al. MiR-873 acts as a novel sensitizer of glioma cells to cisplatin by targeting Bcl-2. Int J Oncol. (2015) 47:1603–11. doi: 10.3892/ijo.2015.3143
277. Wang Q, Shi S, He W, Padilla MT, Zhang L, Wang X, et al. Retaining MKP1 expression and attenuating JNK-mediated apoptosis by RIP1 for cisplatin resistance through miR-940 inhibition. Oncotarget (2014) 5:1304–14. doi: 10.18632/oncotarget.1798
278. He J, Yu J-J, Xu Q, Wang L, Zheng JZ, Liu L-Z, et al. Downregulation of ATG14 by EGR1-MIR152 sensitizes ovarian cancer cells to cisplatin-induced apoptosis by inhibiting cyto-protective autophagy. Autophagy (2015) 11:373–84. doi: 10.1080/15548627.2015.1009781
279. Kim E-A, Kim TG, Sung E-G, Song I-H, Kim J-Y, Doh K-O, et al. miR-148a increases the sensitivity to cisplatin by targeting Rab14 in renal cancer cells. Int J Oncol. (2017) 50:984–92. doi: 10.3892/ijo.2017.3851
280. Wang Q, Chen W, Bai L, Chen W, Padilla MT, Lin AS, et al. Receptor-interacting protein 1 increases chemoresistance by maintaining inhibitor of apoptosis protein levels and reducing reactive oxygen species through a microRNA-146a-mediated catalase pathway. J Biol Chem. (2014) 289:5654–63. doi: 10.1074/jbc.M113.526152
281. Shi H, Pu J, Zhou X-L, Ning Y-Y, Bai C. Silencing long non-coding RNA ROR improves sensitivity of non-small-cell lung cancer to cisplatin resistance by inhibiting PI3K/Akt/mTOR signaling pathway. Tumour Biol. (2017) 39:1010428317697568. doi: 10.1177/1010428317697568
282. Su Z, Zhao J, Rong Z, Geng W, Wang Z. MiR-451, a potential prognostic biomarker and tumor suppressor for gastric cancer. Int J Clin Exp Pathol. (2015) 8:9154–60.
283. Cheng D, Xu Y, Sun C, He Z. MicroRNA-451 sensitizes lung cancer cells to cisplatin through regulation of Mcl-1. Mol Cell Biochem. (2016) 423:85–91. doi: 10.1007/s11010-016-2827-6
284. Soltani I, Douzi K, Gharbi H, Benhassine I, Teber M, Amouri H, et al. Downregulation of miR-451 in Tunisian chronic myeloid leukemia patients: potential implication in imatinib resistance. Hematology (2017) 22:201–7. doi: 10.1080/10245332.2016.1252020
285. Mendell JT. miRiad roles for the miR-17-92 cluster in development and disease. Cell (2008) 133:217–22. doi: 10.1016/j.cell.2008.04.001
286. Zhou D, Wan Y, Xie D, Wang Y, Wei J, Yan Q, et al. DNMT1 mediates chemosensitivity by reducing methylation of miRNA-20a promoter in glioma cells. Exp Mol Med. (2015) 47:e182. doi: 10.1038/emm.2015.57
287. Zhu M, Zhou X, Du Y, Huang Z, Zhu J, Xu J, et al. miR-20a induces cisplatin resistance of a human gastric cancer cell line via targeting CYLD. Mol Med Rep. (2016) 14:1742–50. doi: 10.3892/mmr.2016.5413
288. Wang X, Guo H, Yao B, Helms J. miR-15b inhibits cancer-initiating cell phenotypes and chemoresistance of cisplatin by targeting TRIM14 in oral tongue squamous cell cancer. Oncol Rep. (2017) 37:2720–6. doi: 10.3892/or.2017.5532
289. Zhao Z, Zhang L, Yao Q, Tao Z. miR-15b regulates cisplatin resistance and metastasis by targeting PEBP4 in human lung adenocarcinoma cells. Cancer Gene Ther. (2015) 22:108–14. doi: 10.1038/cgt.2014.73
290. Chen W, Yang Y, Chen B, Lu P, Zhan L, Yu Q, et al. MiR-136 targets E2F1 to reverse cisplatin chemosensitivity in glioma cells. J Neurooncol. (2014) 120:43–53. doi: 10.1007/s11060-014-1535-x
291. Zhao H, Liu S, Wang G, Wu X, Ding Y, Guo G, et al. Expression of miR-136 is associated with the primary cisplatin resistance of human epithelial ovarian cancer. Oncol Rep. (2015) 33:591–8. doi: 10.3892/or.2014.3640
292. Li J, Wang Y, Song Y, Fu Z, Yu W. miR-27a regulates cisplatin resistance and metastasis by targeting RKIP in human lung adenocarcinoma cells. Mol Cancer (2014) 13:193. doi: 10.1186/1476-4598-13-193
293. Tanaka K, Miyata H, Sugimura K, Fukuda S, Kanemura T, Yamashita K, et al. miR-27 is associated with chemoresistance in esophageal cancer through transformation of normal fibroblasts to cancer-associated fibroblasts. Carcinogenesis (2015) 36:894–903. doi: 10.1093/carcin/bgv067
294. Liu G, Yang D, Rupaimoole R, Pecot CV, Sun Y, Mangala LS, et al. Augmentation of response to chemotherapy by microRNA-506 through regulation of RAD51 in serous ovarian cancers. J Natl Cancer Inst. (2015) 107: djv108. doi: 10.1093/jnci/djv108
295. Meng F, Qian L, Lv L, Ding B, Zhou G, Cheng X, et al. miR-193a-3p regulation of chemoradiation resistance in oesophageal cancer cells via the PSEN1 gene. Gene (2016) 579:139–45. doi: 10.1016/j.gene.2015.12.060
296. Jacques C, Calleja LR, Baud'huin M, Quillard T, Heymann D, Lamoureux F, et al. miRNA-193a-5p repression of p73 controls Cisplatin chemoresistance in primary bone tumors. Oncotarget (2016) 7:54503–14. doi: 10.18632/oncotarget.10950
297. Fan Q, Hu X, Zhang H, Wang S, Zhang H, You C, et al. MiR-193a-3p is an important tumour suppressor in lung cancer and directly targets KRAS. Cell Physiol Biochem. (2017) 44:1311–24. doi: 10.1159/000485491
298. Gu S, Chan W-Y. Flexible and versatile as a chameleon-sophisticated functions of microRNA-199a. Int J Mol Sci. (2012) 13:8449–66. doi: 10.3390/ijms13078449
299. Liu MX, Siu MKY, Liu SS, Yam JWP, Ngan HYS, Chan DW. Epigenetic silencing of microRNA-199b-5p is associated with acquired chemoresistance via activation of JAG1-Notch1 signaling in ovarian cancer. Oncotarget (2014) 5:944–58. doi: 10.18632/oncotarget.1458
300. Fan X, Zhou S, Zheng M, Deng X, Yi Y, Huang T. MiR-199a-3p enhances breast cancer cell sensitivity to cisplatin by downregulating TFAM (TFAM). Biomed Pharmacother. (2017) 88:507–14. doi: 10.1016/j.biopha.2017.01.058
301. Hang Q, Sun R, Jiang C, Li Y. Notch 1 promotes cisplatin-resistant gastric cancer formation by upregulating lncRNA AK022798 expression. Anticancer Drugs (2015) 26:632–40. doi: 10.1097/CAD.0000000000000227
302. Yang Y, Li H, Hou S, Hu B, Liu J, Wang J. The noncoding RNA expression profile and the effect of lncRNA AK126698 on cisplatin resistance in non-small-cell lung cancer cell. PLoS ONE (2013) 8:e65309. doi: 10.1371/journal.pone.0065309
303. Yan H, Xia JY, Feng FZ. Long non-coding RNA ENST00000457645 reverses cisplatin resistance in CP70 ovarian cancer cells. Genet Mol Res. (2017) 16. doi: 10.4238/gmr16019411
