- 1Department of Pharmacy, Southeast University, Dhaka, Bangladesh
- 2Pharmakon Neuroscience Research Network, Dhaka, Bangladesh
- 3Center for Neuroscience, Brain Science Institute, Korea Institute of Science and Technology, Seoul, South Korea
- 4Department of Pharmacy, Brac University, Dhaka, Bangladesh
- 5Department of Zoology, College of Science, King Saud University, Riyadh, Saudi Arabia
- 6Department of Biology, Faculty of Sciences, Univesity of Hafr Al Batin, Hafr Al Batin, Saudi Arabia
- 7Glocal School of Life Sciences, Glocal University, Saharanpur, India
- 8King Fahd Medical Research Center, King Abdulaziz University, Jeddah, Saudi Arabia
- 9Department of Medical Laboratory Technology, Faculty of Applied Medical Sciences, King Abdulaziz University, Jeddah, Saudi Arabia
- 10Department of Biology, College of Science, Princess Nourah bint Abdulrahman University, Riyadh, Saudi Arabia
- 11Pharmacology Department, Faculty of Veterinary Medicine, Suez Canal University, Ismailia, Egypt
Neuropathic pain (NP) is the result of irregular processing in the central or peripheral nervous system, which is generally caused by neuronal injury. The management of NP represents a great challenge owing to its heterogeneous profile and the significant undesirable side effects of the frequently prescribed psychoactive agents, including benzodiazepines (BDZ). Currently, several established drugs including antidepressants, anticonvulsants, topical lidocaine, and opioids are used to treat NP, but they exert a wide range of adverse effects. To reduce the burden of adverse effects, we need to investigate alternative therapeutics for the management of NP. Flavonoids are the most common secondary metabolites of plants used in folkloric medicine as tranquilizers, and have been claimed to have a selective affinity to the BDZ binding site. Several studies in animal models have reported that flavonoids can reduce NP. In this paper, we emphasize the potentiality of flavonoids for the management of NP.
Introduction
Neuropathic pain (NP) is caused by damage or disease affecting the somatosensory nervous system (SSNS) (Colloca et al., 2017; Murnion, 2018). NP may be connected with aberrant sensations, known as dysesthesia, or pain from usually non-painful stimuli, called allodynia. The SSNS plays a pivotal role in the transfer of noxious stimuli to the central nervous system (CNS) under normal circumstances (Myers and Bennett, 2008). Therefore, lesion of the SSNS leads to the innervations of nerve cells stopping and causes pain with or without a sensory hypersensitivity event in the painful region (Jensen and Baron, 2003). Furthermore, an injury in the SSNS could reveal itself as negative sensory symptoms or positive sensory symptoms. The positive sensory symptoms occur because of the regeneration as well as disinhibition of the nerve cells, whereas the negative sensory symptoms occur owing to the partial or complete loss of input to the nervous system (Shehla, 2019; von Hehn et al., 2012). Moreover, positive symptoms can be either spontaneous or stimulus-induced.
Paresthesia (i.e., aberrant sensations of the skin including tingling, chilling, numbness, burning, and pricking), spontaneous or shooting stimulus-independent pain, as well as electric shock-like sensations are involved in spontaneous positive symptoms; whereas, stimulus-induced positive symptoms of neuropathy include allodynic and hyperalgesia pain (Rasmussen et al., 2004; Beran, 2015). On the other hand, hypoesthesia (i.e., decreased sensations to non-painful stimuli), hypoalgesia (i.e., decreased sensations to toxic stimuli), pallhypesthesia (i.e., decreased sensations to vibration), and thermohypoesthesia (i.e., decreased sensations to cold/warm stimuli) are negative symptoms of NP (Jensen and Baron, 2003; Toh et al., 2018).
Many studies have revealed the prospective efficacy of phenytoin, mexiletine, dextromethorphan, tricyclic anti-depressants, gabapentin, tramadol, pregabalin, opioids, and lamotrigine for painful sensory neuropathy (Harden, 1999; Attal, 2001). Conversely, these treatments cause a 30–50% decline in pain and are frequently restricted owing to their noticeable adverse effects, with dominant sedative action. Nowadays, natural products like plant secondary metabolites are widely used to treat several chronic diseases due to their limited adverse effects as well as high efficacy (Uddin et al., 2018b, 2020e; Begum et al., 2019; Samsuzzaman et al., 2019; Thangapandiyan et al., 2019; Basu and Basu, 2020). Flavonoids are a broad group of secondary metabolites, extensively found in many fruits, vegetables, wine, cocoa, and tea (Chun et al., 2007; Egert and Rimbach, 2011). Flavonoids are recognized to have antioxidant, analgesic, and anti-inflammatory properties (Uddin and Upaganlawar, 2019). Moreover, these effects are associated with the suppression of nuclear factor kappa B (NF-κB)-dependent pro-inflammatory cytokines (Borghi et al., 2018), vascular endothelial growth factor, intercellular adhesion molecule 1, signal transducer and activator of transcription 3 (Verri et al., 2012), and activation of antioxidant transcription factor including nuclear factor erythroid 2-related factor 2 (Nrf2) (Borghi et al., 2018).
Numerous flavonoids have been demonstrated to be safe natural alternative treatments against neuropathic pain, oxidative stress, and neuroinflammatory diseases (Azevedo et al., 2013; Quintans et al., 2014; Anusha et al., 2017; Carballo-Villalobos et al., 2018; Ginwala et al., 2019). Hence, flavonoids are considered as multi-target drugs, which elucidate their wide range of actions. Here, we have reviewed the recent studies on the promising effects of flavonoids for the treatment of NP.
Mechanisms of Neuropathic Pain
Copious research in animal models have delivered some hint as to the pathophysiological mechanisms that produce NP, which involves both central and peripheral mechanisms (Baron, 2006; Campbell and Meyer, 2006; Gilron et al., 2006) as shown in Figure 1. Furthermore, the peripheral sensitization is performed through unmyelinated C- as well as finely myelinated Aδ-primary afferent neurons, which usually produce the sensation of pain in response to noxious stimuli. Conversely, the peripheral nerve injuries sensitize these neurons that develop a spontaneous activity. Moreover, these injuries result in significant alterations on the molecular and cellular levels activating the nerve cells (Baron, 2006).
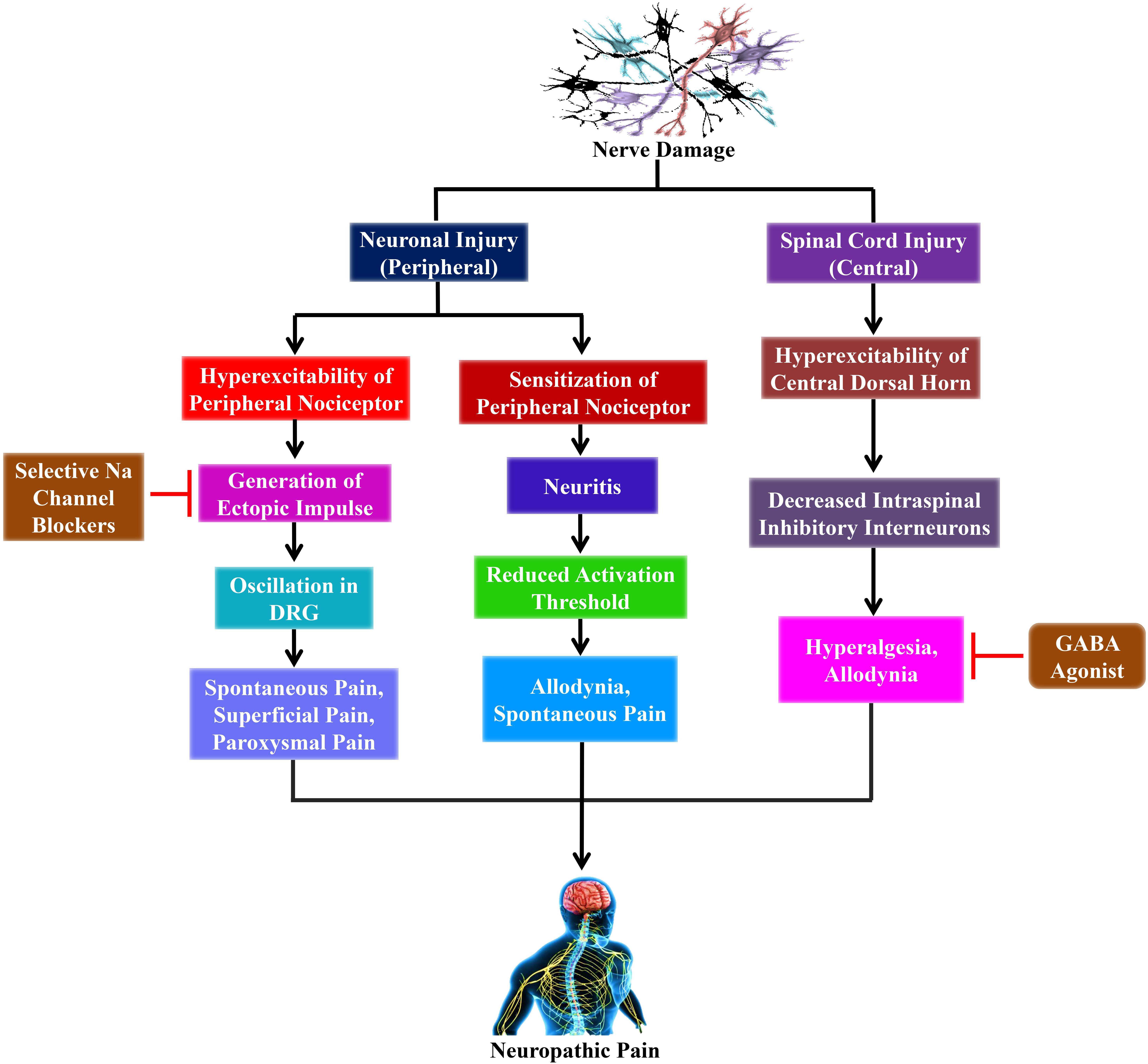
Figure 1. The outlines of the mechanism of neuropathic pain from nerve damage with probable clinical interventions. Nerve damage leads to peripheral nerve injury as well as spinal cord injury (central). The peripheral sensitization and hyperexcitability take place due to the peripheral nerve injury. Furthermore, the hyperexcitability of peripheral nociceptor leads to the generation of ectopic impulses, which plays a crucial role in producing spontaneous pain, superficial pain, and paroxysmal pain that ultimately leads to neuropathic pain. Conversely, selective sodium (Na) channel blockers such as lidocaine and carbamazepine inhibit the generation of ectopic impulses that reduces the sensation of neuropathic pain. On the other hand, the hyperexcitability of the central dorsal horn is caused by spinal cord injury that subsequently decreases intraspinal inhibitory interneurons, which finally leads to neuropathic pain. However, GABA agonists, including baclofen, inhibit the decreased intraspinal inhibitory interneurons that plays an essential role in reducing the sensation of neuropathic pain. DRG, dorsal root ganglion; Na, sodium.
Overexpression of messenger ribonucleic acid (mRNA) for voltage-gated sodium channels in the primary afferent neurons is accountable for ectopic spontaneous activity after nerve damage. This event might cause the clustering of these channels, which declines the action potential threshold, leading to hypersensitivity. Therefore, sodium channel blockers, including lidocaine, demonstrate pain relief action in NP through this mechanism (Lai et al., 2003).
Peripheral nerve injury is also responsible for the upregulation of various receptor proteins. These receptors are usually found at the membranes of the primary afferents and are partly expressed during physiological conditions. Vanilloid receptors, including the transient receptor potential cation channel subfamily V member 1 (TrpV1), play a crucial role in the sensing of toxic heat exceeding 43°C (Patapoutian et al., 2003), while transient receptor potential cation channel subfamily M (melastatin) member 8 (TRPM8) has been recognized as cold and menthol-sensitive which increase at temperature ranges from 8 to 28°C. Furthermore, the TRPM8 receptor is expressed in neurons that are small in diameter from the dorsal root ganglia (McKemy et al., 2002). Nerve injuries can cause the upregulation of this channel, contributing to peripheral sensitization of C-nociceptors, which results in cold hyperalgesia (Wasner, 2004).
In addition, acid-sensing ion channels are thought to take part in static mechanical hyperalgesia (Price et al., 2001). In contrast, both α1- and α2-adrenoceptors situated on the cutaneous afferent fibers play an essential role in hypersensitivity from nerve damage (Baron et al., 1999). Furthermore, adrenergic sensitivity has extensively been expressed in complex regional pain syndromes II, post-traumatic neuralgias, and postherpetic neuralgias; while there is no sensitivity in the primary afferent neurons, which have been claimed in the case of polyneuropathies (Schattschneider et al., 2006). Therefore, sympathetically-induced and temperature-mediated pain can be cured by inhibiting their relevant receptors on nociceptive neurons.
Ectopic activity is mediated by inflammation in both injured and contiguous typical primary afferent nociceptors, which are activated by nerve damage that generates proinflammatory cytokines, particularly tumor necrosis factor-α (TNF-α) (Sommer, 2003). Furthermore, deep proximal, as well as paroxysmal pains are noticeable symptoms in the case of patients who have peripheral neuropathies, including human immunodeficiency virus-neuropathy. Increased concentrations of proinflammatory cytokines and cyclooxygenase-2 (COX-2) have been found in the nerve biopsy specimens of these patients (Lindenlaub and Sommer, 2003).
