- Department of Dermatology, Kagoshima University Graduate School of Medical and Dental Sciences, Kagoshima, Japan
Primary cutaneous lymphomas comprise a group of lymphatic malignancies that occur primarily in the skin. They represent the second most common form of extranodal non-Hodgkin’s lymphoma and are characterized by heterogeneous clinical, histological, immunological, and molecular features. The most common type is mycosis fungoides and its leukemic variant, Sézary syndrome. Both diseases are considered T-helper cell type 2 (Th2) diseases. Not only the tumor cells but also the tumor microenvironment can promote Th2 differentiation, which is beneficial for the tumor cells because a Th1 environment enhances antitumor immune responses. This Th2-dominant milieu also underlies the infectious susceptibility of the patients. Many components, such as tumor-associated macrophages, cancer-associated fibroblasts, and dendritic cells, as well as humoral factors, such as chemokines and cytokines, establish the tumor microenvironment and can modify tumor cell migration and proliferation. Multiagent chemotherapy often induces immunosuppression, resulting in an increased risk of serious infection and poor tolerance. Therefore, overtreatment should be avoided for these types of lymphomas. Interferons have been shown to increase the time to next treatment to a greater degree than has chemotherapy. The pathogenesis and prognosis of cutaneous T-cell lymphoma (CTCL) differ markedly among the subtypes. In some aggressive subtypes of CTCLs, such as primary cutaneous gamma/delta T-cell lymphoma and primary cutaneous CD8+ aggressive epidermotropic cytotoxic T-cell lymphoma, hematopoietic stem cell transplantation should be considered, whereas overtreatment should be avoided with other, favorable subtypes. Therefore, a solid understanding of the pathogenesis and immunological background of cutaneous lymphoma is required to better treat patients who are inflicted with this disease. This review summarizes the current knowledge in the field to attempt to achieve this objective.
Overview of Cutaneous T-Cell Lymphomas (CTCLs)
Non-Hodgkin lymphomas can occur at extranodal sites in approximately 27% of cases, with the gastrointestinal tract and skin being the first and second most common sites of extranodal involvement (1). Most nodal non-Hodgkin lymphomas are B-cell derived, which is in contrast to the approximately 75–85% of primary cutaneous lymphomas that are T-cell derived (2–6). The incidence of CTCLs has been increasing (7); consequently, 4–8 people per million currently suffer from these cancers (8, 9). CTCL represents a series of skin-based neoplasms of T-cell origin, predominantly comprised of peripheral CD4+ T-cells. There are 12 distinct CTCL subtypes (Table 1), with mycosis fungoides (MF) being the most common (10). Primary cutaneous CD30+ T-cell lymphoproliferative disorders are the second most common, except in some countries in the Pacific where adult T-cell leukemia/lymphoma (ATL) ranks second (6, 11).
MF/Sézary Syndrome (SS)
Mycosis fungoides and SS constitute the most common types of primary CTCLs. MF is characterized by erythematous patches, plaques, or tumors on the skin (Figure 1), with the involvement of lymph nodes, blood, and viscera also possible. MF can mimic benign inflammatory skin disorders, such as atopic dermatitis or psoriasis; thus, it is not unusual for MF to remain undiagnosed for years. Although MF is typically an indolent disorder, the disease may progress toward or exhibit de novo more advanced forms including tumors and erythroderma (>80% of the body surface area showing patches/plaques without overt leukemia). This can lead to lymph node or organ involvement, accompanied by increased morbidity and mortality. Patients are classified as having either early-stage (patches/plaques) or advanced-stage (tumors, erythroderma, lymph node, and/or visceral involvement) (12, 13). SS is the leukemic form of the disease, in which erythroderma is accompanied by measurable levels of malignant lymphocytes with cerebriform nuclei [i.e., Sézary cells (SC)] in the blood. Typical SC counts would be ≥1,000/μL, with a CD4/CD8 ratio of ≥10 and a loss of one or more T-cell antigens (CD4+CD7− > 30% or CD4+CD26− > 40%). Furthermore, CD30 expression is associated with a significantly reduced disease-specific survival and is often associated with histologically detectable large cell transformation, hallmarking a more aggressive clinical course (14).
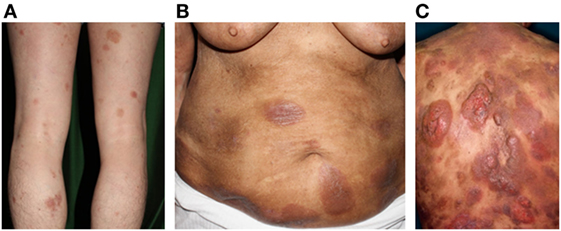
Figure 1. Clinical findings of mycosis fungoides/Sézary syndrome. (A) Patches, (B) plaques, (C) and nodules on the plaque. Written informed consent was obtained from each patient.
In the past, SS has been considered a leukemic and aggressive variant of MF. However, a recent study determined that MF and SS arose from distinct T-cell subsets: SS from central memory T-cells and MF from skin-resident effector memory T-cells (15). CD158k/killer cell immunoglobulin-like receptor 3DL2 represents a specific marker for the evaluation of SC (16); in particular, CD4+ CD158k+ lymphocytes in blood from patients with SS correspond to the malignant clonal cell population (17). In addition, immunohistological finding of CD158k in affected skin is reported to distinguish SS from MF (18). Clonal malignant T-cells from the blood of patients with SS coexpress the lymph node homing molecules C–C motif chemokine receptor 7 (CCR7)/CD197 and CD62L/l-selectin, as well as the CD27 differentiation marker, a characteristic of central memory T-cells. This is consistent with the clinical presentation of peripheral blood disease, lymphadenopathy, and diffuse erythroderma of the skin. In contrast, T-cells from MF skin lesions do not express CCR7, l-selectin, and CD27, but strongly express CCR4 and cutaneous lymphocyte antigen (CLA)/CD162, characteristics of skin-resident effector memory T-cells. This difference in the putative origins between SS (central memory T-cell-derived) and MF (tissue-resident memory-derived) can explain their distinct clinical behaviors; central memory T-cells are long-lived, apoptosis-resistant cells that can be found in the peripheral blood, lymph nodes, and skin, whereas skin-resident memory T-cells remain in the skin and do not enter the general circulation. That MF and SS are derived from different T-cell precursors is also supported by comparative genomic hybridization and gene-expression profiling, demonstrating that the CTCL genotypes are distinct (19, 20). Overall, MF is characterized by gains on chromosomes 1 and 7 and losses on chromosome 9, whereas SS is characterized by gains on chromosomes 8 and 17 and losses on chromosome 10. A multiplatform genomic analysis of patients with SS detected (1) activating CCR4 and caspase recruitment domain-containing protein 11 (CARD11) mutations in nearly one-third of patients; (2) deletion of zinc finger E-box binding homeobox 1 (ZEB1), encoding a transcriptional repressor essential for T-cell differentiation, in over one-half of patients; and (3) overexpression of interleukin 32 (IL-32) and interleukin-2 receptor subunit gamma in nearly all patients (21).
Roles of Chemokines in Development of Skin Pathology
Malignant T-cells are suggested exhibit phenotypes of mature CD4+ memory T-cells, along with type 2 or 17 (Th2 or Th17 (22)) T-helper cell phenotypes, or be comprised of FOXP3 regulatory T-cells (Treg) (23, 24). Many chemokines are also reportedly expressed in the affected skin of patients with CTCL, suggesting that chemokine–receptor interactions play important roles in disease progression (25). Chemokine receptor expression on tumor cells in MF varies with disease stage. In the patch and plaque stages of MF, most infiltrating cells express CXC chemokine receptor (CXCR) 3/CD183 in the affected skin (26). CXCR3 binds three distinct ligands, namely CXC chemokine ligand (CXCL) 9/monokine induced by gamma interferon (MIG), interferon-gamma-inducible protein-10 (CXCL10/IP-10), and interferon-inducible T cell alpha chemoattractant (CXCL11/ITAC)/IP-9; all are expressed in the affected skin in the patch and plaque stages of MF (27–29). Various cell types express these chemokines including keratinocytes, dermal fibroblasts, and Langerhans cells. In the early stages of MF, expression of CXCL9, CXCL10, and CXCR3 is believed to be important for recruitment and accumulation of tumor cells in the skin (25, 28). However, later in the tumor stage, the tumor cells increase in size and tend to express CCR4 instead of CXCR3 (30). The expression levels of CXCL9 and CXCL10 also tend to be lower in the affected skin of patients with MF during the tumor stage than during the patch and plaque stages (31). Moreover, CCR6/CD196 and its ligand CCL20/macrophage inflammatory protein (MIP)-3α are upregulated in advanced CTCL (32). Tumor MF cells exhibit high levels of CCR7 (33), which is considered to be associated with loss of epidermotropism and migration to peripheral lymph nodes, which constitutively synthesize the CCR7 ligands, CCL19 and 21 (34). CCR7 is also expressed at high levels in SC (35), as mentioned in Section “MF/Sézary syndrome (SS)”.
Circulating SC and skin-infiltrating cells in SS also express CCR4 (30, 35, 36). CCR4-expressing T-cells were found in CTCL lesions along with high expression of two CCR4 ligands, namely CC chemokine ligand (CCL) 17/thymus and activation-regulated chemokine and CCL22/macrophage-derived chemokine (30). CCL17 is expressed by endothelial cells and keratinocytes in the affected skin of patients with MF and SS (30, 37). During the tumor stage of MF, serum CCL17 levels are much higher than those during the patch/plaque stages (37), suggesting the importance of CCL17–CCR4 interactions in tumor cell trafficking to the skin of these patients. CCL22 is expressed by dendritic-like cells and keratinocytes (30, 37). Serum CCL22 levels are significantly higher in patients with MF than in healthy controls or patients with psoriasis vulgaris (37).
CC chemokine ligand 27/cutaneous T cell attracting chemokine is a CCR10 ligand that is constitutively produced by activated keratinocytes in various diseases (38). CCR10 is expressed on the tumor cells of MF and SS (35, 39). Strong immunostaining of CCL27 has been observed in the affected skin of patients with MF compared to that of unaffected individuals (39, 40). Serum CCL27 levels and the number of circulating CCR10+ CD4+ cells are both increased in patients with MF compared to that of control patients (39). Therefore, CCR10–CCL27 interactions may also contribute to the migration of lymphoma cells to the affected skin in MF and SS. In addition to CCR4 and CCR10, expression of the receptor for CXCL12/stromal cell-derived factor 1, CXCR4, is observed in SC (36). CXCL12 is a chemoattractant for CXCR4-positive cells and is strongly expressed in the affected skin of patients with MF (41) and SS (36). Therefore, CXCL12–CXCR4 interactions may also facilitate the recruitment of lymphoma cells to the skin.
Th2-Dominant Microenvironment
As the microenvironment in early-stage MF consists of non-malignant Th1 cells and CD8+ tumor-infiltrating T cells, MF and SS are considered Th2-type diseases, which are frequently accompanied by eosinophilia and high serum levels of IgE. In the early 1990s, peripheral blood mononuclear cells in patients with SS and non-leukemic CTCL were reported to be Th2 dominant (42, 43). In 1994, mRNA for Th2 cytokine was detected in the skin of patients with MF (44). T-cell clones from patients with SS were identified thereafter to have Th2-like properties (45). However, in the early stages of MF, affected skin and peripheral blood T-cells express a profile of Th1 cytokines (46, 47). The Th2 phenotype appears to be caused by leukemic T-cells, as culturing benign T-cells away from malignant clones reduces Th2 and enhances Th1 responses (48). The Th2-dominant microenvironment is advantageous for tumor cells, because interferon (IFN)-γ-producing Th1 cells enhance immune responses against the tumor. Indeed, IFN-γ has been shown to be effective for CTCL treatment (49, 50). Adenoviral-mediated gene therapies that increase expression of IFN-γ have also been used successfully in CTCL (51–53). CTCL cells can inhibit T-cell proliferation and suppress dendritic cell (DC) maturation by secretion of Th2 cytokines (54). Skin and nasal colonization with Staphylococcus aureus is common in patients with MF/SS; in particular, a Th2-dominant microenvironment may underlie this susceptibility to infection (55). Infections of S. aureus and sepsis also frequently occur in patients with CTCL (56). Accordingly, the major cause of death in patients with erythrodermic MF and SS is intravenous line sepsis, with S. aureus often being the causative microorganism (57).
In early-stage MF, signal transducers and activators of transcription (STAT) 4, the activation of which is required for Th1 differentiation, are overexpressed by IL-12 signaling via JAK2/TYK2 (58). In later stages, IL-2 and IL-15 signaling via JAK1 and JAK3 kinases activates STAT5, which increases the expression of oncogenic miR-155 (59) and subsequently inhibits STAT4 expression (60), resulting in a switch from Th1 to Th2 phenotype in malignant T cells. Downregulation of STAT4 is also induced by deficiencies in IL-12 expression (58, 61) and lack of the IL-12R β2 chain (58). During this switch, the expression of STAT6 is often upregulated in CTCL (60). STAT5 activation is seen in both early and late stages. Specifically, in the late stage, constitutive STAT5 activation is induced by cytokine-independent JAK1/JAK3 signaling (59). In the advanced stage, such constitutive STAT3 activation, which increases survival and resistance to apoptosis and promotes Th2 and Th17 phenotypes, is induced by an IL-21 autocrine signaling loop (62), the presence of IL-7 and IL-15 in the microenvironment (63), and/or constitutive cytokine-independent activation of JAK1 and JAK3 signaling (64, 65). Moreover, GATA3, a transcriptional regulator of Th2-cells, is overexpressed in SC via proteasome dysregulation (66).
Cancer-Associated Fibroblasts
Fibroblasts are crucial components of the tumor microenvironment, promoting the growth and invasion of cancer cells through various mechanisms (67). The fibroblasts in the affected skin of patients with advanced CTCL promote a Th2-dominant microenvironment by augmenting Th2 and attenuating Th1 immune responses. Increased expression of CCL26/eotaxin-3 is observed in the dermal fibroblasts, keratinocytes, and endothelial cells of the affected skin of patients with advanced MF (68). In addition, serum CCL26 and CCL11/eotaxin-1 levels were shown to be higher in patients with CTCL than in healthy control patients, which correlated with serum soluble interleukin-2 receptor (sIL-2R) levels. However, CCR3/CD193, a receptor for CCL26 and other ligands, is not expressed on lymphoma cells in MF or SS (69). Because mRNA for CCR3 is detected in affected skin (68) and CCR3 is expressed on eosinophils and subpopulations of Th2 cells (70, 71), CCL26 and CCL11 are believed to support the Th2-dominant microenvironment in MF and SS disease lesions (25).
Periostin constitutes an extracellular matrix protein that is expressed in several cancers (72); it is prominent in the stromal area during the patch and plaque stages of MF, but decreases during the tumor stage (73). Fibroblasts are reportedly the source of periostin in MF (74). IL-4 and IL-13 can induce periostin secretion by dermal fibroblasts, periostin mediates thymic stromal protein (TSLP) production by keratinocytes, and TSLP subsequently activates immature myeloid DCs, which modulate Th2 immune responses via CCL17 production (75). Serum (76) and plasma (77) TSLP levels are increased in patients with CTCL, suggesting that TSLP contributes to the Th2-dominant microenvironment in MF lesions. TSLP also induces the growth of CTCL cells (74). Therefore, periostin can directly stimulate the growth of CTCL tumor cells in addition to inducing a Th2-dominant environment in CTCL tumors.
