- 1Shandong Provincial Key Laboratory of Animal Cells and Developmental Biology, School of Life Sciences, Shandong University, Qingdao, China
- 2Department of Otolaryngology-Head and Neck Surgery, The Second Hospital of Shandong University, Jinan, China
- 3Shenzhen Research Institute of Shandong University, Shenzhen, China
- 4Shandong Provincial Collaborative Innovation Center of Cell Biology, Shandong Normal University, Jinan, China
Cyclin-dependent kinase 5 (CDK5) is abundantly expressed in post-mitotic cells including neurons. It is involved in multiple cellular events, such as cytoskeletal dynamics, signaling cascades, gene expression, and cell survival, et al. Dysfunction of CDK5 has been associated with a number of neurological disorders. Here we show that CDK5 is expressed in mouse cochlear hair cells, and CDK5 inactivation in hair cells causes hearing loss in mice. CDK5 inactivation has no effect on stereocilia development in the cochlear hair cells. However, it affects stereocilia maintenance, resulting in stereocilia disorganization and eventually stereocilia loss. Consistently, hair cell loss was significantly elevated by CDK5 inactivation. Despite that CDK5 has been shown to play important roles in synapse development and/or function, CDK5 inactivation does not affect the formation of ribbon synapses of cochlear hair cells. Further investigation showed that CDK5 inactivation causes reduced phosphorylation of ERM (ezrin, radixin, and moesin) proteins, which might contribute to the stereocilia deficits. Taken together, our data suggest that CDK5 plays pivotal roles in auditory hair cells, and CDK5 inactivation causes hearing loss in mice.
Introduction
In the mammalian cochlea, hair cells are arranged into one row of inner hair cells (IHCs) and three rows of outer hair cells (OHCs) that are interlaced with various types of supporting cells (Schwander et al., 2010). As the auditory receptor cells, hair cells are characterized by their hairy-looking, F-actin-based stereocilia on the apical surface. For each hair cell, dozens to hundreds of stereocilia are organized into several rows of increasing heights, forming a staircase-like pattern. The mechanoelectrical transduction (MET) channels have been suggested to localize at the tips of shorter stereocilia (Beurg et al., 2009). Stereocilia are organized in a flattened U-shaped (IHCs) or V-shaped (OHCs) pattern, while the tallest stereocilia are positioned close to the vertex. The vertices of stereocilia in all hair cells point away from the center of the cochlea, establishing the planar cell polarity (PCP) of the cochlear epithelia. A single microtubule-based kinocilium localizes at the vertex of stereocilia, but degenerates at late developmental stage in cochlear hair cells, implying that it is not necessary for MET (Lindeman et al., 1971). Instead, kinocilium was believed to play pivotal roles in stereocilia development and cochlear PCP establishment (Jones et al., 2008). Stereocilia and kinocilium together constitute the so-called hair bundle. The detailed mechanisms of hair bundle development and maintenance as well as hair cell PCP establishment still remain elusive.
Cyclin-dependent kinase 5 (CDK5) is a proline-directed serine/threonine kinase. It is abundantly expressed in post-mitotic cells including neurons and is necessary for cell differentiation (Tsai et al., 1993; Nikolic et al., 1996). It has been shown that CDK5 plays important roles in multiple cellular events, such as cytoskeletal dynamics, signaling cascades, gene expression, and cell survival, et al (Su and Tsai, 2011). Similar to other CDKs whose kinase activity needs to be activated by cyclins, CDK5 is activated by specific activators, including p35, p39, cyclin I (CCNI), and cyclin I-like (CCNI2) (Tsai et al., 1994; Tang et al., 1995; Brinkkoetter et al., 2009; Liu et al., 2017).
Dysfunction of CDK5 has been associated with a number of neurological disorders including Alzheimer’s disease, amyotrophic lateral sclerosis (ALS), and Niemann-Pick type C disease (Su and Tsai, 2011). For example, neurotoxic signals such as β-amyloid (Aβ), excitotoxicity, ischemia, and oxidative stress cause cleavage of p35 into p25 and p10 through activating calpain. The resultant p25 causes constitutive activation and mislocalization of CDK5, which eventually leads to neuronal death (Patrick et al., 1999; Lee et al., 2000). Germline inactivation of Cdk5 gene in mice abolishes cortical laminar structure and cerebellar foliation, and causes abnormal motor axons and neuromuscular synapses, which eventually leads to perinatal mortality and hampers further examination of the physiological function of CDK5 (Ohshima et al., 1996; Fu et al., 2005). To circumvent this obstacle, tissue-specific inactivation of CDK5 has been employed to study the physiological role of CDK5 in different cells such as certain neurons, hippocampus, and adipose (Hirasawa et al., 2004; Hawasli et al., 2007; Guan et al., 2011; Banks et al., 2015).
CDK5 has been shown to be expressed in chicken auditory hair cells and regulate the membrane expression and kinetics of BK channel Slo (Bai et al., 2012). Moreover, inhibition of CDK5 with roscovitine induced differentiation of supernumerary hair cells and supporting cells in the developing rat cochlear explant cultures (Malgrange et al., 2003). These results suggested that CDK5 might play an important role in auditory hair cell differentiation and/or function. In order to investigate the physiological role of CDK5 in hearing, we made use of conditional knockout mice that selectively disrupt Cdk5 gene expression in the hair cells. Our results showed that Cdk5 inactivation causes hair cell loss and leads to deafness in mice.
Materials and Methods
Mice
Cdk5lox/+ mice (Samuels et al., 2007), EIIaCre/+ (Lakso et al., 1996), and Atoh1Cre/+ (Yang et al., 2010) mice were maintained on a mixed genetic background and genotyped as described previously. EIIaCre/+;Cdk5lox/lox mice (“Cdk5 ko mice”) die perinatally, therefore Atoh1Cre/+;Cdk5lox/lox mice (“Cdk5 cko mice”) were used in the present work. Cdk5lox/lox mice (“Cdk5 wt mice”) were included as control. Whole-mount immunostaining (see below) showed that CDK5 is expressed in Cdk5lox/lox auditory hair cells, but absent in Atoh1Cre/+;Cdk5lox/lox auditory hair cells, confirming successful CDK5 inactivation in hair cells of Atoh1Cre/+;Cdk5lox/lox mice.
Whole-Mount Immunostaining
All steps were performed at room temperature unless otherwise indicated. Dissected organ of Corti explants were fixed with 4% paraformaldehyde (PFA) in PBS for 30 min, followed by permeabilization and blocking with PBT1 (0.1% Triton X-100, 1% BSA, and 5% heat-inactivated goat serum in PBS, pH 7.3) for 30 min. Samples were then incubated with rabbit anti-CDK5 antibody (Santa Cruz, Cat. No. sc-173, 1:100 diluted) or rabbit anti-MYO6 (Cell Signaling Technology, Cat. No. 9200, 1:50 diluted) in PBT1 overnight at 4°C, followed by incubation with Alexa Fluor® 488 donkey anti-rabbit secondary antibody (Invitrogen, Cat. No. A21206, 1:200 diluted) in PBT2 (0.1% Triton X-100 and 0.1% BSA in PBS) for 1 h. After that, samples were incubated with TRITC-conjugated phalloidin (Sigma-Aldrich, Cat. No. P1951) in PBS for 30 min, then mounted in PBS/glycerol (1:1) and imaged with a confocal microscope (LSM 700, Zeiss, Germany). For CtBP2 staining, samples were incubated with mouse anti-CtBP2 antibody (BD, Cat. No. 612044, 1:100 diluted) in PBT1 overnight at 4°C, followed by incubation with Alexa Fluor® 568 goat anti-mouse IgG1 (Invitrogen, Cat. No. A21124, 1:200 diluted) in PBT2 for 1 h and DAPI (Gene View Scientific Inc.) in PBS for 1 h.
Cryosection Immunostaining
Mouse cochleae were embedded in OCT compound and sectioned in 8–10 μm thickness. After fixation with 4% PFA in PBS for 30 min, samples were permeabilized and blocked with PBT1 for 30 min, then incubated with rabbit anti-CDK5 antibody (Santa Cruz, Cat. No. sc-173, 1:50 diluted) in PBT1 overnight at 4°C. Afterward, samples were incubated with Alexa Fluor® 488 donkey anti-rabbit secondary antibody (Invitrogen, Cat. No. A21206, 1:200 diluted) in PBT2 for 1 h, followed by incubation with DAPI (Gene View Scientific Inc.) in PBS for 1 h. Lastly, samples were mounted in PBS/glycerol (1:1) and imaged with a confocal microscope (LSM 700, Zeiss, Germany).
Auditory Brainstem Responses (ABR) Measurement
Mice were placed on an isothermal pad to keep the body temperature at 37°C during the whole experiment. A RZ6 workstation and BioSig software (Tucker Davis Technologies) were used for stimulus generation, presentation, ABR acquisition, and data management. After the mice were anesthetized by intraperitoneally injecting 5% chloral hydrate for 0.5 ml/100 g body weight, electrodes were inserted subcutaneously at the vertex and pinna as well as near the tail. Acoustic stimuli (clicks or pure-tone bursts) of decreasing sound level from 90 dB SPL in 10 dB SPL steps were generated using high-frequency transducers. At each sound level, 512 responses were sampled and averaged. Hearing threshold of each mouse was determined as the lowest sound level at which all ABR waves were detectable.
Distortion Product Otoacoustic Emission (DPOAE) Measurement
Mice were anesthetized and maintained as described above. Two sine-wave tones of different frequencies (F1 and F2, F2 = 1.2 × F1) were presented for 1-s durations ranging from 20 to 80 dB SPL in 10 dB SPL steps using two speakers (Tucker-Davis Technologies, MF1, upper frequency limit 88 kHz). The emitted acoustic signal was picked up by a microphone (Etymotic Research, ER10B+, upper frequency limit 32 kHz) and digitized, from which the magnitude of the distortion product (frequency = 2 × F1 – F2) was determined. The surrounding noise floor was calculated by averaging adjacent frequency bins near the distortion product frequency. DPOAE thresholds were calculated when the magnitude of the distortion product was at least 5 dB SPL higher than the noise floor.
Scanning Electron Microscopy (SEM)
Mouse inner ears were fixed with 2.5% glutaraldehyde in 0.1 M phosphate buffer overnight at 4°C. Cochleae were dissected out of the temporal bone, then post-fixed with 1% osmium tetroxide in 0.1 M phosphate buffer at 4°C for 2 h. After dehydration in ethanol, samples were critically point dried using a Leica EM CPD300 (Leica, Germany), then mounted and sputter coated with platinum (15 nm) using a Cressington 108 sputter coater (Cressington, United Kingdom). Images were taken using a Quanta250 field-emission scanning electron microscope (FEI, Netherlands).
