- 1Departamento de Análises Clínicas e Toxicológicas, Faculdade de Ciências Farmacêuticas, Universidade de São Paulo, São Paulo, Brazil
- 2Departamento de Alimentos e Medicamentos, Faculdade de Ciências Farmacêuticas, Universidade Federal de Alfenas, Alfenas, Brazil
- 3Laboratory of Nuclear Medicine (LIM-43), Departamento de Radiologia e Oncologia, Faculdade de Medicina, Universidade de São Paulo, São Paulo, Brazil
- 4Instituto de Pesquisas Energéticas e Nucleares, São Paulo, Brazil
- 5Department of Biomedical Sciences and CNR Institute of Neuroscience, Faculty of Medicine and Surgery, University of Cagliari, Cagliari, Italy
Exposure to environmental tobacco smoke (ETS) is associated with high morbidity and mortality, mainly in childhood. Our aim was to evaluate the effects of postnatal ETS exposure in the brain 2-deoxy-2-[18F]-fluoro-D-glucose (18F-FDG) uptake of mice by positron emission tomography (PET) neuroimaging in a longitudinal study. C57BL/6J mice were exposed to ETS that was generated from 3R4F cigarettes from postnatal day 3 (P3) to P14. PET analyses were performed in male and female mice during infancy (P15), adolescence (P35), and adulthood (P65). We observed that ETS exposure decreased 18F-FDG uptake in the whole brain, both left and right hemispheres, and frontal cortex in both male and female infant mice, while female infant mice exposed to ETS showed decreased 18F-FDG uptake in the cerebellum. In addition, all mice showed reduced 18F-FDG uptake in infancy, compared to adulthood in all analyzed VOIs. In adulthood, ETS exposure during the early postnatal period decreased brain 18F-FDG uptake in adult male mice in the cortex, striatum, hippocampus, cingulate cortex, and thalamus when compared to control group. ETS induced an increase in 18F-FDG uptake in adult female mice when compared to control group in the brainstem and cingulate cortex. Moreover, male ETS-exposed animals showed decreased 18F-FDG uptake when compared to female ETS-exposed in the whole brain, brainstem, cortex, left amygdala, striatum, hippocampus, cingulate cortex, basal forebrain and septum, thalamus, hypothalamus, and midbrain. The present study shows that several brain regions are vulnerable to ETS exposure during the early postnatal period and these effects on 18F-FDG uptake are observed even a long time after the last exposure. This study corroborates our previous findings, strengthening the idea that exposure to tobacco smoke in a critical period interferes with brain development of mice from late infancy to early adulthood.
Introduction
Exposure to environmental tobacco smoke (ETS), one of the most common indoor pollutants, is composed of both mainstream and sidestream smoke. Approximately 40% of children in the world are exposed to ETS, which is related to allergic reactions in the short-term, while it is associated to acute myocardial infarction, lung cancer, and chronic obstructive pulmonary disease in the long term (Oberg et al., 2011).
Clinical studies show that ETS leads to behavioral disorders and deleterious effects on the brain. The exposure to ETS is related to attention deficits and hyperactive behavior during childhood (Pagani, 2014), while maternal smoke during lactation causes sleep and wake disruption (Banderali et al., 2015). Also, paternal smoke in the early postnatal period of childhood has been linked with perinatal mortality, respiratory disease, neurobehavioral problems, decreased academic performance, and brain tumors (Plichart et al., 2008; Hwang et al., 2012). Adolescents exposed to tobacco smoke during prenatal period show distinct brain function in the working memory and alterations in the brain volume, especially in the cerebellum (de Zeeuw et al., 2012; Bennett et al., 2013). In rodents, exposure to mainstream smoke during a critical period of brain development leads to hyperactivity and aggressive behavior (Yochum et al., 2014), while exposure to ETS disturbs cognitive functions, synaptic proteins, and myelination process from late infancy to early adulthood (Torres et al., 2015a, b).
Positron emission tomography (PET) is a molecular imaging technique that enables studying brain function in vivo. The 2-deoxy-2-[18F]-fluoro-D-glucose (18F-FDG) has been widely used to evaluate changes in cerebral glucose metabolism. 18F-FDG is required in metabolically active tissues, and the metabolic activity of a brain region is directly proportional to the amount of 18F-FDG that accumulates in this region (Sokoloff et al., 1977; Welch et al., 2013).
Relatively few studies evaluated the effects of tobacco smoke on brain glucose metabolism by PET imaging and focuses on dependence by nicotine in humans. In a context of tobacco craving and exposure to cues that are related to tobacco, heavy smokers showed rise in glucose metabolism in the anterior cingulate gyrus, orbitofrontal cortex, dorsolateral prefrontal cortex, anterior insula, and sensorimotor cortex (Brody et al., 2002). In addition, smokers treated with bupropion, a norepinephrine and dopamine reuptake inhibitor, showed decrease in glucose metabolism in the anterior cingulate cortex (Brody et al., 2004). Costello et al. (2010) reported that bupropion and practical group counseling reduce glucose metabolism in the posterior cingulate gyrus, with association between cigarette use and 18F-FDG uptake in the occipital gyrus and parietal–temporal junction (Costello et al., 2010). However, there is still a lack of studies evaluating the effects of tobacco smoke on glucose metabolism during the brain development period. Thus, our aim was to investigate the effects of ETS during the early postnatal period on glucose metabolism in a longitudinal preclinical study, by 18F-FDG PET imaging during mice infancy, adolescence, and adulthood.
Materials and Methods
Animals
C57BL/6 mice were obtained from the animal facility of the School of Medicine of University of São Paulo and were housed at 20–22°C with a 12 h/12 h light/dark cycle with water and commercial pellet food for small rodents from Nuvital (Nuvilab CR-1; Colombo, Brazil) ad libitum. All of the procedures were approved by the Ethics Committee of the School of Medicine (027/14) and the School of Pharmaceutical Sciences (P446/14), University of São Paulo.
Experimental Design
The size of each litter was randomly adjusted to six to seven pups within the first day after delivery, as previously described by Torres et al. (2015b). The C57BL/6 pups were exposed to ETS as described by Lobo-Torres et al. (2012). Briefly, the pups, together with their mothers, were subjected to two exposure sessions per day of 1-h each (1 h at 8 a.m. and 1 h at 5 p.m.) to a mixture of mainstream and sidestream tobacco smoke from reference cigarettes 3R4F (College of Agriculture, University of Kentucky). The exposure was performed from the 3rd (P3) to the 14th (P14) days of life, within a chamber measuring 564 × 385 × 371 mm. The levels of CO in the chamber during the exposure (470.2 ± 90.93 ppm) and measurements of the exposure biomarkers (COHb: 21.62 ± 1.80%; plasma nicotine: 139.94 ± 13.02 ng/mL; plasma cotinine: 113.65 ± 16.78 ng/mL) were similar to previous studies from our group (Torres et al., 2015a, b). Control subjects were exposed to the same experimental conditions but inhaled compressed air only.
Based on Vanhove et al. (2015), the number of animals required for imaging studies for changes about 20–25% is four to six animals. Thus, in the present study, we opted to use five animals of each sex in each group. Nonetheless, we used the isogenic C57Bl/6 mice in order to reduce intra-animal variability.
After the exposure period, 19 animals were used from P15 to P65 to evaluate the regional brain metabolism of the animals with 18F-FDG on PET/CT during infancy (P15; n = 5 females ETS-exposed and n = 4 females control; n = 5 males ETS-exposed and n = 5 males control), adolescence (P35; n = 4 females ETS-exposed and n = 4 females control; n = 5 males ETS-exposed and n = 5 males control), and adulthood (P65; n = 5 females ETS-exposed and n = 4 females control; n = 5 males ETS-exposed and n = 5 males control) in a longitudinal study.
18F-FDG-PET/CT Imaging
Positron emission tomography/CT images were acquired using a protocol modified from Welch et al. (2013). Briefly, animals received about 19 MBq (18.86 ± 2.70) of 18F-FDG intraperitoneally (i.p.). After 65 (67 ± 5) min of injection (biodistribution period of the radiotracer), animals were anesthetized with isoflurane (2% in O2) and positioned in an equipment bed with the brain in the center of the field of view (FOV). The scanner used was an Albira PET-SPECT-CT (Bruker Biospin, Valencia, Spain), for small animals. The static PET image was acquired for 50 min with 94.4 mm of trans-axial FOV. A CT scan was acquired immediately after, with 400 projections, 45 kVp, and 400 μA and magnification factor of 1.46. After acquisitions, PET images were reconstructed using maximum-likelihood expectation–maximization (MLEM), with 12 iterations, and corrected for radioactive decay, scatter, and random, but not for attenuation. CT was reconstructed using filtered back projection (FBP) algorithm.
