- 1Mid-Atlantic Mental Illness Research, Education and Clinical Center, Durham VA Medical Center, U.S. Department of Veteran Affairs, Durham, NC, United States
- 2Department of Psychiatry and Behavioral Sciences, Duke University Medical Center, Durham, NC, United States
- 3Brain Imaging and Analysis Center, Duke University, Durham, NC, United States
Background: Neuroactive steroids are endogenous molecules with regenerative and neuroprotective actions. Both cortical thickness and many neuroactive steroid levels decline with age and are decreased in several neuropsychiatric disorders. However, a systematic examination of the relationship between serum neuroactive steroid levels and in vivo measures of cortical thickness in humans is lacking.
Methods: Peripheral serum levels of seven neuroactive steroids were assayed in United States military veterans. All (n = 143) subsequently underwent high-resolution structural MRI, followed by parcellelation of the cortical surface into 148 anatomically defined regions. Regression modeling was applied to test the association between neuroactive steroid levels and hemispheric total gray matter volume as well as region-specific cortical thickness. False discovery rate (FDR) correction was used to control for Type 1 error from multiple testing.
Results: Neuroactive steroid levels of allopregnanolone and pregnenolone were positively correlated with gray matter thickness in multiple regions of cingulate, parietal, and occipital association cortices (r = 0.20–0.47; p < 0.05; FDR-corrected).
Conclusion: Positive associations between serum neuroactive steroid levels and gray matter cortical thickness are found in multiple brain regions. If these results are confirmed, neuroactive steroid levels and cortical thickness may help in monitoring the clinical response in future intervention studies of neuroregenerative therapies.
Introduction
Neuroactive steroids are endogenous molecules that are enriched in human brain, where they are synthesized de novo from cholesterol. They are also produced in the adrenal glands and other peripheral tissues. A number of neuroactive steroids are neuroactive, exhibiting rapid actions at inhibitory GABAA receptors, excitatory NMDA receptors, and other ligand-gated ion channel receptors (Paul and Purdy, 1992). Importantly, a number of neuroactive steroids exhibit neuroregenerative, neuroprotective, and neurotrophic actions, in addition to anti-inflammatory effects. Furthermore, accruing evidence suggests that neuroactive steroids may be effective treatment interventions for several CNS disorders where cortical thickness is reduced and for which neuroactive steroid interventions show potential (Irwin et al., 2014; Vallée, 2016). Neuroactive steroids are thus plausible modulators of brain structure and function, but investigation of neuroactive steroids in conjunction with magnetic resonance imaging (MRI) in humans is currently scant.
The neuroprotective and neuroregenerative potential of neuroactive steroids is particularly promising for developing new therapeutics utilizing neuroactive steroids as interventions and neuroimaging to assess potential treatment effects. For instance, allopregnanolone reduces oxidative stress (Qian et al., 2015) and exhibits neuroprotective actions in animal models of stroke and traumatic brain injury (TBI) (Djebaili et al., 2005; Sayeed et al., 2006), decreases cytokine expression following TBI (He et al., 2004), reduces microglia activation (Chen et al., 2011), and enhances myelin basic protein expression (Ghoumari et al., 2003). Pregnenolone protects against glutamate and amyloid beta protein (Gursoy et al., 2001), while DHEAS and DHEA are protective against excitotoxicity (Kimonides et al., 1998), decrease apoptosis (Charalampopoulos et al., 2004), and enhance axonal and dendritic outgrowth (Compagnone and Mellon, 1998). Allopregnanolone is a GABAergic neuroactive steroid that dose-dependently increases proliferation of rodent and human neural progenitor cells, restores neurogenesis and reverses learning and memory deficits in a mouse model of Alzheimer’s disease (Wang et al., 2010), promotes neurogenesis and survival of newly generated neural cells, and is capable of restoring hippocampal-dependent cognitive performance (Chen et al., 2011; Singh et al., 2012). Consistent with a role for allopregnanolone in the neurobiology and therapeutics of degenerative disorders, allopregnanolone levels are decreased in post-mortem brain tissue of patients with Alzheimer’s disease, and reductions are associated with the presence of the ApoE4 allele (Marx et al., 2006b; Naylor et al., 2010). Peripheral levels of some neuroactive steroids decline with age (Schumacher et al., 2003), and appear to be altered in PTSD (Rasmusson et al., 2006, 2017), schizophrenia (Marx et al., 2009), bipolar disorder (Youssef et al., 2015), depression (Uzunova et al., 1998), and TBI (Marx et al., 2016). Like allopregnanolone, DHEA (Suzuki et al., 2004) and pregnenolone sulfate (Mayo et al., 2005; Xu et al., 2012) also appear to enhance neurogenesis in rodent models.
Few neuroimaging studies in humans have examined associations between neuroactive steroids and brain structure or function despite clear evidence of the regenerative, neuroprotective, neurotrophic, and anti-inflammatory actions of neuroactive steroids in rodents. Many neuroactive steroids in their unsulfated forms are lipophilic and cross the blood-brain barrier. Therefore, closely correlated levels of neuroactive steroids in peripheral blood, cerebrospinal fluid (CSF), and brain would be hypothesized, and there is evidence to support a CSF-brain correlation of DHEA and pregnenolone in human post-mortem brains (Naylor et al., 2008) and a brain-blood correlation of pregnenolone in rats (Marx et al., 2006a). Prior structural MRI investigations have found cortical thickness is associated with DHEA levels during development (Nguyen et al., 2013). Aging is accompanied by cortical thinning throughout adulthood, which appears to be a result of cellular shrinkage and reduced dendritic arborization, rather than neuronal death (Morrison and Hof, 1997). Cortical thinning is also associated with multiple disorders, including first-episode psychosis (Plitman et al., 2016), schizophrenia spectrum symptoms (Watsky et al., 2016), schizophrenia (Rimol et al., 2010; van Erp et al., 2016), bipolar disorder (Hanford et al., 2016), ADHD (Fernández-Jaén et al., 2014), and Alzheimer’s disease (Dickerson et al., 2009), whereas greater cortical thickness appears to be associated with autism (Hyde et al., 2010), and IQ (Narr et al., 2005). Genetic factors also influence cortical thickness, as underscored by its high heritability (h2 = 0.81) (Panizzon et al., 2009). Interestingly, cortical thickness differs between males and females depending on brain region and age, such that generally some brain regions are thicker in men while other brain regions are thicker in women according to a pattern that varies with age (Mutlu et al., 2013).
Our goal was to evaluate possible associations of neuroactive steroid levels with neuronal integrity, inferred from in vivo MRI measurement of cortical gray matter thickness. We quantified seven neuroactive steroids in male military veterans who had undergone MRI scans, followed by parcellelation of the cortical surface. We hypothesized that serum neuroactive steroid levels would be positively correlated with mean cortical thickness of prefrontal and association cortices, given the steroid-induced remodeling that these cortical structures undergo at various developmental periods and the neuroregenerative and neuroprotective properties of these molecules. Finally, we hypothesized that neuroactive steroids may exert stronger influence on cortical thickness for young adults but this influence might be weak in older adults.
Participants and Methods
Participants
Male participants were recruited from a repository of United States military veterans (VA Mid-Atlantic MIRECC, Durham, NC, United States) who served in the United States Military since September 11, 2001. All participants provided written informed consent to procedures that were reviewed and approved by the Institutional Review Boards at Duke University and Durham VA Medical Centers. The overall study included n = 143 participants and a subsample of n = 115 participants, which for convenience are referred to as full-sample (mean age 38.60, SD = 7.14, range 21–65) and subsample (mean age 39.64, SD = 9.78, range 21–65), respectively. Pregnenolone, allopregnanolone, pregnanolone, androsterone, and progesterone levels were only available in a subsample of 115 of the 143 participants. All participants underwent high-resolution structural MRI.
Participant Screening and Clinical Assessment
The screening process excluded major neurological disorders, contraindication to MRI (e.g., metal implants), history of moderate or severe TBI, substance dependence, and age over 65 years. In addition, all Axis I disorders were excluded with the following exceptions: major depressive disorder, PTSD, past alcohol or substance abuse, and current or past nicotine dependence. Specifically, individuals with current and past alcohol or substance dependence, as well as current alcohol or substance abuse, were excluded from participation. Current alcohol or substance abuse is defined as any use in the past 1 month. Use that occurred prior to the past 1 month, is classified as past alcohol or substance abuse. No toxicology screening was done to verify lack of current substance abuse based on empirical evidence that self-report provides an accurate account (Calhoun et al., 2000). Recruitment of United States Military veterans from recent conflicts meant that excluding diagnoses such as PTSD and major depressive disorder was impractical to obtain a sufficient sample size. Therefore, statistical methods were introduced to account for these conditions. Participants were assessed for lifetime occurrence of psychological trauma [Traumatic Life Events Questionnaire (TLEQ; Kubany et al., 2000)], combat exposure [Combat Exposure Scale (CES; Lund et al., 1984)], alcohol abuse [Alcohol Use Disorders Test (AUDIT; Saunders et al., 1993)], smoking status assessed by the Fagerstrom test for nicotine dependence (Heatherton et al., 1991), and depressive symptoms [Beck Depression Inventory-II (BDI-II; Beck et al., 1996)]. Child trauma was coded from the TLEQ based on the number of categories (0, 1, 2, or more) of trauma experienced. Diagnosis of PTSD was ascertained with the Clinician Administered PTSD Scale (CAPS). Severity of TBI was operationalized based on the criteria for mild TBI that are defined by the American College of Rehabilitation Medicine (ACRM). Individuals who exceeded the ACRM criteria were categorized in the moderate to severe category and therefore excluded.
MRI Acquisition
All images were acquired on a GE 3-Tesla scanner equipped with an 8-channel head coil at Duke University using high-resolution T1-weighted whole-brain axial images with 1 mm isotropic voxels. High-resolution T1-weighted whole-brain axial images with 1-mm isotropic voxels were obtained with array spatial sensitivity encoding technique (ASSET) and fast spoiled gradient-recall (3D-FSPGR). Image parameters were optimized for contrast between white matter, gray matter, and CSF on either the (1) GE Discovery MR750 (n = 162) (TR/TE/flip angle = 7.484-ms/2.984-ms/12°, FOV = 256-mm, 1-mm slice thickness, 166 slices, 256 × 256 matrix, 1 excitation), or the (2) GE EXCITE (n = 90) (TR/TE/flip angle = 8.208-ms/3.22-ms/12°, FOV = 256-mm, 1-mm slice thickness, 166 slices, 256 × 256 matrix, 1 excitation).