Expression of herpesvirus entry mediator (HVEM)/CD270, a member of the tumor necrosis factor-receptor superfamily, on dermal fibroblasts in the affected skin of patients with MF and SS is decreased as the disease progresses. In addition, low HVEM expression on dermal fibroblasts in the affected skin of patients with advanced CTCL attenuates the expression of Th1 chemokines, resulting in Th2-dominant microenvironments. This occurs because the interaction between HVEM and tumor necrosis factor superfamily member 14 (also termed LIGHT)/CD258 on dermal fibroblasts increases the secretion of CXCL9–11, which are chemokines that recruit CXCR3-positive Th1 cells (29).
Tumor-Associated Macrophages (TAMs)
Macrophages constitute a major component of the leukocyte infiltrate in the tumor microenvironment (78), in which they are termed TAMs. TAMs usually comprise polarized M2 macrophages that contribute to an immune-suppressive environment and promote tumor cell growth (79). CD163 is recognized as a marker for TAMs. As with many malignancies (80), the presence of M2 macrophages in the affected skin of patients with MF has been correlated with patient prognosis (81, 82), and the presence of M2 macrophages has been correlated with lymph node staging (83); this suggests that TAMs play a significant role in MF pathogenesis. Serum sCD163 levels in patients with CTCL are significantly higher than those in normal controls and they significantly correlate with serum sIL-2R levels. TAMs are believed to play a role in the formation of CTCL by secreting various chemokines (73, 82, 84). Periostin-stimulated macrophages produce CXCL5 and CXCL10 (73), which correlates with MF tumor formation in a xenograft CTCL mouse model (84). CCL18/alternative macrophage activation-associated CC chemokine 1/MIP-4 is secreted by M2 macrophages (85) and binds to its receptor (i.e., CCR8) on Th2 cells (86). The expression of CCR8 on MF or SS tumor cells has not been reported, although the mRNA expression of CCR8 is known to be upregulated in patients with SS (21). TAMs are known to express CCL18 in the skin of patients with CTCL (87, 88). Serum CCL18 levels were significantly higher in patients with CTCL than in healthy controls, and these levels significantly correlated with modified severity-weighted assessment scores, serum sIL-2R, lactate dehydrogenase, Th2 cytokines, and chemokines (88). Furthermore, high serum levels of CCL18 were associated with poor patient prognosis (88). In the affected skin of patients with MF/SS, TAMs highly express CD30, which is the target of the anti-CD30 antibody–drug conjugate, brentuximab vedotin (89).
Dendritic Cells
Dendritic cells are antigen-presenting cells with a unique capacity to induce primary immune responses (90). By secreting Th2 cytokines, CTCL cells can suppress the maturation of DCs (54). Notably, IL-10 downregulates DC functions and may promote tolerance by skin DCs, rather than immune defense (91). Immature DCs can induce tolerance by presenting antigens to T-cells without appropriate costimulation. A significant increase in various DC subsets is seen in the affected dermis of patients with MF/SS, with the majority being immature CD209/DC-specific ICAM-3 grabbing non-integrin (DC-SIGN)-positive DCs. Increases in CD208/DC-lysosome-associated membrane glycoprotein-positive DCs (i.e., mature DCs) and CD303/blood dendritic cell antigen 2-positive DCs (i.e., plasmacytoid DCs) are also observed, but the numbers of cells expressing CD208 or CD303 are few, suggesting that many DCs in the dermis of CTCL lesions are immature. Increased number of immature DCs in CTCL lesions may be important for immunological tolerance against malignant T-cells (92). However, some CD208-positive, mature DCs may attempt to mount an immune response against the cancer cells, as mature CD208-positive DCs are elevated in the skin draining lymph nodes of patients with MF (83).
Other Key Players
The keratinocytes in the affected skin of patients with MF/SS release multiple chemokines including CCL17, CCL26, CCL27, CXCL9, and CXCL10, which help to attract T-cells to the epidermis, as mentioned above. Nerve growth factor (NGF) expression is also elevated in the affected skin of patients with SS, which stimulates the sprouting of nerve fibers. NGF is associated with the severity of pruritus in atopic dermatitis (93), and serum NGF levels are elevated in patients with SS (94). The enhanced expression of NGF is supposedly associated with pruritus in SS.
Mast cells also serve as critical stimulators of the tumor microenvironment (95). Patients with CTCL have increased number of mast cells in their affected skin and this correlates with disease progression (96). Moreover, in a model of cutaneous lymphoma, tumor growth in mast cell-deficient mice was significantly decreased. Therefore, mast cells represent key players in the development of CTCL.
Th22 cells, which produce IL-22 but not IFN-γ, IL-4, or IL-17, express CCR6, CCR4, and CCR10, thus enhancing skin infiltration. IL-22 mediates host defenses against bacterial infection (97). The affected skin of patients with MF/SS expresses high levels of IL-22 and low levels of IL-17 (32). A case of SS reportedly also had high IL-22 expression that was modulated by systemic bacterial infections (98). The serum levels of IL-22 and IL-22-induced CCL20 are increased in patients with MF/SS and are associated with disease severity (32); this suggests an important role of IL-22 in establishing the tumor microenvironment in MF and SS.
Myeloid-derived suppressor cells (MDSCs) are also recognized as key players in tumor immune escape mechanisms (99). The progression from early patch/plaque lesions to tumors in MF is related to an increase in MDSCs (100). Therefore, MDSCs play a role in MF progression by decreasing antitumor immune responses.
T-cell exhaustion via immune checkpoints also constitutes an important factor underlying tumor survival. The expression levels of PD-1 (101, 102), PD-L1 (102), CTLA-4 (103), and ICOS (104) have been described at different stages of the disease, suggesting a role for immune checkpoint inhibitor therapies.
Treatment
There is currently no cure for CTCL, thus treatment is aimed primarily at improving symptoms and quality of life and maintaining remission. Therapies are tailored to the individual patient, based on age, performance status, extent of disease burden, rate of disease progression, and prior treatments (105). A typical MF progression starts at the patch and plaque stage and then advances to dermal-based tumors over many years. Effective immune control in the initial disease stages can slow disease progression. Hughes et al. reported that chemotherapy shortens the median time until the next treatment in patients with MF/SS (106). Multiagent chemotherapy often induces immunosuppression, which leads to an increased risk of infection and poor tolerance (107). Therefore, chemotherapy should be limited until all other options are exhausted. In comparison, IFN and histone deacetylase inhibitors afford greater times to next treatment than those from chemotherapy.
Both IFN-α and IFN-γ represent effective clinical treatments for CTCLs, including MF, via their cytotoxic and immunological effects on tumor-associated T-cells (108–110). A meta-analysis suggested that the overall response rate (ORR) to IFN-α was 70% (109). In all stages of MF, IFN-α achieves a superior time to next treatment compared to that of chemotherapy (106). IFN-γ shifts the Th2-dominant tumor microenvironment to a Th1 environment, as mentioned above. IFN-α2a and IFN-γ have been shown to decrease the expression and production of CCL17 and CCL18 and increase those of CXCL10 and CXCL11. Furthermore, subcutaneous administration of IFN-α increased the number of CXCL11-producing cells in the affected skin of patients with advanced MF (111).
Toll-like receptor (TLR) agonists induce anticancer effects by stimulating the innate immune system. Imiquimod is a topical immunomodulator that stimulates Th1 responses by activating TLR7 on plasmacytoid DCs, which leads to the production of IFN-α, IL-12, and tumor necrosis factor-α (112). The effectiveness of topical imiquimod has been reported in early-stage (113–115), folliculotropic, and tumor-stage MF (116). Resiquimod, a TLR7/8 agonist, is also effective for early-stage MF (117). TLR8 is expressed by myeloid-derived DCs, which are the most abundant DCs in human skin. Resiquimod, but not imiquimod, potently activates these cells (118).
The acetylation of histones plays a critical role in gene expression regulation (119). Histone acetylation and deacetylation control gene transcription and are mediated by histone acetyltransferases and deacetylases, respectively. Histone deacetylase inhibitors enhance the acetylation of histones and non-histone proteins and can induce apoptosis (120). Histone deacetylase inhibitors are potential therapeutic agents for the treatment of lymphoid neoplasms (121–124). Pruritus relief has also been reported with these inhibitors (121, 122, 124–126), supposedly through the reduction in the levels of IL-31-expressing T-cells (127).
Brentuximab vedotin (mentioned above) is an antibody–drug conjugate, in which an anti-CD30 monoclonal antibody is linked with the anti-tubulin agent, monomethyl auristatin E (128). Brentuximab vedotin is effective in the treatment of CD30-positive relapsed/refractory Hodgkin’s lymphoma (129) and anaplastic large cell lymphoma (130). In a phase II study for MF/SS with variable CD30 expression levels, an ORR of 70% was observed with brentuximab vedotin (127). In addition, a significant improvement in objective response was observed in a randomized, phase III clinical trial (131).
Mogamulizumab, a defucosylated humanized anti-CCR4 antibody that was first approved for relapsed ATL, as described in further detail in Section “Adult T-cell leukemia/lymphoma,” is also effective for CTCL including MF/SS (132, 133), and approved for relapsed or refractory CCR4-positive CTCL. In addition, an anti-CD158k monoclonal antibody, IPH4102, has also recently been developed (134), for which clinical studies in CTCL are ongoing (135). Lenalidomide (136), bortezomib (137), and immune checkpoint blockade are also under investigation.
Primary Cutaneous CD30+ T-Cell Lymphoproliferative Disorders
Primary cutaneous CD30+ T-cell lymphoproliferative disorders (PC CD30+ T-LPD) constitute the second most common form of CTCL, representing approximately 30% of all cutaneous lymphomas (2). They comprise a spectrum of diseases from lymphomatoid papulosis (LyP) to primary cutaneous anaplastic large-cell lymphoma (PCALCL) (138). The expression of CD30, a cytokine receptor belonging to the tumor necrosis factor receptor superfamily, by atypical T-cells is the common immunophenotype of this disorder.
Primary cutaneous anaplastic large-cell lymphoma is characterized by large T-cells with prominent nuclear pleomorphisms along with CD30 expression by more than 75% of the tumor cells (2). A single tumor or a group of firm nodules is seen clinically (Figure 2). PCALCL was established as a distinct form of ALCL because its clinical course, phenotype, and genotype are significantly different from those of systemic ALCL, including ALK-positive and ALK-negative forms (139–141). Moreover, IFN regulatory factor-4 translocations are reported to be specific for PCALCL (142). In contrast to that of systemic ALCL, the prognosis of PCALCL is reportedly excellent (143), with the exception of cases in Japan that appear to have a less favorable prognosis (144). PCALCL arising on the legs tends to produce poorer outcomes (145). The typical histology of PCALCL is a circumscribed nodular infiltrate of cohesively arranged large lymphoid cells that extends into the deep dermis or hypodermis. Neutrophil-rich and eosinophil-rich variants have been noted and appear to be associated with immunodeficiency (146). The abundant infiltration of neutrophils can be explained by the release of IL-8, a potent neutrophil chemoattractant, from the tumor cells (147).
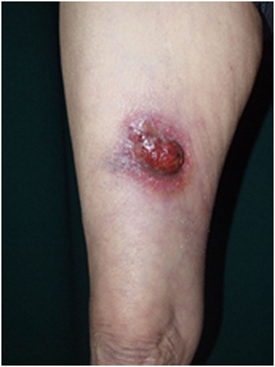
Figure 2. Clinical findings of primary cutaneous CD30+ T-cell lymphoma. Eroded tumor is seen on the right thigh. Written informed consent was obtained from the patient.
The tumor cells in PCALCL possess an activated T-cell phenotype and express CD2, CD4, and CD45RO, with a loss of CD2 and CD5 occurring variably. CD3 may be lacking or expressed at lower levels owing to genetic alterations in the T-cell receptor (TCR) coding regions on chromosome 1 in the tumor cells (148). Additionally, CD25/IL-2R, CD71, human leukocyte antigen–antigen D related, and CLA/CD162, as well as cytotoxic proteins, such as T-cell intracellular antigen 1 (TIA-1), granzyme B, and perforin, are expressed in half of PCALCL cases. PCALCL is often negative for epithelial membrane antigen, which differentiates it from systemic ALCL. Numerous quantities of TAMs are also present.
As opposed to MF/SS, the tumor cells of PCALCL express CCR3. CCL11, a CCR3 ligand, is also expressed by PCALCL cells and is detected in the connective tissue cells in the tumor. The CCR3+ tumor cells abundantly express IL-4 but not IFN-γ (69). The expression of both CCL11 and CCR3 on the tumor cells can lead to homotypic aggregation, which can be observed as cohesive clusters of tumor cells, a characteristic finding in ALCL (149). As CCR3 is also expressed on eosinophils and subpopulations of Th2 cells (70, 71), CCR3+ cells secreting CCL11 and IL-4 may produce a Th2-dominant microenvironment, which is suitable for tumor growth.
Lymphomatoid papulosis was first described by the dermatologist, Warren L. Macaulay, as a chronic recurrent, self-regressing papulonodular skin eruption with histologic features of a malignant lymphoma (138). Five histological variants (types A to E) are recognized as original variants in the updated World Health Organization classification of 2016 (10). LyP type A is the most common subtype, accounting for 75% of LyP cases (150). Type A is characterized by wedge-shaped dermal infiltrates with scattered large CD30+ cells. Histiocytes, eosinophils, and neutrophils comprise the background inflammatory cells. Type B shows epidermotropic infiltrates of small to medium-sized lymphocytes with variable CD30 expression and atypical chromatin-dense nuclei. Type C shows nodular cohesive infiltrates of large CD30+ pleomorphic or anaplastic lymphocytes. Type D shows epidermotropic infiltrates of atypical, small to medium-sized pleomorphic CD8+ cytotoxic cells (151). Type E shows angioinvasive infiltrates of mainly medium-sized pleomorphic CD30+ cells (152). Vascular occlusion by atypical lymphocytes and/or thrombi, hemorrhage, ulceration, and extensive necrosis are observed. LyP can persist for years or decades, but is not life-threatening (143, 153). However, some patients with LyP can develop secondary lymphoid neoplasms, in particular MF, Hodgkin’s lymphoma, and cutaneous or nodal CD30+ ALCL (140, 146, 154). Surgical excision or radiation therapy is the recommended therapy for solitary or grouped lesion(s) of PCALCL, whereas methotrexate is the most prescribed therapy for multifocal lesions (138). The brentuximab vedotin (128) has been granted breakthrough therapy designation; in addition, bexarotene, a retinoid X receptor-specific agonist, has also been shown to be effective for both PCALCL (ORR: 50%) and LyP (ORR: 60%) in clinical trials (155). HDAC inhibitors (156), crizotinib, an ALK inhibitor (157), and anti-PD-1 are under investigation.
Adult T-Cell Leukemia/Lymphoma
Adult T-cell leukemia/lymphoma is a distinct T-cell malignancy caused by human T-lymphotropic virus type I (HTLV-1). HTLV-I infections are endemic in many parts of the world including southwest Japan, the Caribbean basin, and parts of central Africa and South America. Neoplastic T-cells are usually CD4+CD25+CCR4+ (158). The general characteristics of ATL are lymphadenopathy, hepatosplenomegaly, hypercalcemia, abnormal peripheral blood lymphocytes with multilobulated nuclei, and skin lesions (Figure 3).