CNS forms precise anatomical connections with the thalamus, brain stem, cortex, and spinal cord. Furthermore, these relations can connect the sensations that are produced in the high threshold primary afferents with the cortical areas of the CNS, which subsequently processes it into final painful sensations (Woolf, 2011). The constant hyperactivity is produced by damaged nerves that are considered to be a causative factor for central sensitization, as well as triggering activity-dependent synaptic flexibility occurring inside the cortex. Moreover, various factors are involved in central sensitization such as excitatory amino acid, changes in ion channel kinetics, different synaptic modulators, pre- and post-synaptic activation of kinases, and increased bulk of ionotropic receptors.
Most of the patients who have peripheral as well as central neuropathy demonstrate dominant synaptic facilitation leading to hypersensitivity and allodynia (Campbell and Meyer, 2006). Additionally, peripheral nerve damage results in pre-synaptic changes such as alterations in the synthesis of neuromodulators, neurotransmitters, and modifications in the calcium channels density (Hendrich et al., 2008). In contrast, post-synaptic changes take place due to the increased density of receptors on account of increased synthesis of ion channels and scaffold proteins and the phosphorylation of N-methyl-D-aspartate (NMDA) subunits (Cheng et al., 2008). These alterations also lead to aberrant expression of the mitogen-activated protein kinase system and Nav 1.3 (Hains et al., 2004; Ji and Woolf, 2001). Furthermore, pathologically sensitized C-fibers sensitize neuropeptide substance P as well as a spinal dorsal horn (SDH) through the release of glutamate, which cannot be neglected. Subsequently, glutamate demonstrates an excitatory action by acting upon the postsynaptic NMDA receptor contributing to central sensitization (Ultenius et al., 2006). It has been observed that the involvement of loss of function of tonic γ-amino butyric acid-A (GABAA)-conciliated inhibition and enhanced excitatory neurotransmitters are caused by an induction of central sensitization, leading to peripheral hypersensitivity, specifically hyperalgesia and allodynia (Knabl et al., 2008). When this sensitivity is developed, the generally harmless tactile stimuli can trigger Aβ as well as Aδ low threshold mechanoreceptors (Tal and Bennett, 1994).
GABA and Neuropathic Pain
The most abundant inhibitory neurotransmitter in the brain is GABA (Uddin et al., 2018a). GABA regulates diverse physiological functions such as anxiety, sleep, reward, and memory formation (Zeilhofer et al., 2009; Spiering, 2018; Uddin and Amran, 2019). GABA also regulates the excitatory action of neuronal cells of the CNS, assisting and maintaining the neural circuit’s homeostasis. Previously, it has been described that the role of inhibitory neurons, especially in SDH, act and monitor transmission of pain via the periphery to greater intensities of the brain (Melzack and Wall, 1965). After this, GABA was established to be the primary inhibitory neurotransmitters in the brain’s SDH (Yaksh, 1989).
GABA, releasing from presynaptic neurons, acts postsynaptically with several receptors; G protein-coupled channels, GABAA, GABAB, as well as GABAC, are ligand-gated ion channels (Gavande et al., 2011). Generally, ionotropic GABAA receptors are comprised of 5 heteropentameric subunits that form transmembrane protein complexes (Uddin and Rashid, 2020). Meanwhile, the α1β2γ2 subunit is thought to be the most dominant one in the human brain (Wafford, 2005). GABA initiation stimulates the membrane penetrability to chloride and carbonate ions that produce a net inner flow of anions as well as resulting in hyperpolarization. Therefore, this hyperpolarizing post-synaptic reaction is known as inhibitory post-synaptic potential (Semyanov et al., 2003).
Physiologically, GABA-liberating interneurons impose a robust inhibitory regulation through dorsal horn neuronal cells. Besides, damage of these neurons might additionally stimulate the dominant sensitization of the models of peripheral partial nerve injury. In rodents, injury caused the reduction of GABA release from the spine, with reduced GABA-producing glutamic acid decarboxylase (Moore et al., 2002). However, in diseased conditions, an improved excitation state arises that is recognized as an enormous GABAergic neuronal loss or deterioration of interneurons. Therefore, an imbalance of this condition could culminate into several neurological as well as psychiatric diseases such as Alzheimer’s disease, Parkinson’s disease, schizophrenia, epilepsy, NP, and the collective role of inhibitory and excitatory neurons show a dynamic role in regulating many brain activities (Tyson and Anderson, 2014).
It has been found that peripheral and central sensitization causes nerve injury and NP. GABAergic interneuronal loss is considered to be the main contributor to persistent pain states (Bráz et al., 2012). In the spinal cord, pharmacological inhibition of GABAergic neurotransmission causes hyperalgesia and allodynia (Gwak et al., 2006; Jergova et al., 2012). Likewise, GABAA receptor blockage could prompt a behavioral reaction, which was revealed by electrophysiological studies (Hwang and Yaksh, 1997). Furthermore, the GABAergic system impaired chronic NP in animals (Zeilhofer, 2008). As a result, spinal inhibitory neurotransmission may be appreciated as a pharmacological NP treatment.
Additionally, the crucial function of GABA in opioid-mediated antinociception has long been recognized (Ossipov et al., 2010). Also, agonists of GABAA receptor-mediated antinociceptive activity have been recognized to stimulate or inhibit additional neurotransmitters (McCarson and Enna, 2014). As a consequence, the agonists of the GABA receptor might play a dynamic role in considering chronic and acute pain (McCarson and Enna, 2014). Incidentally, isoguvacine and muscimol, agonists of GABAA receptors, are described to oppose nerve injury-stimulated tactile allodynia (Hwang and Yaksh, 1997). These receptors are strictly linked to huge diameter afferents involved in innocuous sensation (Price et al., 1984; Sivilotti and Woolf, 1994; Reeve et al., 1998; Ataka et al., 2000; Riley et al., 2001; Turner, 2003). Pharmacological as well as behavioral examinations have stated that a single or continuous intrathecal GABA response to spinal cord or GABA liberating cells reduce NP (Eaton et al., 1999a, b; Stubley et al., 2001; Malan et al., 2002). In addition, spinal GABAA receptors inhibition shows annoying peripheral nerve injury connected to hyperalgesia (Yamamoto and Yaksh, 1993).
In contrast, intrathecal administration of benzodiazepines (BDZs) and allosteric positive modulators of GABAA receptors have been extensively used in sleep complaints, convulsions, anxiety, and analgesic activity (Tucker et al., 2004). Even though it has analgesic properties, its usage in pain relief is limited due to sedation. Therefore, study is urgently needed in to GABAergic modulators which might play a prominent role in the attenuation of NP.
Flavonoids
Flavonoids are polyphenolic compounds found in fruits, flowers, barks, grains, vegetables, roots, tea, stems, and so on (Uddin et al., 2020a). Chemically, flavonoids are 15-carbon skeletons comprising of two benzene rings (A and B) linked via a heterocyclic pyrane ring (C) (Kumar and Pandey, 2013) as shown in Figure 2. Flavonoids can be divided into diverse subgroups according to the carbon of the C ring whereon the B ring is connected as well as the oxidation and degrees of unsaturation of the C ring (Panche et al., 2016).
In 1930, a novel constituent derived from an orange was believed to be a vitamin and called vitamin P. It was subsequently proved to be a flavonoid, rutin, that played an essential role in the isolation as well as the study of the mechanisms of several individual flavonoids. In fact, several traditional medicines are mainly flavonoids. In past centuries, Tanacetum parthenium has been used as a prophylactic drug in the treatment of migraine, while Matricaria recutita, chamomile flowers, has been used as a tranquilizer for many decades, with both comprising of the active constituent apigenin (Jäger et al., 2009). Moreover, linden flowers, Tilia sp. Tiliaceae, have been used as sedative agents, and Calluna vulgaris might serve as a nerve-calming medicine, which has active components of kaempferol and quercetin (Aguirre-Hernández et al., 2010). Apart from the separation of natural flavonoids, several synthetic and semi-synthetic products have been synthesized and separated for their therapeutic potential (Cushnie and Lamb, 2005). Up to now, 6000 diverse flavonoids have been isolated. Flavonoid compounds show different biological effects, such as neuroprotective (Cho et al., 2013; Uddin et al., 2016; Uddin and Kabir, 2019; Zaplatic et al., 2019), antifungal (Ammar et al., 2013), antimicrobial (Cushnie and Lamb, 2005; Górniak et al., 2019), anticancer (Liu et al., 2010; Abotaleb et al., 2019), anti-inflammatory (Wang et al., 2010; Begum et al., 2019), anxiolytic (Ognibene et al., 2008), antioxidant (Heim et al., 2002; Uddin et al., 2017), antiviral (Orhan et al., 2010; Dai et al., 2019), cardioprotective (Yu et al., 2005; Mahmoud et al., 2019), and antinociceptive activities (Wang et al., 2014; Hossain et al., 2017a, b).
Role of Flavonoid on Ionotropic GABAA Receptors
Flavonoids are widely targeted for their peripheral events; though, their selective affinity for GABAA receptors has extensively been demonstrated in studies using bovine and rat brain membrane binding analyses (Hong and Hopfinger, 2003). Numerous behavioral tests have also widely been performed, which confirm the sedative effects of flavonoids in an animal model of anxiety that was devoid of the additional side effects of BDZs (Griebel et al., 1999). Remarkably, negative, positive, and neutral allosteric modulatory flavonoid actions of an extensive variety of ionotropic GABA receptors have been focused on and intensely supported through enormous evidence. In the 1990s, flavonoids had been well-defined as a novel family of BDZ receptor ligands (Medina et al., 1997; Marder and Paladini, 2002). Typically, they were believed to be acting upon BDZ receptors, as well as many synthetic flavonoids having a remarkable affinity for BDZ binding site (Yao et al., 2007), until they were claimed to be insensitive to the BDZ receptor antagonist, flumazenil, therefore focusing a distinctive site of action (Hanrahan et al., 2011).
It has been found that the replacement at 6- or 3′-positions of flavones with an electronegative functional group improved the affinity toward the receptors of BDZ (Paladini et al., 1999). Moreover, GABA ratios were measured by the impact of ligand binding on the GABA binding site. These ratios displayed that flavones showed substantial biological actions at BDZ receptors (Hanrahan et al., 2011). 6-Bromo-3′-nitroflavone, 6-chloro-3′-nitroflavone, and 6-bromoflavone with a GABA ratio of 1.38, 2.0, and 1.6–2.0 were demonstrated as a partial agonist, an antagonist, and full agonist at these receptors respectively (Marder et al., 1996; Wolfman et al., 1998; Viola et al., 2000).
GABAA receptors were enhanced by the flux of chloride ion that deliver a robust inhibitory effect via positive ionotropic modulators. As a result, these modulators are the strongest candidates for the management of CNS-associated diseases such as generalized anxiety, seizure disorders, sleep disturbances, panic disorders, muscle spasms, and NP (Rudolph and Möhler, 2006). Furthermore, flavonoids might act upon a new binding site, excluding the classical BDZ binding site, which plays a pivotal role in searching for novel therapeutic candidates with limited adverse effects (Rudolph and Möhler, 2006). Incidentally, 6-methoxyflavonone has been described to act as a positive allosteric modulator at α1β2γ2L and α2β2γ2L subunits of GABAA receptors (Hall et al., 2014).
The substitution at 6-position on flavones is linked to its role in recombinant GABAA receptors. 6-Hydroxyflavone showed a remarkable effect at the flumazenil-sensitive BDZ site (Ren et al., 2010). Furthermore, 6-methoxyflavone and 6-methoxyflavanone have been claimed to display anti-allodynic effects in cisplatin- and streptozotocin-stimulated NP models (Akbar et al., 2016; Shahid et al., 2017). Therefore, these defensive properties against NP have been recognized to cause allosteric positive modulatory effects on opioid and GABAA receptors (Akbar et al., 2016).
Additionally, myrcitin and baicalin exerted antiallodynic effects in sciatic nerve ligation models (Cherng et al., 2014; Meotti et al., 2006). Besides, quercetin and rutin have widely been claimed to suppress oxaliplatin-mediated chronic peripheral neuropathic pain (Azevedo et al., 2013). Meanwhile, the antiallodynic potential of streptozotocin-induced painful diabetic neuropathy has been reported by naringin (Kandhare et al., 2012).
Role of Flavonoids in Different Neuropathic Pain Models
Effect of Flavonoids on Diabetic Neuropathy
NP is arduous to treat properly and is related to the remarkable impairment of health conditions as well as economic problems (O’Connor, 2009; Langley et al., 2013). Diabetic neuropathy is one of the most common causes of neuropathy and affects about 382 million people in the world (Boulton et al., 1998). Furthermore, genistein (Valsecchi et al., 2011), luteolin (Li et al., 2015), catechin (Addepalli and Suryavanshi, 2018), rutin (Tian et al., 2016), and pelargonidin (Mirshekar et al., 2010) have been revealed to decrease the levels of malondialdehyde (MDA) in animal models of diabetes. Moreover, MDA serves as a key biomarker for lipid damage as well as oxidative stress that can be caused by free radicals. In diabetic patients, the increased level of MDA has widely been observed in the serum as well as other tissues, which significantly affects the peripheral nerves (Feldman et al., 1994; Perkins et al., 2001). Some flavonoids, such as genistein (Valsecchi et al., 2011), naringenin (Al-Rejaie et al., 2015), luteolin (Li et al., 2015), hesperidin, catechin (Addepalli and Suryavanshi, 2018), kaempferol (Kishore et al., 2018), fisetin (Zhao et al., 2015), rutin (Tian et al., 2016), and morin (Bachewal et al., 2018) have been shown to reduce the ROS level by increasing the level of diverse antioxidative enzymes including glutathione peroxidase, reduced glutathione peroxidase, superoxide dismutase, glutathione reductase, and catalase in various tissues such as the liver, sciatic nerve, and brain of diabetic animals (Table 1). In the diabetic animal model, rutin, luteolin, and morin have been demonstrated to raise the expression of Nrf2 as well as its downstream effector’s heme oxygenase-1 (HO-1) in nerve tissues. Numerous studies have found that Nrf-2/HO-1 could fight against oxidative stress-mediated neuroinflammation and nerve damage in diabetic animal models (Cardozo et al., 2013; Agca et al., 2014; Kumar and Mittal, 2017). Moreover, kaempferol decreased advanced glycation end products and epigallocatechin gallate (EGCG) causes a reduction of 8-hydroxy-2-deoxyguanosine, which is considered as the major form of free radical-mediated oxidative stress in the nucleus and mitochondria (Valavanidis et al., 2009). It has also been found that in the diabetic animal model, genistein and naringenin raised nerve growth factor (NGF) in sciatic nerves (Basu and Basu, 2020). Therefore, NGF servesas the survival and life maintenance of the neurons.
