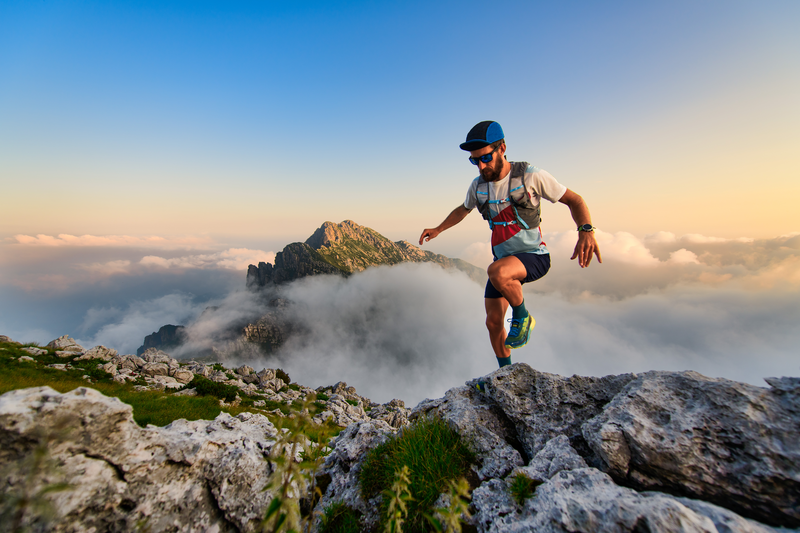
95% of researchers rate our articles as excellent or good
Learn more about the work of our research integrity team to safeguard the quality of each article we publish.
Find out more
MINI REVIEW article
Front. Mol. Neurosci. , 21 December 2018
Sec. Brain Disease Mechanisms
Volume 11 - 2018 | https://doi.org/10.3389/fnmol.2018.00465
This article is part of the Research Topic The Protein Alpha-Synuclein: Its Normal Role (in Neurons) and its Role in Disease View all 13 articles
Characterizing the normal function(s) of the protein α-Synuclein (aSyn) has the potential to illuminate links between Parkinson’s disease (PD) and diabetes and also point the way toward new therapies for these disorders. Here we provide a perspective for consideration based on our discovery that aSyn normally acts to inhibit insulin secretion from pancreatic β-cells by interacting with the Kir6.2 subunit of the ATP-sensitive potassium channel (K-ATP). It is also known that K-ATP channels act to inhibit brain dopamine secretion, and we have also shown that aSyn is a normal inhibitor of dopamine synthesis. The finding, that aSyn modulates Kir6.2 and other proteins involved in dopamine and insulin secretion, suggests that aSyn interacting proteins may be negatively impacted when aSyn aggregates inside cells, whether in brain or pancreas. Furthermore, identifying therapies for PD that can counteract dysfunction found in diabetes, would be highly beneficial. One such compound may be the multiple sclerosis drug, FTY720, which like aSyn can stimulate the activity of the catalytic subunit of protein phosphatase 2A (PP2Ac) as well as insulin secretion. In aging aSyn transgenic mice given long term oral FTY720, the mice had reduced aSyn pathology and increased levels of the protective molecule, brain derived neurotrophic factor (BDNF) (Vidal-Martinez et al., 2016). In collaboration with medicinal chemists, we made two non-immunosuppressive FTY720s that also enhance PP2Ac activity, and BDNF expression (Vargas-Medrano et al., 2014; Enoru et al., 2016; Segura-Ulate et al., 2017a). FTY720 and our novel FTY720-based-derivatives, may thus have therapeutic potential for both diabetes and PD.
We and others have shown that aSyn protein has important normal functions that are associated with its ability to interact with other molecules in a chaperone-like manner (Jenco et al., 1998; Jensen et al., 1999; Ostrerova et al., 1999; Jo et al., 2000; Murphy et al., 2000; Souza et al., 2000; Hashimoto et al., 2002; Seo et al., 2002; Kim et al., 2004; Acosta-Martinez and Levine, 2007; Martinez et al., 2007; Klegeris et al., 2008; Gorbatyuk et al., 2010b; Aoki and Li, 2011; Jin et al., 2011; Oaks and Sidhu, 2011; Bartels et al., 2014; Lautenschläger et al., 2018). Over the years, our laboratory has identified several aSyn-interacting proteins and organelles. These include: tyrosine hydroxylase, also called tyrosine 3-monooxygenase (EC 1.14.16.2) (TH), the rate limiting dopamine biosynthetic enzyme that localizes on vesicles and mitochondria with aSyn (Perez et al., 2002; Jin et al., 2007; Alerte et al., 2008); the next enzyme in the dopamine biosynthetic pathway, aromatic amino acid decarboxylase, AADC, also called dopa decarboxylase (Tehranian et al., 2006); the catalytic subunit of protein phosphatase 2A (PP2Ac) (Peng et al., 2005; Lou et al., 2010); and the 14-3-3ζ adapter protein, which also localize to mitochondria to help regulate dopamine synthesis at that organelle (Wang et al., 2009).
With normal aSyn function(s) in mind and knowing that aSyn normally interacts with and regulates many other molecules, we long ago hypothesized that a loss of aSyn function could be especially detrimental to dopaminergic neurons in a manner to contribute to nigral PD pathology (Perez and Hastings, 2004; Porras and Perez, 2014). We have tested this hypothesis in multiple models over the years. These include using aSyn lentivirus in mice, brains from familial PD and Dementia with Lewy Bodies (DLB) subjects, cell free assays, and aSyn transgenic mice where we confirmed that TH and PP2A activities become dysregulated when aSyn aggregates (Alerte et al., 2008; Wu et al., 2012; Farrell et al., 2014). This demonstrates an important normal role for soluble aSyn in the regulation of key aSyn-interacting molecules. Others have also shown that sustaining normal aSyn levels contributes significantly to neuronal viability, further solidifying a major role for soluble aSyn in optimal brain health (Gorbatyuk et al., 2010a; Kanaan and Manfredsson, 2012; Benskey et al., 2016; Collier et al., 2016).
