- 1Department of Pediatric Oncology, Dana-Farber Cancer Institute, Boston, MA, United States
- 2Division of Pediatric Hematology/Oncology, Boston Children’s Hospital, Boston, MA, United States
- 3Harvard Medical School, Boston, MA, United States
Dramatic advances in the molecular analysis of diffuse intrinsic pontine glioma have occurred over the last decade and resulted in the identification of potential therapeutic targets. In spite of these advances, no significant improvement in the outcome has been achieved and median survival remains approximately 10 months. An understanding of the approaches that have been taken to date, why they failed, and how that information can lead the field forward is critical if we are to change the status quo. In this review, we will discuss the clinical trial landscape in North America with an overview of historical approaches that failed and what might account for this failure. We will then provide a discussion of how our understanding of the genotype of this disease has led to the development of a number of trials targeting the mutations and epigenome of diffuse intrinsic pontine gliomas and the issues related to these trials. Similarly, the introduction of methodologies to address penetration across the blood–brain barrier will be considered in the context of both targeted approaches, epigenetic modification, and immune surveillance of these tumors. The comprehensive analysis of these data, generated through cooperative groups, collaborative clinical trials, and pilot studies in North America will be the focus of the IVth Memorial Alicia Pueyo international symposium in Barcelona on March 12th, 2018 and will be compared and contrasted with a similar comprehensive analysis of the European data with the goal of bringing all of these data together to develop a uniform platform on which new rational trials can be based.
Introduction
Diffuse-intrinsic pontine gliomas (DIPG) are the most common brainstem tumors in children and remain the deadliest cancer diagnosis in this population. It occurs in all age groups but is most commonly seen in children between the ages of 5–10 years with an equal distribution between the sexes (M:F = 1:1). DIPG remains one of the most challenging of all pediatric cancers and its outcomes remain abysmal. Median progression-free survival continues to range from 5 to 9 months with overall survival at 6–16 months despite hundreds of clinical trials.
Clinical Presentation and Diagnosis
Historically, DIPG has been a clinical-radiographic diagnosis as biopsy and thus histopathologic confirmation was deemed unsafe and did not influence either treatment or outcome. Patients with DIPG often have a short latency (<3 months) between symptom onset and diagnosis. At time of diagnosis, acute symptoms include cranial neuropathies, long tract signs, and ataxia with a minority (<10%) of patients presenting with symptoms of raised intracranial pressure. Classic radiographic features on MRI include a T1 hypointense T2 hyperintense mass occupying more than 50% of the pons, causing expansion of the pons, and often encircling the basilar artery. It appears to obey the pontomedullary boundary. Post gadolidium enhancement can be variable from rim enhancement to patchy enhancement to complete absence of enhancement. When present, contrast enhancement is a poor prognostic indicator (1). The presence of these imaging characteristics coupled with acute symptoms has long been sufficient for the diagnosis and treatment of children with DIPG. However, with a growing utilization of biopsies at diagnosis, a new WHO classification applicable to some DIPG tumors has emerged—diffuse midline glioma, H3K27M mutant (discussion to follow).
Standard Treatment of DIPG
The backbone of treatment for children with newly diagnosed DIPG continues to be focal, wide field radiation therapy to the pons. Most centers utilize 3D conformational photon-based radiotherapy to 54–59.4 Gy given in 30–33 fractions of 1.8 Gy daily. Radiation therapy may allow for relief of neurologic symptoms in most patients and a reduction or cessation of systemic steroids for many. However, radiation therapy overall is seen as a means of palliation, taking the overall survival from weeks to months. Increasing the dose of RT using hyper-fractionated protocols (66–78 Gy) does not appear to provide a survival benefit when compared to standard dose and fractionation protocols (2–7). Some international groups have sought to demonstrate the feasibility of hypo-fractionated radiation therapy in an effort to improve palliation (8–10), and thus the ideal fractionation strategy continues to cause some debate.
Radiation Sensitizer Clinical Trails
The only improvement to date in the outcome of DIPG has been the addition of radiation therapy. A rational approach meant to build on this impact has been to add agents that can sensitize or synergize the effects of radiation therapy. The majority of these have focused on improving areas of hypoxia so that the concentration of oxygen radicals needed for the radiation effect can be achieved. While multiple approaches have been tried and some drugs entered pediatric clinical trials (11), none have improved the median or overall survival and most have been associated with a significant increase in toxicity (12).
