- 1Department of Physiology, Faculty of Physical Education, Gdansk University of Physical Education and Sport, Gdańsk, Poland
- 2Department of Athletics, Strength and Conditioning, Poznań University of Physical Education, Poznań, Poland
- 3Sports Neuroscience Division, Advanced Research Initiative for Human High Performance, Faculty of Health and Sport Sciences, University of Tsukuba, Tsukuba, Japan
- 4Division of Sport Science, Incheon National University, Incheon, South Korea
- 5Neurological and Psychiatric Nursing Department, Faculty of Health Sciences, Medical University of Gdańsk, Gdańsk, Poland
- 6Neurology Department, St. Adalbert’s Hospital, Poznań, Poland
- 7Laboratory of Exercise Biochemistry and Neuroendocrinology, Faculty of Health and Sport Sciences, University of Tsukuba, Tsukuba, Japan
There is increasing attention to sprint interval exercise (SIE) training as a time-efficient exercise regime. Recent studies, including our own (Kujach et al., 2018), have shown that acute high-intensity intermittent exercise can improve cognitive function; however, the neurobiological mechanisms underlying the effect still remain unknown. We thus examined the effects of acute SIE on cognitive function by monitoring the peripheral levels of growth and neurotrophic factors as well as blood lactate (LA) as potential mechanisms. Thirty-six young males participated in the current study and were divided into two groups: SIE (n = 20; mean age: 21.0 ± 0.9 years) and resting control (CTR) (n = 16; mean age: 21.7 ± 1.3 years). The SIE session consisted of 5 min of warm-up exercise and six sets of 30 s of all-out cycling exercise followed by 4.5 min of rest on a cycling-ergometer. Blood samples to evaluate the changes of serum concentrations of brain-derived neurotrophic factor (BDNF), insulin-like growth factor-1 (IGF-1), vascular endothelial growth factor (VEGF), and blood LA were obtained at three time points: before, immediately after, and 60 min after each session. A Stroop task (ST) and trail making test (TMT) parts A and B were used to assess cognitive functions. Acute SIE shortened response times for both the ST and TMT A and B. Meanwhile, the peripheral levels of BDNF, IGF-1, and VEGF were significantly increased after an acute bout of SIE compared to those in CTR. In response to acute SIE, blood LA levels significantly increased and correlated with increased levels of BDNF, IGF-1, and VEGF. Furthermore, cognitive function and BDNF are found to be correlated. The current results suggest that SIE could have beneficial effects on cognitive functions with increased neuroprotective factors along with peripheral LA concentration in humans.
Introduction
There is increasing attention to the beneficial effects of exercise on human cognition. Among various types of exercise, many studies have pointed out that moderate-intensity exercise has beneficial effects on cognitive abilities such as information processing or control of inhibition (Knaepen et al., 2010; Alves et al., 2012). However, these recommended aerobic exercise regimens require people to make a considerable time commitment, which is known as a limiting factor of physical activity in the modern era (Gibala and Little, 2010). Recently, high-intensity interval training (HIT) has become very popular among people as a time-efficient exercise regime (Gibala and Little, 2010; Astorino and Sheard, 2019). In addition, recent evidence suggests that HIT is more enjoyable than moderate-intensity endurance exercise (Bartlett et al., 2011; Little et al., 2011a). Since some reported that HIT could also be uncomfortable, causing effects such as active displeasure (i.e., distressed, upset) and lower enjoyment assessed with the Physical Activity Enjoyment Scale, especially for the sedentary population (Decker and Ekkekakis, 2017; Farias-Junior et al., 2019), various transferable forms of high-intensity intermittent exercise have been developed and it was revealed that these exercise regimes have beneficial effects not only on cardiovascular and metabolic adaptation but also on cognitive function (Little et al., 2010; Kujach et al., 2018). Sprint interval exercise (SIE) as a Wingate test-based modality consists of a number of supramaximal “all out” exercise bouts interspersed with recovery periods, achieving ∼20 min of activity in a single session (Burgomaster et al., 2005; Gibala and Little, 2010). This training protocol has been widely used and demonstrated to positively influence cardio-metabolic health parameters (Sloth et al., 2013). However, there is less evidence for how acute SIE improves cognitive functions and underlying neurobiological mechanisms.
Numerous animal and human studies have revealed that exercise enhances human cognition via exercise-enhanced neurotrophins and catecholamine production, which is known to mediate neural plasticity and energy metabolism in the brain (Kohut et al., 2006; Gomez-Pinilla and Hillman, 2013). Several neurochemicals, including brain-derived neurotrophic factor (BDNF), insulin-like growth factor-1 (IGF-1), and vascular endothelial growth factor (VEGF), are currently considered key proteins that mediate downstream effects of exercise on the brain and cognition (Cotman et al., 2007). As a single bout of physical exercise may lead to an increase in BDNF level (Ferris et al., 2007; Griffin et al., 2011), these exercise-increased neurotrophins may contribute to a reduction in mood disorders and to the protection and regeneration of various tissues resulting in increased cognitive performance in humans (Hansen et al., 2001; Rojas Vega et al., 2008; Zoladz and Pilc, 2010).
Interestingly, previous research has indicated that SIE facilitates muscle remodeling, which in turn may lead to the production of BDNF (Little et al., 2011b; Wrann et al., 2013; Phillips et al., 2014). In fact, high-intensity exercises and strength training both stimulate the secretion of IGF-1 (Bermon et al., 1999; Cassilhas et al., 2007), which is needed to transform pro-BDNF into BDNF in the central nervous system and can easily cross the blood–brain-barrier affecting neurogenesis and synaptic plasticity (Ding et al., 2006; Nishijima et al., 2010). In addition, recent animal studies have revealed that IGF-1 mediates exercise-induced angiogenesis, increased central BDNF, and VEGF production (Lopez-Lopez et al., 2004; Ding et al., 2006). Similar to IGF-1, peripheral VEGF also increases during exercise, in part mediating exercise-induced angiogenesis and neurogenesis (Lopez-Lopez et al., 2004). Circulating VEGF may promote neurogenesis and synaptic plasticity by stimulating neural stem cell proliferation and differentiation (Zacchigna et al., 2008; Ruiz de Almodovar et al., 2009) and also increases central endothelial cell and astrocytic productions of VEGF, BDNF, and IGF-1 (Zacchigna et al., 2008; Ruiz de Almodovar et al., 2009).
Moreover, SIE is related to the increase in glucose metabolism and lactate (LA) production, where glucose as well as LA are important energy sources for the human brain (van Hall et al., 2009; Tsukamoto et al., 2016; Hashimoto et al., 2018). Further, among various HIT protocols, SIE is well recognized as inducing important elevation in blood LA concentration (Wood et al., 2016; Olney et al., 2018). Glenn et al. (2015) found that peripherally produced LA is available as a cerebral energy supply after traumatic brain injury. Although the brain metabolism relies mainly on glucose while at rest, the cerebral consumption of glucose decreases during high intensity exercise, along with an increase in blood LA of consequence for the cerebral uptake (Kemppainen et al., 2005). Furthermore, improvement in executive functions may also be related with neuronal activation induced by high intensity intermittent exercise (Kujach et al., 2018). Neuronal activation is associated with an increase in energy requirement (Dalsgaard et al., 2002). Elevated peripheral LA concentration in response to intense exercise promotes the supply of LA as an energy substrate to meet acute neuronal energy requirements (Barros, 2013; Dienel, 2017). Thus, SIE modulating blood LA concentration and neuronal activation could affect cognitive function.
Although SIE may lead to progressive fatigue, causing excessive activation of the central nervous system and subsequent cognitive impairments (Chmura et al., 1994; Tomporowski, 2003), it might also induce the production of neurotrophins such as BDNF or IGF-1, positively affecting neurogenesis and synaptic plasticity in the brain (Lista and Sorrentino, 2010).
Given this evidence, we hypothesized that BDNF, IGF-1, and VEGF would increase in response to SIE and that this may be related to acute-exercise-induced cognitive benefits. Here, we have investigated whether acute SIE affects circulating neuroprotein concentrations, which may play a critical role in the enhancement of cognitive abilities.
Materials and Methods
Subjects
Thirty-six healthy, right-handed Polish-speaking male subjects participated in the study. All volunteers had normal vision (including color vision). No subject had a history of neurological, major medical, or psychiatric disorders, and none were taking medication at the time of measurement. Additionally, they were required to refrain from consumption of caffeine for 12 h prior to the testing session. All the subjects provided written informed consent prior to the study procedures. The participants were assigned to either the SIE group (SIE; n = 20, 21.0 ± 0.9 years) or the control group (CTR; n = 16, 21.7 ± 1.3 years) based on their age, weight, and physical fitness level (O2max). All the procedures were approved by the Bioethical Committee of the Regional Medical Society in Gdańsk.
Study Design
Participants visited the study site three times. One week before starting the experiment the subjects were asked to come to the laboratory for a familiarization session to learn about the testing procedures. Next, participants completed the same set of body composition, aerobic, and cognitive assessments before the main testing session. An overview of the experimental protocol is presented in Figure 1.
Body Composition Measurements
Body mass (BM) and body composition were estimated using a multi-frequency impedance plethysmograph body composition analyzer (InBody 720, Biospace, South Korea). This analyzer accurately measures body water and body composition, including fat mass, free fat mass, skeletal muscle mass, and soft lean mass (Ziemann et al., 2013).
Maximal Oxygen Uptake (
O2max) Test
To determine O2max, participants performed a graded cycle ergometry test on a mechanically braked cycle ergometer (884E Sprint Bike, Monark, Sweden). Subjects were allowed a 5-min warm-up period at an intensity of 1.5 W × kg–1 with a pedaling cadence of 60 rpm. Immediately after the warm-up the participants began
O2max testing by cycling at increasingly difficult workloads in which resistance was increased by 25 W × min–1 until the participant reached the point of volitional exhaustion.
O2max was determined when at least two of the following criteria were satisfied: (1) the respiratory exchange ratio (RER) exceeded 1.05, (2) achievement of 90% of age-predicted peak HR (220–age), and (3) an ratings of perceived exertion (RPE) of 19 or 20 (Suwabe et al., 2017). Breath-by-breath pulmonary gas exchange was measured (MetaMax 3B, Cortex, Germany) throughout the
O2max test; the O2 and CO2 analyzers were calibrated before each test using standard gases of known concentrations in accordance with manufacturer guidelines.