A lesser known function of aSyn is our discovery that the protein is highly expressed in pancreatic beta cells where it interacts with Kir6.2 on insulin secretory granules, acting to downregulate insulin secretion (Geng et al., 2011). In data from co-immunoprecipitation experiments we show that aSyn and Kir6.2 interact with each other in the pancreas and in islet cell cultures, as can be appreciated in Figure 1. The methods used for these experiments are detailed in our figure legend. In this same paper, striking immunohistochemical images generated by Drs. Geng and Drain confirm near perfect overlapping localization of aSyn not only with Kir6.2, but also with Sur1, Insulin, and C peptide in beta cells (Geng et al., 2011).
Figure 1. Co-immunoprecipitation (Co-IP) of Kir6.2 with aSyn from pancreas (A) and from pancreatic islet cell cultures (B,C). (A) Anti-aSyn antibody (BD Biosciences, Cat # BD610787) was used to immunoprecipitate aSyn protein from mouse pancreatic tissue extracts. Immunocomplexes are characterized on immunoblots using anti-Kir6.2 (Santa Cruz Biotechnology, Cat # sc-11228) and anti-aSyn (Santa Cruz Biotechnology, Cat # sc-7011-R) antibodies. Equivalent aliquots of the initial input of each extract (Input) were analyzed. Homogenates in which secondary antibody only was used (SO-IP) served as a negative control. Both aSyn and Kir6.2 were co-immunoprecipitated with the anti-aSyn antibody (Syn Co-IP). (B) Binding interactions between Kir6.2 and aSyn are also seen in a representative Co-IP experiment using mouse islet cells grown in culture. Immunoblots were reacted with anti-aSyn antibody (Santa Cruz Biotechnology, Cat # sc-7011-R; in B and C, bottom panel) or anti-Kir6.2 antibody (Santa Cruz Biotechnology, Cat # sc-20809, H55; in B and C, top panel). Both Kir6.2 and aSyn are present on immunoblots in initial homogenates (Input), and were enriched after anti-aSyn antibody Co-IP (Syn-1, BD 610787; Syn IP). Specificity was confirmed using pre-adsorbed Syn-1 antibody (Pre), which efficiently reduced levels of protein that were co-immunoprecipitated. (C) Binding interactions between Kir6.2 and aSyn in a representative experiment using mouse islet cells also show the presence of Kir6.2 and aSyn in the initial homogenate (Input) as well as in the Co-IP performed using the anti-Kir6.2 antibody (Santa Cruz Biotechnology, Cat # sc-20809, H55; Kir6.2 IP), with specificity demonstrated in a Co-IP using pre-immune serum + beads (Pre). Molecular weights, determined from pre-stained standards, are shown on the left. Data from Geng et al. (2011) reprinted with permission obtained from the Copyright Clearance Center.
The aSyn/Kir6.2 interaction becomes more intriguing because in brain, neuronal Kir6.2 is found in axons and dendrites (Patel et al., 2011; Trimmer, 2015) where it plays an active role in the downregulation of dopamine secretion (Avshalumov and Rice, 2003; Bao et al., 2005; Shi et al., 2008; Patel et al., 2011; Trimmer, 2015). It remains unknown if Kir6.2 and aSyn interact and colocalize on neurotransmitter secretory vesicles in a manner to downregulate dopamine secretion similar to its effects on Kir6.2 in insulin secretory granules. Still, this possibility and other cumulative findings lead us to propose that a loss of aSyn/Kir6.2 interactions that may occur when aSyn aggregates could produce over-secretion of insulin and dopamine, although this remains largely unexplored. This possibility has further implications because there are multiple emerging lines of evidence supporting links between type 2 diabetes mellitus (T2DM) and PD comorbidity (Hu et al., 2007; Driver et al., 2008; Cereda et al., 2011, 2013; Palacios et al., 2011; Schernhammer et al., 2011; Kotagal et al., 2012; Aviles-Olmos et al., 2013; Santiago and Potashkin, 2013; Marcelo et al., 2014; Zhang and Tian, 2014; Santiago et al., 2017; Foltynie et al., 2018), as has been recently been confirmed (De Pablo-Fernandez et al., 2018).
In this regard, protein misfolding and insulin resistance are common to both T2DM and PD (Athauda and Foltynie, 2016). In diabetes, this protein misfolding implicates the islet amyloid polypeptide protein (IAPP, also known as amylin), which is a short peptide that is packaged and secreted along with insulin from pancreatic beta cells (Moore and Cooper, 1991). IAPP/amylin plays a role in glycemic regulation and is known to adopt abnormal conformations that can permeabilize synthetic vesicles in a pore-like manner akin to findings for aSyn protein (Anguiano et al., 2002). This has led some to propose that IAPP/amylin oligomers may act in a prion-like manner in the pancreatic islet cells of diabetics to spur disease onset and/or progression, as some data tend to support (Mukherjee et al., 2015, 2017). In addition, cross-seeding of aSyn and IAPP/amylin has been shown to accelerate the aggregation of both of these aggregation prone proteins (Horvath and Wittung-Stafshede, 2016), raising the possibility that aSyn may accumulate among the amyloids in pancreatic beta cells. This was recently confirmed in pancreatic tissues from subjects with synucleinopathies (Martinez-Valbuena et al., 2018). In addition, there is evidence that in nigral dopamine neurons of individuals with idiopathic/sporadic PD, there is a dysregulation of miR-126, a microRNA involved in the regulation of insulin/IGF-1/phosphatidylinositol-3-kinase (PI3K)/AKT and extracellular signal-regulated kinase (ERK) signaling (Kim et al., 2014; Briggs et al., 2015). Further, it is well-appreciated that insulin signaling contributes significantly to normal brain function and becomes dysregulated in neurodegeneration (Bomfim et al., 2012; Bamji-Mirza et al., 2014; Gao et al., 2015). Together these findings provide strong support for an association between T2DM and PD in which aSyn may play a pivotal role.
It is well-established that aSyn misfolding contributes to PD as well as to other synucleinopathies, such as DLB and multiple system atrophy (MSA) (Galvin et al., 2001; Goedert, 2001; Trojanowski and Lee, 2003). It has further been shown that aSyn oligomerization, to form preformed fibrils (PFF), can induce a prion-like spread of aSyn and cell death in PD models (Volpicelli-Daley et al., 2011, 2014; Dryanovski et al., 2013; Polinski et al., 2018). Also, aSyn PFF uptake in vitro and in vivo is modulated specifically by the LAG3 receptor, which has been shown to contribute to pathological aSyn transmission (Mao et al., 2016). Moreover, LAG3 has also been implicated in autoimmune diabetes (Bettini et al., 2011; Zhang et al., 2017), providing further evidence for potential overlap between diabetes and PD.