Western Blot
Mouse tissues were homogenized in ice-cold lysis buffer consisting of 150 mM NaCl, 50 mM Tris at pH 7.5, 1% NP-40, 0.1% SDS, 1% (vol/vol) Triton X-100, 1 mM PMSF, and 1 × protease inhibitor cocktail (Sigma-Aldrich, Saint Louis, MO, United States). The supernatant was collected after centrifugation at 4°C and separated by polyacrylamide gel electrophoresis (PAGE), then transferred to PVDF membrane. After blocking in PBS containing 5% milk and 0.1% Tween-20, the membrane was incubated with primary antibody at 4°C over night, followed by incubation with HRP-conjugated secondary antibody (Bio-Rad, Cat. No. 170-6515 or 170-6516) at room temperature for an hour. The signals were detected with the ECL system (Cell Signaling Technology, Danvers, MA, United States). Primary antibodies used are as follows: anti-ERM (rabbit, Cell Signaling Technology, Cat. No. 3142), anti-pERM (rabbit, Cell Signaling Technology, Cat. No. 3726), anti-CDK5 (rabbit, Santa Cruz, Cat. No. sc-173), and anti-GAPDH (mouse, Millipore, Cat. No. MAB374).
FM 1-43FX Uptake Assay
FM1-43FX (Molecular Probes, Invitrogen), a fixable analog of FM1-43 [N-(3-triethylammoniumpropyl)-4-(4-(dibutylamino)-styryl) pyridinium dibromide] was used to label functional hair cells. Briefly, dissected mouse basilar membrane was incubated with 3 μM FM1-43FX in PBS for 30 s and rinsed three times in PBS, then fixed with 4% PFA at room temperature for 20 min. The samples were mounted in PBS-glycerol (1:1) and imaged with an epifluorescence microscope (IX53, Olympus, Japan).
Statistical Analysis
Data were shown as means ± standard error of mean. One way ANOVA with Dunnett’s test was used to determine statistical significance, and p < 0.05 was considered statistically significant.
Results
CDK5 Is Expressed in Mouse Cochlear Hair Cells
The expression of CDK5 in mouse cochlea was examined by performing whole-mount immunostaining using a specific anti-CDK5 antibody. The results showed that CDK5 immunoreactivity was present in the cell body of both IHCs and OHCs, but not in the stereocilia (Figure 1A). Cryosection immunostaining confirmed that CDK5 was present in the cell body of both IHCs and OHCs, and the immunoreactivity was more concentrated at the apical surface of hair cells (Supplementary Figure S1A).
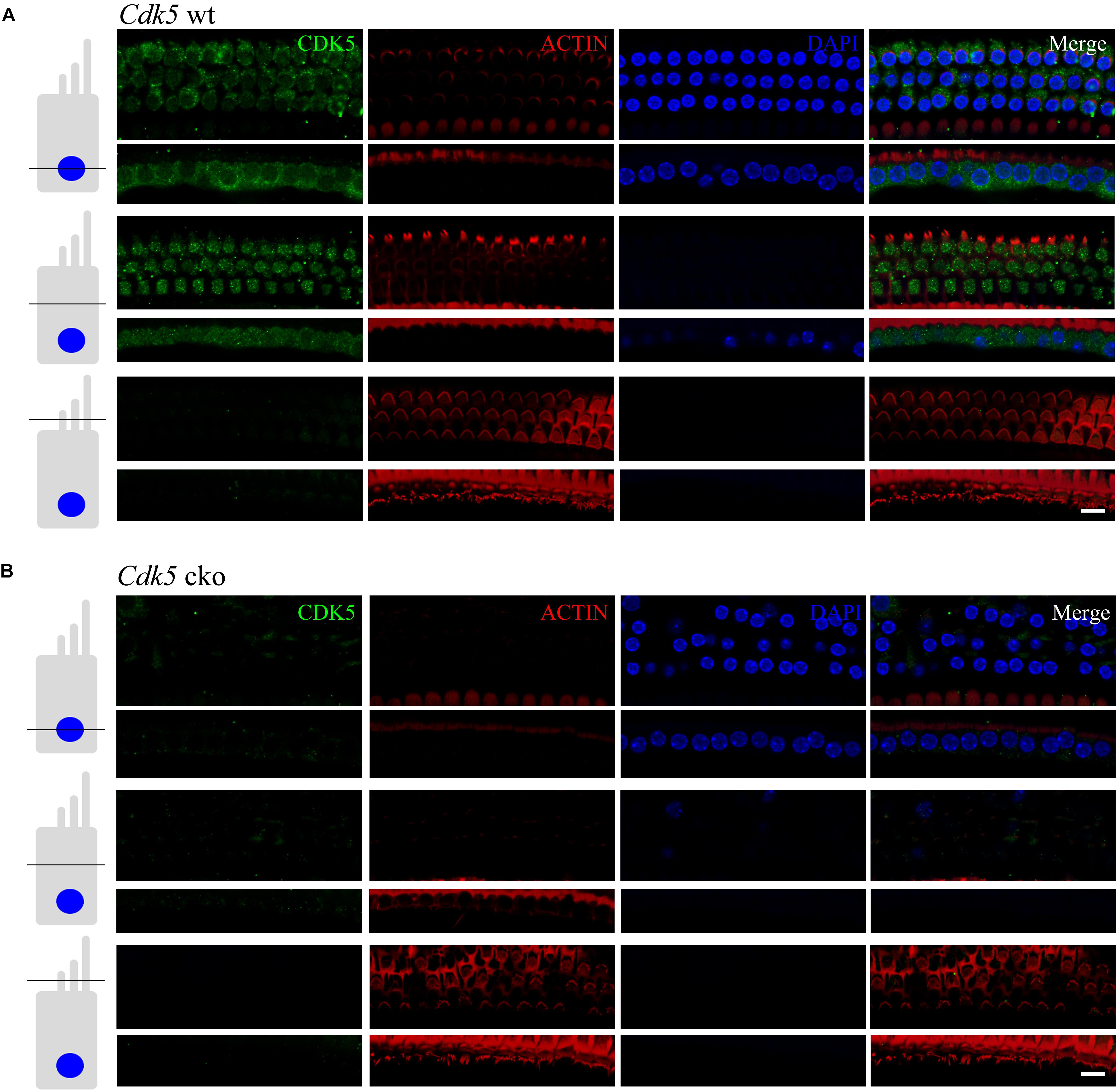
Figure 1. CDK5 is expressed in the cell body of mouse auditory hair cells. Expression of CDK5 in auditory hair cells was examined by performing whole-mount immunostaining of Cdk5lox/lox (A) and Atoh1Cre/+;Cdk5lox/lox (B) cochlea. F-actin core of stereocilia was visualized by TRITC-conjugated phalloidin. Nuclei were visualized by DAPI. Images were taken from the middle turn of 5-month-old mouse cochlea using a confocal microscope at different levels as indicated. Scale bar, 10 μm.
In order to examine the physiological role of CDK5, we crossed Cdk5lox/+ mice with EIIaCre/+ mice to generate EIIaCre/+;Cdk5lox/+ mice, which were then crossed with Cdk5lox/+ mice to obtain EIIaCre/+;Cdk5lox/lox mice. Cdk5 expression is disrupted in all tissues in EIIaCre/+;Cdk5lox/lox mice, hence we designate them as “Cdk5 ko mice” in the following text. RT-PCR and western blot confirmed that CDK5 expression is indeed abolished in various tissues of Cdk5 ko mice (data not shown). Cdk5 ko mice died perinatally as reported (Ohshima et al., 1996), preventing us from further analyzing the effect of Cdk5 inactivation on hearing. To disrupt Cdk5 expression specifically in the hair cells, we utilized Atoh1Cre/+ mice that express Cre recombinase in the developing cochlear hair cells from approximately E14.5 (Yang et al., 2010). We designate the obtained Atoh1Cre/+;Cdk5lox/lox mice as “Cdk5 cko mice” in the following text. Whole-mount and cryosection immunostaining confirmed that CDK5 is absent in the cochlear hair cells of Cdk5 cko mice (Figure 1B and Supplementary Figure S1B). In the following experiments, Cdk5lox/lox mice (“Cdk5 wt mice”) were used as control.
Cdk5 Inactivation in Hair Cells Causes Hearing Loss
The auditory function of Cdk5 cko mice was evaluated by performing auditory brainstem response (ABR) measurement, which detects the sound-evoked electrophysiological potentials in the auditory pathway from the cochlea to the brainstem. The results revealed significant differences in click-induced ABR thresholds between Cdk5 cko mice and control mice. The ABR threshold of 1-month-old Cdk5 cko mice was about 30 dB higher than that of control mice (Figures 2A,B). The ABR threshold elevation of Cdk5 cko mice increased to about 40 dB at age of 3 months, and reached around 50 dB after age of 6 months (Figure 2B). Elevated ABR thresholds were also observed in Cdk5 cko mice to pure tone stimuli of all examined frequencies in an age-dependent manner (Figures 2C,D).