Chemotherapy Clinical Trials
Various chemotherapeutic strategies have been used to treat patients with DIPG including neo-adjuvant chemotherapy, concurrent chemotherapy with RT, adjuvant chemotherapy, and high dose myeloablative chemotherapy with stem cell rescue (13–16). Unfortunately, none have demonstrated improved survival when compared to radiation therapy alone. In particular, the standard of care for adult glioblastoma, which includes radiation therapy with concurrent and adjuvant temozolomide has not been shown to benefit newly diagnosed DIPG patients (17–19). While small series have indicated some effect of antiangiogenic therapy with bevacizumab (20), by and large this therapy has failed to markedly improve survival in both the newly diagnosed and relapse setting (21, 22). While a multitude of experimental agents and chemotherapy regimens have been utilized in DIPG, many of these trials specifically accrued patients with recurrent disease. Given the often short latency from disease recurrence to death, testing agents in this setting may have decreased the likelihood of finding active agents.
Biologic Advances
The lack of treatment progress despite decades of attempts highlighted a lack of understanding of the biologic underpinnings of this devastating disease. In years past, those pretreatment specimens that were available were often atypical cases that had required biopsy and, therefore, may not appropriately reflect typical DIPG biology. Across many centers, a concerted effort was made to obtain postmortem specimens from DIPG patients, which yielded many new insights. However, postmortem specimens are inherently limited as there may be significantly different molecular characteristics between the primary untreated tumor and the posttreatment postmortem tumor due to the selective pressure of radiation and chemotherapy. Given the limitations of postmortem samples, renewed interest in obtaining pretreatment specimens of DIPG bloomed. Large centers were able to demonstrate that surgical biopsy of DIPG at the time of diagnosis was safe and feasible and yielded sufficient sample for meaningful analysis with low morbidity (23–26).
The advent of diagnostic biopsies in DIPG has provided adequate tumor tissue for genome and epigenome sequencing. This revealed recurrent somatic mutations of H3F3A and HIST1H3B resulting in lysine 27 to methionine (K27M) substitution in the encoded histone H3.3 or H3.1. These mutations have been shown to be gain-of-function mutations that alter the polycomb repressive complex 2 leading to aberrant gene expression and thus drives cell transformation (27). Mutations in these histones, the proteins which package DNA into chromatin, have been found in approximately 80% of DIPG (28, 29). This consistent finding led to a revision in the WHO classification of CNS tumors—diffuse midline glioma, H3K27M-mutant (30)—for tumors once referred to as DIPG. This change has important implications as new clinical trials increasingly focus on the 80% of DIPG with histone mutations (and often include thalamic H3K27M mutated tumors, the biology of which may not be identical to classical DIPG). Similarly, the 20% of classic DIPG that lack histone mutations are still DIPG. When reviewing clinical trial data between studies, it will be important to recognize the different population in molecularly classified from radiographically classified DIPG. Equally important is the growing recognition of the heterogeneity in median survival between the different genomic variants of DIPG (31, 32) something that will have to be taken into account as new treatments are compared to historical controls.
Surgical Therapeutic Approaches
An important component of DIPG resistance to traditional chemotherapy is thought related to the blood–brain barrier, which is more impermeable at the pons. Thus, strategies to bypass the blood–brain barrier have been developed (33). One such method is convection-enhanced delivery (CED) whereby catheters are placed stereotactically into the tumor and drugs administered through these small catheters directly into the tumor. The feasibility of this technique was first demonstrated in the 1990s on small animals (34), followed by animal studies to demonstrate the feasibility of this delivery method into the brainstem (35). More recently, brainstem CED has been used safely on a limited clinical basis both outside and within the context of clinical trials (36–39). CED approaches now include both multi-catheter devices, which allow for the coverage of different areas within the tumor, as well as single catheter approaches that can be re-implanted for repeated infusion.