SIE Sessions
Sprint interval exercise is a subcategory of interval exercise, involving “all out” supramaximal intensity (>100% O2max) (Weston et al., 2014). The SIE sessions were performed on a mechanically braked cycle ergometer (884E Sprint Bike, Monark, Sweden). Exercise protocol started with a standard 5-min warm up at 1.5 Watts × kg–1 of BM. After the warmup, subjects performed the interval exercise, which included six sets of 30 s of “all out” sprint cycling exercise. Flywheel resistance equaled 0.075 kG × kg–1 of BM (i.e., Wingate test based) which corresponded to 7.5% of each individual’s BM and was applied on the onset of the SIE (Burgomaster et al., 2008). The interval rest periods between the 30-s bouts were 4 min and 30 s. The participants were instructed to accelerate until they reached their maximal pedaling rate and were verbally encouraged to maintain this pedaling cadence as long as possible throughout the SIE compilation. Only during the first few initial movements of each bout of the test was each participant allowed to pedal in a standing position; this was to help overcome the resistance and to quickly achieve the maximal pedaling rate. During the testing session, oxygen uptake was also monitored. For this purpose, a breath-by-breath pulmonary gas exchange method was used, where MetaMax 3B (Cortex, Germany) was applied. The O2 and CO2 analyzers were calibrated before each test using standard gases of known concentrations in accordance with manufacturer guidelines. Using MetaMax 3B, lung minute ventilation (VE L × min–1) and relative maximal oxygen uptake (
O2max mL × min–1 × kg–1) were obtained. Heartrate (HR b × min–1) was monitored continuously via telemetry (S-625, Polar Electro-Oy, Finland). All training sessions were performed at similar times in the morning at least 2 h after breakfast.
Cognitive Assessments
The assessment of cognitive functions was made using selected neuropsychological timed tasks. TMT A and B were used to measure working memory and the ST was adopted to assess executive functions. Since in meta-analyses, Lambourne and Tomporowski (2010) and Chang et al. (2012) suggest that more pronounced cognitive function facilitation could be observed 11–20 min following acute high-intensity exercise, each task was assessed before and 20 min after the SIE session for the SIE group, and before and 20 min after the resting session for the control group.
Stroop Test
The Polish paper version of the ST was used in this study. The ST consisted of 71 words written in colored ink. The participants’ task was to name the color of the font regardless of the word written. At the beginning of the test the meaning of each word was consistent with the color of the font in which it appeared (e.g., the word “blue” written in blue or “red” written in red; congruent) in order to activate the automatism of reading, and as the test progressed words written in incongruent fonts (e.g., the word “blue” written in green or “red” written in blue; incongruent) were randomly mixed in with the congruent words, requiring cognitive control in order to respond accurately. The numbers of “congruent” and “incongruent” stimuli were the same. The need to inhibit the automatic reading response, rather than to only name the color in which the word was printed, elicits a significant slowing in reaction time called the Stroop interference effect; thus, cognitive control is reflected in the time taken to execute the test and the number of errors. Consequently, the ST is a sensitive measure of cognitive inhibition and information processing speed (Stroop, 1935; Strauss et al., 2006).
Trail Making Test
The trail making test (TMT) is one of the most commonly used tests assessing working memory. This test includes two conditions (i.e., “A” and “B”), where the “A” condition reflects psychomotor speed and the “B” condition requires additional executive control needed to switch between number and letter sequences. TMT-A consists of drawing lines as quickly as possible to link consecutively numbered circles. In contrast, in TMT-B participants must connect circles while alternating between numbers and letters. Performance in both conditions is assessed based on the time (seconds) taken to complete each task (Chang and Etnier, 2009; Alves et al., 2012). The difference in time needed to complete part B and part A reflects the efficiency of the working memory, as both parts of the test are comparable in terms of visual search and motor requirements but do differ in cognitive complexity.
Blood Sampling and Analysis
Samples were collected from the antecubital vein (v. mediana cubiti) between 8:00 and 10:00 a.m. to establish a baseline. Follow-up samples were taken before the warm-up, directly after the last (sixth) 30-s bout, and 1 h after the completion of all SIE bouts in order to evaluate serum concentrations of BDNF, IGF-1, VEGF, and cortisol. Samples for the CTR group were taken following the same timeline as those for the SIE group, except 1 h after the experiment.
The samples were centrifuged at 2000 × g for 10 min at 4°C. The separated serum samples were frozen and kept at −80 °C until later analysis. Serum BDNF, IGF-1, and VEGF were determined by enzyme immunoassay methods using commercial kits (R&D Systems, United States, catalog no. DBD00, DG100, DVE00). The average intra-assay CV was 8.0% for all proteins. Serum cortisol concentration was evaluated using a Demeditec (Germany) ELISA kit. Detection limits were 2.5 ng mL–1, and the intra-assay coefficient of variation for the kits was <7%. For blood LA analysis samples were collected from capillary blood taken from the finger as part of the baseline and after completion of last SIE bout. Immediately after collection, the blood was deproteinized by the addition of ice-cold 0.4 M perchloric acid. After being thoroughly mixed, the samples were centrifuged at 12,000 × g for 10 min. Blood LA was determined using a standard Randox (Crumlin, United Kingdom) kit based on the LA oxidase method (LC2389); assays were performed on a Cecil CE9200 spectrophotometer (Cambridge, United Kingdom).
Statistical Analysis
The statistical calculations were performed using STATISTICA 13. The results are expressed as mean and standard deviation (SD), or standard error of mean (SEM). The normality of data distribution was established using the Shapiro–Wilk W-test. The level of significance was set as p = 0.05 for all of the analyses. Additionally, two-way analysis of variance (ANOVA) with repeated measures was used to investigate the significance of differences between groups and time. For peripheral neurotrophic factors concentration response to acute SIE a one-way repeated measures ANOVA was applied. Significant main effects were further analyzed with the Bonferroni or Tukey’s post hoc test. Changes (delta) in both groups were compared using an independent samples t-test or a Mann–Whitney U test, according to the data distribution. Correlations between variables were evaluated using the Spearman correlation coefficient for non-normally distributed data.
Results
The study participants were physical education students who experience various forms of physical activity during the course of their studies (swimming, gymnastics, athletics, football, or basketball). Moreover, the results of body fat and O2max values demonstrate that the participants were physically active. All participants completed the study. The anthropometric and physical activity parameters are presented in Table 1. At baseline, there were no significant differences in basic anthropometric characteristics or in aerobic performance between groups (Table 1).
Physiological Response to SIE
The evolution of VO2, VE, and HR during the six bouts of SIE are displayed in Figure 2. The obtained data indicate a significant increase in cardio-respiratory parameters compared to the baseline in the SIE group (Figure 2). The average O2, VE, and HR responses to SIE correspond to ∼85, 90, and 90%, respectively, compared to the values reached in the maximal graded exercise test (
O2max test). Moreover, the SIE group had RPE after acute SIE that averaged between 17–18 ± 2 (mean ± SD).
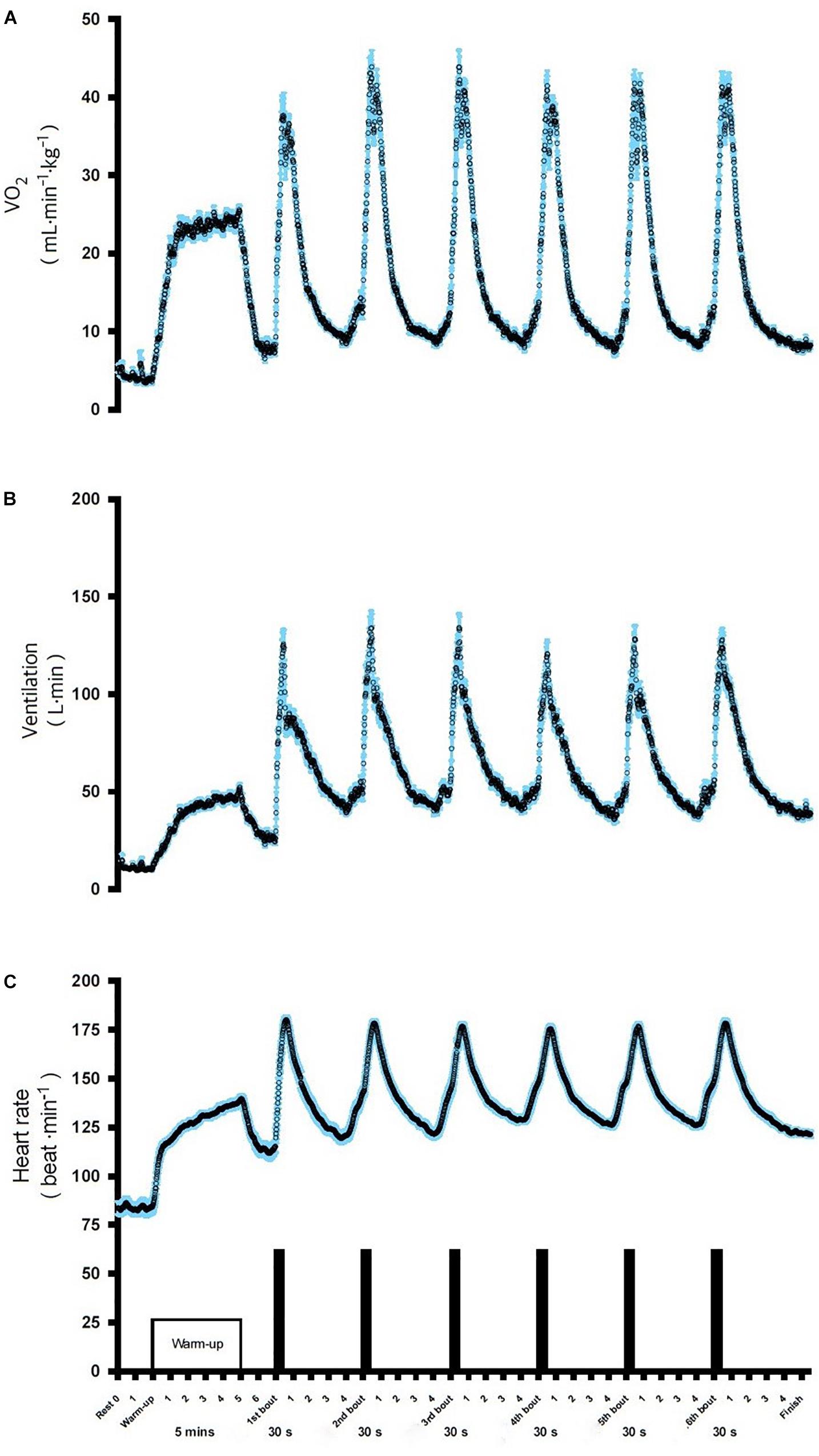
Figure 2. Oxygen consumption VO2 (A), ventilation VE (B), and heart rate HR (C), during SIE. Data shown are means.
Cognitive Performance in Stroop Test and Trail Making Test
The analysis revealed no statistical differences between the SIE and CTR groups in ST performance (p = 0.85), TMT-A (p = 0.24), and TMT-B (p = 0.39) for the pre-sessions (Figure 3). There was significant interaction between group (SIE/CTR) and time (PRE/POST) factors when we performed a two-way ANOVA with repeated measures for ST execution time (p < 0.05; F(1,34) = 9.45). Next, to examine the interaction, we calculated the difference of the degree of ST performance between post- and pre-sessions (delta), contrasted for both the SIE and CTR groups separately, and compared the difference between them. The delta ST performance difference was significantly more negative in the SIE than in the CTR group (z = 62.0; p < 0.001, Mann–Whitney U test) (Figure 3B).