304. Zhou Y, Zhang X, Klibanski A. MEG3 noncoding RNA: a tumor suppressor. J Mol Endocrinol. (2012) 48:R45–53. doi: 10.1530/JME-12-0008
305. Liu J, Wan L, Lu K, Sun M, Pan X, Zhang P, et al. The long noncoding RNA MEG3 contributes to cisplatin resistance of human lung adenocarcinoma. PLoS ONE (2015) 10:e0114586. doi: 10.1371/journal.pone.0114586
306. Kruer TL, Dougherty SM, Reynolds L, Long E, de Silva T, Lockwood WW, et al. Expression of the lncRNA Maternally Expressed Gene 3 (MEG3) contributes to the control of lung cancer cell proliferation by the Rb pathway. PLoS ONE (2016) 11:e0166363. doi: 10.1371/journal.pone.0166363
307. Wang Y, Cheng N, Luo J. Downregulation of lncRNA ANRIL represses tumorigenicity and enhances cisplatin-induced cytotoxicity via regulating microRNA let-7a in nasopharyngeal carcinoma. J Biochem Mol Toxicol. (2017) 31:e21904. doi: 10.1002/jbt.21904
308. Jordan MA, Wilson L. Microtubules as a target for anticancer drugs. Nat Rev Cancer (2004) 4:253–65. doi: 10.1038/nrc1317
309. Fujita Y, Yagishita S, Hagiwara K, Yoshioka Y, Kosaka N, Takeshita F, et al. The clinical relevance of the miR-197/CKS1B/STAT3-mediated PD-L1 network in chemoresistant non-small-cell lung cancer. Mol Ther. (2015) 23:717–27. doi: 10.1038/mt.2015.10
310. Wu H, Huang M, Lu M, Zhu W, Shu Y, Cao P, et al. Regulation of microtubule-associated protein tau (MAPT) by miR-34c-5p determines the chemosensitivity of gastric cancer to paclitaxel. Cancer Chemother Pharmacol. (2013) 71:1159–71. doi: 10.1007/s00280-013-2108-y
311. Weiner-Gorzel K, Dempsey E, Milewska M, McGoldrick A, Toh V, Walsh A, et al. Overexpression of the microRNA miR-433 promotes resistance to paclitaxel through the induction of cellular senescence in ovarian cancer cells. Cancer Med. (2015) 4:745–58. doi: 10.1002/cam4.409
312. Peng X, Cao P, He D, Han S, Zhou J, Tan G, et al. MiR-634 sensitizes nasopharyngeal carcinoma cells to paclitaxel and inhibits cell growth both in vitro and in vivo. Int J Clin Exp Pathol. (2014) 7:6784–91.
313. Ren S, Li G, Liu C, Cai T, Su Z, Wei M, et al. Next generation deep sequencing identified a novel lncRNA n375709 associated with paclitaxel resistance in nasopharyngeal carcinoma. Oncol Rep. (2016) 36:1861–7. doi: 10.3892/or.2016.4981
314. Bar-Eli M. Searching for the “melano-miRs”: miR-214 drives melanoma metastasis. EMBO J. (2011) 30:1880–1. doi: 10.1038/emboj.2011.132
315. Phatak P, Byrnes KA, Mansour D, Liu L, Cao S, Li R, et al. Overexpression of miR-214-3p in esophageal squamous cancer cells enhances sensitivity to cisplatin by targeting survivin directly and indirectly through CUG-BP1. Oncogene (2016) 35:2087–97. doi: 10.1038/onc.2015.271
316. Tsai K-W, Liao Y-L, Wu C-W, Hu L-Y, Li S-C, Chan W-C, et al. Aberrant hypermethylation of miR-9 genes in gastric cancer. Epigenetics (2011) 6:1189–97. doi: 10.4161/epi.6.10.16535
317. Li X, Pan Q, Wan X, Mao Y, Lu W, Xie X, et al. Methylation-associated Has-miR-9 deregulation in paclitaxel- resistant epithelial ovarian carcinoma. BMC Cancer (2015) 15:509. doi: 10.1186/s12885-015-1509-1
318. Munoz JL, Rodriguez-Cruz V, Rameshwar P. High expression of miR-9 in CD133+ glioblastoma cells in chemoresistance to temozolomide. J Cancer Stem Cell Res. (2015) 3:e1003. doi: 10.14343/JCSCR.2015.3e1003
319. Cloonan N, Brown MK, Steptoe AL, Wani S, Chan WL, Forrest ARR, Kolle G, Gabrielli B, Grimmond SM. The miR-17-5p microRNA is a key regulator of the G1/S phase cell cycle transition. Genome Biol. (2008) 9:R127. doi: 10.1186/gb-2008-9-8-r127
320. Liao X-H, Xiang Y, Yu C-X, Li J-P, Li H, Nie Q, et al. STAT3 is required for MiR-17-5p-mediated sensitization to chemotherapy-induced apoptosis in breast cancer cells. Oncotarget (2017) 8:15763–74. doi: 10.18632/oncotarget.15000
321. Llovet JM, Ricci S, Mazzaferro V, Hilgard P, Gane E, Blanc J-F, et al. Sorafenib in advanced hepatocellular carcinoma. N Engl J Med. (2008) 359:378–90. doi: 10.1056/NEJMoa0708857
322. Lu A-Q, Lv B, Qiu F, Wang X-Y, Cao X-H. Upregulation of miR-137 reverses sorafenib resistance and cancer-initiating cell phenotypes by degrading ANT2 in hepatocellular carcinoma. Oncol Rep. (2017) 37:2071–8. doi: 10.3892/or.2017.5498
323. Xu J, Lin H, Li G, Sun Y, Chen J, Shi L, et al. The miR-367-3p increases sorafenib chemotherapy efficacy to suppress hepatocellular carcinoma metastasis through altering the androgen receptor signals. EBioMed. (2016) 12:55–67. doi: 10.1016/j.ebiom.2016.07.013
324. Tang S, Tan G, Jiang X, Han P, Zhai B, Dong X, et al. An artificial lncRNA targeting multiple miRNAs overcomes sorafenib resistance in hepatocellular carcinoma cells. Oncotarget (2016) 7:73257–69. doi: 10.18632/oncotarget.12304
325. Azumi J, Tsubota T, Sakabe T, Shiota G. miR-181a induces sorafenib resistance of hepatocellular carcinoma cells through downregulation of RASSF1 expression. Cancer Sci. (2016) 107:1256–62. doi: 10.1111/cas.13006
326. Xu Y, Huang J, Ma L, Shan J, Shen J, Yang Z, et al. MicroRNA-122 confers sorafenib resistance to hepatocellular carcinoma cells by targeting IGF-1R to regulate RAS/RAF/ERK signaling pathways. Cancer Lett. (2016) 371:171–81. doi: 10.1016/j.canlet.2015.11.034
327. Kishikawa T, Otsuka M, Tan PS, Ohno M, Sun X, Yoshikawa T, et al. Decreased miR122 in hepatocellular carcinoma leads to chemoresistance with increased arginine. Oncotarget (2015) 6:8339–52. doi: 10.18632/oncotarget.3234
328. Jin W, Chen L, Cai X, Zhang Y, Zhang J, Ma D, et al. Long non-coding RNA TUC338 is functionally involved in sorafenib-sensitized hepatocarcinoma cells by targeting RASAL1. Oncol Rep. (2017) 37:273–80. doi: 10.3892/or.2016.5248
329. Xu Z, Yang F, Wei D, Liu B, Chen C, Bao Y, et al. Long noncoding RNA-SRLR elicits intrinsic sorafenib resistance via evoking IL-6/STAT3 axis in renal cell carcinoma. Oncogene (2017) 36:1965–77. doi: 10.1038/onc.2016.356
330. Eisenhauer EA, Vermorken JB. The taxoids. Comparative clinical pharmacology and therapeutic potential. Drugs (1998) 55:5–30.