In addition to CED, others have proposed that intra-arterial chemotherapy administration may be advantageous when compared to systemic chemotherapy for intracranial neoplasms (40). There has been significant effort to disrupt the blood–brain barrier in conjunction with intra-arterial chemotherapy administration (41, 42). These techniques have been used in combination in two cases of brainstem lesions via basilar artery administration (43, 44). While there have been multiple attempts to use blood–brain barrier agents in combination with traditional chemotherapy, these have not generated improved outcomes although significant worsening of toxicity was often observed (45).
Current Strategies
Building upon previous decades’ advances in understanding of DIPG as an entity distinct from adult GBM, new clinical trials seek to further understand and exploit recently discovered molecular underpinnings of this challenging diagnosis. Table 1 provides a current list of North American trials for newly diagnosed DIPG (upper part of table), newly diagnosed DIPG after radiation therapy (middle part of table), and recurrent/progressive DIPG (bottom part of table). Clinical trials from Europe will be detailed in a different manuscript in this edition.
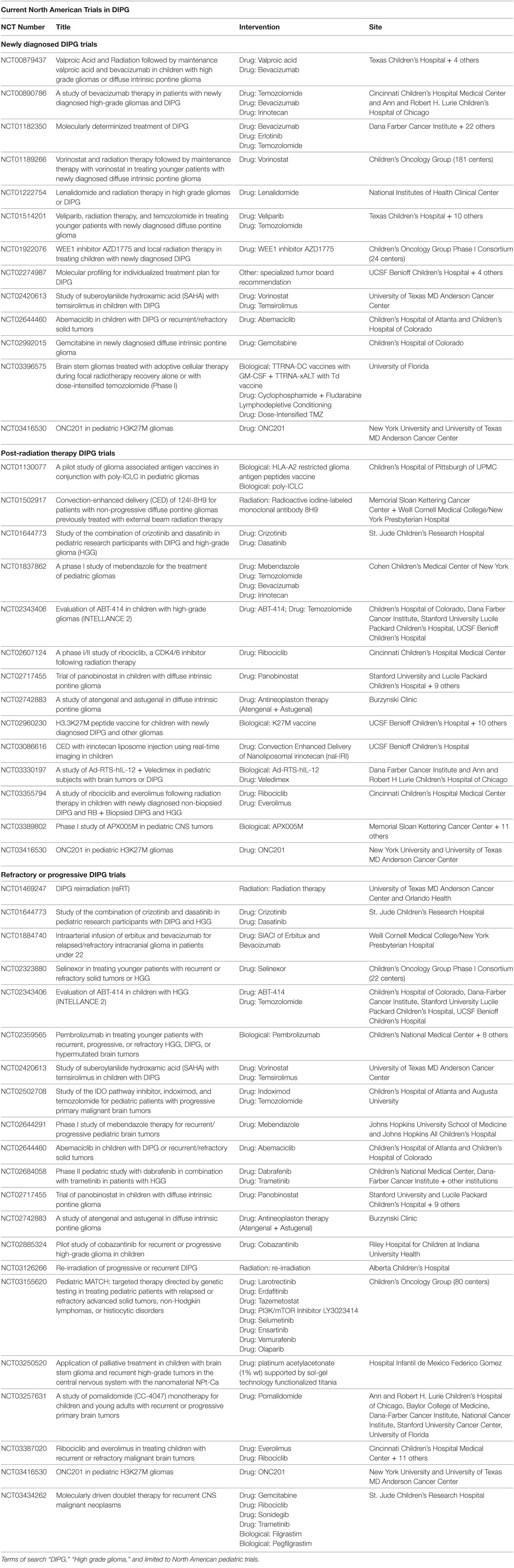
Table 1. Active North American clinical trials for children with diffuse intrinsic pontine gliomas (DIPG) as found on www.Clinicaltrials.gov as of March 1, 2018.
The common DIPG mutation in Histone 3.3 or 3.1 alters the distribution of the repressive trimethylation at position 27, which leads to transcriptional de-repression. Preclinically, researchers utilized histone deactelyase inhibitors to overcome this epigenetic mutation with good effect. This has led to the initiation of a number of phase I clinical trials with agents such as valproic acid, entinostat, and panobinostat (LHB589) for the treatment of children with recurrent or progressive DIPG. While the preclinical data for histone modifications has been very exciting (46–48), the concentrations achieved in humans for these inhibitors before excessive toxicity have limited their clinical activity (47).