Diabetic neuropathy in animal models has widely been marked by evaluating behavioral signs, such as chemical, mechanical, thermal hyperalgesia, and tactile allodynia (Pittenger et al., 2005). It has also been observed that flavonoids considerably downregulated thermal, mechanical, chemical hyperalgesia, and tactile allodynia in diabetic animal models (Figure 3). A number of flavonoids including fisetin (Zhao et al., 2015), baicalin (Li et al., 2018), naringenin (Al-Rejaie et al., 2015), pelargonidin (Mirshekar et al., 2010), rutin (Tian et al., 2016), naringin (Kandhare et al., 2012), hesperidin (Visnagri et al., 2014), and luteolin (Li et al., 2015) reduced diabetes-mediated thermal hyperalgesia, although kaempferol (Kishore et al., 2018), EGCG (Raposo et al., 2015), rutin (Tian et al., 2016), naringenin (Al-Rejaie et al., 2015), luteolin (Li et al., 2015), morin (Bachewal et al., 2018), and naringin (Kandhare et al., 2012) attenuated mechanical hyperalgesia (Figure 3). Furthermore, fisetin (Zhao et al., 2015), baicalein (Li et al., 2018), hesperidin (Visnagri et al., 2014), morin (Bachewal et al., 2018), and puerarin (Liu et al., 2014) ameliorated mechanical allodynia, while naringin (Kandhare et al., 2012) and genistein (Valsecchi et al., 2011) decreased mechano-tactile allodynia, and rutin (Tian et al., 2016) and luteolin (Li et al., 2015) improved cold allodynia. Numerous investigations have demonstrated that short term diabetes mediated mechanical, chemical, and thermal hyperalgesia (Dyck et al., 2000; Freshwater and Calcutt, 2005), however chronic diabetes induces mechanical and thermal hypoalgesia (Calcutt et al., 2004). Besides, baicalein attenuated thermal hypoalgesia (Stavniichuk et al., 2011).
Effect of Flavonoids on Chemotherapy-Induced Peripheral Neuropathy
The use of diverse chemotherapeutic agents and other anticancer drugs leads to the impairment of the peripheral nerves. Chemotherapy-induced peripheral neuropathy (CIPN) is another form of neuropathy caused by anticancer drugs (Hershman et al., 2014). Platinum compounds are extensively used in the management of several solid tumors. Oxaliplatin, a third-generation platinum agent, plays a pivotal role in diminishing antitumoral resistance with noticeable cytotoxicity (Argyriou et al., 2008; Stein and Arnold, 2012). It has been observed that (Azevedo et al., 2013) rutin and quercetin suppressed oxaliplatin-mediated mechanical as well as cold nociceptive thresholds. In a study by Schwingel et al. (2014) it was demonstrated that rutin and nanoemulsion of quercetin ameliorated oxaliplatin-mediated mechanical allodynia. According to the study by Shahid et al. (2017) 6-methoxyflavone showed antinociceptive activity in a rat model of CIPN (Table 1). Hence, 6-methoxyflavone considerably reduced cisplatin-mediated mechanical allodynia by raising the paw withdrawal threshold as well as thermal hypoalgesia (Figure 3) by improving the paw thermal threshold.
Effect of Flavonoids on Sciatic Nerve Chronic Constriction Injury
Chronic constriction injury (CCI) is considered to be the most extensively studied model for chronic neuropathic pain. There are various symptoms of CCI-induced pain including hyperalgesia, allodynia, paraesthesia, dysesthesia, and spontaneous pain (Austin et al., 2012). Flavonoids including hesperidin (Carballo-Villalobos et al., 2016), diosmin (Bertozzi et al., 2017), and grape seed proanthocyanidins (Kaur et al., 2016) decreased both mechanical and thermal hyperalgesia (Figure 3). In contrast, other flavonoids including genistein (Valsecchi et al., 2008), EGCG (Kuang et al., 2012), EGCG-derived compounds (Xifró et al., 2015), morin (Komirishetty et al., 2016), and isoorientin (Zhang et al., 2019) decreased only thermal hyperalgesia (Table 1). As compared to morphine and gabapentin, quercetin decreased the mechanical and thermal hypersensitivities to a greater extent (Çivi et al., 2016). When quercetin was administered in a pre-injury condition, it exerted long term actions on mechanical hypersensitivity, which further suggests the antinociceptive properties of quercetin in the CCI model (Çivi et al., 2016). Additionally, flavonoids including genistein (Valsecchi et al., 2008), EGCG (Kuang et al., 2012), puerarin (Liu et al., 2014), morin (Komirishetty et al., 2016), and isoorientin (Zhang et al., 2019) decreased CCI-mediated mechanical allodynia. On the other hand, cold allodynia was reduced by morin (Komirishetty et al., 2016) and isoorientin (Zhang et al., 2019). Although mechanical and cold hyperalgesia was reduced by luteolin, it did not affect thermal hyperalgesia (Hara et al., 2014). However, thermal hyperalgesia was decreased by fisetin, but did not affect the nociceptive sensitivity to mechanical stimuli (Zhao et al., 2015).
An elevated level of nitro oxidative stress can cause DNA damage, which can cause the activation of poly-ADP ribose polymerase (PARP) (Figure 4), which can further lead to PARP-induced DNA repair by transferring ADP-ribose units to the nuclear proteins. Nevertheless, activation of PARP can cause NF-κB activation, which can subsequently activate various inflammatory markers including interleukin (IL)-6, TNF-α, inducible nitric oxide synthase (iNOS), and cyclooxygenase-2 (COX-2) (Obrosova et al., 2004; Sommer and Kress, 2004) that lead to neuroinflammation (Figure 4). Studies involving the CCI-induced neuropathic pain model revealed that flavonoids exert effects on various pro-inflammatory biomarkers (Bertozzi et al., 2017; Figure 3). A single administration of diosmin decreased the levels of mRNA expressions of IL-33/ST2 and IL-1β, while chronic administration decreased the mRNA expression level of TNF-α along with ST2, IL-33, and IL-1β. Furthermore, a single administration also decreased the expression levels of oligodendrocytes and microglia, whereas chronic treatment decreased astrocytes together with oligodendrocytes and microglia (Bertozzi et al., 2017). Puerarin decreased the elevated immunoreactivity of glial fibrillary acidic protein and ionized calcium-binding adaptor protein-1, which are astroglia and microglial activation markers, successively (Liu et al., 2014). In the CCI-induced neuropathic pain model, morin decreased various inflammatory biomarkers including IL-6, TNF-α, phospho-NF-κB, NF-κB, COX-2, iNOS, and PARP (Komirishetty et al., 2016). Deoxyribonucleic acid (DNA) damage was found to be increased due to the CCI-induced nerve injury, which resulted in PARP overactivation (Jagtap and Szabo, 2005). It was found that overactivation of PARP caused bioenergetic failure because overactivity of PARP requires a high amount of nicotinamide adenine dinucleotide (NAD) during DNA repair, and finally, NAD synthesis also requires adenosine triphosphate (ATP), which can eventually lead to the disruption of biochemical processes that are dependent on ATP (Hyo and Snyder, 1999).
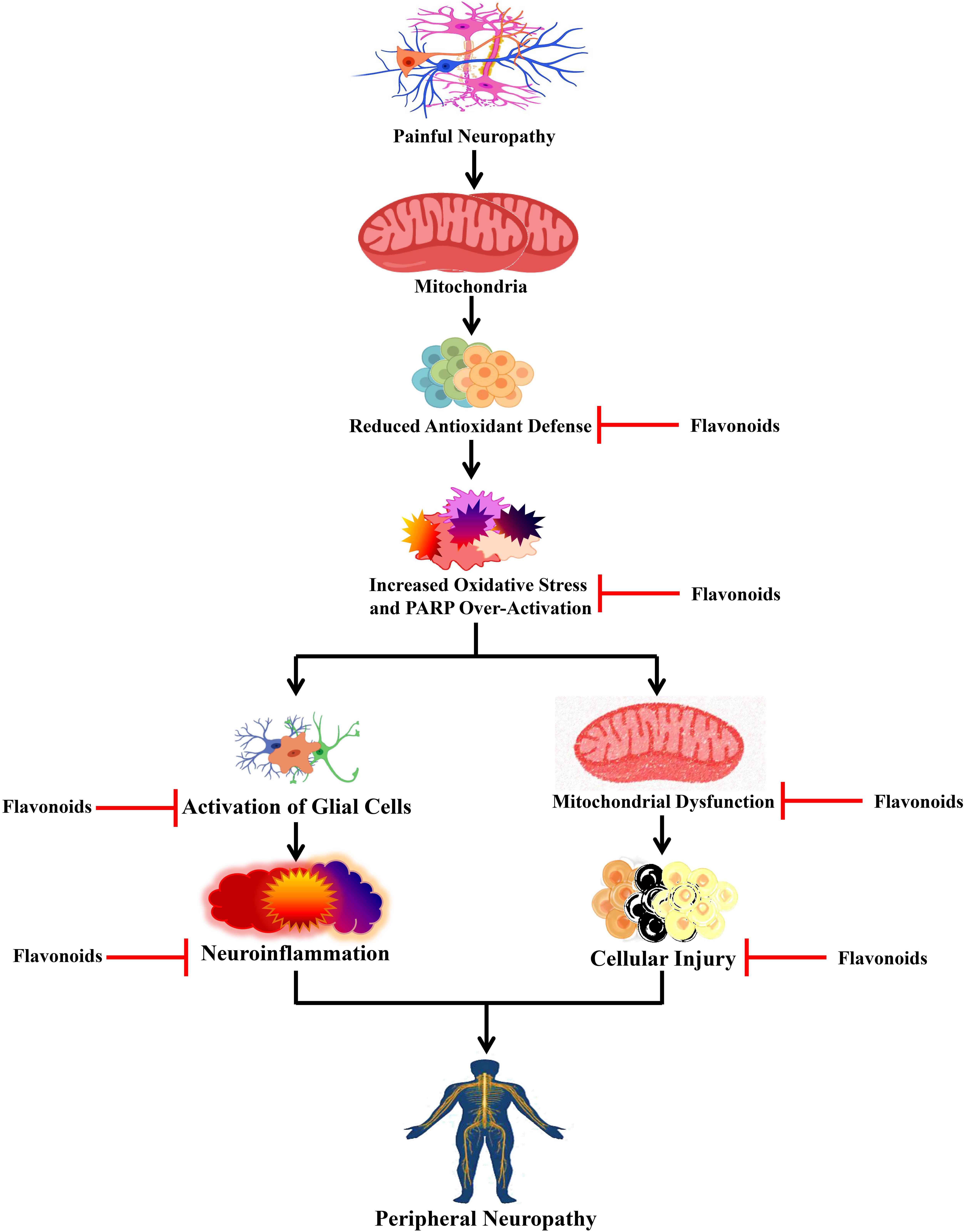
Figure 4. Effects of flavonoids on peripheral neuropathy. Flavonoids act on different peripheral neuropathic pain conditions by blocking oxidative stress, activation of glial cells, and mitochondrial dysfunction. PARP, poly-ADP ribose polymerase.
Treatment with morin caused marked restoration of CCI-mediated reduction in the ATP levels and also restored the neuronal cells from the bioenergetic crisis (Komirishetty et al., 2016). In a study, Kuang et al. (2012) revealed that treatment with EGCG reduced the mRNA and protein expressions of the toll-like receptor (TLR4) and its endogenous ligand HMGB1. It is known that TLR4 is a pattern recognition receptor and plays roles in the immune system and inflammatory diseases. When endogenous ligands bind with TLR4, it gets activated and stimulates the generation of pro-inflammatory cytokines by causing NF-κB activation (Janeway and Medzhitov, 2002; Akira et al., 2006). Furthermore, EGCG elevated the level of IL-10, reduced the downstream pro-inflammatory cytokines (i.e., TNF-α and IL-1β) of the TLR4 signaling pathway, and reduced the expression of NF-κB in the lumbar SDH of CCI rats (Kuang et al., 2012). In the dorsal horn of the spinal cord, an EGCG-derived compound decreased the levels of mRNA and protein expressions of IL-6, NF-κB, IL-1β, and TNF-α (Xifró et al., 2015). Administration of isoorientin and puerarin also decreased the level of CCI-induced pro-inflammatory cytokines including IL-6, IL-1β, and TNF-α (Liu et al., 2014; Zhang et al., 2019). Interestingly, genistein reduced the level of IL-1β expression in the spinal cord and dorsal root ganglion, while genistein also decreased mRNA expressions of both IL-6 and IL-1β in the sciatic nerve (Valsecchi et al., 2008).