PET Image Analysis
Positron emission tomography image analysis was performed with PMOD 3.4 software (PMODTM Technologies Ltd., Switzerland). The scans were manually co-registered to: (1) own animal CT for analysis in the different animals’ age and (2) to a T2 weighted MRI template (available in the PMOD software) in the adult animals’ analysis to facilitate the identification of different brain regions.
In the analysis of the brain in the different ages, manual volumes of interest (VOIs) were drawn in the PET images fused to the CT (Zovein et al., 2004). Due to the small size of the infant animals’ brain, to allow comparison with adolescent and adult mice, the VOIs for all ages were defined as whole brain, left and right brain hemispheres, frontal cortex, and cerebellum, always using the skull defined by the CT as a border line. When analysis was restricted to adult animals, PET image was co-registered to the MRI template and different brain regions considered in the analysis (whole brain, brainstem, cortex, cerebellum, left and right amygdala left and right striatum, left and right hippocampus, cingulate cortex, basal forebrain and septum, thalamus, hypothalamus, and left and right midbrain).
The 18F-FDG uptake is presented as a standardized uptake value (SUV) which is calculated as radioactivity concentration (kBq/cc) divided by the ratio between injected dose (kBq) and animal body weight (g).
Statistical Analysis
As in young animals it is difficult to analyze small brain areas, due to the limited PET imaging spatial resolution, and in order to compare infancy, adolescence, and adulthood, we analyzed brain glucose metabolism in the following brain areas: whole brain, left and right brain hemispheres, frontal cortex, and cerebellum. Thus, we performed a three-way mixed ANOVA with repeated measures, considering groups as between and time and VOIs (whole brain, frontal cortex, cerebellum, right hemisphere, and left hemisphere) in infancy, adolescence, and adulthood as within-subject factors. Bonferroni post hoc test with multiple comparison correction was performed to test 18F-FDG uptake differences between the time points and groups for each VOI (Figure 1). PET imaging of adult animals was analyzed by a two-way ANOVA, considering groups as between and VOIs (whole brain, brainstem, cortex, cerebellum, amygdala, striatum, hippocampus, cingulate cortex, basal forebrain and septum, thalamus, hypothalamus, and midbrain) as within-subject factors. Bonferroni post hoc with multiple comparison correction was performed to test 18F-FDG uptake differences between the groups for each VOI (Figure 3). The data were analyzed using SPSS Statistics 20 Software, Armonk, NY: IBM Corp., United States and data were plotted using GraphPad Prism 6 Software, La Jolla, CA, United States. Results are presented as mean ± standard error. Differences with a probability of 95% (p < 0.05) were considered statistically significant.
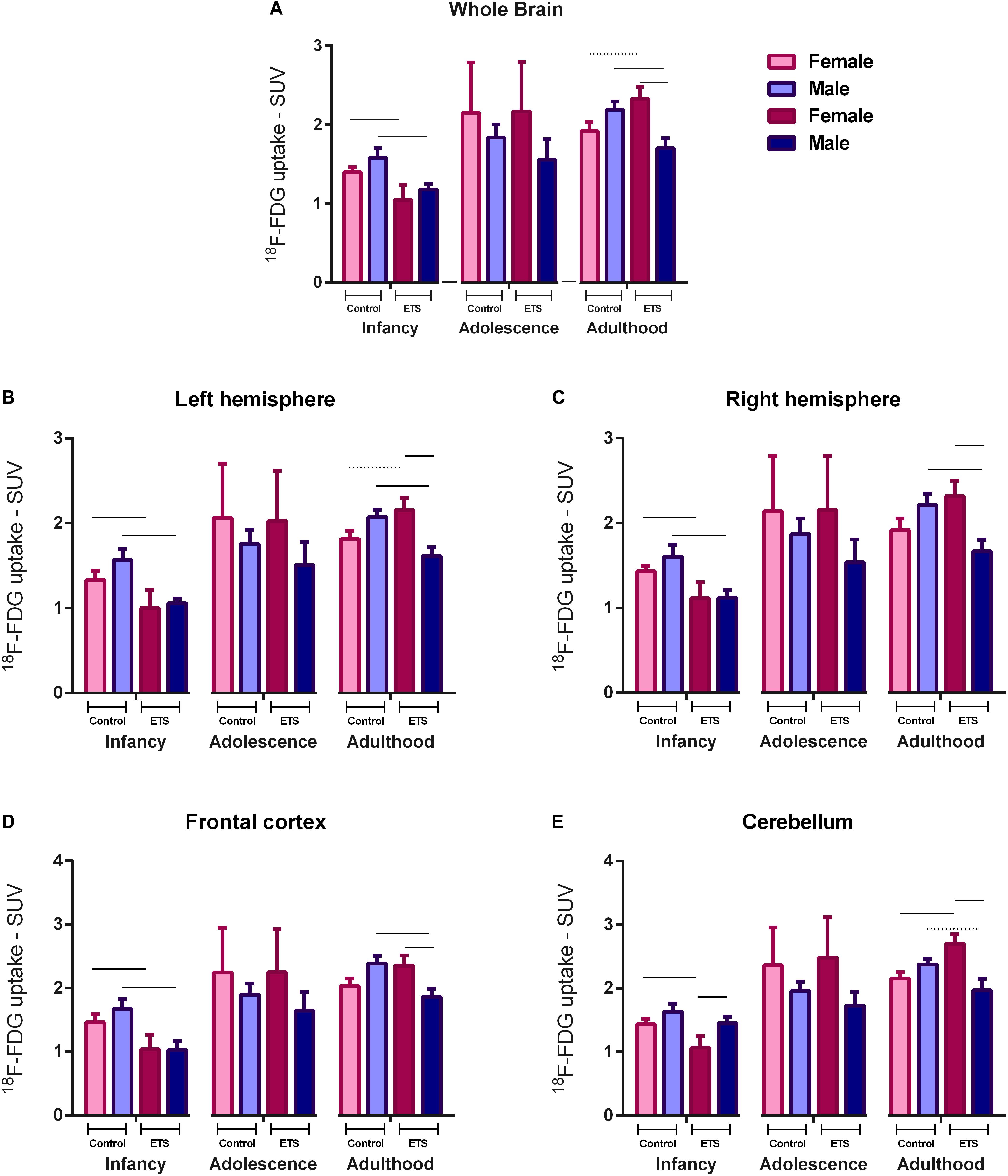
Figure 1. 18F-FDG uptake in the whole brain (A), left hemisphere (B), right hemisphere (C), frontal cortex (D), and cerebellum (E) for female and male infant (n = 5 females ETS-exposed and n = 4 females control; n = 5 males ETS-exposed and n = 5 males control), adolescent (n = 4 females ETS-exposed and n = 4 females control; n = 5 males ETS-exposed and n = 5 males control), and adult (n = 5 females ETS-exposed and n = 4 females control; n = 5 males ETS-exposed and n = 5 males control) mice exposed to ETS during the early postnatal period. Three-way mixed ANOVA with repeated measures (groups × VOIs) and post hoc paired t-test (Bonferroni) with multiple comparison correction. Continuous bar: p < 0.05. Dashed bar: trend toward statistical significance.
Results
ETS During the Early Postnatal Period Decreased Brain 18F-FDG Uptake in Infant Mice
Positron emission tomography scan data of glucose uptake for male and female infant, adolescent, and adult mice exposed to ETS during the early postnatal period were analyzed by a three-way mixed ANOVA with repeated measures (groups × VOIs) and Bonferroni post hoc test with multiple comparison correction. We found a significant effect for the factors VOIs (F4,56 = 33.7333; p < 0.00010) and time (F2,8 = 10.99; p < 0.0001), and a significant VOIs × time interaction (F8,112 = 4.592, p < 0.0001). We also had a significant VOIs × time × group interaction (F24,112 = 1.753; p < 0.05).