The rapid improvements in our understanding of immune regulation have led to a number of new approaches in the treatment of DIPG under the label of immunotherapy. These include vaccines, checkpoint inhibitors, and cellular therapies (NK, T cells, macrophages). Our understanding of immune regulation in the brain may be different from that outside the central nervous system. Thus, approaches that have demonstrated dramatic responses in certain leukemias, lymphoma, and melanoma (49–52) remain to be proven in DIPG and other CNS tumors (53).
Given the demonstrated safety of biopsy in newly diagnosed DIPG as detailed above (23–26), there has been a push for use of biopsy and the molecular information gained from these procedures to improve up front therapy in these devastating tumors. The first North American clinical trial (NCT01172350) tested the feasibility of biopsy followed by molecular stratification based on MGMT promoter methylation, EGFR overexpression, and subsequent treatment stratification (25). Given the success of this pilot study, multiple upfront biopsy protocols are now underway and at least one active clinical trial utilizes a precision medicine approach whereby a specialized treatment recommendation is made based upon RNA expression analysis, whole exome sequencing, and predictive modeling following biopsy in newly diagnosed DIPG (NCT02264987).
Additional trials presently seek to expand the role of convection enhanced delivery in DIPG. One trial involves the direct infusion of the traditional chemotherapy agent, irinotecan (NCT03086616). Another study involves direct delivery via CED of the 124I-8H9 radioactive antibody into the tumor (NCT01502917). Both of these trials employ these interventions after standard radiation therapy but prior to progression.
Still other current clinical trials seek to explore the feasibility and role of intra-arterial treatment in DIPG. One group is evaluating the safety of intra-arterial melphalan in progressive DIPG (NCT01688401). Another group is investigating the safety of intra-arterial erbitux, an EGFR inhibitor, and bevacizumab in relapsed/refractory intracranial glioma including DIPG (NCT01884740).
Thus, as biologic understanding of DIPG and technology advance, a new wave of clinical trials has emerged (Table 1).
Conclusion
For decades, DIPG has stubbornly remained a disease with abysmal outcomes. However, safe biopsy has lead to improved biologic understanding of these challenging tumors as distinct from adult high-grade gliomas. With this understanding, our treatment paradigms have evolved. No longer are we treating DIPG with the same interventions as adult high-grade gliomas and wondering why these approaches are not effective. Instead, we seek to exploit the biologic characteristics of DIPG and employ strategies, which circumvent the unique challenges of the blood–brain barrier in this location. While exciting, we have not yet seen this new molecular understanding translate into more effective therapy. In the future, using these strategies in combination as well as a move toward precision medicine targeting individual mutational profiles of each tumor may finally alter the outcomes in DIPG.
Author Contributions
JC and MK were involved in the composition and editing of the manuscript.
Conflict of Interest Statement
The authors declare that the research was conducted in the absence of any commercial or financial relationships that could be construed as a potential conflict of interest.
Funding
This article is funded by Ellie Kavalieros DIPG Fund.