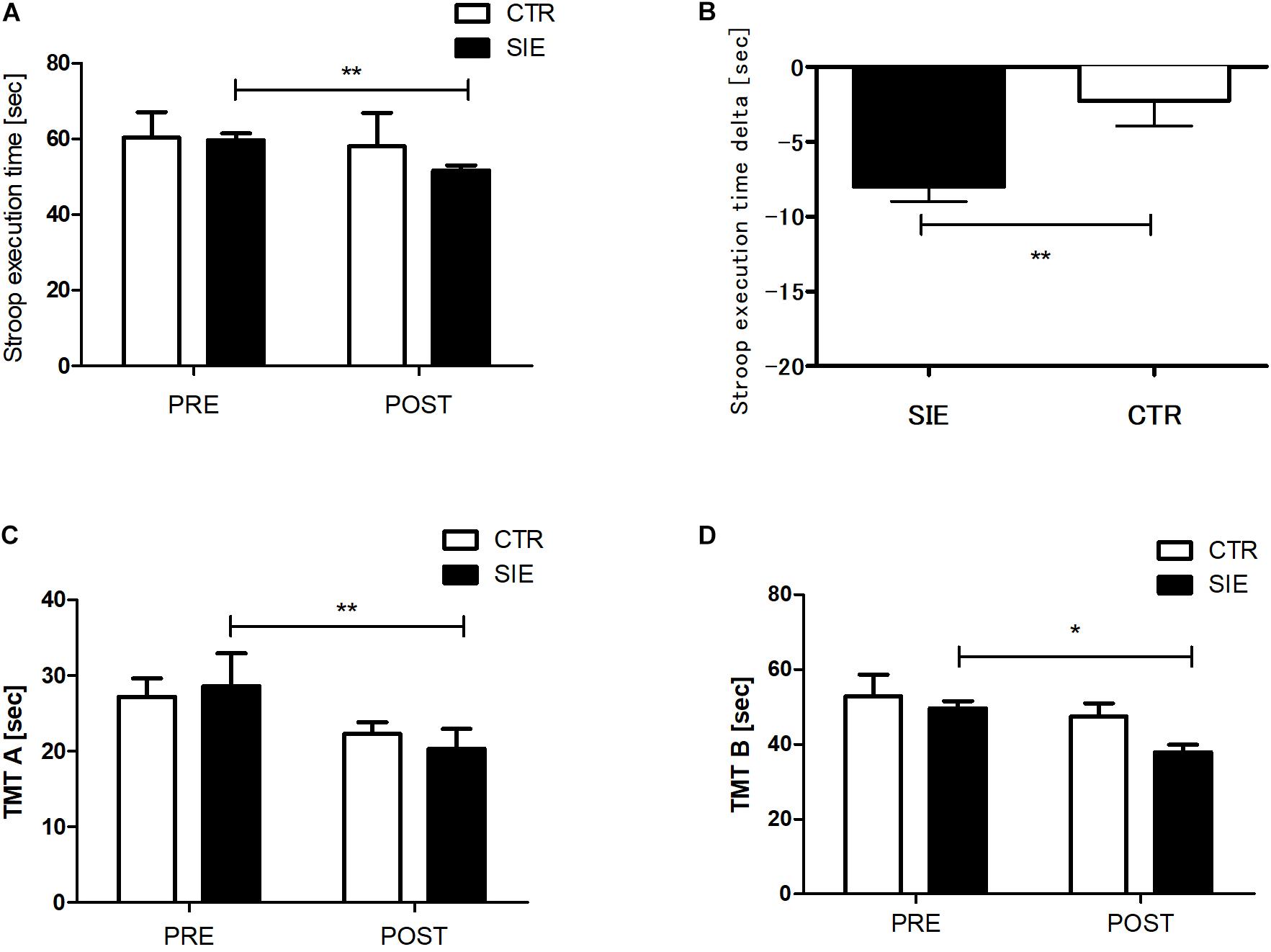
Figure 3. Stroop task (A) and trial making test, parts A and B (C,D) execution times. Contrast between SIE delta (post–pre) and CTR delta (post–pre) for Stroop task performance (B). Values are means. Error bars indicate ± SEM (standard error of mean). *p < 0.05, **p < 0.001.
Moreover there was a significant main effect of time in the TMT parts A and B (p < 0.05; F(1,68) = 21.23 and p < 0.01; F(1,68) = 6.42, respectively; two-way ANOVA), whereas neither main effect of group nor interaction of the factors was significant. Post hoc Tukey’s test analysis revealed a significant decrease in the both test version A (p < 0.01) and B (p < 0.05) execution time in the SIE group 20 min post-exercise (Figures 3C,D).
The Response of Blood Lactate and Cortisol Levels Following Acute SIE
Blood LA concentration and serum cortisol concentration were significantly affected by SIE (Figure 4). The SIE resulted in a rapid increase in blood LA concentration (t = 35.5; p < 0.01). Acute SIE induced an almost twofold cortisol level increase (p < 0.01) sustained to 1 h post exercise (p < 0.05) relative to baseline: one-way repeated measures ANOVA with post hoc Bonferroni test results were p < 0.01; F(2,38) = 18.51.
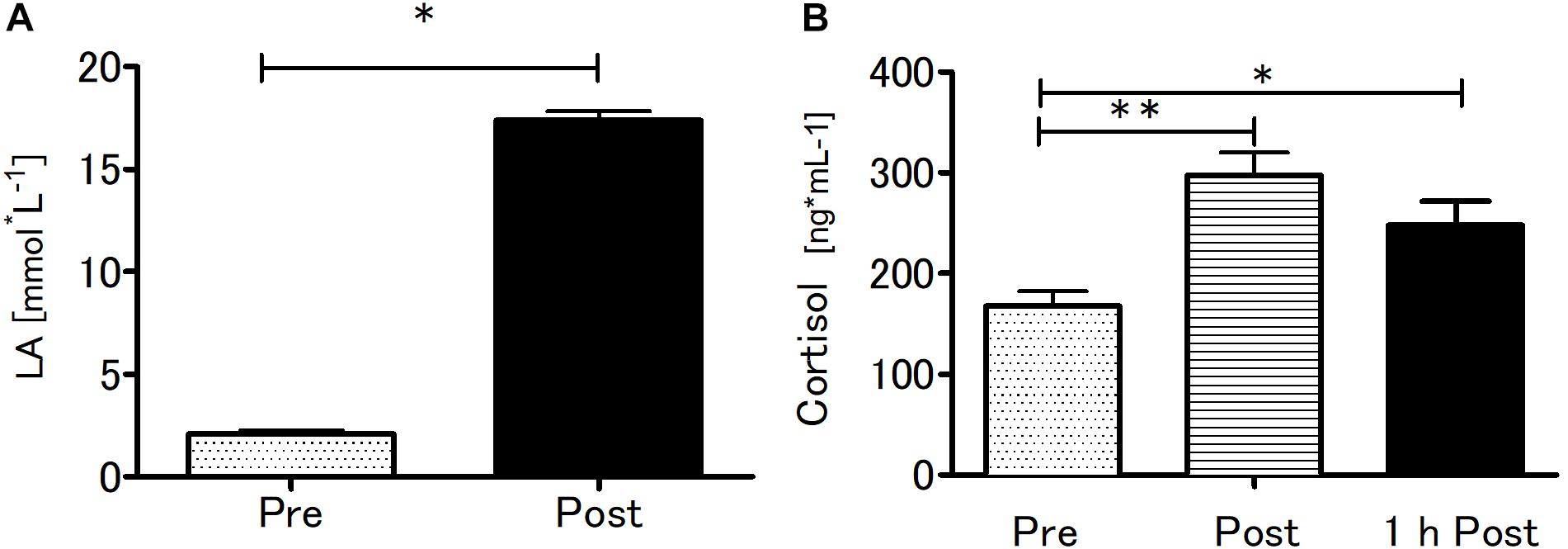
Figure 4. Blood lactate at baseline and after SIE (A) and serum cortisol concentrations pre, immediately post, and 1-h post-SIE (B) for the exercise group. Values are means. Error bars indicate ± SEM. *p < 0.05, **p < 0.001.
Effect of Acute SIE on Peripheral Levels of BDNF, IGF-1, and VEGF
Analyzing the effect of SIE on peripheral trophic factor concentration, a two-way ANOVA for repeated measures with post hoc Bonferroni test revealed a significant interaction (Group x Time) (p < 0.01; F(1,34) = 10.18) in BDNF and VEGF (p < 0.01; F(1,34) = 7.51) peripheral concentration (Figures 5A,C). Further, there was a significant main effect of time (p < 0.05; F(1,34) = 3.87), whereas neither the main effect of group nor interaction of the factors was significant in peripheral IGF-1 concentration (Figure 5B). Moreover, statistically significant differences between the SIE and CTR groups in delta BDNF (t = 3.19; p < 0.01) and VEGF (t = 2.60; p < 0.01) concentrations were found (Figures 5D,F). Despite the increase in IGF-1 following SIE, statistical analysis did not show any significance effect versus the CTR group (t = 1.77; p = 0.08) (Figure 5E).
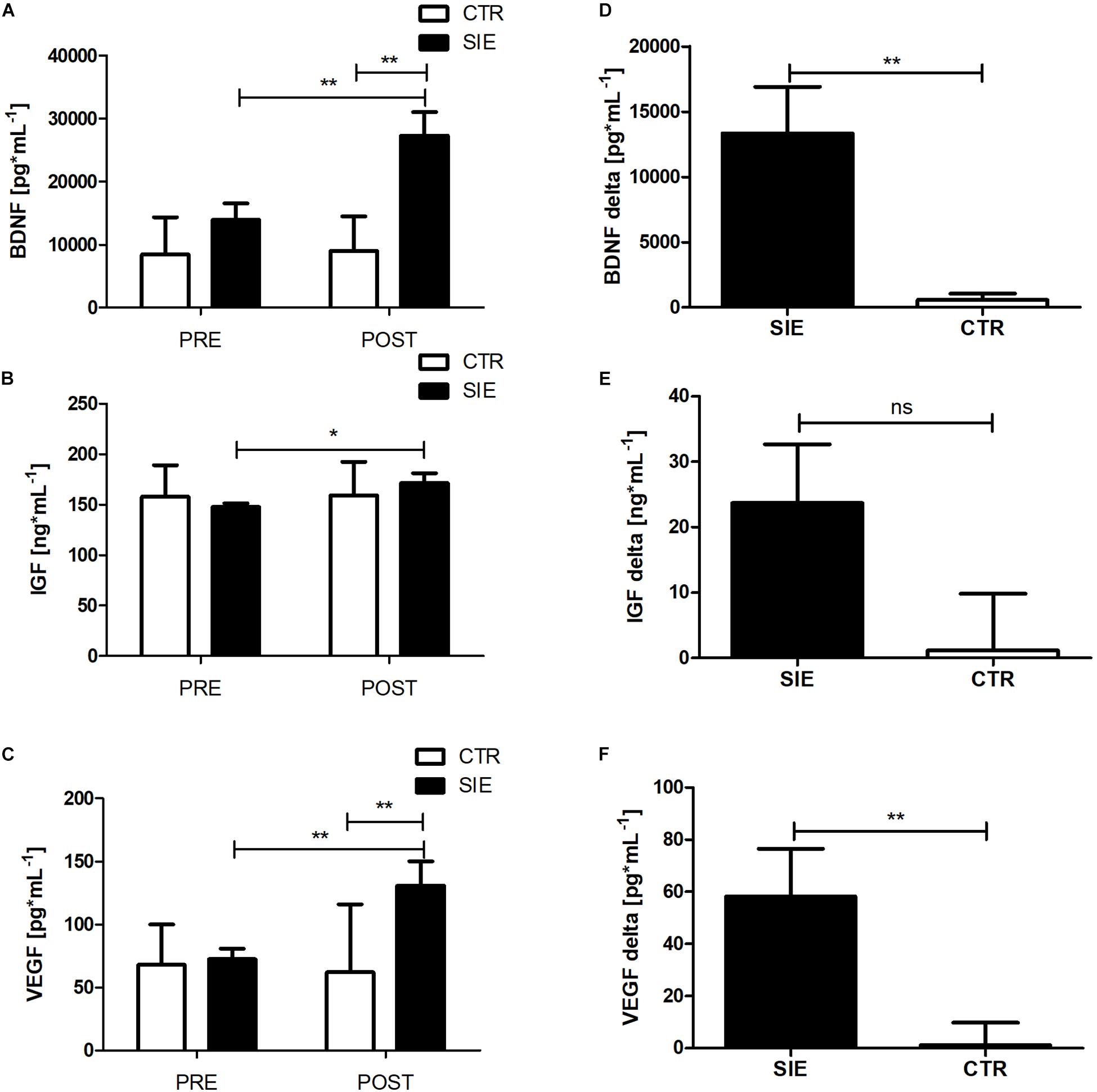
Figure 5. Effect of acute SIE on peripheral BDNF (A), IGF-1 (B), and VEGF (C) concentrations and contrast between SIE versus CTR deltas (post–pre) (D–F). Values are means. Error bars indicate ± SEM. *p < 0.05; **p < 0.001; ns, non-significant.