331. Xiao Y, Li X, Wang H, Wen R, He J, Tang J. Epigenetic regulation of miR-129-2 and its effects on the proliferation and invasion in lung cancer cells. J Cell Mol Med. (2015) 19:2172–80. doi: 10.1111/jcmm.12597
332. Lu C, Shan Z, Li C, Yang L. MiR-129 regulates cisplatin-resistance in human gastric cancer cells by targeting P-gp. Biomed Pharmacother. (2017) 86:450–6. doi: 10.1016/j.biopha.2016.11.139
333. Zhang Y, Wang Y, Wei Y, Li M, Yu S, Ye M, et al. MiR-129-3p promotes docetaxel resistance of breast cancer cells via CP110 inhibition. Sci Rep. (2015) 5:15424. doi: 10.1038/srep15424
334. Zhang J, Zhang H, da Chen L, Sun DW, Mao C, Fei Chen W, et al. β-elemene reverses chemoresistance of breast cancer via regulating MDR-related microRNA expression. Cell Physiol Biochem. (2014) 34:2027–37. doi: 10.1159/000366398
335. Pan B, Feng B, Chen Y, Huang G, Wang R, Chen L, et al. MiR-200b regulates autophagy associated with chemoresistance in human lung adenocarcinoma. Oncotarget (2015) 6:32805–20. doi: 10.18632/oncotarget.5352
336. Hilberg F, Tontsch-Grunt U, Baum A, Le AT, Doebele RC, Lieb S, et al. Triple angiokinase inhibitor nintedanib directly inhibits tumor cell growth and induces tumor shrinkage via blocking oncogenic receptor tyrosine kinases. J Pharmacol Exp Ther. (2018) 364:494–503. doi: 10.1124/jpet.117.244129
337. Popat S, Mellemgaard A, Fahrbach K, Martin A, Rizzo M, Kaiser R, et al. Nintedanib plus docetaxel as second-line therapy in patients with non-small-cell lung cancer: a network meta-analysis. Future Oncol. (2015) 11:409–20. doi: 10.2217/fon.14.290
338. Nishijima N, Seike M, Soeno C, Chiba M, Miyanaga A, Noro R, et al. miR-200/ZEB axis regulates sensitivity to nintedanib in non-small cell lung cancer cells. Int J Oncol. (2016) 48:937–44. doi: 10.3892/ijo.2016.3331
339. Chen D, Huang J, Zhang K, Pan B, Chen J, De W, et al. MicroRNA-451 induces epithelial-mesenchymal transition in docetaxel-resistant lung adenocarcinoma cells by targeting proto-oncogene c-Myc. Eur J Cancer (2014) 50:3050–67. doi: 10.1016/j.ejca.2014.09.008
340. Miyoshi J, Toden S, Yoshida K, Toiyama Y, Alberts SR, Kusunoki M, et al. MiR-139-5p as a novel serum biomarker for recurrence and metastasis in colorectal cancer. Sci Rep. (2017) 7:43393. doi: 10.1038/srep43393
341. Zhang H, Sun D-W, Mao L, Zhang J, Jiang L-H, Li J, et al. MiR-139-5p inhibits the biological function of breast cancer cells by targeting Notch1 and mediates chemosensitivity to docetaxel. Biochem Biophys Res Commun. (2015) 465:702–13. doi: 10.1016/j.bbrc.2015.08.053
342. Liu H, Yin Y, Hu Y, Feng Y, Bian Z, Yao S, et al. miR-139-5p sensitizes colorectal cancer cells to 5-fluorouracil by targeting NOTCH-1. Pathol Res Pract. (2016) 212:643–9. doi: 10.1016/j.prp.2016.04.011
343. Chen J, Zhang K, Song H, Wang R, Chu X, Chen L. Long noncoding RNA CCAT1 acts as an oncogene and promotes chemoresistance in docetaxel-resistant lung adenocarcinoma cells. Oncotarget (2016) 7:62474–89. doi: 10.18632/oncotarget.11518
344. Liang C, Wang Z, Li Y-Y, Yu B-H, Zhang F, Li H-Y. miR-33a suppresses the nuclear translocation of β-catenin to enhance gemcitabine sensitivity in human pancreatic cancer cells. Tumour Biol. (2015) 36:9395–403. doi: 10.1007/s13277-015-3679-5
345. You L, Chang D, Du H-Z, Zhao Y-P. Genome-wide screen identifies PVT1 as a regulator of Gemcitabine sensitivity in human pancreatic cancer cells. Biochem Biophys Res Commun. (2011) 407:1–6. doi: 10.1016/j.bbrc.2011.02.027
346. Cao J, He Y, Liu H-Q, Wang S-B, Zhao B-C, Cheng Y-S. MicroRNA 192 regulates chemo-resistance of lung adenocarcinoma for gemcitabine and cisplatin combined therapy by targeting Bcl-2. Int J Clin Exp Med. (2015) 8:12397–403.