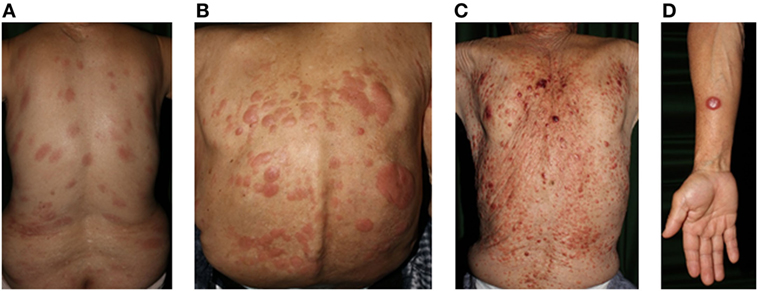
Figure 3. Clinical findings of adult T-cell lymphoma/leukemia. (A) Patch, (B) plaque, (C) multiple nodulotumoral lesion, and (D) tumor. Written informed consent was obtained from each patient.
There are four clinical subtypes of ATL (159): acute, lymphoma, chronic, and smoldering, based on peripheral blood involvement, organ complications, and laboratory examinations. Patients with ATL can be stratified into two groups: aggressive, which consists of the acute, lymphoma, and unfavorable chronic types, and indolent, which consists of the favorable chronic and smoldering types. The chronic type is separated into the favorable and unfavorable subgroups according to significant prognostic factors. This stratification is important for treatment selection, with most patients with aggressive ATL being given systemic chemotherapy, whereas those with indolent ATL are given topical therapy or are placed on observation.
Cutaneous involvement is frequently observed in patients with ATL at 30–70% (160, 161), regardless of ATL subtype. Cutaneous manifestation in the smoldering type of ATL has been suggested to reflect poor prognosis (162), and cutaneous ATL was recently proposed to include the lymphoma type as an extranodal variant (163). The majority of skin lesions are caused by the direct invasion of ATL tumor cells, forming various types of eruptions (164). In addition to these primary invasive lesions, patients with ATL may present with secondary inflammatory or infectious lesions (165). Compared to those of peripheral blood tumor cells, skin-infiltrating ATL tumor cells exhibit enhanced characteristics, such as increased expression of chemokine receptors. The interaction between chemokines and chemokine receptors drives T-cell migration and activation, which plays a critical role in the pathogenesis of various neoplastic and inflammatory disorders. ATL cells produce several chemokines including CCL3/MIP-1α, CCL4/MIP-1β (166), CCL2/monocyte chemoattractant protein-1 (MCP-1) (167), and CCL1/I-309 (168), as well as several chemokine receptors, including CCR4 (158, 169), CCR7 (170), and CCR8/CDw198 (168). Overexpression of chemokine CCL1 and its receptor, CCR8, contributes to autocrine anti-apoptotic effects ATL cells (168). Increased CCR7 expression is associated with lymphoid organ infiltration (170).
Adult T-cell leukemia/lymphoma cells not only express CCR4 but also its ligands, CCL17 and CCL22 (171). Neoplastic T-cells that highly express the Th2 chemokine receptor, CCR4, are found in the peripheral blood and affected skin of patients with ATL. In CTCL, extravasation of lymphoma cells into the skin is mediated by CCL17 and CCL22 released from epidermal cells (30). In contrast, one of the major sources of CCL17 in the affected skin of patients with ATL is the tumor cell itself (171). Moreover, CCL17 and CCL22 can also attract CCR4-expressing Treg cells, which may further suppress cytotoxic T-cells and prevent tumor immunosurveillance of the ATL cells (165). As ATL cells share the CD4+CD25+CCR4+ phenotype with Treg cells, ATL cells have been postulated as being Treg cells. In addition to CD25 and CCR4, ATL cells express CTLA-4 and FoxP3, both of which are expressed in Treg cells (172, 173). However, whether ATL cells can function as Treg cells is controversial because tumor cells possess very limited regulatory ability (174).
Th17 cells play an important role in cutaneous innate immunity. Th17-derived cytokines stimulate keratinocytes to produce antimicrobial peptides (175). ATL tumor cells can reduce the number and/or function of Th17 cells. Studies have shown that cellular immune responses are greatly impaired in patients with ATL, and ATL cells have been shown to secrete immunosuppressive cytokines such as IL-10 and transforming growth factor-β1 in vitro. In particular, ATL cells, as well as Treg or Th2 cells residing in the blood, produce IL-10, thereby suppressing Th17 activity (176). IL-17 enhances the synthesis of various antimicrobial peptides, such as human β-defensin 2, LL-37 (177), and S100A7, in keratinocytes. These peptides are active against fungi, such as those causing ringworm (178). More than 60% of patients with ATL have tinea pedis/unguium/corporis, candidiasis, or other cutaneous fungal infections (165). Other skin infections may occur in these patients in addition to superficial fungal infections. It has been reported that scabies is sometimes superimposed on the skin lesions of patients with ATL (179).
Programmed cell death (PD)-1/CD279 constitutes a cell surface receptor that suppresses the immune system. PD-1 expression on HTLV-1-specific cytotoxic T-cells is dramatically upregulated in HTLV-1 carriers and patients with ATL (180). PD-1 is expressed at high levels on CD4+ neoplastic and non-neoplastic cells, but not on CD8+ cells (181). Because normal CD4+ T-cells can be infected with HTLV-1, they can sometimes express PD-1, leading to immunosuppression. Moreover, it is noteworthy that PD-L1 is expressed in ATL cells (181). Expression of both PD-1 and PD-L1 by the ATL cells suggests a self-destructive state of the tumor cells. However, it may be more important that the PD-L1 expressed by the tumor cells suppresses the function of PD-1-expressing normal CD4+ T-cells, resulting in immune evasion. Of note, 25% of patients with ATL have structural variations in the 3’-region of the gene for PD-L1, which leads to marked elevations of aberrant PDL1 transcripts (182).
The fact that the tumor cells express CCR4 provides a therapeutic strategy for ATL. The anti-CCR4 monoclonal antibody mogamulizumab markedly enhances antibody-dependent cellular cytotoxicity and has been approved for the treatment of patients with CCR4-positive ATL, peripheral T-cell lymphoma, and CTCL. In a phase II trial of patients with relapsed CCR4-positive ATL, the ORR was 50%, with a complete response rate of 30% (183). Mogamulizumab is more effective against the peripheral blood tumor cells than those in the skin and lymph nodes. Cutaneous adverse reactions (CARs) are frequently observed during treatment (183, 184) and are supposedly indicative of favorable prognoses in ATL (185); a reduction in Treg by mogamulizumab is believed to induce CARs (186, 187). Recently, pretransplantation mogamulizumab has been reported to increase the risk of severe acute graft-versus-host disease (188, 189), and non-relapse mortality is significantly higher in patients with pretransplantation mogamulizumab. Therefore, mogamulizumab should be carefully considered and monitored for patients with ATL who are eligible for allogeneic hematopoietic stem-cell transplantation.
Panniculitis-Like T-Cell Lymphoma
Subcutaneous panniculitis-like T-cell lymphoma (SPTCL) with α/β phenotype and SPTCL with γ/δ phenotype have been recognized as unique entities, considering their clinical, histological, and immunological characteristics (2, 190, 191). The term SPTCL is now used exclusively for cases with the α/β T-cell phenotype, whereas those of the γ/δ T-cell phenotype have been reclassified as primary cutaneous gamma/delta T-cell lymphoma (PCGD-TCL) (2). The differential diagnosis of these two diseases is important, as each has a different prognosis and therapeutic strategy. In addition, both entities should be differentiated from other types of malignant lymphoma with preferential subcutaneous involvement and from other forms of lobular panniculitis, especially lupus panniculitis (192, 193).
Subcutaneous Panniculitis-Like T-Cell Lymphoma
Patients with SPTCL present clinically with multiple nodules or deeply seated plaques without ulceration. The skin lesions usually involve the legs, arms, and trunk. Systemic symptoms, such as pyrexia, fatigue, and weight loss, and laboratory abnormalities, including cytopenia and elevated liver function tests, are commonly observed. Hemophagocytic syndrome (HPS) is observed in <20% of patients (194). Dissemination to extracutaneous sites rarely occurs. As many as 20% of patients have associated autoimmune disease, which is commonly systemic lupus erythematosus (194).
The histopathological findings in SPTCL are dense, nodular, or diffuse subcutaneous infiltrates with a pattern similar to lobular panniculitis. The epidermis is not typically involved. The rimming of individual fat cells by neoplastic T-cells is a curious finding, although it is not diagnostic (193). The neoplastic T-cells are interspersed with small reactive lymphocytes and many histiocytes, whereas other inflammatory cells, including neutrophils and eosinophils, as well as the plasma cells and plasmacytoid DCs that are common in lupus panniculitis (195, 196), are usually lacking (193). High-throughput sequencing of the TCR genes can assist in the diagnosis of SPTCL (192). The neoplastic cells have a mature CD3+CD4−CD8+ T-cell phenotype and express cytotoxic proteins, such as granzyme B, TIA-1, and perforin (194). Although the exact mechanisms that neoplastic cells utilize to migrate into the hypodermis are still mostly unknown, CCR5 expression on neoplastic cells and its ligands, CCL3, CCL4, and CCL5, which can be secreted from immunologically activated adipocytes, may contribute to the pathogenesis of SPTCL (197, 198).
The differential diagnosis of SPTCL includes both PCGD-TCL and lupus panniculitis. Differentiation is critical because PCGD-TCL with panniculitis-like features generally has a poor prognosis and requires systemic chemotherapy. In contrast, SPTCL has an excellent prognosis, especially in the cases without HPS (194). Both SPTCL and PCGD-TCL have nodular skin lesions with panniculitis-like features and rimming of fat cells. In contrast to that of SPTCL, PCGD-TCL involves ulceration of the hypodermis, dermis, and/or epidermis (194). Expression of βF1, but not TCRγ/δ or CD56, is useful to differentiate between SPTCL and PCGD-TCL.
Multiagent chemotherapy is not recommended as a first-line treatment for SPTCL without HPS. Systemic corticosteroids or other immunosuppressive agents, such as cyclosporine or methotrexate, are preferred, which is also the case with relapsing disease (199–201). Oral bexarotene has also shown good response rates (202).
Primary Cutaneous Gamma/Delta T-Cell Lymphoma
Primary cutaneous gamma/delta T-cell lymphoma is a lymphoma composed of a clonal proliferation of mature, activated γ/δ T-cells with a cytotoxic phenotype. Most patients present with deep dermal or subcutaneous plaques or tumors, either with or without epidermal ulceration and necrosis (194, 203, 204). The skin lesions are often generalized and involve the extremities. Some patients may present with a single tumor, or scaly patches/plaques, clinically resembling early-stage MF (204). The involvement of mucosal and other extranodal sites is frequently noted, although lymph nodes, spleen, and bone marrow are rarely involved (204, 205). Most patients present with systemic symptoms including B symptoms. PCGD-TCL is frequently accompanied by HPS, particularly in patients with panniculitis-like tumors (194, 203). Chronic antigenic stimulation has been hypothesized to be involved in the pathogenesis of PCGD-TCL (206). PCGD-TCL is also associated with opportunistic infections in patients with congenital or acquired immunosuppression and autoimmunity (207–209).
The lymphoid infiltrates have a variable histological pattern and may be epidermotropic, dermal, and/or subcutaneous (203, 204). In contrast to that of SPTCL, a pure panniculitic pattern is rarely observed (204), and variable patterns can be found in skin biopsies obtained from different sites or different parts of the same biopsy (190, 203, 204). Lichenoid or vascular interface dermatitis-like patterns of epidermal infiltration may occur, which may be associated with intraepidermal vesiculation and necrosis (204). Panniculitis-like lesions may show the rimming of fat cells observed in SPTCL. Angiocentricity, angiodestruction, and tissue necrosis may be seen. Hemophagocytosis may be present, especially in cases with HPS. The tumor cells have a characteristic phenotype of TCR γ/δ+, βF1−, CD3+, CD2+, CD5−, and CD56+, with a strong expression of cytotoxic proteins. PCGD-TCL with subcutaneous panniculitis-like infiltrate preferentially derives from the V2 subtype (205). PCGD-TCL is resistant to multiagent chemotherapy. The effectiveness of hematopoietic stem cell transplantation has been reported in some patients with PCGD-TCL (204, 210, 211).
Primary Cutaneous CD4+ Small/Medium T-Cell Lymphoproliferative Disorder
Primary cutaneous small/medium-sized T-cell lymphoma (PCSM-TCL) has recently been reclassified as primary cutaneous small/medium-sized T-cell lymphoproliferative disorder (PCSM-TCLPD) because of its indolent behavior and uncertain malignancy (10). PCSM-TCL was originally associated with a favorable 5-year survival rate of 60–80% (2). However, fatal outcomes have not been documented in subsequent reports (212, 213).
Primary cutaneous small/medium-sized T-cell lymphoproliferative disorder characteristically presents with a single lesion on the head, neck, or upper arms, but rarely presents as multiple papules, plaques, or tumors (212, 214). Histopathologically, PCSM-TCLPD is characterized by many small- to medium-sized CD3+CD4+CD8− T-cells, with a small number of large CD4+ pleomorphic T-cells and variable admixtures of CD8+ T-cells, B-cells, histiocytes, plasma cells, and eosinophils (2).
The few, large pleomorphic CD4+ T-cells in PCSM-TCLPD express PD-1, BCL6, and CXCL13 (215), all of which are expressed on a particular germinal center T-cell subset, termed follicular helper T (TFH) cells. TFH cells are important in germinal center formation and plasma cell development. The expression of PD-1, BCL6, and CXCL13 by these large CD4+ T-cells suggests that PCSM-TCLPD originates from TFH cells (215). PD-1 is typically expressed by atypical cells in PCSM-TCL and pseudo-T-cell lymphomas (216). The clinical presentation, pathological features, and immunohistochemical findings of PCSM-TCLPD are very similar to those of pseudo-T-cell lymphomas (217, 218). The demonstration of a T-cell clone and loss of pan-T-cell antigens are useful diagnostic criteria for PCSM-TCL (218). The staining pattern for nuclear factor of activated T-cells, cytoplasmic 1 is also reported to be useful for the differential diagnosis between PCSM-TCLPD and pseudo-T-cell lymphomas (219), where NFAT1c nuclear staining indicates PCSM-TCLPD and cytoplasmic staining indicates pseudo-T-cell lymphoma. The cytoplasmic staining pattern is also seen in MF, ALCL, and LyP. The clinical behavior of PCSM-TCLPD is almost always indolent, with most patients showing localized disease. Treatment with local therapies, such as excision or radiation therapy, is often curative (214, 220, 221).
Hydroa Vacciniforme-Like Lymphoproliferative Disorder (HVLL)
Typical hydroa vacciniforme (HV) is characterized by light-induced herpetiform vesiculopapules on the sun-exposed areas. The eruptions form crusts and then heal to leave varicelliform scars. Systemic symptoms are absent, and the disease usually improves spontaneously in adolescence and young adulthood (222). Routine laboratory tests are normal. Since the first report in 1986 (223), peculiar HV-like eruptions have been recognized in children mainly from Asia and Central and South America. HVLL was included for the first time in the 2008 World Health Organization classification of tumors of hematopoietic and lymphoid tissues (224). HVLL is defined as an Epstein–Barr virus (EBV)-positive CTCL that occurs in children and less often in young adults (225). Unlike typical HV, HVLL eruptions become more severe with age, presenting with marked facial edema and vesiclopapules followed by ulceration and crusting. Systemic symptoms, including high-grade fever and liver damage, are usually present. Hepatosplenomegaly and lymphadenopathy are frequently observed during the acute phase. The lesions are associated with EBV infection and frequently possess monoclonal rearrangements of the TCR genes (226, 227). Although the skin lesions are not limited to sun-exposed areas, there is an increased occurrence during the summer. Most cases have a CD8+ T-cell phenotype (228), whereas a small number of cases have been reported to have a natural killer-cell phenotype (229, 230). Regardless of cell-type derivation, the lymphoid cells are positive for cytotoxic markers, such as granzyme B and TIA-1 (231).