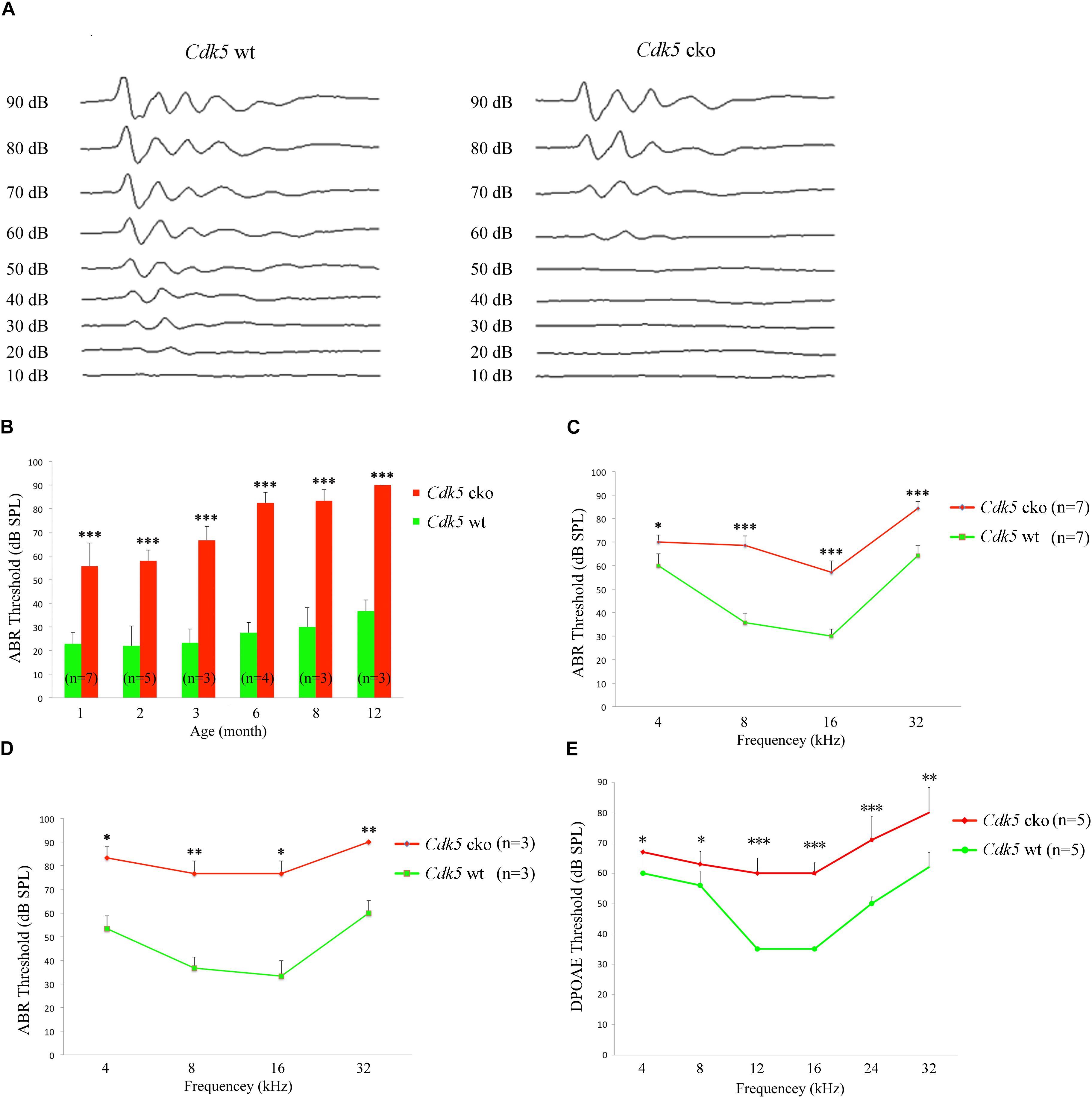
Figure 2. Hearing threshold is significantly elevated in Cdk5 cko mice. (A) Raw traces of ABR responses to click stimuli in 1-month-old Atoh1Cre/+;Cdk5lox/lox and Cdk5lox/lox mice. (B) The ABR thresholds for click stimuli in Atoh1Cre/+;Cdk5lox/lox and Cdk5lox/lox mice at different ages. (C) The ABR thresholds for pure tone stimuli in 1-month-old Atoh1Cre/+;Cdk5lox/lox and Cdk5lox/lox mice. (D) The ABR thresholds for pure tone stimuli in 8-month-old Atoh1Cre/+;Cdk5lox/lox and Cdk5lox/lox mice. (E) The DPOAE thresholds for pure tone stimuli in 1-month-old Atoh1Cre/+;Cdk5lox/lox and Cdk5lox/lox mice. The numbers of animals for each group used in the experiments are indicated. ∗p < 0.05; ∗∗p < 0.01; ∗∗∗p < 0.001.
Distortion product otoacoustic emission (DPOAE) measurements were then performed to examine the function of OHCs in Cdk5 cko mice. DPOAE detects a low-level sound that is generated by functional OHCs and emitted back to the ear canal. The results showed that the DPOAE threshold of Cdk5 cko mice was significantly elevated compared to that of control mice, suggesting that there were OHC function deficits in Cdk5 cko mice (Figure 2E). Taken together, our present data suggested that Cdk5 inactivation in hair cells compromises OHC function and causes hearing loss.
Cdk5 Inactivation in Hair Cells Does Not Affect Stereocilia Development and MET
The morphology of auditory hair cell stereocilia was examined by performing phalloidin staining of stereocilia’s F-actin core. At postnatal day 8 (P8), the morphology of cochlear hair cell stereocilia in Cdk5 cko mice was largely unaffected (Figure 3A). Stereocilia morphology was further examined by performing SEM. Consistent with the phalloidin staining result, SEM showed that the stereocilia morphology of P8 Cdk5 cko OHCs and IHCs was largely unaffected, forming regular staircase pattern and normal PCP (Figures 4A–C). Taken together, these results suggested that stereocilia development was not affected by Cdk5 inactivation in hair cells.
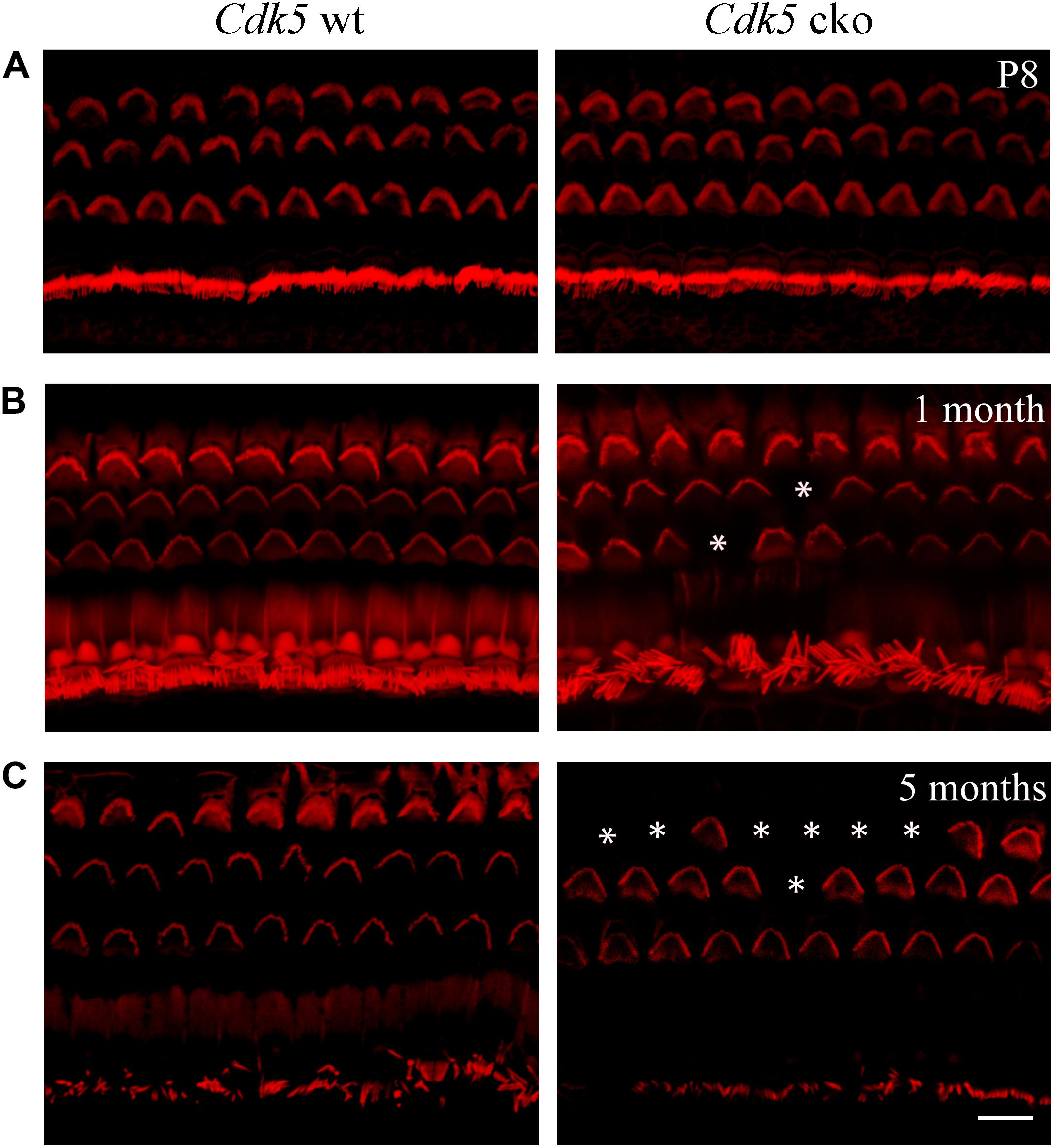
Figure 3. Stereocilia morphology examined by phalloidin staining at different developmental stages. Phalloidin staining and confocal microscopy were employed to examine the morphology of P8 (A), 1-month-old (B), and 5-month-old (C) Atoh1Cre/+; Cdk5lox/lox and Cdk5lox/lox hair cell stereocilia at the middle turn of cochleae. Loss of stereocilia was indicated by asterisks. Scale bar, 10 μm.