347. Pichiorri F, Suh S-S, Rocci A, De Luca L, Taccioli C, Santhanam R, et al. Downregulation of p53-inducible microRNAs 192, 194, and 215 impairs the p53/MDM2 autoregulatory loop in multiple myeloma development. Cancer Cell (2010) 18:367–81. doi: 10.1016/j.ccr.2010.09.005
348. Stojcheva N, Schechtmann G, Sass S, Roth P, Florea A-M, Stefanski A, et al. MicroRNA-138 promotes acquired alkylator resistance in glioblastoma by targeting the Bcl-2-interacting mediator BIM. Oncotarget (2016) 7:12937–50. doi: 10.18632/oncotarget.7346
349. Kozinn SI, Harty NJ, Delong JM, Deliyiannis C, Logvinenko T, Summerhayes IC, et al. MicroRNA profile to predict gemcitabine resistance in bladder carcinoma cell lines. Genes Cancer (2013) 4:61–9. doi: 10.1177/1947601913484495
350. Xu C, Fu H, Gao L, Wang L, Wang W, Li J, et al. BCR-ABL/GATA1/miR-138 mini circuitry contributes to the leukemogenesis of chronic myeloid leukemia. Oncogene (2014) 33:44–54. doi: 10.1038/onc.2012.557
351. Gregory PA, Bert AG, Paterson EL, Barry SC, Tsykin A, Farshid G, et al. The miR-200 family and miR-205 regulate epithelial to mesenchymal transition by targeting ZEB1 and SIP1. Nat Cell Biol. (2008) 10:593–601. doi: 10.1038/ncb1722
352. Singh S, Chitkara D, Kumar V, Behrman SW, Mahato RI. miRNA profiling in pancreatic cancer and restoration of chemosensitivity. Cancer Lett. (2013) 334:211–20. doi: 10.1016/j.canlet.2012.10.008
353. Takiuchi D, Eguchi H, Nagano H, Iwagami Y, Tomimaru Y, Wada H, et al. Involvement of microRNA-181b in the gemcitabine resistance of pancreatic cancer cells. Pancreatology (2013) 13:517–23. doi: 10.1016/j.pan.2013.06.007
354. Wang J, Sai K, Chen F, Chen Z. miR-181b modulates glioma cell sensitivity to temozolomide by targeting MEK1. Cancer Chemother Pharmacol. (2013) 72:147–58. doi: 10.1007/s00280-013-2180-3
355. Huang X, Le Q-T, Giaccia AJ. MiR-210–micromanager of the hypoxia pathway. Trends Mol Med. (2010) 16:230–7. doi: 10.1016/j.molmed.2010.03.004
356. Lee D, Sun S, Zhang XQ, Zhang PD, Ho ASW, Kiang KMY, et al. MicroRNA-210 and endoplasmic reticulum chaperones in the regulation of chemoresistance in glioblastoma. J Cancer (2015) 6:227–32. doi: 10.7150/jca.10765
357. Li Z, Zhao X, Zhou Y, Liu Y, Zhou Q, Ye H, et al. The long non-coding RNA HOTTIP promotes progression and gemcitabine resistance by regulating HOXA13 in pancreatic cancer. J Transl Med. (2015) 13:84. doi: 10.1186/s12967-015-0442-z
358. Wu DI, Wang T, Ren C, Liu L, Kong D, Jin X, et al. Downregulation of BC200 in ovarian cancer contributes to cancer cell proliferation and chemoresistance to carboplatin. Oncol Lett. (2016) 11:1189–94. doi: 10.3892/ol.2015.3983
359. Vitiello M, Tuccoli A, D'Aurizio R, Sarti S, Giannecchini L, Lubrano S, et al. Context-dependent miR-204 and miR-211 affect the biological properties of amelanotic and melanotic melanoma cells. Oncotarget (2017) 8:25395–417. doi: 10.18632/oncotarget.15915
360. Deeds J, Cronin F, Duncan LM. Patterns of melastatin mRNA expression in melanocytic tumors. Hum Pathol. (2000) 31:1346–56.
361. Fattore L, Mancini R, Acunzo M, Romano G, Laganà A, Pisanu ME, et al. miR-579-3p controls melanoma progression and resistance to target therapy. Proc Natl Acad Sci USA. (2016) 113:E5005–13. doi: 10.1073/pnas.1607753113
362. Sha A, Abadi S, Gill S. Utilization of capecitabine plus oxaliplatin and 5-fluorouracil/folinic acid plus oxaliplatin in the adjuvant treatment of stage IIB and stage III colon cancer: a multi-centre, retrospective, chart review study. J Oncol Pharm Pract. (2017) 1078155217718381. doi: 10.1177/1078155217718381
363. Hu J, Cai G, Xu Y, Cai S. The Plasma microRNA miR-1914* and−1915 Suppresses chemoresistant in colorectal cancer patients by down-regulating NFIX. Curr Mol Med. (2016) 16:70–82.
364. Zhang Y, Hu X, Miao X, Zhu K, Cui S, Meng Q, et al. MicroRNA-425-5p regulates chemoresistance in colorectal cancer cells via regulation of Programmed Cell Death 10. J Cell Mol Med. (2016) 20:360–9. doi: 10.1111/jcmm.12742
365. Tan S, Shi H, Ba M, Lin S, Tang H, Zeng X, et al. miR-409-3p sensitizes colon cancer cells to oxaliplatin by inhibiting Beclin-1-mediated autophagy. Int J Mol Med. (2016) 37:1030–8. doi: 10.3892/ijmm.2016.2492
366. Linc00152 Functions as a competing endogenous RNA to confer oxaliplatin resistance and holds prognostic values in colon cancer. Available online at: https://www.ncbi.nlm.nih.gov/pubmed/?term=27633443 (Accessed May 1, 2018)
367. Efficacy of adjuvant fluorouracil and folinic acid in colon cancer. International Multicentre Pooled Analysis of Colon Cancer Trials (IMPACT) investigators. Lancet (1995) 345:939–44.
368. Longley DB, Harkin DP, Johnston PG. 5-fluorouracil: mechanisms of action and clinical strategies. Nat Rev Cancer (2003) 3:330–8. doi: 10.1038/nrc1074
369. Zhang Y, Talmon G, Wang J. MicroRNA-587 antagonizes 5-FU-induced apoptosis and confers drug resistance by regulating PPP2R1B expression in colorectal cancer. Cell Death Dis. (2015) 6:e1845. doi: 10.1038/cddis.2015.200
370. Liu N, Li J, Zhao Z, Han J, Jiang T, Chen Y, et al. MicroRNA-302a enhances 5-fluorouracil-induced cell death in human colon cancer cells. Oncol Rep. (2017) 37:631–9. doi: 10.3892/or.2016.5237
371. Huang Z, Huang S, Wang Q, Liang L, Ni S, Wang L, et al. MicroRNA-95 promotes cell proliferation and targets sorting Nexin 1 in human colorectal carcinoma. Cancer Res. (2011) 71:2582–9. doi: 10.1158/0008-5472.CAN-10-3032
372. Wang Q, Huang Z, Guo W, Ni S, Xiao X, Wang L, et al. microRNA-202-3p inhibits cell proliferation by targeting ADP-ribosylation factor-like 5A in human colorectal carcinoma. Clin Cancer Res. (2014) 20:1146–57. doi: 10.1158/1078-0432.CCR-13-1023
373. Zhang Y, Geng L, Talmon G, Wang J. MicroRNA-520g confers drug resistance by regulating p21 expression in colorectal cancer. J Biol Chem. (2015) 290:6215–25. doi: 10.1074/jbc.M114.620252
374. Deng J, Wang Y, Lei J, Lei W, Xiong JP. Insights into the involvement of noncoding RNAs in 5-fluorouracil drug resistance. Tumour Biol. (2017) 39:1010428317697553. doi: 10.1177/1010428317697553
375. Kim C, Hong Y, Lee H, Kang H, Lee EK. MicroRNA-195 desensitizes HCT116 human colon cancer cells to 5-fluorouracil. Cancer Lett. (2018) 412:264–71. doi: 10.1016/j.canlet.2017.10.022
376. Liu X, Xie T, Mao X, Xue L, Chu X, Chen L. MicroRNA-149 Increases the sensitivity of colorectal cancer cells to 5-Fluorouracil by targeting forkhead box transcription factor FOXM1. Cell Physiol Biochem. (2016) 39:617–29. doi: 10.1159/000445653
