- 1Department of Psychology, Franciscan University of Steubenville, Steubenville, OH, United States
- 2School of Medicine, Universidad San Sebastián, Conceptión, Chile
Given the significant physiological changes that take place during and resulting from pregnancy, as well as the relative absence of such information in relation to pregnancy termination, this study investigated the potential for developing a valid animal model to objectively assess the biological, physiological and behavioral consequences of drug-induced pregnancy termination. Female Long-Evans rats were divided into four groups (n = 19–21/group), controlling for drug [mifepristone (50 mg/kg/3 ml, i.g.)/misoprostol (0.3 mg/kg/ml, i.g.) or vehicle (1% Carboxymethylcellulose Sodium/0.2% Tween® 80 suspension, i.g.)] and pregnancy. Drug administration took place on days 12–14 of gestation (days 28–40 human gestational equivalent). Vehicle was administered to the controls on the same days. Parameters measured included rat body weight, food intake, vaginal impedance, sucrose consumption/preference, locomotor activity, forced swim test, and home-cage activity. At the termination of the study, rats were deeply anesthetized using urethane, and blood, brain, and liver were collected for biochemical analysis. Following drug/vehicle administration, only the pregnancy termination group (pregnant, drug) displayed a significant decrease in body weight, food intake, locomotor activity-related behaviors and home-cage activity relative to the control group (non-pregnant, vehicle). Additionally, the pregnancy termination group was the only group that displayed a significant reduction in sucrose consumption/preference during Treatment Week relative to Pre-Treatment Week. Vaginal impedance did not significantly decrease over time in parous rats in contrast to all other groups, including the rats in the pregnancy termination group. Biochemical analysis indicated putative drug- and pregnancy-specific influences on oxidative balance. Regression analysis indicated that pregnancy termination was a predictor variable for body weight, food intake and all locomotor activity parameters measured. Moreover, pertaining to body weight and food intake, the pregnancy termination group displayed significant changes, which were not present in a group of naturally miscarrying rats following pregnancy loss. Overall, our results appear to suggest negative biological and behavioral effects following pregnancy termination, that appear to also be distinct from natural miscarriage, and potential benefits of parity pertaining to fecundity. Thus, our findings indicate the importance for further objective investigation of the physiological and behavioral consequences of medical abortion, in order to provide further insight into the potential implications in humans.
Introduction
The potential for abortion-related consequences on mental health has been extensively debated in the scientific literature for more than 20 years, with little clear resolution (Tsoi et al., 1976; Gibbons, 1984; Adler et al., 1992; Thorp et al., 2005; Major et al., 2009; Coleman, 2011; Reardon, 2018). These consequences include an increased risk of mood disorders (including depression), anxiety (Cougle et al., 2003; Fergusson et al., 2006; Pedersen, 2008), substance abuse (Reardon et al., 2004a; Fergusson et al., 2006, 2008; Dingle et al., 2008) and suicide (Reardon et al., 2004b; Thorp et al., 2005; Fergusson et al., 2006; Luo et al., 2018). Given that approximately 20% of pregnancies in the United States end in abortion (Jones and Kooistra, 2011; Finer and Zolna, 2016), the seriousness of the potential mental health consequences, and the clinical difficulties associated with their treatment and/or prevention (Crosby et al., 2011; Lau and Rapee, 2011; Kupfer et al., 2012), it becomes necessary to objectively investigate any potential links between abortion and specific negative consequences that may arise from the procedure.
A number of recent studies have sought to investigate the comorbid relationship between abortion and psychopathological behaviors (e.g., depression, anxiety etc.) with reports of positive (Reardon and Cougle, 2002; Cougle et al., 2003; Reardon et al., 2004a; Thorp et al., 2005; Dingle et al., 2008; Fergusson et al., 2008; Pedersen, 2008; Mota et al., 2010; Coleman, 2011; Huang et al., 2012; Luo et al., 2018), negative (Foster et al., 2015; Biggs et al., 2016b) or no relationship (Rees and Sabia, 2007; American Psychological Association, 2008; Robinson et al., 2009; Warren et al., 2010; Munk-Olsen et al., 2011; Biggs et al., 2015, 2016a; Foster et al., 2015; van Ditzhuijzen et al., 2018). These conflicting findings are likely to arise due to the retrospective nature of most studies, differences in the follow-up period of the subjects involved in the study after the abortion procedure took place (Coleman, 2011; Coleman et al., 2012; Reardon, 2014; Biggs et al., 2016a) or the perspective of interpretation of those utilizing the data (Reardon, 2018). Irrespective of the reason, further investigation is warranted to address these issues.
As the process of pregnancy termination (abortion) in both the early and late stages of pregnancy involves a significant disturbance (Hamoda and Templeton, 2010; Lee et al., 2010) to the complex normal physical course of events associated with a normal physical state (pregnancy) (Hill and Pickinpaugh, 2008), it is reasonable to also expect physiological consequences, including an impact on reproduction (Lv et al., 2012). Among the normal changes that take place during pregnancy are those to the hypothalamic-pituitary-adrenal (HPA) axis (Lindsay and Nieman, 2005; Duthie and Reynolds, 2013), which is crucial for the control of various hormones, including glucocorticoids, and has been implicated in depression (Pariante and Lightman, 2008; Belvederi Murri et al., 2014; Juruena, 2014; Ancelin et al., 2017). Therefore, interrupting the normal course of changes in the HPA axis makes it plausible that pregnancy termination may result in physiological alterations leading to changes in mental health, irrespective of whether the abortion is medical or surgical. Relative to surgical abortion, previous work in rats has reported reduced protection from the influence of carcinogens in rats that have their fetuses removed via hysterectomy at mid-pregnancy (day 12) relative to those allowed to deliver and nurse their litters (Russo and Russo, 1980; Sinha et al., 1988; Yang et al., 1999; Rajkumar et al., 2001; Russo et al., 2005). This work appears to support human data reflecting a link between abortion and breast cancer development (Yuasa and MacMahon, 1970; Lanfranchi, 2005, 2014; Russo et al., 2005; Naieni et al., 2007; Xing et al., 2010; Huang et al., 2013; Lanfranchi and Fagan, 2014a,b).
In the case of medical abortion, consideration needs to be given to the pharmacological effects of mifepristone (RU486), in addition to any procedural consequences. Mifepristone is utilized to induce cervical ripening (Radestad et al., 1990), along with misoprostol or gemeprost (agonists at prostaglandin E1 receptor), which induce uterine contractions (Wing et al., 1995). Mifepristone produces its actions both by modulating progesterone action (as a progesterone receptor antagonist or partial agonist) and blocking the glucocorticoid receptors (GR) (Spitz and Bardin, 1993). It has also been reported to increase cytokine expression, which is thought to be a contributing factor to the mechanism of action of mifepristone in the termination of pregnancy (Li et al., 2004). Furthermore, increased levels of inflammatory cytokines have been reported under stressful conditions and may be a factor in triggering depression (Nguyen et al., 1998; Ishikawa et al., 2001; Steptoe et al., 2007; Shapira-Lichter et al., 2008; Kumar et al., 2011). Moreover, as a result of its actions on the GR, mifepristone has been reported to potentiate cytokine-induced depression-like behavior in rats, as measured by various parameters, including sweet-solution (saccharin) intake and locomotor behavior (Wang et al., 2011).
At the biochemical level, lowered levels of antioxidants (e.g., glutathione) and increased oxidative stress in the blood, peripheral tissue and the brain have been consistently reported in animal models of depression (Maes et al., 2011). An imbalance in oxidative stress has also been reported as a result of depression in humans (Liu et al., 2015). Redox alterations brought about by diverse insults are reflected by changes in the redox potential of the redox pair glutathione (GSH) and oxidized glutathione (GSSG) (Schafer and Buettner, 2001; Alzoubi et al., 2012; Schiavone et al., 2013), as increased levels of reactive oxygen species (ROS) can lead to an increase in the production of GSSG (Lushchak, 2012). An increase of lipid peroxidation end products, such as malondialdehyde (MDA), has also been observed as a sign of redox imbalance (Halliwell and Chirico, 1993). Given the potential for physiological consequences of pregnancy termination, it would be reasonable to expect alterations in the GSH/GSSG balance, toward a more oxidizing potential, as well as an increase in lipid peroxidation end products.
Collectively, the evidence appears to indicate the potential for physiological and biochemical changes arising from the termination of pregnancy, which may cause significant behavioral and psychological changes that could ultimately lead to various mental health consequences. The challenging nature of prospective studies on this subject in humans demands for a more objective methodology for measuring the physiological/biochemical and behavioral changes associated with the procedure of abortion. This would aid in providing some clarity into any potential causal links between the procedure and the development of psychological disorders. Thus, we proposed that the examination of pregnancy termination in an animal model, as a means to further our understanding of the physiological and behavioral consequences of abortion in a more controlled setting, with less unknown variables, would potentially provide clearer insight into the repercussions of the abortion procedure in humans.
Our work sought to create a valid animal model in order to examine the impact of treatments known to induce the pharmacological termination of pregnancy relative to sham treatments in rats. Given that medical abortions in humans are performed up to the first 10 weeks of pregnancy, the pregnancy termination for this study was performed at mid-term (days 12–14) of the rat’s gestational period (∼23 days). This compares to days 28–40 (4–6 weeks) in human embryonic development (Witschi, 1962; O’Rahilly, 1979; Hill, 2019). Our study measured behaviors characteristic of symptoms associated with depression, including, but not limited to, sucrose consumption/preference (as a measure of anhedonia; Papp et al., 1991; Willner, 1991), spontaneous locomotor activity (as a measure of psychomotor activity; Willner, 1991), immobility in the forced swim test (as a measure of behavioral despair; Porsolt et al., 1977; Castagné et al., 2010; Slattery and Cryan, 2012), as well as oxidative stress (as a biochemical outcome associated with behavioral alterations; Schafer and Buettner, 2001; Maes et al., 2011; Alzoubi et al., 2012; Schiavone et al., 2013; Liu et al., 2015).
Materials and Methods
Drugs
Mifepristone and misoprostol (neat oil) were purchased from Cayman Chemical (Ann Arbor, MI, United States). Urethane, Tween® 80, 5,5′-dithiobis(2-nitrobenzoic acid), 2-thiobarbituric acid (TBA), L-glutathione reduced (GSH) and glutathione reductase from baker’s yeast were purchased from Sigma-Aldrich (St. Louis, MO, United States). Carboxymethylcellulose sodium (CMC-Na), 2-vinylpyridine, NADPH, 1-chloro-2,4-dinitrobenzene and trichloroacetic acid (TCA) were purchased from VWR (Philadelphia, PA, United States). All other chemicals were of the highest grade commercially available.
Subjects
Female Long-Evans rats (n = 81) were carefully bred with male rats of the same species and raised in-house, avoiding any inbreeding. The original breeder pairs were purchased from Hilltop Lab Animals (Scottdale, PA, United States). All animal protocols were approved by the Franciscan University Institutional Animal Care and Use Committee (Protocol Number: 2013-01) and adhere to the Guide for the Care and Use of Laboratory Animals published by the USPHS. Animals were housed on Aspen shavings (Nepco®), were single-housed (beginning 9 weeks of age) and positioned in such a way that they could see, hear and smell other animals of the same species, under a 12/12 h light-dark cycle (Lights on: 2.15 a.m. or 3.15 a.m.) and controlled temperature and humidity (20–26°C, 30–70% relative humidity), with ad libitum access to standard laboratory chow (RMH 1800, LabDiet) and water, aside from the 1 h duration of the experiment. Animal behaviors were monitored daily as an indicator of their health and well-being (NIH, 2016).
Experimental Procedure
Following single-housing, rats were acclimated to training tips on their water bottles in the home-cage, similar to those used during the experimental procedure itself. The rats continued with access to these tips in their home-cages until the end of the experiment. Five weeks prior to breeding, rat weight and impedance measurements were initiated. While rat weight was measured throughout the entire length of the experiment, impedance, measured using a Vaginal-Estrous Cycle-Monitor (MK-11, Stoelting, Wood Dale, IL, United States), was only collected until the rats were bred or assigned to a non-breeding control group. Food weight was measured beginning the week before breeding and continued through the end of the experiment. Sucrose consumption/preference and locomotor testing commenced 3 weeks prior to breeding (14 weeks of age). Following this acclimation period, the rats were assigned to an experimental group and bred accordingly (17 weeks of age). Drug or vehicle was administered at mid-term of gestation (Treatment Week; see below under “Chemical termination, experimental groups and drug administration”). A forced swim test (FST) was carried out 3 days post-partum (D3PP; Day 17 (D17) of gestation for rats undergoing pregnancy termination and their controls, or 3 days post-birth for rats giving natural birth and their controls). Home-cage activity was monitored on D11 of gestation (day prior to drug/vehicle administration), as well as D3PP. Sucrose consumption/preference and locomotor parameters continued to be measured for 12 weeks post-breeding. Impedance was also re-measured for 2 weeks (11 and 12 weeks post-breeding and equivalent days for control groups), immediately prior to tissue collection. For tissue collection, rats were deeply anesthetized using urethane (1.5–2 g/kg/ml, i.p.) (Field et al., 1993) and decapitated, and blood, liver and brain were collected for further analysis (see Supplementary Figure 1).
Breeding
Vaginal impedance was measured daily (∼3.5 h prior to the start of the dark cycle), as described under “Experimental procedure” to determine estrus (Singletary et al., 2005). A peak in impedance was considered as an indication of estrus and was not present in pregnant rats (Bartos, 1977; Taradach, 1982; Singletary et al., 2005). Rats not showing consistent cycles (n = 13), as measured by vaginal impedance, were removed from the study prior to breeding and never underwent treatment. Female rats (17 weeks of age), whose impedance indicated estrus, were paired with a fertile male at the beginning of the dark cycle of the day of estrus (Yang et al., 2000). The male was removed after 8 h and the female was checked for signs of mating. The presence of a vaginal plug and daily weight gain were considered as presumptive evidence of pregnancy. Day 1 (D1) of pregnancy was considered to be the day following breeding.