References
1. Poussaint TY, Kocak M, Vajapeyam S, Packer RI, Robertson RL, Geyer R, et al. MRI as a central component of clinical trials analysis in brainstem glioma: a report from the pediatric brain tumor consortium (PBTC). Neuro Oncol (2011) 13(4):417–27. doi:10.1093/neuonc/noq200
2. Edwards MS, Wara WM, Urtasun RC, Prados M, Levin VA, Fulton D, et al. Hyperfractionated radiation therapy for brain-stem glioma: a phase I-II trial. J Neurosurg (1989) 70(5):691–700. doi:10.3171/jns.1989.70.5.0691
3. Freeman CR, Krischer J, Sanford RA, Burger PC, Cohen M, Norris D. Hyperfractionated radiotherapy in brain stem tumors: results of a Pediatric Oncology Group study. Int J Radiat Oncol Biol Phys (1988) 15(2):311–8. doi:10.1016/S0360-3016(98)90010-4
4. Freeman CR, Krischer J, Sanford RA, Cohen ME, Burger PC, Kun L, et al. Hyperfractionated radiation therapy in brain stem tumors. Results of treatment at the 7020 cGy dose level of Pediatric Oncology Group study #8495. Cancer (1991) 68(3):474–81. doi:10.1002/1097-0142(19910801)68:3<474::AID-CNCR2820680305>3.0.CO;2-7
5. Packer RJ, Boyett JM, Zimmerman RA, Albright AL, Kaplan AM, Rorke LB, et al. Outcome of children with brain stem gliomas after treatment with 7800 cGy of hyperfractionated radiotherapy. A Childrens Cancer Group Phase I/II Trial. Cancer (1994) 74(6):1827–34. doi:10.1002/1097-0142(19940915)74:6<1827::AID-CNCR2820740628>3.0.CO;2-Q
6. Packer RJ, Boyett JM, Zimmerman RA, Rorke LB, Kaplan AM, Albright AL, et al. Hyperfractionated radiation therapy (72 Gy) for children with brain stem gliomas. A Childrens Cancer Group phase I/II trial. Cancer (1993) 72(4):1414–21. doi:10.1002/1097-0142(19930815)72:4<1414::AID-CNCR2820720442>3.0.CO;2-C
7. Packer RJ, Littman PA, Sposto RM, D’Angio G, Priest JR, Heideman RL, et al. Results of a pilot study of hyperfractionated radiation therapy for children with brain stem gliomas. Int J Radiat Oncol Biol Phys (1987) 13(11):1647–51. doi:10.1016/0360-3016(87)90160-X
8. Janssens GO, Gidding CE, Van Lindert EJ, Oldenburger FR, Erasmus CE, Schouten-Meeteren AY, et al. The role of hypofractionation radiotherapy for diffuse intrinsic brainstem glioma in children: a pilot study. Int J Radiat Oncol Biol Phys (2009) 73(3):722–6. doi:10.1016/j.ijrobp.2008.05.030
9. Janssens GO, Jansen MH, Lauwers SJ, Nowak PJ, Oldenburger FR, Bouffet E, et al. Hypofractionation vs conventional radiation therapy for newly diagnosed diffuse intrinsic pontine glioma: a matched-cohort analysis. Int J Radiat Oncol Biol Phys (2013) 85(2):315–20. doi:10.1016/j.ijrobp.2012.04.006
10. Negretti L, Bouchireb K, Levy-Piedbois C, Habrand JL, Dhermain F, Kalifa C, et al. Hypofractionated radiotherapy in the treatment of diffuse intrinsic pontine glioma in children: a single institution’s experience. J Neurooncol (2011) 104(3):773–7. doi:10.1007/s11060-011-0542-4
11. Marcus KJ, Dutton SC, Barnes P, Coleman CN, Pomeroy SL, Goumnerova L, et al. A phase I trial of etanidazole and hyperfractionated radiotherapy in children with diffuse brainstem glioma. Int J Radiat Oncol Biol Phys (2003) 55(5):1182–5. doi:10.1016/S0360-3016(02)04391-2
12. Aguilera TA, Giaccia AJ. The end of the hypoxic EPOch. Int J Radiat Oncol Biol Phys (2015) 91(5):895–7. doi:10.1016/j.ijrobp.2015.01.041
13. Vanan MI, Eisenstat DD. DIPG in children – what can we learn from the past? Front Oncol (2015) 5:237. doi:10.3389/fonc.2015.00237