Effect of Flavonoids on Other Neuropathic Pain Signaling Pathways
Flavonoids show anti-inflammatory as well as antioxidant effects due to their action on GABAA receptors (Hanrahan et al., 2011). Maximum metabolic disorders are the result of oxidative stress. Along with exogenous factors, regular metabolism of oxygen inside the tissues and cells produce reactive oxygen species (ROS) and free radicals that steadily endanger them (Forrester et al., 2018; Uddin et al., 2019). Flavonoids are well-recognized for their antioxidant properties and are also confirmed to show beneficial effects in several chronic diseases, including neurodegenerative disease, diabetes, atherosclerosis, and cancer (de Teles et al., 2018; Kozłowska and Szostak-Węgierek, 2019; Uddin and Kabir, 2019; Uddin et al., 2020c, d). Moreover, certain flavonoids play a crucial role in the iron chelation thus stopping the development of free radicals (Nelson et al., 1992; Ferrali et al., 1997). Rutin and epicatechin are shown to have the capability to be oxidized themselves through free radicals, producing a less reactive and stable species (Hanasaki et al., 1994). Correspondingly, quercetin, a plant pigment flavonoid, prevents nitric oxide (NO)-mediated cell injury. A combination of NO and free radicals generates the enormously injurious peroxynitrite, which directly oxidizes low-density lipoprotein and plays a crucial role in the permanent damage of the cell membrane. Therefore, free radicals are scavenged by quercetin and restrained from reacting with NO, whereas, silibin reacts directly with NO (Dehmlow et al., 1996; Shutenko et al., 1999). Mechanical allodynia induced by spinal nerve ligation (SNL) was found to be decreased by various flavonoids including myricetin (Hagenacker et al., 2010), EGCG (Choi et al., 2012), and baicalein (Cherng et al., 2014). SNL-induced thermal hyperalgesia was reduced by myricetin (Hagenacker et al., 2010) and baicalein (Cherng et al., 2014), while quercetin decreased both cold and thermal hyperalgesia in SNL rats (Ji et al., 2017). In addition to this, hesperetin and quercetin decreased partial sciatic nerve ligation-stimulated neuropathic pain and spared nerve injury (Figure 3; Aswar et al., 2014; Muto et al., 2018).
Physiologically, xanthine dehydrogenase plays an important role in the metabolism of xanthine to uric acid, however, this enzyme alters into xanthine oxidase in the case of ischemic-reperfusion, which works as a precursor of free radicals. There are various flavonoids, such as quercetin, silibinin, and luteolin, that are recognized to work as antioxidants through stopping xanthine oxidase (Chang et al., 1993; Shoskes, 1998). Similarly, reperfusion is also caused by the mobilization of leucocytes producing the subsequent release of inflammatory mediators as well as cytotoxic oxidants, which provokes the complement system. Many flavonoids play a key role in the immobilization of leucocytes, eventually resulting in a decline in the serum complement system as well as inflammation (Friesenecker et al., 1995; Ferrándiz et al., 1996). It has been observed that the connection of the same pathophysiological mechanisms takes place with both NP of peripheral origin and inflammation. Both kinds of pathologies express as hyperalgesia and allodynia (Clatworthy et al., 1995; DeLeo and Yezierski, 2001; Jin et al., 2003). Moreover, inflammatory cells infiltration and their main secretory products, including cytokines and arachidonic acid, affect peripheral nerve damage, which is accountable for the production and maintenance of the constant pain (Tracey and Walker, 1995; Cui et al., 2000; Ma and Eisenach, 2003). When cytokines such as IL-1, IL-6, and TNF-α were injected into a rat paw, it would result in the initiation of thermal and mechanical hyperalgesia (Cunha et al., 1992; Ferreira et al., 1993). On the other hand, the inhibition of TNF-α in the animal models with painful neuropathy led to the reduction of hyperalgesia (Sommer et al., 1998). The release of cytokines also activates COX-2 dependent prostanoid releases. Furthermore, prostaglandins (PGs) also play a pivotal role in triggering inflammation that increases sensitivity to pain (Uddin et al., 2020b). It had been found that intrathecal injection of PGs such as PGE2 and PGF2α triggered allodynia in conscious mice (Minami et al., 1992, 1994), while intrathecal administration of PGD2 and PGE2 led to the initiation of hyperalgesia (Uda et al., 1990). Additionally, synthesis of NO and PG through COX-2 as well as iNOS is increased in the microglia on account of peripheral nerve damage, leading to hypersensitization (Hanisch, 2002). It is evident that flavonoids show anti-inflammatory activity both in vitro and in vivo. One of the imperative mechanisms of anti-inflammatory action is recognized by inhibiting eicosanoid producing enzymes such as phospholipase A2, lipoxygenases, and COX (Kim et al., 2004). Along with anti-inflammatory activity, flavonoids also block arachidonic acid metabolism (Ferrándiz and Alcaraz, 1991).
Conclusion
In this review, we discuss the effects of flavonoids in improving different NP conditions and how flavonoids control diverse pain biomarkers in animal models of NP. Allosteric modulators at GABAA receptors can alter either the affinity or efficacy of agonists including GABA, subsequently controlling their activity. Flavonoids are strong allosteric modulators and may serve as valuable candidates in the management of NP. Hence, it can be said that there is huge potentiality in flavonoids for the development of novel therapeutics agents for NP, however, further studies are needed.
Author Contributions
MU conceived the original idea and designed the outlines of the study. MU, AM, MR, and MK wrote the draft of the manuscript. MU and AM prepared the figures for the manuscript. SA, IA, AP, GA, MB-J, and MA-D revised and improved the draft. All authors have read and approved the final manuscript.
Conflict of Interest
The authors declare that the research was conducted in the absence of any commercial or financial relationships that could be construed as a potential conflict of interest.
Acknowledgments
This work was funded by the Deanship of Scientific Research at Princess Nourah bint Abdulrahman University, through the Fast-track Research Funding Program.
Abbreviations
BDZ, benzodiazepines; CNS, central nervous system; CIPN, chemotherapy-induced peripheral neuropathy; CCI, chronic constriction injury; EGCG, epigallocatechin gallate; GABA, γ -amino butyric acid; MDA, malondialdehyde; NMDA, N-methyl -D-aspartate; NP, neuropathic pain; NF- κ B, nuclear factor kappa B; Nrf2, nuclear factor erythroid 2-related factor 2; SDH, spinal dorsal horn; SNL, spinal nerve ligation; SSNS, somatosensory nervous system.
References
Abotaleb, M., Samuel, S. M., Varghese, E., Varghese, S., Kubatka, P., Liskova, A., et al. (2019). Flavonoids in cancer and apoptosis. Cancers 11:28. doi: 10.3390/cancers11010028
Addepalli, V., and Suryavanshi, S. V. (2018). Catechin attenuates diabetic autonomic neuropathy in streptozotocin induced diabetic rats. Biomed. Pharmacother. 108, 1517–1523. doi: 10.1016/j.biopha.2018.09.179
Agca, C. A., Tuzcu, M., Hayirli, A., and Sahin, K. (2014). Taurine ameliorates neuropathy via regulating NF-κB and Nrf2/HO-1 signaling cascades in diabetic rats. Food Chem. Toxicol. 71, 116–121. doi: 10.1016/j.fct.2014.05.023
Aguirre-Hernández, E., González-Trujano, M. E., Martínez, A. L., Moreno, J., Kite, G., Terrazas, T., et al. (2010). HPLC/MS analysis and anxiolytic-like effect of quercetin and kaempferol flavonoids from Tilia americana var. mexicana. J. Ethnopharmacol. 127, 91–97. doi: 10.1016/j.jep.2009.09.044
Akbar, S., Subhan, F., Karim, N., Shahid, M., Ahmad, N., Ali, G., et al. (2016). 6-Methoxyflavanone attenuates mechanical allodynia and vulvodynia in the streptozotocin-induced diabetic neuropathic pain. Biomed. Pharmacother. 84, 962–971. doi: 10.1016/j.biopha.2016.10.017
Akira, S., Uematsu, S., and Takeuchi, O. (2006). Pathogen recognition and innate immunity. Cell 124, 783–801. doi: 10.1016/j.cell.2006.02.015
Al-Rejaie, S. S., Aleisa, A. M., Abuohashish, H. M., Parmar, M. Y., Ola, M. S., Al-Hosaini, A. A., et al. (2015). Naringenin neutralises oxidative stress and nerve growth factor discrepancy in experimental diabetic neuropathy. Neurol. Res. 37, 924–933. doi: 10.1179/1743132815Y.0000000079
Ammar, M. I., Nenaah, G. E., and Mohamed, A. H. H. (2013). Antifungal activity of prenylated flavonoids isolated from Tephrosia apollinea L. against four phytopathogenic fungi. Crop Prot. 49, 21–25. doi: 10.1016/j.cropro.2013.02.012
Anjaneyulu, M., and Chopra, K. (2003). Quercetin, a bioflavonoid, attenuates thermal hyperalgesia in a mouse model of diabetic neuropathic pain. Prog. Neuro Psychopharm. Biol. Psychiatry 27, 1001–1005. doi: 10.1016/S0278-5846(03)00160-X
Anjaneyulu, M., and Chopra, K. (2004). Quercetin attenuates thermal hyperalgesia and cold allodynia in STZ-induced diabetic rats. Indian J. Exp. Biol. 42, 766–769.
Anusha, C., Sumathi, T., and Joseph, L. D. (2017). Protective role of apigenin on rotenone induced rat model of Parkinson’s disease: suppression of neuroinflammation and oxidative stress mediated apoptosis. Chem. Biol. Interact. 269, 67–79. doi: 10.1016/j.cbi.2017.03.016
Argyriou, A. A., Polychronopoulos, P., Iconomou, G., Chroni, E., and Kalofonos, H. P. (2008). A review on oxaliplatin-induced peripheral nerve damage. Cancer Treat. Rev. 34, 368–377. doi: 10.1016/j.ctrv.2008.01.003
Aswar, M., Kute, P., Mahajan, S., Mahajan, U., Nerurkar, G., and Aswar, U. (2014). Protective effect of hesperetin in rat model of partial sciatic nerve ligation induced painful neuropathic pain: an evidence of anti-inflammatory and anti-oxidative activity. Pharmacol. Biochem. Behav. 124, 101–107. doi: 10.1016/j.pbb.2014.05.013
Ataka, T., Kumamoto, E., Shimoji, K., and Yoshimura, M. (2000). Baclofen inhibits more effectively C-afferent than Aδ-afferent glutamatergic transmission in substantia gelatinosa neurons of adult rat spinal cord slices. Pain 86, 273–282. doi: 10.1016/S0304-3959(00)00255-4
Austin, P. J., Wu, A., and Moalem-Taylor, G. (2012). Chronic constriction of the sciatic nerve and pain hypersensitivity testing in rats. J. Vis. Exp. 61:3393. doi: 10.3791/3393
Azevedo, M. I., Pereira, A. F., Nogueira, R. B., Rolim, F. E., Brito, G. A. C., Wong, D. V. T., et al. (2013). The antioxidant effects of the flavonoids rutin and quercetin inhibit oxaliplatin-induced chronic painful peripheral neuropathy. Mol. Pain 9:53. doi: 10.1186/1744-8069-9-53
Bachewal, P., Gundu, C., Yerra, V. G., Kalvala, A. K., Areti, A., and Kumar, A. (2018). Morin exerts neuroprotection via attenuation of ROS induced oxidative damage and neuroinflammation in experimental diabetic neuropathy. Biofactors 44, 109–122. doi: 10.1002/biof.1397
Baron, R. (2006). Mechanisms of disease: neuropathic pain - A clinical perspective. Nat. Clin. Pract. Neurol. 2, 95–106. doi: 10.1038/ncpneuro0113
Baron, R., Levine, J. D., and Fields, H. L. (1999). Causalgia and reflex sympathetic dystrophy: does the sympathetic nervous system contribute to the generation of pain? Muscle Nerve 22, 678–695. doi: 10.1002/(SICI)1097-4598(199906)22:6<678:AID-MUS4<3.0.CO;2-P
Basu, P., and Basu, A. (2020). In vitro and in vivo effects of flavonoids on peripheral neuropathic pain. Molecules 25:1171. doi: 10.3390/molecules25051171
Begum, M. M., Islam, A., Begum, R., Uddin, M. S., Rahman, M. S., Alam, S., et al. (2019). Ethnopharmacological inspections of organic extract of Oroxylum indicum in rat models: a promising natural gift. Evidence-based complement. Altern. Med. 2019, 1–13. doi: 10.1155/2019/1562038
Bertozzi, M. M., Rossaneis, A. C., Fattori, V., Longhi-Balbinot, D. T., Freitas, A., Cunha, F. Q., et al. (2017). Diosmin reduces chronic constriction injury-induced neuropathic pain in mice. Chem. Biol. Interact. 273, 180–189. doi: 10.1016/j.cbi.2017.06.014
Borghi, S. M., Mizokami, S. S., Pinho-Ribeiro, F. A., Fattori, V., Crespigio, J., Clemente-Napimoga, J. T., et al. (2018). The flavonoid quercetin inhibits titanium dioxide (TiO 2)-induced chronic arthritis in mice. J. Nutr. Biochem. 53, 81–95. doi: 10.1016/j.jnutbio.2017.10.010
Boulton, A. J. M., Gries, F. A., and Jervell, J. A. (1998). Guidelines for the diagnosis and outpatient management of diabetic peripheral neuropathy. Diabet. Med. 15, 508–514. doi: 10.1002/(SICI)1096-9136(199806)15:6<508:AID-DIA613<3.0.CO;2-L
Bráz, J. M., Sharif-Naeini, R., Vogt, D., Kriegstein, A., Alvarez-Buylla, A., Rubenstein, J. L., et al. (2012). Forebrain GABAergic neuron precursors integrate into adult spinal cord and reduce injury-induced neuropathic pain. Neuron 74, 663–675. doi: 10.1016/j.neuron.2012.02.033
Calcutt, N. A., Freshwater, J. D., and Mizisin, A. P. (2004). Prevention of sensory disorders in diabetic Sprague-Dawley rats by aldose reductase inhibition or treatment with ciliary neurotrophic factor. Diabetologia 47, 718–724. doi: 10.1007/s00125-004-1354-2
Campbell, J. N., and Meyer, R. A. (2006). Mechanisms of neuropathic pain. Neuron 52, 77–92. doi: 10.1016/j.neuron.2006.09.021
Carballo-Villalobos, A. I., González-Trujano, M. E., Pellicer, F., Alvarado-Vásquez, N., and López-Muñoz, F. J. (2018). Central and peripheral anti-hyperalgesic effects of diosmin in a neuropathic pain model in rats. Biomed. Pharmacother. 97, 310–320. doi: 10.1016/j.biopha.2017.10.077
Carballo-Villalobos, A. I., González-Trujano, M.-E., Pellicer, F., and López-Muñoz, F. J. (2016). Antihyperalgesic effect of Hesperidin improves with diosmin in experimental neuropathic pain. Biomed Res. Int. 2016:8263463. doi: 10.1155/2016/8263463
Cardozo, L. F. M. F., Pedruzzi, L. M., Stenvinkel, P., Stockler-Pinto, M. B., Daleprane, J. B., Leite, M., et al. (2013). Nutritional strategies to modulate inflammation and oxidative stress pathways via activation of the master antioxidant switch Nrf2. Biochimie 95, 1525–1533. doi: 10.1016/j.biochi.2013.04.012
Chang, W. S., Lee, Y. J., Lu, F. J., and Chiang, H. C. (1993). Inhibitory effects of flavonoids on xanthine oxidase. Anticancer. Res. 13, 2165–2170.