Severe Mosquito Bite Allergy
An associated cutaneous disorder is a severe allergy/hypersensitivity to mosquito bites (232). It is defined as an EBV+ NK-cell lymphoproliferation that is characterized by high fever, ulcers, skin necrosis, and deep scarring, with the potential to progress into overt NK/T-cell lymphoma or aggressive NK-cell leukemia in the protracted clinical course (233). Severe mosquito bite allergy was included for the first time in the 2017 World Health Organization classification of tumors of hematopoietic and lymphoid tissues (234).
Primary Cutaneous Acral CD8+ T-Cell Lymphoma
Primary cutaneous acral CD8+ T-cell lymphoma is characterized as a solitary, slow-growing nodule without prior patches or plaques (235), but with precedence of bilateral, symmetrical disease and recurrent disease (236). Most cases appear on the ear, although other peripheral locations, such as the nose, hands, and feet, have been noted (237).
Primary cutaneous acral CD8+ T-cell lymphoma and PCSM-TCLPD are often indistinguishable morphologically. Moreover, the overt clinical features of both diseases are similar, such as targeting adults, a preference for the face and neck, solitary tumors without ulceration, and an indolent behavior. However, T follicular markers, such as CD10, Bcl-6, PD-1, and CXCL13, which are expressed on neoplastic cells of PCSM-TCLPD, are negative in primary cutaneous acral CD8+ T-cell lymphoma (236). Granzyme B expression is also typically negative in the latter (238). The clinical course for primary cutaneous acral CD8+ T-cell lymphoma is invariably indolent; cutaneous relapse may occur, but there have been no reports of progression to extracutaneous sites, and overtreatment should be avoided (238). Localized therapy, such as topical steroids, radiotherapy, and surgical excision, or careful monitoring, is preferred. IFN, psoralen-ultraviolet A phototherapy, and methotrexate have been used for patients with multifocal cutaneous disease (238).
Primary Cutaneous CD8+ Aggressive Epidermotropic Cytotoxic T-Cell Lymphoma
Primary cutaneous CD8+ aggressive epidermotropic cytotoxic T-cell lymphoma (PCAETCL) is characterized by disseminated, rapidly developing papules, plaques, and nodules with central ulceration or necrosis. PCAETCL may spread to other visceral organs including the lungs, testes, central nervous system, and oral mucosa (239–241); it carries an overall poor prognosis. However, the lymph nodes are rarely involved. Histological findings demonstrate prominent epidermotropism, with necrotic keratinocytes and ulceration (240). Dermal infiltrates consist of atypical lymphocytes, often extending into the deep dermis and subcutaneous fat. Adnexal invasion is frequently observed (242). Blistering, angiocentricity, angioinvasion, riming of adipocytes, and destruction of adnexal structures may be seen (240). Cells invariably demonstrate CD8+CD4− phenotypes and usually express CD3, β-F1, and TIA-1. CD45RA is expressed in the majority of cases (239). T-cell clonality is usually demonstrated. Conventional therapies for CTCL are ineffective and multiagent chemotherapies have unsatisfactory outcomes (240). Hematopoietic stem cell transplantation is a reasonable treatment choice for PCAETCL (243).
Author Contributions
The author confirms being the sole contributor of this work and approved it for publication.
Conflict of Interest Statement
The author declares that the research was conducted in the absence of any commercial or financial relationships that could be construed as a potential conflict of interest.
References
1. Groves FD, Linet MS, Travis LB, Devesa SS. Cancer surveillance series: non-Hodgkin’s lymphoma incidence by histologic subtype in the United States from 1978 through 1995. J Natl Cancer Inst (2000) 92:1240–51. doi:10.1093/jnci/92.15.1240
2. Willemze R, Jaffe ES, Burg G, Cerroni L, Berti E, Swerdlow SH, et al. WHO-EORTC classification for cutaneous lymphomas. Blood (2005) 105:3768–85. doi:10.1182/blood-2004-09-3502
3. Criscione VD, Weinstock MA. Incidence of cutaneous T-cell lymphoma in the United States, 1973-2002. Arch Dermatol (2007) 143:854–9. doi:10.1001/archderm.143.7.854
4. Assaf C, Gellrich S, Steinhoff M, Nashan D, Weisse F, Dippel E, et al. Cutaneous lymphomas in Germany: an analysis of the central cutaneous lymphoma registry of the German society of dermatology (DDG). J Dtsch Dermatol Ges (2007) 5:662–8. doi:10.1111/j.1610-0387.2007.06337.x
5. Bradford PT, Devesa SS, Anderson WF, Toro JR. Cutaneous lymphoma incidence patterns in the United States: a population-based study of 3884 cases. Blood (2009) 113:5064–73. doi:10.1182/blood-2008-10-184168
6. Hamada T, Iwatsuki K. Cutaneous lymphoma in Japan: a nationwide study of 1733 patients. J Dermatol (2014) 41:3–10. doi:10.1111/1346-8138.12299
7. Weinstock MA, Horm JW. Mycosis fungoides in the United States. Increasing incidence and descriptive epidemiology. JAMA (1988) 260:42–6. doi:10.1001/jama.1988.03410010050033
8. Holterhues C, Vries E, Louwman MW, Koljenovic S, Nijsten T. Incidence and trends of cutaneous malignancies in the Netherlands, 1989-2005. J Invest Dermatol (2010) 130:1807–12. doi:10.1038/jid.2010.58
9. Sokolowska-Wojdylo M, Olek-Hrab K, Ruckemann-Dziurdzinska K. Primary cutaneous lymphomas: diagnosis and treatment. Postepy Dermatol Alergol (2015) 32:368–83. doi:10.5114/pdia.2015.54749
10. Swerdlow SH, Campo E, Pileri SA, Harris NL, Stein H, Siebert R, et al. The 2016 revision of the World Health Organization classification of lymphoid neoplasms. Blood (2016) 127:2375–90. doi:10.1182/blood-2016-01-643569
11. Ruiz R, Morante Z, Mantilla R, Mas L, Casanova L, Gomez HL. Primary cutaneous T-cell lymphoma: experience from the Peruvian National Cancer Institute. An Bras Dermatol (2017) 92:649–54. doi:10.1590/abd1806-4841.20176825
12. Olsen E, Vonderheid E, Pimpinelli N, Willemze R, Kim Y, Knobler R, et al. Revisions to the staging and classification of mycosis fungoides and Sezary syndrome: a proposal of the International Society for Cutaneous Lymphomas (ISCL) and the cutaneous lymphoma task force of the European Organization of Research and Treatment of Cancer (EORTC). Blood (2007) 110:1713–22. doi:10.1182/blood-2007-03-055749
13. Agar NS, Wedgeworth E, Crichton S, Mitchell TJ, Cox M, Ferreira S, et al. Survival outcomes and prognostic factors in mycosis fungoides/Sezary syndrome: validation of the revised International Society for Cutaneous Lymphomas/European Organisation for Research and treatment of cancer staging proposal. J Clin Oncol (2010) 28:4730–9. doi:10.1200/JCO.2009.27.7665
14. Benner MF, Jansen PM, Vermeer MH, Willemze R. Prognostic factors in transformed mycosis fungoides: a retrospective analysis of 100 cases. Blood (2012) 119(7):1643–9. doi:10.1182/blood-2011-08-376319
15. Campbell JJ, Clark RA, Watanabe R, Kupper TS. Sezary syndrome and mycosis fungoides arise from distinct T-cell subsets: a biologic rationale for their distinct clinical behaviors. Blood (2010) 116:767–71. doi:10.1182/blood-2009-11-251926
16. Bagot M, Moretta A, Sivori S, Biassoni R, Cantoni C, Bottino C, et al. CD4(+) cutaneous T-cell lymphoma cells express the p140-killer cell immunoglobulin-like receptor. Blood (2001) 97(5):1388–91. doi:10.1182/blood.V97.5.1388
17. Poszepczynska-Guigne E, Schiavon V, D’Incan M, Echchakir H, Musette P, Ortonne N, et al. CD158k/KIR3DL2 is a new phenotypic marker of Sezary cells: relevance for the diagnosis and follow-up of Sezary syndrome. J Invest Dermatol (2004) 122(3):820–3. doi:10.1111/j.0022-202X.2004.22326.x
18. Wechsler J, Bagot M, Nikolova M, Parolini S, Martin-Garcia N, Boumsell L, et al. Killer cell immunoglobulin-like receptor expression delineates in situ Sezary syndrome lymphocytes. J Pathol (2003) 199(1):77–83. doi:10.1002/path.1251
19. van Doorn R, van Kester MS, Dijkman R, Vermeer MH, Mulder AA, Szuhai K, et al. Oncogenomic analysis of mycosis fungoides reveals major differences with Sezary syndrome. Blood (2009) 113:127–36. doi:10.1182/blood-2008-04-153031
20. Laharanne E, Oumouhou N, Bonnet F, Carlotti M, Gentil C, Chevret E, et al. Genome-wide analysis of cutaneous T-cell lymphomas identifies three clinically relevant classes. J Invest Dermatol (2010) 130:1707–18. doi:10.1038/jid.2010.8
21. Wang L, Ni X, Covington KR, Yang BY, Shiu J, Zhang X, et al. Genomic profiling of Sezary syndrome identifies alterations of key T cell signaling and differentiation genes. Nat Genet (2015) 47:1426–34. doi:10.1038/ng.3444
22. Krejsgaard T, Ralfkiaer U, Clasen-Linde E, Eriksen KW, Kopp KL, Bonefeld CM, et al. Malignant cutaneous T-cell lymphoma cells express IL-17 utilizing the Jak3/Stat3 signaling pathway. J Invest Dermatol (2011) 131(6):1331–8. doi:10.1038/jid.2011.27
23. Berger CL, Tigelaar R, Cohen J, Mariwalla K, Trinh J, Wang N, et al. Cutaneous T-cell lymphoma: malignant proliferation of T-regulatory cells. Blood (2005) 105(4):1640–7. doi:10.1182/blood-2004-06-2181
24. Heid JB, Schmidt A, Oberle N, Goerdt S, Krammer PH, Suri-Payer E, et al. FOXP3+CD25− tumor cells with regulatory function in Sezary syndrome. J Invest Dermatol (2009) 129(12):2875–85. doi:10.1038/jid.2009.175
25. Sugaya M. Chemokines and cutaneous lymphoma. J Dermatol Sci (2010) 59:81–5. doi:10.1016/j.jdermsci.2010.05.005
26. Lu D, Duvic M, Medeiros LJ, Luthra R, Dorfman DM, Jones D. The T-cell chemokine receptor CXCR3 is expressed highly in low-grade mycosis fungoides. Am J Clin Pathol (2001) 115:413–21. doi:10.1309/3N7P-J84L-JQ9K-G89R
27. Sarris AH, Esgleyes-Ribot T, Crow M, Broxmeyer HE, Karasavvas N, Pugh W, et al. Cytokine loops involving interferon-gamma and IP-10, a cytokine chemotactic for CD4+ lymphocytes: an explanation for the epidermotropism of cutaneous T-cell lymphoma? Blood (1995) 86:651–8.
28. Tensen CP, Vermeer MH, van der Stoop PM, van Beek P, Scheper RJ, Boorsma DM, et al. Epidermal interferon-gamma inducible protein-10 (IP-10) and monokine induced by gamma-interferon (Mig) but not IL-8 mRNA expression is associated with epidermotropism in cutaneous T cell lymphomas. J Invest Dermatol (1998) 111:222–6. doi:10.1046/j.1523-1747.1998.00263.x
29. Miyagaki T, Sugaya M, Suga H, Morimura S, Ohmatsu H, Fujita H, et al. Low herpesvirus entry mediator (HVEM) expression on dermal fibroblasts contributes to a Th2-dominant microenvironment in advanced cutaneous T-cell lymphoma. J Invest Dermatol (2012) 132:1280–9. doi:10.1038/jid.2011.470
30. Ferenczi K, Fuhlbrigge RC, Pinkus J, Pinkus GS, Kupper TS. Increased CCR4 expression in cutaneous T cell lymphoma. J Invest Dermatol (2002) 119:1405–10. doi:10.1046/j.1523-1747.2002.19610.x
31. Miyagaki T, Sugaya M. Immunological milieu in mycosis fungoides and Sezary syndrome. J Dermatol (2014) 41:11–8. doi:10.1111/1346-8138.12305
32. Miyagaki T, Sugaya M, Suga H, Kamata M, Ohmatsu H, Fujita H, et al. IL-22, but not IL-17, dominant environment in cutaneous T-cell lymphoma. Clin Cancer Res (2011) 17(24):7529–38. doi:10.1158/1078-0432.CCR-11-1192
33. Kallinich T, Muche JM, Qin S, Sterry W, Audring H, Kroczek RA. Chemokine receptor expression on neoplastic and reactive T cells in the skin at different stages of mycosis fungoides. J Invest Dermatol (2003) 121(5):1045–52. doi:10.1046/j.1523-1747.2003.12555.x
34. Wu XS, Lonsdorf AS, Hwang ST. Cutaneous T-cell lymphoma: roles for chemokines and chemokine receptors. J Invest Dermatol (2009) 129(5):1115–9. doi:10.1038/jid.2009.45
35. Sokolowska-Wojdylo M, Wenzel J, Gaffal E, Lenz J, Speuser P, Erdmann S, et al. Circulating clonal CLA(+) and CD4(+) T cells in Sezary syndrome express the skin-homing chemokine receptors CCR4 and CCR10 as well as the lymph node-homing chemokine receptor CCR7. Br J Dermatol (2005) 152:258–64. doi:10.1111/j.1365-2133.2004.06325.x
36. Narducci MG, Scala E, Bresin A, Caprini E, Picchio MC, Remotti D, et al. Skin homing of Sezary cells involves SDF-1-CXCR4 signaling and down-regulation of CD26/dipeptidylpeptidase IV. Blood (2006) 107:1108–15. doi:10.1182/blood-2005-04-1492
37. Kakinuma T, Sugaya M, Nakamura K, Kaneko F, Wakugawa M, Matsushima K, et al. Thymus and activation-regulated chemokine (TARC/CCL17) in mycosis fungoides: serum TARC levels reflect the disease activity of mycosis fungoides. J Am Acad Dermatol (2003) 48:23–30. doi:10.1067/mjd.2003.132
38. Morales J, Homey B, Vicari AP, Hudak S, Oldham E, Hedrick J, et al. CTACK, a skin-associated chemokine that preferentially attracts skin-homing memory T cells. Proc Natl Acad Sci U S A (1999) 96:14470–5. doi:10.1073/pnas.96.25.14470
39. Fujita Y, Abe R, Sasaki M, Honda A, Furuichi M, Asano Y, et al. Presence of circulating CCR10+ T cells and elevated serum CTACK/CCL27 in the early stage of mycosis fungoides. Clin Cancer Res (2006) 12:2670–5. doi:10.1158/1078-0432.CCR-05-1513
40. Goteri G, Rupoli S, Campanati A, Zizzi A, Picardi P, Cardelli M, et al. Serum and tissue CTACK/CCL27 chemokine levels in early mycosis fungoides may be correlated with disease-free survival following treatment with interferon alfa and psoralen plus ultraviolet A therapy. Br J Dermatol (2012) 166:948–52. doi:10.1111/j.1365-2133.2012.10818.x
41. Maj J, Jankowska-Konsur AM, Halon A, Wozniak Z, Plomer-Niezgoda E, Reich A. Expression of CXCR4 and CXCL12 and their correlations to the cell proliferation and angiogenesis in mycosis fungoides. Postepy Dermatol Alergol (2015) 32:437–42. doi:10.5114/pdia.2015.48034
42. Vowels BR, Cassin M, Vonderheid EC, Rook AH. Aberrant cytokine production by Sezary syndrome patients: cytokine secretion pattern resembles murine Th2 cells. J Invest Dermatol (1992) 99:90–4. doi:10.1111/1523-1747.ep12611877
43. Dummer R, Kohl O, Gillessen J, Kagi M, Burg G. Peripheral blood mononuclear cells in patients with nonleukemic cutaneous T-cell lymphoma. Reduced proliferation and preferential secretion of a T helper-2-like cytokine pattern on stimulation. Arch Dermatol (1993) 129:433–6. doi:10.1001/archderm.1993.01680250045005
44. Vowels BR, Lessin SR, Cassin M, Jaworsky C, Benoit B, Wolfe JT, et al. Th2 cytokine mRNA expression in skin in cutaneous T-cell lymphoma. J Invest Dermatol (1994) 103:669–73. doi:10.1111/1523-1747.ep12398454
45. Dummer R, Heald PW, Nestle FO, Ludwig E, Laine E, Hemmi S, et al. Sezary syndrome T-cell clones display T-helper 2 cytokines and express the accessory factor-1 (interferon-gamma receptor beta-chain). Blood (1996) 88:1383–9.