The Response of Peripheral BDNF, IGF-1, and VEGF Following Acute SIE
To verify the peripheral BDNF, IGF-1, and VEGF concentration response to acute SIE a one-way repeated measures ANOVA and post hoc Bonferroni was applied. Acute SIE induced an increase in serum BDNF, IGF-1, and, VEGF concentration (Figure 6). There was a significant difference between time points in BDNF, IGF-1, and VEGF concentration (p < 0.01; F(2,38) = 9.97, p < 0.01; F(2,38) = 5.54, and p < 0.01; F(2,38) = 5.23, respectively; one-way repeated measures ANOVA and post hoc Bonferroni test). Post hoc analysis revealed a significant increase in serum BDNF concentration immediately post-SIE (p < 0.01), sustained to 1 h post-SIE (p < 0.01), relative to baseline, as well as for VEGF concentration immediately post-SIE (p < 0.05), sustained to 1 h post-SIE (p < 0.05), relative to baseline. Acute SIE also significantly altered serum IGF-1 concentration immediately post exercise (p < 0.01) whereas at 1-h post-SIE serum IGF-1 concentration decreased and was not significantly different from the baseline. Changes in serum BDNF, IGF-1, and VEGF concentration after SIE are displayed in Figure 6.
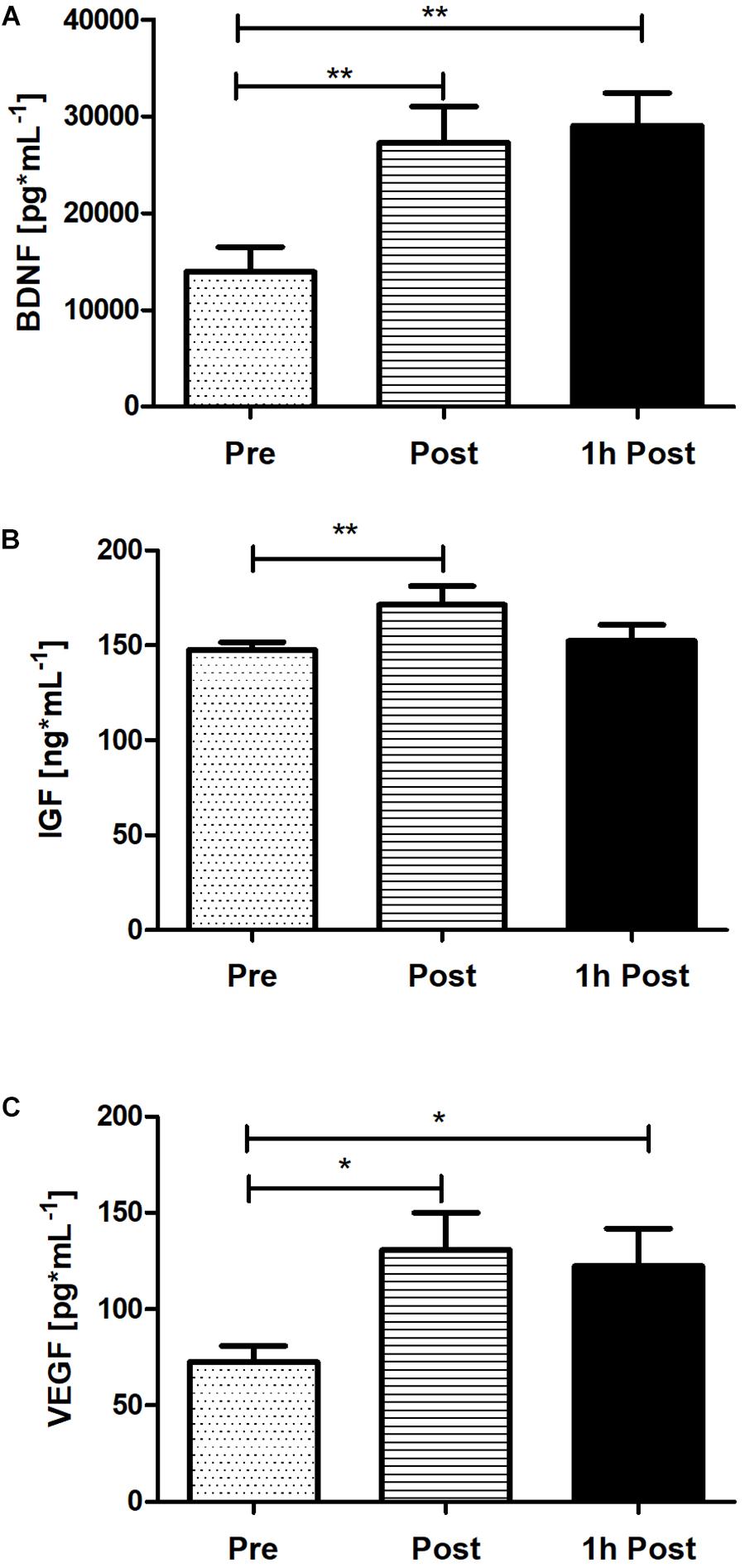
Figure 6. The response of serum BDNF (A), IGF-1 (B), and VEGF (C) concentration immediately post and 1 h following acute SIE. Values are means. Error bars indicate ± SEM. *p < 0.05, **p < 0.001.
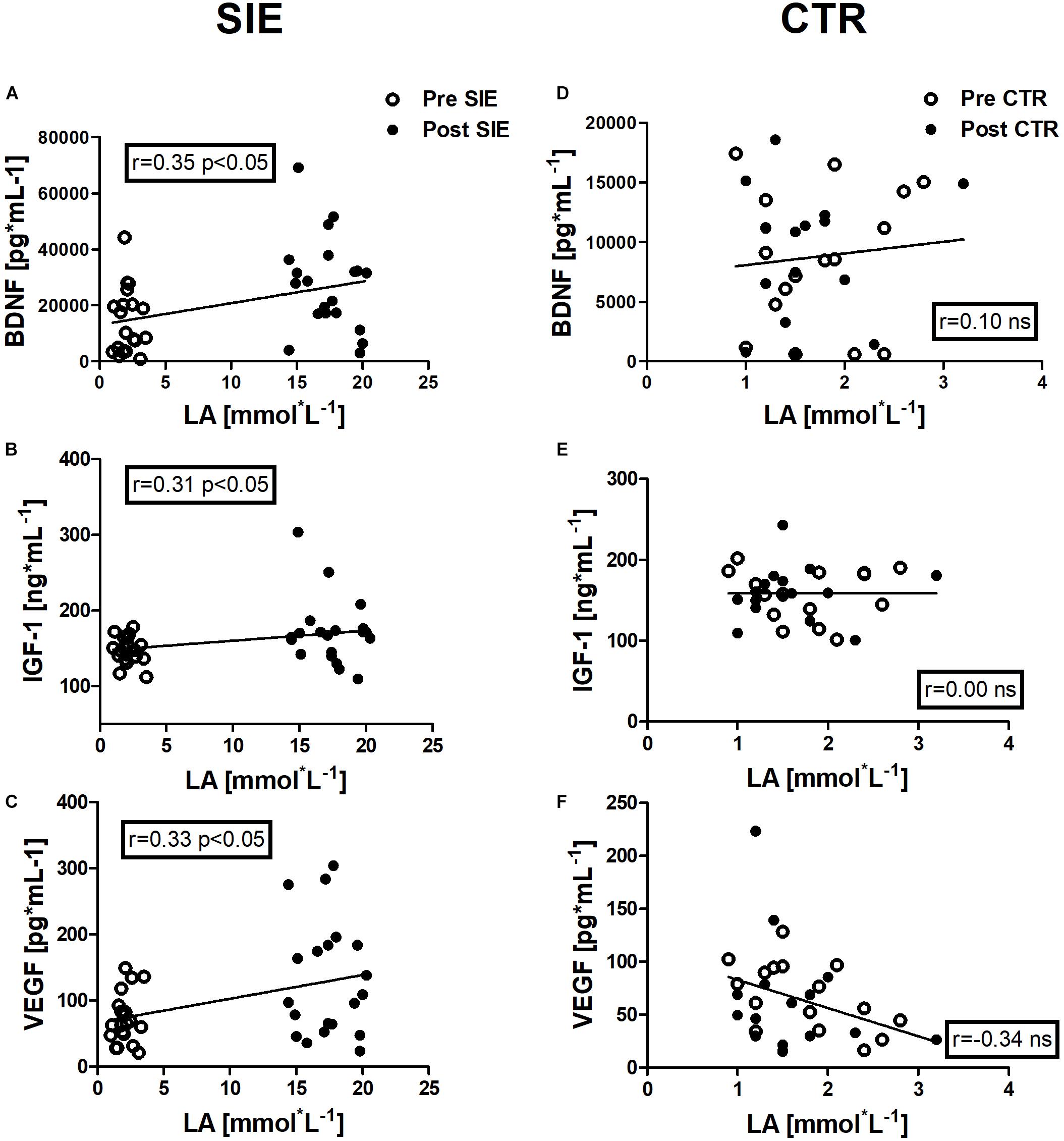
Figure 7. Association between LA and BDNF, IGF-1, and VEGF concentrations, of pre- and post-sessions in the SIE and CTR groups. Significant positive correlations between LA and BDNF concentration (A), LA and IGF-1 concentration (B), and LA and VEGF concentration (C) were found. No significant associations were observed in the concentration of neurotrophic factors and LA in the control group (D–F). Data are presented as open circles: pre, and closed circles: post-SIE or CTR. ns, non-significant.