Cheng, H. T., Suzuki, M., Hegarty, D. M., Xu, Q., Weyerbacher, A. R., South, S. M., et al. (2008). Inflammatory pain-induced signaling events following a conditional deletion of the N-methyl-d-aspartate receptor in spinal cord dorsal horn. Neuroscience 155, 948–958. doi: 10.1016/j.neuroscience.2008.06.024
Cherng, C. H., Lee, K. C., Chien, C. C., Chou, K. Y., Cheng, Y. C., Hsin, S. T., et al. (2014). Baicalin ameliorates neuropathic pain by suppressing HDAC1 expression in the spinal cord of spinal nerve ligation rats. J. Formos. Med. Assoc. 113, 513–520. doi: 10.1016/j.jfma.2013.04.007
Cho, N., Lee, K. Y., Huh, J., Choi, J. H., Yang, H., Jeong, E. J., et al. (2013). Cognitive-enhancing effects of Rhus verniciflua bark extract and its active flavonoids with neuroprotective and anti-inflammatory activities. Food Chem. Toxicol. 58, 355–361. doi: 10.1016/j.fct.2013.05.007
Choi, J. II, Kim, W. M., Lee, H. G., Kim, Y. O., and Yoon, M. H. (2012). Role of neuronal nitric oxide synthase in the antiallodynic effects of intrathecal EGCG in a neuropathic pain rat model. Neurosci. Lett. 510, 53–57. doi: 10.1016/j.neulet.2011.12.070
Chtourou, Y., Gargouri, B., Kebieche, M., and Fetoui, H. (2015). Naringin abrogates cisplatin-induced cognitive deficits and cholinergic dysfunction through the down-regulation of AChE expression and iNOS signaling pathways in hippocampus of aged rats. J. Mol. Neurosci. 56, 349–362. doi: 10.1007/s12031-015-0547-0
Chun, O. K., Chung, S. J., and Song, W. O. (2007). Estimated Dietary Flavonoid Intake and Major Food Sources of U.S. Adults. J. Nutr. 137, 1244–1252. doi: 10.1093/jn/137.5.1244
Çivi, S., Emmez, G., Dere, ÜA., Börcek, A. Ö, and Emmez, H. (2016). Effects of quercetin on chronic constriction nerve injury in an experimental rat model. Acta Neurochir. 158, 959–965. doi: 10.1007/s00701-016-2761-0
Clatworthy, A. L., Illich, P. A., Castro, G. A., and Walters, E. T. (1995). Role of peri-axonal inflammation in the development of thermal hyperalgesia and guarding behavior in a rat model of neuropathic pain. Neurosci. Lett. 184, 5–8. doi: 10.1016/0304-3940(94)11154-B
Colloca, L., Ludman, T., Bouhassira, D., Baron, R., Dickenson, A. H., Yarnitsky, D., et al. (2017). Neuropathic pain. Nat. Rev. Dis. Prim. 3:17002. doi: 10.1038/nrdp.2017.2
Cui, J. G., Holmin, S., Mathiesen, T., Meyerson, B. A., and Linderoth, B. (2000). Possible role of inflammatory mediators in tactile hypersensitivity in rat models of mononeuropathy. Pain 88, 239–248. doi: 10.1016/S0304-3959(00)00331-6
Cunha, F. Q., Poole, S., Lorenzetti, B. B., and Ferreira, S. H. (1992). The pivotal role of tumour necrosis factor α in the development of inflammatory hyperalgesia. Br. J. Pharmacol. 107, 660–664. doi: 10.1111/j.1476-5381.1992.tb14503.x
Cushnie, T. P. T., and Lamb, A. J. (2005). Antimicrobial activity of flavonoids. Int. J. Antimicrob. Agents 26, 343–356. doi: 10.1016/j.ijantimicag.2005.09.002
Dai, W., Bi, J., Li, F., Wang, S., Huang, X., Meng, X., et al. (2019). Antiviral efficacy of flavonoids against enterovirus 71 infection in vitro and in newborn mice. Viruses 11:625. doi: 10.3390/v11070625
de Teles, R. B. A., Diniz, T. C., Pinto, T. C. C., de Júnior, R. G. O., Silva, M. G. E., de Lavor, E. M., et al. (2018). Flavonoids as therapeutic agents in Alzheimer’s and Parkinson’s diseases: a systematic review of preclinical evidences. Oxid. Med. Cell. Longev. 2018:7043213. doi: 10.1155/2018/7043213
Dehmlow, C., Erhard, J., and de Groot, H. (1996). Inhibition of Kupffer cell functions as an explanation for the hepatoprotective properties of silibinin. Hepatology 23, 749–754. doi: 10.1002/hep.510230415
DeLeo, J. A., and Yezierski, R. P. (2001). The role of neuroinflammation and neuroimmune activation in persistent pain. Pain 90, 1–6. doi: 10.1016/S0304-3959(00)00490-5
Dyck, P. J., Dyck, P. J. B., Velosa, J. A., Larson, T. S., and O’Brien, P. C. (2000). Patterns of quantitative sensation testing of hypoesthesia and hyperalgesia are predictive of diabetic polyneuropathy: a study of three cohorts. Diabetes Care 23, 510–517. doi: 10.2337/diacare.23.4.510
Eaton, M. J., Martinez, M. A., and Karmally, S. (1999a). A single intrathecal injection of GABA permanently reverses neuropathic pain after nerve injury. Brain Res. 835, 334–339. doi: 10.1016/S0006-8993(99)01564-4
Eaton, M. J., Plunkett, J. A., Martinez, M. A., Lopez, T., Karmally, S., Cejas, P., et al. (1999b). Transplants of neuronal cells bioengineered to synthesize GABA alleviate chronic neuropathic pain. Cell Transplant. 8, 87–101. doi: 10.1177/096368979900800102
Egert, S., and Rimbach, G. (2011). Which sources of flavonoids: complex diets or dietary supplements? Adv. Nutr. 2, 8–14. doi: 10.3945/an.110.000026
Feldman, E. L., Stevens, M. J., Thomas, P. K., Brown, M. B., Canal, N., and Greene, D. A. (1994). A practical two-step quantitative clinical and electrophysiological assessment for the diagnosis and staging of diabetic neuropathy. Diabetes Care 17, 1281–1289. doi: 10.2337/diacare.17.11.1281
Ferrali, M., Signorini, C., Caciotti, B., Sugherini, L., Ciccoli, L., Giachetti, D., et al. (1997). Protection against oxidative damage of erythrocyte membrane by the flavonoid quercetin and its relation to iron chelating activity. FEBS Lett. 416, 123–129. doi: 10.1016/S0014-5793(97)01182-4
Ferrándiz, M. L., and Alcaraz, M. J. (1991). Anti-inflammatory activity and inhibition of arachidonic acid metabolism by flavonoids. Agents Actions 32, 283–288. doi: 10.1007/BF01980887
Ferrándiz, M. L., Gil, B., Sanz, M. J., Ubeda, A., Erazo, S., González, E., et al. (1996). Effect of bakuchiol on leukocyte functions and some inflammatory responses in mice. J. Pharm. Pharmacol. 48, 975–980. doi: 10.1111/j.2042-7158.1996.tb06016.x
Ferreira, S. H., Lorenzetti, B. B., and Poole, S. (1993). Bradykinin initiates cytokine-mediated inflammatory hyperalgesia. Br. J. Pharmacol. 110, 1227–1231. doi: 10.1111/j.1476-5381.1993.tb13946.x
Forrester, S. J., Kikuchi, D. S., Hernandes, M. S., Xu, Q., and Griendling, K. K. (2018). Reactive oxygen species in metabolic and inflammatory signaling. Circ. Res. 122, 877–902. doi: 10.1161/CIRCRESAHA.117.311401
Freshwater, J. D., and Calcutt, N. A. (2005). Low doses of formalin reveal allodynia in diabetic rats. J. Neuropathic Pain Symptom Palliation 1, 39–46. doi: 10.3109/j426v01n01_08
Friesenecker, B., Tsai, A. G., and Lntaglietta, M. (1995). Cellular Basis of Inflammation, Edema and the Activity of Daflon 500 mg. Int. J. Microcirc. 15, 17–21. doi: 10.1159/000179090
Gao, W., Zan, Y., Wang, Z. J. J., Hu, X. Y., and Huang, F. (2016). Quercetin ameliorates paclitaxel-induced neuropathic pain by stabilizing mast cells, and subsequently blocking PKCε-dependent activation of TRPV1. Acta Pharmacol. Sin. 37, 1166–1177. doi: 10.1038/aps.2016.58
Gavande, N., Karim, N., Johnston, G. A. R., Hanrahan, J. R., and Chebib, M. (2011). Identification of Benzopyran-4-one Derivatives (Isoflavones) as Positive Modulators of GABAA Receptors. ChemMedChem 6, 1340–1346. doi: 10.1002/cmdc.201100120
Gilron, I., Watson, C. P. N., Cahill, C. M., and Moulin, D. E. (2006). Neuropathic pain: a practical guide for the clinician. CMAJ 175, 265–275. doi: 10.1503/cmaj.060146
Ginwala, R., Bhavsar, R., Chigbu, D. G. I., Jain, P., and Khan, Z. K. (2019). Potential role of flavonoids in treating chronic inflammatory diseases with a special focus on the anti-inflammatory activity of apigenin. Antioxidants 8:35. doi: 10.3390/antiox8020035
Górniak, I., Bartoszewski, R., and Króliczewski, J. (2019). Comprehensive review of antimicrobial activities of plant flavonoids. Phytochem. Rev. 18, 241–272. doi: 10.1007/s11101-018-9591-z
Griebel, G., Perrault, G., Tan, S., Schoemaker, H., and Sanger, D. J. (1999). Pharmacological studies on synthetic flavonoids: comparison with diazepam. Neuropharmacology 38, 965–977. doi: 10.1016/S0028-3908(99)00026-X
Gui, Y., Zhang, J., Chen, L., Duan, S., Tang, J., Xu, W., et al. (2018). Icariin, a flavonoid with anti-cancer effects, alleviated paclitaxel-induced neuropathic pain in a SIRT1-dependent manner. Mol. Pain 14:1744806918768970. doi: 10.1177/1744806918768970
Gwak, Y. S., Tan, H. Y., Nam, T. S., Paik, K. S., Hulsebosch, C. E., and Leem, J. W. (2006). Activation of spinal GABA receptors attenuates chronic central neuropathic pain after spinal cord injury. J. Neurotrauma 23, 1111–1124. doi: 10.1089/neu.2006.23.1111
Hagenacker, T., Hillebrand, I., Wissmann, A., Büsselberg, D., and Schäfers, M. (2010). Anti-allodynic effect of the flavonoid myricetin in a rat model of neuropathic pain: involvement of p38 and protein kinase C mediated modulation of Ca2+ channels. Eur. J. Pain 14, 992–998. doi: 10.1016/j.ejpain.2010.04.005
Hains, B. C., Saab, C. Y., Klein, J. P., Craner, M. J., and Waxman, S. G. (2004). Altered sodium channel expression in second-order spinal sensory neurons contributes to pain after peripheral nerve injury. J. Neurosci. 24, 4832–4839. doi: 10.1523/JNEUROSCI.0300-04.2004
Hall, B. J., Karim, N., Chebib, M., Johnston, G. A. R., and Hanrahan, J. R. (2014). Modulation of ionotropic GABA receptors by 6-methoxyflavanone and 6-methoxyflavone. Neurochem. Res. 39, 1068–1078. doi: 10.1007/s11064-013-1157-2
Hanasaki, Y., Ogawa, S., and Fukui, S. (1994). The correlation between active oxygens scavenging and antioxidative effects of flavonoids. Free Radic. Biol. Med. 16, 845–850. doi: 10.1016/0891-5849(94)90202-X
Hanisch, U.-K. (2002). Microglia as a source and target of cytokines. Glia 40, 140–155. doi: 10.1002/glia.10161
Hanrahan, J. R., Chebib, M., and Johnston, G. A. R. (2011). Flavonoid modulation of GABAA receptors. Br. J. Pharmacol. 163, 234–245. doi: 10.1111/j.1476-5381.2011.01228.x
Hara, K., Haranishi, Y., Terada, T., Takahashi, Y., Nakamura, M., and Sata, T. (2014). Effects of intrathecal and intracerebroventricular administration of luteolin in a rat neuropathic pain model. Pharmacol. Biochem. Behav. 125, 78–84. doi: 10.1016/j.pbb.2014.08.011
Harden, R. N. (1999). Gabapentin: a new tool in the treatment of neuropathic pain. Acta Neurol. Scand. 100, 43–47. doi: 10.1111/j.1600-0404.1999.tb07389.x
Heim, K. E., Tagliaferro, A. R., and Bobilya, D. J. (2002). Flavonoid antioxidants: chemistry, metabolism and structure-activity relationships. J. Nutr. Biochem. 13, 572–584. doi: 10.1016/S0955-2863(02)00208-5
Hendrich, J., Van Minh, A. T., Heblich, F., Nieto-Rostro, M., Watschinger, K., Striessnig, J., et al. (2008). Pharmacological disruption of calcium channel trafficking by the α 2 δ ligand gabapentin. Proc. Natl. Acad. Sci. U.S.A. 105, 3628–3633. doi: 10.1073/pnas.0708930105