It is also becoming accepted that aSyn plays a role in inducing innate and adaptive immunity in PD (Allen Reish and Standaert, 2015), arising, at least in part, by aSyn activating microglial cells, which stimulates neuroimmunity (Sanchez-Guajardo et al., 2013). A role for aSyn in metabolism has also been reported in the Thy1 promoter parkinsonian A53T mice, where aSyn pathology was found to drive metabolic abnormalities in that PD model (Rothman et al., 2014). Inflammation and activated innate immunity have been shown to play a role in the pathogenesis of T2DM (Pickup, 2004) and inflammation is known to be common in diabetes and other metabolic disorders (Hotamisligil et al., 1993; Zhong et al., 2017). Based on these findings, it thus would be prudent to evaluate parkinsonian mouse models for potential overlapping pathology related to PD and T2DM.
First described by James Parkinson in the early 1800s, it is remarkable to find that in his initial description of the disorder that was later named after him, he was among the first to suggest that the “shaking palsy” may be caused by “compression of the brain, or dependent on partial exhaustion of the energy of that organ” (Parkinson, 2002). This suggests that Parkinson himself had anticipated a potential role for metabolic dysregulation in brain as contributing to the disease pathology. Yet, even 200 years later the scientific community continues to search to identify the cause for PD and for successful therapies that will counteract PD pathology.
In our search to identify protective therapies for PD, we began studying FTY720 (fingolimod, Gilenya), a Food and Drug Administration approved therapy for the demyelinating brain disorder, multiple sclerosis (Brinkmann et al., 2010). We first evaluated FTY720 based on its ability to stimulate PP2A activity (Oaks et al., 2013; Vargas-Medrano et al., 2014). This is because our research had revealed that aSyn is a normal stimulator of PP2A catalytic subunit activity (Peng et al., 2005), and that PP2A activity is significantly diminished in vivo if aSyn becomes insoluble and accumulates in Lewy bodies (Wu et al., 2012; Farrell et al., 2014). Later, others showed that FTY720 stimulates the expression of the protective molecule BDNF in vitro and in vivo (Deogracias et al., 2012). Thus, we began testing FTY720 in aging parkinsonian aSyn A53T transgenic mice and found that the mice not only tolerate long term FTY720 treatment, but also have behavioral improvement, increased BDNF expression, and reduced Lewy body-like aSyn pathology when compared to transgenic littermates treated with a vehicle control solution (Vidal-Martinez et al., 2016). In control experiments Vidal-Martinez et al. (2016) also show that blocking BDNF signaling accelerates aSyn aggregation that is reversed by co-delivering FTY720 with the TrkB blocker, ANA-12. Moreover, in addition to being able to improve both glial and neuronal cell functions (Balatoni et al., 2007; Miron et al., 2008; Kim et al., 2011; Gao et al., 2012; Vargas-Medrano et al., 2014; Cipriani et al., 2015; Segura-Ulate et al., 2017b), FTY720 has been shown to have potent anti-diabetic activity including an ability to stimulate insulin secretion (Fu et al., 2001; Yang et al., 2003; Kendall and Hupfeld, 2008; Zhao et al., 2012; Moon et al., 2013). Remarkably, insulin itself can stimulate dopamine release (Stouffer et al., 2015; Sulzer et al., 2016), confirming related effects on insulin and dopamine in brain and pancreas that are highly relevant to PD and T2DM. In addition, there is compelling evidence that dopamine itself is produced within beta cells of the human pancreas, where it becomes packaged along with insulin and acts to negatively regulate insulin secretion (Simpson et al., 2012). Future studies will be required to determine if aSyn binding to Kir6.2 occurs in brain to modulate dopamine similarly to its effects on insulin release. Additional studies to assess potential benefits of our novel FTY720-derivative compounds in pancreatic beta cells and neurons are also required. Cumulatively, the findings concerning the comorbidity of diabetes with PD, and the overlapping interactions between aSyn and key regulatory molecules in brain and pancreas open the door to further explore potential novel therapies that may benefit both disorders that affect a large percentage of our rapidly aging population, worldwide.
All authors contributed to literature searches, reading, writing, and/or editing of this manuscript. The review was conceived of by RP who also obtained permission to reprint data via Copyright Clearance Center for Figure 1. BY and JV-M provided intellectual content at all stages. After review, recommendations led us to seek the expertise of GV-M to further improve writing and content.
The authors are grateful for support from Lizanell and Colbert Coldwell Foundation, Hoy Family Research, Perez Family Research, and the El Paso Community Foundation.
RP has filed a patent for FTY720 derivative compounds. Compositions and Methods for the Treatment of Parkinson’s Disease. Publication# 2015/0290145.
The remaining authors declare that the research was conducted in the absence of any commercial or financial relationships that could be construed as a potential conflict of interest.