Correlation Analyses
Positive correlations were identified between LA and BDNF concentration (r = 0.35, p < 0.05), between LA and IGF-1 concentration (r = 0.31, p < 0.05), and between LA and VEGF concentration (r = 0.33, p < 0.05) in the pre- and post-session in the SIE group.
In addition, we performed Spearman correlation analysis to examine the association between Stroop execution time and BDNF concentration (r = −0.26, p < 0.05), and a blood LA concentration (r = −0.40, p < 0.01) (Figures 8A,B).
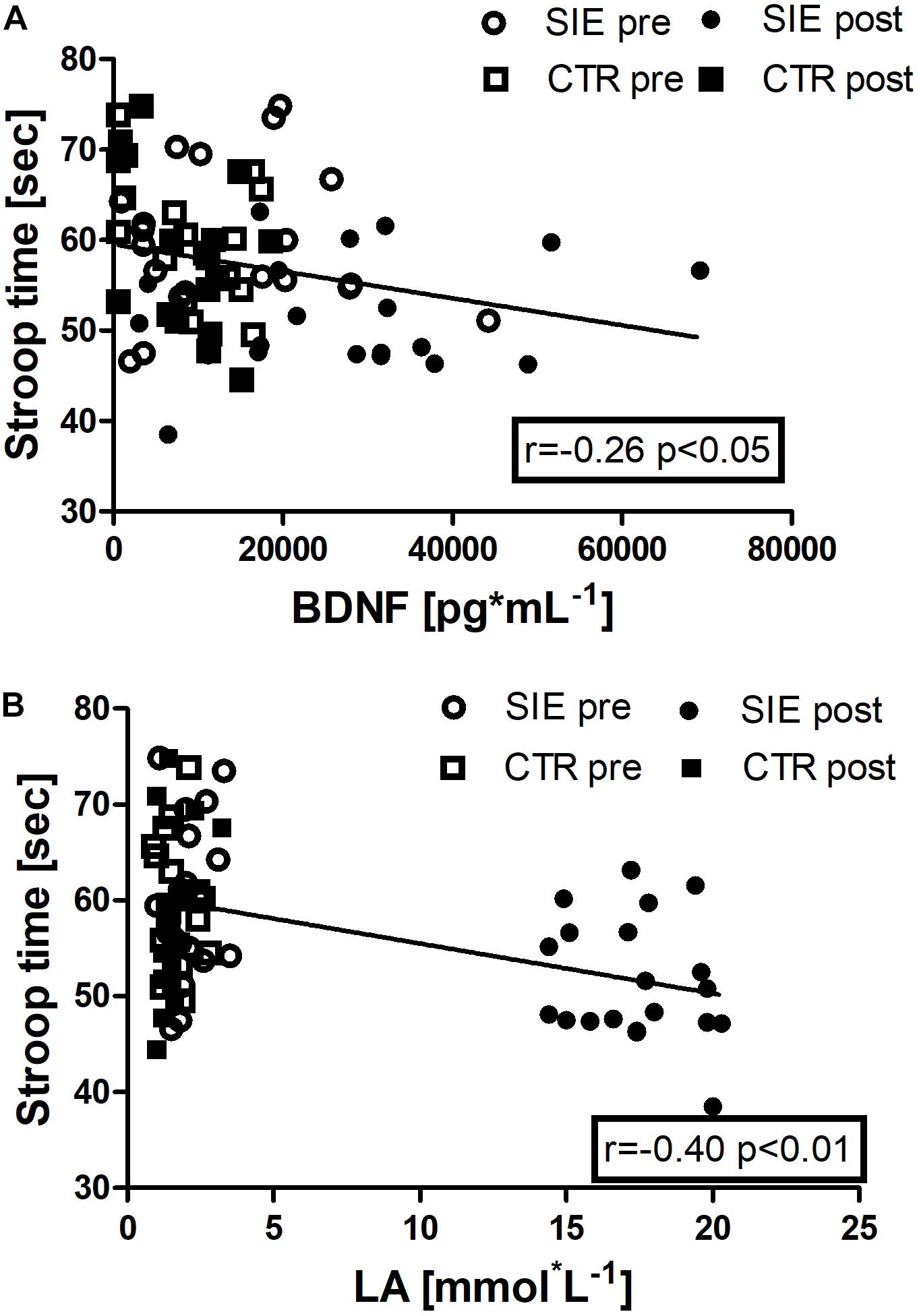
Figure 8. Association between Stroop execution time and BDNF concentration (r = –0.26, p < 0.05) (A), and Stroop execution time and blood LA concentration (r = –0.40, p < 0.01) (B). Data are presented as open circles: pre, and closed circles: post-SIE and open squares: pre, and closed squares: post-CTR.
Discussion
In the present study, acute SIE led to an improvement in prefrontal-dependent cognitive performance. Also, SIE resulted in significant elevations of serum BDNF, IGF-1, and VEGF levels and these changes are accompanied by an increased peripheral LA concentration. These findings suggest that acute bouts of SIE are beneficial to improve cognitive performance 20 min following exercise in young adults.
We illustrated that acute SIE improved cognitive performance as indicated by shorter response times for the ST and faster completion time of the TMT parts A and B. These results are consistent with the findings of recent neuroimaging studies revealing improved cognitive performance on the ST and TMT A and B after acute aerobic exercise as well as with SIE, both of which are associated with changes of neural activation in the prefrontal cortex (Yanagisawa et al., 2010; Lee et al., 2014; Kujach et al., 2018). Therefore, we can postulate that the current SIE model is beneficial to the prefrontal-dependent cognitive functions in young, healthy adults.
Blood LA elevated through SIE is, potentially, a factor that leads to improved cognitive function together with SIE-induced neuroprotective protein levels. It has been demonstrated that glucose and LA are important energy sources not only in muscle, but also in the human brain (van Hall et al., 2009). At rest, the brain mainly relies on glucose, whereas during high-intensity exercise, glucose uptake significantly decreases with increased blood LA concentration (Kemppainen et al., 2005). In contrast, LA is used by the brain in order to compensate for the increased energy required to maintain neuronal activity during high-intensity exercise (Kemppainen et al., 2005; Weston et al., 2014). Recently, Hashimoto et al. (2018) showed that arterial LA and brain LA uptake (arterial–venous differences across the brain) increases after SIE, suggesting that systemic LA affects brain LA uptake and influences executive function after exercise (Weston et al., 2014). Moreover, we found a positive correlation between blood LA and neuro-supporting protein concentrations. Similarly, Ferris et al. (2007) found that the increased blood LA level induced by acute exercise correlated with blood BDNF level. Furthermore, it has been pointed out, that LA is the “missing exercise factor” inducing BDNF synthesis (El Hayek et al., 2019). Very recently, El Hayek et al. (2019) observed that LA modulates the redox status of neurons by altering the NAD+/NADH ratio which leads to SIRT1 activation and in turn engages the hippocampal PGC1-α/FNDC5 pathway to induce BDNF expression (Koltai et al., 2010; Wrann et al., 2013). Moreover LA released from exercising muscles mediates cerebral angiogenesis through the activation of the LA receptor HCAR1, a key regulator of VEGF (Morland et al., 2017). Additionally, LA induces IGF-1 mRNA expression via the somatotropic axis stimulation (Salgueiro et al., 2014). Since blood LA following SIE greatly increased in the present study, we cannot rule out that the improvement in cognitive functions resulted from the acceleration of cerebral LA metabolism along with neuroprotective proteins induction. However, various high-intensity interval training protocols could differently affect blood LA synthesis (Wood et al., 2016; Warr-di Piero et al., 2018). SIE is characterized by high LA production whereas longer protocols could rely more on aerobic metabolism, with lower LA synthesis (Astorino and Sheard, 2019). Therefore, interval training protocols could differently modulate the central nervous system activation and cognitive performance.
The present data also revealed that peripheral BDNF, IGF-1, and VEGF secretion are modulated by acute SIE. Recent studies proposed a hypothesis of the role of exercise-induced BDNF synthesis (Kemppainen et al., 2005; Wrann et al., 2013; Saucedo Marquez et al., 2015). Wrann et al. (2013) suggested that the activation of peroxisome proliferator-activated receptor-alfa coactivator (PGC)-1α is induced by exercise in skeletal muscle cells, and that it could activate FNDC5 gene expression, which is a positive regulator of BDNF levels in the brain, mainly in the hippocampus. Interestingly, an increase in PGC1-α has also been observed after exercise adopted in an interval exercise protocol (Little et al., 2011b). Moreover, Ferris et al. (2007) indicated that intensive exercise leads to greater increases in peripheral BDNF concentration than does low-intensity, continuous exercise, suggesting that exercise intensity could be a key factor. Saucedo Marquez et al. (2015) have speculated that skeletal muscle contractions during high-intensity exercise may trigger this biochemical pathway, inducing elevated BDNF levels in the brain. They revealed that the SIE protocol (10 × 1-min bouts at 90% of maximal work load, alternating with 1-min rest at 60 W for a total duration of 20 min) is a more effective and preferred intervention for elevating BDNF levels than traditional, continuous, moderate-intensity exercise (Saucedo Marquez et al., 2015). Furthermore, the high-intensity interval exercise protocol could also have induced optimal short bursts of oxidative stress and inflammation, leading to the activation of the prefrontal cortex a brain region involved in cognitive processing, including executive function (Gomez-Pinilla and Hillman, 2013). Our protocol was “all out” and characterized by a very high intensity which on the one hand stimulates dramatic BDNF increase and on the other hand could increase oxidative stress and inflammation to a greater extent. Thus, high-intensity exercises such as SIE, which is associated with increased inflammation and oxidative stress, will certainly be better tolerated by young active people in comparison to inactive persons or the elderly.
Moreover, it has been revealed that BDNF could modulate presynaptic neurotransmitter release and evoke excitatory postsynaptic currents via TrkB receptors, directly inducing neuronal depolarization (Kafitz et al., 1999; Jovanovic et al., 2000). Furthermore, we found a positive association between improved ST performance and BDNF concentration. Accordingly, other animal and human studies have shown significant, positive associations between serum BDNF and cortex BDNF (r = 0.81) at rest as well as elevated BDNF and improved cognitive performance in response to acute exercise (Karege et al., 2002; Kraus et al., 2004; Winter et al., 2007; Griffin et al., 2011). These findings suggest that acute SIE stimulates BDNF and may induce cognitive enhancement related to the above-mentioned changes in neural activation in the prefrontal cortex (Yanagisawa et al., 2010; Lee et al., 2014).