Regional Cortical Thickness Analysis
All T1-images were visually inspected to assure sufficient quality for automated segmentation and labeling, which were performed using the FreeSurfer image analysis suite1 (version 5.3.0) and its library tool recon-all. Details of FreeSurfer parcellations have been previously described (Fischl et al., 2004; Destrieux et al., 2010). Briefly, the technique automatically assigns a neuroanatomical label to each location of a cortical surface model based on probabilistic information estimated from a manually labeled training set and geometric information derived from the cortical model. Mean cortical thickness measures were calculated for 148 neuroanatomical regions (74 per hemisphere) with the aparc.a2009s template (Destrieux et al., 2010).
Quantification of Serum Neuroactive Steroid Levels
All serum samples were drawn between 10:30 AM and 2:30 PM to minimize the effects of diurnal variations and collected at the time that participants entered the registry. Peripheral serum levels of three neuroactive steroids were quantified via radioimmunoassay: dehydroepiandrosterone (DHEA; full-sample), dehydroepiandrosterone sulfate (DHEAS; full-sample), and progesterone (subsample). Peripheral serum levels of four neuroactive steroids (allopregnanolone, pregnenolone, androsterone, and pregnanolone) were quantified in the subsample by mass spectrometry in male military veterans. For the DHEA quantifications (Beckman Coulter), the sensitivity of the RIA is 0.06 ng/mL, the intra-assay coefficient of variation is 3.8%, and the inter-assay coefficient of variation is 8.6%. For the DHEAS quantifications (Beckman Coulter), the sensitivity of the RIA is 12.33 ng/mL, the intra-assay coefficient of variation is 5.2%, and the inter-assay coefficient of variation is 5.3% (please note, DHEAS quantifications can only be quantified indirectly by RIA following cleavage of the sulfate group). For both DHEA and DHEAS, cross-reactivity with other steroids was described by the manufacturer as being “extremely low.” For progesterone (MP Biomedical), the sensitivity is 0.05 ng/mL, the commercial intra-assay coefficient of variation is 2.3% and the inter-assay coefficient of variation is 4.9%. Cross-reactivity for the progesterone RIA was reported by the manufacturer for 20 steroids as follows: for two steroids tested, cross-reactivity was <5.5% (i.e., 20α-dihydroprogesterone = 5.41%, desoxycorticosterone 3.80%), for five additional steroids tested cross-reactivity was <1.0%, and for the remaining 13 steroids tested cross-reactivity was <0.01%. Secondary to limited serum volume availability, RIAs were not run in duplicate, and the above coefficients of variation represent commercial values reported by the manufacturer.
NS quantification of the remaining neuroactive steroids was performed by highly sensitive and specific GC/MS preceded by a high performance liquid chromatography (HPLC) purification step. Serum was homogenized in distilled water containing 2 fmol of tritiated NS of interest (New England Nuclear) and 400 pg of the deuterium-labeled NS of interest (Cambridge Isotope Laboratories). Supernatants were extracted three times with ethyl acetate and dried under nitrogen prior to HPLC, as described by Sripada et al. (2013a, b). HPLC purification was performed on an 1100 Series Agilent HPLC utilizing hexane, tetrahydrofuran, and ethanol as the mobile phase and an Alltech LiChrosorb DIOl 250 mm × 4.6 mm column. The HPLC fractions containing the NS of interest were derivatized utilizing heptafluorobutyric acid anhydride (HFBA).
Mass spectrometry for the derivatized steroids was performed in the EI mode utilizing helium as the carrier gas on an Agilent 5973 MS coupled to an Agilent 6890N GC equipped with an Agilent HP-5MS 30 m × 0.250 mm × 0.25 um capillary column. In addition to the GC retention time characteristic of each steroid, the structural identification of each NS assayed was provided by its unique mass fragmentation pattern. We utilized the single ion-monitoring (SIM) mode of the mass spectrometer to focus on the most abundant ion fragment for each steroid derivative. For NS quantification, the standard curve for the steroid of interest was prepared by combining varying known quantities of steroids (Steraloids) ranging from 1 to 3000 pg with a constant amount of deuterated internal standard. The area under the peak of the internal standard was divided by the area under the peak of the known quantity of each steroid and plotted against the quantity of each steroid to generate the standard curve. Limit of NS detection with this method is 1 pg for each NS. A subset of the samples (7%) was quantified in duplicate; intra-assay coefficients of variation were 4.3% for allopregnanolone, 1.8% for pregnenolone, 1.0% for pregnanolone, and 2.0% for androsterone.
The blood sample was obtained in the resting state with the participant seated comfortably in an ambulatory clinic setting. The participant had not eaten or smoked for at least 30 min prior to the blood draw based on the duration of study procedures that preceded the blood draw. The blood draw preceded the MRI scan for all subjects (sample-1 mean = 1.37 years SD = 1.83; sample-2 mean 1.25 years, SD = 1.80). RIA was utilized to quantify serum levels of DHEA (Beckman Coulter), progesterone (MP Biomedical), and DHEAS (Beckman Coulter), according to manufacturer directions. The time range between blood draw and MRI scan for sample-1 and sample-2 was from 1 day (0.003 years) up to 3,259 days (8.9 years). Moreover 87.4% (125/143) of participants underwent MRI scanning within 3 years of blood draw.
Statistical Analysis
Analyses of serum neuroactive steroid levels and MRI cortical thickness were conducted in male participants for whom both an MRI scan and neuroactive steroid levels were available (n = 115 for allopregnanolone, pregnenolone, androsterone, pregnanolone, progesterone; n = 143 for DHEA and DHEAS levels). Zero-order (bivariate) Pearson correlations were computed in the initial stage between all pairings of seven neuroactive steroids and left hemisphere, right hemisphere, and bilateral global gray matter volume. The corresponding correlation coefficient (r) and p-value for each correlation was Bonferroni corrected for 21 multiple tests (7 neuroactive steroids × 3 brain measures). Local and regional neurostructural associations were investigated in the initial stage with zero-order (bivariate) Pearson correlations computed between all pairings of seven neuroactive steroid and 148 regional cortical thickness measures (1,036 comparisons). The corresponding t-statistic and p-value for each correlation was corrected for multiple testing by controlling the False Discovery Rate (FDR) as reported by Benjamini and Hochberg (1995). For comparison, the adaptive FDR procedure developed by Storey et al. (2004) and Bonferroni correction (Perneger, 1998) were also applied. In cases where a high proportion of the null hypotheses are false, as in the case of widespread effects such as cortical thinning, adaptive FDR methods can paradoxically reject more null hypotheses than using uncorrected p-values (Reiss et al., 2012).
The main analyses were performed with ordinary least squares (OLS) regression procedures on the findings from the initial stage that survived the Benjamini and Hochberg FDR correction. Regional cortical thickness was regressed on a set of independent variables including serum neuroactive steroid level, age, depression score, alcohol use, PTSD diagnosis, childhood trauma exposure, antidepressant medication use, and smoking status. All participants were male, and thus no adjustment for sex was necessary. Five neuroactive steroids out of the seven had significant associations with cortical regions that persisted following FDR correction (p < 0.05) for multiple testing based on 1,036 tests (7 neuroactive steroids × 148 brain regions). These included allopreganolone (59 regions), pregnenolone (46 regions), DHEAS (46 regions), DHEA (13 regions), and androsterone (6 regions). To avoid overfitting, predictor variables lacking associations (p > 0.1) were removed from the regression model and the model was re-estimated with the remaining predictors. Among the tested covariates, only age was a consistent predictor of cortical thickness for all five neuroactive steroid candidates. Based on results that frequently showed both age and neuroactive steroid level as significant predictors, we estimated a second set of models testing age as a modulating (interaction) factor in the association between neuroactive steroid levels and cortical thickness. To visualize the results of the statistical interaction between age and neuroactive steroid level, we dichotomized age to young (<40 years) and old (>40 years). An age-cutoff of 40 years was chosen based on (1) an observed significant decline at age 40 years (polynomial fit), particularly for allopreganolone, and (2) median age of the sample was 40 years. Finally, we examined whether the time elapsed between the blood draw and MRI scan differentially influenced cortical thickness based on the neuroactive steroid level by calculating the interaction of years elapsed and neuroactive steroid level.
We tested whether the correlation between neursteroid level and cortical thickness grows stronger (or weaker) when the duration between blood draw and MRI scan is short (<200 days) or when it is long (>200 days). We selected 200 days because it was the median split in the number of days between blood draw and MRI scan. A significant difference in the correlation between short and long intervals, which could be visualized as different slopes of the fitted line for short and long durations, would indicate that the elapsed time between blood draw and MRI is affecting the relationship between neuroactive steroid level and cortical thickness. The interaction was tested with the R function cocor (Diedenhofen and Musch, 2015).
Vertex-Wide Analysis
Cortical thickness surface maps were generated following registration of all participants’ cortical reconstructions to the FreeSurfer common average surface. Surface maps were smoothed by use of default kernel of 10 mm FWHM. The statistical parametric analysis was performed by the built-in Qdec pipeline implemented in FreeSurfer, by use of a general linear model. Cortical thickness across the whole cortical surface was regressed on a set of independent variables including serum neuroactive steroid level, age, depression score, alcohol use, PTSD diagnosis, childhood trauma exposure, antidepressant medication use, and smoking status. All participants were male, and thus no adjustment for sex was necessary. Correction for multiple comparisons was achieved with a voxel-wise cluster-forming threshold (CFT) of p < 0.01 and cluster-wise threshold of p < 0.05, Monte Carlo corrected based on 10,000 simulations (Greve and Fischl, 2017).
Results
Demographic and Clinical Characteristics
Demographic and clinical information are reported in Table 1 with group mean and descriptive statistics reported separately for the subsample (n = 115) and full-sample (n = 143). Assays for pregnenolone, allopregnanolone, pregnanolone, androsterone, and progesterone were performed in only the subsample.
Association of Neuroactive Steroids and Regional Cortical Thickness
We found highly significant correlations of left (L), right (R), and bilateral (B/L) hemisphere gray matter volume with allopregnanolone (L: r = 0.458, p = 1.86 × 10–6; R: r = 0.459, p = 1.64 × 10–6; B/L: r = 0.460, p = 1.62 × 10–6; corrected) and pregnenolone (L: r = 0.355, p = 0.001; R: r = 0.360, p = 0.001; B/L: r = 0.358, p = 0.001; corrected). The relationships of hemispheric cortical volume with the remaining neuroactive steroids were non-significant after correction for the number of neuroactive steroids tested (Table 2). The significant associations of global mean values with neuroactive steroids prompted a finer grained investigation of the association between regional cortical thickness and neuroactive steroid levels.