Chemical Termination, Experimental Groups, and Drug Administration
Methodology pertaining to pharmacological pregnancy termination, drug dosing, preparation, and administration was adapted from He et al. (2003) and Cabrol et al. (1991). Dosage for mifepristone and misoprostol was based on a combination of resources (He et al., 2003; Hu et al., 2012; Maddalena et al., 2013), as well as taking into consideration the doses utilized in human abortions (Fiala and Danielsson, 2006) and applying the formula for dose translation based on the Body Surface Area (BSA), as described by Reagan-Shaw et al. (2008) in order to calculate the rat equivalent dose.
In order to qualify as a successful pregnancy termination, it was necessary for blood to be evident vaginally and on cage bedding, and no further weight gain observed. Moreover, in order to avoid any potential ambiguity in interpretation of results, unsuccessful pregnancy termination procedures [e.g., problem with intragastric (i.g.) administration, n = 1] and natural pregnancy termination (miscarriage, n = 0) during the course of the pregnancy were considered as exclusion criteria for the study. The rats described under “Results,” pertaining to the miscarriage observations, were not bred as part of the original study.
Rats were assigned to one of four groups. The pregnant, drug group (D+P+) was administered mifepristone (50.0 mg/kg/3 ml, i.g.) on days 12, 13, and 14 of pregnancy, while misoprostol (0.3 mg/kg/ml, i.g.) was administered on day 14 of pregnancy, 2 h after the final administration of mifepristone (He et al., 2003). Drugs were administered in a 0.5–1 ml volume of a CMC-Na (1%) and Tween® 80 (0.2%) suspension. The same mifepristone and misoprostol protocol was also administered to non-pregnant, age-matched female rats (D+P-: non-pregnant, drug group). Pregnant, vehicle (D-P+) and non-pregnant, vehicle (D-P-) control groups were administered vehicle (1% CMC-Na/0.2% Tween® 80 suspension) on the same days (D12–14) as the D+P+ and D+P- groups.
Behavioral Testing
The behavioral studies described below were performed on all rat groups.
Forced Swim Test (FST) and Home-Cage Activity
The forced swim test has been extensively utilized as a measure of behavioral despair and the efficacy of antidepressants (Porsolt et al., 1978a,b; Cryan et al., 2002; Slattery and Cryan, 2012). Non-depressed rats placed in a cylinder containing water display swimming and climbing activity, while reduced mobility reflects behavioral despair (Porsolt et al., 1978b). The experiment was conducted in a plexiglass cylinder (20 cm in diameter, made in-house) filled with water to a depth of 30 cm with sufficient space from the top of the cylinder to prevent the rats from escaping during the test. Water temperature was set at 23–25°C. Rats were placed in the water-cylinder for a 5 min test session on D3PP and swimming behavior was recorded using video cameras. The forced swim test was conducted during the light phase of the light/dark cycle (Castagné et al., 2010). Forced swim test videos were processed using Smart® software (Panlab Harvard Apparatus) assessing passive behavior: immobility (when a rat remained floating in the water, making only the necessary movements to keep its head above water) (Cervo et al., 1988); and active behaviors: swimming (when rats made active swimming motions) and climbing (when rats made vigorous movements with its forepaws in and out of the water, usually directed against walls) (Detke and Lucki, 1995; Slattery and Cryan, 2012).
For home-cage activity recordings, rats were recorded using cameras, in their home-cages, for the first 5 min of the dark cycle under red LED lighting. Recordings were conducted on D11 (Pre-Treatment) and D3PP (Post-Treatment). Home-cage activity videos were also processed using Smart® software, measuring periods of immobility.
Sucrose Consumption/Preference
As rats are more active during the dark period, both sucrose consumption/preference and locomotor tests were conducted in the first hour of the dark cycle (Laakso et al., 1995; Navarre et al., 2010), twice a week, on the same days each week.
Consumption/preference of a sweet palatable solution, such as sucrose or saccharin, has been utilized as a measure of changes in the sensitivity of the reward system, specifically, a reduction in consumption/preference being reflective of anhedonia (Katz, 1981; Papp et al., 1991; Grønli et al., 2005; Wang et al., 2009; Hayase, 2011).
The testing involved a two-bottle choice test containing plain water or 2% sucrose solution. Sucrose consumption/preference was measured for 1 h, using the Drinking/Feeding module of the PhenoMaster (TSE Systems, Chesterfield, MO, United States). Following the hour of sucrose access, the rats were returned to their home cages. Rats not consuming greater than 1.0 g of sucrose solution prior to breeding were eliminated from the study, as a lack of consumption could mask any potential treatment (drug, pregnancy) effect.
Locomotor Activity
Altered locomotor activity has also been utilized as a measure of depression (Willner, 1991; Grønli et al., 2005; Kumar et al., 2011; Wang et al., 2011; Zhu et al., 2011). Recordings were carried out concurrently with the sucrose consumption/preference testing and were measured using the InfraMot module of the PhenoMaster (TSE Systems, Chesterfield, MO, United States).
Both sucrose consumption/preference and locomotor activity data were processed using the TSE PhenoMaster V5.0.1 program (TSE Systems, Chesterfield, MO, United States). The parameters analyzed were comprised of activity time/time active, defined as the time during which the rat moved above the threshold (TSE Systems, Chesterfield, MO, United States) of 6cm/s (determined from previous tests in laboratory); distance active, defined as the distance moved during activity time; overall speed, defined as distance in activity time over total time (TSE Systems, Chesterfield, MO, United States), as well as rearings, defined as standing on hind legs for a duration >3 s (Horowitz et al., 1997).
Further analysis was conducted pertaining to activity in the corners of the cage. Corners were defined as 12 light beams in the x-axis by 6 light beams in the y-axis per corner. The corners were labeled as NW, NE, SW, SE, with the front of the cage (furthest from the wall) being the two east corners. Given the presence of the bottles in the NW corner and that on average, the rats spent less than 5% (relative to total testing time and total time in corners) of their testing time in this corner, the analyses only take into consideration the NE, SW, and SE corners. Time spent in each corner as a percentage of the total testing time was calculated for all groups.
Tissue Collection
At the end of the experiment, the rats were deeply anesthetized using urethane (1.5–2 g/kg/ml, i.p.) (Field et al., 1993) and decapitated. Prior to the decapitation, blood samples were collected from the portal vein into a blood collection vial (VWR, BD367983). Blood-containing vials were then centrifuged in a clinical centrifuge (Clinaspin, VUL-6C) at 3000 rpm for 30 min and serum was aliquoted into 0.5–1 ml samples. Immediately following euthanasia, livers were dissected and perfused with NaCl (0.9% W/V) to remove all blood, and brains were dissected. Serum and tissues were frozen at -80°C until further analysis.
Measures of Oxidative Stress
Preparation of Serum Samples
Serum samples were thawed on ice and aliquoted for measurement of the total glutathione pool (reduced plus oxidized, GSH+GSSG), GSSG, thiobarbituric acid substances (TBARS) and glutathione S-transferase (GST) activity. Aliquots were used directly for assaying GSH+GSSG or GST activity, while an aliquot was alkylated with 1M 2-vinylpyridine for at least 2 h at room temperature in the dark for the GSSG assay. TBARS assay was conducted on samples deproteinized with 0.24M TCA and incubated for 10 min at 4°C; protein precipitate was removed by centrifugation at 10,000 g for 10 min at 4°C.
Preparation of Brain and Liver Samples
For the analysis of brain and liver tissue, samples were thawed on ice, weighed, and homogenized in five volumes of 0.154M KCl by eight strokes in a Dounce Wheaton B homogenizer. GST activity was assayed directly in homogenates, while GSH+GSSG and GSSG were measured in homogenates deproteinized with 30% W/V TCA and neutralized with NaOH (5M). Removal of precipitated protein was achieved by centrifugation at 14,000 g for 10 min at room temperature. GSSG levels were determined in deproteinized homogenates following incubation with 1M 2-vinylpyridine for at least 2 h at room temperature in the dark. TBARS assay was conducted on samples deproteinized with 0.24M TCA and incubated for 10 min at 4°C; protein precipitate was removed by centrifugation at 10,000 g for 10 min at 4°C.
Spectrophotometric Measurements
Spectrophotometric measurements were conducted utilizing Shimadzu UV160U or GENESYS 10S Series UV-VIS (Thermo Fisher Scientific) spectrophotometers. Glutathione pool was assayed as previously described (Tietze, 1969; Griffith, 1980), using known GSH concentration solutions as standard. TBARS assay was performed as previously reported (Ohkawa et al., 1979) and reported as μmol-eq malondialdehyde (MDA) per liter, using the molar extinction coefficient for the MDA-TBA adduct (1.56 × 105 M-1cm-1). GST activity was assayed continuously for 1–2 min at room temperature as previously described (Habig et al., 1974), in the presence of saturating concentrations of 1-chloro-2,4-dinitrobenzene as substrate (final 1 mM) and GSH as cofactor (final 4 mM) in 100 mM phosphate buffer (pH 6.5). Enzymatic activity was reported as nmol/min/ml of serum or nmol/min/g wet weight for liver and brain, using the molar extinction coefficient for the S-glutathionylated product (9.6 mM-1cm-1).
Statistical Analysis
Analyses were conducted on all data (nD+P+ = 20, nD+P- = 21, nD-P+ = 19 and nD-P- = 21). One rat in the pregnant, drug group (D+P+) died 3 days post-pregnancy termination. The data collected from this rat to that point was still included in the analysis, as it did not deviate in any way from the behaviors of the rest of the group. In order to investigate whether or not the biochemical parameters differed significantly if collected closer to the time of the drug/vehicle administration and pregnancy termination/delivery relative to tissue collection, potentially warranting further investigation, each group also consisted of 1–2 rats whose blood, brain and liver samples were collected on D3 post-partum/pregnancy termination. Independent t-tests of the data with or without these data points indicated no significant differences. Data analysis was conducted using SigmaPlot 11.0 (Systat Software, Inc.). A Two-Way Repeated Measures (RM) ANOVA with one factor repetition (week) was utilized to determine if the groups were significantly different from each other prior to Treatment Week (sucrose/water consumption, rearings, distance active, percentage time active, and overall speed). A Two-Way RM ANOVA with one factor repetition (time: day or week) was utilized to analyze the following parameters: rat weight and food intake (daily); sucrose and water consumption (weekly average; Pre-Treatment vs. Treatment Week). Sucrose and water consumption were additionally analyzed using a One-Way ANOVA (within Treatment Week). Rearings, distance active, percentage time active, and overall speed were also analyzed using a One-Way ANOVA. A Two-Way ANOVA was utilized to analyze vaginal impedance (pre-breeding vs. post). A One-Way ANOVA was utilized to analyze immobility time in the FST (n = 11–12 per group) across the groups and percentage time immobile in home-cage activity (n = 4–7 per group). Where applicable, post hoc analysis was completed using a Tukey Test. A Kruskal-Wallis One-Way ANOVA on Ranks was utilized to analyze the biochemical variables, followed by Dunn’s post hoc analysis where appropriate. Backward Stepwise Elimination Regression was utilized to determine the relationship between various biological, behavioral and biochemical variables. Two models were utilized in our analysis: model 1 included drug and pregnancy as binomial predictor variables, while model 2 introduced abortion as an additional possible binomial predictor (i.e., drug, pregnancy and abortion). Various biochemical variables were also included as additional predictors in both models, with the exception of the home-cage activity. The contribution of oxidative (see below under “Oxidative stress markers”) and non-oxidative (see below under “GST activity”) glutathione (GSH) consumption were analyzed separately. Differences were considered significant at p < 0.05 for all analyses.
Results
Biological Parameters
Rat Weight
Analysis of percentage rat weight change (relative to D1 of the gestation period) revealed a significant difference across both animal group [F(3, 1443) = 192.36] and gestational day [F(20, 1443) = 186.20], as well as the interaction between group and gestational day [F(60, 1443) = 193.97], all p < 0.001. Beginning on Day 3 (D3) of the gestation period, post hoc analysis indicated a significant increase (p < 0.01; Figure 1) in percentage weight change in the rats that carried their pregnancy to full-term (D-P+) relative to both non-pregnant groups (D-P- and D+P-). This significant increase in percentage weight continued throughout the rest of the gestation period (Relative to D-P- and D+P- on D4–21: p < 0.001). Rats whose pregnancy was terminated at mid-term (D+P+) experienced an initial similar significant increase in percentage weight change (relative to both D-P- and D+P- on D3–4: p < 0.01; D5–12: p < 0.001). However, this increase was only until the administration of first injection of mifepristone (D12).