According to our initial hypothesis, acute SIE significantly increased peripheral IGF-1 concentration. Although IGF-1 concentration in the serum has been reported to increase following high-intensity exercise (Kraemer et al., 2004) other studies have reported a lack of post-exercise increase in peripheral IGF-1 concentration (Jahreis et al., 1989; Schiffer et al., 2009). Circulating IGF-1 is mainly derived from the liver, but its secretion is also found in the brain, skeletal muscle, or bones (Yakar et al., 1999; Dall et al., 2001). Therefore, it is assumed that for post-exercise IGF-1 concentration, peripheral and cerebral sources are partly responsible (Dall et al., 2001). Additionally, acute exercise stimulates the expression and release of liver IGF-1 and results in elevated brain uptake of IGF-1 (Carro et al., 2000). Also, peripheral IGF is necessary for exercise-induced hippocampal neurogenesis and for functional recovery after brain injury in rodents (Trejo et al., 2001; Duman et al., 2009). Similarly, to IGF-1 we found significant increase in VEGF concentrations. The effects of acute exercise on peripheral VEGF have produced conflicting results with some investigators finding an increase after acute exercise (Kraus et al., 2004; Wahl et al., 2011) and others finding no differences in VEGF concentration in healthy subjects after physical exercise (Landers-Ramos et al., 2014). Interestingly the level of VEGF in the hippocampus decreases with age, while post-exercise VEGF elevation could play an important therapeutic role increasing brain functions (Shetty et al., 2005). Peripherally circulating VEGF can affect neurogenesis and synaptic plasticity by inducing proliferation and differentiation of neural stem cells (Zacchigna et al., 2008; Ruiz de Almodovar et al., 2009). It appears that the IGF-1 as well as VEGF response are largely dependent on exercise intensity and a lack of consistency in study designs as far as exercise type, intensity, duration, and timing of blood sampling after exercise may explain some of these discordant findings (Griffin et al., 2011; Wahl et al., 2011; Skriver et al., 2014).
There is strong evidence from studies of both human and animal subjects that BDNF, IGF-1, and VEGF are important pathways by which chronic exercise modulates brain function (Cotman et al., 2007; Voss et al., 2013). Recent studies suggest that the three growth factors have an impact on functional brain connectivity in the medial and lateral temporal cortices (Voss et al., 2013). Moreover, BDNF may also influence functional connectivity by increasing synaptogenesis and dendritic spine density, therefore improving long-term potentiation (LTP) via increased synaptic plasticity (Schinder and Poo, 2000; Rex et al., 2006; Vaynman et al., 2006; Stranahan et al., 2007). In addition, periphery-produced IGF-1 and VEGF support exercise-induced neurogenesis and angiogenesis (Ding et al., 2006). Further, exercise-induced neurovascular adaptations in the hippocampus have been associated with cognitive function (Clark et al., 2009). Taken together, a growing number of evidence supports the importance of neurotrophic factors for synaptic plasticity and structural brain changes. However, these findings are mostly observed in chronic exercise intervention and were not replicated in the current study.
It has been revealed that exercise intensity may differently affect cognitive performance (Tomporowski, 2003; Kohut et al., 2006). Low- and moderate-intensity exercise improves cognition, whereas high-intensity exercise may lead to increased arousal resulting in impaired cognitive performance. For example, Chmura et al. (1994) observed gradual shortening in reaction time during a multiple-choice reaction task with an exercise intensity of up to ∼75% O2peak, beyond which reaction time was rapidly impaired, suggesting that exercise intensity plays a role in the effects of acute exercise on some aspects of cognitive performance (Chmura et al., 1994). Moreover, prolonged high-intensity exercise exposure causes impairments in cognitive control and has neurotoxic effects in the human brain (Farias-Junior et al., 2019). In contrast, not only did our study not reveal any decrements in cognitive performance after an SIE session, but it also identified improvements in cognitive control and working memory. This is consistent with previous research adopting higher-intensity intermittent exercise, in which cognition parameters remained unaffected or even improved (Winter et al., 2007; Salgueiro et al., 2014). In addition, these findings are generally in agreement with other studies revealing that a single bout of HIT could improve executive performance and that this facilitation is sustained even for as long as 30 min (Yakar et al., 1999; Duman et al., 2009; Alves et al., 2014). The heterogeneity in the pattern of results of previous studies maybe due to differences in methodologies involving exercise mode and protocol, the participants’ fitness levels, cognitive task type, post-cognitive test timing, and other confounding factors (Chang et al., 2012).
It should also be noted that the high intensity of SIE could produce negative affective responses, thus making people unlikely to habitually perform the exercise (Hardcastle et al., 2014; Biddle and Batterham, 2015). We did not determine the mood state (enjoyment, pleasure, or affect) of our participants, so it is difficult to conclude whether they would like to repeat this kind of exercise in the future. However, Olney et al. (2018) demonstrated that healthy adults unaccustomed to interval training perceived high-intensity and SIE to be as enjoyable as time-consuming moderate-intensity continuous exercise. It is worth mentioning that the structure of interval training can be easily modified, allowing its application in people with diverse physical fitness levels. Therefore, there is a need for further research verifying the impact of various SIE protocols, especially since Townsend et al. (2017) showed that shorter sprint bouts (<30 s) in SIE produce greater pleasure among subjects. The proposed SIE can be successfully and safety applied by subjects with physical activity experience, such as physical education students or athletes. Collectively, we propose that even high intensity exercise, such as SIE, can be a fruitful and time-efficient intervention providing cognitive benefits to young, physically active people.
Limitations
In this study we tested young, active, healthy men, making it difficult to generalize our results to the general population. Moreover, we did not control for BDNF polymorphism, which could also influence BDNF secretion in response to exercise.
Conclusion
In summary, the current findings indicate that acute SIE enhances human cognitive function. The improvement in cognitive performance may result from the synthesis nor release of neuroprotective proteins modulated by high post-exercise blood LA concentration.
Data Availability Statement
The datasets generated for this study are available on request to the corresponding author.
Ethics Statement
The studies involving human participants were reviewed and approved by the Bioethical Committee of the Regional Medical Society in Gdańsk. The patients/participants provided their written informed consent to participate in this study.
Author Contributions
SK, RO, and RL conceived of and designed the experiment. SK, RO, EZ, and RL collected the data. SK, KB, and ES performed the statistical analyses and interpreted the data. SK, RO, KB, ES, EZ, RL, and HS participated in drafting the article or revising it critically for important intellectual content. SK, RO, KB, KS, EZ, ES, RL, and HS approved the final version of the manuscript.
Funding
This research was supported by a grant from the National Science Center (Poland) under grant no: 2019/33/B/NZ7/01980.
Conflict of Interest
The authors declare that the research was conducted in the absence of any commercial or financial relationships that could be construed as a potential conflict of interest.
Acknowledgments
The authors are grateful to the participants who devoted their precious time to the study.
References
Alves, C. R., Gualano, B., Takao, P. P., Avakian, P., Fernandes, R. M., Morine, D., et al. (2012). Effects of acute physical exercise on executive functions: a comparison between aerobic and strength exercise. J. Sport Exerc. Psychol. 34, 539–549. doi: 10.1123/jsep.34.4.539
Alves, C. R., Tessaro, V. H., Teixeira, L. A., Murakava, K., Roschel, H., Gualano, B., et al. (2014). Influence of acute high-intensity aerobic interval exercise bout on selective attention and short-term memory tasks. Percept. Mot. Skills 118, 63–72. doi: 10.2466/22.06.PMS.118k10w4
Astorino, T. A., and Sheard, A. C. (2019). Does sex mediate the affective response to high intensity interval exercise? Physiol. Behav. 204, 27–32. doi: 10.1016/j.physbeh.2019.02.005
Barros, L. F. (2013). Metabolic signaling by lactate in the brain. Trends Neurosci. 36, 396–404. doi: 10.1016/j.tins.2013.04.002
Bartlett, J. D., Close, G. L., MacLaren, D. P., Gregson, W., Drust, B., and Morton, J. P. (2011). High-intensity interval running is perceived to be more enjoyable than moderate-intensity continuous exercise: implications for exercise adherence. J. Sports Sci. 29, 547–553. doi: 10.1080/02640414.2010.545427
Bermon, S., Ferrari, P., Bernard, P., Altare, S., and Dolisi, C. (1999). Responses of total and free insulin-like growth factor-I and insulin-like growth factor binding protein-3 after resistance exercise and training in elderly subjects. Acta Physiol. Scand. 165, 51–56. doi: 10.1046/j.1365-201x.1999.00471.x
Biddle, S. J., and Batterham, A. M. (2015). High-intensity interval exercise training for public health: a big HIT or shall we HIT it on the head? Int. J. Behav. Nutr. Phys. Act. 12:95. doi: 10.1186/s12966-015-0254-259
Burgomaster, K. A., Howarth, K. R., Phillips, S. M., Rakobowchuk, M., Macdonald, M. J., McGee, S. L., et al. (2008). Similar metabolic adaptations during exercise after low volume sprint interval and traditional endurance training in humans. J. Physiol. 586, 151–160. doi: 10.1113/jphysiol.2007.142109