14. Bouffet E, Raquin M, Doz F, Gentet JC, Rodary C, Demeocq F, et al. Radiotherapy followed by high dose busulfan and thiotepa: a prospective assessment of high dose chemotherapy in children with diffuse pontine gliomas. Cancer (2000) 88(3):685–92. doi:10.1002/(SICI)1097-0142(20000201)88:3<685::AID-CNCR27>3.0.CO;2-K
15. Hargrave D, Bartels U, Bouffet E. Diffuse brainstem glioma in children: critical review of clinical trials. Lancet Oncol (2006) 7(3):241–8. doi:10.1016/S1470-2045(06)70615-5
16. Grimm SA, Chamberlain MC. Brainstem glioma: a review. Curr Neurol Neurosci Rep (2013) 13(5):346. doi:10.1007/s11910-013-0346-3
17. Cohen KJ, Heideman RL, Zhou T, Holmes EJ, Lavey RS, Bouffet E, et al. Temozolomide in the treatment of children with newly diagnosed diffuse intrinsic pontine gliomas: a report from the Children’s Oncology Group. Neuro Oncol (2011) 13(4):410–6. doi:10.1093/neuonc/noq205
18. Chassot A, Canale S, Varlet P, Puget S, Roujeau T, Negretti L, et al. Radiotherapy with concurrent and adjuvant temozolomide in children with newly diagnosed diffuse intrinsic pontine glioma. J Neurooncol (2012) 106(2):399–407. doi:10.1007/s11060-011-0681-7
19. Sharp JR, Bouffet E, Stempak D, Gammon J, Stephens D, Johnston DL, et al. A multi-centre Canadian pilot study of metronomic temozolomide combined with radiotherapy for newly diagnosed paediatric brainstem glioma. Eur J Cancer (2010) 46(18):3271–9. doi:10.1016/j.ejca.2010.06.115
20. Zaky W, Wellner M, Brown RJ, Blüml S, Finlay JL, Dhall G. Treatment of children with diffuse intrinsic pontine gliomas with chemoradiotherapy followed by a combination of temozolomide, irinotecan, and bevacizumab. Pediatr Hematol Oncol (2013) 30(7):623–32. doi:10.3109/08880018.2013.829895
21. Gururangan S, Chi SN, Young Poussaint T, Onar-Thomas A, Gilbertson RJ, Vajapeyam S, et al. Lack of efficacy of bevacizumab plus irinotecan in children with recurrent malignant glioma and diffuse brainstem glioma: a pediatric brain tumor consortium study. J Clin Oncol (2010) 28(18):3069–75. doi:10.1200/JCO.2009.26.8789
22. Hummel TR, Salloum R, Drissi R, Kumar S, Sobo M, Goldman S, et al. A pilot study of bevacizumab-based therapy in patients with newly diagnosed high-grade gliomas and diffuse intrinsic pontine gliomas. J Neurooncol (2016) 127(1):53–61. doi:10.1007/s11060-015-2008-6
23. Cage TA, Samagh SP, Mueller S, Nicolaides T, Haas-Kogan D, Prados M, et al. Feasibility, safety, and indications for surgical biopsy of intrinsic brainstem tumors in children. Childs Nerv Syst (2013) 29(8):1313–9. doi:10.1007/s00381-013-2101-0
24. Puget S, Beccaria K, Blauwblomme T, Roujeau T, James S, Grill J, et al. Biopsy in a series of 130 pediatric diffuse intrinsic Pontine gliomas. Childs Nerv Syst (2015) 31(10):1773–80. doi:10.1007/s00381-015-2832-1
25. Hamisch C, Kickingereder P, Fischer M, Simon T, Ruge MI. Update on the diagnostic value and safety of stereotactic biopsy for pediatric brainstem tumors: a systematic review and meta-analysis of 735 cases. J Neurosurg Pediatr (2017) 20(3):261–8. doi:10.3171/2017.2.PEDS1665
26. Gupta N, Goumnerova L, Ayyanar K, Gump W, Bendel A, Nagib M, et al. PDCT-20. Feasibility and safety of surgical biopsy for patients with DIPG: preliminary results from DIPG-BATS. Neuro Oncol (2017) 19(Suppl_6, 6):vi188. doi:10.1093/neuonc/nox168.762
27. Lewis PW, Müller MM, Koletsky MS, Cordero F, Lin S, Banaszynski LA, et al. Inhibition of PRC2 activity by a gain-of-function H3 mutation found in pediatric glioblastoma. Science (2013) 340(6134):857–61. doi:10.1126/science.1232245