Acosta-Martinez, M., and Levine, J. E. (2007). Regulation of KATP channel subunit gene expression by hyperglycemia in the mediobasal hypothalamus of female rats. Am. J. Physiol. Endocrinol. Metab. 292, E1801–E1807. doi: 10.1152/ajpendo.00700.2006
Alerte, T. N., Akinfolarin, A. A., Friedrich, E. E., Mader, S. A., Hong, C. S., and Perez, R. G. (2008). Alpha-synuclein aggregation alters tyrosine hydroxylase phosphorylation and immunoreactivity: lessons from viral transduction of knockout mice. Neurosci. Lett. 435, 24–29. doi: 10.1016/j.neulet.2008.02.014
Allen Reish, H. E., and Standaert, D. G. (2015). Role of alpha-synuclein in inducing innate and adaptive immunity in Parkinson disease. J. Parkinsons Dis. 5, 1–19. doi: 10.3233/JPD-140491
Anguiano, M., Nowak, R. J., and Lansbury, P. T. (2002). Protofibrillar islet amyloid polypeptide permeabilizes synthetic vesicles by a pore-like mechanism that may be relevant to type II diabetes. Biochemistry 41, 11338–11343. doi: 10.1021/bi020314u
Aoki, R., and Li, Y. R. (2011). Alpha-synuclein promotes neuroprotection through NF-kappaB-mediated transcriptional regulation of protein kinase Cdelta. Sci. Signal. 4:jc6. doi: 10.1126/scisignal.2002425
Athauda, D., and Foltynie, T. (2016). Insulin resistance and Parkinson’s disease: a new target for disease modification? Prog. Neurobiol. 145–146, 98–120. doi: 10.1016/j.pneurobio.2016.10.001
Aviles-Olmos, I., Limousin, P., Lees, A., and Foltynie, T. (2013). Parkinson’s disease, insulin resistance and novel agents of neuroprotection. Brain 136, 374–384. doi: 10.1093/brain/aws009
Avshalumov, M. V., and Rice, M. E. (2003). Activation of ATP-sensitive K+ (K(ATP)) channels by H2O2 underlies glutamate-dependent inhibition of striatal dopamine release. Proc. Natl. Acad. Sci. U.S.A. 100, 11729–11734. doi: 10.1073/pnas.1834314100
Balatoni, B., Storch, M. K., Swoboda, E. M., Schonborn, V., Koziel, A., Lambrou, G. N., et al. (2007). FTY720 sustains and restores neuronal function in the DA rat model of MOG-induced experimental autoimmune encephalomyelitis. Brain Res. Bull. 74, 307–316. doi: 10.1016/j.brainresbull.2007.06.023
Bamji-Mirza, M., Callaghan, D., Najem, D., Shen, S., Hasim, M. S., Yang, Z., et al. (2014). Stimulation of insulin signaling and inhibition of JNK-AP1 activation protect cells from amyloid-beta-induced signaling dysregulation and inflammatory response. J. Alzheimers Dis. 40, 105–122. doi: 10.3233/JAD-131949
Bao, L., Avshalumov, M. V., and Rice, M. E. (2005). Partial mitochondrial inhibition causes striatal dopamine release suppression and medium spiny neuron depolarization via H2O2 elevation, not ATP depletion. J. Neurosci. 25, 10029–10040. doi: 10.1523/JNEUROSCI.2652-05.2005
Bartels, T., Kim, N. C., Luth, E. S., and Selkoe, D. J. (2014). N-alpha-acetylation of alpha-synuclein increases its helical folding propensity, GM1 binding specificity and resistance to aggregation. PLoS One 9:e103727. doi: 10.1371/journal.pone.0103727
Benskey, M. J., Perez, R. G., and Manfredsson, F. P. (2016). The contribution of alpha synuclein to neuronal survival and function - Implications for Parkinson’s disease. J. Neurochem. 137, 331–359. doi: 10.1111/jnc.13570
Bettini, M., Szymczak-Workman, A. L., Forbes, K., Castellaw, A. H., Selby, M., Pan, X., et al. (2011). Cutting edge: accelerated autoimmune diabetes in the absence of LAG-3. J. Immunol. 187, 3493–3498. doi: 10.4049/jimmunol.1100714
Bomfim, T. R., Forny-Germano, L., Sathler, L. B., Brito-Moreira, J., Houzel, J. C., Decker, H., et al. (2012). An anti-diabetes agent protects the mouse brain from defective insulin signaling caused by Alzheimer’s disease- associated Abeta oligomers. J. Clin. Invest. 122, 1339–1353. doi: 10.1172/JCI57256
Briggs, C. E., Wang, Y., Kong, B., Woo, T. -U. W., Iyer, L. K., and Sonntag, K. C. (2015). Midbrain dopamine neurons in Parkinson’s disease exhibit a dysregulated miRNA and target-gene network. Brain Res. 1618, 111–121. doi: 10.1016/j.brainres.2015.05.021
Brinkmann, V., Billich, A., Baumruker, T., Heining, P., Schmouder, R., Francis, G., et al. (2010). Fingolimod (FTY720): discovery and development of an oral drug to treat multiple sclerosis. Nat. Rev. Drug Discov. 9, 883–897. doi: 10.1038/nrd3248
Cereda, E., Barichella, M., Pedrolli, C., Klersy, C., Cassani, E., Caccialanza, R., et al. (2011). Diabetes and risk of Parkinson’s disease: a systematic review and meta-analysis. Diabetes Care 34, 2614–2623. doi: 10.2337/dc11-1584
Cereda, E., Barichella, M., Pedrolli, C., Klersy, C., Cassani, E., Caccialanza, R., et al. (2013). Diabetes and risk of Parkinson’s disease. Mov. Disord. 28, 257–261. doi: 10.1002/mds.25211
Cipriani, R., Chara, J. C., Rodriguez-Antiguedad, A., and Matute, C. (2015). FTY720 attenuates excitotoxicity and neuroinflammation. J. Neuroinflammation 12:86. doi: 10.1186/s12974-015-0308-6
Collier, T. J., Redmond, D. E. Jr., Steece-Collier, K., Lipton, J. W., and Manfredsson, F. P. (2016). Is alpha-synuclein loss-of-function a contributor to Parkinsonian pathology? evidence from non-human primates. Front. Neurosci. 10:12. doi: 10.3389/fnins.2016.00012
De Pablo-Fernandez, E., Goldacre, R., Pakpoor, J., Noyce, A. J., and Warner, T. T. (2018). Association between diabetes and subsequent Parkinson disease: a record-linkage cohort study. Neurology 91, e139–e142. doi: 10.1212/WNL.0000000000005771