The number of significant results from bivariate correlations of the seven neuroactive steroids with 148 cortical regions are shown in Figure 1 using various approaches to multiple comparison testing: (1) uncorrected, (2) adaptive FDR correction of Storey (Storey et al., 2004), (3) FDR correction of Benjamini and Hochberg (1995), and (4) Bonferroni correction (Perneger, 1998). Final regression analyses were conducted on significant results identified by FDR criteria (Benjamini and Hochberg, 1995).
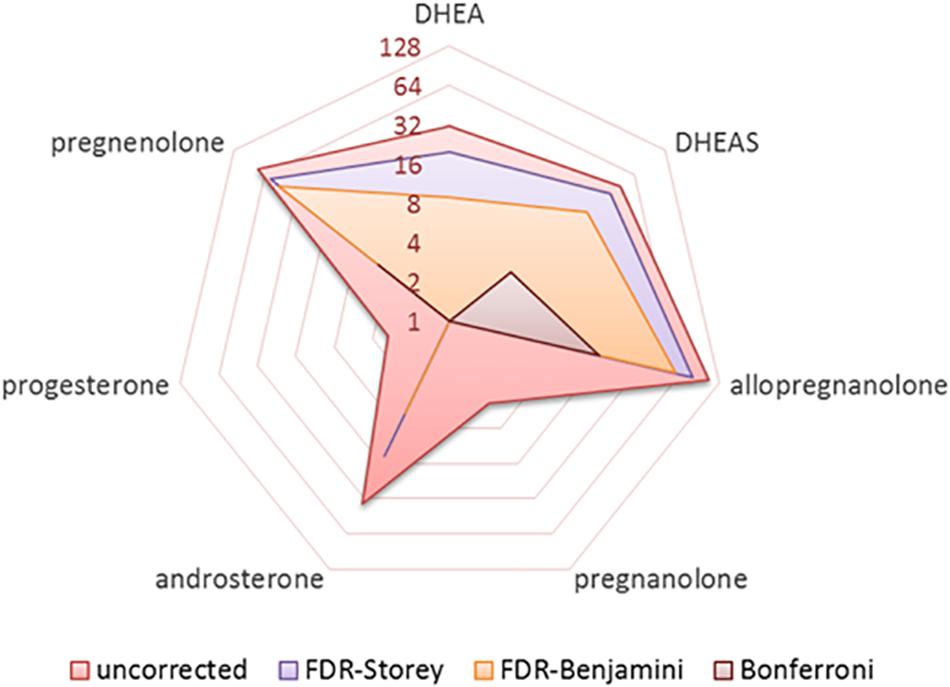
Figure 1. Radar plot showing the number of significant results (log base-2) obtained from bivariate correlations of seven neurosteroids with 148 cortical regions (1036 total tests) for various approaches to handling multiple comparisons including uncorrected, FDR correction of Benjamini and Hochberg, pFDR correction of Storey, and Bonferroni correction.
Five neuroactive steroids, specifically allopregnanolone, pregnenolone, DHEAS, DHEA, and androsterone, were significantly correlated with cortical thickness in multiple brain regions [p < 0.05; FDR-corrected (1995)]. Two neuroactive steroids, pregnanolone and progesterone, did not exhibit significant bivariate associations with cortical regions that persisted following FDR correction (1995).
Allopregnanolone
Bivariate correlations revealed that allopregnanolone had significant associations with gray matter cortical thickness in 59 cortical regions (p < 0.05; FDR-corrected). Regression analysis with all predictor variables revealed that aside from neuroactive steroid level, only age contributed significantly to the model. The remaining variables, depression score, alcohol use, PTSD diagnosis, childhood trauma exposure, antidepressant medication use, and smoking status were non-significant with respect to cortical thickness (FDR-corrected). Results from a restricted model including only age and neuroactive steroid level showed age was significantly associated with all 59 regions that were identified in the bivariate correlations, and that neuroactive steroid level was significantly associated with cortical thickness in 21 regions, including: left middle posterior cingulate gyrus/sulcus, left cuneus gyrus, left middle occipital gyrus, left superior occipital gyrus, left occipito-temporal and medial lingual gyrus, left orbital gyrus, left superior parietal gyrus, left intraparietal sulcus, left occipital and middle lunatus, left occipital superior gyrus, and transversal sulcus, right middle posterior cingulate gyrus/sulcus, right cuneus, right middle occipital gyrus, right superior occipital gyrus, right occipito-temporal lateral fusiform, right occipito-temporal medial and lingual gyrus, right superior parietal gyrus, right rectus gyrus, right marginal cingulate sulcus, right transverse posterior collateral sulcus, and right postcentral sulcus (Figure 2 and Table 3).
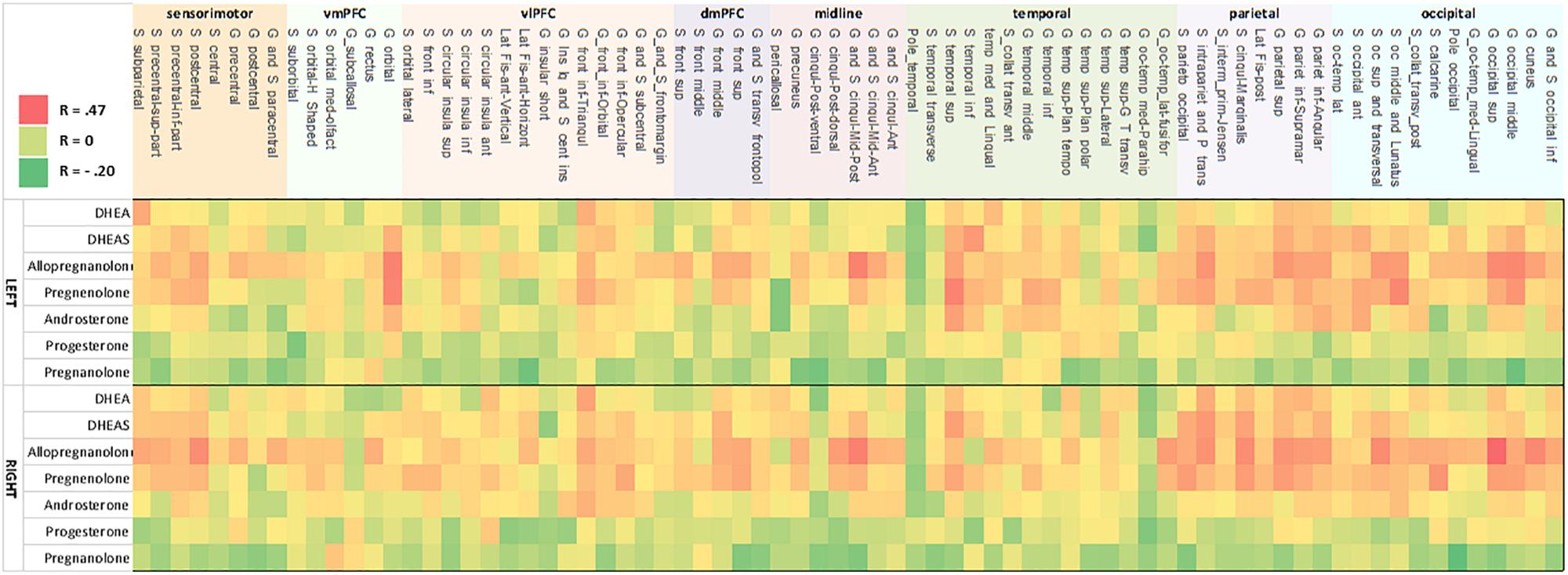
Figure 2. Heat map of correlation between neurosteroid level and cortical thickness. Correlation strength between neurosteroid level (y-axis) and cortical thickness (x-axis) for the left hemisphere (upper heat map) and right hemisphere (lower heat map). The pattern shows concordant relationships among DHEAS, allopregnanolone, pregnenolone, and to a lesser extent with androsterone as well as concordant results between the left and right hemisphere. The strongest correlations are present in inferior frontal cortex, temporoparietal, occipitoparietal, and occiptiotemporal association cortices. Nomenclature of cortical structure is per definitions provided by Destrieux et al. (2010).
Pregnenolone
Bivariate correlations revealed pregnenolone had significant associations with 46 cortical regions (p < 0.05; FDR-corrected). Regression analysis with all predictor variables revealed that only age contributed significantly to the model (FDR-corrected). Results showed a significant association of age with cortical thickness for all 46 regions and a significant association of neuroactive steroid level with cortical thickness for eight regions, including: left middle occipital gyrus, left orbital gyrus, left occipital and middle lunatus, left superior temporal gyrus, right middle occipital gyrus, right superior occipital gyrus, right superior parietal gyrus, right calcarine sulcus (Figure 2 and Table 4). Associations between cortical thickness and neuroactive steroid levels, particularly for allopregnanolone and pregnenolone, modulated many of the same neuroanatomical regions. For instance, the results of bivariate correlations revealed that allopregnanolone and pregnenolone showed concordance in modulating 33 of the same neuroanatomical regions in the initial model utilizing all of the above predictor variables (Table 4).
Androsterone
Bivariate correlations revealed androsterone had significant associations (p < 0.05; FDR-corrected) with six cortical regions (Figure 2 and Table 5). In subsequent regression modeling, only age and androsterone retained significant associations with cortical thickness. Accordingly, the models were re-estimated in each of the six identified regions with only the latter two covariates retained. Regression analysis with all predictor variables revealed that age contributed significantly to the model (FDR-corrected). Results were similar across each of the six models: only age remained significantly associated with cortical thickness (Table 5).
DHEA
Bivariate correlations identified significant (p < 0.05; FDR-corrected) associations between thickness and DHEA in 13 cortical regions (Figure 2 and Table 6). In subsequent regression modeling, only age and DHEA retained significant associations with cortical thickness. Accordingly, the models were re-estimated in each of the 13 identified regions with only the latter two covariates retained. Results from a restricted model including only age and neuroactive steroid level showed a significant association with age for all 13 regions and a significant association with cortical thickness in the triangularis portion of the left and right inferior frontal gyrus (Table 6).
DHEAS
Bivariate correlations revealed DHEAS had significant associations (p < 0.05; FDR-corrected) with 46 cortical regions (Figure 2 and Table 7). Regression analysis with all predictor variables revealed that only age contributed significantly to the model. Results from a reduced model including only age and neuroactive steroid level showed a significant association with age for all 46 regions and a significant association with cortical thickness in the left inferior temporal sulcus (Table 7).
Interaction of Age and Neuroactive Steroids With Regional Cortical Thickness
The association of cortical thickness with the interaction of neuroactive steroid level × age revealed significant results for DHEAS with left orbital gyrus (p = 0.04) (Figure 3A), DHEAS with the anterior horizontal part of the right lateral fissure (p = 0.02) (Figure 3B). This finding of positive associations between cortical thickness and DHEA and DHEAS levels in younger participants only (i.e., those younger than 40 years of age) was restricted to these two neuroactive steroids and to only two brain regions, and may thus potentially reflect Type 1 error in this exploratory analysis.