The post hoc analysis revealed that all mice showed reduced 18F-FDG uptake in infancy, compared to adulthood in all analyzed VOIs. Regarding the females of ETS group, the 18F-FDG uptake was also lower during infancy when compared to adolescence. As detailed at Table 1, in infancy, both male and female mice ETS-exposed had lower 18F-FDG uptake in the whole brain, left and right hemisphere and frontal cortex, compared to the control group. Females showed decreased 18F-FDG uptake in the cerebellum (Figure 1 and Table 1). When mice reached adulthood, males showed a reduction in 18F-FDG uptake when compared to controls in all VOIs analyzed (Figure 1 and Table 1). However, in the females a different pattern occurred, as they had higher 18F-FDG uptake in the whole brain, left hemisphere, and cerebellum, when compared to the control group (Figure 1 and Table 1). When both ETS-exposed groups were compared, the post hoc analysis showed a reduction in 18F-FDG uptake in the males in all the VOIs evaluated when compared to females (Figure 1 and Table 1). Figure 2 shows a representative brain PET/CT scans of mice exposed to ETS during the early postnatal period and the control group during infancy, adolescence, and adulthood.
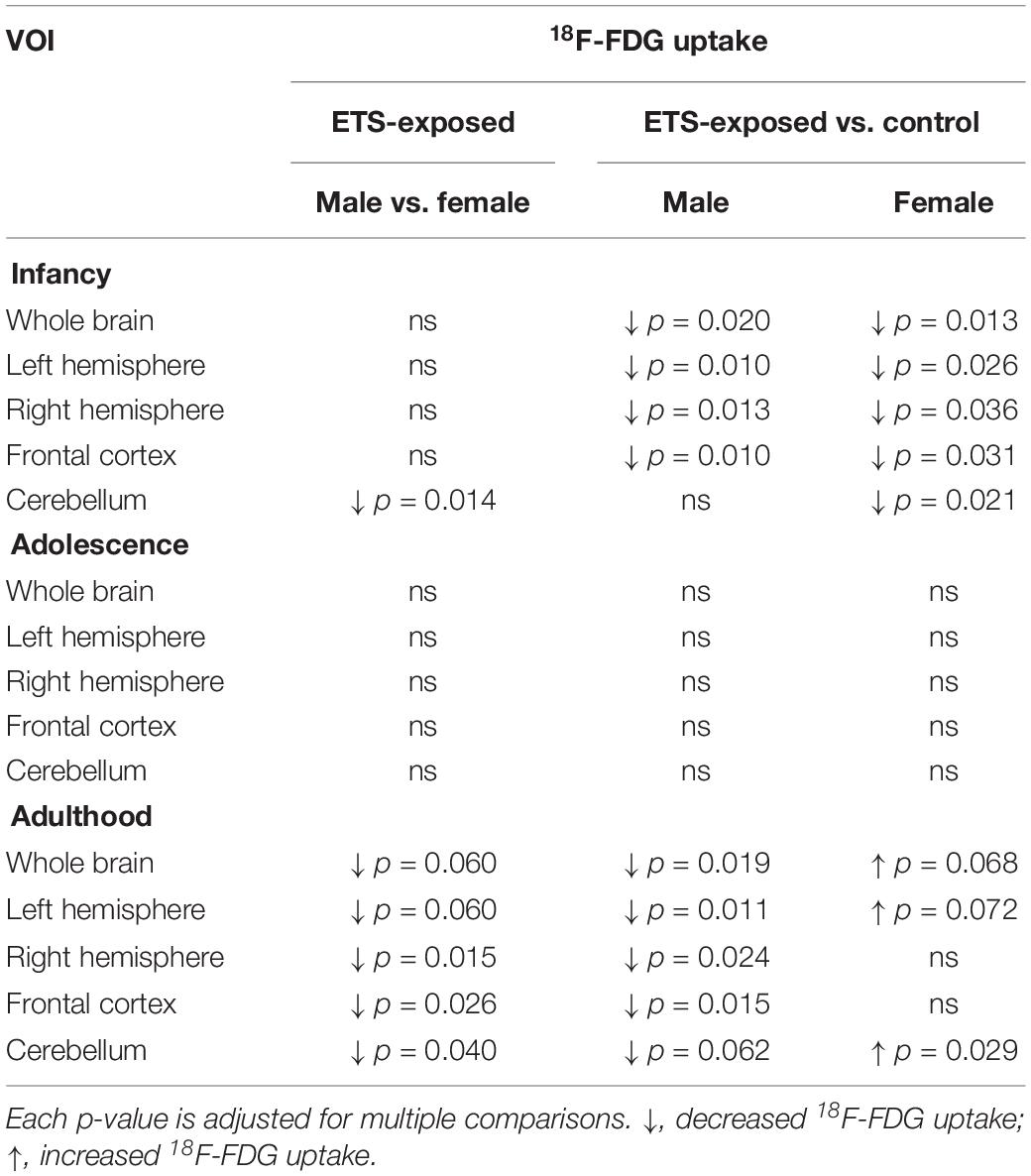
Table 1. Detailed description of the statistical analysis of 18F-FDG uptake in infancy, adolescence, and adulthood mice in distinct brain regions.
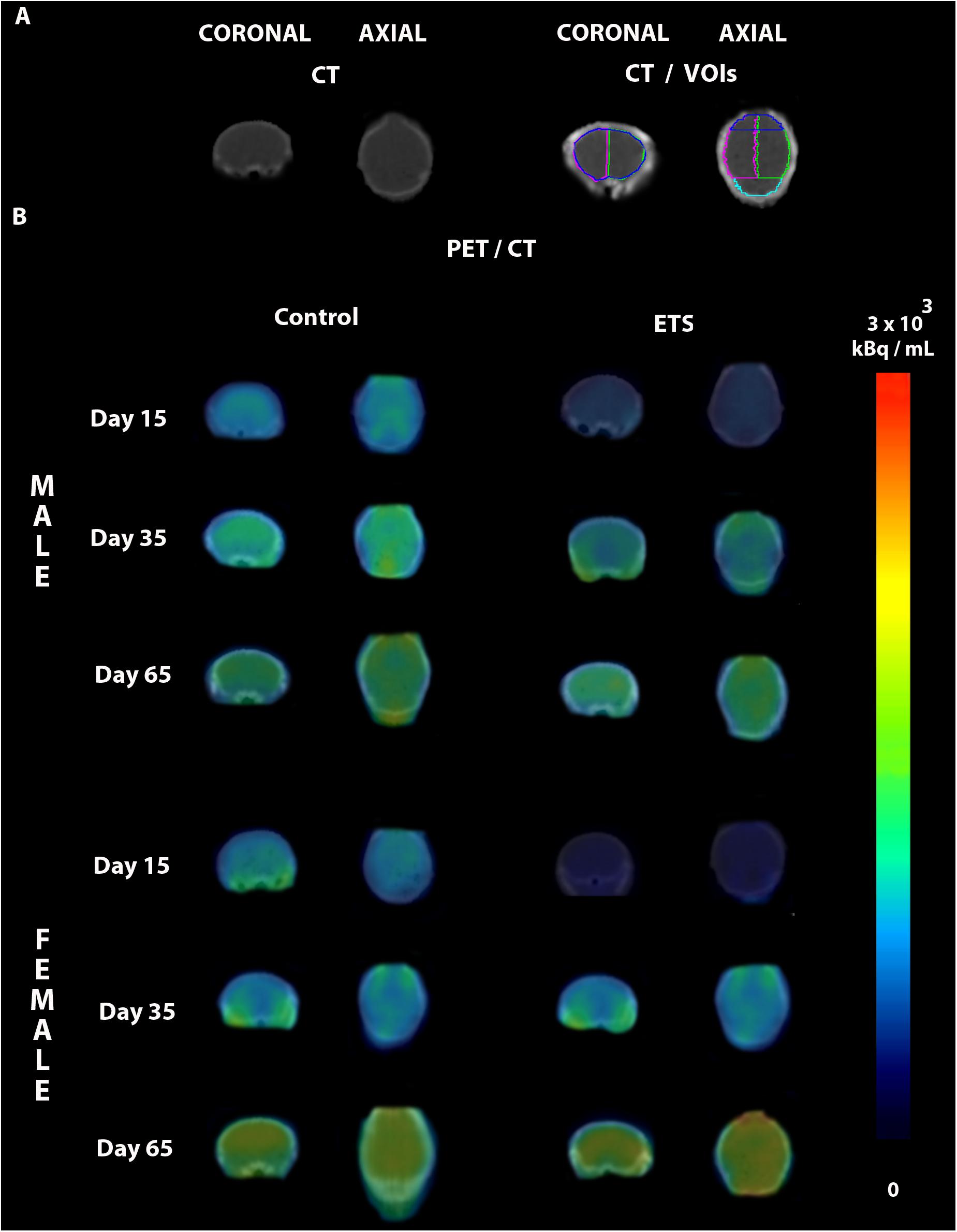
Figure 2. Representative PET/CT brain images of male and female mice exposed to ETS during the early postnatal period and the control group. (A) CT images in different anatomical planes in the top left, and CT with the drawn volumes of interest (VOIs) used for quantification in the top right (whole brain, left hemisphere, right hemisphere, frontal cortex, and cerebellum). (B) PET images fused to the CT images of infant (Day 15), adolescent (Day 35), and adult (Day 65) male and female mice of the control and ETS groups.