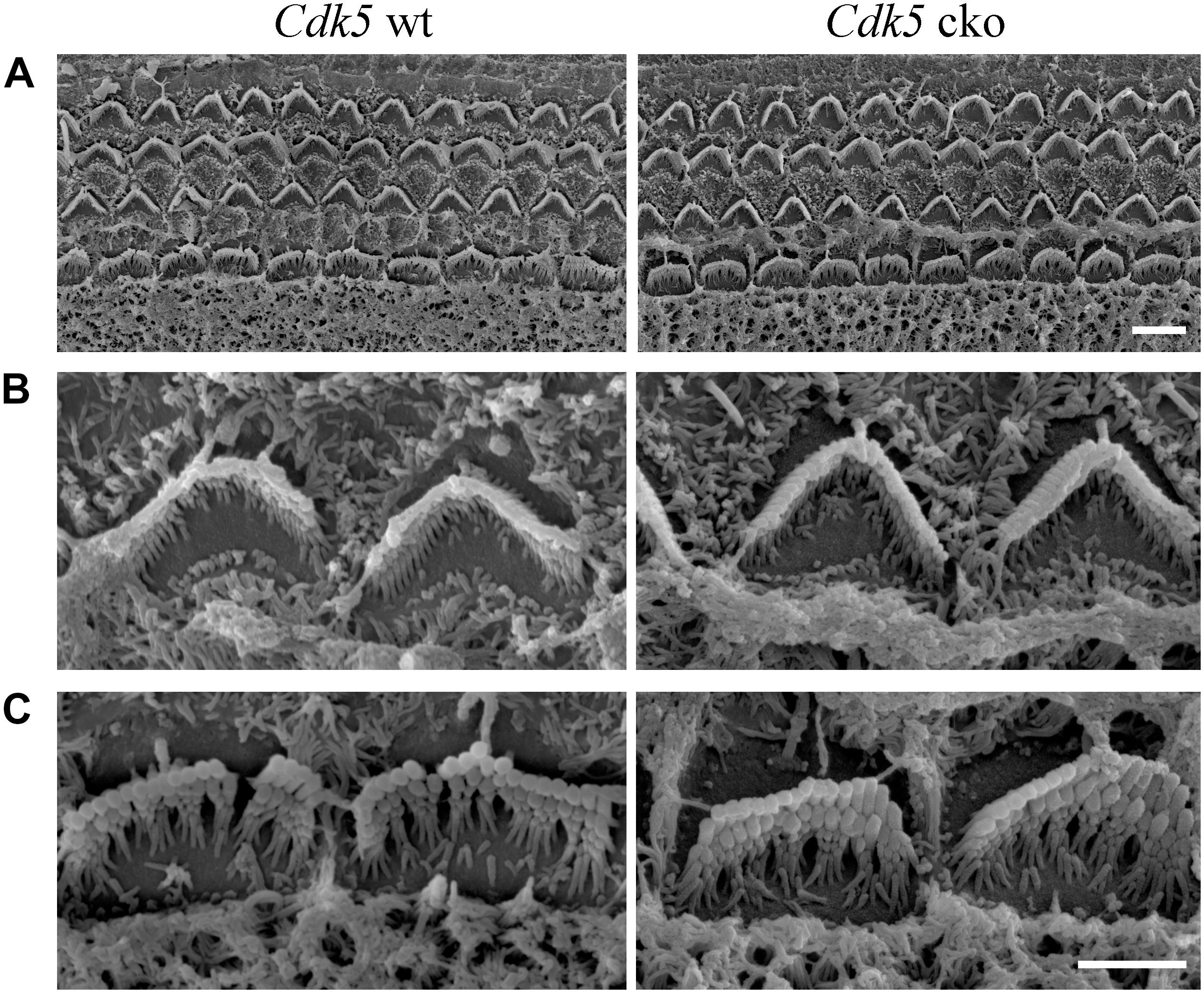
Figure 4. Hair bundle morphology of P8 Cdk5 cko mice examined by SEM. (A) Low magnification images of hair bundles at the middle turn of Cdk5lox/lox and Atoh1Cre/+; Cdk5lox/lox cochleae. Scale bar, 5 μm. (B) High magnification images of hair bundles of Cdk5lox/lox and Atoh1Cre/+; Cdk5lox/lox OHCs. (C) High magnification images of hair bundles of Cdk5lox/lox and Atoh1Cre/+; Cdk5lox/lox IHCs. Scale bar, 2.5 μm.
Normal stereocilia development in Cdk5 cko hair cells prompted us to examine whether the Cdk5 cko hair cells are functionally intact. Florescent dye FM1-43 has been shown to enter hair cells through the MET channels, providing an indicator of the functional integrity of hair cells (Gale et al., 2001; Meyers et al., 2003). Here we used FM1-43FX, a fixable analog of FM1-43, to examine the function integrity of Cdk5 cko hair cells. The results showed that FM1-43FX uptake of P8 Cdk5 cko hair cells was indistinguishable from that of control hair cells, suggesting that hair cell function is not affected in newborn Cdk5 cko mice (Supplementary Figure S2).
Cdk5 Inactivation in Hair Cells Affects Stereocilia Maintenance and Causes Hair Cell Loss
In contrast to the normal stereocilia morphology observed at P8, phalloidin staining revealed that stereocilia degeneration took place in some of the Cdk5 cko OHCs at age of 1-month, which was exacerbated furthermore at age of 5-months (Figures 3B,C). SEM confirmed the phalloidin staining results. By age of 1-month, complete stereocilia degeneration could be observed in some Cdk5 cko OHCs (Figures 5A–E). By age of 5-months, more OHCs of Cdk5 cko mice showed stereocilia degeneration, especially at the basal turn (Figures 6A–D). Meanwhile, stereocilia fusion could be observed in some IHCs of 5-month-old Cdk5 cko mice (Figure 6E). Taken together, our data suggested that although Cdk5 inactivation does not affect stereocilia development, it causes serious stereocilia maintenance deficits.
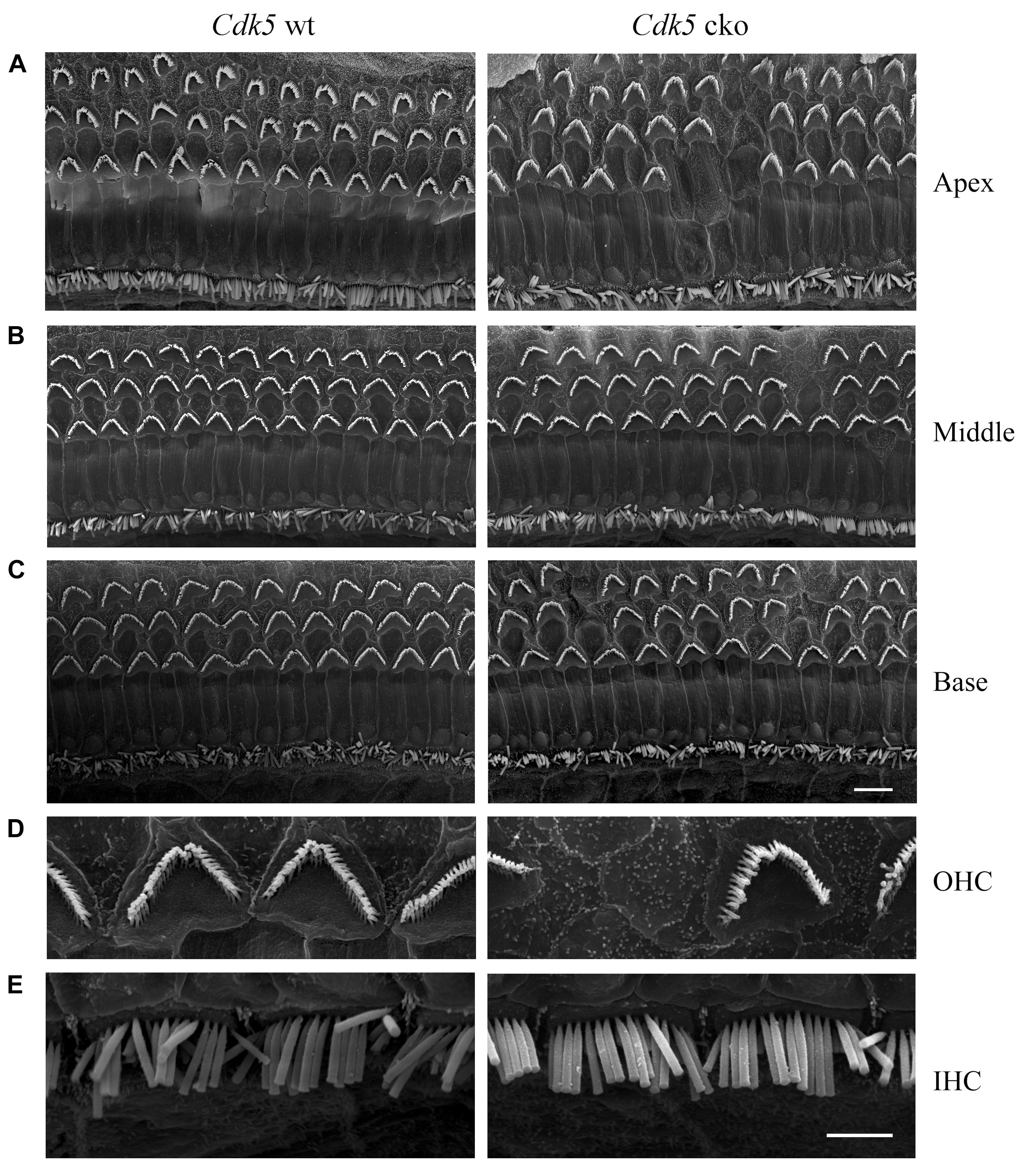
Figure 5. Hair bundle morphology of 1-month-old Cdk5 cko mice examined by SEM. (A) Low magnification images of hair bundles of Cdk5lox/lox and Atoh1Cre/+; Cdk5lox/lox cochleae at apical turn. (B) Low magnification images of hair bundles of Cdk5lox/lox and Atoh1Cre/+; Cdk5lox/lox cochleae at middle turn. (C) Low magnification images of hair bundles of Cdk5lox/lox and Atoh1Cre/+; Cdk5lox/lox cochleae at basal turn. Scale bar, 5 μm. (D) High magnification images of hair bundles of middle turn Cdk5lox/lox and Atoh1Cre/+; Cdk5lox/lox OHCs. (E) High magnification images of hair bundles of middle turn Cdk5lox/lox and Atoh1Cre/+; Cdk5lox/lox IHCs. Scale bar, 2 μm.