377. Li T, Gao F, Zhang X-P. miR-203 enhances chemosensitivity to 5-fluorouracil by targeting thymidylate synthase in colorectal cancer. Oncol Rep. (2015) 33:607–14. doi: 10.3892/or.2014.3646
378. Karaayvaz M, Zhai H, Ju J. miR-129 promotes apoptosis and enhances chemosensitivity to 5-fluorouracil in colorectal cancer. Cell Death Dis. (2013) 4:e659. doi: 10.1038/cddis.2013.193
379. Fu Q, Cheng J, Zhang J, Zhang Y, Chen X, Luo S, et al. miR-20b reduces 5-FU resistance by suppressing the ADAM9/EGFR signaling pathway in colon cancer. Oncol Rep. (2017) 37:123–30. doi: 10.3892/or.2016.5259
380. To KKW, Leung WW, Ng SSM. Exploiting a novel miR-519c-HuR-ABCG2 regulatory pathway to overcome chemoresistance in colorectal cancer. Exp Cell Res. (2015) 338:222–31. doi: 10.1016/j.yexcr.2015.09.011
381. Wang Y, Baskerville S, Shenoy A, Babiarz JE, Baehner L, Blelloch R. Embryonic stem cell-specific microRNAs regulate the G1-S transition and promote rapid proliferation. Nat Genet. (2008) 40:1478–83. doi: 10.1038/ng.250
382. Mattie MD, Benz CC, Bowers J, Sensinger K, Wong L, Scott GK, et al. Optimized high-throughput microRNA expression profiling provides novel biomarker assessment of clinical prostate and breast cancer biopsies. Mol Cancer (2006) 5:24. doi: 10.1186/1476-4598-5-24
383. Hamfjord J, Stangeland AM, Hughes T, Skrede ML, Tveit KM, Ikdahl T, et al. Differential expression of miRNAs in colorectal cancer: comparison of paired tumor tissue and adjacent normal mucosa using high-throughput sequencing. PLoS ONE (2012) 7:e34150. doi: 10.1371/journal.pone.0034150
384. Ress AL, Stiegelbauer V, Winter E, Schwarzenbacher D, Kiesslich T, Lax S, et al. MiR-96-5p influences cellular growth and is associated with poor survival in colorectal cancer patients. Mol Carcinog. (2015) 54:1442–50. doi: 10.1002/mc.22218
385. Kim S-A, Kim I, Yoon SK, Lee EK, Kuh H-J. Indirect modulation of sensitivity to 5-fluorouracil by microRNA-96 in human colorectal cancer cells. Arch Pharm Res. (2015) 38:239–48. doi: 10.1007/s12272-014-0528-9
386. Shang J, Yang F, Wang Y, Wang Y, Xue G, Mei Q, et al. MicroRNA-23a antisense enhances 5-fluorouracil chemosensitivity through APAF-1/caspase-9 apoptotic pathway in colorectal cancer cells. J Cell Biochem. (2014) 115:772–84. doi: 10.1002/jcb.24721
387. Li X, Li X, Liao D, Wang X, Wu Z, Nie J, et al. Elevated microRNA-23a Expression enhances the chemoresistance of colorectal cancer cells with microsatellite instability to 5-fluorouracil by directly targeting ABCF1. Curr Protein Pept Sci. (2015) 16:301–9.
388. Vergani E, Di Guardo L, Dugo M, Rigoletto S, Tragni G, Ruggeri R, et al. Overcoming melanoma resistance to vemurafenib by targeting CCL2-induced miR-34a, miR-100 and miR-125b. Oncotarget (2016) 7:4428–41. doi: 10.18632/oncotarget.6599
389. Parrott AM, Tsai M, Batchu P, Ryan K, Ozer HL, Tian B, et al. The evolution and expression of the snaR family of small non-coding RNAs. Nucleic Acids Res. (2011) 39:1485–500. doi: 10.1093/nar/gkq856
390. Lee H, Kim C, Ku J-L, Kim W, Yoon SK, Kuh H-J, et al. A long non-coding RNA snaR contributes to 5-fluorouracil resistance in human colon cancer cells. Mol Cells (2014) 37:540–6. doi: 10.14348/molcells.2014.0151
391. Yin Y, Zhang B, Wang W, Fei B, Quan C, Zhang J, et al. miR-204-5p inhibits proliferation and invasion and enhances chemotherapeutic sensitivity of colorectal cancer cells by downregulating RAB22A. Clin Cancer Res. (2014) 20:6187–99. doi: 10.1158/1078-0432.CCR-14-1030
392. Wu H, Liang Y, Shen L, Shen L. MicroRNA-204 modulates colorectal cancer cell sensitivity in response to 5-fluorouracil-based treatment by targeting high mobility group protein A2. Biol Open (2016) 5:563–70. doi: 10.1242/bio.015008
393. Zheng D, Dai Y, Wang S, Xing X. MicroRNA-299-3p promotes the sensibility of lung cancer to doxorubicin through directly targeting ABCE1. Int J Clin Exp Pathol. (2015) 8:10072–81.
394. Jiang M, Huang O, Xie Z, Wu S, Zhang X, Shen A, et al. A novel long non-coding RNA-ARA: adriamycin resistance-associated. Biochem Pharmacol. (2014) 87:254–83. doi: 10.1016/j.bcp.2013.10.020
395. Zhou J, Wu S, Chen Y, Zhao J, Zhang K, Wang J, et al. microRNA-143 is associated with the survival of ALDH1+CD133+ osteosarcoma cells and the chemoresistance of osteosarcoma. Exp Biol Med. (2015) 240:867–75. doi: 10.1177/1535370214563893
396. Zhang Y, Duan G, Feng S. MicroRNA-301a modulates doxorubicin resistance in osteosarcoma cells by targeting AMP-activated protein kinase alpha 1. Biochem Biophys Res Commun. (2015) 459:367–73. doi: 10.1016/j.bbrc.2015.02.101
397. Hu H, Li S, Cui X, Lv X, Jiao Y, Yu F, et al. The overexpression of hypomethylated miR-663 induces chemotherapy resistance in human breast cancer cells by targeting heparin sulfate proteoglycan 2 (HSPG2). J Biol Chem. (2013) 288:10973–85. doi: 10.1074/jbc.M112.434340
398. Zhao L, Wang Y, Jiang L, He M, Bai X, Yu L, et al. MiR-302a/b/c/d cooperatively sensitizes breast cancer cells to adriamycin via suppressing P-glycoprotein(P-gp) by targeting MAP/ERK kinase kinase 1 (MEKK1). J Exp Clin Cancer Res. (2016) 35:25. doi: 10.1186/s13046-016-0300-8
399. Lv J, Fu Z, Shi M, Xia K, Ji C, Xu P, et al. Systematic analysis of gene expression pattern in has-miR-760 overexpressed resistance of the MCF-7 human breast cancer cell to doxorubicin. Biomed Pharmacother. (2015) 69:162–9. doi: 10.1016/j.biopha.2014.11.028
400. Zhang C-L, Zhu K-P, Shen G-Q, Zhu Z-S. A long non-coding RNA contributes to doxorubicin resistance of osteosarcoma. Tumour Biol. (2016) 37:2737–48. doi: 10.1007/s13277-015-4130-7
401. Li C, Gao Y, Li Y, Ding D. TUG1 mediates methotrexate resistance in colorectal cancer via miR-186/CPEB2 axis. Biochem Biophys Res Commun. (2017) 491:552–7. doi: 10.1016/j.bbrc.2017.03.042
402. Song B, Shu Z-B, Du J, Ren J-C, Feng Y. Anti-cancer effect of low dose of celecoxib may be associated with lnc-SCD-1:13 and lnc-PTMS-1:3 but not COX-2 in NCI-N87 cells. Oncol Lett. (2017) 14:1775–9. doi: 10.3892/ol.2017.6316
403. Pommier Y, Leo E, Zhang H, Marchand C. DNA topoisomerases and their poisoning by anticancer and antibacterial drugs. Chem Biol. (2010) 17:421–33. doi: 10.1016/j.chembiol.2010.04.012
404. Tribollet V, Barenton B, Kroiss A, Vincent S, Zhang L, Forcet C, et al. miR-135a Inhibits the Invasion of Cancer Cells via Suppression of ERRα. PLoS ONE (2016) 11:e0156445. doi: 10.1371/journal.pone.0156445
405. Ho T-T, He X, Mo Y-Y, Beck WT. Transient resistance to DNA damaging agents is associated with expression of microRNAs-135b and−196b in human leukemia cell lines. Int J Biochem Mol Biol. (2016) 7:27–47.