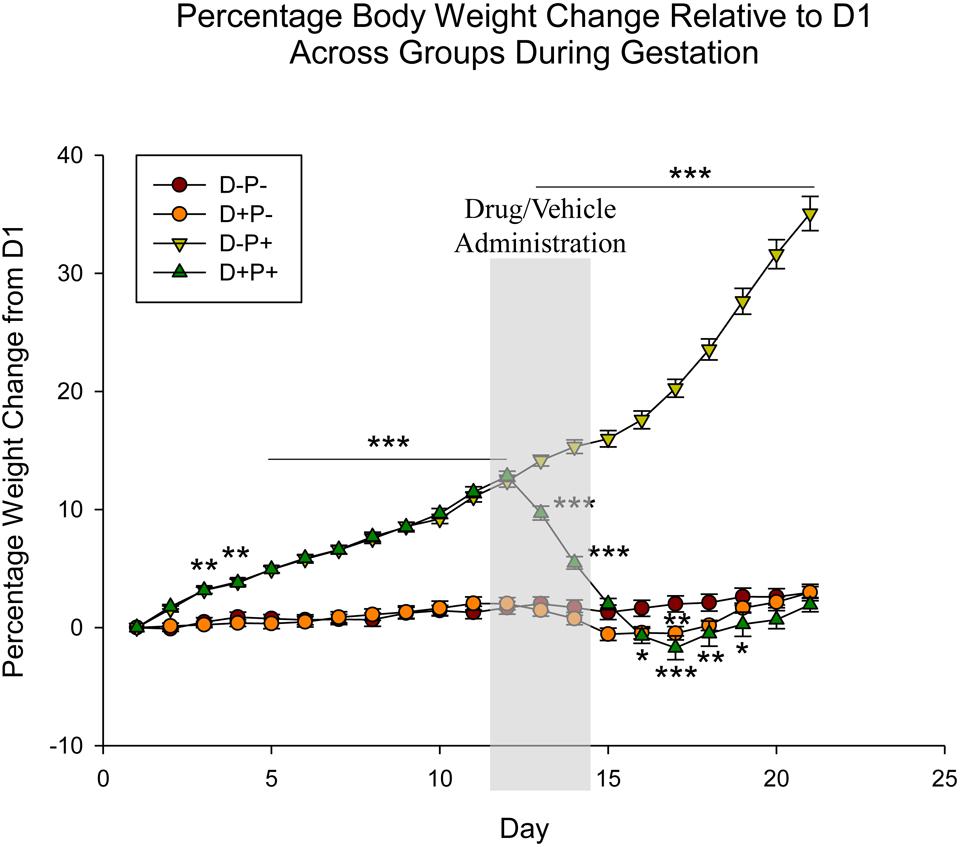
Figure 1. Percentage rat body weight change across gestation period relative to Day 1 (D1) of pregnancy. Following pregnancy termination, rats in the D+P+ group showed significant weight loss, decreasing below baseline (D-P-). n = 19–20 rats/group; ∗p < 0.05, ∗∗p < 0.01, ∗∗∗p < 0.001 (Relative to D-P-). Data is expressed as mean ± SEM. Shaded area indicates days of drug/vehicle administration.
Beginning on D13, rats in the D+P+ group experienced a steady and significant decrease in percentage weight relative to the D-P+ group (D13–17: p < 0.001), with the maximum percentage weight loss being D17. Starting on D16, the percentage weight was also significantly lower than the D-P- group (D16, 19: p < 0.05; D18: p < 0.01 D17: p < 0.001). The percentage weight of the D+P+ group following D19 remained below that of the D-P- group until D22. These differences, however, were not significant (p > 0.05). Additionally, the D+P+ group also remained significantly lower than the D-P+ group through full-term (D21, p < 0.001).
Non-pregnant rats who received mifepristone and misoprostol (D+P-) also experienced a decrease in percentage weight following drug administration; however, the decrease was only significantly lower than the percentage weights of the control group (D-P-) on D16 (p < 0.05) and D17 (p < 0.01). The D+P- group remained below the percentage weights of the D-P- group until D20.
Rat weight: comparison of full-term pregnancy (D-P+), medical abortion (D+P+) and miscarriage (spontaneous abortion)
Interestingly, the observations just described pertaining to changes in rat weight in the D+P+ group appear to be specific to rats who underwent a drug-induced termination, but not to rats that experienced a natural miscarriage (spontaneous abortion). A group of rats (n = 5) that was being bred for the maintenance of the colony experienced a spontaneous abortion (n = 4 between D13 and 16, n = 1 at D6 of gestation). A pregnancy was considered miscarried if the rat weight relative to D1 of gestation was equal to or less than the previous day for more than 2 days, and did not display a return to normal weight (potentially indicative of pseudopregnancy; Kennedy and Mitra, 1963; Dewar, 1964). The day of miscarriage was considered as the last day of weight gain.
Analysis of the miscarriage data relative to the D-P+ (full-term pregnancy) and D+P+ (medical abortion) groups revealed significant effects of group [F(2, 798) = 123.43], day [F(20, 798) = 120.30] and the interaction between group and day [F(40, 798) = 170.33], all p < 0.001. Post hoc analysis revealed no significant difference between the three groups through D12 (p > 0.05). As previously described, the rat weights of the D-P+ group were significantly higher than those of the D+P+ group beginning D13 through D21 (all p < 0.001). Following D13, the rat weights of the miscarriage group stabilized, showing no significant differences (all p > 0.05) between each day relative to D13, as well as to the previous day.
As a result of the stabilization in rat weight that occurred following the miscarriage, beginning D16 through D21, the miscarriage group was significantly lower than the rats that carried the pregnancy to full-term (D-P+; D16: p < 0.05; D17–21: p < 0.001).
The miscarriage group also showed significant differences relative to the rats that underwent a pregnancy termination (D+P+). Specifically, the latter group displayed a significant weight loss relative to the miscarriage group starting D13 through D21 (D13: p < 0.05; D14–21: p < 0.001) (Figure 2).
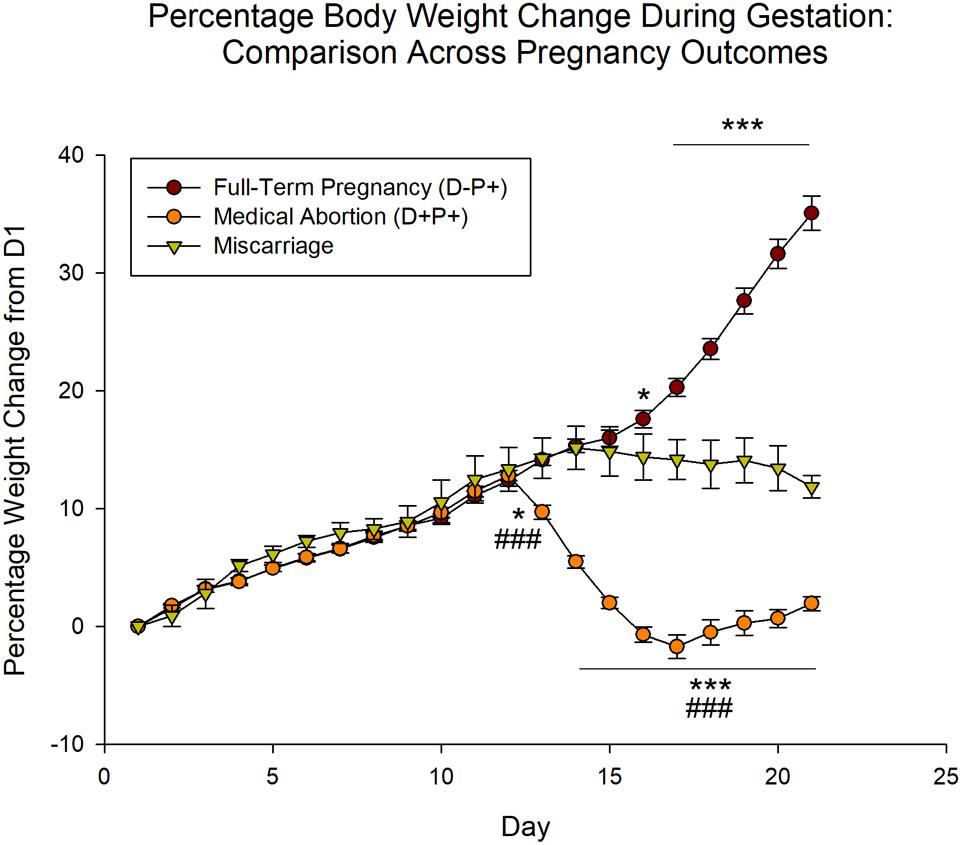
Figure 2. Percentage rat body weight change during gestation period relative to Day 1 (D1) across pregnancy outcomes. Unlike rats undergoing pregnancy termination, which displayed a significant weight loss, the body weight of rats that naturally miscarried did not decrease significantly, but stabilized following the miscarriage. n = 19–20 rats/group (D+P+ and D-P+); n = 5 rats (miscarriage group). ∗p < 0.05, ∗∗∗p < 0.001 (Relative to Miscarriage Group); ###p < 0.001 (Relative to D-P+). Data is expressed as mean ± SEM.
Food Intake
Food intake was analyzed as a percentage of rat weight, given that rats will consume food in proportion to their weight. Statistical analysis indicated a significant difference across both animal group [F(3, 1180) = 42.50] and gestational day [F(20, 1180) = 28.33], as well as the interaction between group and day [F(60, 1180) = 9.18], all p < 0.001. Days prior to D12 revealed random significant differences (p < 0.05) between the two pregnant groups (D-P+ and D+P+) and the two non-pregnant groups (D+P- and D-P-). However, starting D13, food intake in the D+P+ group was significantly reduced relative to the D-P+ group until D20 (D13–19: p < 0.001; D20: p < 0.05). In the rats whose pregnancy was terminated (D+P+), there was a significant decrease in food intake relative to the control group (D-P-) following the pregnancy termination on D13 to D18 (D13: p < 0.05; D14–18: p < 0.001; Figure 3). Moreover, rats whose pregnancy was terminated (D+P+) consumed significantly less food than the non-pregnant rats who received drug (D+P-) following drug administration (D14–18: p < 0.001, D19: p < 0.01). Although food intake was also reduced in the drug group (D+P-) relative to D-P-, it was only significant on D15–16 (p < 0.05).
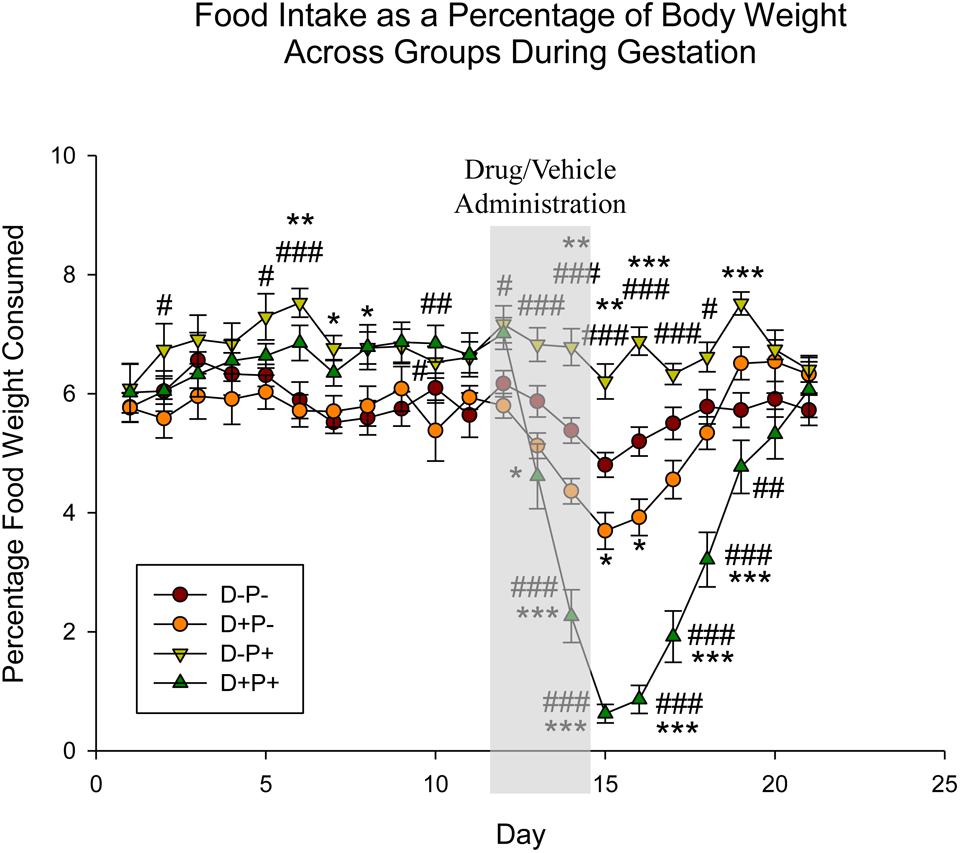
Figure 3. Food intake as a percentage of body weight across gestation period. Pregnancy termination induced a significant reduction in food intake. n = 15–17 rats/group; ∗p < 0.05, ∗∗p < 0.01, ∗∗∗p < 0.001 (Relative to D-P-); #p < 0.05, ##p < 0.01, ###p < 0.001 (Relative to D+P-). Data is expressed as mean ± SEM. Shaded area indicates days of drug/vehicle administration.
Food intake: comparison of full-term pregnancy (D-P+), medical abortion (D+P+) and miscarriage (spontaneous abortion)
Similar to the rat weight, the observations just described pertaining to food intake appear to be specific to rats who underwent a drug-induced termination, but not to rats that experienced a natural miscarriage. Analysis of the data comparing food intake (n = 4; one rat was not included in analysis due to hoarding of food, which did not enable the accurate measurement of food intake) across the various pregnancy outcomes (D-P+, D+P+ and miscarriage), setting the day of miscarriage as D0, and aligning this day with D12 (first day of drug/vehicle administration, also assigned as D0 for the purpose of this analysis) in the D+P+ and D-P+ groups, revealed a significant difference across group [F(2, 248) = 39.90], day [F(8, 248) = 20.61] and the interaction of group and day [F(16, 248) = 22.44], all p < 0.001.
Post hoc analysis revealed no significant difference in food intake in the miscarriage and D-P+ groups across both group and day from D-4 through D4 (all p > 0.05). There was also no significant difference in the D+P+ group, both across day and relative to the other two groups from D-4 through D0 (all p > 0.05). Beginning D1 through D4, food intake for the D+P+ group was significantly lower than both the miscarriage (D1: p < 0.01; D2–4: p < 0.001) and the D-P+ groups (all p < 0.001) (Figure 4).