Deogracias, R., Yazdani, M., Dekkers, M. P., Guy, J., Ionescu, M. C., Vogt, K. E., et al. (2012). Fingolimod, a sphingosine-1 phosphate receptor modulator, increases BDNF levels and improves symptoms of a mouse model of Rett syndrome. Proc. Natl. Acad. Sci. U.S.A. 109, 14230–14235. doi: 10.1073/pnas.1206093109
Driver, J. A., Smith, A., Buring, J. E., Gaziano, J. M., Kurth, T., and Logroscino, G. (2008). Prospective cohort study of type 2 diabetes and the risk of Parkinson’s disease. Diabetes Care 31, 2003–2005. doi: 10.2337/dc08-0688
Dryanovski, D. I., Guzman, J. N., Xie, Z., Galteri, D. J., Volpicelli-Daley, L. A., Lee, V. M., et al. (2013). Calcium entry and alpha-synuclein inclusions elevate dendritic mitochondrial oxidant stress in dopaminergic neurons. J. Neurosci. 33, 10154–10164. doi: 10.1523/JNEUROSCI.5311-12.2013
Enoru, J. O., Yang, B., Krishnamachari, S., Villanueva, E., DeMaio, W., Watanyar, A., et al. (2016). Preclinical metabolism, pharmacokinetics and in vivo analysis of new blood-brain-barrier penetrant fingolimod analogues: FTY720-C2 and FTY720-mitoxy. PLoS One 11:e0162162. doi: 10.1371/journal.pone.0162162
Farrell, K. F., Krishnamachari, S., Villanueva, E., Lou, H., Alerte, T. N., Peet, E., et al. (2014). Non-motor Parkinsonian pathology in aging A53T alpha-synuclein mice is associated with progressive synucleinopathy and altered enzymatic function. J. Neurochem. 128, 536–546. doi: 10.1111/jnc.12481
Foltynie, T., Politis, M., Tolosa, E., and Stefanis, L. (2018). The Relationship Between Diabetes and Parkinson’s Disease, Online Blog MDS The International Parkinson and Movement Disorder Society (MDS). Available at: https://www.movementdisorders.org/MDS/Scientific-Issues-Committee-Blog/The-Relationship-Between-Diabetes-and-Parkinsons-Disease.htm
Fu, F., Hu, S., Li, S., DeLeo, J., Hoover, J., Wang, S., et al. (2001). FTY720, a novel immunosuppressive agent with insulinotropic activity, prolongs graft survival in a mouse islet transplantation model. Transplant. Proc. 33, 672–673. doi: 10.1016/S0041-1345(00)02195-3
Galvin, J. E., Lee, V. M., and Trojanowski, J. Q. (2001). Synucleinopathies: clinical and pathological implications. Arch. Neurol. 58, 186–190. doi: 10.1001/archneur.58.2.186
Gao, F., Liu, Y., Li, X., Wang, Y., Wei, D., and Jiang, W. (2012). Fingolimod (FTY720) inhibits neuroinflammation and attenuates spontaneous convulsions in lithium-pilocarpine induced status epilepticus in rat model. Pharmacol. Biochem. Behav. 103, 187–196. doi: 10.1016/j.pbb.2012.08.025
Gao, S., Duan, C., Gao, G., Wang, X., and Yang, H. (2015). Alpha-synuclein overexpression negatively regulates insulin receptor substrate 1 by activating mTORC1/S6K1 signaling. Int. J. Biochem. Cell Biol. 64, 25–33. doi: 10.1016/j.biocel.2015.03.006
Geng, X., Lou, H., Wang, J., Li, L., Swanson, A. L., Sun, M., et al. (2011). alpha-Synuclein binds the K(ATP) channel at insulin-secretory granules and inhibits insulin secretion. Am. J. Physiol. Endocrinol. Metab. 300, E276–E286. doi: 10.1152/ajpendo.00262.2010
Goedert, M. (2001). Alpha-synuclein and neurodegenerative diseases. Nat. Rev. Neurosci. 2, 492–501. doi: 10.1038/35081564
Gorbatyuk, O. S., Li, S., Nash, K., Gorbatyuk, M., Lewin, A. S., Sullivan, L. F., et al. (2010a). In vivo RNAi-mediated alpha-synuclein silencing induces nigrostriatal degeneration. Mol. Ther. 18, 1450–1457. doi: 10.1038/mt.2010.115
Gorbatyuk, O. S., Li, S., Nha Nguyen, F., Manfredsson, F. P., Kondrikova, G., Sullivan, L. F., et al. (2010b). alpha-Synuclein expression in rat Substantia nigra suppresses phospholipase D2 toxicity and nigral neurodegeneration. Mol. Ther. 18, 1758–1768. doi: 10.1038/mt.2010.137
Hashimoto, M., Hsu, L. J., Rockenstein, E., Takenouchi, T., Mallory, M., and Masliah, E. (2002). alpha-Synuclein protects against oxidative stress via inactivation of the c-Jun N-terminal kinase stress-signaling pathway in neuronal cells. J. Biol. Chem. 277, 11465–11472. doi: 10.1074/jbc.M111428200
Horvath, I., and Wittung-Stafshede, P. (2016). Cross-talk between amyloidogenic proteins in type-2 diabetes and Parkinson’s disease. Proc. Natl. Acad. Sci. U.S.A. 113, 12473–12477. doi: 10.1073/pnas.1610371113
Hotamisligil, G. S., Shargill, N. S., and Spiegelman, B. M. (1993). Adipose expression of tumor necrosis factor-alpha: direct role in obesity-linked insulin resistance. Science 259, 87–91. doi: 10.1126/science.7678183
Hu, G., Jousilahti, P., Bidel, S., Antikainen, R., and Tuomilehto, J. (2007). Type 2 diabetes and the risk of Parkinson’s disease. Diabetes Care 30, 842–847. doi: 10.2337/dc06-2011
Jenco, J. M., Rawlingson, A., Daniels, B., and Morris, A. J. (1998). Regulation of phospholipase D2: selective inhibition of mammalian phospholipase D isoenzymes by alpha- and beta-synucleins. Biochemistry 37, 4901–4909. doi: 10.1021/bi972776r
Jensen, P. H., Hager, H., Nielsen, M. S., Hojrup, P., Gliemann, J., and Jakes, R. (1999). alpha-synuclein binds to Tau and stimulates the protein kinase A-catalyzed tau phosphorylation of serine residues 262 and 356. J. Biol. Chem. 274, 25481–25489. doi: 10.1074/jbc.274.36.25481