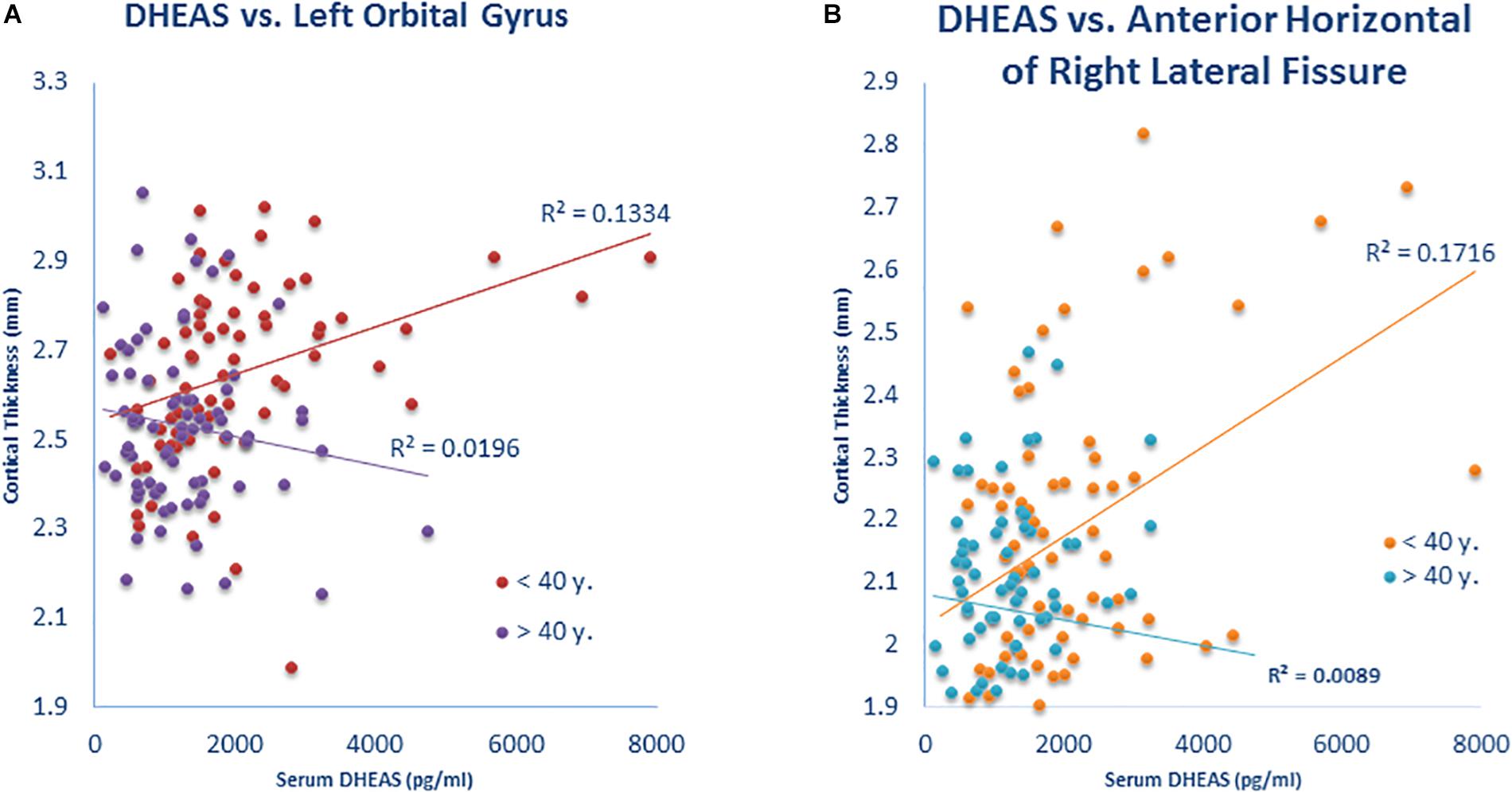
Figure 3. Interaction of neurosteroid level × age is associated with cortical thickness. (A) Participants under 40 years showed a positive correlation (r = 0.365) of DHEAS with cortical thickness in the left orbital gyrus but the correlation was not present (r = –0.140) in participants over 40 years (Z = 3.08; p = 0.002). (B) Participants under 40 years showed a positive correlation (r = 0.414) of DHEAS with cortical thickness in the anterior horizontal portion of the right lateral fissure but the correlation was not present (r = –0.094) in participants over 40 years (Z = 3.14; p = 0.002).
Association of Clinical Variables With Neuroactive Steroid Levels
There were significant negative correlations of age with DHEA (r = −0.536; p = 1.5 × 10–8; FDR-corrected), DHEAS (r = −0.446; p = 9.4 × 10–6; FDR-corrected), allopregnanolone (r = −0.403; p = 7.7 × 10–5; FDR-corrected), androsterone (r = −0.323; p = 3.5 × 10–3; FDR-corrected), and pregnenolone (r = −0.407; p = 7.7 × 10–5; FDR-corrected). Age was not correlated with pregnanolone or with progesterone. All the associations between the remaining clinical variables (depression score, PTSD diagnosis, childhood trauma, alcohol use, medication use, smoking status) and each of the neuroactive steroids were non-significant (FDR-corrected).
Variability in Neuroactive Steroid Levels
We quantified variability or dispersion within our sample with the coefficient of variation (CV = standard deviation/mean). The coefficient of variation was similar for all the neuroactive steroids in the current sample, except for progesterone, which had higher values (Table 8). However, the results reported in the present manuscript had no significant associations of progesterone with cortical thickness. These results were consistent with the coefficient of variation in a superset of males (n = 380) who had neuroactive steroid measures but lacked MRI scans (Table 8).
Stability of Neuroactive Steroids Over Time
We did not detect a significant differential effect of time between blood draw and MRI scan on the association between neuroactive steroid levels and cortical thickness that was determined by calculating the interaction of years elapsed and neuroactive steroid level. We further tested for the stability of neuroactive steroid levels over time given the time elapsed between blood draw and MRI scan (sample-1 mean = 1.37 years, SD = 1.83 years; sample-2 mean 1.25 years, SD = 1.80 years). The sample was binned by median split of the time between blood draw and MRI scan. Half the subjects received early scans, within 0.548 years (200 days), and half who received late scans, after 0.548 years. We plotted the log base-10 transformed neuroactive steroid levels against cortical thickness separately for the early and late subgroups. The scatter plots show the correlation between both allopregnanolone (Figure 4) and pregnenolone (Figure 5). Mean cortical thickness was not significantly affected by the elapsed time between blood draw and MRI scan (allopregnanolone, p = 0.367; pregnenolone, p = 0.501). Similarly, the other five neuroactive steroids lacked a relationship with cortical thickness that was significantly influenced by time.
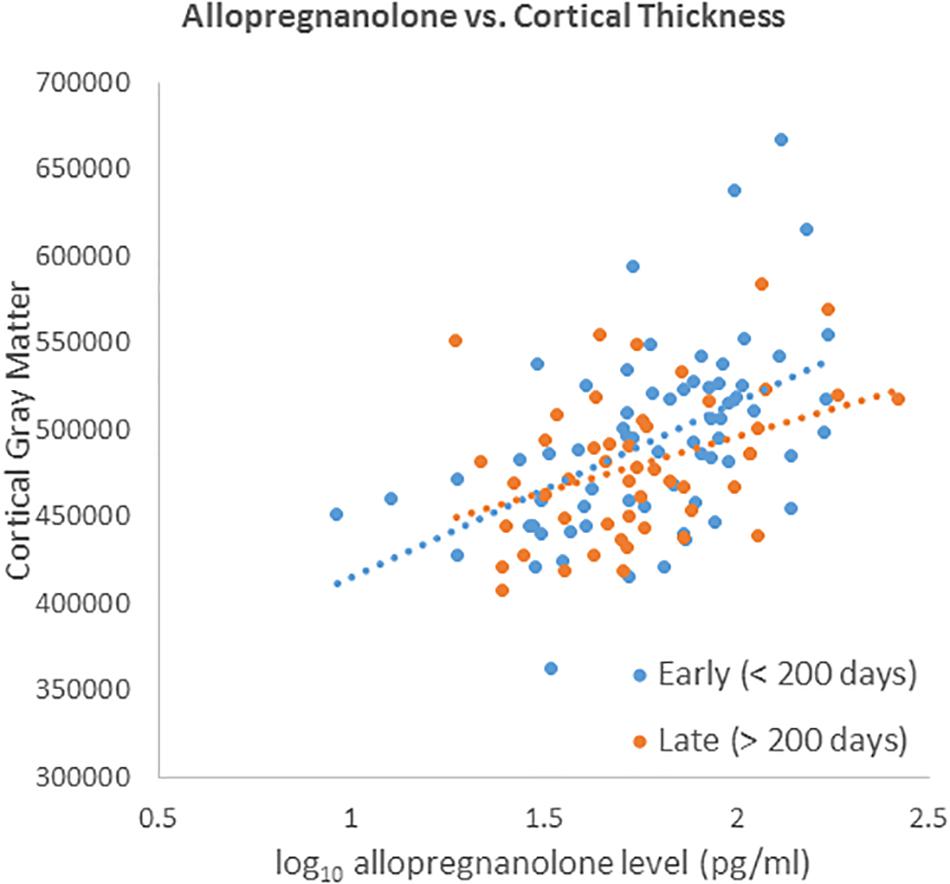
Figure 4. Scatter plot of log10 transformed allopregnanolone levels (x-axis) against cortical thickness (y-axis) separately for the early (blue dots/line) and late subgroups (red dots/line). Fisher’s r-to-z showed no significant difference between early (<200) and late (>200) correlations (Z = 0.901, p = 0.367).