Hershman, D. L., Lacchetti, C., Dworkin, R. H., Lavoie Smith, E. M., Bleeker, J., Cavaletti, G., et al. (2014). Prevention and management of chemotherapy-induced peripheral neuropathy in survivors of adult cancers: american society of clinical oncology clinical practice guideline. J. Clin. Oncol. 32, 1941–1967. doi: 10.1200/JCO.2013.54.0914
Hong, X., and Hopfinger, A. J. (2003). 3D-Pharmacophores of Flavonoid binding at the Benzodiazepine GABA A receptor site using 4D-QSAR analysis. J. Chem. Inf. Comput. Sci. 43, 324–336. doi: 10.1021/ci0200321
Hossain, M. S., Uddin, M. S., Asaduzzaman, M., Shirajum Munira, M., Josim Uddin, M., Rajdoula Rafe, M., et al. (2017b). Inquiry of analgesic and anti-inflammatory activities of Xanthosoma sagittifolium L.: an effective medicinal plant. J. Coast. Life Med. 5, 22–26. doi: 10.12980/jclm.5.2017J6-229
Hossain, M. S., Uddin, M. S., Kabir, M. T., Akhter, S., Goswami, S., Mamun, A. A., et al. (2017a). In vivo screening for analgesic and anti-inflammatory activities of Syngonium podophyllum L.: a remarkable herbal medicine. Annu. Res. Rev. Biol. 16, 1–12. doi: 10.9734/ARRB/2017/35692
Hwang, J. H., and Yaksh, T. L. (1997). The effect of spinal GABA receptor agonists on tactile allodynia in a surgically-induced neuropathic pain model in the rat. Pain 70, 15–22. doi: 10.1016/S0304-3959(96)03249-6
Hyo, C. H., and Snyder, S. H. (1999). Poly(ADP-ribose) polymerase is a mediator of necrotic cell death by ATP depletion. Proc. Natl. Acad. Sci. U.S.A. 96, 13978–13982. doi: 10.1073/pnas.96.24.13978
Jäger, A. K., Krydsfeldt, K., and Rasmussen, H. B. (2009). Bioassay-guided isolation of apigenin with GABA-benzodiazepine activity from Tanacetum parthenium. Phyther. Res. 23, 1642–1644. doi: 10.1002/ptr.2816
Jagtap, P., and Szabo, C. (2005). Poly(ADP-ribose) polymerase and the therapeutic effects of its inhibitors. Nat. Rev. Drug Discov. 4, 421–440. doi: 10.1038/nrd1718
Janeway, C. A., and Medzhitov, R. (2002). Innate immune recognition. Annu. Rev. Immunol. 20, 197–216. doi: 10.1146/annurev.immunol.20.083001.084359
Jensen, T. S., and Baron, R. (2003). Translation of symptoms and signs into mechanisms in neuropathic pain. Pain 102, 1–8. doi: 10.1016/s0304-3959(03)00006-x
Jergova, S., Hentall, I. D., Gajavelli, S., Varghese, M. S., and Sagen, J. (2012). Intraspinal transplantation of GABAergic neural progenitors attenuates neuropathic pain in rats: a pharmacologic and neurophysiological evaluation. Exp. Neurol. 234, 39–49. doi: 10.1016/j.expneurol.2011.12.005
Ji, C., Xu, Y., Han, F., Sun, D., Zhang, H., Li, X., et al. (2017). Quercetin alleviates thermal and cold hyperalgesia in a rat neuropathic pain model by inhibiting Toll-like receptor signaling. Biomed. Pharmacother. 94, 652–658. doi: 10.1016/j.biopha.2017.07.145
Ji, G., Yang, Q., Hao, J., Guo, L., Chen, X., Hu, J., et al. (2011). Anti-inflammatory effect of genistein on non-alcoholic steatohepatitis rats induced by high fat diet and its potential mechanisms. Int. Immunopharmacol. 11, 762–768. doi: 10.1016/j.intimp.2011.01.036
Ji, R. R., and Woolf, C. J. (2001). Neuronal plasticity and signal transduction in nociceptive neurons: implications for the initiation and maintenance of pathological pain. Neurobiol. Dis. 8, 1–10. doi: 10.1006/nbdi.2000.0360
Jin, S. X., Zhuang, Z. Y., Woolf, C. J., and Ji, R. R. (2003). p38 mitogen-activated protein kinase is activated after a spinal nerve ligation in spinal cord microglia and dorsal root ganglion neurons and contributes to the generation of neuropathic pain. J. Neurosci. 23, 4017–4022. doi: 10.1523/jneurosci.23-10-04017.2003
Kandhare, A. D., Raygude, K. S., Ghosh, P., Ghule, A. E., and Bodhankar, S. L. (2012). Neuroprotective effect of naringin by modulation of endogenous biomarkers in streptozotocin induced painful diabetic neuropathy. Fitoterapia 83, 650–659. doi: 10.1016/j.fitote.2012.01.010
Kaur, G., Bedi, O., Sharma, N., Singh, S., Deshmukh, R., and Kumar, P. (2016). Anti-hyperalgesic and anti-nociceptive potentials of standardized grape seed proanthocyanidin extract against CCI-induced neuropathic pain in rats. J. Basic Clin. Physiol. Pharmacol. 27, 9–17. doi: 10.1515/jbcpp-2015-0026
Kim, H. P., Son, K. H., Chang, H. W., and Kang, S. S. (2004). Anti-inflammatory plant flavonoids and cellular action mechanisms. J. Pharmacol. Sci. 96, 229–245. doi: 10.1254/jphs.CRJ04003X
Kishore, L., Kaur, N., and Singh, R. (2018). Effect of Kaempferol isolated from seeds of Eruca sativa on changes of pain sensitivity in Streptozotocin-induced diabetic neuropathy. Inflammopharmacology 26, 993–1003. doi: 10.1007/s10787-017-0416-2
Knabl, J., Witschi, R., Hösl, K., Reinold, H., Zeilhofer, U. B., Ahmadi, S., et al. (2008). Reversal of pathological pain through specific spinal GABAA receptor subtypes. Nature 451, 330–334. doi: 10.1038/nature06493
Komirishetty, P., Areti, A., Sistla, R., and Kumar, A. (2016). Morin Mitigates Chronic Constriction Injury (CCI)-induced peripheral neuropathy by inhibiting oxidative stress induced PARP over-activation and neuroinflammation. Neurochem. Res. 41, 2029–2042. doi: 10.1007/s11064-016-1914-0
Kozłowska, A., and Szostak-Węgierek, D. (2019). Flavonoids – Food Sources, Health Benefits, and Mechanisms Involved. Cham: Springer, 53–78. doi: 10.1007/978-3-319-78030-6_54
Kuang, X., Huang, Y., Gu, H. F., Zu, X. Y., Zou, W. Y., Song, Z. B., et al. (2012). Effects of intrathecal epigallocatechin gallate, an inhibitor of Toll-like receptor 4, on chronic neuropathic pain in rats. Eur. J. Pharmacol. 676, 51–56. doi: 10.1016/j.ejphar.2011.11.037
Kumar, A., and Mittal, R. (2017). Nrf2: a potential therapeutic target for diabetic neuropathy. Inflammopharmacology 25, 393–402. doi: 10.1007/s10787-017-0339-y
Kumar, S., and Pandey, A. K. (2013). Chemistry and biological activities of flavonoids: an overview. Sci. World J. 2013, 162750. doi: 10.1155/2013/162750
Lai, J., Hunter, J. C., and Porreca, F. (2003). The role of voltage-gated sodium channels in neuropathic pain. Curr. Opin. Neurobiol. 13, 291–297. doi: 10.1016/S0959-4388(03)00074-6
Langley, P. C., Van Litsenburg, C., Cappelleri, J. C., and Carroll, D. (2013). The burden associated with neuropathic pain in Western Europe. J. Med. Econ. 16, 85–95. doi: 10.3111/13696998.2012.729548
Li, M., Li, Q., Zhao, Q., Zhang, J., and Lin, J. (2015). Luteolin improves the impaired nerve functions in diabetic neuropathy: behavioral and biochemical evidences. Int. J. Clin. Exp. Pathol. 8, 10112–10120.
Li, P., Xiong, D. L., Sun, W. P., and Xu, S. Y. (2018). Effects of baicalin on diabetic neuropathic pain involving transient receptor potential vanilloid 1 in the dorsal root ganglia of rats. Neuroreport 29, 1492–1498. doi: 10.1097/WNR.0000000000001138
Lindenlaub, T., and Sommer, C. (2003). Cytokines in sural nerve biopsies from inflammatory and non-inflammatory neuropathies. Acta Neuropathol. 105, 593–602. doi: 10.1007/s00401-003-0689-y
Liu, H., Jiang, W., and Xie, M. (2010). Flavonoids: recent advances as anticancer drugs. Recent Pat. Anticancer. Drug Discov. 5, 152–164. doi: 10.2174/157489210790936261
Liu, M., Liao, K., Yu, C., Li, X., Liu, S., and Yang, S. (2014). Puerarin alleviates neuropathic pain by inhibiting neuroinflammation in spinal cord. Media. Inflamm. 2014:485927. doi: 10.1155/2014/485927
Liu, L., Zuo, Z., Lu, S., Liu, A., and Liu, X. (2017). Naringin attenuates diabetic retinopathy by inhibiting inflammation, oxidative stress and NF-κB activation in Vivo and in Vitro. Iran. J. Basic Med. Sci. 20, 814–822. doi: 10.22038/ijbms.2017.9017
Ma, W., and Eisenach, J. C. (2003). Cyclooxygenase 2 in infiltrating inflammatory cells in injured nerve is universally up-regulated following various types of peripheral nerve injury. Neuroscience 121, 691–704. doi: 10.1016/S0306-4522(03)00495-0
Mahmoud, A. M., Hernández Bautista, R. J., Sandhu, M. A., and Hussein, O. E. (2019). Beneficial effects of citrus flavonoids on cardiovascular and metabolic health. Oxid. Med. Cell. Longev. 2019:5484138. doi: 10.1155/2019/5484138
Malan, T. P., Mata, H. P., and Porreca, F. (2002). Spinal GABA A and GABA B receptor pharmacology in a rat model of neuropathic pain. Anesthesiology 96, 1161–1167. doi: 10.1097/00000542-200205000-00020
Marder, M., and Paladini, A. (2002). GABA-A-receptor ligands of flavonoid structure. Curr. Top. Med. Chem. 2, 853–867. doi: 10.2174/1568026023393462
Marder, M., Viola, H., Wasowski, C., Wolfman, C., Waterman, P. G., Cassels, B. K., et al. (1996). 6-bromoflavone, a high affinity ligand for the central benzodiazepine receptors is a member of a family of active flavonoids. Biochem. Biophys. Res. Commun. 223, 384–389. doi: 10.1006/bbrc.1996.0903
McCarson, K. E., and Enna, S. J. (2014). GABA pharmacology: the search for analgesics. Neurochem. Res. 39, 1948–1963. doi: 10.1007/s11064-014-1254-x
McKemy, D. D., Neuhausser, W. M., and Julius, D. (2002). Identification of a cold receptor reveals a general role for TRP channels in thermosensation. Nature 416, 52–58. doi: 10.1038/nature719
Medina, J. H., Viola, H., Wolfman, C., Marder, M., Wasowski, C., Calvo, D., et al. (1997). Overview - Flavonoids: a new family of benzodiazepine receptor ligands. Neurochem. Res. 22, 419–425. doi: 10.1023/A:1027303609517
Melzack, R., and Wall, P. D. (1965). Pain mechanisms: a new theory. Science 150, 971–979. doi: 10.1126/science.150.3699.971
Meotti, F. C., Missau, F. C., Ferreira, J., Pizzolatti, M. G., Mizuzaki, C., Nogueira, C. W., et al. (2006). Anti-allodynic property of flavonoid myricitrin in models of persistent inflammatory and neuropathic pain in mice. Biochem. Pharmacol. 72, 1707–1713. doi: 10.1016/j.bcp.2006.08.028
Minami, T., Uda, R., Horiguchi, S., Ito, S., Hyodo, M., and Hayaishi, O. (1992). Allodynia evoked by intrathecal administration of prostaglandin F2α to conscious mice. Pain 50, 223–229. doi: 10.1016/0304-3959(92)90166-9
Minami, T., Uda, R., Horiguchi, S., Ito, S., Hyodo, M., and Hayaishi, O. (1994). Allodynia evoked by intrathecal administration of prostaglandin E2 to conscious mice. Pain 57, 217–223. doi: 10.1016/0304-3959(94)90226-7
Mirshekar, M., Roghani, M., Khalili, M., Baluchnejadmojarad, T., and Arab Moazzen, S. (2010). Chronic oral pelargonidin alleviates streptozotocin-induced diabetic neuropathic hyperalgesia in rat: involvement of oxidative stress. Iran. Biomed. J. 14, 33–39.