28. Schwartzentruber J, Korshunov A, Liu XY, Jones DT, Pfaff E, Jacob K, et al. Driver mutations in histone H3.3 and chromatin remodelling genes in paediatric glioblastoma. Nature (2012) 482(7384):226–31. doi:10.1038/nature10833
29. Wu G, Broniscer A, McEachron TA, Lu C, Paugh BS, Becksfort J, et al. Somatic histone H3 alterations in pediatric diffuse intrinsic pontine gliomas and non-brainstem glioblastomas. Nat Genet (2012) 44(3):251–3. doi:10.1038/ng.1102
30. Louis DN, Perry A, Reifenberger G, von Deimling A, Figarella-Branger D, Cavenee WK, et al. The 2016 world health organization classification of tumors of the central nervous system: a summary. Acta Neuropathol (2016) 131(6):803–20. doi:10.1007/s00401-016-1545-1
31. Khuong-Quang DA, Buczkowicz P, Rakopoulos P, Liu XY, Fontebasso AM, Bouffet E, et al. K27M mutation in histone H3.3 defines clinically and biologically distinct subgroups of pediatric diffuse intrinsic pontine gliomas. Acta Neuropathol (2012) 124(3):439–47. doi:10.1007/s00401-012-0998-0
32. Castel D, Philippe C, Calmon R, Le Dret L, Truffaux N, Boddaert N, et al. Histone H3F3A and HIST1H3B K27M mutations define two subgroups of diffuse intrinsic pontine gliomas with different prognosis and phenotypes. Acta Neuropathol (2015) 130(6):815–27. doi:10.1007/s00401-015-1478-0
33. Goodwin CR, Xu R, Iyer R, Sankey EW, Liu A, Abu-Bonsrah N, et al. Local delivery methods of therapeutic agents in the treatment of diffuse intrinsic brainstem gliomas. Clin Neurol Neurosurg (2016) 142:120–7. doi:10.1016/j.clineuro.2016.01.007
34. Bobo RH, Laske DW, Akbasak A, Morrison PF, Dedrick RL, Oldfield EH. Convection-enhanced delivery of macromolecules in the brain. Proc Natl Acad Sci U S A (1994) 91(6):2076–80. doi:10.1073/pnas.91.6.2076
35. Sandberg DI, Edgar MA, Souweidane MM. Convection-enhanced delivery into the rat brainstem. J Neurosurg (2002) 96(5):885–91. doi:10.3171/jns.2002.96.5.0885
36. Anderson RC, Kennedy B, Yanes CL, Garvin J, Needle M, Canoll P, et al. Convection-enhanced delivery of topotecan into diffuse intrinsic brainstem tumors in children. J Neurosurg Pediatr (2013) 11(3):289–95. doi:10.3171/2012.10.PEDS12142
37. Barua NU, Lowis SP, Woolley M, O’Sullivan S, Harrison R, Gill SS. Robot-guided convection-enhanced delivery of carboplatin for advanced brainstem glioma. Acta Neurochir (Wien) (2013) 155(8):1459–65. doi:10.1007/s00701-013-1700-6
38. Chittiboina P, Heiss JD, Warren KE, Lonser RR. Magnetic resonance imaging properties of convective delivery in diffuse intrinsic pontine gliomas. J Neurosurg Pediatr (2014) 13(3):276–82. doi:10.3171/2013.11.PEDS136
39. Lonser RR, Warren KE, Butman JA, Quezado Z, Robison RA, Walbridge S, et al. Real-time image-guided direct convective perfusion of intrinsic brainstem lesions. Technical note. J Neurosurg (2007) 107(1):190–7. doi:10.3171/JNS-07/07/0190
40. Gobin YP, Cloughesy TF, Chow KL, Duckwiler GR, Sayre JW, Milanese K, et al. Intraarterial chemotherapy for brain tumors by using a spatial dose fractionation algorithm and pulsatile delivery. Radiology (2001) 218(3):724–32. doi:10.1148/radiology.218.3.r01mr41724
41. Neuwelt EA, Barnett PA, McCormick CI, Frenkel EP, Minna JD. Osmotic blood-brain barrier modification: monoclonal antibody, albumin, and methotrexate delivery to cerebrospinal fluid and brain. Neurosurgery (1985) 17(3):419–23. doi:10.1227/00006123-198509000-00004
42. Neuwelt EA, Gilmer-Knight K, Lacy C, Nicholson HS, Kraemer DF, Doolittle ND, et al. Toxicity profile of delayed high dose sodium thiosulfate in children treated with carboplatin in conjunction with blood-brain-barrier disruption. Pediatr Blood Cancer (2006) 47(2):174–82. doi:10.1002/pbc.20529