46. Asadullah K, Haeussler A, Sterry W, Docke WD, Volk HD. Interferon gamma and tumor necrosis factor alpha mRNA expression in mycosis fungoides progression. Blood (1996) 88:757–8.
47. Asadullah K, Docke WD, Haeussler A, Sterry W, Volk HD. Progression of mycosis fungoides is associated with increasing cutaneous expression of interleukin-10 mRNA. J Invest Dermatol (1996) 107:833–7. doi:10.1111/1523-1747.ep12330869
48. Guenova E, Watanabe R, Teague JE, Desimone JA, Jiang Y, Dowlatshahi M, et al. TH2 cytokines from malignant cells suppress TH1 responses and enforce a global TH2 bias in leukemic cutaneous T-cell lymphoma. Clin Cancer Res (2013) 19:3755–63. doi:10.1158/1078-0432.CCR-12-3488
49. Kaplan EH, Rosen ST, Norris DB, Roenigk HH Jr., Saks SR, Bunn PA Jr. Phase II study of recombinant human interferon gamma for treatment of cutaneous T-cell lymphoma. J Natl Cancer Inst (1990) 82:208–12. doi:10.1093/jnci/82.3.208
50. Sugaya M, Tokura Y, Hamada T, Tsuboi R, Moroi Y, Nakahara T, et al. Phase II study of i.v. interferon-gamma in Japanese patients with mycosis fungoides. J Dermatol (2014) 41:50–6. doi:10.1111/1346-8138.12341
51. Dummer R, Hassel JC, Fellenberg F, Eichmuller S, Maier T, Slos P, et al. Adenovirus-mediated intralesional interferon-gamma gene transfer induces tumor regressions in cutaneous lymphomas. Blood (2004) 104:1631–8. doi:10.1182/blood-2004-01-0360
52. Urosevic M, Fujii K, Calmels B, Laine E, Kobert N, Acres B, et al. Type I IFN innate immune response to adenovirus-mediated IFN-gamma gene transfer contributes to the regression of cutaneous lymphomas. J Clin Invest (2007) 117:2834–46. doi:10.1172/JCI32077
53. Dummer R, Eichmuller S, Gellrich S, Assaf C, Dreno B, Schiller M, et al. Phase II clinical trial of intratumoral application of TG1042 (adenovirus-interferon-gamma) in patients with advanced cutaneous T-cell lymphomas and multilesional cutaneous B-cell lymphomas. Mol Ther (2010) 18:1244–7. doi:10.1038/mt.2010.52
54. Thumann P, Luftl M, Moc I, Bagot M, Bensussan A, Schuler G, et al. Interaction of cutaneous lymphoma cells with reactive T cells and dendritic cells: implications for dendritic cell-based immunotherapy. Br J Dermatol (2003) 149:1128–42. doi:10.1111/j.1365-2133.2003.05674.x
55. Talpur R, Bassett R, Duvic M. Prevalence and treatment of Staphylococcus aureus colonization in patients with mycosis fungoides and Sezary syndrome. Br J Dermatol (2008) 159:105–12. doi:10.1111/j.1365-2133.2008.08612.x
56. Tsambiras PE, Patel S, Greene JN, Sandin RL, Vincent AL. Infectious complications of cutaneous t-cell lymphoma. Cancer Control (2001) 8:185–8. doi:10.1177/107327480100800213
57. Talpur R, Singh L, Daulat S, Liu P, Seyfer S, Trynosky T, et al. Long-term outcomes of 1,263 patients with mycosis fungoides and Sezary syndrome from 1982 to 2009. Clin Cancer Res (2012) 18:5051–60. doi:10.1158/1078-0432.CCR-12-0604
58. Showe LC, Fox FE, Williams D, Au K, Niu Z, Rook AH. Depressed IL-12-mediated signal transduction in T cells from patients with Sezary syndrome is associated with the absence of IL-12 receptor beta 2 mRNA and highly reduced levels of STAT4. J Immunol (1999) 163(7):4073–9.
59. Kopp KL, Ralfkiaer U, Gjerdrum LM, Helvad R, Pedersen IH, Litman T, et al. STAT5-mediated expression of oncogenic miR-155 in cutaneous T-cell lymphoma. Cell Cycle (2013) 12(12):1939–47. doi:10.4161/cc.24987
60. Netchiporouk E, Litvinov IV, Moreau L, Gilbert M, Sasseville D, Duvic M. Deregulation in STAT signaling is important for cutaneous T-cell lymphoma (CTCL) pathogenesis and cancer progression. Cell Cycle (2014) 13(21):3331–5. doi:10.4161/15384101.2014.965061
61. Rook AH, Kubin M, Fox FE, Niu Z, Cassin M, Vowels BR, et al. The potential therapeutic role of interleukin-12 in cutaneous T-cell lymphoma. Ann N Y Acad Sci (1996) 795:310–8. doi:10.1111/j.1749-6632.1996.tb52680.x
62. van der Fits L, Out-Luiting JJ, van Leeuwen MA, Samsom JN, Willemze R, Tensen CP, et al. Autocrine IL-21 stimulation is involved in the maintenance of constitutive STAT3 activation in Sezary syndrome. J Invest Dermatol (2012) 132(2):440–7. doi:10.1038/jid.2011.293
63. Qin JZ, Kamarashev J, Zhang CL, Dummer R, Burg G, Dobbeling U. Constitutive and interleukin-7- and interleukin-15-stimulated DNA binding of STAT and novel factors in cutaneous T cell lymphoma cells. J Invest Dermatol (2001) 117(3):583–9. doi:10.1046/j.0022-202x.2001.01436.x
64. Nielsen M, Kaestel CG, Eriksen KW, Woetmann A, Stokkedal T, Kaltoft K, et al. Inhibition of constitutively activated Stat3 correlates with altered Bcl-2/Bax expression and induction of apoptosis in mycosis fungoides tumor cells. Leukemia (1999) 13(5):735–8. doi:10.1038/sj.leu.2401415
65. van Kester MS, Out-Luiting JJ, von dem Borne PA, Willemze R, Tensen CP, Vermeer MH. Cucurbitacin I inhibits Stat3 and induces apoptosis in Sezary cells. J Invest Dermatol (2008) 128(7):1691–5. doi:10.1038/sj.jid.5701246
66. Gibson HM, Mishra A, Chan DV, Hake TS, Porcu P, Wong HK. Impaired proteasome function activates GATA3 in T cells and upregulates CTLA-4: relevance for Sezary syndrome. J Invest Dermatol (2013) 133(1):249–57. doi:10.1038/jid.2012.265
67. Liotta LA, Kohn EC. The microenvironment of the tumour-host interface. Nature (2001) 411:375–9. doi:10.1038/35077241
68. Miyagaki T, Sugaya M, Fujita H, Ohmatsu H, Kakinuma T, Kadono T, et al. Eotaxins and CCR3 interaction regulates the Th2 environment of cutaneous T-cell lymphoma. J Invest Dermatol (2010) 130:2304–11. doi:10.1038/jid.2010.128
69. Kleinhans M, Tun-Kyi A, Gilliet M, Kadin ME, Dummer R, Burg G, et al. Functional expression of the eotaxin receptor CCR3 in CD30+ cutaneous T-cell lymphoma. Blood (2003) 101:1487–93. doi:10.1182/blood-2002-02-0475
70. Sallusto F, Mackay CR, Lanzavecchia A. Selective expression of the eotaxin receptor CCR3 by human T helper 2 cells. Science (1997) 277:2005–7. doi:10.1126/science.277.5334.2005
71. Forssmann U, Uguccioni M, Loetscher P, Dahinden CA, Langen H, Thelen M, et al. Eotaxin-2, a novel CC chemokine that is selective for the chemokine receptor CCR3, and acts like eotaxin on human eosinophil and basophil leukocytes. J Exp Med (1997) 185:2171–6. doi:10.1084/jem.185.12.2171
72. Kikuchi Y, Kunita A, Iwata C, Komura D, Nishiyama T, Shimazu K, et al. The niche component periostin is produced by cancer-associated fibroblasts, supporting growth of gastric cancer through ERK activation. Am J Pathol (2014) 184:859–70. doi:10.1016/j.ajpath.2013.11.012
73. Furudate S, Fujimura T, Kakizaki A, Kambayashi Y, Asano M, Watabe A, et al. The possible interaction between periostin expressed by cancer stroma and tumor-associated macrophages in developing mycosis fungoides. Exp Dermatol (2016) 25:107–12. doi:10.1111/exd.12873
74. Takahashi N, Sugaya M, Suga H, Oka T, Kawaguchi M, Miyagaki T, et al. Thymic stromal chemokine TSLP Acts through Th2 cytokine production to induce cutaneous T-cell lymphoma. Cancer Res (2016) 76:6241–52. doi:10.1158/0008-5472.CAN-16-0992
75. Soumelis V, Reche PA, Kanzler H, Yuan W, Edward G, Homey B, et al. Human epithelial cells trigger dendritic cell mediated allergic inflammation by producing TSLP. Nat Immunol (2002) 3:673–80. doi:10.1038/ni805
76. Miyagaki T, Sugaya M, Fujita H, Saeki H, Tamaki K. Increased serum thymic stromal lymphopoietin levels in patients with cutaneous T cell lymphoma. Clin Exp Dermatol (2009) 34:539–40. doi:10.1111/j.1365-2230.2008.02990.x
77. Tuzova M, Richmond J, Wolpowitz D, Curiel-Lewandrowski C, Chaney K, Kupper T, et al. CCR4+T cell recruitment to the skin in mycosis fungoides: potential contributions by thymic stromal lymphopoietin and interleukin-16. Leuk Lymphoma (2015) 56:440–9. doi:10.3109/10428194.2014.919634
78. Mantovani A, Bottazzi B, Colotta F, Sozzani S, Ruco L. The origin and function of tumor-associated macrophages. Immunol Today (1992) 13:265–70. doi:10.1016/0167-5699(92)90008-U
79. Hao NB, Lu MH, Fan YH, Cao YL, Zhang ZR, Yang SM. Macrophages in tumor microenvironments and the progression of tumors. Clin Dev Immunol (2012) 2012:948098. doi:10.1155/2012/948098
80. Bingle L, Brown NJ, Lewis CE. The role of tumour-associated macrophages in tumour progression: implications for new anticancer therapies. J Pathol (2002) 196:254–65. doi:10.1002/path.1027
81. Sugaya M, Miyagaki T, Ohmatsu H, Suga H, Kai H, Kamata M, et al. Association of the numbers of CD163(+) cells in lesional skin and serum levels of soluble CD163 with disease progression of cutaneous T cell lymphoma. J Dermatol Sci (2012) 68:45–51. doi:10.1016/j.jdermsci.2012.07.007
82. Assaf C, Hwang ST. Mac attack: macrophages as key drivers of cutaneous T-cell lymphoma pathogenesis. Exp Dermatol (2016) 25:105–6. doi:10.1111/exd.12894
83. Tada K, Hamada T, Asagoe K, Umemura H, Mizuno-Ikeda K, Aoyama Y, et al. Increase of DC-LAMP+ mature dendritic cell subsets in dermatopathic lymphadenitis of mycosis fungoides. Eur J Dermatol (2014) 24:670–5. doi:10.1684/ejd.2014.2437
84. Wu X, Schulte BC, Zhou Y, Haribhai D, Mackinnon AC, Plaza JA, et al. Depletion of M2-like tumor-associated macrophages delays cutaneous T-cell lymphoma development in vivo. J Invest Dermatol (2014) 134:2814–22. doi:10.1038/jid.2014.206
85. Kodelja V, Muller C, Politz O, Hakij N, Orfanos CE, Goerdt S. Alternative macrophage activation-associated CC-chemokine-1, a novel structural homologue of macrophage inflammatory protein-1 alpha with a Th2-associated expression pattern. J Immunol (1998) 160:1411–8.