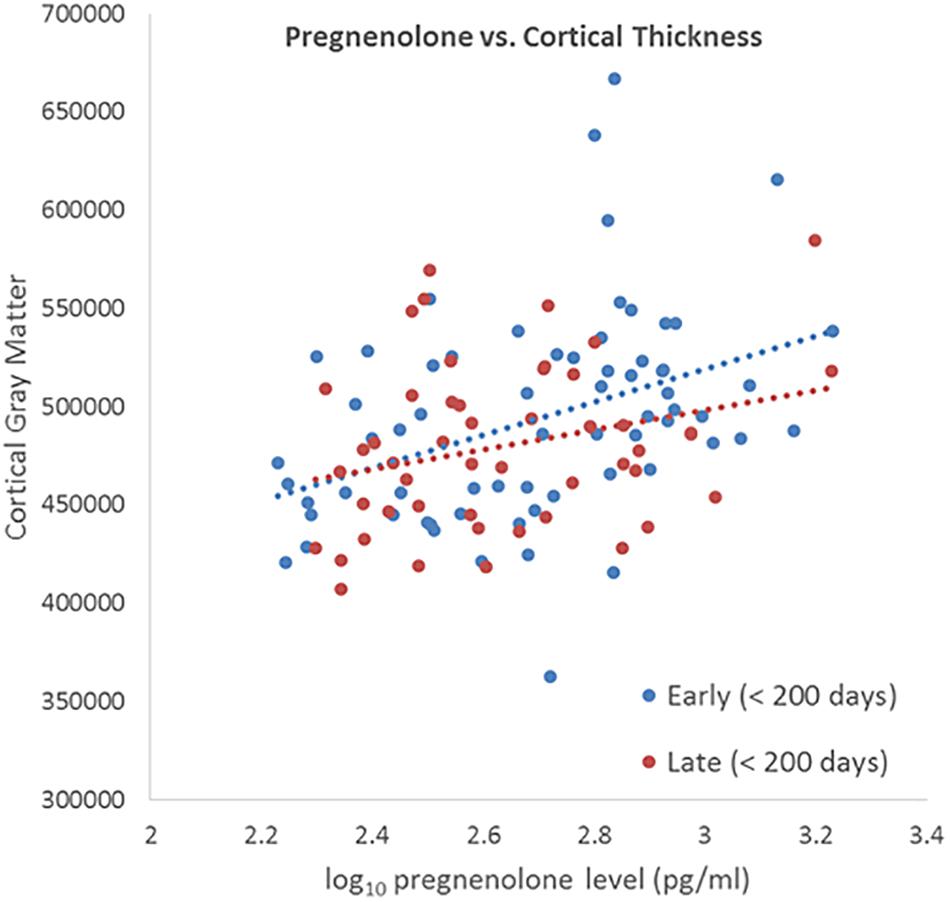
Figure 5. Scatter plot of log10 transformed pregnenolone levels (x-axis) against cortical thickness (y-axis) separately for the early (blue dots/line) and late subgroups (red dots/line). Fisher’s r-to-z showed no significant difference between early (<200) and late (>200) correlations (Z = 0.673, p = 0.501).
The correlation between neuroactive steroids levels across participants is provided as a cross-correlation (Table 9). As expected, specific neuroactive steroids were highly correlated with each other; allopregnanolone and pregnenolone were the most highly correlated (r = 0.494; p = 3.0 × 10 –8), as well as pregnenolone and DHEA (r = 0.492; p = 4.1 × 10–10).
Vertex-Wide Cortical Thickness Association With Neuroactive Steroids
Vertex-wide cortical thickness analysis showed an association with pregnenolone in the left lateral occipto-temporal cortex (Figure 6A), and with allopregnanolone in the right medial occipital cortex (Figure 6B) and right inferior medial temporo-occipital cortex (Figure 6C) (CFT p < 0.01; corrected).
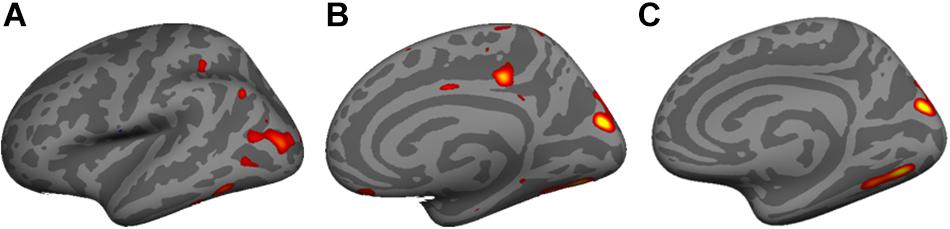
Figure 6. Vertex-wide cortical thickness analysis showed an association with (A) pregnenolone in the left lateral occipto-temporal cortex, and (B) with allopregnanolone in the right medial occipital cortex and (C) right inferior medial temporo-occipital cortex (Cluster Forming Threshold (CFT) p < 0.01; corrected).
Discussion
Neuroactive steroid levels of allopregnanolone and pregnenolone in the peripheral circulation were positively associated with gray matter cortical thickness in multiple brain regions after FDR correction, primarily in cingulate, parietal and occipital association cortices of the brain. The effects of allopregnanolone and pregnenolone showed a similar pattern of associations with cortical thickness across many of the same brain regions. The relatively widespread nature of the FDR-corrected results suggest that the association between neuroactive steroids and cortical thickness may be more global than we report, such that brain regions with negative findings might be explained by inadequate statistical power and the influence of other modulators of cortical thickness that were not included in our model. Nevertheless, the present results are preliminary and will therefore require confirmation as elaborated below. Among the covariates we considered in the initial statistical model, only age significantly impacted the association between neuroactive steroid level and cortical thickness. Given the lack of significant covariate effects, and to avoid overfitting the model, only age was utilized as a covariate in the final set of analyses. Other covariates, while relevant to our military veteran sample (depression, past alcohol abuse, smoking, PTSD, combat exposure, and childhood trauma), were omitted. Results of the moderator analysis show that correlations between age and cortical thickness were largely independent of the association between neuroactive steroid levels and cortical thickness (except for DHEA and DHEAS in specific brain regions). We found that DHEA and DHEAS possess a strong positive correlation with specific cortical regions, such as the circular sulcus of the insula, lateral fissure, and orbital gyrus in young adults, but that this relationship is absent in relatively old adults. To our knowledge, our study is the first to describe an association between cortical thickness and neuroactive steroids in adults.
Cortical thickness quantification obtained from MRI can be influenced by a number of factors. Histology suggests cortical thinning is unlikely to originate from neuronal death, as careful post-mortem studies have found relatively comparable neuronal counts between older and younger subjects, a finding that is supported in non-human primates. Rather, cellular shrinkage and reduction in dendritic arborization are more likely to account for cortical thinning (Morrison and Hof, 1997). Developmental cortical gray-matter thinning is thought to result from both synaptic pruning and myelination (Sowell et al., 2001, 2004; O’donnell et al., 2005; Dosenbach et al., 2010). Over the course of childhood, white-matter volume expands via myelin proliferation and replaces gray matter (Sowell et al., 2004; #12189). This process results in smaller estimates of cortical gray-matter thickness (Kharitonova et al., 2013). Therefore, cortical thinning can be a consequence of increased myelination that may occur well into adulthood. For instance, myelination of a key relay zone in the hippocampal formation occurs in the human brain during childhood, adolescence, and adulthood (Benes et al., 1994). Alternatively, increase in cortical thickness accompanies learning, which correlates histologically with cellular events in gray matter that may be detected by MRI such as axon sprouting, dendritic branching and synaptogenesis, neurogenesis, changes in glial number and morphology, and angiogenesis (Zatorre et al., 2012). Given the regenerative, neuroprotective, and anti-inflammatory actions of neuroactive steroids, among other pleiotropic effects, the possible mechanistic processes of neuroactive steroids’ modulatory effects on cortical thickness are multi-faceted. Allopregnanolone is a positive allosteric modulator of both extrasynaptic and synaptic GABAA receptors (potentiating GABAA responses more potently than benzodiazepines or barbiturates) (Morrow et al., 1987, 1990; Lambert et al., 2009; Carver and Reddy, 2013; Reddy, 2018), which is another candidate mechanism that explains the actions of neuroactive steroids as modulators of cortical thickness (Belelli and Lambert, 2005), as well as their therapeutic potential (Irwin et al., 2014; Vallée, 2016). The neuroactive steroids allopregnanolone and pregnenolone seemed to particularly impact cortical thickness. These neuroactive steroids are not necessarily more abundant in brain (based on recent human post-mortem brain tissue investigations), and it is unclear whether they are more or less efficiently transported in the brain. While allopregnanolone and pregnenolone positively impact myelination/synaptogenesis, less is known about other neuroactive steroids such as androsterone and pregnanolone.
Although enhanced neurogenesis and other neuroactive steroid actions represent plausible mechanisms, non-neuronal factors such as changes to vasculature, which accounts for 5% of gray matter, and glial cells, which outnumber neurons 6:1, could also contribute to enhanced cortical thickness (Zatorre et al., 2012). However, because our findings are correlational and not causal, an alternative or parallel explanation is that participants who possess greater cortical thickness have more cortical gray matter volume that is capable of synthesizing neuroactive steroids (i.e., an increased capacity for synthesis of neuroactive steroids within cortical gray matter could be reflected in increased neuroactive steroid levels in the periphery). While possible, this scenario requires that neuroactive steroids synthesized in the brain make their way into the peripheral circulation, where our quantifications were obtained. Given the lipophilicity of many neuroactive steroids and their ability to cross the blood-brain-barrier (BBB) in their unsulfated forms, determining the compartmentalization and potential movements of these molecules in brain and blood has been very challenging. To date, the evidence from multiple laboratories shows that peripheral neuroactive steroids cross the BBB and subsequently cause elevations in neuroactive steroid levels in brain (Wang et al., 1997; Marx et al., 2003, 2006a). To our knowledge, there is no evidence at this time that the converse occurs, but this possibility appears tenable. It is also possible that other factors may independently impact neuroactive steroid levels and cortical thickness, such as the expression of genes regulating cellular proliferation in the brain, adrenals, and/or other regions.
Findings of relatively widespread positive correlations between cortical thickness and neuroactive steroids warrants further investigation into whether there is a possible causal relationship between neuroactive steroid levels and cortical thickness. If confirmed, further research could be pursued to assess neuroactive steroids as therapeutic agents for psychiatric and neurological conditions that are affected by cortical thinning. Reductions in neuroactive steroid molecules have been reported in multiple CNS disorders. If a contributory link between low neuroactive steroids and CNS disorder is established, amelioration of neuroactive steroid deficits through exogenous supplementation could be clinically efficacious (Irwin et al., 2014; Vallée, 2016), as supported by evidence in schizophrenia (Marx et al., 2011, 2014), bipolar disorder (Osuji et al., 2010; Brown et al., 2014), severe postpartum depression (Kanes et al., 2016), super-refractory status epilepticus (Reddy and Kuruba, 2013; Broomall et al., 2014), and TBI (Marx et al., 2016), which are associated with cortical thinning (Shaw et al., 2006; Dickerson et al., 2009; Rimol et al., 2010; Hanford et al., 2016; van Erp et al., 2016). Investigation of neuroactive steroids in CNS conditions is supported by previous neuroimaging investigations involving one-time neuroactive steroid administration and fMRI paradigms (pregnenolone 400 mg or DHEA 400 mg). We previously showed that peripheral serum allopregnanolone levels were associated with enhanced activation of emotion regulation circuits following pregnenolone administration (Sripada et al., 2013b). DHEA also modulated resting-state amygdala connectivity (Sripada et al., 2014), and DHEA administration-enhanced emotion regulation circuits and modulated memory for emotional stimuli (Sripada et al., 2013a). A causal relationship between neuroactive steroid supplementation and enhanced cortical thickness will be important to establish in future investigations.