Moore, K. A., Kohno, T., Karchewski, L. A., Scholz, J., Baba, H., and Woolf, C. J. (2002). Partial peripheral nerve injury promotes a selective loss of GABAergic inhibition in the superficial dorsal horn of the spinal cord. J. Neurosci. 22, 6724–6731. doi: 10.1523/jneurosci.22-15-06724.2002
Murnion, B. P. (2018). Neuropathic pain: current definition and review of drug treatment. Aust. Prescr. 41, 60–63. doi: 10.18773/austprescr.2018.022
Muto, N., Matsuoka, Y., Arakawa, K., Kurita, M., Omiya, H., Taniguchi, A., et al. (2018). Quercetin attenuates neuropathic pain in rats with spared nerve injury. Acta Med. Okayama 72, 457–465. doi: 10.18926/AMO/56243
Myers, S. A., and Bennett, T. (2008). xPharm: The Comprehensive Pharmacology Reference. Amsterdam: Elsevier, doi: 10.1016/B978-008055232-3.60980-8
Nelson, C. W., Wei, E. P., Povlishock, J. T., Kontos, H. A., and Moskowitz, M. A. (1992). Oxygen radicals in cerebral ischemia. Am. J. Physiol. Hear. Circ. Physiol. 263, H1356–H1362. doi: 10.1152/ajpheart.1992.263.5.h1356
Obrosova, I. G., Li, F., Abatan, O. I., Forsell, M. A., Komjáti, K., Pacher, P., et al. (2004). Role of Poly(ADP-Ribose) polymerase activation in diabetic neuropathy. Diabetes Metab. Res. Rev. 53, 711–720. doi: 10.2337/diabetes.53.3.711
O’Connor, A. B. (2009). Neuropathic pain: quality-of-life impact, costs and cost effectiveness of therapy. Pharmacoeconomics 27, 95–112. doi: 10.2165/00019053-200927020-00002
Ognibene, E., Bovicelli, P., Adriani, W., Saso, L., and Laviola, G. (2008). Behavioral effects of 6-bromoflavanone and 5-methoxy-6,8-dibromoflavanone as anxiolytic compounds. Prog. Neuro Psychopharmacol. Biol. Psychiatry 32, 128–134. doi: 10.1016/j.pnpbp.2007.07.023
Orhan, D. D., Özçelik, B., Özgen, S., and Ergun, F. (2010). Antibacterial, antifungal, and antiviral activities of some flavonoids. Microbiol. Res. 165, 496–504. doi: 10.1016/j.micres.2009.09.002
Ossipov, M. H., Dussor, G. O., and Porreca, F. (2010). Central modulation of pain. J. Clin. Invest. 120, 3779–3787. doi: 10.1172/JCI43766
Paladini, A. C., Marder, M., Viola, H., Wolfman, C., Wasowski, C., and Medina, J. H. (1999). Flavonoids and the central nervous system: from forgotten factors to potent anxiolytic compounds. J. Pharm. Pharmacol. 51, 519–526. doi: 10.1211/0022357991772790
Panche, A. N., Diwan, A. D., and Chandra, S. R. (2016). Flavonoids: an overview. J. Nutr. Sci. 5:e47. doi: 10.1017/jns.2016.41
Patapoutian, A., Peier, A. M., Story, G. M., and Viswanath, V. (2003). Thermotrp channels and beyond: mechanisms of temperature sensation. Nat. Rev. Neurosci. 4, 529–539. doi: 10.1038/nrn1141
Perkins, B. A., Olaleye, D., Zinman, B., and Bril, V. (2001). Simple screening test for peripheral neuropathy in the diabetes clinic. Diabetes Care 24, 250–256. doi: 10.2337/diacare.24.2.250
Pittenger, G. L., Mehrabyan, A., Simmons, K., Rice, A., Dublins, C., Barlow, P., et al. (2005). Small fiber neuropathy is associated with the metabolic syndrome. Metab. Syndr. Relat. Disord. 3, 113–121. doi: 10.1089/met.2005.3.113
Price, G. W., Wilkin, G. P., Turnbull, M. J., and Bowery, N. G. (1984). Are baclofen-sensitive GABAB receptors present on primary afferent terminals of the spinal cord? Nature 307, 71–74. doi: 10.1038/307071a0
Price, M. P., McIlwrath, S. L., Xie, J., Cheng, C., Qiao, J., Tarr, D. E., et al. (2001). The DRASIC cation channel contributes to the detection of cutaneous touch and acid stimuli in mice. Neuron 32, 1071–1083. doi: 10.1016/S0896-6273(01)00547-5
Quintans, J. S. S., Antoniolli, ÂR., Almeida, J. R. G. S., Santana-Filho, V. J., and Quintans-Júnior, L. J. (2014). Natural products evaluated in neuropathic pain models - a systematic review. Basic Clin. Pharmacol. Toxicol. 114, 442–450. doi: 10.1111/bcpt.12178
Quintão, N. L. M., Antonialli, C. S., Da Silva, G. F., Rocha, L. W., De Souza, M. M., Malheiros, A., et al. (2012). Aleurites moluccana and its main active ingredient, the flavonoid 2″-O-rhamnosylswertisin, have promising antinociceptive effects in experimental models of hypersensitivity in mice. Pharmacol. Biochem. Behav. 102, 302–311. doi: 10.1016/j.pbb.2012.05.005
Raposo, D., Morgado, C., Pereira-Terra, P., and Tavares, I. (2015). Nociceptive spinal cord neurons of laminae I-III exhibit oxidative stress damage during diabetic neuropathy which is prevented by early antioxidant treatment with epigallocatechin-gallate (EGCG). Brain Res. Bull. 110, 68–75. doi: 10.1016/j.brainresbull.2014.12.004
Rasmussen, P. V., Sindrup, S. H., Jensen, T. S., and Bach, F. W. (2004). Symptoms and signs in patients with suspected neuropathic pain. Pain 110, 461–469. doi: 10.1016/j.pain.2004.04.034
Reeve, A. J., Dickenson, A. H., and Kerr, N. C. (1998). Spinal effects of Bicuculline: modulation of an Allodynia-like state by an A 1 -receptor agonist, morphine, and an NMDA-receptor antagonist. J. Neurophysiol. 79, 1494–1507. doi: 10.1152/jn.1998.79.3.1494
Ren, L., Wang, F., Xu, Z., Chan, W. M., Zhao, C., and Xue, H. (2010). GABAA receptor subtype selectivity underlying anxiolytic effect of 6-hydroxyflavone. Biochem. Pharmacol. 79, 1337–1344. doi: 10.1016/j.bcp.2009.12.024
Riley, R. C., Trafton, J. A., Chi, S. I., and Basbaum, A. I. (2001). Presynaptic regulation of spinal cord tachykinin signaling via GABAB but not GABAA receptor activation. Neuroscience 103, 725–737. doi: 10.1016/S0306-4522(00)00571-6
Rudolph, U., and Möhler, H. (2006). GABA-based therapeutic approaches: GABAA receptor subtype functions. Curr. Opin. Pharmacol. 6, 18–23. doi: 10.1016/j.coph.2005.10.003
Samsuzzaman, M., Uddin, M. S., Shah, M. A., and Mathew, B. (2019). Natural inhibitors on airway mucin: molecular insight into the therapeutic potential targeting MUC5AC expression and production. Life Sci. 231:116485. doi: 10.1016/J.LFS.2019.05.041
Schattschneider, J., Uphoff, J., Binder, A., Wasner, G., and Baron, R. (2006). No adrenergic sensitization of afferent neurons in painful sensory polyneuropathy. J. Neurol. 253, 280–286. doi: 10.1007/s00415-005-0976-8
Schwingel, T. E., Klein, C. P., Nicoletti, N. F., Dora, C. L., Hadrich, G., Bica, C. G., et al. (2014). Effects of the compounds resveratrol, rutin, quercetin, and quercetin nanoemulsion on oxaliplatin-induced hepatotoxicity and neurotoxicity in mice. Naunyn. Schmiedebergs. Arch. Pharmacol. 387, 837–848. doi: 10.1007/s00210-014-0994-0
Semyanov, A., Walker, M. C., and Kullmann, D. M. (2003). GABA uptake regulates cortical excitability via cell type-specific tonic inhibition. Nat. Neurosci. 6, 484–490. doi: 10.1038/nn1043
Shahid, M., Subhan, F., Ahmad, N., and Sewell, R. D. E. (2017). The flavonoid 6-methoxyflavone allays cisplatin-induced neuropathic allodynia and hypoalgesia. Biomed. Pharmacother. 95, 1725–1733. doi: 10.1016/j.biopha.2017.09.108
Shehla, A. (2019). Flavonoids in neuropathic pain management: a new player on an old target. African J. Pharm. Pharmacol. 13, 100–112. doi: 10.5897/ajpp2019.5023
Shoskes, D. A. (1998). Effect of bioflavonoids quercetin and curcumin on ischemic renal injury: a new class of renoprotective agents. Transplantation 66, 147–152. doi: 10.1097/00007890-199807270-00001
Shutenko, Z., Henry, Y., Pinard, E., Seylaz, J., Potier, P., Berthet, F., et al. (1999). Influence of the antioxidant quercetin in vivo on the level of nitric oxide determined by electron paramagnetic resonance in rat brain during global ischemia and reperfusion. Biochem. Pharmacol. 57, 199–208. doi: 10.1016/S0006-2952(98)00296-2
Sivilotti, L., and Woolf, C. J. (1994). The contribution of GABA(A) and glycine receptors to central sensitization: disinhibition and touch-evoked allodynia in the spinal cord. J. Neurophysiol. 72, 169–179. doi: 10.1152/jn.1994.72.1.169
Sommer, C. (2003). Painful neuropathies. Curr. Opin. Neurol. 16, 623–628. doi: 10.1097/01.wco.0000093106.34793.06
Sommer, C., and Kress, M. (2004). Recent findings on how proinflammatory cytokines cause pain: peripheral mechanisms in inflammatory and neuropathic hyperalgesia. Neurosci. Lett. 361, 184–187. doi: 10.1016/j.neulet.2003.12.007
Sommer, C., Schmidt, C., and George, A. (1998). Hyperalgesia in experimental neuropathy is dependent on the TNF receptor 1. Exp. Neurol. 151, 138–142. doi: 10.1006/exnr.1998.6797
Spiering, M. J. (2018). The discovery of GABA in the brain. J. Biol. Chem. 293, 19159–19160. doi: 10.1074/jbc.CL118.006591
Stavniichuk, R., Drel, V. R., Shevalye, H., Maksimchyk, Y., Kuchmerovska, T. M., Nadler, J. L., et al. (2011). Baicalein alleviates diabetic peripheral neuropathy through inhibition of oxidative-nitrosative stress and p38 MAPK activation. Exp. Neurol. 230, 106–113. doi: 10.1016/j.expneurol.2011.04.002
Stein, A., and Arnold, D. (2012). Oxaliplatin: a review of approved uses. Expert Opin. Pharmacother. 13, 125–137. doi: 10.1517/14656566.2012.643870
Stubley, L. A., Martinez, M. A., Karmally, S., Lopez, T., Cejas, P., and Eaton, M. J. (2001). Only early intervention with Gamma-Aminobutyric acid cell therapy is able to Reverse Neuropathic pain after partial nerve injury. J. Neurotrauma 18, 471–477. doi: 10.1089/089771501750171092
Tal, M., and Bennett, G. J. (1994). Extra-territorial pain in rats with a peripheral mononeuropathy: mechano-hyperalgesia and mechano-allodynia in the territory of an uninjured nerve. Pain 57, 375–382. doi: 10.1016/0304-3959(94)90013-2
Thangapandiyan, S., Ramesh, M., Hema, T., Miltonprabu, S., Uddin, M. S., Nandhini, V., et al. (2019). Sulforaphane potentially ameliorates arsenic induced hepatotoxicity in Albino Wistar rats: implication of PI3K/Akt/Nrf2 signaling pathway. Cell. Physiol. Biochem. 52, 1203–1222. doi: 10.33594/000000082
Tian, R., Yang, W., Xue, Q., Gao, L., Huo, J., Ren, D., et al. (2016). Rutin ameliorates diabetic neuropathy by lowering plasma glucose and decreasing oxidative stress via Nrf2 signaling pathway in rats. Eur. J. Pharmacol. 771, 84–92. doi: 10.1016/j.ejphar.2015.12.021
Tiwari, V., Kuhad, A., and Chopra, K. (2011). Amelioration of functional, biochemical and molecular deficits by epigallocatechin gallate in experimental model of alcoholic neuropathy. Eur. J. Pain 15, 286–292. doi: 10.1016/j.ejpain.2010.07.005
Toh, H.-S., Maharjan, J., Thapa, R., Neupane, K. D., Shah, M., Baral, S., et al. (2018). Diagnosis and impact of neuropathic pain in leprosy patients in Nepal after completion of multidrug therapy. PLoS Negl. Trop. Dis. 12:e0006610. doi: 10.1371/journal.pntd.0006610
Tracey, D. J., and Walker, J. S. (1995). Pain due to nerve damage: are inflammatory mediators involved. Inflamm. Res. 44, 407–411. doi: 10.1007/BF01757696
Tucker, A. P., Mezzatesta, J., Nadeson, R., and Goodchild, C. S. (2004). Intrathecal midazolam II: combination with intrathecal fentanyl for labor pain. Anesth. Analg. 98, 1521–1527. doi: 10.1213/01.ANE.0000112434.68702.E4
Turner, M. S. (2003). Intrathecal drug delivery 2002. Acta Neurochir. Suppl. 87, 29–35. doi: 10.1007/978-3-7091-6081-7_7
Tyson, J. A., and Anderson, S. A. (2014). GABAergic interneuron transplants to study development and treat disease. Trends Neurosci. 37, 169–177. doi: 10.1016/j.tins.2014.01.003
Uda, R., Horiguchi, S., Ito, S., Hyodo, M., and Hayaishi, O. (1990). Nociceptive effects induced by intrathecal administration of prostaglandin D2, E2, or F2α to conscious mice. Brain Res. 510, 26–32. doi: 10.1016/0006-8993(90)90723-O
Uddin, M. S., and Amran, M. S. (2019). Comprehensive MCQs in Pharmacology. Hauppauge, NY: Nova Science Publishers.