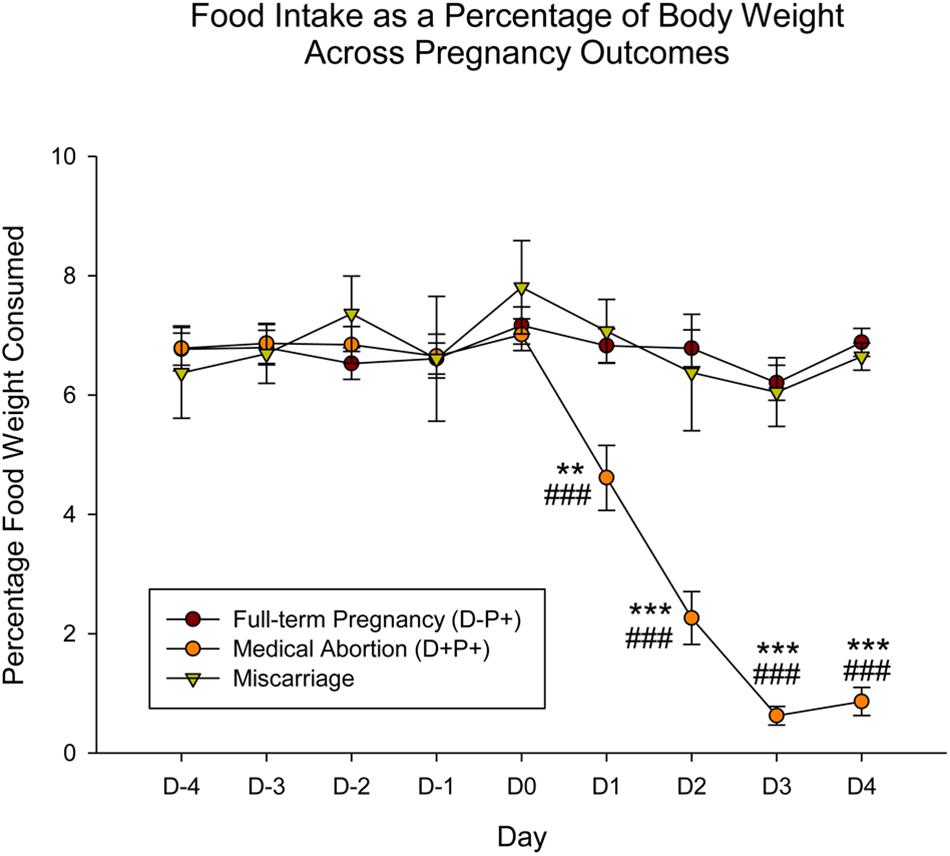
Figure 4. Food intake as a percentage of body weight across gestation period across pregnancy outcomes. In contrast to the significant decrease in food intake displayed by rats in the D+P+ group, rats that naturally miscarried did not display any change in food intake. Pregnancy termination induced a significant reduction in food intake. n = 15 rats/group (D+P+ and D-P+); n = 4 rats (miscarriage group). ∗∗p < 0.01, ∗∗∗p < 0.001 (Relative to Miscarriage Group); ###p < 0.001 (Relative to D-P+). Data is expressed as mean ± SEM.
Vaginal Impedance
The collective average impedance of each group was analyzed comparing the measurements collected prior to breeding (pre-breeding) with 11 and 12 weeks post-breeding (post-breeding) and equivalent days for control groups. Statistical analysis revealed a significant difference in vaginal impedance across time [F(1, 2503) = 67.20, p < 0.001], as well as experimental group [F(3, 2503) = 3.70, p < 0.05] and the interaction between group and time [F(3, 2503) = 3.15, p < 0.05, Figure 5].
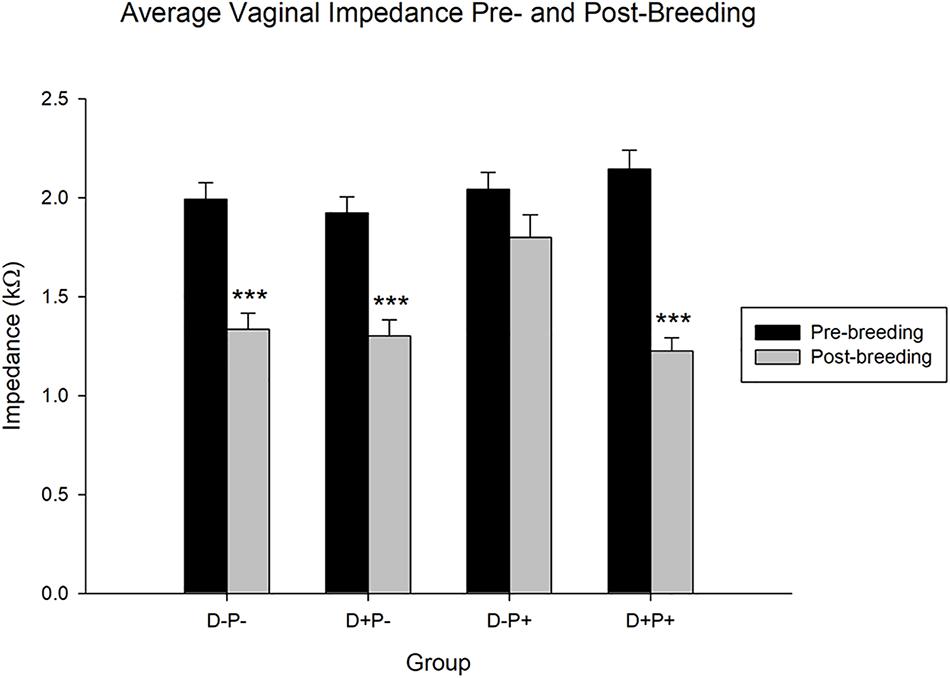
Figure 5. Average vaginal impedance (kΩ) pre- and post-breeding. All groups with the exception of the D-P+ group showed a significant decrease in the average vaginal impedance over time. Data points include the impedance of rats from n = 19–21 rats/group for pre-breeding and n = 11–13 rats/group for post-breeding. Data is expressed as mean ± SEM. ∗∗∗p < 0.001 (Relative to pre-breeding levels).
Post hoc analysis indicated no significant difference (p > 0.05) in pre-breeding impedance measurements between the groups. However, the same test revealed that the average vaginal impedance levels of all groups except the rats that carried their pregnancy to full-term (D-P+) decreased significantly post-breeding (all p < 0.001) relative to their pre-breeding levels. Thus, the rats that carried their pregnancy to full-term and delivered (D-P+) did not experience a significant decrease (p > 0.05) in their vaginal impedance over the timeframe of the experiment. Moreover, while the post-breeding vaginal impedance of the D-P-, D+P-, and D+P+ groups were not significantly different from each other (p > 0.05), the vaginal impedance of the D-P+ group was significantly higher post-breeding (all p < 0.05) relative to the other three groups (D-P-, D+P-, D+P+).
Behavioral Parameters
Comparison of Behaviors Prior to Treatment Week
Analysis of the data pertaining to sucrose/water consumption, rearings, distance active, percentage time active and overall speed, in weeks prior to treatment indicated no significant difference between the experimental groups in any of these behaviors (all p > 0.05).
Sucrose and Water Consumption/Preference
Pertaining to sucrose consumption (g/100 g rat weight), analysis indicated a significant difference between groups [F(3, 77) = 2.85, p < 0.05] in Treatment Week. Post hoc analysis revealed a significant difference in sucrose consumption between D+P+ and D+P- (p < 0.05). Sucrose consumption for the D+P+ group in Treatment Week was not significantly different relative to any other group (p > 0.05) (Figure 6A).
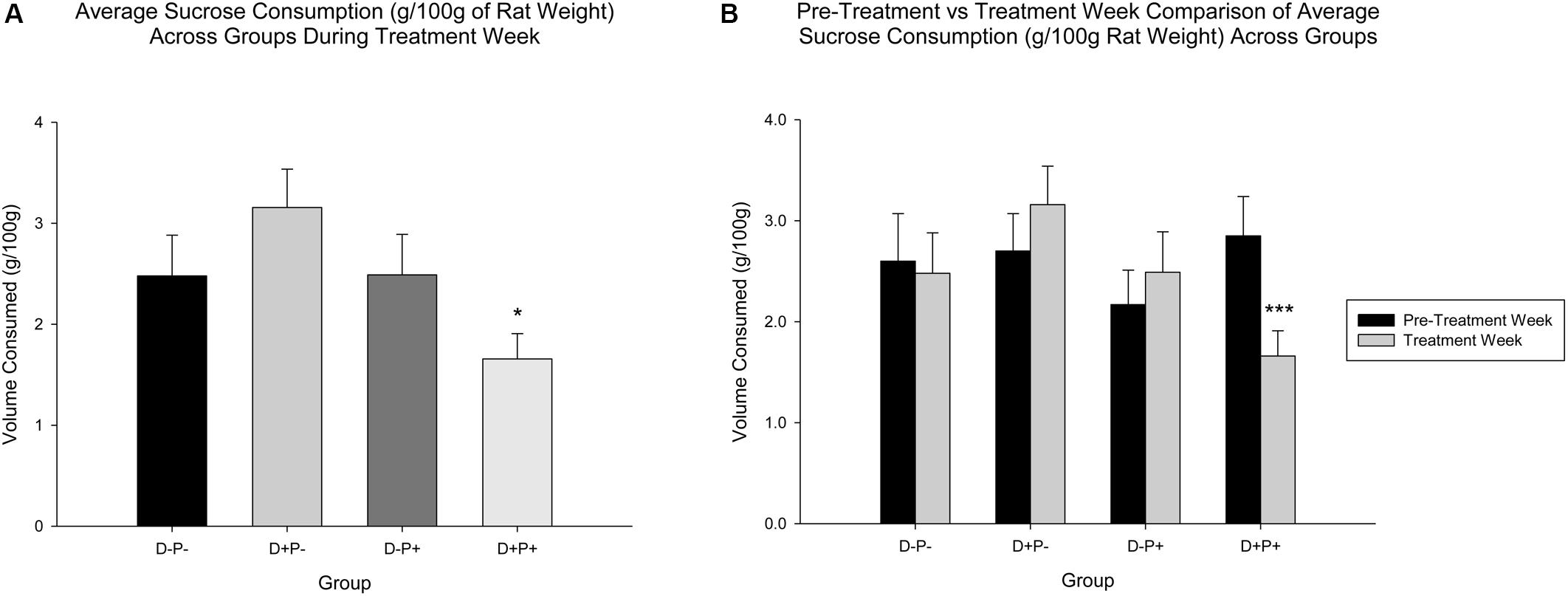
Figure 6. (A) Average sucrose consumption (g/100 g rat weight) during treatment week. Sucrose consumption was significantly reduced only in the pregnancy termination group (D+P+) relative to the D+P- group. n = 19–21 rats/group; ∗p < 0.05. (B) Average sucrose consumption (g/100 g rat weight) in pre-treatment vs. treatment week. The D+P+ group was the only group with a significant reduction in average sucrose consumption, during treatment week relative to pre-treatment week. n = 15–17 rats/group; ∗∗∗p < 0.001. Data is expressed as mean ± SEM.
A comparison of Treatment Week relative to Pre-Treatment Week, however, revealed no significant differences across group [F(3, 61) = 1.43, p > 0.05) or week [F(1, 61) = 1.13, p > 0.05], but a significant interaction of group and week [F(3, 61) = 5.17, p < 0.01]. Post hoc analysis revealed that sucrose consumption was significantly reduced in Treatment Week relative to Pre-Treatment Week, only in the D+P+ group (p < 0.001). All other differences across the weeks, within the other groups (D-P-, D+P-, D-P+) were not significant (all p > 0.05) (Figure 6B).
Water consumption was not significantly different during Treatment Week across groups [F(3, 77) = 0.91, p > 0.05]. There was also no significant difference in water consumption across group, week and the interaction between group and week when comparing Treatment Week to Pre-Treatment Week [group: F(3, 61) = 0.27; week: F(1, 61) = 1.34, group × week: F(3, 61) = 0.85, all p > 0.05].
Rearings
Relative to the number of rearings, there was a significant difference between the treatment groups [F(3, 77) = 15.09, p < 0.001]. Post hoc analysis revealed that rats in the pregnancy termination group (D+P+) displayed a significant decrease in number of rearings during Treatment Week relative to all other groups (D-P- and D+P-: p < 0.001; D-P+: p < 0.01; Figure 7).