Jin, H., Kanthasamy, A., Ghosh, A., Yang, Y., Anantharam, V., and Kanthasamy, A. G. (2011). alpha-Synuclein negatively regulates protein kinase Cdelta expression to suppress apoptosis in dopaminergic neurons by reducing p300 histone acetyltransferase activity. J. Neurosci. 31, 2035–2051. doi: 10.1523/JNEUROSCI.5634-10.2011
Jin, J., Li, G. J., Davis, J., Zhu, D., Wang, Y., Pan, C., et al. (2007). Identification of novel proteins interacting with both alpha-synuclein and DJ-1. Mol. Cell. Proteomics 6, 845–859. doi: 10.1016/j.neulet.2009.04.060
Jo, E., McLaurin, J., Yip, C. M., St George-Hyslop, P., and Fraser, P. E. (2000). alpha-Synuclein membrane interactions and lipid specificity. J. Biol. Chem. 275, 34328–34334. doi: 10.1074/jbc.M004345200
Kanaan, N. M., and Manfredsson, F. P. (2012). Loss of functional alpha-synuclein: a toxic event in Parkinson’s disease? J. Parkinsons Dis. 2, 249–267. doi: 10.3233/JPD-012138
Kendall, M. R., and Hupfeld, C. J. (2008). FTY720, a sphingosine-1-phosphate receptor modulator, reverses high-fat diet-induced weight gain, insulin resistance and adipose tissue inflammation in C57BL/6 mice. Diabetes Obes. Metab. 10, 802–805. doi: 10.1111/j.1463-1326.2008.00910.x
Kim, H. J., Miron, V. E., Dukala, D., Proia, R. L., Ludwin, S. K., Traka, M., et al. (2011). Neurobiological effects of sphingosine 1-phosphate receptor modulation in the cuprizone model. FASEB J. 25, 1509–1518. doi: 10.1096/fj.10-173203
Kim, T., Choi, E., Rhim, H., Paik, S., and Yang, C. (2004). alpha-Synuclein has structural and functional similarities to small heat shock proteins. Biochem. Biophys. Res. Commun. 324, 1352–1359. doi: 10.1016/j.bbrc.2004.09.208
Kim, W., Lee, Y., McKenna, N. D., Yi, M., Simunovic, F., Wang, Y., et al. (2014). miR-126 contributes to Parkinson’s disease by dysregulating the insulin-like growth factor/phosphoinositide 3-kinase signaling. Neurobiol. Aging 35, 1712–1721. doi: 10.1016/j.neurobiolaging.2014.01.021
Klegeris, A., Pelech, S., Giasson, B. I., Maguire, J., Zhang, H., McGeer, E. G., et al. (2008). Alpha-synuclein activates stress signaling protein kinases in THP-1 cells and microglia. Neurobiol. Aging 29, 739–752. doi: 10.1016/j.neurobiolaging.2006.11.013
Kotagal, V., Albin, R. L., Muller, M. L., and Bohnen, N. I. (2012). Clinical features of Parkinson disease when onset of diabetes came first: a case-control study. Neurology 79, 1835–1836; author rely 1836.
Lautenschläger, J., Stephens, A. D., Fusco, G., Ströhl, F., Curry, N., Zacharopoulou, M., et al. (2018). C-terminal calcium binding of α-synuclein modulates synaptic vesicle interaction. Nat. Commun. 9:712. doi: 10.1038/s41467-018-03111-4
Lou, H., Montoya, S. E., Alerte, T. N., Wang, J., Wu, J., Peng, X., et al. (2010). Serine 129 phosphorylation reduces the ability of alpha-synuclein to regulate tyrosine hydroxylase and protein phosphatase 2A in vitro and in vivo. J. Biol. Chem. 285, 17648–17661. doi: 10.1074/jbc.M110.100867
Mao, X., Ou, M. T., Karuppagounder, S. S., Kam, T. I., Yin, X., Xiong, Y., et al. (2016). Pathological alpha-synuclein transmission initiated by binding lymphocyte-activation gene 3. Science 353:aah3374. doi: 10.1126/science.aah3374
Marcelo, M. S. L., Adriano, D. S. T., Ana, C. D. N., Lais, S. R., Ana Marcia, D., Fabiola, V. D. S., et al. (2014). Does Parkinson’s disease and type-2 diabetes mellitus present common pathophysiological mechanisms and treatments? CNS Neurol. Disord. Drug Targets 13, 418–428. doi: 10.2174/18715273113126660155
Martinez, Z., Zhu, M., Han, S., and Fink, A. L. (2007). GM1 specifically interacts with alpha-synuclein and inhibits fibrillation. Biochemistry 46, 1868–1877. doi: 10.1021/bi061749a
Martinez-Valbuena, I., Amat-Villegas, I., Valenti-Azcarate, R., Carmona-Abellan, M. D. M., Marcilla, I., Tunon, M. T., and Luquin, M. R. (2018). Interaction of amyloidogenic proteins in pancreatic beta cells from subjects with synucleinopathies. Acta Neuropathol. 135, 877–886. doi: 10.1007/s00401-018-1832-0
Miron, V. E., Schubart, A., and Antel, J. P. (2008). Central nervous system-directed effects of FTY720 (fingolimod). J. Neurol. Sci. 274, 13–17. doi: 10.1016/j.jns.2008.06.031
Moon, H., Chon, J., Joo, J., Kim, D., In, J., Lee, H., et al. (2013). FTY720 preserved islet beta-cell mass by inhibiting apoptosis and increasing survival of beta-cells in db/db mice. Diabetes Met. Res. Rev. 29, 19–24. doi: 10.1002/dmrr.2341
Moore, C. X., and Cooper, G. J. (1991). Co-secretion of amylin and insulin from cultured islet beta-cells: modulation by nutrient secretagogues, islet hormones and hypoglycemic agents. Biochem. Biophys. Res. Commun. 179, 1–9. doi: 10.1016/0006-291X(91)91325-7
Mukherjee, A., Morales-Scheihing, D., Butler, P. C., and Soto, C. (2015). Type 2 diabetes as a protein misfolding disease. Trends Mol. Med. 21, 439–449. doi: 10.1016/j.molmed.2015.04.005
Mukherjee, A., Morales-Scheihing, D., Salvadores, N., Moreno-Gonzalez, I., Gonzalez, C., Taylor-Presse, K., et al. (2017). Induction of IAPP amyloid deposition and associated diabetic abnormalities by a prion-like mechanism. J. Exp. Med. 214, 2591–2610. doi: 10.1084/jem.20161134
Murphy, D. D., Rueter, S. M., Trojanowski, J. Q., and Lee, V. M. (2000). Synucleins are developmentally expressed, and alpha-synuclein regulates the size of the presynaptic vesicular pool in primary hippocampal neurons. J. Neurosci. 20, 3214–3220. doi: 10.1523/JNEUROSCI.20-09-03214.2000