Uddin, M. S., Hossain, M. F., Al Mamun, A., Shah, M. A., Hasana, S., Bulbul, I. J., et al. (2020a). Exploring the multimodal role of phytochemicals in the modulation of cellular signaling pathways to combat age-related neurodegeneration. Sci. Total Environ. 2020:138313. doi: 10.1016/j.scitotenv.2020.138313
Uddin, M. S., Kabir, M. T., and Al Mamun, A. (2020b). Pharmacological approaches to mitigate neuroinflammation in Alzheimer’s disease. Int. Immunopharmacol. 2020:106479. doi: 10.1016/j.intimp.2020.106479
Uddin, M. S., Kabir, M. T., Niaz, K., Jeandet, P., Clément, C., Mathew, B., et al. (2020c). Molecular insight into the therapeutic promise of flavonoids against Alzheimer’s disease. Moleclues. 25:1267. doi: 10.3390/MOLECULES25061267
Uddin, M. S., Kabir, M. T., Tewari, D., Mathew, B., and Aleya, L. (2020d). Emerging signal regulating potential of small molecule biflavonoids to combat neuropathological insults of Alzheimer’s disease. Sci. Total Environ. 700:134836. doi: 10.1016/j.scitotenv.2019.134836
Uddin, M. S., Mamun, A. Al, Jakaria, M., Thangapandiyan, S., Ahmad, J., Rahman, M. A., et al. (2020e). Emerging promise of sulforaphane-mediated Nrf2 signaling cascade against neurological disorders. Sci. Total Environ. 2019:135624. doi: 10.1016/j.scitotenv.2019.135624
Uddin, M. S., Mamun, A. A., Kabir, M. T., Nasrullah, M., Wahid, F., Begum, M. M., et al. (2018a). Neurochemistry of neurochemicals: messengers of brain functions. J. Intellect. Disabil. Diagnosis Treat. 5, 137–151. doi: 10.6000/2292-2598.2017.05.04.6
Uddin, M. S., Hossain, M. S., Al Mamun, A., Tewari, D., Asaduzzaman, M., Islam, M. S., et al. (2018b). Phytochemical analysis and antioxidant profile of methanolic extract of seed, pulp and peel of Baccaurea ramiflora Lour. Asian Pac. J. Trop. Med. 11, 443–450. doi: 10.4103/1995-7645.237189
Uddin, M. S., and Kabir, M. T. (2019). Emerging signal regulating potential of genistein against Alzheimer’s disease: a promising molecule of interest. Front. Cell Dev. Biol. 7:197. doi: 10.3389/fcell.2019.00197
Uddin, M. S., Kabir, M. T., Jakaria, M., Mamun, A. Al, Niaz, K., Amran, M. S., et al. (2019). Endothelial PPARγ is crucial for averting age-related vascular dysfunction by stalling oxidative stress and ROCK. Neurotox. Res. 36, 583–601. doi: 10.1007/s12640-019-00047-5
Uddin, M. S., Mamun, A. A., Hossain, M. S., Akter, F., Iqbal, M. A., and Asaduzzaman, M. (2016). Exploring the effect of Phyllanthus emblica L. on cognitive performance, brain antioxidant markers and acetylcholinesterase activity in rats: promising natural gift for the mitigation of Alzheimer’s disease. Ann. Neurosci. 23, 218–229. doi: 10.1159/000449482
Uddin, M. S., and Rashid, M. (2020). Advances in Neuropharmacology: Drugs and Therapeutics. Canada: Apple Academic Press.
Uddin, M. S., Uddin, G. M. S., Begum, M. M., Begum, Y., Herrera-Calderon, O., Islam, M. M., et al. (2017). Inspection of phytochemical content and in vitro antioxidant profile of Gnaphalium luteoalbum L.: an unexplored phytomedicine. J. Pharm. Nutr. Sci. 7, 136–146. doi: 10.6000/1927-5951.2017.07.03.10
Uddin, M. S., and Upaganlawar, A. B. (2019). Oxidative Stress and Antioxidant Defense: Biomedical Value in Health and Diseases. Hauppauge, NY: Nova Science Publishers.
Ultenius, C., Linderoth, B., Meyerson, B. A., and Wallin, J. (2006). Spinal NMDA receptor phosphorylation correlates with the presence of neuropathic signs following peripheral nerve injury in the rat. Neurosci. Lett. 399, 85–90. doi: 10.1016/j.neulet.2006.01.018
Valavanidis, A., Vlachogianni, T., and Fiotakis, C. (2009). 8-Hydroxy-2’ -deoxyguanosine (8-OHdG): a critical biomarker of oxidative stress and carcinogenesis. J. Environ. Sci. Heal. Part C Environ. Carcinog. Ecotoxicol. Rev. 27, 120–139. doi: 10.1080/10590500902885684
Valsecchi, A. E., Franchi, S., Panerai, A. E., Rossi, A., Sacerdote, P., and Colleoni, M. (2011). The soy isoflavone genistein reverses oxidative and inflammatory state, neuropathic pain, neurotrophic and vasculature deficits in diabetes mouse model. Eur. J. Pharmacol. 650, 694–702. doi: 10.1016/j.ejphar.2010.10.060
Valsecchi, A. E., Franchi, S., Panerai, A. E., Sacerdote, P., Trovato, A. E., and Colleoni, M. (2008). Genistein, a natural phytoestrogen from soy, relieves neuropathic pain following chronic constriction sciatic nerve injury in mice: anti-inflammatory and antioxidant activity. J. Neurochem. 107, 230–240. doi: 10.1111/j.1471-4159.2008.05614.x
Verri, W. A., Vicentini, F. T. M. C., Baracat, M. M., Georgetti, S. R., Cardoso, R. D. R., Cunha, T. M., et al. (2012). “Flavonoids as anti-inflammatory and analgesic drugs: mechanisms of action and perspectives in the development of pharmaceutical forms,” in Studies in Natural Products Chemistry, Ed. A. U. Rahman (Amsterdam: Elsevier B.V), 297–330. doi: 10.1016/B978-0-444-53836-9.00026-8
Viola, H., Wolfman, C., Marder, M., Goutman, J. D., Bianchin, M., Wasowski, C., et al. (2000). 6-Chloro-3’-nitroflavone is a potent ligand for the benzodiazepine binding site of the GABA(A) receptor devoid of intrinsic activity. Pharmacol. Biochem. Behav. 65, 313–320. doi: 10.1016/S0091-3057(99)00199-9
Visnagri, A., Kandhare, A. D., Chakravarty, S., Ghosh, P., and Bodhankar, S. L. (2014). Hesperidin, a flavanoglycone attenuates experimental diabetic neuropathy via modulation of cellular and biochemical marker to improve nerve functions. Pharm. Biol. 52, 814–828. doi: 10.3109/13880209.2013.870584
von Hehn, C. A., Baron, R., and Woolf, C. J. (2012). Deconstructing the neuropathic pain phenotype to reveal neural mechanisms. Neuron 73, 638–652. doi: 10.1016/j.neuron.2012.02.008
Wafford, K. A. (2005). GABAA receptor subtypes: any clues to the mechanism of benzodiazepine dependence? Curr. Opin. Pharmacol. 5, 47–52. doi: 10.1016/j.coph.2004.08.006
Wang, D., Tang, W., Yang, G. M., and Cai, B. C. (2010). Anti-inflammatory, antioxidant and cytotoxic activities of flavonoids from oxytropis falcata bunge. Chin. J. Nat. Med. 8, 461–465. doi: 10.1016/S1875-5364(11)60008-3
Wang, Y., Chen, P., Tang, C., Wang, Y., Li, Y., and Zhang, H. (2014). Antinociceptive and anti-inflammatory activities of extract and two isolated flavonoids of Carthamus tinctorius L. J. Ethnopharmacol. 151, 944–950. doi: 10.1016/j.jep.2013.12.003
Wasner, G. (2004). Topical menthol–a human model for cold pain by activation and sensitization of C nociceptors. Brain 127, 1159–1171. doi: 10.1093/brain/awh134
Wolfman, C., Viola, H., Marder, M., Ardenghi, P., Wasowski, C., Schröder, N., et al. (1998). Pharmacological characterization of 6-bromo-3′-nitroflavone, a synthetic flavonoid with high affinity for the benzodiazepine receptors. Pharmacol. Biochem. Behav. 61, 239–246. doi: 10.1016/S0091-3057(98)00088-4
Woolf, C. J. (2011). Central sensitization: implications for the diagnosis and treatment of pain. Pain 152, S2–S15. doi: 10.1016/j.pain.2010.09.030
Xifró, X., Vidal-Sancho, L., Boadas-Vaello, P., Turrado, C., Alberch, J., Puig, T., et al. (2015). Novel Epigallocatechin-3-Gallate (EGCG) derivative as a new therapeutic strategy for reducing neuropathic pain after chronic constriction nerve injury in mice. PLoS One 10:e0123122. doi: 10.1371/journal.pone.0123122
Xu, C., Xu, W., Xu, H., Xiong, W., Gao, Y., Li, G., et al. (2012). Role of puerarin in the signalling of neuropathic pain mediated by P2X3 receptor of dorsal root ganglion neurons. Brain Res. Bull. 87, 37–43. doi: 10.1016/j.brainresbull.2011.10.007
Yaksh, T. L. (1989). Behavioral and autonomic correlates of the tactile evoked allodynia produced by spinal glycine inhibition: effects of modulatory receptor systems and excitatory amino acid antagonists. Pain 37, 111–123. doi: 10.1016/0304-3959(89)90160-7
Yamamoto, T., and Yaksh, T. L. (1993). Effects of intrathecal strychnine and bicuculline on nerve compression-induced thermal hyperalgesia and selective antagonism by MK-801. Pain 54, 79–84. doi: 10.1016/0304-3959(93)90102-U
Yao, N., Song, A., Wang, X., Dixon, S., and Lam, K. S. (2007). Synthesis of flavonoid analogues as scaffolds for natural product-based combinatorial libraries. J. Comb. Chem. 9, 668–676. doi: 10.1021/cc070009y
Yu, L. L., Zhou, K. K., and Parry, J. (2005). Antioxidant properties of cold-pressed black caraway, carrot, cranberry, and hemp seed oils. Food Chem. 91, 723–729. doi: 10.1016/j.foodchem.2004.06.044
Zaplatic, E., Bule, M., Shah, S. Z. A., Uddin, M. S., and Niaz, K. (2019). Molecular mechanisms underlying protective role of quercetin in attenuating Alzheimer’s disease. Life Sci. 224, 109–119. doi: 10.1016/j.lfs.2019.03.055
Zeilhofer, H. U. (2008). Loss of glycinergic and GABAergic inhibition in chronic pain-contributions of inflammation and microglia. Int. Immunopharmacol. 8, 182–187. doi: 10.1016/j.intimp.2007.07.009
Zeilhofer, H. U., Möhler, H., and Di Lio, A. (2009). GABAergic analgesia: new insights from mutant mice and subtype-selective agonists. Trends Pharmacol. Sci. 30, 397–402. doi: 10.1016/j.tips.2009.05.007
Zhang, G., Liu, N., Zhu, C., Ma, L., Yang, J., Du, J., et al. (2019). Antinociceptive effect of isoorientin against neuropathic pain induced by the chronic constriction injury of the sciatic nerve in mice. Int. Immunopharmacol. 75, 105753. doi: 10.1016/j.intimp.2019.105753
Keywords: neuropathic pain, neuronal injury, flavonoids, benzodiazepines, GABA
Citation: Uddin MS, Mamun AA, Rahman MA, Kabir MT, Alkahtani S, Alanazi IS, Perveen A, Ashraf GM, Bin-Jumah MN and Abdel-Daim MM (2020) Exploring the Promise of Flavonoids to Combat Neuropathic Pain: From Molecular Mechanisms to Therapeutic Implications. Front. Neurosci. 14:478. doi: 10.3389/fnins.2020.00478
Received: 15 January 2020; Accepted: 17 April 2020;
Published: 09 June 2020.
Edited by:
Rajeev K. Singla, West China Hospital, Sichuan University, ChinaReviewed by:
Nasiara Karim, University of Malakand, PakistanRohit Gundamaraju, University of Tasmania, Australia
Copyright © 2020 Uddin, Mamun, Rahman, Kabir, Alkahtani, Alanazi, Perveen, Ashraf, Bin-Jumah and Abdel-Daim. This is an open-access article distributed under the terms of the Creative Commons Attribution License (CC BY). The use, distribution or reproduction in other forums is permitted, provided the original author(s) and the copyright owner(s) are credited and that the original publication in this journal is cited, in accordance with accepted academic practice. No use, distribution or reproduction is permitted which does not comply with these terms.
*Correspondence: Md. Sahab Uddin, msu-neuropharma@hotmail.com; msu_neuropharma@hotmail.com
†These authors have contributed equally to this work