We hypothesized that neuroactive steroids may exert stronger influence on cortical thickness for young adults but this influence might be weak in older adults, but this hypothesis was not confirmed except for the relationship of DHEAS level with thickness of the left orbital gyrus, and DHEAS levels with the anterior horizontal portion of the right lateral fissure (Figure 3). Our hypothesis was based on the declining response of the aging brain to a variety of neuromodulators. This age by neuroactive steroid interaction is also relevant to the potential for neuroactive steroids as therapeutic agents in older adults who are particularly affected by deleterious effects of cortical thinning (Morrison and Hof, 1997). The lack of an age by neuroactive steroid interaction on cortical thickness, combined with lower neuroactive steroid levels in older participants, raises the possibility that neuroactive steroids could be attractive therapeutic agents in elderly individuals who experience cortical thinning and associated cognitive decline. This proposition awaits future confirmation that neuroactive steroids effectively stimulate/preserve cortical gray matter.
Limitations
Important caveats and limitations of this study deserve some careful discussion. The current sample of United States military veterans who served after September 11, 2001 has unique health concerns and exposures associated with deployment to Iraq and Afghanistan, which may limit the ability to generalize these results to other populations despite our efforts to statistically control for relevant variables. Confirmation of our findings in a larger independent cohort that is more representative would address this limitation. Although smoking, trauma history, depression, PTSD, and variables other than age did not appear to impact the association between neuroactive steroid levels and cortical thickness, it is possible that a larger sample may be necessary to discern these effects. Because only males participated in the current study, it is unclear whether the current findings generalize to women. We quantified peripheral (serum) neuroactive steroid levels rather than brain neuroactive steroid levels, and the precise relationships between serum and brain neuroactive steroid levels remains to be definitively elucidated. However, evidence to date suggests correlated levels between serum, CSF, and cortical regions (Marx et al., 2006a; Naylor et al., 2008; Kancheva et al., 2011). The significant time lag between obtaining blood for neurosteroid assays and the MRI scan acquisition used for ascertaining cortical thickness is a concern. However, we showed that results were consistent in a subsample who received an MRI scan within 200 days of the blood draw. Furthermore we did not detect a significant association between neuroactive steroid levels and cortical thickness that was modulated by the time elapsed between the blood draw and the MRI scan. While neuroactive steroid levels in the brain may be regionally specific, very few human studies have been conducted to date. Recent clinical and preclinical findings lend credence to an important role for allopregnanolone and pregnenolone relative to other neuroactive steroids in the modulation of brain structure and function. The current study is also cross-sectional, and thus changes in brain morphology (and potential correlations with neuroactive steroids) could not be assessed longitudinally within an individual in this pilot effort. Future studies will be required to understand these regional differences and their neurobiological underpinnings.
Conclusion
Neuroactive steroid levels in the periphery are positively correlated with cortical thickness derived from high-resolution structural MRI in multiple brain regions. Given that neuroactive steroids exhibit pleiotropic actions that include the enhancement of neurogenesis, neuroprotection, neurotrophic properties, and anti-inflammatory effects (among others), there are likely to be multiple mechanisms that contribute to the putative modulatory actions of neuroactive steroids on cortical thickness. If our findings are confirmed in a larger and prospectively designed study, neuroactive steroid quantification and MRI assessment of cortical thickness are potential biomarkers for illness identification, therapeutic response prediction following intervention, and defining biomarker signatures of risk and resilience. Thus, our findings may stimulate new hypotheses and future avenues for investigation in this promising area.
Data Availability Statement
Neurosteroid data is not publicly available since it is protected VA data. For access to neuroimaging data, go to https://openneuro.org/, click public database, and then search for Serum Grey Matter Cortical Thickness.
Ethics Statement
The study has been approved by the Institutional Review Board at Duke University Medical Center and the Durham VA Medical Center. The research has been performed in accordance with the ethical standards as laid down in the 1964 Declaration of Helsinki and its later amendments or comparable ethical standards. Informed consent was obtained from all individual participants included in the study.
Author Contributions
RM: writing the manuscript, data analysis, and final approval of the manuscript. SD and CS: data collection and final approval of the manuscript. CH and DS: data analysis and final approval of the manuscript. JN and SS: study design and final approval of the manuscript. JK: database management and final approval of the manuscript. LS and GP: neurosteroid assays and final approval of the manuscript. HW: biostatistics and final approval of the manuscript. CM: obtaining funding, writing of the manuscript, study design, and final approval of the manuscript.
Funding
This work was supported in part by the U.S. Department of Veterans Affairs, Mid-Atlantic Mental Illness Research, Education and Clinical Center (MIRECC) of the VA Office of Mental Health Services, the Mid-Atlantic Healthcare Network, and the Office of Research and Development (ORD; 5I01CX000748 and 5I01CX000120). Additional financial support was provided by VA Merit Review, RR&D (5I01RX000571; CM) and the National Institute of Neurological Disorders and Stroke (R01NS086885-01A1; RM). JN was supported by a VA Career Development Award (1lK2RX000908) from the Rehabilitation Research and Development (RR&D). SS was supported by a VA Career Development Award (IK2CX001397) from VA Clinical Science Research and Development (CSR&D). The contents of this presentation do not represent the views of the Department of Veterans Affairs or the United States Government.
Conflict of Interest
CM is a co-applicant on pending patent applications focusing on the use of neuroactive steroids and derivatives in CNS disorders. No patents issued. No licensing in place. VA-208 waiver in place.
The remaining authors declare that the research was conducted in the absence of any commercial or financial relationships that could be construed as a potential conflict of interest.
Footnotes
References
Beck, A. T., Steer, R. A., and Brown, G. K. (eds) (1996). Manual for the Beck Depression Inventory-II. San Antonio, TX: Psychological Corp.
Belelli, D., and Lambert, J. J. (2005). Neurosteroids: endogenous regulators of the GABAA receptor. Nat. Rev. Neurosci. 6, 565–575. doi: 10.1038/nrn1703
Benes, F. M., Turtle, M., Khan, Y., and Farol, P. (1994). Myelination of a key relay zone in the hippocampal formation occurs in the human brain during childhood, adolescence, and adulthood. Arch. Gen. Psychiatry 51, 477–484.
Benjamini, Y., and Hochberg, Y. (1995). Controlling the false discovery rate: a practical and powerful approach to multiple testing. J. R. Stat. Soc. B 57, 289–300. doi: 10.1111/j.2517-6161.1995.tb02031.x
Broomall, E., Natale, J. E., Grimason, M., Goldstein, J., Smith, C. M., Chang, C., et al. (2014). Pediatric super-refractory status epilepticus treated with allopregnanolone. Ann. Neurol. 76, 911–915. doi: 10.1002/ana.24295
Brown, E. S., Park, J., Marx, C. E., Hynan, L. S., Gardner, C., Davila, D., et al. (2014). A randomized, double-blind, placebo-controlled trial of pregnenolone for bipolar depression. Neuropsychopharmacology 39, 2867–2873. doi: 10.1038/npp.2014.138
Calhoun, P. S., Sampson, W. S., Bosworth, H. B., Feldman, M. E., Kirby, A. C., Hertzberg, M. A., et al. (2000). Drug use and validity of substance use self-reports in veterans seeking help for posttraumatic stress disorder. J. Consult. Clin. Psychol. 68, 923–927. doi: 10.1037//0022-006x.68.5.923
Carver, C. M., and Reddy, D. S. (2013). Neurosteroid interactions with synaptic and extrasynaptic GABA A receptors: regulation of subunit plasticity, phasic and tonic inhibition, and neuronal network excitability. Psychopharmacology 230, 151–188. doi: 10.1007/s00213-013-3276-5
Charalampopoulos, I., Tsatsanis, C., Dermitzaki, E., Alexaki, V.-I., Castanas, E., Margioris, A. N., et al. (2004). Dehydroepiandrosterone and allopregnanolone protect sympathoadrenal medulla cells against apoptosis via antiapoptotic Bcl-2 proteins. Proc. Natl. Acad. Sci. U.S.A. 101, 8209–8214. doi: 10.1073/pnas.0306631101
Chen, S., Wang, J. M., Irwin, R. W., Yao, J., Liu, L., and Brinton, R. D. (2011). Allopregnanolone promotes regeneration and reduces beta-amyloid burden in a preclinical model of Alzheimer’s disease. PLoS One 6:e24293. doi: 10.1371/journal.pone.0024293
Compagnone, N. A., and Mellon, S. H. (1998). Dehydroepiandrosterone: a potential signalling molecule for neocortical organization during development. Proc. Natl. Acad. Sci. U.S.A. 95, 4678–4683. doi: 10.1073/pnas.95.8.4678
Destrieux, C., Fischl, B., Dale, A., and Halgren, E. (2010). Automatic parcellation of human cortical gyri and sulci using standard anatomical nomenclature. Neuroimage 53, 1–15. doi: 10.1016/j.neuroimage.2010.06.010
Dickerson, B. C., Bakkour, A., Salat, D. H., Feczko, E., Pacheco, J., Greve, D. N., et al. (2009). The cortical signature of Alzheimer’s disease: regionally specific cortical thinning relates to symptom severity in very mild to mild AD dementia and is detectable in asymptomatic amyloid-positive individuals. Cereb. Cortex 19, 497–510. doi: 10.1093/cercor/bhn113
Diedenhofen, B., and Musch, J. (2015). cocor: a comprehensive solution for the statistical comparison of correlations. PLoS One 10:e0121945. doi: 10.1371/journal.pone.0121945
Djebaili, M., Guo, Q., Pettus, E. H., Hoffman, S. W., and Stein, D. G. (2005). The neurosteroids progesterone and allopregnanolone reduce cell death, gliosis, and functional deficits after traumatic brain injury in rats. J. Neurotrauma 22, 106–118. doi: 10.1089/neu.2005.22.106
Dosenbach, N. U., Nardos, B., Cohen, A. L., Fair, D. A., Power, J. D., Church, J. A., et al. (2010). Prediction of individual brain maturity using fMRI. Science 329, 1358–1361. doi: 10.1126/science.1194144
Fernández-Jaén, A., López-Martín, S., Albert, J., Fernández-Mayoralas, D. M., Fernández-Perrone, A. L., Tapia, D. Q., et al. (2014). Cortical thinning of temporal pole and orbitofrontal cortex in medication-naive children and adolescents with ADHD. Psychiatry Res. Neuroimaging 224, 8–13. doi: 10.1016/j.pscychresns.2014.07.004
Fischl, B., van der Kouwe, A., Destrieux, C., Halgren, E., Segonne, F., Salat, D. H., et al. (2004). Automatically parcellating the human cerebral cortex. Cereb. Cortex 14, 11–22. doi: 10.1093/cercor/bhg087
Ghoumari, A., Ibanez, C., El-Etr, M., Leclerc, P., Eychenne, B., O’malley, B., et al. (2003). Progesterone and its metabolites increase myelin basic protein expression in organotypic slice cultures of rat cerebellum. J. Neurochem. 86, 848–859. doi: 10.1046/j.1471-4159.2003.01881.x
Greve, D. N., and Fischl, B. (2017). False positive rates in surface-based anatomical analysis. Neuroimage 171, 6–14. doi: 10.1016/j.neuroimage.2017.12.072
Gursoy, E., Cardounel, A., and Kalimi, M. (2001). Pregnenolone protects mouse hippocampal (HT-22) cells against glutamate and amyloid beta protein toxicity. Neurochem. Res. 26, 15–21.