ETS During the Early Postnatal Period Decreased 18F-FDG Uptake in Adult Male Mice in Distinct Brain Regions
Positron emission tomography scan data of glucose uptake for male and female adult mice exposed to ETS during the early postnatal period were analyzed by two-way ANOVA (VOIs × treatment) with Bonferroni post hoc test with p-values corrected for multiplicity. We found a significant effect for VOIs (F15,240 = 7.101, p < 0.0001) and treatment (F3,240 = 59.5, p < 0.0001) factors; however, the interaction between them was not significant (F45,240 = 0.033, p > 0.999; see Table 2 for detailed description of the statistical analysis).
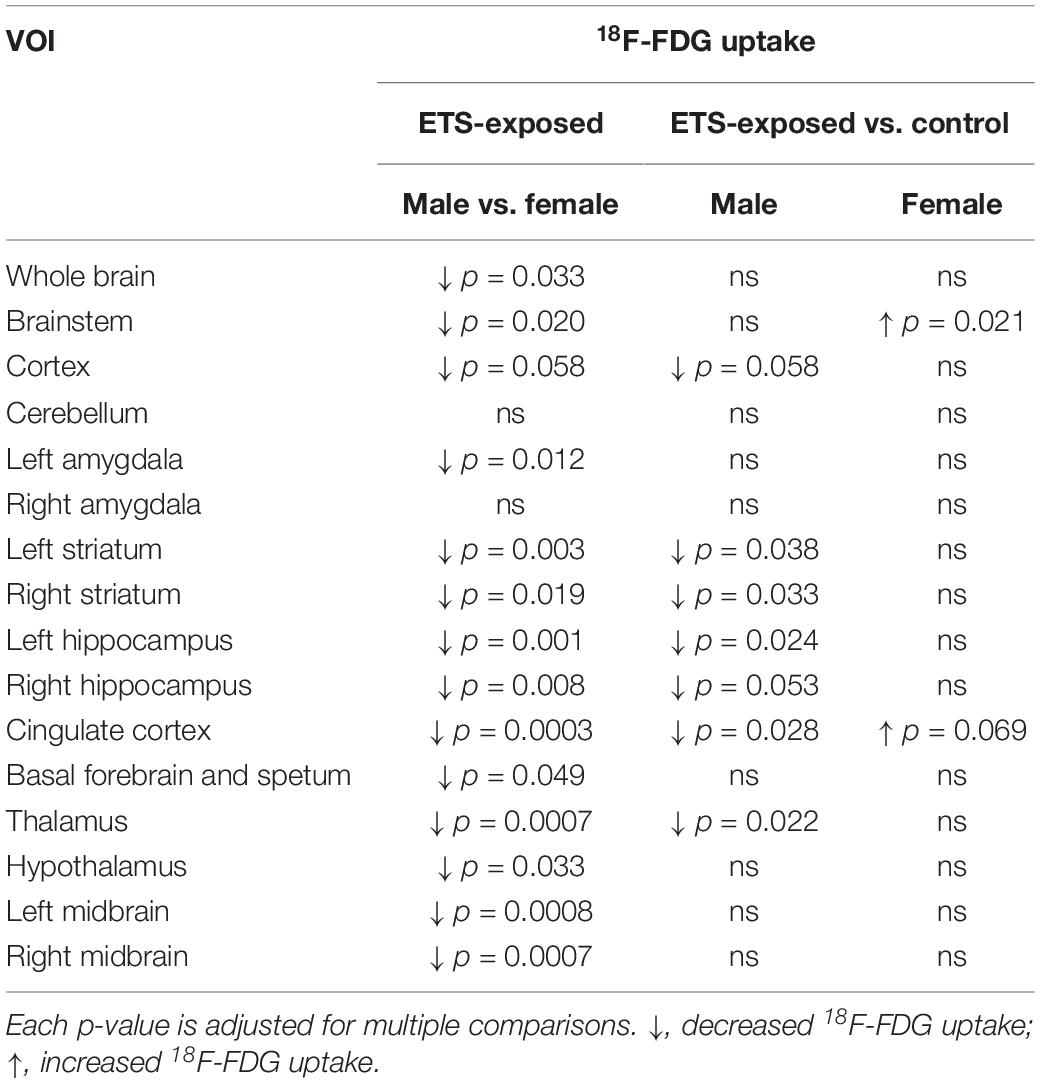
Table 2. Detailed description of the statistical analysis of 18F-FDG uptake in adult mice in distinct brain regions.
The post hoc analysis showed that exposure to ETS during the early postnatal period decreased 18F-FDG uptake in adult male mice when compared with adult female mice in the whole brain, brainstem, left amygdala, left and right striatum, left and right hippocampus, cingulate cortex, basal forebrain and septum, thalamus, hypothalamus, and left and right midbrain. There was a trend of statistical significance in the cortex (Figure 3 and Table 2). We also observed that adult male mice exposed to ETS showed a decrease in glucose metabolism when compared with male mice from the control group in the left and right striatum, left hippocampus, cingulate cortex, and thalamus (Figure 3 and Table 2). It was also detected a trend toward statistical significance in the cortex, and right hippocampus (Figure 3 and Table 2). Regarding adult female mice exposed to ETS, we observed an increase in glucose uptake when compared with female mice from the control group in the brainstem, with trend toward statistical significance in the cingulate cortex. Figure 4 shows brain PET/CT average scans of female and male adult mice exposed to ETS during the early postnatal period and the control group.