43. Rajappa P, Krass J, Riina HA, Boockvar JA, Greenfield JP. Super-selective basilar artery infusion of bevacizumab and cetuximab for multiply recurrent pediatric ependymoma. Interv Neuroradiol (2011) 17(4):459–65. doi:10.1177/159101991101700410
44. Riina HA, Knopman J, Greenfield JP, Fralin S, Gobin YP, Tsiouris AJ, et al. Balloon-assisted superselective intra-arterial cerebral infusion of bevacizumab for malignant brainstem glioma. A technical note. Interv Neuroradiol (2010) 16(1):71–6. doi:10.1177/159101991001600109
45. Warren KE. Novel therapeutic delivery approaches in development for pediatric gliomas. CNS Oncol (2013) 2(5):427–35. doi:10.2217/cns.13.37
46. Killick-Cole CL, Singleton WGB, Bienemann AS, Asby DJ, Wyatt MJ, Boulter LJ, et al. Repurposing the anti-epileptic drug sodium valproate as an adjuvant treatment for diffuse intrinsic pontine glioma. PLoS One (2017) 12(5):e0176855. doi:10.1371/journal.pone.0176855
47. Hennika T, Hu G, Olaciregui NG, Barton KL, Ehteda A, Chitranjan A, et al. Pre-Clinical study of panobinostat in xenograft and genetically engineered murine diffuse intrinsic pontine glioma models. PLoS One (2017) 12(1):e0169485. doi:10.1371/journal.pone.0169485
48. Grasso CS, Tang Y, Truffaux N, Berlow NE, Liu L, Debily MA, et al. Functionally defined therapeutic targets in diffuse intrinsic pontine glioma. Nat Med (2015) 21(6):555–9. doi:10.1038/nm.3855
49. Lakin N, Rulach R, Nowicki S, Kurian KM. Current advances in checkpoint inhibitors: lessons from non-central nervous system cancers and potential for glioblastoma. Front Oncol (2017) 7:141. doi:10.3389/fonc.2017.00141
50. Hao C, Tian J, Liu H, Li F, Niu H, Zhu B. Efficacy and safety of anti-PD-1 and anti-PD-1 combined with anti-CTLA-4 immunotherapy to advanced melanoma: a systematic review and meta-analysis of randomized controlled trials. Medicine (Baltimore) (2017) 96(26):e7325. doi:10.1097/MD.0000000000007325
51. Geyer MB, Brentjens RJ. Review: current clinical applications of chimeric antigen receptor (CAR) modified T cells. Cytotherapy (2016) 18(11):1393–409. doi:10.1016/j.jcyt.2016.07.003
52. Hu Z, Ott PA, Wu CJ. Towards personalized, tumour-specific, therapeutic vaccines for cancer. Nat Rev Immunol (2017) 18(3):168–82. doi:10.1038/nri.2017.131
Keywords: diffuse intrinsic pontine gliomas, brainstem glioma, targeted therapy, convection-enhanced delivery, immunotherapy, clinical trials
Citation: Clymer J and Kieran MW (2018) The Integration of Biology Into the Treatment of Diffuse Intrinsic Pontine Glioma: A Review of the North American Clinical Trial Perspective. Front. Oncol. 8:169. doi: 10.3389/fonc.2018.00169
Received: 01 March 2018; Accepted: 01 May 2018;
Published: 18 May 2018
Edited by:
Rimas J. Orentas, Seattle Children’s Research Institute, United StatesReviewed by:
Nicholas Vitanza, Seattle Children’s Hospital, United StatesOren Becher, Northwestern University, United States
Copyright: © 2018 Clymer and Kieran. This is an open-access article distributed under the terms of the Creative Commons Attribution License (CC BY). The use, distribution or reproduction in other forums is permitted, provided the original author(s) and the copyright owner are credited and that the original publication in this journal is cited, in accordance with accepted academic practice. No use, distribution or reproduction is permitted which does not comply with these terms.
*Correspondence: Jessica Clymer, jessicar_clymer@dfci.harvard.edu