86. Islam SA, Ling MF, Leung J, Shreffler WG, Luster AD. Identification of human CCR8 as a CCL18 receptor. J Exp Med (2013) 210:1889–98. doi:10.1084/jem.20130240
87. Gunther C, Zimmermann N, Berndt N, Grosser M, Stein A, Koch A, et al. Up-regulation of the chemokine CCL18 by macrophages is a potential immunomodulatory pathway in cutaneous T-cell lymphoma. Am J Pathol (2011) 179:1434–42. doi:10.1016/j.ajpath.2011.05.040
88. Miyagaki T, Sugaya M, Suga H, Ohmatsu H, Fujita H, Asano Y, et al. Increased CCL18 expression in patients with cutaneous T-cell lymphoma: association with disease severity and prognosis. J Eur Acad Dermatol Venereol (2013) 27:e60–7. doi:10.1111/j.1468-3083.2012.04495.x
89. Kim YH, Tavallaee M, Sundram U, Salva KA, Wood GS, Li S, et al. Phase II investigator-initiated study of brentuximab vedotin in mycosis fungoides and Sezary syndrome with variable CD30 expression level: a multi-institution collaborative project. J Clin Oncol (2015) 33:3750–8. doi:10.1200/JCO.2014.60.3969
90. Banchereau J, Steinman RM. Dendritic cells and the control of immunity. Nature (1998) 392:245–52. doi:10.1038/32588
91. Luftl M, Feng A, Licha E, Schuler G. Dendritic cells and apoptosis in mycosis fungoides. Br J Dermatol (2002) 147:1171–9. doi:10.1046/j.1365-2133.2002.04994.x
92. Schlapbach C, Ochsenbein A, Kaelin U, Hassan AS, Hunger RE, Yawalkar N. High numbers of DC-SIGN+ dendritic cells in lesional skin of cutaneous T-cell lymphoma. J Am Acad Dermatol (2010) 62:995–1004. doi:10.1016/j.jaad.2009.06.082
93. Yamaguchi J, Aihara M, Kobayashi Y, Kambara T, Ikezawa Z. Quantitative analysis of nerve growth factor (NGF) in the atopic dermatitis and psoriasis horny layer and effect of treatment on NGF in atopic dermatitis. J Dermatol Sci (2009) 53:48–54. doi:10.1016/j.jdermsci.2008.08.011
94. Suga H, Sugaya M, Miyagaki T, Ohmatsu H, Fujita H, Kagami S, et al. Association of nerve growth factor, chemokine (C-C motif) ligands and immunoglobulin E with pruritus in cutaneous T-cell lymphoma. Acta Derm Venereol (2013) 93:144–9. doi:10.2340/00015555-1428
95. Ribatti D, Crivellato E. Mast cells, angiogenesis and cancer. Adv Exp Med Biol (2011) 716:270–88. doi:10.1007/978-1-4419-9533-9_14
96. Rabenhorst A, Schlaak M, Heukamp LC, Forster A, Theurich S, von Bergwelt-Baildon M, et al. Mast cells play a protumorigenic role in primary cutaneous lymphoma. Blood (2012) 120:2042–54. doi:10.1182/blood-2012-03-415638
97. Zheng Y, Valdez PA, Danilenko DM, Hu Y, Sa SM, Gong Q, et al. Interleukin-22 mediates early host defense against attaching and effacing bacterial pathogens. Nat Med (2008) 14:282–9. doi:10.1038/nm1720
98. Shimauchi T, Sasada K, Kito Y, Mori T, Hata M, Fujiyama T, et al. CD8+ Sezary syndrome with interleukin-22 production modulated by bacterial sepsis. Br J Dermatol (2013) 168:881–3. doi:10.1111/bjd.12051
99. Gabrilovich DI, Nagaraj S. Myeloid-derived suppressor cells as regulators of the immune system. Nat Rev Immunol (2009) 9:162–74. doi:10.1038/nri2506
100. Pileri A, Agostinelli C, Sessa M, Quaglino P, Santucci M, Tomasini C, et al. Langerhans, plasmacytoid dendritic and myeloid-derived suppressor cell levels in mycosis fungoides vary according to the stage of the disease. Virchows Arch (2017) 470:575–82. doi:10.1007/s00428-017-2107-1
101. Samimi S, Benoit B, Evans K, Wherry EJ, Showe L, Wysocka M, et al. Increased programmed death-1 expression on CD4+ T cells in cutaneous T-cell lymphoma: implications for immune suppression. Arch Dermatol (2010) 146(12):1382–8. doi:10.1001/archdermatol.2010.200
102. Kantekure K, Yang Y, Raghunath P, Schaffer A, Woetmann A, Zhang Q, et al. Expression patterns of the immunosuppressive proteins PD-1/CD279 and PD-L1/CD274 at different stages of cutaneous T-cell lymphoma/mycosis fungoides. Am J Dermatopathol (2012) 34(1):126–8. doi:10.1097/DAD.0b013e31821c35cb
103. Wong HK, Wilson AJ, Gibson HM, Hafner MS, Hedgcock CJ, Berger CL, et al. Increased expression of CTLA-4 in malignant T-cells from patients with mycosis fungoides – cutaneous T cell lymphoma. J Invest Dermatol (2006) 126(1):212–9. doi:10.1038/sj.jid.5700029
104. Bosisio FM, Cerroni L. Expression of T-follicular helper markers in sequential biopsies of progressive mycosis fungoides and other primary cutaneous T-cell lymphomas. Am J Dermatopathol (2015) 37(2):115–21. doi:10.1097/DAD.0000000000000258
105. Wilcox RA. Cutaneous T-cell lymphoma: 2017 update on diagnosis, risk-stratification, and management. Am J Hematol (2017) 92:1085–102. doi:10.1002/ajh.24876
106. Hughes CF, Khot A, McCormack C, Lade S, Westerman DA, Twigger R, et al. Lack of durable disease control with chemotherapy for mycosis fungoides and Sezary syndrome: a comparative study of systemic therapy. Blood (2015) 125:71–81. doi:10.1182/blood-2014-07-588236
107. Akilov OE, Geskin L. Therapeutic advances in cutaneous T-cell lymphoma. Skin Therapy Lett (2011) 16:1–5.
108. Olsen EA, Rosen ST, Vollmer RT, Variakojis D, Roenigk HH Jr., Diab N, et al. Interferon alfa-2a in the treatment of cutaneous T cell lymphoma. J Am Acad Dermatol (1989) 20:395–407. doi:10.1016/S0190-9622(89)70049-9
109. Suchin KR, Cucchiara AJ, Gottleib SL, Wolfe JT, DeNardo BJ, Macey WH, et al. Treatment of cutaneous T-cell lymphoma with combined immunomodulatory therapy: a 14-year experience at a single institution. Arch Dermatol (2002) 138:1054–60. doi:10.1001/archderm.138.8.1054
110. Pichardo DA, Querfeld C, Guitart J, Kuzel TM, Rosen ST. Cutaneous T-cell lymphoma: a paradigm for biological therapies. Leuk Lymphoma (2004) 45:1755–65. doi:10.1080/10428190410001693560
111. Furudate S, Fujimura T, Kakizaki A, Hidaka T, Asano M, Aiba S. Tumor-associated M2 macrophages in mycosis fungoides acquire immunomodulatory function by interferon alpha and interferon gamma. J Dermatol Sci (2016) 83:182–9. doi:10.1016/j.jdermsci.2016.05.004
112. Sauder DN. Immunomodulatory and pharmacologic properties of imiquimod. J Am Acad Dermatol (2000) 43(1 Pt 2):S6–11. doi:10.1067/mjd.2000.107808
113. Suchin KR, Junkins-Hopkins JM, Rook AH. Treatment of stage IA cutaneous T-Cell lymphoma with topical application of the immune response modifier imiquimod. Arch Dermatol (2002) 138:1137–9. doi:10.1001/archderm.138.9.1137
114. Dummer R, Urosevic M, Kempf W, Kazakov D, Burg G. Imiquimod induces complete clearance of a PUVA-resistant plaque in mycosis fungoides. Dermatology (2003) 207:116–8. doi:10.1159/000070962
115. Coors EA, Schuler G, Von Den Driesch P. Topical imiquimod as treatment for different kinds of cutaneous lymphoma. Eur J Dermatol (2006) 16:391–3.
116. Gordon MC, Sluzevich JC, Jambusaria-Pahlajani A. Clearance of folliculotropic and tumor mycosis fungoides with topical 5% imiquimod. JAAD Case Rep (2015) 1:348–50. doi:10.1016/j.jdcr.2015.08.007
117. Rook AH, Gelfand JM, Wysocka M, Troxel AB, Benoit B, Surber C, et al. Topical resiquimod can induce disease regression and enhance T-cell effector functions in cutaneous T-cell lymphoma. Blood (2015) 126:1452–61. doi:10.1182/blood-2015-02-630335
118. Gorden KB, Gorski KS, Gibson SJ, Kedl RM, Kieper WC, Qiu X, et al. Synthetic TLR agonists reveal functional differences between human TLR7 and TLR8. J Immunol (2005) 174:1259–68. doi:10.4049/jimmunol.174.3.1259
119. Marks P, Rifkind RA, Richon VM, Breslow R, Miller T, Kelly WK. Histone deacetylases and cancer: causes and therapies. Nat Rev Cancer (2001) 1:194–202. doi:10.1038/35106079
120. Marks PA, Jiang X. Histone deacetylase inhibitors in programmed cell death and cancer therapy. Cell Cycle (2005) 4:549–51. doi:10.4161/cc.4.4.1564
121. Olsen EA, Kim YH, Kuzel TM, Pacheco TR, Foss FM, Parker S, et al. Phase IIb multicenter trial of vorinostat in patients with persistent, progressive, or treatment refractory cutaneous T-cell lymphoma. J Clin Oncol (2007) 25:3109–15. doi:10.1200/JCO.2006.10.2434
122. Duvic M, Talpur R, Ni X, Zhang C, Hazarika P, Kelly C, et al. Phase 2 trial of oral vorinostat (suberoylanilide hydroxamic acid, SAHA) for refractory cutaneous T-cell lymphoma (CTCL). Blood (2007) 109:31–9. doi:10.1182/blood-2006-06-025999
123. Piekarz RL, Frye R, Turner M, Wright JJ, Allen SL, Kirschbaum MH, et al. Phase II multi-institutional trial of the histone deacetylase inhibitor romidepsin as monotherapy for patients with cutaneous T-cell lymphoma. J Clin Oncol (2009) 27:5410–7. doi:10.1200/JCO.2008.21.6150
124. Whittaker SJ, Demierre MF, Kim EJ, Rook AH, Lerner A, Duvic M, et al. Final results from a multicenter, international, pivotal study of romidepsin in refractory cutaneous T-cell lymphoma. J Clin Oncol (2010) 28:4485–91. doi:10.1200/JCO.2010.28.9066
125. Wada H, Tsuboi R, Kato Y, Sugaya M, Tobinai K, Hamada T, et al. Phase I and pharmacokinetic study of the oral histone deacetylase inhibitor vorinostat in Japanese patients with relapsed or refractory cutaneous T-cell lymphoma. J Dermatol (2012) 39:823–8. doi:10.1111/j.1346-8138.2012.01554.x
126. Kim YH, Demierre MF, Kim EJ, Lerner A, Rook AH, Duvic M, et al. Clinically meaningful reduction in pruritus in patients with cutaneous T-cell lymphoma treated with romidepsin. Leuk Lymphoma (2013) 54:284–9. doi:10.3109/10428194.2012.711829
127. Cedeno-Laurent F, Singer EM, Wysocka M, Benoit BM, Vittorio CC, Kim EJ, et al. Improved pruritus correlates with lower levels of IL-31 in CTCL patients under different therapeutic modalities. Clin Immunol (2015) 158:1–7. doi:10.1016/j.clim.2015.02.014
128. Francisco JA, Cerveny CG, Meyer DL, Mixan BJ, Klussman K, Chace DF, et al. cAC10-vcMMAE, an anti-CD30-monomethyl auristatin E conjugate with potent and selective antitumor activity. Blood (2003) 102:1458–65. doi:10.1182/blood-2003-01-0039
129. Younes A, Gopal AK, Smith SE, Ansell SM, Rosenblatt JD, Savage KJ, et al. Results of a pivotal phase II study of brentuximab vedotin for patients with relapsed or refractory Hodgkin’s lymphoma. J Clin Oncol (2012) 30:2183–9. doi:10.1200/JCO.2011.38.0410
130. Forero-Torres A, Fanale M, Advani R, Bartlett NL, Rosenblatt JD, Kennedy DA, et al. Brentuximab vedotin in transplant-naive patients with relapsed or refractory Hodgkin lymphoma: analysis of two phase I studies. Oncologist (2012) 17:1073–80. doi:10.1634/theoncologist.2012-0133
131. Prince HM, Kim YH, Horwitz SM, Dummer R, Scarisbrick J, Quaglino P, et al. Brentuximab vedotin or physician’s choice in CD30-positive cutaneous T-cell lymphoma (ALCANZA): an international, open-label, randomised, phase 3, multicentre trial. Lancet (2017) 390:555–66. doi:10.1016/S0140-6736(17)31266-7
132. Ogura M, Ishida T, Hatake K, Taniwaki M, Ando K, Tobinai K, et al. Multicenter phase II study of mogamulizumab (KW-0761), a defucosylated anti-cc chemokine receptor 4 antibody, in patients with relapsed peripheral T-cell lymphoma and cutaneous T-cell lymphoma. J Clin Oncol (2014) 32(11):1157–63. doi:10.1200/JCO.2013.52.0924
133. Duvic M, Pinter-Brown LC, Foss FM, Sokol L, Jorgensen JL, Challagundla P, et al. Phase 1/2 study of mogamulizumab, a defucosylated anti-CCR4 antibody, in previously treated patients with cutaneous T-cell lymphoma. Blood (2015) 125(12):1883–9. doi:10.1182/blood-2014-09-600924
134. Sicard H, Bonnafous C, Morel A, Bagot M, Bensussan A, Marie-Cardine A. A novel targeted immunotherapy for CTCL is on its way: anti-KIR3DL2 mAb IPH4102 is potent and safe in non-clinical studies. Oncoimmunology (2015) 4(9):e1022306. doi:10.1080/2162402X.2015.1022306
135. Bagot M. New targeted treatments for cutaneous T-cell Lymphomas. Indian J Dermatol (2017) 62(2):142–5. doi:10.4103/ijd.IJD_73_17
136. Querfeld C, Rosen ST, Guitart J, Duvic M, Kim YH, Dusza SW, et al. Results of an open-label multicenter phase 2 trial of lenalidomide monotherapy in refractory mycosis fungoides and Sezary syndrome. Blood (2014) 123(8):1159–66. doi:10.1182/blood-2013-09-525915
137. Zinzani PL, Musuraca G, Tani M, Stefoni V, Marchi E, Fina M, et al. Phase II trial of proteasome inhibitor bortezomib in patients with relapsed or refractory cutaneous T-cell lymphoma. J Clin Oncol (2007) 25(27):4293–7. doi:10.1200/JCO.2007.11.4207
138. Kempf W, Pfaltz K, Vermeer MH, Cozzio A, Ortiz-Romero PL, Bagot M, et al. EORTC, ISCL, and USCLC consensus recommendations for the treatment of primary cutaneous CD30-positive lymphoproliferative disorders: lymphomatoid papulosis and primary cutaneous anaplastic large-cell lymphoma. Blood (2011) 118:4024–35. doi:10.1182/blood-2011-05-351346
139. Stein H, Foss HD, Durkop H, Marafioti T, Delsol G, Pulford K, et al. CD30(+) anaplastic large cell lymphoma: a review of its histopathologic, genetic, and clinical features. Blood (2000) 96:3681–95.
140. Kadin ME, Carpenter C. Systemic and primary cutaneous anaplastic large cell lymphomas. Semin Hematol (2003) 40:244–56. doi:10.1016/S0037-1963(03)00138-0
141. Fornari A, Piva R, Chiarle R, Novero D, Inghirami G. Anaplastic large cell lymphoma: one or more entities among T-cell lymphoma? Hematol Oncol (2009) 27:161–70. doi:10.1002/hon.897
142. Wada DA, Law ME, Hsi ED, Dicaudo DJ, Ma L, Lim MS, et al. Specificity of IRF4 translocations for primary cutaneous anaplastic large cell lymphoma: a multicenter study of 204 skin biopsies. Mod Pathol (2011) 24(4):596–605. doi:10.1038/modpathol.2010.225
143. Bekkenk MW, Geelen FA, van Voorst Vader PC, Heule F, Geerts ML, van Vloten WA, et al. Primary and secondary cutaneous CD30(+) lymphoproliferative disorders: a report from the Dutch Cutaneous Lymphoma Group on the long-term follow-up data of 219 patients and guidelines for diagnosis and treatment. Blood (2000) 95:3653–61.