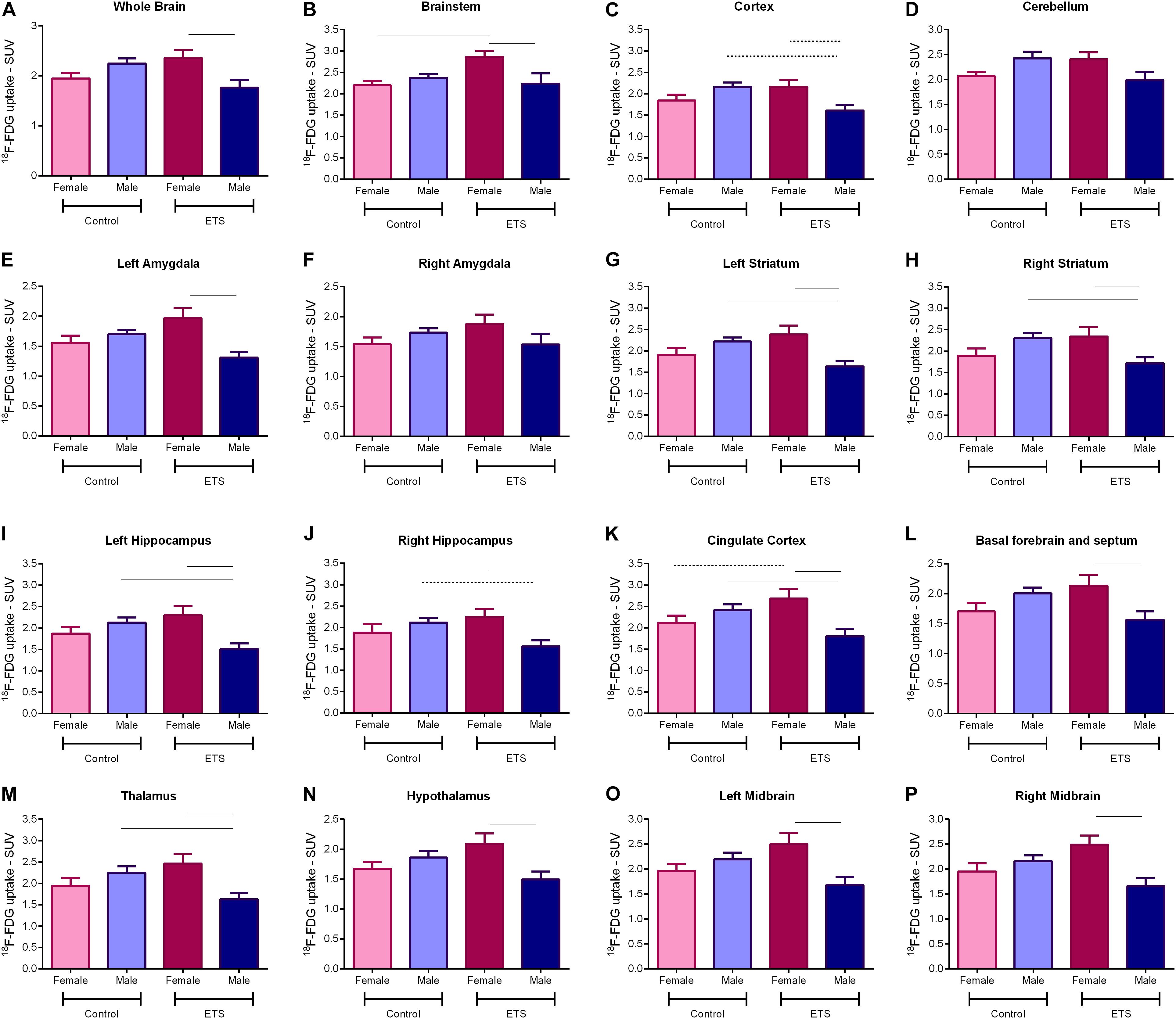
Figure 3. 18F-FDG uptake for adult mice (n = 5 females ETS-exposed and n = 4 females control; n = 5 males ETS-exposed and n = 5 males control) in the whole brain (A), brainstem (B), cortex (C), cerebellum (D), left amygdala (E), right amygdala (F), left striatum (G), right striatum (H), left hippocampus (I), right hippocampus (J), cingulate cortex (K), basal forebrain and septum (L), thalamus (M), hypothalamus (N), left midbrain (O), and right midbrain (P). Two-way ANOVA with Bonferroni post hoc test and adjusted p-values for multiple comparisons. Continuous bar: p < 0.05. Dashed bar: trend toward statistical significance.
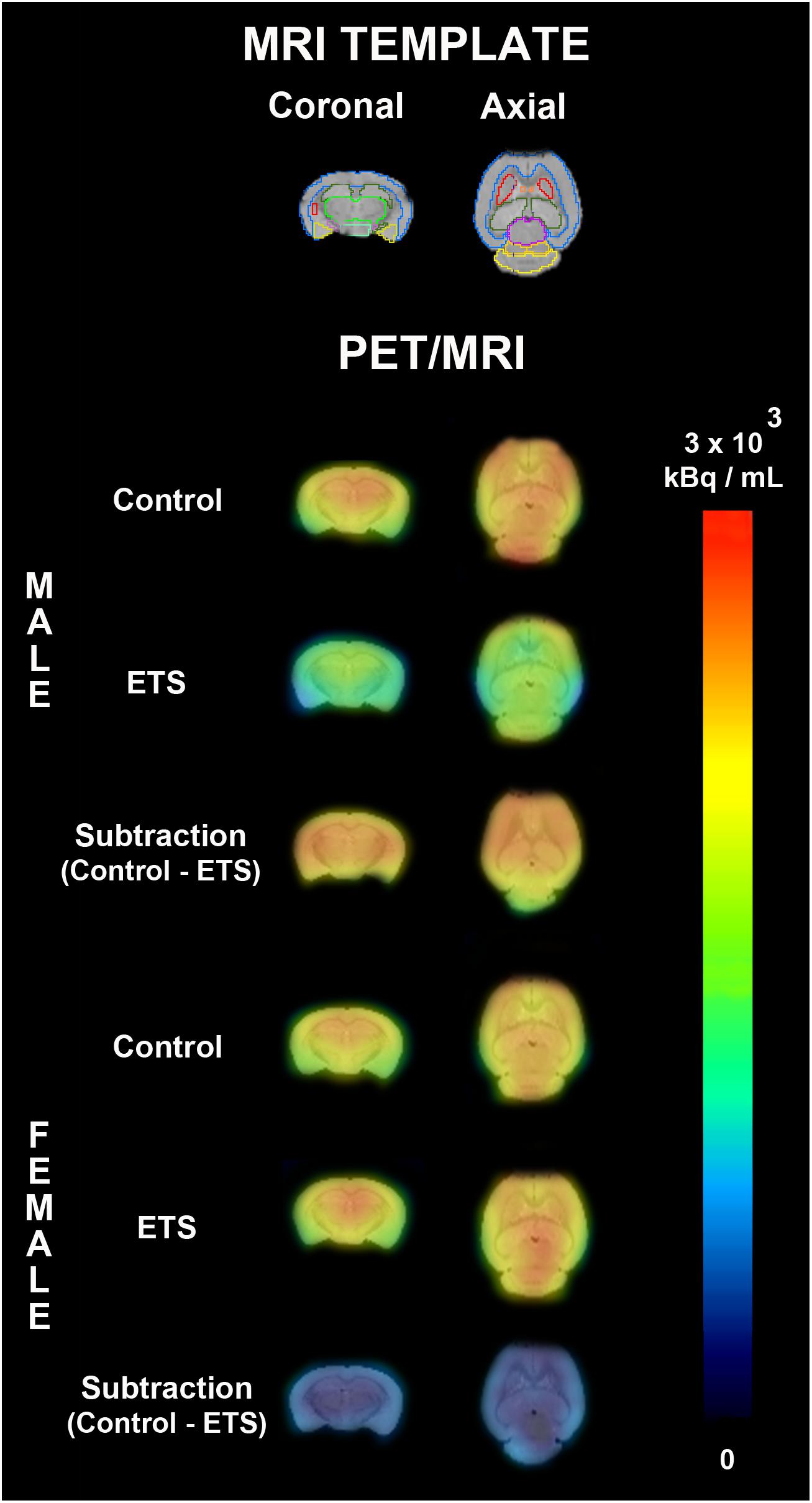
Figure 4. PET average images of female and male adult mice exposed to ETS during the early postnatal period and the control group. On the top, a MRI template used for drawing volumes of interest (whole brain, brainstem, cortex, cerebellum, left amygdala, right amygdala, left striatum, right striatum, left hippocampus, right hippocampus, cingulate cortex, basal forebrain and septum, thalamus, hypothalamus, left midbrain, and right midbrain). The first two rows represent PET average images fused to MRI template and the third row represent the difference of PET average image fused to MRI template between control group and ETS exposed mice.
Discussion
To the best of our knowledge, this is the first study that investigated the effects of ETS exposure during brain development on 18F-FDG uptake in the brain of mice. By PET imaging, we observed that ETS exposure during the early postnatal period decreased brain 18F-FDG uptake in both male and female infant mice and in adult male mice in distinct brain regions and increased 18F-FDG uptake in adult female mice in the brainstem and cingulate cortex. In addition, male ETS-exposed mice showed decreased 18F-FDG uptake when compared to female ETS-exposed. These results are in accordance with previous studies of our group and with studies that show that exposure to tobacco smoke during brain development can affect the central nervous system. Exposure to tobacco smoke extract during gestational period of Sprague–Dawley rats decreased nicotinic and serotonin receptors in different brain regions (Slotkin et al., 2017). In addition, postnatal exposure to tobacco smoke leads to impairment in the myelination, learning, and memory, and induces oxidative stress and lower brain-derived neurotrophic factor (BDNF) and synaptic proteins levels (Stangherlin et al., 2009; Lobo-Torres et al., 2012; Torres et al., 2015a, b).