Burgomaster, K. A., Hughes, S. C., Heigenhauser, G. J., Bradwell, S. N., and Gibala, M. J. (2005). Six sessions of sprint interval training increases muscle oxidative potential and cycle endurance capacity in humans. J. Appl. Physiol. 98, 1985–1990. doi: 10.1152/japplphysiol.01095.2004
Carro, E., Nunez, A., Busiguina, S., and Torres-Aleman, I. (2000). Circulating insulin-like growth factor I mediates effects of exercise on the brain. J. Neurosci. 20, 2926–2933. doi: 10.1523/jneurosci.20-08-02926.2000
Cassilhas, R. C., Viana, V. A., Grassmann, V., Santos, R. T., Santos, R. F., Tufik, S., et al. (2007). The impact of resistance exercise on the cognitive function of the elderly. Med. Sci. Sports Exerc. 39, 1401–1407. doi: 10.1249/mss.0b013e318060111f
Chang, Y. K., and Etnier, J. L. (2009). Effects of an acute bout of localized resistance exercise on cognitive performance in middle-aged adults: a randomized controlled trial study. Psychol. Sport Exerc. 10, 19–24. doi: 10.1016/j.psychsport.2008.05.004
Chang, Y. K., Labban, J. D., Gapin, J. I., and Etnier, J. L. (2012). The effects of acute exercise on cognitive performance: a meta-analysis. Brain Res. 1453, 87–101. doi: 10.1016/j.brainres.2012.02.068
Chmura, J., Nazar, K., and Kaciuba-Uscilko, H. (1994). Choice reaction time during graded exercise in relation to blood lactate and plasma catecholamine thresholds. Int. J. Sports Med. 15, 172–176. doi: 10.1055/s-2007-1021042
Clark, P. J., Brzezinska, W. J., Puchalski, E. K., Krone, D. A., and Rhodes, J. S. (2009). Functional analysis of neurovascular adaptations to exercise in the dentate gyrus of young adult mice associated with cognitive gain. Hippocampus 19, 937–950. doi: 10.1002/hipo.20543
Cotman, C. W., Berchtold, N. C., and Christie, L. A. (2007). Exercise builds brain health: key roles of growth factor cascades and inflammation. Trends Neurosci. 30, 464–472. doi: 10.1016/j.tins.2007.06.011
Dall, R., Lange, K. H., Kjaer, M., Jorgensen, J. O., Christiansen, J. S., Orskov, H., et al. (2001). No evidence of insulin-like growth factor-binding protein 3 proteolysis during a maximal exercise test in elite athletes. J. Clin. Endocrinol. Metab. 86, 669–674. doi: 10.1210/jcem.86.2.7180
Dalsgaard, M. K., Ide, K., Cai, Y., Quistorff, B., and Secher, N. H. (2002). The intent to exercise influences the cerebral O(2)/carbohydrate uptake ratio in humans. J. Physiol. 540(Pt 2), 681–689. doi: 10.1113/jphysiol.2001.013062
Decker, E. S., and Ekkekakis, P. (2017). More efficient, perhaps, but at what price? Pleasure and enjoyment responses to high-intensity interval exercise in low-active women with obesity. Psychol. Sport Exerc. 28, 1–10. doi: 10.1016/j.psychsport.2016.09.005
Dienel, G. A. (2017). The metabolic trinity, glucose-glycogen-lactate, links astrocytes and neurons in brain energetics, signaling, memory, and gene expression. Neurosci. Lett. 637, 18–25. doi: 10.1016/j.neulet.2015.02.052
Ding, Q., Vaynman, S., Akhavan, M., Ying, Z., and Gomez-Pinilla, F. (2006). Insulin-like growth factor I interfaces with brain-derived neurotrophic factor-mediated synaptic plasticity to modulate aspects of exercise-induced cognitive function. Neuroscience 140, 823–833. doi: 10.1016/j.neuroscience.2006.02.084
Duman, C. H., Schlesinger, L., Terwilliger, R., Russell, D. S., Newton, S. S., and Duman, R. S. (2009). Peripheral insulin-like growth factor-I produces antidepressant-like behavior and contributes to the effect of exercise. Behav. Brain Res. 198, 366–371. doi: 10.1016/j.bbr.2008.11.016
El Hayek, L., Khalifeh, M., Zibara, V., Abi Assaad, R., Emmanuel, N., Karnib, N., et al. (2019). Lactate mediates the effects of exercise on learning and memory through SIRT1-dependent activation of hippocampal brain-derived neurotrophic factor (BDNF). J. Neurosci. 39, 2369–2382. doi: 10.1523/JNEUROSCI.1661-18.2019
Farias-Junior, L. F., Browne, R. A. V., Freire, Y. A., Oliveira-Dantas, F. F., Lemos, T., Galvao-Coelho, N. L., et al. (2019). Psychological responses, muscle damage, inflammation, and delayed onset muscle soreness to high-intensity interval and moderate-intensity continuous exercise in overweight men. Physiol. Behav. 199, 200–209. doi: 10.1016/j.physbeh.2018.11.028
Ferris, L. T., Williams, J. S., and Shen, C. L. (2007). The effect of acute exercise on serum brain-derived neurotrophic factor levels and cognitive function. Med. Sci. Sports Exerc. 39, 728–734. doi: 10.1249/mss.0b013e31802f04c7
Gibala, M. J., and Little, J. P. (2010). Just HIT it! a time-efficient exercise strategy to improve muscle insulin sensitivity. J. Physiol. 588(Pt 18), 3341–3342. doi: 10.1113/jphysiol.2010.196303
Glenn, T. C., Martin, N. A., Horning, M. A., McArthur, D. L., Hovda, D. A., Vespa, P., et al. (2015). Lactate: brain fuel in human traumatic brain injury: a comparison with normal healthy control subjects. J. Neurotrauma 32, 820–832. doi: 10.1089/neu.2014.3483
Gomez-Pinilla, F., and Hillman, C. (2013). The influence of exercise on cognitive abilities. Compr. Physiol. 3, 403–428. doi: 10.1002/cphy.c110063
Griffin, E. W., Mullally, S., Foley, C., Warmington, S. A., O’Mara, S. M., and Kelly, A. M. (2011). Aerobic exercise improves hippocampal function and increases BDNF in the serum of young adult males. Physiol. Behav. 104, 934–941. doi: 10.1016/j.physbeh.2011.06.005
Hansen, C. J., Stevens, L. C., and Coast, J. R. (2001). Exercise duration and mood state: how much is enough to feel better? Health Psychol. 20, 267–275. doi: 10.1037/0278-6133.20.4.267
Hardcastle, S. J., Ray, H., Beale, L., and Hagger, M. S. (2014). Why sprint interval training is inappropriate for a largely sedentary population. Front. Psychol. 5:1505. doi: 10.3389/fpsyg.2014.01505
Hashimoto, T., Tsukamoto, H., Takenaka, S., Olesen, N. D., Petersen, L. G., Sorensen, H., et al. (2018). Maintained exercise-enhanced brain executive function related to cerebral lactate metabolism in men. FASEB J. 32, 1417–1427. doi: 10.1096/fj.201700381RR
Jahreis, G., Hesse, V., Schmidt, H. E., and Scheibe, J. (1989). Effect of endurance exercise on somatomedin-C/insulin-like growth factor I concentration in male and female runners. Exp. Clin. Endocrinol. 94, 89–96. doi: 10.1055/s-0029-1210884
Jovanovic, J. N., Czernik, A. J., Fienberg, A. A., Greengard, P., and Sihra, T. S. (2000). Synapsins as mediators of BDNF-enhanced neurotransmitter release. Nat. Neurosci. 3, 323–329. doi: 10.1038/73888
Kafitz, K. W., Rose, C. R., Thoenen, H., and Konnerth, A. (1999). Neurotrophin-evoked rapid excitation through TrkB receptors. Nature 401, 918–921. doi: 10.1038/44847
Karege, F., Schwald, M., and Cisse, M. (2002). Postnatal developmental profile of brain-derived neurotrophic factor in rat brain and platelets. Neurosci. Lett. 328, 261–264. doi: 10.1016/s0304-3940(02)00529-3
Kemppainen, J., Aalto, S., Fujimoto, T., Kalliokoski, K. K., Langsjo, J., Oikonen, V., et al. (2005). High intensity exercise decreases global brain glucose uptake in humans. J. Physiol. 568(Pt 1), 323–332. doi: 10.1113/jphysiol.2005.091355
Knaepen, K., Goekint, M., Heyman, E. M., and Meeusen, R. (2010). Neuroplasticity – exercise-induced response of peripheral brain-derived neurotrophic factor: a systematic review of experimental studies in human subjects. Sports Med. 40, 765–801. doi: 10.2165/11534530-000000000-00000
Kohut, M. L., McCann, D. A., Russell, D. W., Konopka, D. N., Cunnick, J. E., Franke, W. D., et al. (2006). Aerobic exercise, but not flexibility/resistance exercise, reduces serum IL-18, CRP, and IL-6 independent of beta-blockers, BMI, and psychosocial factors in older adults. Brain Behav. Immun. 20, 201–209. doi: 10.1016/j.bbi.2005.12.002
Koltai, E., Szabo, Z., Atalay, M., Boldogh, I., Naito, H., Goto, S., et al. (2010). Exercise alters SIRT1, SIRT6, NAD and NAMPT levels in skeletal muscle of aged rats. Mech. Ageing Dev. 131, 21–28. doi: 10.1016/j.mad.2009.11.002
Kraemer, R. R., Durand, R. J., Acevedo, E. O., Johnson, L. G., Kraemer, G. R., Hebert, E. P., et al. (2004). Rigorous running increases growth hormone and insulin-like growth factor-I without altering ghrelin. Exp. Biol. Med. 229, 240–246. doi: 10.1177/153537020422900304
Kraus, R. M., Stallings, H. W. III, Yeager, R. C., and Gavin, T. P. (2004). Circulating plasma VEGF response to exercise in sedentary and endurance-trained men. J. Appl. Physiol. 96, 1445–1450. doi: 10.1152/japplphysiol.01031.2003
Kujach, S., Byun, K., Hyodo, K., Suwabe, K., Fukuie, T., Laskowski, R., et al. (2018). A transferable high-intensity intermittent exercise improves executive performance in association with dorsolateral prefrontal activation in young adults. Neuroimage 169, 117–125. doi: 10.1016/j.neuroimage.2017.12.003
Lambourne, K., and Tomporowski, P. (2010). The effect of exercise-induced arousal on cognitive task performance: a meta-regression analysis. Brain Res. 1341, 12–24. doi: 10.1016/j.brainres.2010.03.091
Landers-Ramos, R. Q., Jenkins, N. T., Spangenburg, E. E., Hagberg, J. M., and Prior, S. J. (2014). Circulating angiogenic and inflammatory cytokine responses to acute aerobic exercise in trained and sedentary young men. Eur. J. Appl. Physiol. 114, 1377–1384. doi: 10.1007/s00421-014-2861-2866
Lee, N. R., Wallace, G. L., Raznahan, A., Clasen, L. S., and Giedd, J. N. (2014). Trail making test performance in youth varies as a function of anatomical coupling between the prefrontal cortex and distributed cortical regions. Front. Psychol. 5:496. doi: 10.3389/fpsyg.2014.00496
Lista, I., and Sorrentino, G. (2010). Biological mechanisms of physical activity in preventing cognitive decline. Cell Mol. Neurobiol. 30, 493–503. doi: 10.1007/s10571-009-9488-x
Little, J. P., Gillen, J. B., Percival, M. E., Safdar, A., Tarnopolsky, M. A., Punthakee, Z., et al. (2011a). Low-volume high-intensity interval training reduces hyperglycemia and increases muscle mitochondrial capacity in patients with type 2 diabetes. J. Appl. Physiol. 111, 1554–1560. doi: 10.1152/japplphysiol.00921.2011
Little, J. P., Safdar, A., Bishop, D., Tarnopolsky, M. A., and Gibala, M. J. (2011b). An acute bout of high-intensity interval training increases the nuclear abundance of PGC-1alpha and activates mitochondrial biogenesis in human skeletal muscle. Am. J. Physiol. Regul. Integr. Comp. Physiol. 300, R1303–R1310. doi: 10.1152/ajpregu.00538.2010