144. Fujita A, Hamada T, Iwatsuki K. Retrospective analysis of 133 patients with cutaneous lymphomas from a single Japanese medical center between 1995 and 2008. J Dermatol (2011) 38:524–30. doi:10.1111/j.1346-8138.2010.01049.x
145. Lee WJ, Moon IJ, Lee SH, Won CH, Chang SE, Choi JH, et al. Cutaneous anaplastic large-cell lymphoma (ALCL): A comparative clinical feature and survival outcome analysis of 52 cases according to primary tumor site. J Am Acad Dermatol (2016) 74:1135–43. doi:10.1016/j.jaad.2015.12.053
146. Kempf W. A new era for cutaneous CD30-positive T-cell lymphoproliferative disorders. Semin Diagn Pathol (2017) 34:22–35. doi:10.1053/j.semdp.2016.11.005
147. Burg G, Kempf W, Kazakov DV, Dummer R, Frosch PJ, Lange-Ionescu S, et al. Pyogenic lymphoma of the skin: a peculiar variant of primary cutaneous neutrophil-rich CD30+ anaplastic large-cell lymphoma. Clinicopathological study of four cases and review of the literature. Br J Dermatol (2003) 148:580–6. doi:10.1046/j.1365-2133.2003.05248.x
148. Geissinger E, Sadler P, Roth S, Grieb T, Puppe B, Muller N, et al. Disturbed expression of the T-cell receptor/CD3 complex and associated signaling molecules in CD30+ T-cell lymphoproliferations. Haematologica (2010) 95:1697–704. doi:10.3324/haematol.2009.021428
149. Kadin ME. Primary Ki-1-positive anaplastic large-cell lymphoma: a distinct clinicopathologic entity. Ann Oncol (1994) 5(Suppl 1):25–30. doi:10.1093/annonc/5.suppl_1.S25
150. El Shabrawi-Caelen L, Kerl H, Cerroni L. Lymphomatoid papulosis: reappraisal of clinicopathologic presentation and classification into subtypes A, B, and C. Arch Dermatol (2004) 140:441–7. doi:10.1001/archderm.140.4.441
151. Saggini A, Gulia A, Argenyi Z, Fink-Puches R, Lissia A, Magana M, et al. A variant of lymphomatoid papulosis simulating primary cutaneous aggressive epidermotropic CD8+ cytotoxic T-cell lymphoma. Description of 9 cases. Am J Surg Pathol (2010) 34:1168–75. doi:10.1097/PAS.0b013e3181e75356
152. Kempf W, Kazakov DV, Scharer L, Rutten A, Mentzel T, Paredes BE, et al. Angioinvasive lymphomatoid papulosis: a new variant simulating aggressive lymphomas. Am J Surg Pathol (2013) 37:1–13. doi:10.1097/PAS.0b013e3182648596
153. Paulli M, Berti E, Rosso R, Boveri E, Kindl S, Klersy C, et al. CD30/Ki-1-positive lymphoproliferative disorders of the skin – clinicopathologic correlation and statistical analysis of 86 cases: a multicentric study from the European Organization for Research and Treatment of Cancer Cutaneous Lymphoma Project Group. J Clin Oncol (1995) 13:1343–54. doi:10.1200/JCO.1995.13.6.1343
154. Zackheim HS, Jones C, Leboit PE, Kashani-Sabet M, McCalmont TH, Zehnder J. Lymphomatoid papulosis associated with mycosis fungoides: a study of 21 patients including analyses for clonality. J Am Acad Dermatol (2003) 49:620–3. doi:10.1067/S0190-9622(03)01577-9
155. Weichenthal M, Goldinger S, Wehkamp U, Beyer M, Stein A, Tsianakas A, et al. Response of rare variants of cutaneous T cell lymphoma (CTCL) to treatment with bexarotene. A prospective German DeCOG trial. Blood (2013) 122:4379.
156. Foss F, Advani R, Duvic M, Hymes KB, Intragumtornchai T, Lekhakula A, et al. A Phase II trial of Belinostat (PXD101) in patients with relapsed or refractory peripheral or cutaneous T-cell lymphoma. Br J Haematol (2015) 168(6):811–9. doi:10.1111/bjh.13222
157. Lamant L, Pileri S, Sabattini E, Brugieres L, Jaffe ES, Delsol G. Cutaneous presentation of ALK-positive anaplastic large cell lymphoma following insect bites: evidence for an association in five cases. Haematologica (2010) 95(3):449–55. doi:10.3324/haematol.2009.015024
158. Yoshie O, Fujisawa R, Nakayama T, Harasawa H, Tago H, Izawa D, et al. Frequent expression of CCR4 in adult T-cell leukemia and human T-cell leukemia virus type 1-transformed T cells. Blood (2002) 99:1505–11. doi:10.1182/blood.V99.5.1505
159. Shimoyama M. Diagnostic criteria and classification of clinical subtypes of adult T-cell leukaemia-lymphoma. A report from the Lymphoma Study Group (1984-87). Br J Haematol (1991) 79:428–37. doi:10.1111/j.1365-2141.1991.tb08051.x
160. Nosaka K, Iwanaga M, Imaizumi Y, Ishitsuka K, Ishizawa K, Ishida Y, et al. Epidemiological and clinical features of adult T-cell leukemia-lymphoma in Japan, 2010-2011: a nationwide survey. Cancer Sci (2017) 108:2478–86. doi:10.1111/cas.13398
161. Bittencourt AL, Barbosa HS, Vieira MD, Farre L. Adult T-cell leukemia/lymphoma (ATL) presenting in the skin: clinical, histological and immunohistochemical features of 52 cases. Acta Oncol (2009) 48:598–604. doi:10.1080/02841860802657235
162. Setoyama M, Katahira Y, Kanzaki T. Clinicopathologic analysis of 124 cases of adult T-cell leukemia/lymphoma with cutaneous manifestations: the smouldering type with skin manifestations has a poorer prognosis than previously thought. J Dermatol (1999) 26:785–90. doi:10.1111/j.1346-8138.1999.tb02093.x
163. Tsukasaki K, Imaizumi Y, Tokura Y, Ohshima K, Kawai K, Utsunomiya A, et al. Meeting report on the possible proposal of an extranodal primary cutaneous variant in the lymphoma type of adult T-cell leukemia-lymphoma. J Dermatol (2014) 41:26–8. doi:10.1111/1346-8138.12374
164. Sawada Y, Hino R, Hama K, Ohmori S, Fueki H, Yamada S, et al. Type of skin eruption is an independent prognostic indicator for adult T-cell leukemia/lymphoma. Blood (2011) 117:3961–7. doi:10.1182/blood-2010-11-316794
165. Tokura Y, Sawada Y, Shimauchi T. Skin manifestations of adult T-cell leukemia/lymphoma: clinical, cytological and immunological features. J Dermatol (2014) 41:19–25. doi:10.1111/1346-8138.12328
166. Tanaka Y, Mine S, Figdor CG, Wake A, Hirano H, Tsukada J, et al. Constitutive chemokine production results in activation of leukocyte function-associated antigen-1 on adult T-cell leukemia cells. Blood (1998) 91:3909–19.
167. Mori N, Ueda A, Ikeda S, Yamasaki Y, Yamada Y, Tomonaga M, et al. Human T-cell leukemia virus type I tax activates transcription of the human monocyte chemoattractant protein-1 gene through two nuclear factor-kappaB sites. Cancer Res (2000) 60:4939–45.
168. Ruckes T, Saul D, Van Snick J, Hermine O, Grassmann R. Autocrine antiapoptotic stimulation of cultured adult T-cell leukemia cells by overexpression of the chemokine I-309. Blood (2001) 98:1150–9. doi:10.1182/blood.V98.4.1150
169. Ishida T, Utsunomiya A, Iida S, Inagaki H, Takatsuka Y, Kusumoto S, et al. Clinical significance of CCR4 expression in adult T-cell leukemia/lymphoma: its close association with skin involvement and unfavorable outcome. Clin Cancer Res (2003) 9(10 Pt 1):3625–34.
170. Hasegawa H, Nomura T, Kohno M, Tateishi N, Suzuki Y, Maeda N, et al. Increased chemokine receptor CCR7/EBI1 expression enhances the infiltration of lymphoid organs by adult T-cell leukemia cells. Blood (2000) 95:30–8.
171. Shimauchi T, Imai S, Hino R, Tokura Y. Production of thymus and activation-regulated chemokine and macrophage-derived chemokine by CCR4+ adult T-cell leukemia cells. Clin Cancer Res (2005) 11:2427–35. doi:10.1158/1078-0432.CCR-04-0491
172. Karube K, Ohshima K, Tsuchiya T, Yamaguchi T, Kawano R, Suzumiya J, et al. Expression of FoxP3, a key molecule in CD4CD25 regulatory T cells, in adult T-cell leukaemia/lymphoma cells. Br J Haematol (2004) 126:81–4. doi:10.1111/j.1365-2141.2004.04999.x
173. Kohno T, Yamada Y, Akamatsu N, Kamihira S, Imaizumi Y, Tomonaga M, et al. Possible origin of adult T-cell leukemia/lymphoma cells from human T lymphotropic virus type-1-infected regulatory T cells. Cancer Sci (2005) 96:527–33. doi:10.1111/j.1349-7006.2005.00080.x
174. Shimauchi T, Kabashima K, Tokura Y. Adult T-cell leukemia/lymphoma cells from blood and skin tumors express cytotoxic T lymphocyte-associated antigen-4 and Foxp3 but lack suppressor activity toward autologous CD8+ T cells. Cancer Sci (2008) 99:98–106. doi:10.1111/j.1349-7006.2007.00646.x
175. Eyerich K, Pennino D, Scarponi C, Foerster S, Nasorri F, Behrendt H, et al. IL-17 in atopic eczema: linking allergen-specific adaptive and microbial-triggered innate immune response. J Allergy Clin Immunol (2009) 123:59–66e4. doi:10.1016/j.jaci.2008.10.031
176. Sawada Y, Nakamura M, Kabashima-Kubo R, Shimauchi T, Kobayashi M, Tokura Y. Defective epidermal innate immunity and resultant superficial dermatophytosis in adult T-cell leukemia/lymphoma. Clin Cancer Res (2012) 18:3772–9. doi:10.1158/1078-0432.CCR-12-0292
177. Peric M, Koglin S, Kim SM, Morizane S, Besch R, Prinz JC, et al. IL-17A enhances vitamin D3-induced expression of cathelicidin antimicrobial peptide in human keratinocytes. J Immunol (2008) 181:8504–12. doi:10.4049/jimmunol.181.12.8504
178. Sawada Y, Nakamura M, Kabashima-Kubo R, Shimauchi T, Kobayashi M, Tokura Y. Defective epidermal induction of S100A7/psoriasin associated with low frequencies of skin-infiltrating Th17 cells in dermatophytosis-prone adult T cell leukemia/lymphoma. Clin Immunol (2013) 148:1–3. doi:10.1016/j.clim.2013.03.013
179. Kabashima R, Kabashima K, Hino R, Shimauchi T, Tokura Y. Scabies superimposed on skin lesions of adult T-cell leukemia/lymphoma: case report and literature review. Int J Dermatol (2008) 47:1168–71. doi:10.1111/j.1365-4632.2008.03707.x
180. Kozako T, Yoshimitsu M, Fujiwara H, Masamoto I, Horai S, White Y, et al. PD-1/PD-L1 expression in human T-cell leukemia virus type 1 carriers and adult T-cell leukemia/lymphoma patients. Leukemia (2009) 23:375–82. doi:10.1038/leu.2008.272
181. Shimauchi T, Kabashima K, Nakashima D, Sugita K, Yamada Y, Hino R, et al. Augmented expression of programmed death-1 in both neoplastic and non-neoplastic CD4+ T-cells in adult T-cell leukemia/lymphoma. Int J Cancer (2007) 121:2585–90. doi:10.1002/ijc.23042
182. Kataoka K, Shiraishi Y, Takeda Y, Sakata S, Matsumoto M, Nagano S, et al. Aberrant PD-L1 expression through 3’-UTR disruption in multiple cancers. Nature (2016) 534:402–6. doi:10.1038/nature18294
183. Ishida T, Joh T, Uike N, Yamamoto K, Utsunomiya A, Yoshida S, et al. Defucosylated anti-CCR4 monoclonal antibody (KW-0761) for relapsed adult T-cell leukemia-lymphoma: a multicenter phase II study. J Clin Oncol (2012) 30:837–42. doi:10.1200/JCO.2011.37.3472
184. Tokunaga M, Yonekura K, Nakamura D, Haraguchi K, Tabuchi T, Fujino S, et al. Clinical significance of cutaneous adverse reaction to mogamulizumab in relapsed or refractory adult T-cell leukaemia-lymphoma. Br J Haematol (2018) 181:539–42. doi:10.1111/bjh.14634
185. Yonekura K, Tokunaga M, Kawakami N, Takeda K, Kanzaki T, Nakano N, et al. Cutaneous adverse reaction to mogamulizumab may indicate favourable prognosis in adult T-cell leukaemia-lymphoma. Acta Derm Venereol (2016) 96:1000–2. doi:10.2340/00015555-2421
186. Ishida T, Ito A, Sato F, Kusumoto S, Iida S, Inagaki H, et al. Stevens-Johnson syndrome associated with mogamulizumab treatment of adult T-cell leukemia/lymphoma. Cancer Sci (2013) 104:647–50. doi:10.1111/cas.12116
187. Yonekura K, Kanzaki T, Gunshin K, Kawakami N, Takatsuka Y, Nakano N, et al. Effect of anti-CCR4 monoclonal antibody (mogamulizumab) on adult T-cell leukemia-lymphoma: cutaneous adverse reactions may predict the prognosis. J Dermatol (2014) 41:239–44. doi:10.1111/1346-8138.12419
188. Fuji S, Inoue Y, Utsunomiya A, Moriuchi Y, Uchimaru K, Choi I, et al. Pretransplantation Anti-CCR4 antibody mogamulizumab against adult T-cell leukemia/lymphoma is associated with significantly increased risks of severe and corticosteroid-refractory graft-versus-host disease, nonrelapse mortality, and overall mortality. J Clin Oncol (2016) 34:3426–33. doi:10.1200/JCO.2016.67.8250
189. Sugio T, Kato K, Aoki T, Ohta T, Saito N, Yoshida S, et al. Mogamulizumab treatment prior to allogeneic hematopoietic stem cell transplantation induces severe acute graft-versus-host disease. Biol Blood Marrow Transplant (2016) 22:1608–14. doi:10.1016/j.bbmt.2016.05.017
190. Salhany KE, Macon WR, Choi JK, Elenitsas R, Lessin SR, Felgar RE, et al. Subcutaneous panniculitis-like T-cell lymphoma: clinicopathologic, immunophenotypic, and genotypic analysis of alpha/beta and gamma/delta subtypes. Am J Surg Pathol (1998) 22:881–93. doi:10.1097/00000478-199807000-00010
191. Massone C, Chott A, Metze D, Kerl K, Citarella L, Vale E, et al. Subcutaneous, blastic natural killer (NK), NK/T-cell, and other cytotoxic lymphomas of the skin: a morphologic, immunophenotypic, and molecular study of 50 patients. Am J Surg Pathol (2004) 28:719–35. doi:10.1097/01.pas.0000126719.71954.4f
192. LeBlanc RE, Tavallaee M, Kim YH, Kim J. Useful parameters for distinguishing subcutaneous panniculitis-like T-cell lymphoma from lupus erythematosus panniculitis. Am J Surg Pathol (2016) 40:745–54. doi:10.1097/PAS.0000000000000596
193. Willemze R. Cutaneous lymphomas with a panniculitic presentation. Semin Diagn Pathol (2017) 34:36–43. doi:10.1053/j.semdp.2016.11.009
194. Willemze R, Jansen PM, Cerroni L, Berti E, Santucci M, Assaf C, et al. Subcutaneous panniculitis-like T-cell lymphoma: definition, classification, and prognostic factors: an EORTC Cutaneous Lymphoma Group Study of 83 cases. Blood (2008) 111:838–45. doi:10.1182/blood-2007-04-087288
195. Massone C, Kodama K, Salmhofer W, Abe R, Shimizu H, Parodi A, et al. Lupus erythematosus panniculitis (lupus profundus): clinical, histopathological, and molecular analysis of nine cases. J Cutan Pathol (2005) 32:396–404. doi:10.1111/j.0303-6987.2005.00351.x
196. Liau JY, Chuang SS, Chu CY, Ku WH, Tsai JH, Shih TF. The presence of clusters of plasmacytoid dendritic cells is a helpful feature for differentiating lupus panniculitis from subcutaneous panniculitis-like T-cell lymphoma. Histopathology (2013) 62:1057–66. doi:10.1111/his.12105
197. Honda Y, Otsuka A, Nonomura Y, Kaku Y, Dainichi T, Miyachi Y, et al. CCR5 and CXCR3 expression in a case of subcutaneous panniculitis-like T-cell lymphoma. J Eur Acad Dermatol Venereol (2016) 30:1413–5. doi:10.1111/jdv.13258
198. Kitayama N, Otsuka A, Honda Y, Matsumura Y, Honda T, Kabashima K. CCR4 and CCR5 expression in a case of subcutaneous panniculitis-like T-cell lymphoma. Eur J Dermatol (2017) 27:414–5. doi:10.1684/ejd.2017.3016
199. Guenova E, Schanz S, Hoetzenecker W, DeSimone JA, Mehra T, Voykov B, et al. Systemic corticosteroids for subcutaneous panniculitis-like T-cell lymphoma. Br J Dermatol (2014) 171:891–4. doi:10.1111/bjd.13053
200. Rojnuckarin P, Nakorn TN, Assanasen T, Wannakrairot P, Intragumtornchai T. Cyclosporin in subcutaneous panniculitis-like T-cell lymphoma. Leuk Lymphoma (2007) 48:560–3. doi:10.1080/10428190601078456
201. Mizutani S, Kuroda J, Shimura Y, Kobayashi T, Tsutsumi Y, Yamashita M, et al. Cyclosporine A for chemotherapy-resistant subcutaneous panniculitis-like T cell lymphoma with hemophagocytic syndrome. Acta Haematol (2011) 126:8–12. doi:10.1159/000323565
202. Mehta N, Wayne AS, Kim YH, Hale GA, Alvarado CS, Myskowski P, et al. Bexarotene is active against subcutaneous panniculitis-like T-cell lymphoma in adult and pediatric populations. Clin Lymphoma Myeloma Leuk (2012) 12:20–5. doi:10.1016/j.clml.2011.06.016
203. Toro JR, Liewehr DJ, Pabby N, Sorbara L, Raffeld M, Steinberg SM, et al. Gamma-delta T-cell phenotype is associated with significantly decreased survival in cutaneous T-cell lymphoma. Blood (2003) 101:3407–12. doi:10.1182/blood-2002-05-1597
204. Guitart J, Weisenburger DD, Subtil A, Kim E, Wood G, Duvic M, et al. Cutaneous gammadelta T-cell lymphomas: a spectrum of presentations with overlap with other cytotoxic lymphomas. Am J Surg Pathol (2012) 36:1656–65. doi:10.1097/PAS.0b013e31826a5038
205. Przybylski GK, Wu H, Macon WR, Finan J, Leonard DG, Felgar RE, et al. Hepatosplenic and subcutaneous panniculitis-like gamma/delta T cell lymphomas are derived from different Vdelta subsets of gamma/delta T lymphocytes. J Mol Diagn (2000) 2:11–9. doi:10.1016/S1525-1578(10)60610-1
206. Tripodo C, Iannitto E, Florena AM, Pucillo CE, Piccaluga PP, Franco V, et al. Gamma-delta T-cell lymphomas. Nat Rev Clin Oncol (2009) 6:707–17. doi:10.1038/nrclinonc.2009.169
207. Arnulf B, Copie-Bergman C, Delfau-Larue MH, Lavergne-Slove A, Bosq J, Wechsler J, et al. Nonhepatosplenic gammadelta T-cell lymphoma: a subset of cytotoxic lymphomas with mucosal or skin localization. Blood (1998) 91:1723–31.