The exposure biomarkers of the present study were similar to Obot et al. (2004), Amos-Kroohs et al. (2013), and Torres et al. (2015a, b, 2019a, 2019b). Nwosu and Kum-Nji (2018) suggested that the classification as passive or active smoking could be done according to serum cotinine levels. Thus, serum cotinine levels between 0.05 and 10 ng/mL can be considered as passive smokers and >10 ng/mL as active smoking. Although cotinine concentration of the present study could be considered as active smoker by Nwosu and Kum-Nji (2018), the classification was based in children and adolescent under 17 years old and not in rodents. Moreover, the authors did not mention how long after tobacco smoke exposure the blood was collected. In the present study, due to the weaker binding affinity of CO for mouse hemoglobin when compared to that of human hemoglobin (Watson et al., 1987), blood collection for the quantification of the biological markers was performed immediately after the ETS exposure. Thus, the cotinine levels data reflect the peak of cotinine, as the half-life of plasma nicotine in rodents is 0.9–1.1 h.
Neuronal activity requires high energy, mainly in the level of synaptic connections and signaling transduction pathways (Sokoloff, 1999). In this scenario, 18F-FDG brain uptake correlates with brain metabolic activity, since we can predict brain function through the relationship between energy consumption and neuronal activity (Shulman et al., 2004). Small animal PET imaging in longitudinal studies allows in vivo quantification of brain metabolic activity in the same animal during different periods of life making possible to analyze how brain behave in different stages and how xenobiotics might be able to interfere in the homeostatic state. Our data showed decreased 18F-FDG uptake in infancy, suggesting that ETS exposure is affecting brain neuronal activity during this important period of brain development. In fact, it is known that in humans, childhood is a period in which the central nervous system is under active development, maturation, and with open critical periods of synaptic plasticity, with the higher levels of metabolism, reaching a peak on the fourth year of life (Chugani and Phelps, 1991). Until the ninth year of life there is a plateau, followed by a steady decline until adulthood, in the second decade of life, when the prefrontal cortex has completed its maturation (Kennedy and Sokoloff, 1957). During normal aging, brain passes through structural and function changes in white and gray matters, reflecting in a declined metabolic activity (Moeller et al., 1996).
It is interesting to note that even a long time after the last exposure, adult mice showed changes in 18F-FDG uptake in distinct brain regions, which were sex dependent. Adult male mice exposed to ETS during brain development showed decreased 18F-FDG uptake compared with adult female mice or with male controls in different brain regions. In fact, sex seems to be an important factor in brain response to ETS. A clinical study showed that nicotine administered by patch induced increased brain 18F-FDG uptake in females than males during a Continuous Performance Task or the Bushman Competition and Retaliation Task tests (Fallon et al., 2005). A previous study of our group also showed that the effects of ETS exposure during the early postnatal period are sex-dependent, as infant female mice showed poorer performance in learning and memory tests than males (Torres et al., 2015b).
Garcia et al. (2013) revealed that sex is determinant for post-hypoxic depression and recovery. Persistent post-hypoxic depression is more recurrent in male mice than female and glucose supplementation improves post-hypoxic recovery rhythmogenesis only in female mice (Garcia et al., 2013). These data are relevant, since post-hypoxic depression is involved in the pathogenesis of sudden infant death syndrome (SIDS). About 60% of the children affected by SIDS are male (Richardson et al., 2010). The exposure to ETS is related to higher risk of SIDS, a syndrome that has no known mechanism, but it requires immature cardiorespiratory control and impairments in sleep arousal (Mitchell and Milerad, 2006; Moon et al., 2016). The brainstem is associated with respiratory and cardiovascular responses, therefore is related to pathogenesis of SIDS and it is susceptible to ETS exposure. Previous studies showed that exposure to ETS in the early postnatal period decreased myelin-specific proteins and alter receptors and enzymes of the endocannabinoid system in the brainstem (Torres et al., 2015a, 2019a). The present study corroborates these findings since we observed that ETS exposure decreased 18F-FDG uptake in ETS-exposed male mice compared with female mice and increased 18F-FDG uptake in ETS-exposed female mice compared with control group.
Environmental tobacco smoke exposure during the early postnatal period induced a similar result in the left amygdala, which have a key role in the acquisition of memory related to fear conditioning (Fujisaki et al., 2004). Amygdala is associated to emotional experience, including fear and anxiety (De Bellis et al., 2000; de Oliveira et al., 2013). In fact, pediatric generalized anxiety disorder was associated to higher amygdala volumes (De Bellis et al., 2000). Active smokers have significantly reduced amygdala volumes compared with non-smokers (Luhar et al., 2013). In line with these results, preclinical studies have shown that the exposure to ETS during postnatal periods leads to anxiety-like behavior in a short- and long-term withdrawal (Abreu-Villaça et al., 2015; de la Peña et al., 2016; Torres et al., 2019b). Taken together, these data suggest that the anxiety behavioral disorders related to tobacco smoke may be associated with amygdala alterations.
In the present study, we observed that exposure to ETS decreased 18F-FDG uptake in striatum of adult male mice. This data are consistent with studies that revealed that ETS exposure during a critical period induced oxidative stress, decreased synaptic proteins levels and BDNF, and modified elements of the endocannabinoid system in the striatum (Lobo-Torres et al., 2012; Torres et al., 2019a, b). The striatum, constituted by the caudate nucleus and putamen, is involved in motor, cognitive, and limbic functions, and is involved in the neurobiology of addiction (Burton et al., 2015). Addictive drugs increase dopamine levels in mesolimbic system, especially in the dorsal and ventral striatum/nucleus accumbens (Hyman et al., 2006). Romoli et al. (2019) reported that exposure to nicotine during the early postnatal period increased nicotine consumption during adulthood, effect mediated by dopaminergic neurons in the midbrain, that contains dopaminergic neurons that are located in the ventral tegmental area and in the substantia nigra, regions that are important for the development of drug addiction (Björklund and Dunnett, 2007; Romoli et al., 2019).
Weinstein et al. (2010) observed that smokers showed increased craving scores after watching a videotape with smoking scenes, which were associated with brain 18F-FDG uptake in the ventral striatum, anterior cingulate, orbitofrontal cortex, middle temporal lobe, hippocampus, insula, midbrain, and thalamus (Weinstein et al., 2010). In addition, Domino et al. (2000) showed that nicotine increases regional cerebral blood flow, evaluated by PET, in the thalamus, pons, primary visual cortex, and cerebellum of tobacco smokers. These individuals also showed reduction in the hippocampal area (Domino et al., 2000; Hanlon et al., 2014). We observed that ETS decreased glucose metabolism in ETS-exposed male mice compared with female mice and with control group in the hippocampus, one of the main areas that is involved in learning and memory. Indeed, ETS exposure during the early postnatal period decreased synaptic proteins and BDNF in hippocampus and induced impairment in the learning and memory from late infancy to early adulthood (Torres et al., 2015b).
It is important to point out the limitations of the present protocol. In order to evaluate the long-lasting effect of tobacco smoke exposure during the early postnatal period, we used a longitudinal study to measure 18F-FDG uptake from infancy to adulthood. Although longitudinal studies have the advantage of evaluating the same animal throughout life, this type of protocol does not allow other measures to be performed, since euthanasia is only done when the animal reaches adulthood.
In summary, we showed that several brain regions are vulnerable to ETS exposure during the early postnatal period and these effects on 18F-FDG uptake were observed even a long time after the last exposure. This study corroborates our previous studies, supporting the hypothesis that exposure to tobacco smoke in a critical period interferes with brain development of mice from late infancy to early adulthood.
Data Availability Statement
The datasets generated for this study are available on request to the corresponding author.
Ethics Statement
The animal study was reviewed and approved by the Ethics Committee of the School of Medicine (027/14) and the School of Pharmaceutical Sciences (P446/14), University of São Paulo.
Author Contributions
LT and TM conceived and designed the project. LT, LS, AS, and TA performed all the experiments related to exposure to tobacco smoke and biomarkers quantification under TM supervision. LT, WT, LP, and PS performed the experiments related to PET/CT image acquisition. WT, CR, and DP performed the image processing and analysis. LT, WT, MP, CR, DP, and TM wrote and edited the manuscript. All authors contributed to the manuscript revision, and read and approved the submitted version of the manuscript.
Funding
This work was supported by the São Paulo Research Foundation (Fundação de Amparo à Pesquisa do Estado de São Paulo – FAPESP, Grants 2013/08881-9 and 2013/12769-7); Coordination for the Improvement of Higher Education Personnel (Coordenação de Aperfeiçoamento de Pessoal de Nível Superior – Brazil – CAPES – Finance Code 001); and the National Counsel of Technological and Scientific Development (Conselho Nacional de Desenvolvimento Científico e Tecnológico – CNPq Grant 405353/2016-2). TM is a research fellow of CNPq.