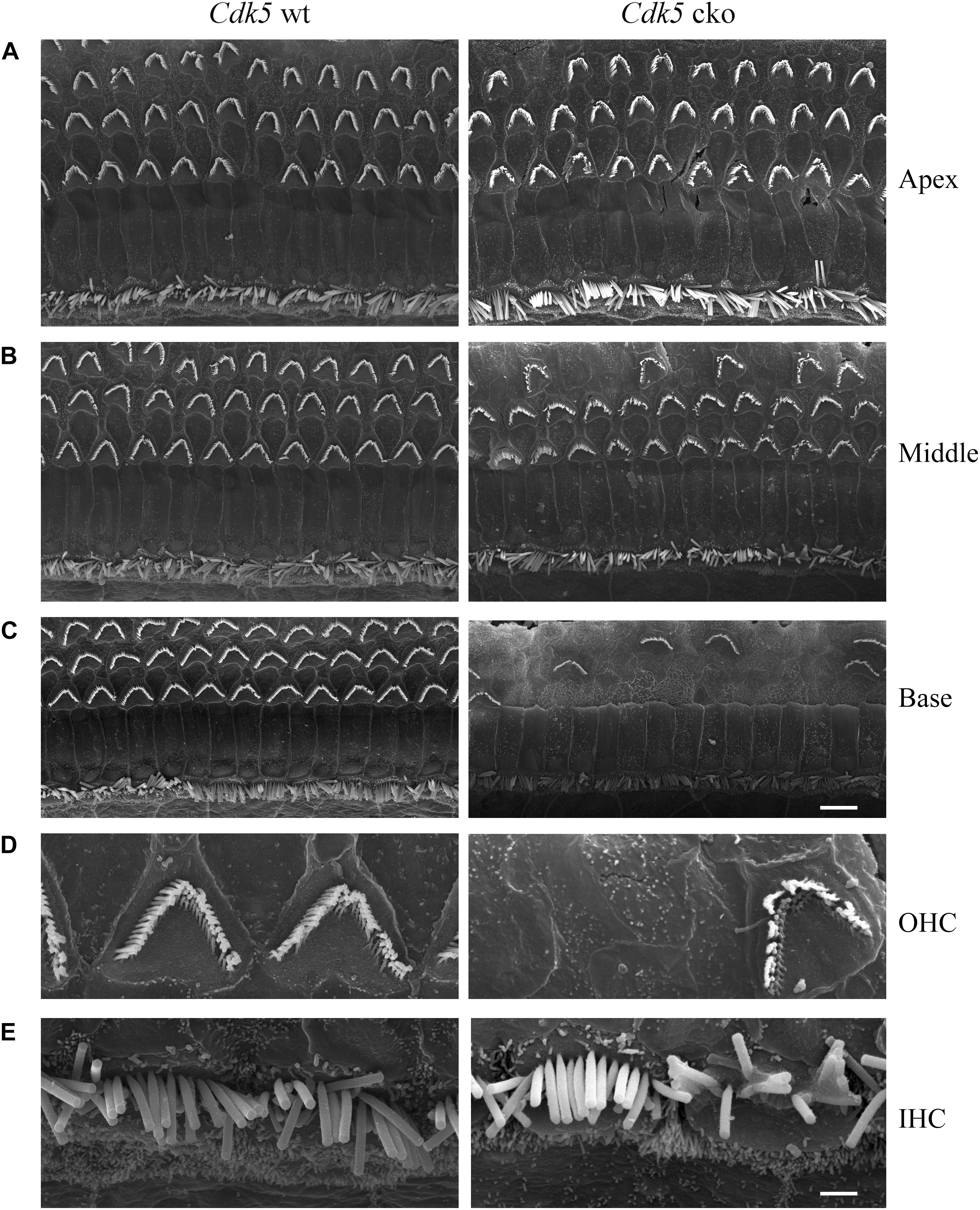
Figure 6. Hair bundle morphology of 5-month-old Cdk5 cko mice examined by SEM. (A) Low magnification images of hair bundles of Cdk5lox/lox and Atoh1Cre/+; Cdk5lox/lox cochleae at apical turn. (B) Low magnification images of hair bundles of Cdk5lox/lox and Atoh1Cre/+; Cdk5lox/lox cochleae at middle turn. (C) Low magnification images of hair bundles of Cdk5lox/lox and Atoh1Cre/+;Cdk5lox/lox cochleae at basal turn. Scale bar, 5 μm. (D) High magnification images of hair bundles of middle turn Cdk5lox/lox and Atoh1Cre/+; Cdk5lox/lox OHCs. (E) High magnification images of hair bundles of middle turn Cdk5lox/lox and Atoh1Cre/+; Cdk5lox/lox IHCs. Scale bar, 1 μm.
Stereocilia degeneration is usually followed by hair cell loss, hence we examined hair cells by performing whole-mount immunostaining using an antibody that detects hair cell marker myosin VI (MYO6) (Torii et al., 2016; Wong et al., 2016). The results showed that compared to control mice, there was increased OHC loss in Cdk5 cko mice, which is exacerbated when mice age (Figure 7A). At age of 1-month, less than 10% of OHC was lost at the basal-middle turn cochlea of Cdk5 cko mice, and this number reached around 80% by age of 6-months (Figure 7B). In contrast, IHC loss in Cdk5 cko mice was negligible (Figures 7A,C).
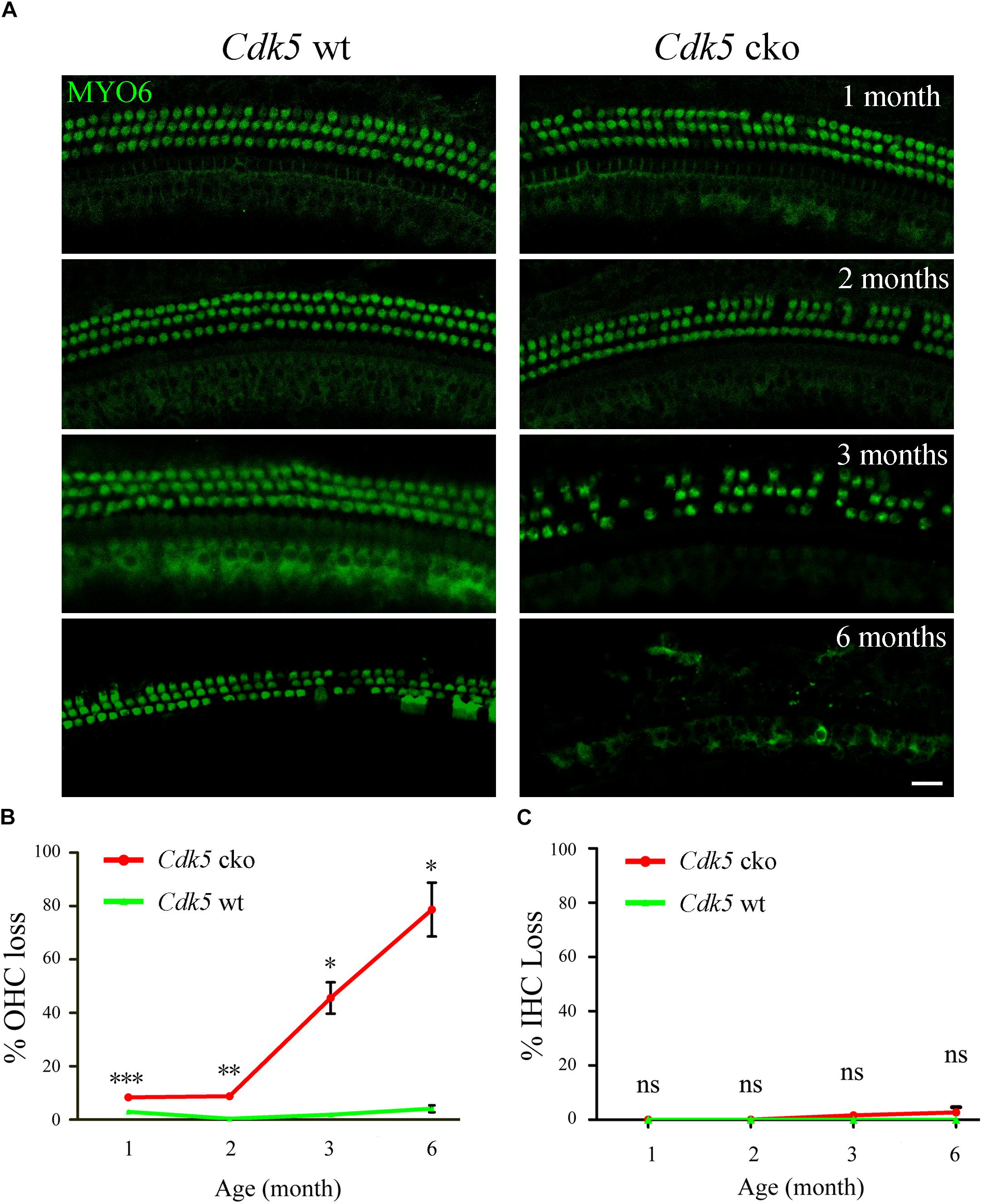
Figure 7. OHC loss was increased in Cdk5 cko mice. (A) Whole-mount immunostaining was performed using an anti-MYO6 antibody at different ages as indicated. Shown are single confocal images of basal-middle turn cochlea. Scale bar, 20 μm. Please notice that MYO6 immunoreactivity in most IHCs is rather weak because they are not at the same focus plane as the OHCs. (B) OHC loss was quantified according to the results from (A). (C) IHC loss was quantified according to the results from (A). Numbers of mice used in each group are more than three. ∗p < 0.05; ∗∗p < 0.01; ∗∗∗p < 0.001; ns, not significant.
Ribbon Synapse Formation Is Unaffected in Cdk5 cko Mice
CDK5 has been shown to play important roles in synapse development and function (Tan et al., 2003; Fu et al., 2005; Lin et al., 2005; Lai and Ip, 2015). IHCs contain specialized synapses named ribbon synapses, which are also present in retinal photoreceptors, etc (Matthews and Fuchs, 2010). Ribeye/CtBP2 is one of the most abundant proteins in ribbon synapses and is commonly used as a marker for ribbon synapses (Schmitz et al., 2000). We then examined the integrity of hair cell ribbon synapses by performing whole-mount immunostaining with an anti-CtBP2 antibody. The results revealed no difference between Cdk5 cko mice and control mice at different developmental stages (Figures 8A–D), suggesting that CDK5 inactivation does not affect the formation of ribbon synapses of cochlear hair cells.