208. Toro JR, Beaty M, Sorbara L, Turner ML, White J, Kingma DW, et al. Gamma delta T-cell lymphoma of the skin: a clinical, microscopic, and molecular study. Arch Dermatol (2000) 136:1024–32. doi:10.1001/archderm.136.8.1024
209. Koens L, Senff NJ, Vermeer MH, Ronday HK, Willemze R, Jansen PM. Cutaneous gamma/delta T-cell lymphoma during treatment with etanercept for rheumatoid arthritis. Acta Derm Venereol (2009) 89:653–4. doi:10.2340/00015555-0728
210. Alaibac M, Berti E, Pigozzi B, Chiarion V, Aversa S, Marino F, et al. High-dose chemotherapy with autologous blood stem cell transplantation for aggressive subcutaneous panniculitis-like T-cell lymphoma. J Am Acad Dermatol (2005) 52(5 Suppl 1):S121–3. doi:10.1016/j.jaad.2004.05.042
211. Gibson JF, Alpdogan O, Subtil A, Girardi M, Wilson LD, Roberts K, et al. Hematopoietic stem cell transplantation for primary cutaneous gammadelta T-cell lymphoma and refractory subcutaneous panniculitis-like T-cell lymphoma. J Am Acad Dermatol (2015) 72:1010.e–5.e. doi:10.1016/j.jaad.2015.01.003
212. Beltraminelli H, Leinweber B, Kerl H, Cerroni L. Primary cutaneous CD4+ small-/medium-sized pleomorphic T-cell lymphoma: a cutaneous nodular proliferation of pleomorphic T lymphocytes of undetermined significance? A study of 136 cases. Am J Dermatopathol (2009) 31:317–22. doi:10.1097/DAD.0b013e31819f19bb
213. Baum CL, Link BK, Neppalli VT, Swick BL, Liu V. Reappraisal of the provisional entity primary cutaneous CD4+ small/medium pleomorphic T-cell lymphoma: a series of 10 adult and pediatric patients and review of the literature. J Am Acad Dermatol (2011) 65:739–48. doi:10.1016/j.jaad.2010.07.028
214. Grogg KL, Jung S, Erickson LA, McClure RF, Dogan A. Primary cutaneous CD4-positive small/medium-sized pleomorphic T-cell lymphoma: a clonal T-cell lymphoproliferative disorder with indolent behavior. Mod Pathol (2008) 21:708–15. doi:10.1038/modpathol.2008.40
215. Rodriguez Pinilla SM, Roncador G, Rodriguez-Peralto JL, Mollejo M, Garcia JF, Montes-Moreno S, et al. Primary cutaneous CD4+ small/medium-sized pleomorphic T-cell lymphoma expresses follicular T-cell markers. Am J Surg Pathol (2009) 33:81–90. doi:10.1097/PAS.0b013e31818e52fe
216. Cetinozman F, Jansen PM, Willemze R. Expression of programmed death-1 in primary cutaneous CD4-positive small/medium-sized pleomorphic T-cell lymphoma, cutaneous pseudo-T-cell lymphoma, and other types of cutaneous T-cell lymphoma. Am J Surg Pathol (2012) 36:109–16. doi:10.1097/PAS.0b013e318230df87
217. Rijlaarsdam JU, Willemze R. Cutaneous pseudolymphomas: classification and differential diagnosis. Semin Dermatol (1994) 13:187–96.
218. Bakels V, van Oostveen JW, van der Putte SC, Meijer CJ, Willemze R. Immunophenotyping and gene rearrangement analysis provide additional criteria to differentiate between cutaneous T-cell lymphomas and pseudo-T-cell lymphomas. Am J Pathol (1997) 150:1941–9.
219. Magro CM, Momtahen S. Differential NFATc1 expression in primary cutaneous CD4+ small/medium-sized pleomorphic T-cell lymphoma and other forms of cutaneous T-cell lymphoma and pseudolymphoma. Am J Dermatopathol (2017) 39:95–103. doi:10.1097/DAD.0000000000000597
220. Garcia-Herrera A, Colomo L, Camos M, Carreras J, Balague O, Martinez A, et al. Primary cutaneous small/medium CD4+ T-cell lymphomas: a heterogeneous group of tumors with different clinicopathologic features and outcome. J Clin Oncol (2008) 26:3364–71. doi:10.1200/JCO.2008.16.1307
221. Williams VL, Torres-Cabala CA, Duvic M. Primary cutaneous small- to medium-sized CD4+ pleomorphic T-cell lymphoma: a retrospective case series and review of the provisional cutaneous lymphoma category. Am J Clin Dermatol (2011) 12:389–401. doi:10.2165/11590390-000000000-00000
222. Goldgeier MH, Nordlund JJ, Lucky AW, Sibrack LA, McCarthy MJ, McGuire J. Hydroa vacciniforme: diagnosis and therapy. Arch Dermatol (1982) 118:588–91. doi:10.1001/archderm.118.8.588
223. Oono T, Arata J, Masuda T, Ohtsuki Y. Coexistence of hydroa vacciniforme and malignant lymphoma. Arch Dermatol (1986) 122:1306–9. doi:10.1001/archderm.122.11.1306
224. Quintanilla-Martinez L, Ridaura C, Nagl F, Saez-de-Ocariz M, Duran-McKinster C, Ruiz-Maldonado R, et al. Hydroa vacciniforme-like lymphoma: a chronic EBV+ lymphoproliferative disorder with risk to develop a systemic lymphoma. Blood (2013) 122:3101–10. doi:10.1182/blood-2013-05-502203
225. Cho KH, Kim CW, Heo DS, Lee DS, Choi WW, Rim JH, et al. Epstein-Barr virus-associated peripheral T-cell lymphoma in adults with hydroa vacciniforme-like lesions. Clin Exp Dermatol (2001) 26:242–7. doi:10.1046/j.1365-2230.2001.00805.x
226. Magana M, Sangueza P, Gil-Beristain J, Sanchez-Sosa S, Salgado A, Ramon G, et al. Angiocentric cutaneous T-cell lymphoma of childhood (hydroa-like lymphoma): a distinctive type of cutaneous T-cell lymphoma. J Am Acad Dermatol (1998) 38:574–9. doi:10.1016/S0190-9622(98)70120-3
227. Iwatsuki K, Xu Z, Takata M, Iguchi M, Ohtsuka M, Akiba H, et al. The association of latent Epstein-Barr virus infection with hydroa vacciniforme. Br J Dermatol (1999) 140:715–21. doi:10.1046/j.1365-2133.1999.02777.x
228. Barrionuevo C, Anderson VM, Zevallos-Giampietri E, Zaharia M, Misad O, Bravo F, et al. Hydroa-like cutaneous T-cell lymphoma: a clinicopathologic and molecular genetic study of 16 pediatric cases from Peru. Appl Immunohistochem Mol Morphol (2002) 10:7–14. doi:10.1097/00129039-200203000-00002
229. Kawa K, Okamura T, Yagi K, Takeuchi M, Nakayama M, Inoue M. Mosquito allergy and Epstein-Barr virus-associated T/natural killer-cell lymphoproliferative disease. Blood (2001) 98:3173–4. doi:10.1182/blood.V98.10.3173
230. Iwatsuki K, Satoh M, Yamamoto T, Oono T, Morizane S, Ohtsuka M, et al. Pathogenic link between hydroa vacciniforme and Epstein-Barr virus-associated hematologic disorders. Arch Dermatol (2006) 142:587–95. doi:10.1001/archderm.142.5.587
231. Wu YH, Chen HC, Hsiao PF, Tu MI, Lin YC, Wang TY. Hydroa vacciniforme-like Epstein-Barr virus-associated monoclonal T-lymphoproliferative disorder in a child. Int J Dermatol (2007) 46:1081–6. doi:10.1111/j.1365-4632.2007.03102.x
232. Tokura Y, Tamura Y, Takigawa M, Koide M, Satoh T, Sakamoto T, et al. Severe hypersensitivity to mosquito bites associated with natural killer cell lymphocytosis. Arch Dermatol (1990) 126:362–8. doi:10.1001/archderm.126.3.362
233. Kimura H, Ito Y, Kawabe S, Gotoh K, Takahashi Y, Kojima S, et al. EBV-associated T/NK-cell lymphoproliferative diseases in nonimmunocompromised hosts: prospective analysis of 108 cases. Blood (2012) 119:673–86. doi:10.1182/blood-2011-10-381921
234. Quintanilla-Martinez L, Ko YH, Kimura H, Jaffe ES. EBV-positive T-cell and NK-cell lymphoproliferative diseases of childhood. In: Swerdlow SH, Campo E, Harris NL, Jaffe ES, Pileri SA, Stein H, et al. editors. WHO Classification of Tumours of Haematopoietic and Lymphoid Tissues. Lyon: International Agency for Research on Cancer (2017). p. 355–63.
235. Kempf W, Kazakov DV, Cozzio A, Kamarashev J, Kerl K, Plaza T, et al. Primary cutaneous CD8(+) small- to medium-sized lymphoproliferative disorder in extrafacial sites: clinicopathologic features and concept on their classification. Am J Dermatopathol (2013) 35:159–66. doi:10.1097/DAD.0b013e31825c3a33
236. Greenblatt D, Ally M, Child F, Scarisbrick J, Whittaker S, Morris S, et al. Indolent CD8(+) lymphoid proliferation of acral sites: a clinicopathologic study of six patients with some atypical features. J Cutan Pathol (2013) 40:248–58. doi:10.1111/cup.12045
237. Hathuc VM, Hristov AC, Smith LB. Primary cutaneous acral CD8(+) T-cell lymphoma. Arch Pathol Lab Med (2017) 141:1469–75. doi:10.5858/arpa.2017-0230-RA
238. Kluk J, Kai A, Koch D, Taibjee SM, O’Connor S, Persic M, et al. Indolent CD8-positive lymphoid proliferation of acral sites: three further cases of a rare entity and an update on a unique patient. J Cutan Pathol (2016) 43:125–36. doi:10.1111/cup.12633
239. Berti E, Tomasini D, Vermeer MH, Meijer CJ, Alessi E, Willemze R. Primary cutaneous CD8-positive epidermotropic cytotoxic T cell lymphomas. A distinct clinicopathological entity with an aggressive clinical behavior. Am J Pathol (1999) 155:483–92. doi:10.1016/S0002-9440(10)65144-9
240. Nofal A, Abdel-Mawla MY, Assaf M, Salah E. Primary cutaneous aggressive epidermotropic CD8+ T-cell lymphoma: proposed diagnostic criteria and therapeutic evaluation. J Am Acad Dermatol (2012) 67:748–59. doi:10.1016/j.jaad.2011.07.043
241. Robson A, Assaf C, Bagot M, Burg G, Calonje E, Castillo C, et al. Aggressive epidermotropic cutaneous CD8+ lymphoma: a cutaneous lymphoma with distinct clinical and pathological features. Report of an EORTC cutaneous lymphoma task force workshop. Histopathology (2015) 67:425–41. doi:10.1111/his.12371
242. Guitart J, Martinez-Escala ME, Subtil A, Duvic M, Pulitzer MP, Olsen EA, et al. Primary cutaneous aggressive epidermotropic cytotoxic T-cell lymphomas: reappraisal of a provisional entity in the 2016 WHO classification of cutaneous lymphomas. Mod Pathol (2017) 30:761–72. doi:10.1038/modpathol.2016.240
Keywords: cutaneous T-cell lymphoma, mycosis fungoides, Sézary syndrome, primary cutaneous CD30+ T-cell lymphoproliferative disorders, adult T-cell leukemia/lymphoma
Citation: Fujii K (2018) New Therapies and Immunological Findings in Cutaneous T-Cell Lymphoma. Front. Oncol. 8:198. doi: 10.3389/fonc.2018.00198
Received: 13 March 2018; Accepted: 17 May 2018;
Published: 04 June 2018
Edited by:
Atsushi Otsuka, Kyoto University, JapanReviewed by:
Yu Sawada, University of Occupational and Environmental Health Japan, JapanDaniel Olive, Aix Marseille Université, France
Copyright: © 2018 Fujii. This is an open-access article distributed under the terms of the Creative Commons Attribution License (CC BY). The use, distribution or reproduction in other forums is permitted, provided the original author(s) and the copyright owner are credited and that the original publication in this journal is cited, in accordance with accepted academic practice. No use, distribution or reproduction is permitted which does not comply with these terms.
*Correspondence: Kazuyasu Fujii, kazfujii@m2.kufm.kagoshima-u.ac.jp