406. Pan Y, Meng M, Zhang G, Han H, Zhou Q. Oncogenic microRNAs in the genesis of leukemia and lymphoma. Curr Pharm Des. (2014) 20:5260–7.
407. Ren D, Lin B, Zhang X, Peng Y, Ye Z, Ma Y, et al. Maintenance of cancer stemness by miR-196b-5p contributes to chemoresistance of colorectal cancer cells via activating STAT3 signaling pathway. Oncotarget (2017) 8:49807–23. doi: 10.18632/oncotarget.17971
408. Jeong J-H, Park S-J, Dickinson SI, Luo J-L. A Constitutive intrinsic inflammatory signaling circuit composed of miR-196b, Meis2, PPP3CC, and p65 drives prostate cancer castration resistance. Mol Cell (2017) 65:154–67. doi: 10.1016/j.molcel.2016.11.034
409. Kim E-A, Kim S-W, Nam J, Sung E-G, Song I-H, Kim J-Y, et al. Inhibition of c-FLIPL expression by miRNA-708 increases the sensitivity of renal cancer cells to anti-cancer drugs. Oncotarget (2016) 7:31832–46. doi: 10.18632/oncotarget.7149
410. Kollinerová S, Dostál Z, Modrianský M. MicroRNA hsa-miR-29b potentiates etoposide toxicity in HeLa cells via down-regulation of Mcl-1. Toxicol In Vitro (2017) 40:289–96. doi: 10.1016/j.tiv.2017.02.005
411. Yan B, Guo Q, Fu F-J, Wang Z, Yin Z, Wei Y-B, et al. The role of miR-29b in cancer: regulation, function, and signaling. Onco Targets Ther. (2015) 8:539–48. doi: 10.2147/OTT.S75899
412. Sugio A, Iwasaki M, Habata S, Mariya T, Suzuki M, Osogami H, et al. BAG3 upregulates Mcl-1 through downregulation of miR-29b to induce anticancer drug resistance in ovarian cancer. Gynecol Oncol. (2014) 134:615–23. doi: 10.1016/j.ygyno.2014.06.024
413. Han Z, Shi L. Long non-coding RNA LUCAT1 modulates methotrexate resistance in osteosarcoma via miR-200c/ABCB1 axis. Biochem Biophys Res Commun. (2018) 495:947–53. doi: 10.1016/j.bbrc.2017.11.121
414. Xu F, Liao J-Z, Xiang G-Y, Zhao P-X, Ye F, Zhao Q, et al. MiR-101 and doxorubicin codelivered by liposomes suppressing malignant properties of hepatocellular carcinoma. Cancer Med. (2017) 6:651–61. doi: 10.1002/cam4.1016
415. Jin Z, Selaru FM, Cheng Y, Kan T, Agarwal R, Mori Y, et al. MicroRNA-192 and−215 are upregulated in human gastric cancer in vivo and suppress ALCAM expression in vitro. Oncogene (2011) 30:1577–85. doi: 10.1038/onc.2010.534
416. Wang L, Wang YM, Xu S, Wang WG, Chen Y, Mao JY, et al. MicroRNA-215 is upregulated by treatment with Adriamycin and leads to the chemoresistance of hepatocellular carcinoma cells and tissues. Mol Med Rep. (2015) 12:5274–80. doi: 10.3892/mmr.2015.4012
417. Shen Y, Liu S, Fan J, Jin Y, Tian B, Zheng X, et al. Nuclear retention of the lncRNA SNHG1 by doxorubicin attenuates hnRNPC-p53 protein interactions. EMBO Rep. (2017) 18:536–48. doi: 10.15252/embr.201643139
418. Nicholson RI, Johnston SR. Endocrine therapy–current benefits and limitations. Breast Cancer Res Treat. (2005) 93(Suppl. 1):S3–10. doi: 10.1007/s10549-005-9036-4
419. Early Breast Cancer Trialists' Collaborative Group (EBCTCG). Effects of chemotherapy and hormonal therapy for early breast cancer on recurrence and 15-year survival: an overview of the randomised trials. Lancet (2005) 365:1687–717. doi: 10.1016/S0140-6736(05)66544-0
420. Cai Y, He J, Zhang D. [Suppression of long non-coding RNA CCAT2 improves tamoxifen-resistant breast cancer cells' response to tamoxifen]. Mol Biol. (2016) 50:821–7. doi: 10.7868/S0026898416030046
421. Takahashi R, Miyazaki H, Takeshita F, Yamamoto Y, Minoura K, Ono M, et al. Loss of microRNA-27b contributes to breast cancer stem cell generation by activating ENPP1. Nat Commun. (2015) 6:7318. doi: 10.1038/ncomms8318
422. Zhu J, Zou Z, Nie P, Kou X, Wu B, Wang S, et al. Downregulation of microRNA-27b-3p enhances tamoxifen resistance in breast cancer by increasing NR5A2 and CREB1 expression. Cell Death Dis. (2016) 7:e2454. doi: 10.1038/cddis.2016.361
423. Jin L, Wessely O, Marcusson EG, Ivan C, Calin GA, Alahari SK. Prooncogenic factors miR-23b and miR-27b are regulated by Her2/Neu, EGF, and TNF-α in breast cancer. Cancer Res. (2013) 73:2884–96. doi: 10.1158/0008-5472.CAN-12-2162
424. Liu F, Zhang S, Zhao Z, Mao X, Huang J, Wu Z, et al. MicroRNA-27b up-regulated by human papillomavirus 16 E7 promotes proliferation and suppresses apoptosis by targeting polo-like kinase2 in cervical cancer. Oncotarget (2016) 7:19666–79. doi: 10.18632/oncotarget.7531
425. Thiery JP, Acloque H, Huang RYJ, Nieto MA. Epithelial-mesenchymal transitions in development and disease. Cell (2009) 139:871–90. doi: 10.1016/j.cell.2009.11.007
426. Liu H, Zhang H, Sun X, Guo X, He Y, Cui S, et al. Tamoxifen-resistant breast cancer cells possess cancer stem-like cell properties. Chin Med J. (2013) 126:3030–4.