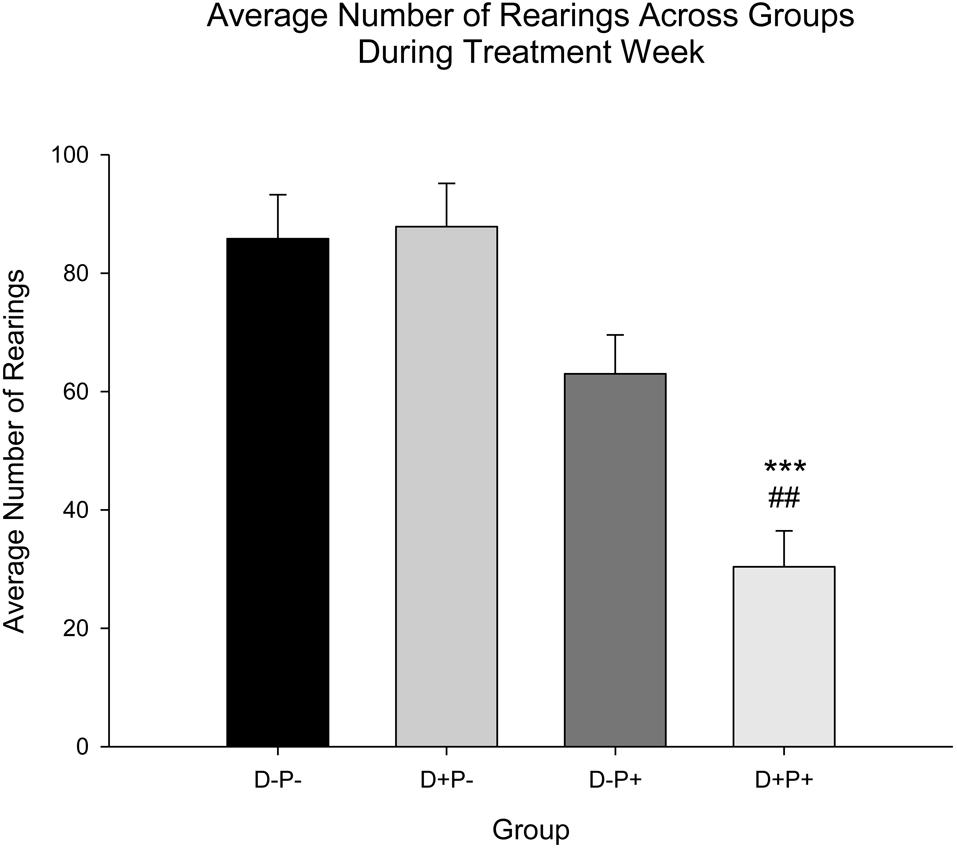
Figure 7. Average number of rearings during treatment week. The number of rearings was only significantly reduced during Treatment Week in the pregnancy termination group (D+P+) relative to all other groups (D-P-, D+P-, D-P+). n = 19–21 rats/group; ∗∗∗p < 0.001 (Relative to D-P- and D+P-); ##p < 0.01 (Relative to D-P+). Data is expressed as mean ± SEM.
Distance Active, Percentage Time Active, and Overall Speed
Additional locomotor parameters measured included distance active (Figure 8A), percentage time active (Figure 8B), and overall speed (Figure 8C). Analysis revealed a significant difference between treatment groups during Treatment Week for distance active [F(3, 76) = 14.15], percentage time active [F(3, 76) = 13.35], and overall speed [F(3, 76) = 14.18], all p < 0.001. Post hoc analysis revealed a significant decrease in all locomotor parameters (distance active, percentage time active and overall speed) in rats whose pregnancy was terminated (D+P+) relative to all other groups (all parameters: Relative to D+P- and D-P-p < 0.001; D-P+ p < 0.01).
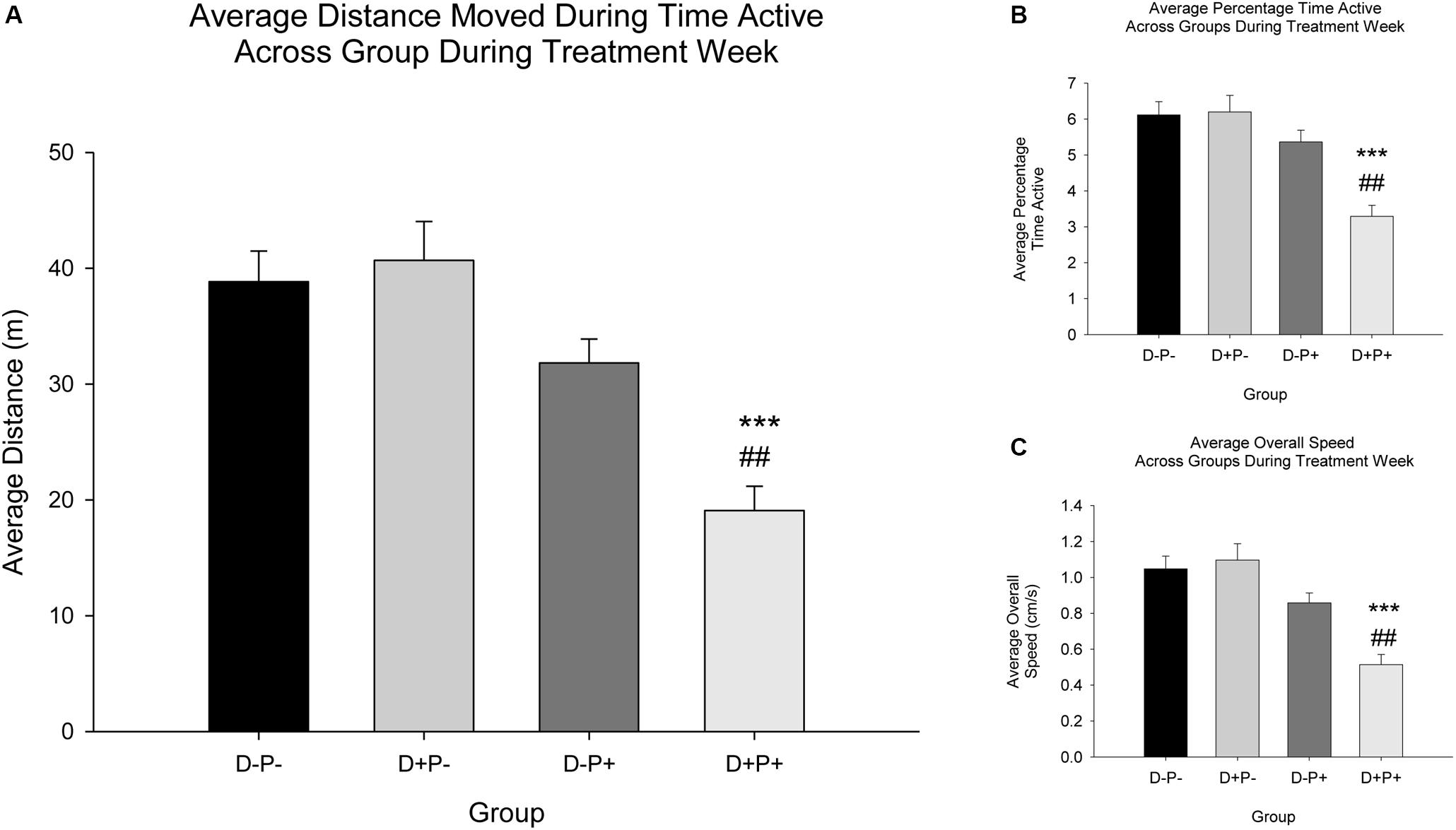
Figure 8. Locomotor parameters across groups during treatment week. (A) Average distance moved during time active (m); (B) Average percentage time active; (C) Average overall speed (cm/s). A significant decrease in all three parameters (A–C) was observed in the D+P+ group relative to all other groups (D-P-, D+P-, D-P+). ∗∗∗ p < 0.001 (Relative to D-P- and D+P-); ##p < 0.01 (Relative to D-P+). Data is expressed as mean ± SEM.
Behaviors Post-delivery
The changes in sucrose consumption (including relative to the previous week), rearings, distance active, percentage time active and overall speed reported above, pertaining to the D+P+ group following pregnancy termination, were not observed in the D-P+ group following delivery relative to the non-pregnant control group (D-P-) (all p > 0.05).
Testing Cage Corner Activity
Corner activity (NE, SE, SW; see Figure 9 inset), measured as time spent in corner as a percentage of the total testing time, during Treatment Week was significantly different between groups [F(3, 152) = 5.29, p < 0.01], corner [F(2, 152) = 13.92, p < 0.001] and the interaction of group and corner [F(6, 152) = 6.12, p < 0.001]. Post hoc analysis revealed a significant difference between the D+P+ group and all other groups (D-P-, D-P+, D+P-) within the SW corner (all p < 0.001; Figure 9). Moreover, the percentage time spent in the SW corner by the D+P+ group was also significantly higher than the two other corners (both p < 0.001). All other comparisons were not significant (all p > 0.05).
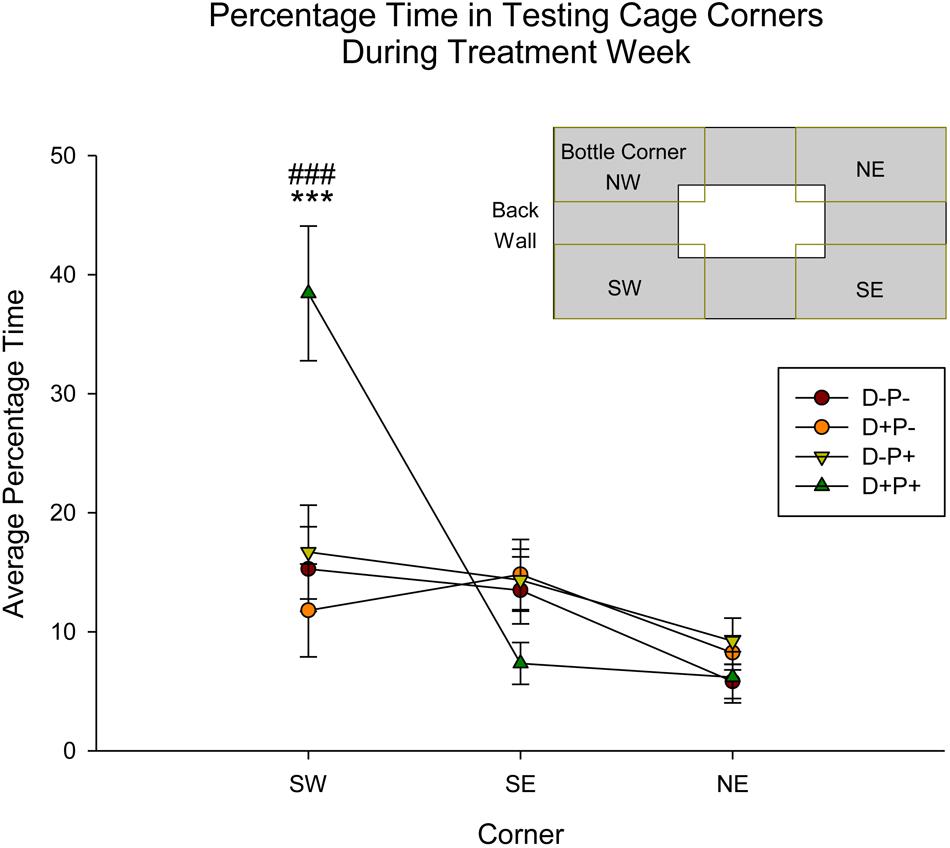
Figure 9. Percentage time in specific testing cage corners during treatment week. The D+P+ group spent significantly more time in the SW corner of the testing cage relative to all other groups, as well as relative to the SE and NE corners. ∗∗∗p < 0.001 (Relative to all groups); ###p < 0.001 (Relative to SE and NE corner). Data is expressed as mean ± SEM. Inset: Cage and corner orientation.
Home-Cage Activity
Analysis of the home-cage activity (percentage time immobile) revealed no significant difference between any of the groups Pre-Treatment (D11) [F(3, 19) = 0.39, p > 0.05]. However, analysis did indicate a significant difference in home-cage immobility between groups Post-Treatment [D3PP; F(3, 19) = 4.69, p < 0.05]. Post hoc analysis revealed a significant increase in immobility in the D+P+ group relative to both D-P- and D+P- (both p < 0.05, Figure 10). There was no significant difference between the D+P+ and D-P+ groups (p > 0.05).
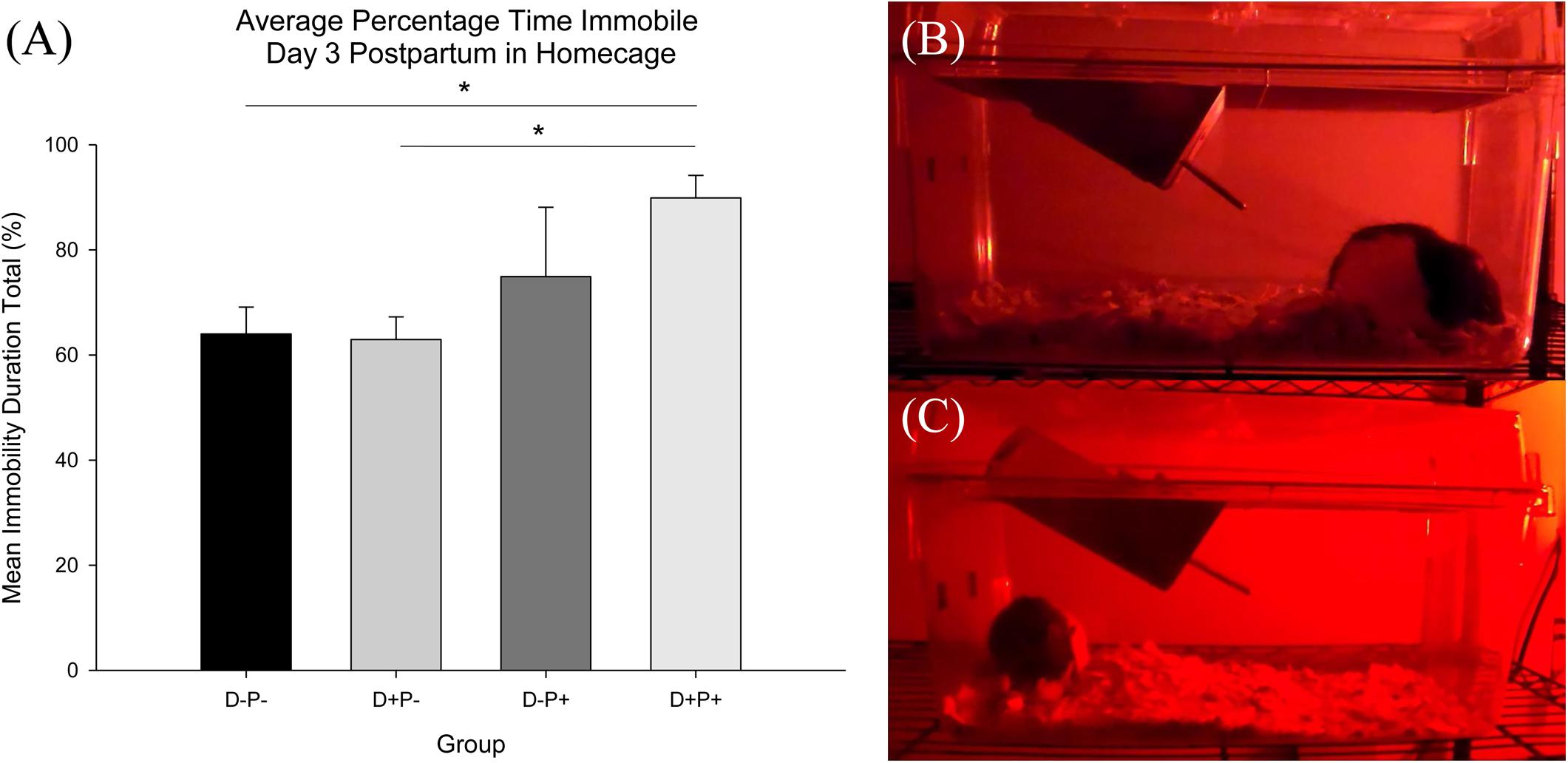
Figure 10. (A) Average percentage time immobile in home-cage. Percentage immobility time was significantly reduced in the D+P+ group relative to D-P- and D+P-. ∗p < 0.05. Data is expressed as mean ± SEM. (B,C) Freeze-frame shot of home-cage behavior recording during dark cycle day 3 postpartum. (B) D+P+; hunched posture typical of D+P+ group and (C) D-P+; mother nursing pups.