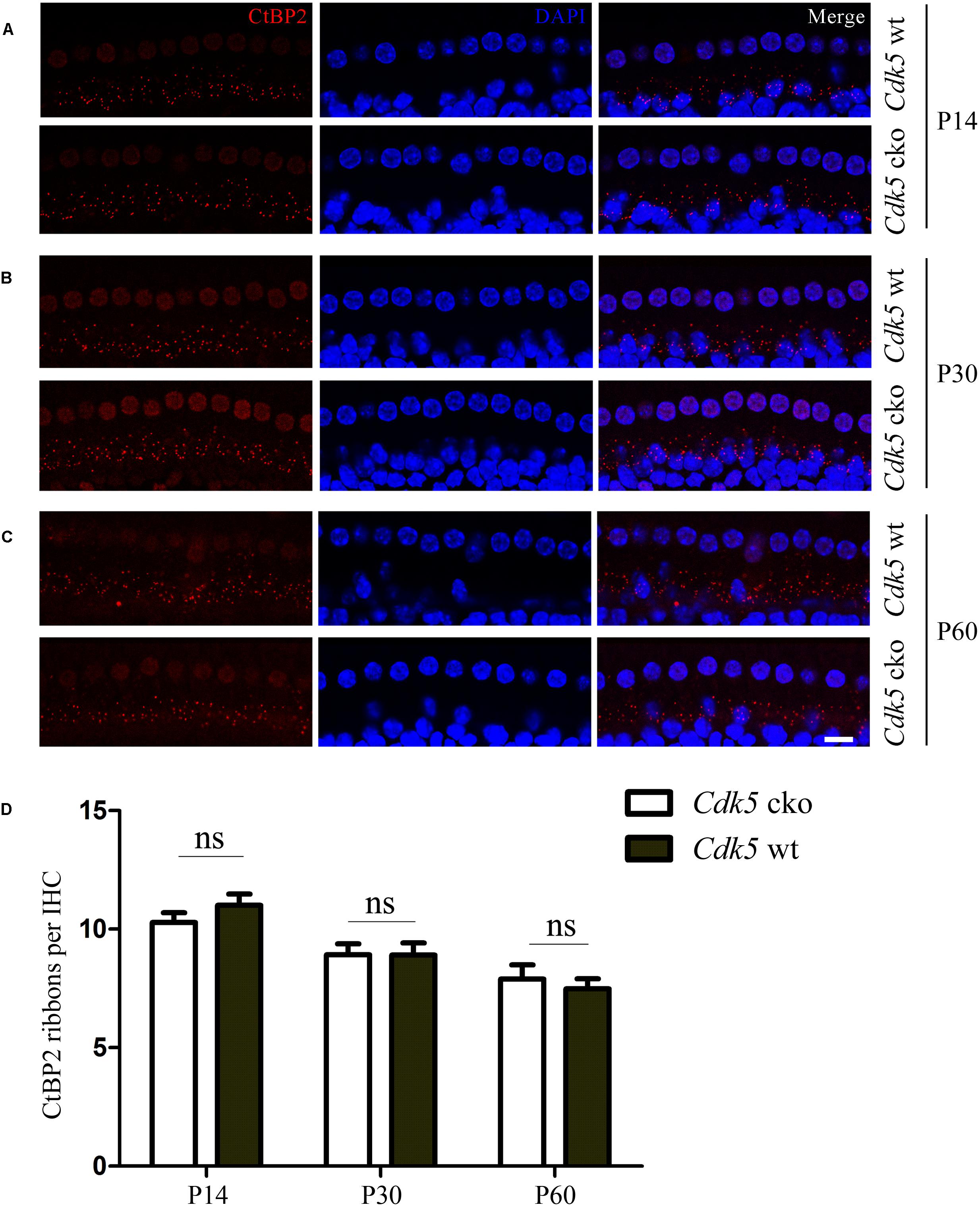
Figure 8. Ribbon synapses are unaffected in Cdk5 cko mice. (A) Whole-mount immunostaining of CtBP2 in P14 Atoh1Cre/+; Cdk5lox/lox and Cdk5lox/lox IHCs. (B) Whole-mount immunostaining of CtBP2 in P30 Atoh1Cre/+; Cdk5lox/lox and Cdk5lox/lox IHCs. (C) Whole-mount immunostaining of CtBP2 in P60 Atoh1Cre/+; Cdk5lox/lox and Cdk5lox/lox IHCs. Nuclei were visualized by DAPI. Images were taken from the middle turn of cochleae using a confocal microscope. Scale bar, 10 μm. (D) CtBP2-positive ribbon synapses were quantified according to the results from (A–C).
ERM Phosphorylation Is Decreased in the Inner Ear of Cdk5 cko Mice
CDK5 phosphorylates many proteins that are involved in F-actin assembly and maintenance. ERM (ezrin, radixin, and moesin) proteins are known CDK5 substrates (Yang et al., 2003; Yang and Hinds, 2003), and have been shown to play important roles in stereocilia development and/or maintenance (Kitajiri et al., 2004; Pataky et al., 2004; Han et al., 2015). Hence we examined the effect of Cdk5 inactivation on the phosphorylation status of ERM proteins in the cochleae. Whole-mount immunostaining showed that phosphorylated ERM (pERM) was readily detected in the stereocilia of control mice, but significantly decreased in the stereocilia of newborn Cdk5 cko mice (Figure 9A and Supplementary Figure S3). Furthermore, western blot results confirmed that pERM was significantly reduced in the Cdk5 ko inner ear at E18.5 (Figure 9B). Taken together, our data suggested that pERM is decreased in the stereocilia of Cdk5 cko mice, which might contribute to the abnormal stereocilia maintenance.
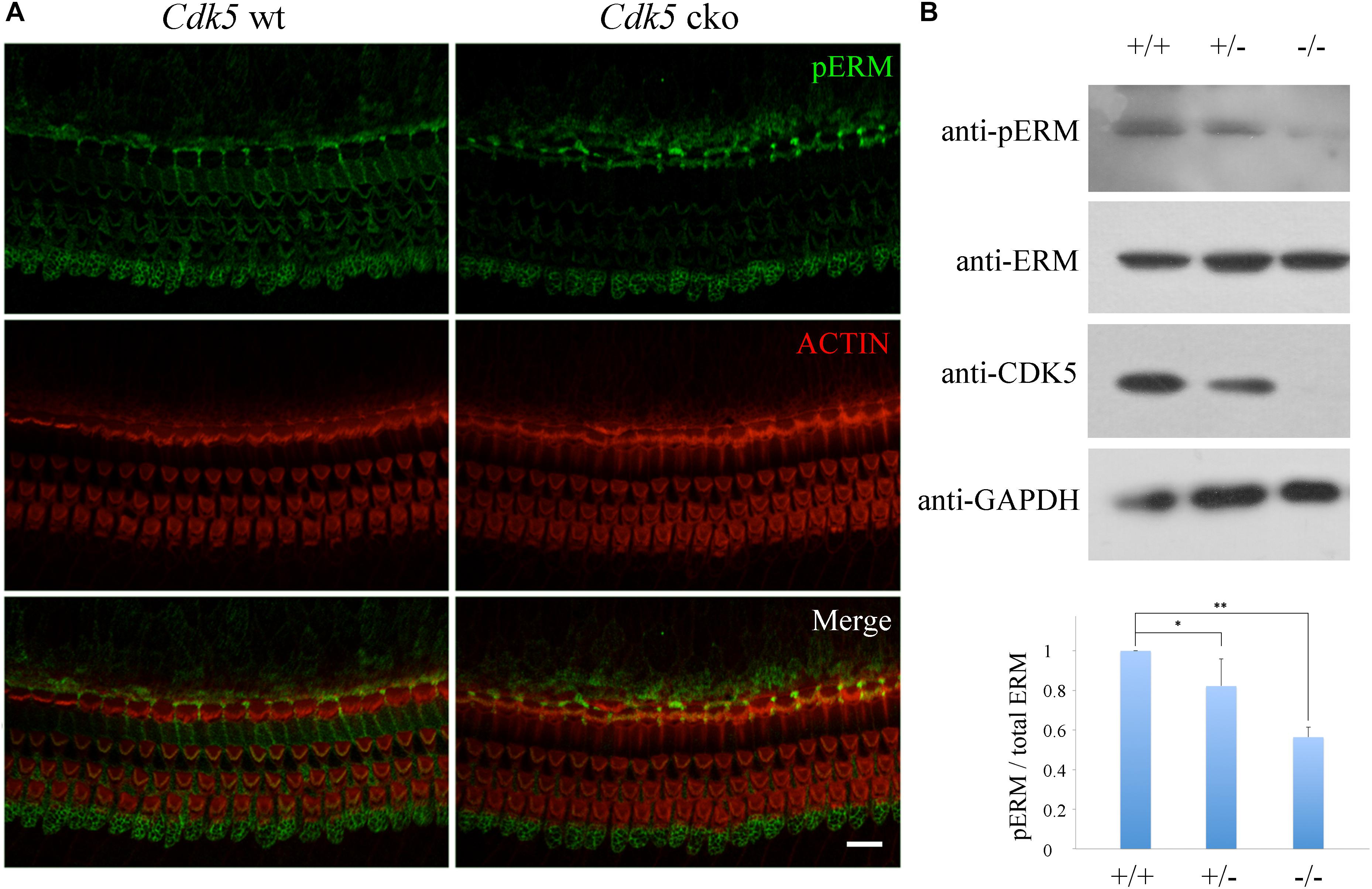
Figure 9. ERM phosphorylation is reduced by Cdk5 inactivation. (A) Whole-mount immunostaining of phosphorylated ERM (pERM) in P8 Atoh1Cre/+; Cdk5lox/lox and Cdk5lox/lox organ of Corti. F-actin core of stereocilia was visualized by TRITC-conjugated phalloidin. Images were taken from the middle turn of cochleae using a confocal microscope. Scale bar, 10 μm. (B) Expression level of pERM, ERM, and CDK5 in the inner ear of E18.5 Cdk5+/+, Cdk5+/-, and Cdk5-/- mice was examined by western blot. GAPDH was included as internal control. The bar graph shows quantification of three independent experiments. ∗p < 0.05; ∗∗p < 0.01.
Discussion
CDK5 plays important roles in neural development and function, and dysfunction of CDK5 is associated with a number of neurological disorders (Su and Tsai, 2011). The role of CDK5 in the central nervous system has been extensively studied, whereas its role in peripheral sensory systems is less understood. It was shown that CDK5 and its activator p35 are highly expressed in primary afferent nociceptive fibers in mouse dorsal root ganglia, where they modulate nociceptive signaling through phosphorylation of transient receptor potential vanilloid 1 (TRPV1) (Pareek et al., 2006, 2007; Yang et al., 2007). CDK5 has also been shown to express in chicken auditory hair cells and regulate the membrane expression and kinetics of BK channel Slo through phosphorylation (Bai et al., 2012), but its physiological role in hearing has not been investigated.
In the present work, we showed that CDK5 was expressed in the cell body of mouse cochlear hair cells. The expression of Cdk5 in mouse cochlear hair cells was supported by RNA transcriptome sequencing result (SHIELD1) (Shen et al., 2015). We found that CDK5 inactivation in hair cells led to severe stereocilia degeneration. Stereocilia are F-actin-based, microvilli-like protrusions on the apical surface of hair cells, and are indispensable for hearing transduction (Schwander et al., 2010). Stereocilia degeneration was mainly detected in the OHCs of Cdk5 cko mice, while OHCs are known to play important roles in amplifying sound-evoked vibrations. Consequently, the observed OHC stereocilia degeneration might explain the profound hearing loss in Cdk5 cko mice. Meanwhile, stereocilia disorganization was also observed in some IHCs, which might also contribute to the hearing loss in Cdk5 cko mice.