427. Li X, Wu Y, Liu A, Tang X. MiR-27b is epigenetically downregulated in tamoxifen resistant breast cancer cells due to promoter methylation and regulates tamoxifen sensitivity by targeting HMGB3. Biochem Biophys Res Commun. (2016) 477:768–73. doi: 10.1016/j.bbrc.2016.06.133
428. Elgamal OA, Park J-K, Gusev Y, Azevedo-Pouly ACP, Jiang J, Roopra A, et al. Tumor suppressive function of mir-205 in breast cancer is linked to HMGB3 regulation. PLoS ONE (2013) 8:e76402. doi: 10.1371/journal.pone.0076402
429. Chen M-J, Cheng Y-M, Chen C-C, Chen Y-C, Shen C-J. MiR-148a and miR-152 reduce tamoxifen resistance in ER+ breast cancer via downregulating ALCAM. Biochem Biophys Res Commun. (2017) 483:840–6. doi: 10.1016/j.bbrc.2017.01.012
430. Ren Y-Q, Wang H-J, Zhang Y-Q, Liu Y-B. WBP2 modulates G1/S transition in ER+ breast cancer cells and is a direct target of miR-206. Cancer Chemother Pharmacol. (2017) 79:1003–11. doi: 10.1007/s00280-017-3302-0
431. Tan S, Ding K, Chong Q-Y, Zhao J, Liu Y, Shao Y, et al. Post-transcriptional regulation of ERBB2 by miR26a/b and HuR confers resistance to tamoxifen in estrogen receptor-positive breast cancer cells. J Biol Chem. (2017) 292:13551–64. doi: 10.1074/jbc.M117.780973
432. Zhao N, Wang R, Zhou L, Zhu Y, Gong J, Zhuang S-M. MicroRNA-26b suppresses the NF-κB signaling and enhances the chemosensitivity of hepatocellular carcinoma cells by targeting TAK1 and TAB3. Mol Cancer (2014) 13:35. doi: 10.1186/1476-4598-13-35
433. Alamolhodaei NS, Behravan J, Mosaffa F, Karimi G. MiR 221/222 as new players in tamoxifen resistance. Curr Pharm Des. (2016) 22:6946–55. doi: 10.2174/1381612822666161102100211
434. Martin EC, Conger AK, Yan TJ, Hoang VT, Miller DFB, Buechlein A, Rusch DB, Nephew KP, Collins-Burow BM, Burow ME. MicroRNA-335-5p and−3p synergize to inhibit estrogen receptor alpha expression and promote tamoxifen resistance. FEBS Lett. (2017) 591:382–92. doi: 10.1002/1873-3468.12538
435. Huang N-S, Chi Y-Y, Xue J-Y, Liu M-Y, Huang S, Mo M, et al. Long non-coding RNA metastasis associated in lung adenocarcinoma transcript 1 (MALAT1) interacts with estrogen receptor and predicted poor survival in breast cancer. Oncotarget (2016) 7:37957–65. doi: 10.18632/oncotarget.9364
436. Arun G, Diermeier S, Akerman M, Chang K-C, Wilkinson JE, Hearn S, et al. Differentiation of mammary tumors and reduction in metastasis upon Malat1 lncRNA loss. Genes Dev. (2016) 30:34–51. doi: 10.1101/gad.270959.115
437. Jiao F, Hu H, Han T, Yuan C, Wang L, Jin Z, et al. Long noncoding RNA MALAT-1 enhances stem cell-like phenotypes in pancreatic cancer cells. Int J Mol Sci. (2015) 16:6677–93. doi: 10.3390/ijms16046677
438. Redis RS, Sieuwerts AM, Look MP, Tudoran O, Ivan C, Spizzo R, et al. CCAT2, a novel long non-coding RNA in breast cancer: expression study and clinical correlations. Oncotarget (2013) 4:1748–62. doi: 10.18632/oncotarget.1292
439. Zhang C-H, Wang J, Zhang L-X, Lu Y-H, Ji T-H, Xu L, et al. Shikonin reduces tamoxifen resistance through long non-coding RNA uc.57. Oncotarget (2017) 8:88658–69. doi: 10.18632/oncotarget.20809
440. Soverini S, Mancini M, Bavaro L, Cavo M, Martinelli G. Chronic myeloid leukemia: the paradigm of targeting oncogenic tyrosine kinase signaling and counteracting resistance for successful cancer therapy. Mol Cancer (2018) 17:49. doi: 10.1186/s12943-018-0780-6
441. Rinn JL, Kertesz M, Wang JK, Squazzo SL, Xu X, Brugmann SA, et al. Functional demarcation of active and silent chromatin domains in human HOX loci by noncoding RNAs. Cell (2007) 129:1311–23. doi: 10.1016/j.cell.2007.05.022
442. Gupta RA, Shah N, Wang KC, Kim J, Horlings HM, Wong DJ, et al. Long non-coding RNA HOTAIR reprograms chromatin state to promote cancer metastasis. Nature (2010) 464:1071–6. doi: 10.1038/nature08975
443. Wang H, Li Q, Tang S, Li M, Feng A, Qin L, et al. The role of long noncoding RNA HOTAIR in the acquired multidrug resistance to imatinib in chronic myeloid leukemia cells. Hematology (2017) 22:208–16. doi: 10.1080/10245332.2016.1258152
444. Wang J, Liu X, Wu H, Ni P, Gu Z, Qiao Y, et al. CREB up-regulates long non-coding RNA, HULC expression through interaction with microRNA-372 in liver cancer. Nucleic Acids Res. (2010) 38:5366–83. doi: 10.1093/nar/gkq285
445. Wang Y, Wu P, Lin R, Rong L, Xue Y, Fang Y. LncRNA NALT interaction with NOTCH1 promoted cell proliferation in pediatric T cell acute lymphoblastic leukemia. Sci Rep. (2015) 5:13749. doi: 10.1038/srep13749
446. Lu Y, Li Y, Chai X, Kang Q, Zhao P, Xiong J, et al. Long noncoding RNA HULC promotes cell proliferation by regulating PI3K/AKT signaling pathway in chronic myeloid leukemia. Gene. (2017) 607:41–46. doi: 10.1016/j.gene.2017.01.004
447. Ichigozaki Y, Fukushima S, Jinnin M, Miyashita A, Nakahara S, Tokuzumi A, et al. Serum long non-coding RNA, snoRNA host gene 5 level as a new tumor marker of malignant melanoma. Exp Dermatol. (2016) 25:67–9. doi: 10.1111/exd.12868
448. Zhao L, Han T, Li Y, Sun J, Zhang S, Liu Y, et al. The lncRNA SNHG5/miR-32 axis regulates gastric cancer cell proliferation and migration by targeting KLF4. FASEB J. (2017) 31:893–903. doi: 10.1096/fj.201600994R
449. He B, Bai Y, Kang W, Zhang X, Jiang X. LncRNA SNHG5 regulates imatinib resistance in chronic myeloid leukemia via acting as a CeRNA against MiR-205-5p. Am J Cancer Res. (2017) 7:1704–13.