Forced Swim Test
There was no statistically significant difference between the groups in percentage immobility duration in the Forced Swim Test on Day 3 post-partum [D-P-: M = 23.82, SEM = 4.47; D+P-: M = 30.67, SEM = 5.19; D-P+: M = 32.39; SEM = 4.90; D+P+: M = 31.73, SEM = 5.03; F(3, 43) = 0.67], p > 0.05. Potential confounds and limitations pertaining to this test will be explained in the Discussion.
Biochemical Parameters
Oxidative Stress Markers
Markers of oxidative stress relative to the glutathione pool [GSH-reduced (GSH), GSH-oxidized (GSSG), GSH/GSSG ratio (Ratio), and redox potential (Redox)] and lipid peroxidation end products (TBARS) were measured in serum (s), brain (b), and liver (l) samples collected from the rats at the end of the study, which are summarized in Table 1. Analysis indicated a significant difference between groups in sGSH (H = 20.85; p < 0.001), sGSSG (H = 13.46; p < 0.01), sRatio (H = 25.45; p < 0.001), and sRedox (H = 24.06; p < 0.001). Post hoc analysis revealed a significant difference (p < 0.05) between D-P- and both D+P- and D-P+, as well as between D+P+ and D+P- in sGSH, sRatio (Figure 11A) and sRedox (Figure 11B). There was also a significant difference (p < 0.05) in sGSSG between D+P- and both D-P- and D+P+. All other comparisons were non-significant across the groups (p > 0.05).
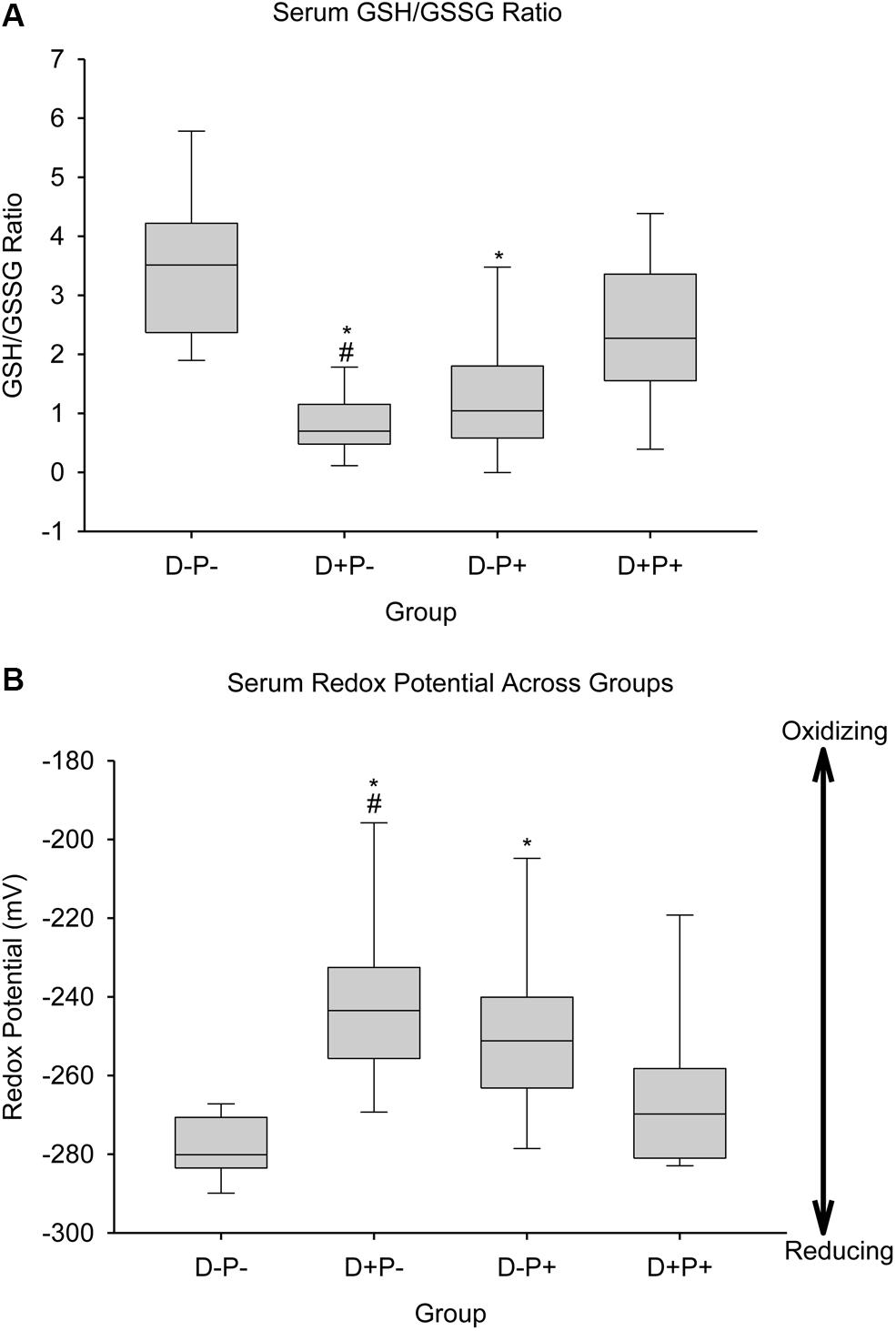
Figure 11. Biochemical parameters reflecting oxidative consumption of glutathione. (A) Serum GSH/GSSG ratio and (B) Serum redox potential of the glutathione pair. Data for serum GSH/GSSG ratio and redox potential of the serum glutathione pair are shown as boxes (25–75% percentiles) and whiskers (min to max values) for each group, with inside line indicating the median of each group. n = 10–13 rats per group; ∗p < 0.05 (Relative to D-P-); #p < 0.05 (Relative to D+P+).
GST Activity
As a marker of non-oxidative consumption of GSH, GST activity was assayed at the end of the study in serum, brain and liver samples. Statistical analysis did not reveal any significant differences between the different treatment groups (p > 0.05; Table 1).
Multiple Regression Analysis
In order to evaluate potential correlations between the biological, behavioral and biochemical parameters measured in this study and potential predictor variables for the behaviors reported, multiple linear regression analyses were conducted using the backward stepwise elimination method. Biological and behavioral parameters during Treatment Week were considered dependent variables (outcomes). Detailed effect sizes (β-values) with their p-values are shown in the supplemental data included with this article (see Supplementary Tables 1–15), while Tables 2, 3 summarize the significant variables associated with oxidative consumption included as final predictors of each behavioral variable.
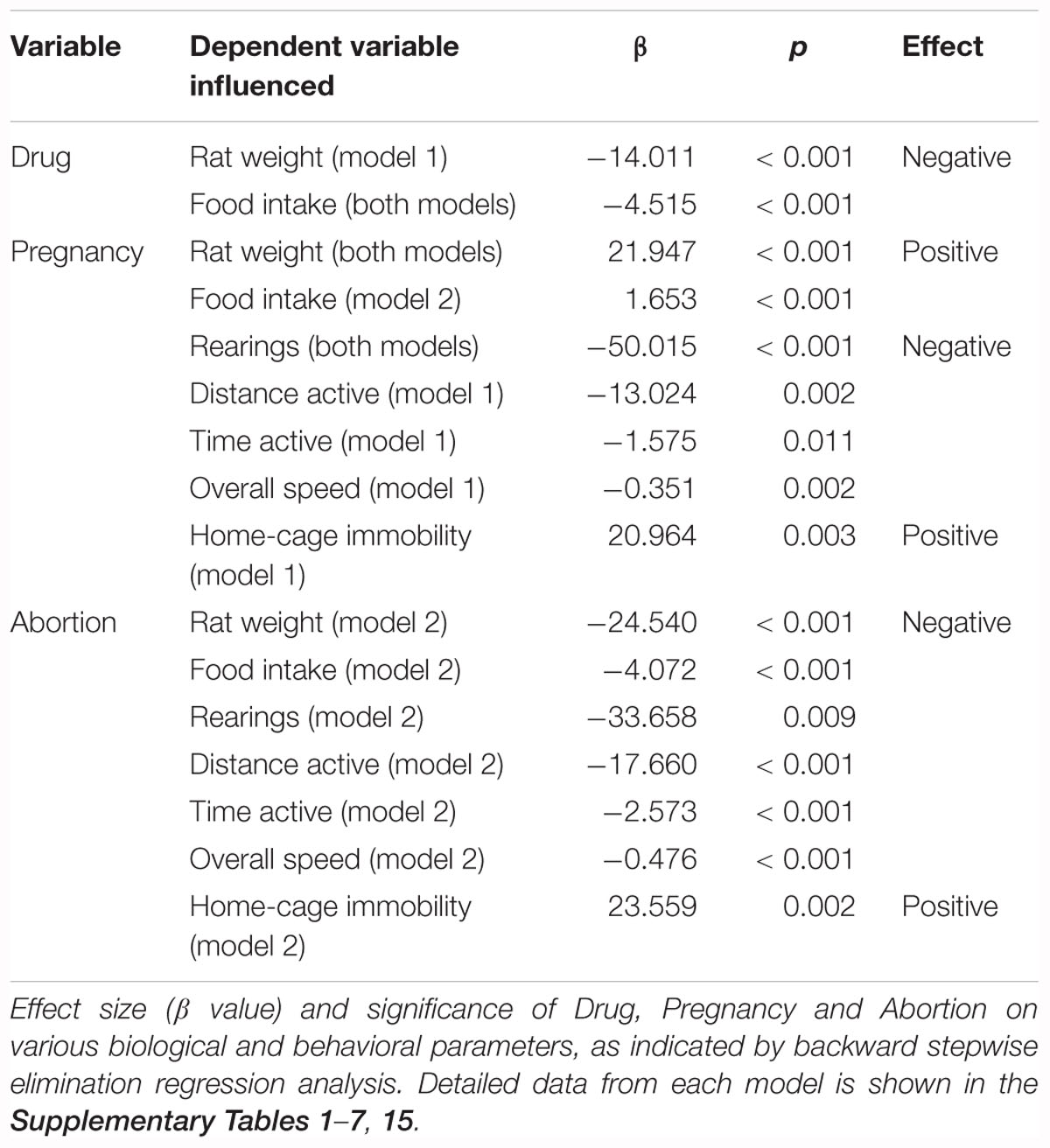
Table 2. Summary of treatment variables (drug, pregnancy, abortion) as final significant predictors for biological and behavioral variables.
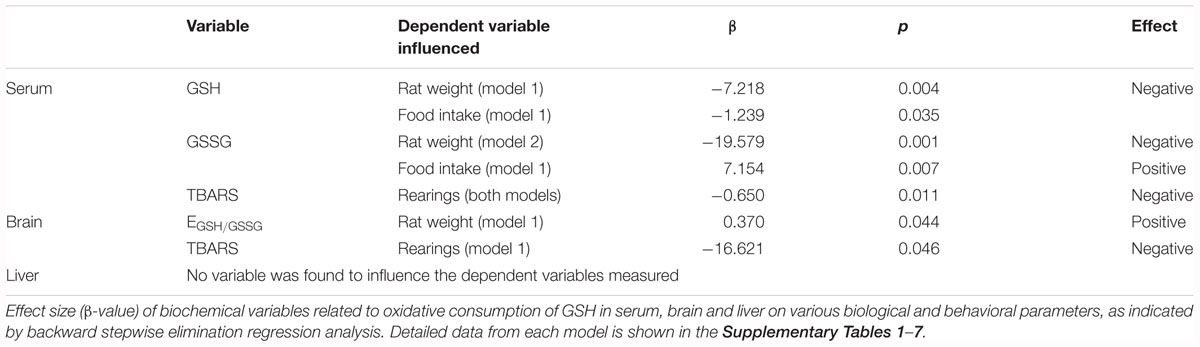
Table 3. Summary of final significant biochemical (oxidative consumption) predictors for biological and behavioral variables.