Oaks, A. W., and Sidhu, A. (2011). Synuclein modulation of monoamine transporters. FEBS Lett. 585, 1001–1006. doi: 10.1016/j.febslet.2011.03.009
Oaks, J. J., Santhanam, R., Walker, C. J., Roof, S., Harb, J. G., Ferenchak, G., et al. (2013). Antagonistic activities of the immunomodulator and PP2A-activating drug FTY720 (Fingolimod, Gilenya) in Jak2-driven hematologic malignancies. Blood 122, 1923–1934. doi: 10.1182/blood-2013-03-492181
Ostrerova, N., Petrucelli, L., Farrer, M., Mehta, N., Choi, P., Hardy, J., et al. (1999). alpha-Synuclein shares physical and functional homology with 14-3-3 proteins. J. Neurosci. 19, 5782–5791. doi: 10.1523/JNEUROSCI.19-14-05782.1999
Palacios, N., Gao, X., McCullough, M. L., Jacobs, E. J., Patel, A. V., Mayo, T., et al. (2011). Obesity, diabetes, and risk of Parkinson’s disease. Mov. Disord. 26, 2253–2259. doi: 10.1002/mds.23855
Parkinson, J. (2002). An essay on the shaking palsy. 1817. J. Neuropsychiatry Clin. Neurosci. 14, 223–236; discussion 222. doi: 10.1176/jnp.14.2.223
Patel, J. C., Witkovsky, P., Coetzee, W. A., and Rice, M. E. (2011). Subsecond regulation of striatal dopamine release by pre-synaptic KATP channels. J. Neurochem. 118, 721–736. doi: 10.1111/j.1471-4159.2011.07358.x
Peng, X., Tehranian, R., Dietrich, P., Stefanis, L., and Perez, R. G. (2005). Alpha-synuclein activation of protein phosphatase 2A reduces tyrosine hydroxylase phosphorylation in dopaminergic cells. J. Cell Sci. 118, 3523–3530. doi: 10.1242/jcs.02481
Perez, R. G., and Hastings, T. G. (2004). Could a loss of alpha-synuclein function put dopaminergic neurons at risk? J. Neurochem. 89, 1318–1324. doi: 10.1111/j.1471-4159.2004.02423.x
Perez, R. G., Waymire, J. C., Lin, E., Liu, J. J., Guo, F., and Zigmond, M. J. (2002). A role for alpha-synuclein in the regulation of dopamine biosynthesis. J. Neurosci. 22, 3090–3099. doi: 10.1523/JNEUROSCI.22-08-03090.2002
Pickup, J. C. (2004). Inflammation and activated innate immunity in the pathogenesis of type 2 diabetes. Diabetes Care 27, 813–823. doi: 10.2337/diacare.27.3.813
Polinski, N. K., Volpicelli-Daley, L. A., Sortwell, C. E., Luk, K. C., Cremades, N., Gottler, L. M., et al. (2018). Best practices for generating and using alpha-synuclein pre-formed fibrils to model Parkinson’s disease in rodents. J. Parkinsons Dis. 8, 303–322. doi: 10.3233/JPD-171248
Porras, J. L., and Perez, R. G. (2014). “Potential contribution of alpha-synuclein dysregulation to Parkinson’s disease pathology,” in Nova Publishers - Alpha-Synuclein, ed. M. P. A. H. C. Kanowitz (Hauppauge, NY: NOVA Science Publishers), 51–70.
Rothman, S. M., Griffioen, K. J., Fishbein, K. W., Spencer, R. G., Makrogiannis, S., Cong, W. N., et al. (2014). Metabolic abnormalities and hypoleptinemia in alpha-synuclein A53T mutant mice. Neurobiol. Aging 35, 1153–1161. doi: 10.1016/j.neurobiolaging.2013.10.088
Sanchez-Guajardo, V., Barnum, C. J., Tansey, M. G., and Romero-Ramos, M. (2013). Neuroimmunological processes in Parkinson’s disease and their relation to α-synuclein: microglia as the referee between neuronal processes and peripheral immunity. ASN Neuro 5:e00112. doi: 10.1042/AN20120066
Santiago, J. A., Bottero, V., and Potashkin, J. A. (2017). Biological and clinical implications of comorbidities in Parkinson’s disease. Front. Aging Neurosci. 9:394. doi: 10.3389/fnagi.2017.00394
Santiago, J. A., and Potashkin, J. A. (2013). Shared dysregulated pathways lead to Parkinson’s disease and diabetes. Trends Mol. Med. 19, 176–186. doi: 10.1016/j.molmed.2013.01.002
Schernhammer, E., Hansen, J., Rugbjerg, K., Wermuth, L., and Ritz, B. (2011). Diabetes and the risk of developing Parkinson’s disease in Denmark. Diabetes Care 34, 1102–1108. doi: 10.2337/dc10-1333
Segura-Ulate, I., Belcher, T. K., Vidal-Martinez, G., Vargas-Medrano, J., and Perez, R. G. (2017a). FTY720-derivatives do not induce FTY720-like lymphopenia. J. Pharmacol. Sci. 133, 187–189. doi: 10.1016/j.jphs.2017.02.006
Segura-Ulate, I., Yang, B., Vargas-Medrano, J., and Perez, R. G. (2017b). FTY720 (Fingolimod) reverses alpha-synuclein-induced downregulation of brain-derived neurotrophic factor mRNA in OLN-93 oligodendroglial cells. Neuropharmacology 117, 149–157. doi: 10.1016/j.neuropharm.2017.01.028
Seo, J. H., Rah, J. C., Choi, S. H., Shin, J. K., Min, K., Kim, H. S., et al. (2002). Alpha-synuclein regulates neuronal survival via Bcl-2 family expression and PI3/Akt kinase pathway. FASEB J. 16, 1826–1828. doi: 10.1096/fj.02-0041fje
Shi, X. R., Chang, J., Ding, J. H., Fan, Y., Sun, X. L., and Hu, G. (2008). Kir6.2 knockout alters neurotransmitter release in mouse striatum: an in vivo microdialysis study. Neurosci. Lett. 439, 230–234. doi: 10.1016/j.neulet.2008.05.024
Simpson, N., Maffei, A., Freeby, M., Burroughs, S., Freyberg, Z., Javitch, J., et al. (2012). Dopamine-mediated autocrine inhibitory circuit regulating human insulin secretion in vitro. Mol. Endocrinol. 26, 1757–1772. doi: 10.1210/me.2012-1101
Souza, J. M., Giasson, B. I., Lee, V. M., and Ischiropoulos, H. (2000). Chaperone-like activity of synucleins. FEBS Lett. 474, 116–119. doi: 10.1016/S0014-5793(00)01563-5