Conflict of Interest
The authors declare that the research was conducted in the absence of any commercial or financial relationships that could be construed as a potential conflict of interest.
Acknowledgments
The authors gratefully acknowledge Fábio Luís de Souza Duran for his technical assistance.
References
Abreu-Villaça, Y., Filgueiras, C. C., Correa-Santos, M., Cavina, C. C., Naiff, V. F., Krahe, T. E., et al. (2015). Tobacco smoke containing high or low levels of nicotine during adolescence: effects on novelty-seeking and anxiety-like behaviors in mice. Psychopharmacology 232, 1693–1703. doi: 10.1007/s00213-014-3801-1
Amos-Kroohs, R. M., Williams, M. T., Braun, A. A., Graham, D. L., Webb, C. L., Birtle, T. S., et al. (2013). Neurobehavioral phenotype of C57BL/6J mice prenatally and neonatally exposed to cigarette smoke. Neurotoxicol. Teratol. 35, 34–45. doi: 10.1016/j.ntt.2013.01.001
Banderali, G., Martelli, A., Landi, M., Moretti, F., Radaelli, G., Lassandro, C., et al. (2015). Short and long term health effects of parental tobacco smoking during pregnancy and lactation: a descriptive review. J. Transl. Med. 13:327. doi: 10.1186/s12967-015-0690-y
Bennett, D. S., Mohamed, F. B., Carmody, D. P., Malik, M., Faro, S. H., and Lewis, M. (2013). Prenatal tobacco exposure predicts differential brain function during working memory in early adolescence: a preliminary investigation. Brain Imaging Behav. 7, 49–59. doi: 10.1007/s11682-012-9192-1
Björklund, A., and Dunnett, S. B. (2007). Dopamine neuron systems in the brain: an update. Trends Neurosci. doi: 10.1016/j.tins.2007.03.006
Brody, A. L., Mandelkern, M. A., Lee, G., Smith, E., Sadeghi, M., Saxena, S., et al. (2004). Attenuation of cue-induced cigarette craving and anterior cingulate cortex activation in bupropion-treated smokers: a preliminary study. Psychiatry Res. Neuroimaging 130, 269–281. doi: 10.1016/j.pscychresns.2003.12.006
Brody, A. L., Mandelkern, M. A., London, E. D., Childress, A. R., Lee, G. S., Bota, R. G., et al. (2002). Brain Metabolic changes during cigarette craving. Arch. Gen. Psychiatry 59, 1162–1172. doi: 10.1001/archpsyc.59.12.1162
Burton, A. C., Nakamura, K., and Roesch, M. R. (2015). From ventral-medial to dorsal-lateral striatum: neural correlates of reward-guided decision-making. Neurobiol. Learn. Mem. 117, 51–59. doi: 10.1111/j.1743-6109.2008.01122.x.Endothelial
Chugani, H. T., and Phelps, M. E. (1991). Imaging human brain development with positron emission tomography. J. Nucl. Med. 32, 23–26.
Costello, M. R., Mandelkern, M. A., Shoptaw, S., Shulenberger, S., Baker, S. K., Abrams, A. L., et al. (2010). Effects of treatment for tobacco dependence on resting cerebral glucose metabolism. Neuropsychopharmacology 35, 605–612. doi: 10.1038/npp.2009.165
De Bellis, M. D., Casey, B. J., Dahl, R. E., Birmaher, B., Williamson, D. E., Thomas, K. M., et al. (2000). A pilot study of amygdala volumes in pediatric generalized anxiety disorder. Biol. Psychiatry 48, 51–57. doi: 10.1016/S0006-3223(00)00835-0
de la Peña, J. B., Ahsan, A. H., Botanas, C. J., dela Peña, I. J., Woo, T., and Kim, H. J. (2016). Cigarette smoke exposure during adolescence but not adulthood induces anxiety-like behavior and locomotor stimulation in rats during withdrawal. Int. J. Dev. Neurosci. 55, 49–55. doi: 10.1016/j.ijdevneu.2016.09.007
de Oliveira, A. R., Reimer, A. E., Reis, F. M., and Brandão, M. L. (2013). Conditioned fear response is modulated by a combined action of the hypothalamic–pituitary–adrenal axis and dopamine activity in the basolateral amygdala. Eur. Neuropsychopharmacol. 23, 379–389. doi: 10.1016/J.EURONEURO.2012.05.007
de Zeeuw, P. D., Zwart, F., Schrama, R., Engeland, H. V., and Durston, S. (2012). Prenatal exposure to cigarette smoke or alcohol and cerebellum volume in attention-deficit/hyperactivity disorder and typical development. Transl. Psychiatry 2, e84–e89. doi: 10.1038/tp.2012.12
Domino, E. F., Minoshima, S., Guthrie, S., Ohl, L., Ni, L., Koeppe, R. A., et al. (2000). Nicotine effects on regional cerebral, blood flow in awake, resting tobacco smokers. Synapse 38, 313–321. doi: 10.1002/1098-2396(20001201)38:3<313::AID-SYN10>3.0.CO;2-6
Fallon, J. H., Keator, D. B., Mbogori, J., Taylor, D., and Potkin, S. G. (2005). Gender: a major determinant of brain response to nicotine. Int. J. Neuropsychopharmacol. 8, 17–26. doi: 10.1017/S1461145704004730
Fujisaki, M., Hashimoto, K., Iyo, M., and Chiba, T. (2004). Role of the amygdalo-hippocampal transition area in the fear expression: evaluation by behavior and immediate early gene expression. Neuroscience 124, 247–260. doi: 10.1016/j.neuroscience.2003.11.022
Garcia, A. J., Rotem-Kohavi, N., Doi, A., and Ramirez, J. M. (2013). Post-hypoxic recovery of respiratory rhythm generation is gender dependent. PLoS One 8:e60695. doi: 10.1371/journal.pone.0060695
Hanlon, C. A., Dowdle, L. T., Naselaris, T., Canterberry, M., and Cortese, B. M. (2014). Visual cortex activation to drug cues: a meta-analysis of functional neuroimaging papers in addiction and substance abuse literature. Drug Alcohol Depend. 143, 206–212. doi: 10.1016/J.DRUGALCDEP.2014.07.028
Hwang, S., Hwang, J. H., Moon, J. S., and Lee, D. (2012). Environmental tobacco smoke and children’s health. Korean J. Pediatr. 55, 35–41. doi: 10.3345/kjp.2012.55.2.35
Hyman, S. E., Malenka, R. C., and Nestler, E. J. (2006). Neural mechanisms of addiction: the role of reward-related learning and memory. Ann. Rev. Neurosci. 29, 565–98. doi: 10.1146/annurev.neuro.29.051605.113009
Kennedy, C., and Sokoloff, L. (1957). An adaptation of the nitrous oxide method to the study of the cerebral circulation in children: normal values for cerebral blood flow and cerebral metabolic rate in childhood. J. Clin. Invest. 36, 1130–1137. doi: 10.1172/JCI103509
Lobo-Torres, L. H., Moreira, W. L., Garcia, R. C. T., Annoni, R., Carvalho, A. L. N., Teixeira, S. A., et al. (2012). Environmental tobacco smoke induces oxidative stress in distinct brain regions of infant mice. J. Toxicol. Environ. Health Part A 75, 971–980. doi: 10.1080/15287394.2012.695985
Luhar, R. B., Sawyer, K. S., Gravitz, Z., Ruiz, S. M., and Oscar-Berman, M. (2013). Brain volumes and neuropsychological performance are related to current smoking and alcoholism history. Neuropsychiatr. Dis. Treat. 9, 1767–1784. doi: 10.2147/NDT.S52298
Mitchell, E. A., and Milerad, J. (2006). Smoking and the sudden infant death syndrome. Rev. Environ. Health 21, 81–103. doi: 10.1515/REVEH.2006.21.2.81
Moeller, J. R., Ishikawa, T., Dhawan, V., Spetsieris, P. G., Mandel, F., Alexander, G. E., et al. (1996). The metabolic topography of normal aging. J. Cereb. Blood Flow Metab. 16, 385–398. doi: 10.1097/00004647-199605000-00005
Moon, R. Y., Darnall, R. A., Feldman-Winter, L., Goodstein, M. H., and Hauck, F. R. (2016). SIDS and other sleep-related infant deaths: evidence base for 2016 updated recommendations for a safe infant sleeping environment. Pediatrics 138:e20162940. doi: 10.1542/peds.2016-2940