The analysis revealed various relationships between drug, pregnancy and abortion and the dependent variables (biological and behavioral) previously mentioned (i.e., rat weight, impedance, food intake, rearings, distance active, percentage time active and overall speed). Our results from the analysis of variables involved in oxidative consumption of GSH (Table 2) indicated that drug was a predictor of rat weight only in model 1 (excludes abortion as a predictor variable). However, pregnancy was found to be a predictor of rat weight regardless of whether or not abortion was considered as a predictor (model 1: R2= 0.760, model 2: R2= 0.923). Food intake was predicted by drug in both models (model 1: R2= 0.826; model 2: R2= 0.896), while it was predicted by pregnancy in model 2 only (when abortion was included as a predictor variable). Similar relationships were present with the behavioral variables (i.e., rearings, distance active, percentage time active and overall speed). Pregnancy predicted distance active, percentage time active and overall speed only when abortion was not included as a variable in the model (i.e., model 1; R2 range = 0.147–0.214). However, pregnancy predicted rearings in both models (model 1: R2= 0.473, model 2: R2= 0.510). The inclusion of abortion as a predictor variable (i.e., model 2, R2 range = 0.264–0.923) indicated that this variable predicted rat weight, food intake and all behavioral variables measured.
Pertaining to the biochemical predictor variables, rat weight was found to be predicted by serum GSH and brain redox potential in model 1, while it was only predicted by serum GSSG levels when abortion was included as a possible predictor (model 2) (Table 3). Moreover, food intake was predicted by both serum GSH and GSSG levels when abortion was not included in the model (model 1) (Table 3). The biochemical variables measured were not significant predictors of the distance active, percentage time active and overall speed. However, serum TBARS predicted rearings in both models, while brain TBARS was a predictor only in model 1.
Regression analysis with the non-oxidative biochemical variables (see Supplementary Tables 8–14) revealed identical effects of similar magnitude to that of the analysis with the oxidative variables (Table 2). The only exceptions to this were impedance that was found to be predicted by abortion (in model 2) and rearings that was predicted by pregnancy only in model 1. Serum, liver and brain GST were not found to influence any of the dependent variables measured.
Regression analysis with home-cage immobility as the dependent variable indicated that while pregnancy was a significant predictor in model 1 (abortion not included as a predictor), when abortion was added as a potential predictor (model 2), pregnancy no longer predicted the home-cage immobility behavior. In this model (model 2), abortion was the only predictor of the observed behavior (see Supplementary Table 15). Biochemical parameters were not measured in these rats, and therefore, were not included in the regression analysis.
Thus, the results from the regression analyses appear to corroborate the observations from the previously mentioned analyses.
Discussion
This study investigated the biological, behavioral and physiological consequences of pharmacologically terminating a pregnancy at mid-term (first-trimester human equivalent) in an animal model. Taken together, our analyses appear to indicate a significant effect of pregnancy termination on the biological (rat weight, food intake, vaginal impedance), physiological (oxidative balance) and most especially, behavioral parameters (sucrose consumption, rearings, distance active, percentage time active, overall speed) measured.
Body Weight and Food Intake
Body weight and food intake were most notably affected in the pregnancy termination group (D+P+) relative to all other groups. Although there was a decrease in these parameters in the drug group (D+P-), the behavior in this specific group is clearly not indicative of a lack of well-being of the rat, in contrast to the D+P+ group. This is evident in the difference of the magnitude of the observed effects, as well as observations relative to grooming, exploratory behavior and general activity, which will be further discussed below under “Home-cage behavior and locomotor activity.” Furthermore, the specific influence of the drug-induced termination on food intake was further confirmed by the absence of similar observations in rats who naturally miscarried.
Previous literature indicates an effect of stress on both body weight and food intake. In humans, stress has been shown to be associated with both an increase and decrease in body weight and is influenced by various variables unique to the specific situations (Korkeila et al., 1998; Kivimaki et al., 2006; Stinson et al., 2018). In animals, however, stress has been primarily associated with weight loss (Alario et al., 1987; Harris et al., 2002; Dallman et al., 2003). Our findings appear to suggest a similar impact of pregnancy termination on body weight. Regarding food intake, the literature reports a variety of effects on food consumption and appetite following periods of stress or depression at both the level of the animal (Marti et al., 1994; Brown and Grunberg, 1996; Torres and Nowson, 2007) and human (Cartwright et al., 2003; Torres and Nowson, 2007; Mikolajczyk et al., 2009; Konttinen et al., 2010). Previous literature (Torres and Nowson, 2007; Harris, 2015) also indicates that in animal models, the specific effect of stress on food intake is dependent on the level of the stressor. Mild stressors have been shown to generally not alter food consumption in rats (Bertiere et al., 1984; Marti et al., 1994; Willner, 1997), whereas moderate and severe stressors have resulted in decreased food consumption (Alario et al., 1987; Monteiro et al., 1989; Krebs et al., 1996; Valles et al., 2000; Armario et al., 2004). Thus, our food intake results from the pregnancy termination group (D+P+) suggest that their behavior demonstrates a similarity to the findings observed in the case of moderate to severe stressors. Our results further indicate that the observed biological changes are distinguished from what was observed following natural miscarriages.
Sucrose Consumption
Relative to sucrose consumption, previous studies have reported a decrease in sweet solution consumption relative to baseline and control, following various chronic mild stress protocols, which is interpreted to be indicative of a putative anhedonic effect (Willner et al., 1987; Muscat and Willner, 1992; Willner, 1997, 2017; Grippo et al., 2003, 2006; Grønli et al., 2005; Rossetti et al., 2016). Our results appear to suggest a similar effect (decrease) of pregnancy termination (D+P+) on sucrose consumption, with the effects being reported during the exposure to the stressor (Willner et al., 1987; Muscat and Willner, 1992; Grippo et al., 2003; Rossetti et al., 2016). Moreover, the findings of Grippo and colleagues (Grippo et al., 2003) indicate a recovery of the behavior following the discontinuation of the chronic mild stress protocol.
Home-Cage Behavior, Locomotor Activity, and Corner Activity
Subjective observations of the rats in their home cages, made by the investigators, indicated the following distinct negative behaviors (NIH, 2016) in the D+P+ group following pregnancy termination, relative to all other groups: a clear reduction in grooming, an unkempt coat, a reduction in exploratory behavior (e.g., rearing, sniffing) when investigators entered the housing room, a reduction in general activity, increased immobility and the assumption of a stooped/hunched posture in the corner of the cage (see Figures 10B,C). Importantly, these parameters are indicators of a lack of a general well-being and health of the rat (NIH, 2016). Home-cage recordings were conducted in an effort to objectively capture and analyze aspects of these behaviors, most specifically, the increased immobility. The objective analysis of the recordings confirmed the subjective observations, indicating decreased activity in the home cage in the D+P+ group 3 days post pregnancy termination.
These observations also appear to be supported by the behavioral measures collected in the testing cage during the experimental testing period. This included a significant reduction in all locomotor activity parameters measured (i.e., distance active, percentage time active and overall speed, as well as rearings) the week of the pregnancy termination in the D+P+ group, relative to all other groups. Similar to body weight and food intake, previous literature indicates a variety of effects on locomotor activity following periods of stress or depression in both animals (Hooks et al., 1991; Gorka et al., 1996; Willner, 1997; Grønli et al., 2005; You et al., 2011) and humans (American Psychiatric Association, 2013). Additionally, the corner activity appears to further support the other locomotor measures, indicating a potentially increased level of anxiety-like behavior in the D+P+ group, as displayed by the significantly higher percentage of time spent in the back corner of the testing cage. This is potentially reflective of behaviors similar to those reported in open-field studies (Sestakova et al., 2013; Crumeyrolle-Arias et al., 2014; Sarkaki et al., 2015).
FST Immobility
Relative to the forced swim test, no significant differences were observed. Given the negative behaviors reported above, as well as the effects of stress on this behavior, previously reported in the literature (Porsolt et al., 1977; Castagné et al., 2010; Slattery and Cryan, 2012), this was somewhat unexpected. However, the literature appears to be divided relative to the effects of various stressors on the forced swim test, with reports of depressant, antidepressant and no effect (Bogdanova et al., 2013), including in chronic mild stress (Harro et al., 2001; Haidkind et al., 2003; Bourke and Neigh, 2011). Therefore, it is potentially possible that the forced swim test is not sensitive to the apparent stress observed following the induced pregnancy termination procedure.
Vaginal Impedance and Fecundity
Another parameter measured in our study was vaginal impedance, as an indicator of estrus and therefore of an increased sexual receptivity (Bartos, 1977; Taradach, 1982; Rezac, 2008; Hockey et al., 2010). The relationship between (a) the estrous cycle, (b) estrus and the associated increased sexual receptivity, (c) fecundity, and (d) the use of vaginal impedance measurements as an objective method for the purpose of fertility management has been previously documented in the literature (Singletary et al., 2005; Rezac, 2008; Luno et al., 2013).
The reduced impedance, between the beginning and the end of the experimental period, in all groups except the rats that carried the pregnancy to full-term (D-P+), appears to indicate a potential effect of pregnancy on the duration of fecundity (the ability to reproduce). Our results appear to suggest that parity is more important than gravidity for fecundity, given that the impedance of the rats whose pregnancy was terminated (D+P+) decreased significantly over the experimental time-period, yet was equivalent to the non-pregnant, vehicle group (D-P-). This potentially corroborates previous research, suggesting that the act of conceiving is not sufficient to lead to the protective effects of pregnancy in humans (Baird and Dunson, 2003; Russo et al., 2005; Lanfranchi and Fagan, 2014a) and in rats (Russo and Russo, 1980; Sinha et al., 1988; Walker et al., 2001; Russo et al., 2005). Our impedance results also appear to be in agreement with previous reports indicating that prior pregnancy reduced the infertility and impaired fecundity levels in humans (Chandra et al., 2013) and that medical abortion affected reproductive capacity and reduced the success of subsequent pregnancies in mice (Lv et al., 2012).
Pregnancy and Oxidative Stress
Our results indicate an increased oxidative stress in the rats who carried the pregnancy to full-term (D-P+). This corresponded to a lower GSH/GSSG ratio and a higher redox potential, indicative of a lower antioxidant capacity (Schafer and Buettner, 2001; Wu et al., 2004; Maes et al., 2011; Zitka et al., 2012; Liu et al., 2015). The literature indicates that oxidative stress is associated with a negative effect on fertility (Ruder et al., 2009; Agarwal et al., 2012). However, this does not represent the full picture of oxidative stress. In our results, this is evident in the effects observed in the vaginal impedance measurements in the D-P+ group, as described above. Moreover, this appears to be consistent with research that indicates that an increase in oxidative stress is beneficial and necessary for a successful pregnancy to occur and be maintained (Jenkins et al., 2000; Wiener-Megnazi et al., 2004; Patil et al., 2007; Michel and Bonnet, 2014; Pereira et al., 2015; Mannaerts et al., 2018). While similar increases have been reported in cases of miscarriage and other pathophysiological complications of pregnancy (Al-Gubory et al., 2010; Burton and Jauniaux, 2011; Ramiro-Cortijo et al., 2016), the difference appears to be in cellular adaptations that take place in the peripheral blood, which offer protection from oxidative damage in the successful pregnancies (Jenkins et al., 2000). This could potentially reflect our biochemical observations in the serum several weeks following the pregnancy in the D-P+ group.
Drug Administration and Oxidative Stress
Our results also indicate an increased oxidative stress in the drug group (D+P-). As previously mentioned, this corresponded to a lower GSH/GSSG ratio and a higher redox potential, indicative of a lower antioxidant capacity (Schafer and Buettner, 2001; Wu et al., 2004; Maes et al., 2011; Zitka et al., 2012; Liu et al., 2015). Although these results are similar to those of the parous rats in the experiment (D-P+), it would appear that the mechanism and reason behind such changes, and thus the overall effect, are potentially different. This appears to be supported by the observations in the vaginal impedance, which showed a decrease over time in all groups except for the D-P+ group.
From a pharmacological perspective, mifepristone acts as both a progesterone and a GR antagonist (Spitz and Bardin, 1993). A resulting effect of the latter mechanism is a disinhibition of cortisol release (Bertagna et al., 1984, 1986; Laue et al., 1990; Young, 2004). Additionally, glucocorticoids have been reported to reduce glutathione peroxidase activity (McIntosh et al., 1998; Patel et al., 2002; Beytut et al., 2018). Thus, as an antagonist of glucocorticoid receptors, mifepristone may potentially have contributed to the changes we observed in oxidative balance toward a lower antioxidant capacity in the non-pregnant, drug group (D+P-). In addition, liver drug biotransformation can lead to oxidative stress (McGill et al., 2015; Du et al., 2016); however, there were no significant changes in liver redox parameters between treatment groups, including glutathione S-transferase activity (a class II biotransformation enzyme), suggesting that drug biotransformation is not likely a contributor to the observed changes in redox parameters.
Pregnancy Termination and Oxidative Stress
Given the necessity for a certain level of oxidative stress in order to maintain a pregnancy, as well as the relationship between carrying a pregnancy to full-term and fecundity (as indicated by the vaginal impedance measurements), it would appear, from our results, that the rats whose pregnancy was terminated (D+P+) are precluded from the potential benefits of pregnancy. This was potentially reflected in some of the biochemical observations in our study.
The changes in oxidative balance observed in the rats that carried their pregnancy to term (D-P+), as well as those present in the non-pregnant, drug group (D+P-) were not observed following the termination of the pregnancy (D+P+). We argue that such changes, described above, in the D-P+ group, may be indicative of potentially positive effects of carrying the pregnancy to term, while in the D+P- group, the pharmacological imbalance associated with the interaction between mifepristone and glucocorticoids may potentially be the cause of the oxidative changes observed. Thus, the absence of similarity to either pregnancy alone or drug administration alone at the biochemical level, as well as the negative behaviors observed in the pregnancy termination group (D+P+) indicate a more complex dynamic that requires more in-depth investigation of the consequences arising from and specific to the termination. Moreover, the presence of distant physiological changes relative to the stressor is supported by research showing a prolonged effect on other physiological parameters (e.g., cardiovascular change), following chronic mild stress, despite the recovery of the behavioral parameters measured (sucrose intake and activity levels) (Grippo et al., 2003).