Further investigation showed that phosphorylation of CDK5 substrate ERM proteins was significantly decreased in Cdk5 cko hair cells, which might contribute to the eventual stereocilia degeneration. ERM proteins are evolutionary conserved group of three related proteins including ezrin, radixin, and moesin. ERM proteins crosslink actin filaments with plasma membrane, and play important roles in the organization of microvilli (Fehon et al., 2010). In the mouse cochlear hair cells, ezrin is expressed in the stereocilia at very low level, whereas moesin is not detected at all (Kitajiri et al., 2004). In contrast, radixin is intensely expressed in the stereocilia (Kitajiri et al., 2004). Mutations in the human RDX gene that encodes radixin are associated with non-syndromic hearing loss DFNB24 (Khan et al., 2007). In mice, Rdx deficiency causes progressive degeneration of cochlear stereocilia that eventually leads to hearing loss (Kitajiri et al., 2004). Similar phenotypes of stereocilia degeneration in Rdx ko mice and Cdk5 cko mice are consistent with the hypothesis that CDK5 regulates stereocilia maintenance through phosphorylation of ERM proteins.
CDK5 has been suggested to play pivotal roles in synapse development and/or function (Lai and Ip, 2015). For example, it was shown that phosphorylation of liprinα1 by CDK5 mediates neuronal activity-dependent synapse development in the brain (Huang et al., 2017). Cdk5 inactivation causes morphological abnormalities at the neuromuscular junction (NMJ) both pre- and post-synaptically (Fu et al., 2005). However, our present data showed that CtBP2 immunoreactivity in Cdk5 cko hair cells was comparable to that in control mice, suggesting that Cdk5 inactivation does not affect the development of hair cell ribbon synapses. Whether the function of hair cell ribbon synapses is affected by Cdk5 inactivation is not examined in the present study and awaits further investigation.
Besides stereocilia degeneration, robust OHC loss was also observed in Cdk5 cko mice. Our present hypothesis is that Cdk5 inactivation causes stereocilia degeneration through dysregulated ERM phosphorylation, which then leads to hair cell degeneration. However, possibility remains that stereocilia degeneration and hair cell degeneration might happen independently to each other in Cdk5 cko mice, or alternatively, stereocilia degeneration is even a result of hair cell degeneration. It has been reported that CDK5 could directly regulate cell survival, and that CDK5 dysfunction could lead to cell death (Zhou et al., 2015; Shi et al., 2016; Nikhil and Shah, 2017; NavaneethaKrishnan et al., 2018). Identification of hair cell-expressed CDK5 substrates other than ERM and Slo will help us to address this question.
A pharmacological inhibitor of CDK5, roscovitine, has been shown to significantly increase the number of hair cells and supporting cells in rat cochlear cultures through triggering differentiation of precursor cells (Malgrange et al., 2003). In the present work, however, supernumerary hair cells and supporting cells were not observed in Cdk5 cko cochleae. This discrepancy might result from the fact that CDK5 was inactivated only in hair cells of Cdk5 cko mice in the present work, whereas roscovitine affected both hair cell and supporting cells in the cochlear cultures. Additionally, it remains possible that roscovitine might trigger differentiation of cochlear precursor cells through inhibition of kinases other than CDK5. In line with this, roscovitine has been shown to also inhibit CDK1and CDK2, though with a lower efficiency (Meijer et al., 1997), and expression of CDK1 and CDK2 has been detected in rat hair cells and/or supporting cells (Malgrange et al., 2003).
As mentioned above, the kinase activity of CDK5 needs to be activated by specific activators. Three CDK5 activators have been identified, namely p35, p39, and CCNI (Tsai et al., 1994; Tang et al., 1995; Brinkkoetter et al., 2009). Inactivation of CDK5 activator(s) has been shown to cause neuronal deficits (Su and Tsai, 2011; Luo et al., 2016; Kamiki et al., 2018). Recently, our group reported a novel CDK5 activator, CCNI2, which is present in human, chicken, and zebrafish, but not in mouse and rat (Liu et al., 2017). The expression of CDK5 activators in the inner ear has not been reported. However, RNA transcriptome sequencing revealed that p35 and Ccni, but not p39 transcript, were expressed in mouse cochlear hair cells (Shen et al., 2015). At present, whether p35 or CCNI is necessary for CDK5 activation in hair cells remains unknown and awaits further investigation.
Ethics Statement
All animal experimental procedures were approved by the Animal Ethics Committee of Shandong University School of Life Sciences (Permit Number: SYDWLL-2017-05) and performed accordingly.
Author Contributions
ZX conceived the study. XZ, CL, BZ, and YW performed the experiments. All authors analyzed the data. ZX wrote the manuscript.
Funding
This work was supported by grants from the National Natural Science Foundation of China (81771001), Technology and Innovation Commission of Shenzhen Municipality (JCYJ20160331174108379), and the Fundamental Research Funds of Shandong University (2018JC025). The funders had no role in study design, data collection and analysis, decision to publish, or preparation of the manuscript.
Conflict of Interest Statement
The authors declare that the research was conducted in the absence of any commercial or financial relationships that could be construed as a potential conflict of interest.
Supplementary Material
The Supplementary Material for this article can be found online at: https://www.frontiersin.org/articles/10.3389/fnmol.2018.00461/full#supplementary-material
Footnotes
References
Bai, J. P., Surguchev, A., Joshi, P., Gross, L., and Navaratnam, D. (2012). CDK5 interacts with Slo and affects its surface expression and kinetics through direct phosphorylation. Am. J. Physiol. Cell Physiol. 302, C766–C780. doi: 10.1152/ajpcell.00339.2011
Banks, A. S., McAllister, F. E., Camporez, J. P., Zushin, P. J., Jurczak, M. J., Laznik-Bogoslavski, D., et al. (2015). An ERK/Cdk5 axis controls the diabetogenic actions of PPARgamma. Nature 517, 391–395. doi: 10.1038/nature13887
Beurg, M., Fettiplace, R., Nam, J. H., and Ricci, A. J. (2009). Localization of inner hair cell mechanotransducer channels using high-speed calcium imaging. Nat. Neurosci. 12, 553–558. doi: 10.1038/nn.2295
Brinkkoetter, P. T., Olivier, P., Wu, J. S., Henderson, S., Krofft, R. D., Pippin, J. W., et al. (2009). Cyclin I activates Cdk5 and regulates expression of Bcl-2 and Bcl-XL in postmitotic mouse cells. J. Clin. Invest. 119, 3089–3101. doi: 10.1172/JCI37978
Fehon, R. G., McClatchey, A. I., and Bretscher, A. (2010). Organizing the cell cortex: the role of ERM proteins. Nat. Rev. Mol. Cell Biol. 11, 276–287. doi: 10.1038/nrm2866
Fu, A. K., Ip, F. C., Fu, W. Y., Cheung, J., Wang, J. H., Yung, W. H., et al. (2005). Aberrant motor axon projection, acetylcholine receptor clustering, and neurotransmission in cyclin-dependent kinase 5 null mice. Proc. Natl. Acad. Sci. U.S.A. 102, 15224–15229. doi: 10.1073/pnas.0507678102
Gale, J. E., Marcotti, W., Kennedy, H. J., Kros, C. J., and Richardson, G. P. (2001). FM1-43 dye behaves as a permeant blocker of the hair-cell mechanotransducer channel. J. Neurosci. 21, 7013–7025. doi: 10.1523/JNEUROSCI.21-18-07013.2001
Guan, J. S., Su, S. C., Gao, J., Joseph, N., Xie, Z., Zhou, Y., et al. (2011). Cdk5 is required for memory function and hippocampal plasticity via the cAMP signaling pathway. PLoS One 6:e25735. doi: 10.1371/journal.pone.0025735
Han, Y., Wang, X., Chen, J., and Sha, S. H. (2015). Noise-induced cochlear F-actin depolymerization is mediated via ROCK2/p-ERM signaling. J. Neurochem. 133, 617–628. doi: 10.1111/jnc.13061
Hawasli, A. H., Benavides, D. R., Nguyen, C., Kansy, J. W., Hayashi, K., Chambon, P., et al. (2007). Cyclin-dependent kinase 5 governs learning and synaptic plasticity via control of NMDAR degradation. Nat. Neurosci. 10, 880–886. doi: 10.1038/nn1914
Hirasawa, M., Ohshima, T., Takahashi, S., Longenecker, G., Honjo, Y., Veeranna, et al. (2004). Perinatal abrogation of Cdk5 expression in brain results in neuronal migration defects. Proc. Natl. Acad. Sci. U.S.A. 101, 6249–6254. doi: 10.1073/pnas.0307322101
Huang, H., Lin, X., Liang, Z., Zhao, T., Du, S., Loy, M. M. T., et al. (2017). Cdk5-dependent phosphorylation of liprinalpha1 mediates neuronal activity-dependent synapse development. Proc. Natl. Acad. Sci. U.S.A. 114, E6992–E7001. doi: 10.1073/pnas.1708240114
Jones, C., Roper, V. C., Foucher, I., Qian, D., Banizs, B., Petit, C., et al. (2008). Ciliary proteins link basal body polarization to planar cell polarity regulation. Nat. Genet. 40, 69–77. doi: 10.1038/ng.2007.54
Kamiki, E., Boehringer, R., Polygalov, D., Ohshima, T., and McHugh, T. J. (2018). Inducible knockout of the Cyclin-dependent kinase 5 activator p35 alters hippocampal spatial doding and neuronal excitability. Front. Cell Neurosci. 12:138. doi: 10.3389/fncel.2018.00138
Khan, S. Y., Ahmed, Z. M., Shabbir, M. I., Kitajiri, S., Kalsoom, S., Tasneem, S., et al. (2007). Mutations of the RDX gene cause nonsyndromic hearing loss at the DFNB24 locus. Hum. Mutat. 28, 417–423. doi: 10.1002/humu.20469