Nwosu, B. U., and Kum-Nji, P. (2018). Tobacco smoke exposure is an independent predictor of vitamin D deficiency in US children. PLoS One 13:e0205342. doi: 10.1371/journal.pone.0205342
Oberg, M., Jaakkola, M. S., Woodward, A., Peruga, A., and Prüss-Ustün, A. (2011). Worldwide burden of disease from exposure to second-hand smoke: a retrospective analysis of data from 192 countries. Lancet 377, 139–146. doi: 10.1016/S0140-6736(10)61388-8
Obot, C. J., Lee, K. M., Fuciarelli, A. F., Renne, R. A., and McKinney, W. J. (2004). Characterization of mainstream cigarette smoke-induced biomarker responses in ICR and C57BI/6 mice. Inhal. Toxicol. 16, 701–719. doi: 10.1080/08958370490476604
Pagani, L. S. (2014). Environmental tobacco smoke exposure and brain development: the case of attention deficit/hyperactivity disorder. Neurosci. Biobehav. Rev. 44, 195–205. doi: 10.1016/j.neubiorev.2013.03.008
Plichart, M., Menegaux, F., Lacour, B., Hartmann, O., Frappaz, D., Doz, F., et al. (2008). Parental smoking, maternal alcohol, coffee and tea consumption during pregnancy and childhood malignant central nervous system tumours: the ESCALE study (SFCE). Eur. J. Cancer Prev. 17, 376–383. doi: 10.1097/CEJ.0b013e3282f75e6f
Richardson, H. L., Walker, A. M., and Horne, R. S. C. (2010). Sleeping like a baby – does gender influence infant arousability? Sleep 33, 1055–1060. doi: 10.1093/sleep/33.8.1055
Romoli, B., Lozada, A. F., Sandoval, I. M., Manfredsson, F. P., Hnasko, T. S., and Berg, D. K. (2019). Neonatal nicotine exposure primes midbrain neurons to a dopaminergic phenotype and increases adult drug consumption. Biol. Psychiatry 86, 344–355. doi: 10.1016/j.biopsych.2019.04.019
Shulman, R. G., Rothman, D. L., Behar, K. L., and Hyder, F. (2004). Energetic basis of brain activity: implications for neuroimaging. Trends Neurosci. 27, 489–495. doi: 10.1016/j.tins.2004.06.005
Slotkin, T. A., Stadler, A., Skavicus, S., Card, J., Ruff, J., and Levin, E. D. (2017). Is there a critical period for the developmental neurotoxicity of low-level tobacco smoke exposure? Toxicol. Sci. 155, 75–84. doi: 10.1093/toxsci/kfw180
Sokoloff, L. (1999). Energetics of functional activiation in neural tissues. Neurochem. Res. 24, 321–329. doi: 10.1023/a:1022534709672
Sokoloff, L., Reivich, M., Kennedy, C., Rosiers, M. H. D., Patlak, C. S., Pettigrew, K. D., et al. (1977). The [14 C]deoxyglucose method for the measurement of local cerebral glucose utilization: theory, procedure, and normal values in the conscious and anesthetized albino rat. J. Neurochem. 28, 897–916. doi: 10.1111/j.1471-4159.1977.tb10649.x
Stangherlin, E. C., Luchese, C., Ardais, A. P., and Nogueira, C. W. (2009). Passive smoke exposure induces oxidative damage in brains of rat pups: protective role of diphenyl diselenide. Inhal. Toxicol. 21, 868–874. doi: 10.1080/08958370802526881
Torres, L. H., Annoni, R., Balestrin, N. T., Coleto, P. L., Duro, S. O., Garcia, R. C. T., et al. (2015a). Environmental tobacco smoke in the early postnatal period induces impairment in brain myelination. Arch. Toxicol. 89, 2051–2058. doi: 10.1007/s00204-014-1343-2
Torres, L. H., Balestrin, N. T., Spelta, L. E. W., Duro, S. O., Pistis, M., and Marcourakis, T. (2019a). Exposure to tobacco smoke during the early postnatal period modifies receptors and enzymes of the endocannabinoid system in the brainstem and striatum in mice. Toxicol. Lett. 302, 35–41. doi: 10.1016/j.toxlet.2018.12.002
Torres, L. H., Garcia, R. C. T., Blois, A. M. M., Dati, L. M. M., Durão, A. C., Alves, A. S., et al. (2015b). Exposure of neonatal mice to tobacco smoke disturbs synaptic proteins and spatial learning and memory from late Infancy to early adulthood. PLoS One 10:e0136399. doi: 10.1371/journal.pone.0136399
Torres, L. H., Garcia, R. C. T., Blois, A. M. M., Pacheco-Neto, M., Camarini, R., and Britto, L. R. (2019b). Early postnatal tobacco smoke exposure triggers anxiety-like behavior and decreases synaptic proteins even after a long exposure-free period in mice. Brain Res. 1707, 99–106. doi: 10.1016/j.brainres.2018.11.022
Vanhove, C., Bankstahl, J. P., Kramer, S. D., Visser, E., Belcari, N., and Vandenberghe, S. (2015). Accurate molecular imaging of small animals taking into account animal models, handling, anaesthesia, quality control and imaging system. EJNMMI Phys. 2:31. doi: 10.1186/s40658-015-0135-y
Watson, E. S., Jones, A. B., Ashfaq, M. K., and Barrett, J. T. (1987). Spectrophotometric evaluation of carboxyhemoglobin in blood of mice after exposure to marijuana or tobacco smoke in a modified walton horizontal smoke exposure machine. J. Anal. Toxicol. 11, 19–23. doi: 10.1093/jat/11.1.19
Weinstein, A., Greif, J., Yemini, Z., Lerman, H., Weizman, A., and ven-Sapir, E. E. (2010). Attenuation of cue-induced smoking urges and brain reward activity in smokers treated successfully with bupropion. J. Psychopharmacol. 24, 829–838. doi: 10.1177/0269881109105456
Welch, K. D., Pfister, J. A., Lima, F. G., Green, B. T., and Gardner, D. R. (2013). Effect of α7 nicotinic acetylcholine receptor agonists and antagonists on motor function in mice. Toxicol. Appl. Pharmacol. 266, 366–374. doi: 10.1016/j.taap.2012.11.024
Yochum, C., Doherty-Lyon, S., Hoffman, C., Hossain, M. M., Zelikoff, J. T., and Richardson, J. R. (2014). Prenatal cigarette smoke exposure causes hyperactivity and aggressive behavior: role of altered catecholamines and BDNF. Exp. Neurol. 254, 145–152. doi: 10.1016/j.expneurol.2014.01.016
Keywords: environmental tobacco smoke, passive smoke, neuroimaging, positron emission tomography, 18F-FDG uptake, glucose metabolism, longitudinal study, brain
Citation: Torres LH, Real CC, Turato WM, Spelta LW, dos Santos Durão ACC, Andrioli TC, Pozzo L, Squair PL, Pistis M, de Paula Faria D and Marcourakis T (2020) Environmental Tobacco Smoke During the Early Postnatal Period of Mice Interferes With Brain 18 F-FDG Uptake From Infancy to Early Adulthood – A Longitudinal Study. Front. Neurosci. 14:5. doi: 10.3389/fnins.2020.00005
Received: 23 September 2019; Accepted: 07 January 2020;
Published: 29 January 2020.
Edited by:
Carla Cannizzaro, University of Palermo, ItalyReviewed by:
Ramon Guirado, University of Valencia, SpainYongsoo Kim, Penn State Health Milton S. Hershey Medical Center, United States
Copyright © 2020 Torres, Real, Turato, Spelta, dos Santos Durão, Andrioli, Pozzo, Squair, Pistis, de Paula Faria and Marcourakis. This is an open-access article distributed under the terms of the Creative Commons Attribution License (CC BY). The use, distribution or reproduction in other forums is permitted, provided the original author(s) and the copyright owner(s) are credited and that the original publication in this journal is cited, in accordance with accepted academic practice. No use, distribution or reproduction is permitted which does not comply with these terms.
*Correspondence: Tania Marcourakis, tmarcour@usp.br