Little, J. P., Safdar, A., Wilkin, G. P., Tarnopolsky, M. A., and Gibala, M. J. (2010). A practical model of low-volume high-intensity interval training induces mitochondrial biogenesis in human skeletal muscle: potential mechanisms. J. Physiol. 588, 1011–1022. doi: 10.1113/jphysiol.2009.181743
Lopez-Lopez, C., LeRoith, D., and Torres-Aleman, I. (2004). Insulin-like growth factor I is required for vessel remodeling in the adult brain. Proc. Natl. Acad. Sci. U.S.A. 101, 9833–9838. doi: 10.1073/pnas.0400337101
Morland, C., Andersson, K. A., Haugen, O. P., Hadzic, A., Kleppa, L., Gille, A., et al. (2017). Exercise induces cerebral VEGF and angiogenesis via the lactate receptor HCAR1. Nat. Commun. 8:15557. doi: 10.1038/ncomms15557
Nishijima, T., Piriz, J., Duflot, S., Fernandez, A. M., Gaitan, G., Gomez-Pinedo, U., et al. (2010). Neuronal activity drives localized blood-brain-barrier transport of serum insulin-like growth factor-I into the CNS. Neuron 67, 834–846. doi: 10.1016/j.neuron.2010.08.007
Olney, N., Wertz, T., LaPorta, Z., Mora, A., Serbas, J., and Astorino, T. A. (2018). Comparison of acute physiological and psychological responses between moderate-intensity continuous exercise and three regimes of high-intensity interval training. J. Strength Cond. Res. 32, 2130–2138. doi: 10.1519/JSC.0000000000002154
Phillips, C., Baktir, M. A., Srivatsan, M., and Salehi, A. (2014). Neuroprotective effects of physical activity on the brain: a closer look at trophic factor signaling. Front. Cell Neurosci. 8:170. doi: 10.3389/fncel.2014.00170
Rex, C. S., Lauterborn, J. C., Lin, C. Y., Kramar, E. A., Rogers, G. A., Gall, C. M., et al. (2006). Restoration of long-term potentiation in middle-aged hippocampus after induction of brain-derived neurotrophic factor. J. Neurophysiol. 96, 677–685. doi: 10.1152/jn.00336.2006
Rojas Vega, S., Abel, T., Lindschulten, R., Hollmann, W., Bloch, W., and Struder, H. K. (2008). Impact of exercise on neuroplasticity-related proteins in spinal cord injured humans. Neuroscience 153, 1064–1070. doi: 10.1016/j.neuroscience.2008.03.037
Ruiz de Almodovar, C., Lambrechts, D., Mazzone, M., and Carmeliet, P. (2009). Role and therapeutic potential of VEGF in the nervous system. Physiol. Rev. 89, 607–648. doi: 10.1152/physrev.00031.2008
Salgueiro, R. B., Peliciari-Garcia, R. A., do Carmo Buonfiglio, D., Peroni, C. N., and Nunes, M. T. (2014). Lactate activates the somatotropic axis in rats. Growth Horm. IGF Res. 24, 268–270. doi: 10.1016/j.ghir.2014.09.003
Saucedo Marquez, C. M., Vanaudenaerde, B., Troosters, T., and Wenderoth, N. (2015). High-intensity interval training evokes larger serum BDNF levels compared with intense continuous exercise. J. Appl. Physiol. 119, 1363–1373. doi: 10.1152/japplphysiol.00126.2015
Schiffer, T., Schulte, S., Hollmann, W., Bloch, W., and Struder, H. K. (2009). Effects of strength and endurance training on brain-derived neurotrophic factor and insulin-like growth factor 1 in humans. Horm. Metab. Res. 41, 250–254. doi: 10.1055/s-0028-1093322
Schinder, A. F., and Poo, M. (2000). The neurotrophin hypothesis for synaptic plasticity. Trends Neurosci. 23, 639–645. doi: 10.1016/s0166-2236(00)01672-1676
Shetty, A. K., Hattiangady, B., and Shetty, G. A. (2005). Stem/progenitor cell proliferation factors FGF-2, IGF-1, and VEGF exhibit early decline during the course of aging in the hippocampus: role of astrocytes. Glia 51, 173–186. doi: 10.1002/glia.20187
Skriver, K., Roig, M., Lundbye-Jensen, J., Pingel, J., Helge, J. W., Kiens, B., et al. (2014). Acute exercise improves motor memory: exploring potential biomarkers. Neurobiol. Learn. Mem. 116, 46–58. doi: 10.1016/j.nlm.2014.08.004
Sloth, M., Sloth, D., Overgaard, K., and Dalgas, U. (2013). Effects of sprint interval training on VO2max and aerobic exercise performance: a systematic review and meta-analysis. Scand. J. Med. Sci. Sports 23, e341–e352. doi: 10.1111/sms.12092
Stranahan, A. M., Khalil, D., and Gould, E. (2007). Running induces widespread structural alterations in the hippocampus and entorhinal cortex. Hippocampus 17, 1017–1022. doi: 10.1002/hipo.20348
Strauss, E., Sherman, E. M. S., and Spreen, O. (2006). A Compendium of Neuropsychological Tests: Administration, Norms, and Commentary. New York, NY: Oxford University Press.
Stroop, J. R. (1935). Studies of interference in serial verbal reactions. J. Exp. Psychol. 18, 643–662. doi: 10.1037/h0054651
Suwabe, K., Hyodo, K., Byun, K., Ochi, G., Fukuie, T., Shimizu, T., et al. (2017). Aerobic fitness associates with mnemonic discrimination as a mediator of physical activity effects: evidence for memory flexibility in young adults. Sci. Rep. 7:5140. doi: 10.1038/s41598-017-04850-y
Tomporowski, P. D. (2003). Effects of acute bouts of exercise on cognition. Acta Psychol. 112, 297–324. doi: 10.1016/s0001-6918(02)00134-8
Townsend, L. K., Islam, H., Dunn, E., Eys, M., Robertson-Wilson, J., and Hazell, T. J. (2017). Modified sprint interval training protocols. Part II. Psychological responses. Appl. Physiol. Nutr. Metab. 42, 347–353. doi: 10.1139/apnm-2016-2479
Trejo, J. L., Carro, E., and Torres-Aleman, I. (2001). Circulating insulin-like growth factor I mediates exercise-induced increases in the number of new neurons in the adult hippocampus. J. Neurosci. 21, 1628–1634. doi: 10.1523/jneurosci.21-05-01628.2001
Tsukamoto, H., Suga, T., Takenaka, S., Tanaka, D., Takeuchi, T., Hamaoka, T., et al. (2016). Greater impact of acute high-intensity interval exercise on post-exercise executive function compared to moderate-intensity continuous exercise. Physiol. Behav. 155, 224–230. doi: 10.1016/j.physbeh.2015.12.021
van Hall, G., Stromstad, M., Rasmussen, P., Jans, O., Zaar, M., Gam, C., et al. (2009). Blood lactate is an important energy source for the human brain. J. Cereb. Blood Flow Metab. 29, 1121–1129. doi: 10.1038/jcbfm.2009.35
Vaynman, S. S., Ying, Z., Yin, D., and Gomez-Pinilla, F. (2006). Exercise differentially regulates synaptic proteins associated to the function of BDNF. Brain Res. 1070, 124–130. doi: 10.1016/j.brainres.2005.11.062
Voss, M. W., Erickson, K. I., Prakash, R. S., Chaddock, L., Kim, J. S., Alves, H., et al. (2013). Neurobiological markers of exercise-related brain plasticity in older adults. Brain Behav. Immun. 28, 90–99. doi: 10.1016/j.bbi.2012.10.021
Wahl, P., Zinner, C., Achtzehn, S., Behringer, M., Bloch, W., and Mester, J. (2011). Effects of acid-base balance and high or low intensity exercise on VEGF and bFGF. Eur. J. Appl. Physiol. 111, 1405–1413. doi: 10.1007/s00421-010-1767-1761
Warr-di Piero, D., Valverde-Esteve, T., Redondo-Castan, J. C., Pablos-Abella, C., and Sanchez-Alarcos Diaz-Pintado, J. V. (2018). Effects of work-interval duration and sport specificity on blood lactate concentration, heart rate and perceptual responses during high intensity interval training. PLoS One 13:e0200690. doi: 10.1371/journal.pone.0200690
Weston, K. S., Wisloff, U., and Coombes, J. S. (2014). High-intensity interval training in patients with lifestyle-induced cardiometabolic disease: a systematic review and meta-analysis. Br. J. Sports Med. 48, 1227–1234. doi: 10.1136/bjsports-2013-092576
Winter, B., Breitenstein, C., Mooren, F. C., Voelker, K., Fobker, M., Lechtermann, A., et al. (2007). High impact running improves learning. Neurobiol. Learn. Mem. 87, 597–609. doi: 10.1016/j.nlm.2006.11.003
Wood, K. M., Olive, B., LaValle, K., Thompson, H., Greer, K., and Astorino, T. A. (2016). Dissimilar physiological and perceptual responses between sprint interval training and high-intensity interval training. J. Strength Cond. Res. 30, 244–250. doi: 10.1519/JSC.0000000000001042
Wrann, C. D., White, J. P., Salogiannnis, J., Laznik-Bogoslavski, D., Wu, J., Ma, D., et al. (2013). Exercise induces hippocampal BDNF through a PGC-1alpha/FNDC5 pathway. Cell Metab. 18, 649–659. doi: 10.1016/j.cmet.2013.09.008
Yakar, S., Liu, J. L., Stannard, B., Butler, A., Accili, D., Sauer, B., et al. (1999). Normal growth and development in the absence of hepatic insulin-like growth factor I. Proc. Natl. Acad. Sci. U.S.A. 96, 7324–7329. doi: 10.1073/pnas.96.13.7324
Yanagisawa, H., Dan, I., Tsuzuki, D., Kato, M., Okamoto, M., Kyutoku, Y., et al. (2010). Acute moderate exercise elicits increased dorsolateral prefrontal activation and improves cognitive performance with Stroop test. Neuroimage 50, 1702–1710. doi: 10.1016/j.neuroimage.2009.12.023
Zacchigna, S., Lambrechts, D., and Carmeliet, P. (2008). Neurovascular signalling defects in neurodegeneration. Nat. Rev. Neurosci. 9, 169–181. doi: 10.1038/nrn2336
Ziemann, E., Olek, R. A., Grzywacz, T., Antosiewicz, J., Kujach, S., Luszczyk, M., et al. (2013). Whole-body cryostimulation as an effective method of reducing low-grade inflammation in obese men. J. Physiol. Sci. 63, 333–343. doi: 10.1007/s12576-013-0269-264
Keywords: sprint interval exercise, BDNF, IGF-1, VEGF, blood lactate, cognitive function
Citation: Kujach S, Olek RA, Byun K, Suwabe K, Sitek EJ, Ziemann E, Laskowski R and Soya H (2020) Acute Sprint Interval Exercise Increases Both Cognitive Functions and Peripheral Neurotrophic Factors in Humans: The Possible Involvement of Lactate. Front. Neurosci. 13:1455. doi: 10.3389/fnins.2019.01455
Received: 08 August 2019; Accepted: 31 December 2019;
Published: 23 January 2020.
Edited by:
Pierrette Gaudreau, Université de Montréal, CanadaReviewed by:
Eduardo Caldas Costa, Federal University of Rio Grande do Norte, BrazilBen Nephew, Worcester Polytechnic Institute, United States
Copyright © 2020 Kujach, Olek, Byun, Suwabe, Sitek, Ziemann, Laskowski and Soya. This is an open-access article distributed under the terms of the Creative Commons Attribution License (CC BY). The use, distribution or reproduction in other forums is permitted, provided the original author(s) and the copyright owner(s) are credited and that the original publication in this journal is cited, in accordance with accepted academic practice. No use, distribution or reproduction is permitted which does not comply with these terms.
*Correspondence: Sylwester Kujach, a3VqYWNoc3lsd2VzdGVyQGdtYWlsLmNvbQ==; c3lsd2VrLWt1amFjaEBvMi5wbA==; Hideaki Soya, c295YS5oaWRlYWtpLmd0QHUudHN1a3ViYS5hYy5qcA==