Stouffer, M. A., Woods, C. A., Patel, J. C., Lee, C. R., Witkovsky, P., Bao, L., et al. (2015). Insulin enhances striatal dopamine release by activating cholinergic interneurons and thereby signals reward. Nat. Commun. 6:8543. doi: 10.1038/ncomms9543
Sulzer, D., Cragg, S. J., and Rice, M. E. (2016). Striatal dopamine neurotransmission: regulation of release and uptake. Basal Ganglia 6, 123–148. doi: 10.1016/j.baga.2016.02.001
Tehranian, R., Montoya, S. E., Van Laar, A. D., Hastings, T. G., and Perez, R. G. (2006). Alpha-synuclein inhibits aromatic amino acid decarboxylase activity in dopaminergic cells. J. Neurochem. 99, 1188–1196. doi: 10.1111/j.1471-4159.2006.04146.x
Trimmer, J. S. (2015). Subcellular localization of K(+) channels in mammalian brain neurons: remarkable precision in the midst of extraordinary complexity. Neuron 85, 238–256. doi: 10.1016/j.neuron.2014.12.042
Trojanowski, J. Q., and Lee, V. M. (2003). Parkinson’s disease and related alpha-synucleinopathies are brain amyloidoses. Ann. N. Y. Acad. Sci. 991, 107–110. doi: 10.1111/j.1749-6632.2003.tb07468.x
Vargas-Medrano, J., Krishnamachari, S., Villanueva, E., Godfrey, W. H., Lou, H., Chinnasamy, R., et al. (2014). Novel FTY720-based compounds stimulate neurotrophin expression and phosphatase activity in dopaminergic cells. ACS Med. Chem. Lett. 5, 782–786. doi: 10.1021/ml500128g
Vidal-Martinez, G., Vargas-Medrano, J., Gil-Tommee, C., Medina, D., Garza, N. T., Yang, B., et al. (2016). G., FTY720/Fingolimod reduces synucleinopathy and improves gut motility in A53T Mice: contributions of pro-brain-derived neurotrophic factor (Pro-BDNF) and mature BDNF. J. Biol. Chem. 291, 20811–20821. doi: 10.1074/jbc.M116.744029
Volpicelli-Daley, L. A., Gamble, K. L., Schultheiss, C. E., Riddle, D. M., West, A. B., and Lee, V. M. (2014). Formation of alpha-synuclein Lewy neurite-like aggregates in axons impedes the transport of distinct endosomes. Mol. Biol. Cell 25, 4010–4023. doi: 10.1091/mbc.E14-02-0741
Volpicelli-Daley, L. A., Luk, K. C., Patel, T. P., Tanik, S. A., Riddle, D. M., Stieber, A., et al. (2011). Exogenous alpha-synuclein fibrils induce Lewy body pathology leading to synaptic dysfunction and neuron death. Neuron 72, 57–71. doi: 10.1016/j.neuron.2011.08.033
Wang, J., Lou, H., Pedersen, C. J., Smith, A. D., and Perez, R. G. (2009). 14-3-3zeta contributes to tyrosine hydroxylase activity in MN9D cells: localization of dopamine regulatory proteins to mitochondria. J. Biol. Chem. 284, 14011–14019. doi: 10.1074/jbc.M901310200
Wu, J., Lou, H., Alerte, T. N., Stachowski, E. K., Chen, J., Singleton, A. B., et al. (2012). Lewy-like aggregation of alpha-synuclein reduces protein phosphatase 2A activity in vitro and in vivo. Neuroscience 207, 288–297. doi: 10.1016/j.neuroscience.2012.01.028
Yang, Z., Chen, M., Fialkow, L. B., Ellett, J. D., Wu, R., Brinkmann, V., et al. (2003). The immune modulator FYT720 prevents autoimmune diabetes in nonobese diabetic mice. Clin. Immunol. 107, 30–35. doi: 10.1016/S1521-6616(02)00054-2
Zhang, P., and Tian, B. (2014). Metabolic syndrome: an important risk factor for Parkinson’s disease. Oxid. Med. Cell. Longev. 2014:729194. doi: 10.1155/2014/729194
Zhang, Q., Chikina, M., Szymczak-Workman, A. L., Horne, W., Kolls, J. K., Vignali, K. M., et al. (2017). LAG3 limits regulatory T cell proliferation and function in autoimmune diabetes. Sci. Immunol. 2:eaah4569. doi: 10.1126/sciimmunol.aah4569
Zhao, Z., Choi, J., Zhao, C., and Ma, Z. A. (2012). FTY720 normalizes hyperglycemia by stimulating beta-cell in vivo regeneration in db/db mice through regulation of cyclin D3 and p57(KIP2). J. Biol. Chem. 287, 5562–5573. doi: 10.1074/jbc.M111.305359
Keywords: alpha-synuclein, dopamine, insulin, Kir6.2, LAG3, Parkinson’s disease, type 2 diabetes
Citation: Vidal-Martinez G, Yang B, Vargas-Medrano J and Perez RG (2018) Could α-Synuclein Modulation of Insulin and Dopamine Identify a Novel Link Between Parkinson’s Disease and Diabetes as Well as Potential Therapies?. Front. Mol. Neurosci. 11:465. doi: 10.3389/fnmol.2018.00465
Received: 19 October 2018; Accepted: 30 November 2018;
Published: 21 December 2018.
Edited by:
Yunjong Lee, Sungkyunkwan University, South KoreaReviewed by:
Ali Keshavarzian, Rush University, United StatesCopyright © 2018 Vidal-Martinez, Yang, Vargas-Medrano and Perez. This is an open-access article distributed under the terms of the Creative Commons Attribution License (CC BY). The use, distribution or reproduction in other forums is permitted, provided the original author(s) and the copyright owner(s) are credited and that the original publication in this journal is cited, in accordance with accepted academic practice. No use, distribution or reproduction is permitted which does not comply with these terms.
*Correspondence: Ruth G. Perez, cnV0aC5nLnBlcmV6QHR0dWhzYy5lZHU=; NDdyZ3BlcmV6QGdtYWlsLmNvbQ==
Disclaimer: All claims expressed in this article are solely those of the authors and do not necessarily represent those of their affiliated organizations, or those of the publisher, the editors and the reviewers. Any product that may be evaluated in this article or claim that may be made by its manufacturer is not guaranteed or endorsed by the publisher.
Research integrity at Frontiers
Learn more about the work of our research integrity team to safeguard the quality of each article we publish.