Kitajiri, S., Fukumoto, K., Hata, M., Sasaki, H., Katsuno, T., Nakagawa, T., et al. (2004). Radixin deficiency causes deafness associated with progressive degeneration of cochlear stereocilia. J. Cell Biol. 166, 559–570. doi: 10.1083/jcb.200402007
Lai, K. O., and Ip, N. Y. (2015). Cdk5: a key player at neuronal synapse with diverse functions. Mini Rev. Med. Chem. 15, 390–395. doi: 10.2174/1389557515666150324122321
Lakso, M., Pichel, J. G., Gorman, J. R., Sauer, B., Okamoto, Y., Lee, E., et al. (1996). Efficient in vivo manipulation of mouse genomic sequences at the zygote stage. Proc. Natl. Acad. Sci. U.S.A. 93, 5860–5865. doi: 10.1073/pnas.93.12.5860
Lee, M. S., Kwon, Y. T., Li, M., Peng, J., Friedlander, R. M., and Tsai, L. H. (2000). Neurotoxicity induces cleavage of p35 to p25 by calpain. Nature 405, 360–364. doi: 10.1038/35012636
Lin, W., Dominguez, B., Yang, J., Aryal, P., Brandon, E. P., Gage, F. H., et al. (2005). Neurotransmitter acetylcholine negatively regulates neuromuscular synapse formation by a Cdk5-dependent mechanism. Neuron 46, 569–579. doi: 10.1016/j.neuron.2005.04.002
Lindeman, H. H., Ades, H. W., Bredberg, G., and Engstrom, H. (1971). The sensory hairs and the tectorial membrane in the development of the cat s organ of Corti. A scanning electron microscopic study. Acta Otolaryngol. 72, 229–242. doi: 10.3109/00016487109122478
Liu, C., Zhai, X., Zhao, B., Wang, Y., and Xu, Z. (2017). Cyclin I-like (CCNI2) is a cyclin-dependent kinase 5 (CDK5) activator and is involved in cell cycle regulation. Sci. Rep. 7:40979. doi: 10.1038/srep40979
Luo, F., Zhang, J., Burke, K., Miller, R. H., and Yang, Y. (2016). The activators of Cyclin-dependent kinase 5 p35 and p39 are essential for oligodendrocyte maturation, process formation, and myelination. J. Neurosci. 36, 3024–3037. doi: 10.1523/JNEUROSCI.2250-15.2016
Malgrange, B., Knockaert, M., Belachew, S., Nguyen, L., Moonen, G., Meijer, L., et al. (2003). The inhibition of cyclin-dependent kinases induces differentiation of supernumerary hair cells and Deiters’ cells in the developing organ of Corti. FASEB J. 17, 2136–2138. doi: 10.1096/fj.03-0035fje
Matthews, G., and Fuchs, P. (2010). The diverse roles of ribbon synapses in sensory neurotransmission. Nat. Rev. Neurosci. 11, 812–822. doi: 10.1038/nrn2924
Meijer, L., Borgne, A., Mulner, O., Chong, J. P., Blow, J. J., Inagaki, N., et al. (1997). Biochemical and cellular effects of roscovitine, a potent and selective inhibitor of the cyclin-dependent kinases cdc2, cdk2 and cdk5. Eur. J. Biochem. 243, 527–536. doi: 10.1111/j.1432-1033.1997.t01-2-00527.x
Meyers, J. R., MacDonald, R. B., Duggan, A., Lenzi, D., Standaert, D. G., Corwin, J. T., et al. (2003). Lighting up the senses: FM1-43 loading of sensory cells through nonselective ion channels. J. Neurosci. 23, 4054–4065. doi: 10.1523/JNEUROSCI.23-10-04054.2003
NavaneethaKrishnan, S., Rosales, J. L., and Lee, K. Y. (2018). Loss of Cdk5 in breast cancer cells promotes ROS-mediated cell death through dysregulation of the mitochondrial permeability transition pore. Oncogene 37, 1788–1804. doi: 10.1038/s41388-017-0103-1
Nikhil, K., and Shah, K. (2017). The Cdk5-Mcl-1 axis promotes mitochondrial dysfunction and neurodegeneration in a model of Alzheimer’s disease. J. Cell Sci. 130, 3023–3039. doi: 10.1242/jcs.205666
Nikolic, M., Dudek, H., Kwon, Y. T., Ramos, Y. F., and Tsai, L. H. (1996). The cdk5/p35 kinase is essential for neurite outgrowth during neuronal differentiation. Genes Dev. 10, 816–825. doi: 10.1101/gad.10.7.816
Ohshima, T., Ward, J. M., Huh, C. G., Longenecker, G., Veeranna, Pant, H. C., et al. (1996). Targeted disruption of the cyclin-dependent kinase 5 gene results in abnormal corticogenesis, neuronal pathology and perinatal death. Proc. Natl. Acad. Sci. U.S.A. 93, 11173–11178. doi: 10.1073/pnas.93.20.11173
Pareek, T. K., Keller, J., Kesavapany, S., Agarwal, N., Kuner, R., Pant, H. C., et al. (2007). Cyclin-dependent kinase 5 modulates nociceptive signaling through direct phosphorylation of transient receptor potential vanilloid 1. Proc. Natl. Acad. Sci. U.S.A. 104, 660–665. doi: 10.1073/pnas.0609916104
Pareek, T. K., Keller, J., Kesavapany, S., Pant, H. C., Iadarola, M. J., Brady, R. O., et al. (2006). Cyclin-dependent kinase 5 activity regulates pain signaling. Proc. Natl. Acad. Sci. U.S.A. 103, 791–796. doi: 10.1073/pnas.0510405103
Pataky, F., Pironkova, R., and Hudspeth, A. J. (2004). Radixin is a constituent of stereocilia in hair cells. Proc. Natl. Acad. Sci. U.S.A. 101, 2601–2606. doi: 10.1073/pnas.0308620100
Patrick, G. N., Zukerberg, L., Nikolic, M., de la Monte, S., Dikkes, P., and Tsai, L. H. (1999). Conversion of p35 to p25 deregulates Cdk5 activity and promotes neurodegeneration. Nature 402, 615–622. doi: 10.1038/45159
Samuels, B. A., Hsueh, Y. P., Shu, T., Liang, H., Tseng, H. C., Hong, C. J., et al. (2007). Cdk5 promotes synaptogenesis by regulating the subcellular distribution of the MAGUK family member CASK. Neuron 56, 823–837. doi: 10.1016/j.neuron.2007.09.035
Schmitz, F., Konigstorfer, A., and Sudhof, T. C. (2000). RIBEYE, a component of synaptic ribbons: a protein’s journey through evolution provides insight into synaptic ribbon function. Neuron 28, 857–872. doi: 10.1016/S0896-6273(00)00159-8
Schwander, M., Kachar, B., and Muller, U. (2010). Review series: the cell biology of hearing. J. Cell Biol. 190, 9–20. doi: 10.1083/jcb.201001138
Shen, J., Scheffer, D. I., Kwan, K. Y., and Corey, D. P. (2015). SHIELD: an integrative gene expression database for inner ear research. Database 2015:bav071. doi: 10.1093/database/bav071
Shi, C., Viccaro, K., Lee, H. G., and Shah, K. (2016). Cdk5-Foxo3 axis: initially neuroprotective, eventually neurodegenerative in Alzheimer’s disease models. J. Cell Sci. 129, 1815–1830. doi: 10.1242/jcs.185009
Su, S. C., and Tsai, L. H. (2011). Cyclin-dependent kinases in brain development and disease. Annu. Rev. Cell Dev. Biol. 27, 465–491. doi: 10.1146/annurev-cellbio-092910-154023
Tan, T. C., Valova, V. A., Malladi, C. S., Graham, M. E., Berven, L. A., Jupp, O. J., et al. (2003). Cdk5 is essential for synaptic vesicle endocytosis. Nat. Cell Biol. 5, 701–710. doi: 10.1038/ncb1020
Tang, D., Yeung, J., Lee, K. Y., Matsushita, M., Matsui, H., Tomizawa, K., et al. (1995). An isoform of the neuronal cyclin-dependent kinase 5 (Cdk5) activator. J. Biol. Chem. 270, 26897–26903. doi: 10.1074/jbc.270.45.26897
Torii, H., Yoshida, A., Katsuno, T., Nakagawa, T., Ito, J., Omori, K., et al. (2016). Septin7 regulates inner ear formation at an early developmental stage. Dev. Biol. 419, 217–228. doi: 10.1016/j.ydbio.2016.09.012
Tsai, L. H., Delalle, I., Caviness, V. S. Jr., Chae, T., and Harlow, E. (1994). p35 is a neural-specific regulatory subunit of cyclin-dependent kinase 5. Nature 371, 419–423. doi: 10.1038/371419a0
Tsai, L. H., Takahashi, T., Caviness, V. S. Jr., and Harlow, E. (1993). Activity and expression pattern of cyclin-dependent kinase 5 in the embryonic mouse nervous system. Development 119, 1029–1040.
Wong, E. Y., Xu, C. Y., Brahmachary, M., and Xu, P. X. (2016). A novel ENU-induced mutation in Myo6 causes vestibular dysfunction and deafness. PLoS One 11:e0154984. doi: 10.1371/journal.pone.0154984
Yang, H., Xie, X., Deng, M., Chen, X., and Gan, L. (2010). Generation and characterization of Atoh1-Cre knock-in mouse line. Genesis 48, 407–413. doi: 10.1002/dvg.20633
Yang, H. S., Alexander, K., Santiago, P., and Hinds, P. W. (2003). ERM proteins and Cdk5 in cellular senescence. Cell Cycle 2, 517–520. doi: 10.4161/cc.2.6.582
Yang, H. S., and Hinds, P. W. (2003). Increased ezrin expression and activation by CDK5 coincident with acquisition of the senescent phenotype. Mol. Cell. 11, 1163–1176. doi: 10.1016/S1097-2765(03)00135-7
Yang, Y. R., He, Y., Zhang, Y., Li, Y., Li, Y., Han, Y., et al. (2007). Activation of cyclin-dependent kinase 5 (Cdk5) in primary sensory and dorsal horn neurons by peripheral inflammation contributes to heat hyperalgesia. Pain 127, 109–120. doi: 10.1016/j.pain.2006.08.008
Keywords: inner ear, hair cells, stereocilia, hearing loss, CDK5
Citation: Zhai X, Liu C, Zhao B, Wang Y and Xu Z (2018) Inactivation of Cyclin-Dependent Kinase 5 in Hair Cells Causes Hearing Loss in Mice. Front. Mol. Neurosci. 11:461. doi: 10.3389/fnmol.2018.00461
Received: 13 April 2018; Accepted: 29 November 2018;
Published: 11 December 2018.
Edited by:
David Z. He, Creighton University School of Medicine, United StatesReviewed by:
Bifeng Pan, Decibel Therapeutics, Inc., United StatesWei-Jia Kong, Huazhong University of Science and Technology, China
Thomas Schimmang, Consejo Superior de Investigaciones Científicas (CSIC), Spain
Copyright © 2018 Zhai, Liu, Zhao, Wang and Xu. This is an open-access article distributed under the terms of the Creative Commons Attribution License (CC BY). The use, distribution or reproduction in other forums is permitted, provided the original author(s) and the copyright owner(s) are credited and that the original publication in this journal is cited, in accordance with accepted academic practice. No use, distribution or reproduction is permitted which does not comply with these terms.
*Correspondence: Zhigang Xu, xuzg@sdu.edu.cn
†These authors have contributed equally to this work