Hanford, L. C., Nazarov, A., Hall, G. B., and Sassi, R. B. (2016). Cortical thickness in bipolar disorder: a systematic review. Bipolar Disord. 18, 4–18. doi: 10.1111/bdi.12362
He, J., Evans, C.-O., Hoffman, S. W., Oyesiku, N. M., and Stein, D. G. (2004). Progesterone and allopregnanolone reduce inflammatory cytokines after traumatic brain injury. Exp. Neurol. 189, 404–412. doi: 10.1016/j.expneurol.2004.06.008
Heatherton, T. F., Kozlowski, L. T., Frecker, R. C., and Fagerstrom, K. O. (1991). The Fagerström test for nicotine dependence: a revision of the fagerstrom tolerance questionnaire. Addiction 86, 1119–1127. doi: 10.1111/j.1360-0443.1991.tb01879.x
Hyde, K. L., Samson, F., Evans, A. C., and Mottron, L. (2010). Neuroanatomical differences in brain areas implicated in perceptual and other core features of autism revealed by cortical thickness analysis and voxel-based morphometry. Hum. Brain Mapp. 31, 556–566. doi: 10.1002/hbm.20887
Irwin, R. W., Solinsky, C. M., and Brinton, R. D. (2014). Frontiers in therapeutic development of allopregnanolone for Alzheimer’s disease and other neurological disorders. Front. Cell. Neurosci. 8:203. doi: 10.3389/fncel.2014.00203
Kancheva, R., Hill, M., Novak, Z., Chrastina, J., Kancheva, L., and Starka, L. (2011). Neuroactive steroids in periphery and cerebrospinal fluid. Neuroscience 191, 22–27. doi: 10.1016/j.neuroscience.2011.05.054
Kanes, S., Colquhoun, H., Gunduz-Bruce, H., Doherty, J., Jonas, J., Rubinow, D., et al. (2016). SAGE-47 for the treatment of severe postpartum depression. ACNP Conf. Abstr. 41, S116–S288.
Kharitonova, M., Martin, R. E., Gabrieli, J. D., and Sheridan, M. A. (2013). Cortical gray-matter thinning is associated with age-related improvements on executive function tasks. Dev. Cogn. Neurosci. 6, 61–71. doi: 10.1016/j.dcn.2013.07.002
Kimonides, V., Khatibi, N., Svendsen, C., Sofroniew, M., and Herbert, J. (1998). Dehydroepiandrosterone (DHEA) and DHEA-sulfate (DHEAS) protect hippocampal neurons against excitatory amino acid-induced neurotoxicity. Proc. Natl. Acad. Sci. 95, 1852–1857. doi: 10.1073/pnas.95.4.1852
Kubany, E. S., Haynes, S. N., Leisen, M. B., Owens, J. A., Kaplan, A. S., Watson, S. B., et al. (2000). Development and preliminary validation of a brief broad-spectrum measure of trauma exposure: the traumatic life events questionnaire. Psychol. Assess. 12, 210–224. doi: 10.1037//1040-3590.12.2.210
Lambert, J. J., Cooper, M. A., Simmons, R. D., Weir, C. J., and Belelli, D. (2009). Neurosteroids: endogenous allosteric modulators of GABAA receptors. Psychoneuroendocrinology 34, S48–S58.
Lund, M., Foy, D., Sipprelle, C., and Strachan, A. (1984). The combat exposure scale: a systematic assessment of trauma in the vietnam war. J. Clin. Psychol. 40, 1323–1328. doi: 10.1002/1097-4679(198411)40:6<1323::aid-jclp2270400607>3.0.co;2-i
Marx, C. E., Bradford, D. W., Hamer, R. M., Naylor, J. C., Allen, T. B., Lieberman, J. A., et al. (2011). Pregnenolone as a novel therapeutic candidate in schizophrenia: emerging preclinical and clinical evidence. Neuroscience 191, 78–90. doi: 10.1016/j.neuroscience.2011.06.076
Marx, C. E., Keefe, R. S., Buchanan, R. W., Hamer, R. M., Kilts, J. D., Bradford, D. W., et al. (2009). Proof-of-concept trial with the neurosteroid pregnenolone targeting cognitive and negative symptoms in schizophrenia. Neuropsychopharmacology 34, 1885–1903. doi: 10.1038/npp.2009.26
Marx, C. E., Lee, J., Subramaniam, M., Rapisarda, A., Bautista, D. C., Chan, E., et al. (2014). Proof-of-concept randomized controlled trial of pregnenolone in schizophrenia. Psychopharmacology 231, 3647–3662. doi: 10.1007/s00213-014-3673-4
Marx, C. E., Naylor, J. C., Kilts, J. D., Dunn, C. E., Tupler, L. A., Szabo, S. T., et al. (2016). “Neurosteroids and traumatic brain injury: translating biomarkers to therapeutics; overview and pilot investigations in Iraq and Afghanistan era veterans,” in Translational Research in Traumatic Brain Injury, eds D. Laskowitz, and G. Grant, (Boca Raton, FL: CRC Press/Taylor and Francis Group).
Marx, C. E., Shampine, L. J., Duncan, G. E., VanDoren, M. J., Grobin, A. C., Massing, M. W., et al. (2006a). Clozapine markedly elevates pregnenolone in rat hippocampus, cerebral cortex, and serum: candidate mechanism for superior efficacy? Pharmacol. Biochem. Behav. 84, 598–608. doi: 10.1016/j.pbb.2006.07.026
Marx, C. E., Trost, W. T., Shampine, L. J., Stevens, R. D., Hulette, C. M., Steffens, D. C., et al. (2006b). The neurosteroid allopregnanolone is reduced in prefrontal cortex in Alzheimer’s disease. Biol. Psychiatry 60, 1287–1294. doi: 10.1016/j.biopsych.2006.06.017
Marx, C. E., VanDoren, M. J., Duncan, G. E., Lieberman, J. A., and Morrow, A. L. (2003). Olanzapine and clozapine increase the GABAergic neuroactive steroid allopregnanolone in rodents. Neuropsychopharmacology 28, 1–13. doi: 10.1038/sj.npp.1300015
Mayo, W., Lemaire, V., Malaterre, J., Rodriguez, J., Cayre, M., Stewart, M., et al. (2005). Pregnenolone sulfate enhances neurogenesis and PSA-NCAM in young and aged hippocampus. Neurobiol. Aging 26, 103–114. doi: 10.1016/j.neurobiolaging.2004.03.013
Morrison, J. H., and Hof, P. R. (1997). Life and death of neurons in the aging brain. Science 278, 412–419. doi: 10.1126/science.278.5337.412
Morrow, A. L., Pace, J. R., Purdy, R. H., and Paul, S. M. (1990). Characterization of steroid interactions with gamma-aminobutyric acid receptor-gated chloride ion channels: evidence for multiple steroid recognition sites. Mol. Pharmacol. 37, 263–270.
Morrow, A. L., Suzdak, P. D., and Paul, S. M. (1987). Steroid hormone metabolites potentiate GABA receptor-mediated chloride ion flux with nanomolar potency. Eur. J. Pharmacol. 142, 483–485. doi: 10.1016/0014-2999(87)90094-x
Mutlu, A. K., Schneider, M., Debbané, M., Badoud, D., Eliez, S., and Schaer, M. (2013). Sex differences in thickness, and folding developments throughout the cortex. Neuroimage 82, 200–207. doi: 10.1016/j.neuroimage.2013.05.076
Narr, K. L., Bilder, R. M., Toga, A. W., Woods, R. P., Rex, D. E., Szeszko, P. R., et al. (2005). Mapping cortical thickness and gray matter concentration in first episode schizophrenia. Cereb. Cortex 15, 708–719. doi: 10.1093/cercor/bhh172
Naylor, J. C., Hulette, C. M., Steffens, D. C., Shampine, L. J., Ervin, J. F., Payne, V. M., et al. (2008). Cerebrospinal fluid dehydroepiandrosterone levels are correlated with brain dehydroepiandrosterone levels, elevated in Alzheimer’s disease, and related to neuropathological disease stage. J. Clin. Endocrinol. Metab. 93, 3173–3178. doi: 10.1210/jc.2007-1229
Naylor, J. C., Kilts, J. D., Hulette, C. M., Steffens, D. C., Blazer, D. G., Ervin, J. F., et al. (2010). Allopregnanolone levels are reduced in temporal cortex in patients with Alzheimer’s disease compared to cognitively intact control subjects. Biochim. Biophys. Acta 1801, 951–959. doi: 10.1016/j.bbalip.2010.05.006
Nguyen, T. V., McCracken, J. T., Ducharme, S., Cropp, B. F., Botteron, K. N., Evans, A. C., et al. (2013). Interactive effects of dehydroepiandrosterone and testosterone on cortical thickness during early brain development. J. Neurosci. 33, 10840–10848. doi: 10.1523/JNEUROSCI.5747-12.2013
O’donnell, S., Noseworthy, M. D., Levine, B., and Dennis, M. (2005). Cortical thickness of the frontopolar area in typically developing children and adolescents. Neuroimage 24, 948–954. doi: 10.1016/j.neuroimage.2004.10.014
Osuji, I. J., Vera-Bolaños, E., Carmody, T. J., and Brown, E. S. (2010). Pregnenolone for cognition and mood in dual diagnosis patients. Psychiatry Res. 178, 309–312. doi: 10.1016/j.psychres.2009.09.006
Panizzon, M. S., Fennema-Notestine, C., Eyler, L. T., Jernigan, T. L., Prom-Wormley, E., Neale, M., et al. (2009). Distinct genetic influences on cortical surface area and cortical thickness. Cereb. Cortex 19, 2728–2735. doi: 10.1093/cercor/bhp026
Perneger, T. V. (1998). What’s wrong with Bonferroni adjustments. Br. Med. J. 316, 1236–1238. doi: 10.1136/bmj.316.7139.1236
Plitman, E., Patel, R., Chung, J. K., Pipitone, J., Chavez, S., Reyes-Madrigal, F., et al. (2016). Glutamatergic metabolites, volume and cortical thickness in antipsychotic-naive patients with first-episode psychosis: implications for excitotoxicity. Neuropsychopharmacology 41, 2606–2613. doi: 10.1038/npp.2016.84
Qian, X., Cao, H., Ma, Q., Wang, Q., He, W., Qin, P., et al. (2015). Allopregnanolone attenuates Aβ25-35-induced neurotoxicity in PC12 cells by reducing oxidative stress. Int. J. Clin. Exp. Med. 8, 13610–13615.