450. Fang L, Yang N, Ma J, Fu Y, Yang G-S. microRNA-1301-mediated inhibition of tumorigenesis. Oncol Rep. (2012) 27:929–34. doi: 10.3892/or.2011.1589
451. Liang W-C, Wang Y, Xiao L-J, Wang Y-B, Fu W-M, Wang W-M, et al. Identification of miRNAs that specifically target tumor suppressive KLF6-FL rather than oncogenic KLF6-SV1 isoform. RNA Biol. (2014) 11:845–54. doi: 10.4161/rna.29356
452. Lin T-Y, Chen K-C, Liu H-JE, Liu A-J, Wang K-L, Shih C-M. MicroRNA-1301-mediated RanGAP1 downregulation induces BCR-ABL nuclear entrapment to enhance imatinib efficacy in chronic myeloid leukemia cells. PLoS ONE (2016) 11:e0156260. doi: 10.1371/journal.pone.0156260
453. Gu D-N, Jiang M-J, Mei Z, Dai J-J, Dai C-Y, Fang C, et al. microRNA-7 impairs autophagy-derived pools of glucose to suppress pancreatic cancer progression. Cancer Lett. (2017) 400:69–78. doi: 10.1016/j.canlet.2017.04.020
454. Fang Y, Xue J-L, Shen Q, Chen J, Tian L. MicroRNA-7 inhibits tumor growth and metastasis by targeting the phosphoinositide 3-kinase/Akt pathway in hepatocellular carcinoma. Hepatology (2012) 55:1852–62. doi: 10.1002/hep.25576
455. Kharas MG, Janes MR, Scarfone VM, Lilly MB, Knight ZA, et al. Ablation of PI3K blocks BCR-ABL leukemogenesis in mice, and a dual PI3K/mTOR inhibitor prevents expansion of human BCR-ABL+ leukemia cells. J Clin Invest. (2008) 118:3038–3050. doi: 10.1172/JCI33337
456. Jiang M-J, Dai J-J, Gu D-N, Huang Q, Tian L. MicroRNA-7 inhibits cell proliferation of chronic myeloid leukemia and sensitizes it to imatinib in vitro. Biochem Biophys Res Commun. (2017) 494:372–378. doi: 10.1016/j.bbrc.2017.10.001
457. Shi Y, Gao X, Hu Q, Li X, Xu J, Lu S, et al. PIK3C2A is a gene-specific target of microRNA-518a-5p in imatinib mesylate-resistant gastrointestinal stromal tumor. Lab Invest. (2016) 96:652–60. doi: 10.1038/labinvest.2015.157
458. Awasthi N, Yen PL, Schwarz MA, Schwarz RE. The efficacy of a novel, dual PI3K/mTOR inhibitor NVP-BEZ235 to enhance chemotherapy and antiangiogenic response in pancreatic cancer. J Cell Biochem. (2012) 113:784–91. doi: 10.1002/jcb.23405
459. Deng L, Jiang L, Lin X-H, Tseng K-F, Liu Y, Zhang X, et al. The PI3K/mTOR dual inhibitor BEZ235 suppresses proliferation and migration and reverses multidrug resistance in acute myeloid leukemia. Acta Pharmacol Sin. (2017) 38:382–91. doi: 10.1038/aps.2016.121
460. Wang W, Peng B, Wang D, Ma X, Jiang D, Zhao J, et al. Human tumor microRNA signatures derived from large-scale oligonucleotide microarray datasets. Int J Cancer (2011) 129:1624–34. doi: 10.1002/ijc.25818
461. Zhou S, Ye W, Zhang Y, Yu D, Shao Q, Liang J, et al. miR-144 reverses chemoresistance of hepatocellular carcinoma cell lines by targeting Nrf2-dependent antioxidant pathway. Am J Transl Res. (2016) 8:2992–3002.
462. Liu L, Wang S, Chen R, Wu Y, Zhang B, Huang S, et al. Myc induced miR-144/451 contributes to the acquired imatinib resistance in chronic myelogenous leukemia cell K562. Biochem Biophys Res Commun. (2012) 425:368–73. doi: 10.1016/j.bbrc.2012.07.098
463. Liu F, Wang J, Fu Q, Zhang X, Wang Y, Liu J, et al. VEGF-activated miR-144 regulates autophagic survival of prostate cancer cells against Cisplatin. Tumour Biol. (2015) doi: 10.1007/s13277-015-4383-1
464. Liu J, Feng L, Zhang H, Zhang J, Zhang Y, Li S, et al. Effects of miR-144 on the sensitivity of human anaplastic thyroid carcinoma cells to cisplatin by autophagy regulation. Cancer Biol Ther. (2018) 19:484–96. doi: 10.1080/15384047.2018.1433502
465. Xiao W, Lou N, Ruan H, Bao L, Xiong Z, Yuan C, et al. Mir-144-3p promotes cell proliferation, metastasis, sunitinib resistance in clear cell renal cell carcinoma by downregulating ARID1A. Cell Physiol Biochem. (2017) 43:2420–33. doi: 10.1159/000484395
466. Berns K, Sonnenblick A, Gennissen A, Brohée S, Hijmans EM, Evers B, et al. Loss of ARID1A Activates ANXA1, which Serves as a predictive biomarker for trastuzumab resistance. Clin Cancer Res. (2016) 22:5238–48. doi: 10.1158/1078-0432.CCR-15-2996
467. Wuerkenbieke D, Wang J, Li Y, Ma C. miRNA-150 downregulation promotes pertuzumab resistance in ovarian cancer cells via AKT activation. Arch Gynecol Obstet. (2015) 292:1109–16. doi: 10.1007/s00404-015-3742-x
468. Sukegawa M, Wang X, Nishioka C, Pan B, Xu K, Ohkawara H, et al. The BCR/ABL tyrosine kinase inhibitor, nilotinib, stimulates expression of IL-1β in vascular endothelium in association with downregulation of miR-3p. Leuk Res. (2017) 58:83–90. doi: 10.1016/j.leukres.2017.05.005
469. Chen Y, Zeng L, Wang Y, Tolleson WH, Knox B, Chen S, et al. The expression, induction and pharmacological activity of CYP1A2 are post-transcriptionally regulated by microRNA hsa-miR-132-5p. Biochem Pharmacol. (2017) 145:178–91. doi: 10.1016/j.bcp.2017.08.012
Keywords: non-coding RNAs, chemoresistance, drug sensitivity, miRNA, lncRNA, cancer, gene networks
Citation: Corrà F, Agnoletto C, Minotti L, Baldassari F and Volinia S (2018) The Network of Non-coding RNAs in Cancer Drug Resistance. Front. Oncol. 8:327. doi: 10.3389/fonc.2018.00327
Received: 01 June 2018; Accepted: 31 July 2018;
Published: 29 August 2018.
Edited by:
Marilena Valeria Iorio, Istituto Nazionale dei Tumori (IRCCS), ItalyReviewed by:
Francesco Russo, Novo Nordisk Foundation Center for Protein Research, University of Copenhagen, DenmarkAjaikumar B. Kunnumakkara, Indian Institute of Technology Guwahati, India
Copyright © 2018 Corrà, Agnoletto, Minotti, Baldassari and Volinia. This is an open-access article distributed under the terms of the Creative Commons Attribution License (CC BY). The use, distribution or reproduction in other forums is permitted, provided the original author(s) and the copyright owner(s) are credited and that the original publication in this journal is cited, in accordance with accepted academic practice. No use, distribution or reproduction is permitted which does not comply with these terms.
*Correspondence: Stefano Volinia, s.volinia@unife.it