Rasmusson, A. M., Marx, C. E., Pineles, S. L., Locci, A., Scioli-Salter, E. R., Nillni, Y. I., et al. (2017). Neuroactive steroids and PTSD treatment. Neurosci. Lett. 649, 156–163. doi: 10.1016/j.neulet.2017.01.054
Rasmusson, A. M., Pinna, G., Paliwal, P., Weisman, D., Gottschalk, C., Charney, D., et al. (2006). Decreased cerebrospinal fluid allopregnanolone levels in women with posttraumatic stress disorder. Biol. Psychiatry 60, 704–713. doi: 10.1016/j.biopsych.2006.03.026
Reddy, D. S. (2018). GABA-A receptors mediate tonic inhibition and neurosteroid sensitivity in the brain. Vitam. Horm. 107, 177–191. doi: 10.1016/bs.vh.2017.12.001
Reddy, D. S., and Kuruba, R. (2013). Experimental models of status epilepticus and neuronal injury for evaluation of therapeutic interventions. Int. J. Mol. Sci. 14, 18284–18318. doi: 10.3390/ijms140918284
Reiss, P. T., Schwartzman, A., Lu, F., Huang, L., and Proal, E. (2012). Paradoxical results of adaptive false discovery rate procedures in neuroimaging studies. Neuroimage 63, 1833–1840. doi: 10.1016/j.neuroimage.2012.07.040
Rimol, L. M., Hartberg, C. B., Nesvåg, R., Fennema-Notestine, C., Hagler, D. J. Jr., Pung, C. J., et al. (2010). Cortical thickness and subcortical volumes in schizophrenia and bipolar disorder. Biol. Psychiatry 68, 41–50. doi: 10.1016/j.biopsych.2010.03.036
Saunders, J. B., Aasland, O. G., Babor, T. F., de la Fuente, J. R., and Grant, M. (1993). Development of the Alcohol Use Disorders Identification Test (AUDIT): WHO collaborative project on early detection of persons with harmful alcohol consumption–II. Addiction 88, 791–804. doi: 10.1111/j.1360-0443.1993.tb02093.x
Sayeed, I., Guo, Q., Hoffman, S. W., and Stein, D. G. (2006). Allopregnanolone, a progesterone metabolite, is more effective than progesterone in reducing cortical infarct volume after transient middle cerebral artery occlusion. Ann. Emerg. Med. 47, 381–389. doi: 10.1016/j.annemergmed.2005.12.011
Schumacher, M., Weill-Engerer, S., Liere, P., Robert, F., Franklin, R., Garcia-Segura, L. M., et al. (2003). Steroid hormones and neurosteroids in normal and pathological aging of the nervous system. Prog. Neurobiol. 71, 3–29. doi: 10.1016/j.pneurobio.2003.09.004
Shaw, P., Greenstein, D., Lerch, J., Clasen, L., Lenroot, R., Gogtay, N., et al. (2006). Intellectual ability and cortical development in children and adolescents. Nature 440, 676–679. doi: 10.1038/nature04513
Singh, C., Liu, L., Wang, J. M., Irwin, R. W., Yao, J., Chen, S., et al. (2012). Allopregnanolone restores hippocampal-dependent learning and memory and neural progenitor survival in aging 3xTgAD and nonTg mice. Neurobiol. Aging 33, 1493–1506. doi: 10.1016/j.neurobiolaging.2011.06.008
Sowell, E. R., Delis, D., Stiles, J., and Jernigan, T. L. (2001). Improved memory functioning and frontal lobe maturation between childhood and adolescence: a structural MRI study. J. Int. Neuropsychol. Soc. 7, 312–322. doi: 10.1017/s135561770173305x
Sowell, E. R., Thompson, P. M., Leonard, C. M., Welcome, S. E., Kan, E., and Toga, A. W. (2004). Longitudinal mapping of cortical thickness and brain growth in normal children. J. Neurosci. 24, 8223–8231. doi: 10.1523/jneurosci.1798-04.2004
Sripada, R. K., Marx, C. E., King, A. P., Rajaram, N., Garfinkel, S. N., Abelson, J. L., et al. (2013a). DHEA enhances emotion regulation neurocircuits and modulates memory for emotional stimuli. Neuropsychopharmacology 38, 1798–1807. doi: 10.1038/npp.2013.79
Sripada, R. K., Marx, C. E., King, A. P., Rampton, J. C., Ho, S. S., and Liberzon, I. (2013b). Allopregnanolone elevations following pregnenolone administration are associated with enhanced activation of emotion regulation neurocircuits. Biol. Psychiatry 73, 1045–1053. doi: 10.1016/j.biopsych.2012.12.008
Sripada, R. K., Welsh, R. C., Marx, C. E., and Liberzon, I. (2014). The neurosteroids allopregnanolone and dehydroepiandrosterone modulate resting-state amygdala connectivity. Hum. Brain Mapp. 35, 3249–3261. doi: 10.1002/hbm.22399
Storey, J. D., Taylor, J. E., and Siegmund, D. (2004). Strong control, conservative point estimation and simultaneous conservative consistency of false discovery rates: a unified approach. J. R. Stat. Soc. 66, 187–205. doi: 10.1111/j.1467-9868.2004.00439.x
Suzuki, M., Wright, L. S., Marwah, P., Lardy, H. A., and Svendsen, C. N. (2004). Mitotic and neurogenic effects of dehydroepiandrosterone (DHEA) on human neural stem cell cultures derived from the fetal cortex. Proc. Natl. Acad. Sci. U.S.A. 101, 3202–3207. doi: 10.1073/pnas.0307325101
Uzunova, V., Sheline, Y., Davis, J., Rasmusson, A., Uzunov, D., Costa, E., et al. (1998). Increase in the cerebrospinal fluid content of neurosteroids in patients with unipolar major depression who are receiving fluoxetine or fluvoxamine. Proc. Natl. Acad. Sci. U.S.A. 95, 3239–3244. doi: 10.1073/pnas.95.6.3239
Vallée, M. (2016). Neurosteroids and potential therapeutics: focus on pregnenolone. J. Steroid Biochem. Mol. Biol. 160, 78–87. doi: 10.1016/j.jsbmb.2015.09.030
van Erp, T. G., Hibar, D. P., Rasmussen, J. M., Glahn, D. C., Pearlson, G. D., Andreassen, O. A., et al. (2016). Subcortical brain volume abnormalities in 2028 individuals with schizophrenia and 2540 healthy controls via the ENIGMA consortium. Mol. Psychiatry 21, 547–553. doi: 10.1038/mp.2015.63
Wang, J. M., Singh, C., Liu, L., Irwin, R. W., Chen, S., Chung, E. J., et al. (2010). Allopregnanolone reverses neurogenic and cognitive deficits in mouse model of Alzheimer’s disease. Proc. Natl. Acad. Sci. U.S.A. 107, 6498–6503. doi: 10.1073/pnas.1001422107
Wang, M.-D., Wahlström, G., and Bäckström, T. (1997). The regional brain distribution of the neurosteroids pregnenolone and pregnenolone sulfate following intravenous infusion. J. Steroid Biochem. Mol. Biol. 62, 299–306. doi: 10.1016/s0960-0760(97)00041-1
Watsky, R. E., Ludovici Pollard, K., Greenstein, D., Shora, L., Dillard-Broadnax, D., Gochman, P., et al. (2016). Severity of cortical thinning correlates with schizophrenia spectrum symptoms. J. Am. Acad. Child Adolesc. Psychiatry 55, 130–136. doi: 10.1016/j.jaac.2015.11.008
Xu, B., Yang, R., Chang, F., Chen, L., Xie, G., Sokabe, M., et al. (2012). Neurosteroid PREGS protects neurite growth and survival of newborn neurons in the hippocampal dentate gyrus of APPswe/PS1dE9 mice. Curr. Alzheimer Res. 9, 361–372. doi: 10.2174/156720512800107591
Youssef, N. A., Bradford, D. W., Kilts, J. D., Szabo, S. T., Naylor, J. C., Allen, T. B., et al. (2015). Exploratory investigation of biomarker candidates for suicide in schizophrenia and bipolar disorder. Crisis 36, 46–54. doi: 10.1027/0227-5910/a000280
Keywords: neuroactive steroids, cortical thickness, MRI, neuroregeneration, neuroprotection, gray matter
Citation: Morey RA, Davis SL, Haswell CC, Naylor JC, Kilts JD, Szabo ST, Shampine LJ, Parke GJ, Sun D, Swanson CA, Wagner HR, Mid-Atlantic MIRECC Workgroup and Marx CE (2019) Widespread Cortical Thickness Is Associated With Neuroactive Steroid Levels. Front. Neurosci. 13:1118. doi: 10.3389/fnins.2019.01118
Received: 13 June 2019; Accepted: 03 October 2019;
Published: 12 November 2019.
Edited by:
A. Leslie Morrow, The University of North Carolina at Chapel Hill, United StatesReviewed by:
Michael Schumacher, Institut National de la Santé et de la Recherche Médicale (INSERM), FranceWilliam Davies, Cardiff University, United Kingdom
Copyright © 2019 Morey, Davis, Haswell, Naylor, Kilts, Szabo, Shampine, Parke, Sun, Swanson, Wagner, Mid-Atlantic MIRECC Workgroup and Marx. This is an open-access article distributed under the terms of the Creative Commons Attribution License (CC BY). The use, distribution or reproduction in other forums is permitted, provided the original author(s) and the copyright owner(s) are credited and that the original publication in this journal is cited, in accordance with accepted academic practice. No use, distribution or reproduction is permitted which does not comply with these terms.
*Correspondence: Rajendra A. Morey, cmFqZW5kcmEubW9yZXlAZHVrZS5lZHU=; bW9yZXlAYmlhYy5kdWtlLmVkdQ==