Limitations
As with all animal models, while the findings from our study cannot be directly extrapolated to the level of the human person, they provide the possibility of objectively and ethically investigating the putative consequences of pregnancy termination at the biological, behavioral and physiological levels.
Given the novelty of this investigation, certain limitations should be taken into consideration in the interpretation of certain results, as well as for future experiments, including the absence of physiological, histological and anatomical measures related to the reproductive system (e.g., uterus, ovary, vagina) and its health. Additionally, a further limitation to consider is the inevitable lack of precise alignment of the experimental days during which the behavioral measurements (e.g., sucrose/water consumption/preference and locomotor activity) were conducted, relative to the pregnancy termination. It is possible that the absence of significance in sucrose consumption, between the D+P+ group and both the D-P- and D-P+ groups within Treatment Week may reflect a sensitivity to the necessity for more exact alignment of this particular parameter, especially given our observations relative to Pre-Treatment Week. An additional factor for consideration, and related to timing, is that due to the nature of this experiment, there is a limited capacity for adjustment.
Another potential distinction may also arise from the fact that in other studies, rats were deprived of water (and in some cases food) for extended periods of time prior to the sucrose test (Willner et al., 1992; Wang et al., 2009). This was not an option in our study, as it would have added a significant confounding variable given the potential effects of inappropriate nutrition on pregnancy.
Future Direction
Future experiments will focus on investigating the potential reversal of the behaviors observed in our study, including through the administration of antidepressants. Moreover, given the role of the hypothalamic-pituitary-adrenal axis and its significance in stress, the changes involved in pregnancy, glucocorticoid regulation, the perception of reward, the electrophysiological and electrochemical dynamics within the various brain regions potentially affected will also be investigated, as well as behaviors that could potentially be influenced by such altered dynamics, such as drug-addiction and maternal care of future litters. Additionally, similar experiments will be carried out to investigate the parameters addressed in this study following late-term surgical pregnancy termination.
Conclusion
To our knowledge, our study is the first report addressing the potential biological, behavioral and biochemical effects associated with pregnancy termination in an animal model. Additionally, the findings of this study also appear to provide additional support to the current literature pertaining to the benefits of carrying a pregnancy to full-term. Moreover, we believe that our findings support the use of this model as an objective method for the investigation of potential physical (biological and physiological) and behavioral effects of induced pregnancy termination. Our findings strongly suggest that pregnancy termination at mid-term (first-trimester human equivalent) induces significant negative biological and behavioral changes in the rat. Additionally, such a procedure appears to be associated with a potential absence of beneficial effects of carrying a pregnancy to full-term. Moreover, our findings also appear to indicate a significant difference between induced pregnancy termination (medical abortion) and natural miscarriage. Our study, therefore, indicates the importance and necessity for further objective research into the abortion procedure, including at the physiological and neurophysiological levels. Such work may further our understanding and potentially shed some clarity into the potential biobehavioral impact of such a procedure at the level of the human person.
Data Availability
Datasets are available on request. The raw data supporting the conclusions of this manuscript will be made available by the authors, without undue reservation, to any qualified researcher.
Ethics Statement
This study was carried out in accordance with the recommendations of the Guide for the Care and Use of Laboratory Animals published by the USPHS. The protocol was approved by the Franciscan University Institutional Animal Care and Use Committee (IACUC; Protocol Number. 2013-01).
Author Contributions
SS contributed to the conception, design, supervision and acquisition of funding of the study. SS, CC, and RB conducted the behavioral experiments. PA-S contributed to the design and supervision of the biochemistry aspect of the study. SS, CC, and PA-S performed the statistical analyses. SS and CC wrote the first draft of the manuscript. SS, CC, PA-S, and LP contributed to research investigation and writing of the biochemistry section of the manuscript. All authors contributed to manuscript revision, read and approved the submitted version.
Funding
This research was funded by a grant from the Watson Bowes Research Institute (https://watsonbowesresearchinstitute.org/) (SS). The funders had no role in study design, data collection and analysis, interpretation of results, decision to publish, or preparation of the manuscript.
Conflict of Interest Statement
The authors declare that the research was conducted in the absence of any commercial or financial relationships that could be construed as a potential conflict of interest.
Supplementary Material
The Supplementary Material for this article can be found online at: https://www.frontiersin.org/articles/10.3389/fnins.2019.00544/full#supplementary-material
References
Adler, N. E., David, H. P., Major, B. N., Roth, S. H., Russo, N. F., and Wyatt, G. E. (1992). Psychological factors in abortion. Am. Psychol. 47, 1194–1204. doi: 10.1037//0003-066x.47.10.1194
Agarwal, A., Aponte-Mellado, A., Premkumar, B. J., Shaman, A., and Gupta, S. (2012). The effects of oxidative stress on female reproduction: a review. Reprod. Biol. Endocrinol. 10:49. doi: 10.1186/1477-7827-10-49
Alario, P., Gamallo, A., Beato, M. J., and Trancho, G. (1987). Body weight gain, food intake and adrenal development in chronic noise stressed rats. Physiol. Behav. 40, 29–32. doi: 10.1016/0031-9384(87)90181-8
Al-Gubory, K. H., Fowler, P. A., and Garrel, C. (2010). The roles of cellular reactive oxygen species, oxidative stress and antioxidants in pregnancy outcomes. Int. J. Biochem. Cell Biol. 42, 1634–1650. doi: 10.1016/j.biocel.2010.06.001
Alzoubi, K. H., Khabour, O. F., Rashid, B. A., Damaj, I. M., and Salah, H. A. (2012). The neuroprotective effect of vitamin E on chronic sleep deprivation-induced memory impairment: the role of oxidative stress. Behav. Brain Res. 226, 205–210. doi: 10.1016/j.bbr.2011.09.017
American Psychiatric Association (2013). Diagnostic and Statistical Manual of Mental Disorders. Washington, DC: American Psychiatric Association.
American Psychological Association (2008). American Psychological Association Task Force on Mental Health and Abortion. Report of the APA Task Force on Mental Health and Abortion. Washington, DC: APA.
Ancelin, M. L., Scali, J., Norton, J., Ritchie, K., Dupuy, A. M., Chaudieu, I., et al. (2017). Heterogeneity in HPA axis dysregulation and serotonergic vulnerability to depression. Psychoneuroendocrinology 77, 90–94. doi: 10.1016/j.psyneuen.2016.11.016
Armario, A., Valles, A., Dal-Zotto, S., Marquez, C., and Belda, X. (2004). A single exposure to severe stressors causes long-term desensitisation of the physiological response to the homotypic stressor. Stress 7, 157–172. doi: 10.1080/10253890400010721
Baird, D. D., and Dunson, D. B. (2003). Why is parity protective for uterine fibroids? Epidemiology 14, 247–250. doi: 10.1097/01.EDE.0000054360.61254.27
Bartos, L. (1977). Vaginal impedance measurement used for mating in the rat. Lab. Anim. 11, 53–55. doi: 10.1258/002367777780959148
Belvederi Murri, M., Pariante, C., Mondelli, V., Masotti, M., Atti, A. R., Mellacqua, Z., et al. (2014). HPA axis and aging in depression: systematic review and meta-analysis. Psychoneuroendocrinology 41, 46–62. doi: 10.1016/j.psyneuen.2013.12.004
Bertagna, X., Bertagna, C., Laudat, M. H., Husson, J. M., Girard, F., and Luton, J. P. (1986). Pituitary-adrenal response to the antiglucocorticoid action of RU 486 in cushing’s syndrome. J. Clin. Endocrinol. Metab. 63, 639–643. doi: 10.1210/jcem-63-3-639
Bertagna, X., Bertagna, C., Luton, J. P., Husson, J. M., and Girard, F. (1984). The new steroid analog RU 486 inhibits glucocorticoid action in man. J. Clin. Endocrinol. Metab. 59, 25–28. doi: 10.1210/jcem-59-1-25
Bertiere, M. C., Sy, T. M., Baigts, F., Mandenoff, A., and Apfelbaum, M. (1984). Stress and sucrose hyperphagia: role of endogenous opiates. Pharmacol. Biochem. Behav. 20, 675–679. doi: 10.1016/0091-3057(84)90183-7
Beytut, E., Yilmaz, S., Aksakal, M., and Polat, S. (2018). The possible protective effects of vitamin E and selenium administration in oxidative stress caused by high doses of glucocorticoid administration in the brain of rats. J. Trace Elem. Med. Biol. 45, 131–135. doi: 10.1016/j.jtemb.2017.10.005
Biggs, M. A., Neuhaus, J. M., and Foster, D. G. (2015). Mental health diagnoses 3 years after receiving or being denied an abortion in the united states. Am. J. Public Health 105, 2557–2563. doi: 10.2105/AJPH.2015.302803
Biggs, M. A., Rowland, B., McCulloch, C. E., and Foster, D. G. (2016a). Does abortion increase women’s risk for post-traumatic stress? Findings from a prospective longitudinal cohort study. BMJ Open 6:e009698. doi: 10.1136/bmjopen-2015-009698
Biggs, M. A., Upadhyay, U. D., McCulloch, C. E., and Foster, D. G. (2016b). Women’s mental health and well-being 5 years after receiving or being denied an abortion: a prospective, longitudinal cohort study. JAMA Psychiatry 74, 169–178. doi: 10.1001/jamapsychiatry.2016.3478
Bogdanova, O. V., Kanekar, S., D’Anci, K. E., and Renshaw, P. F. (2013). Factors influencing behavior in the forced swim test. Physiol. Behav. 118, 227–239. doi: 10.1016/j.physbeh.2013.05.012
Bourke, C. H., and Neigh, G. N. (2011). Behavioral effects of chronic adolescent stress are sustained and sexually dimorphic. Horm. Behav. 60, 112–120. doi: 10.1016/j.yhbeh.2011.03.011
Brown, K. J., and Grunberg, N. E. (1996). Effects of environmental conditions on food consumption in female and male rats. Physiol. Behav. 60, 293–297. doi: 10.1016/0031-9384(96)00020-0
Burton, G. J., and Jauniaux, E. (2011). Oxidative stress. Best Pract. Res. Clin. Obstet. Gynaecol. 25, 287–299. doi: 10.1016/j.bpobgyn.2010.10.016
Cabrol, D., Carbonne, B., Bienkiewicz, A., Dallot, E., Alj, A. E., and Cedard, L. (1991). Induction of labor and cervical maturation using mifepristone (RU 486) in the late pregnant rat. Influence of a cyclooxygenase inhibitor (Diclofenac). Prostaglandins 42, 71–79. doi: 10.1016/0090-6980(91)90095-w
Cartwright, M., Wardle, J., Steggles, N., Simon, A. E., Croker, H., and Jarvis, M. J. (2003). Stress and dietary practices in adolescents. Health Psychol. 22, 362–369. doi: 10.1037/0278-6133.22.4.362
Castagné, V., Moser, P., Roux, S., and Porsolt, R. D. (2010). “Rodent models of depression: forced swim and tail suspension behavioral despair tests in rats and mice,” in Current Protocols in Neuroscience, ed. J. Crawley (Hoboken, NY: John Wiley & Sons, Inc.).
Cervo, L., Grignaschi, G., and Samanin, R. (1988). 8-Hydroxy-2-(di-n-propylamino)tetralin, a selective serotonin1A receptor agonist, reduces the immobility of rats in the forced swimming test by acting on the nucleus raphe dorsalis. Eur. J. Pharmacol. 158, 53–59. doi: 10.1016/0014-2999(88)90252-X
Chandra, A., Copen, C. E., and Stephen, E. H. (2013). Infertility and impaired fecundity in the United States, 1982-2010: data from the national survey of family Growth. Natl. Health Stat. Rep. 67, 1–18.
Coleman, P. K. (2011). Abortion and mental health: quantitative synthesis and analysis of research published 1995-2009. Br. J. Psychiatry 199, 180–186. doi: 10.1192/bjp.bp.110.077230
Coleman, P. K., Reardon, D. C., and Calhoun, B. C. (2012). ). Reproductive history patterns and long-term mortality rates: a danish, population-based record linkage study. Eur. J. Public Health. 23, 569–574. doi: 10.1093/eurpub/cks107
Cougle, J. R., Reardon, D. C., and Coleman, P. K. (2003). Depression associated with abortion and childbirth: a long-term analysis of the NLSY cohort. Med. Sci. Monit. 9, CR105–CR112.
Crosby, A. E., Han, B., Ortega, L. A., Parks, S. E., and Gfroerer, J. (2011). Suicidal thoughts and behaviors among adults aged ≥ 18 years–United States, 2008-2009. MMWR Surveill. Summ. 60, 1–22.
Crumeyrolle-Arias, M., Jaglin, M., Bruneau, A., Vancassel, S., Cardona, A., Dauge, V., et al. (2014). Absence of the gut microbiota enhances anxiety-like behavior and neuroendocrine response to acute stress in rats. Psychoneuroendocrinology 42, 207–217. doi: 10.1016/j.psyneuen.2014.01.014
Cryan, J. F., Markou, A., and Lucki, I. (2002). Assessing antidepressant activity in rodents: recent developments and future needs. Trends Pharmacol. Sci. 23, 238–245. doi: 10.1016/s0165-6147(02)02017-5
Dallman, M. F., Pecoraro, N., Akana, S. F., La Fleur, S. E., Gomez, F., Houshyar, H., et al. (2003). Chronic stress and obesity: a new view of “comfort food”. Proc. Natl. Acad. Sci. U.S.A. 100, 11696–11701. doi: 10.1073/pnas.1934666100
Detke, M. J., and Lucki, I. (1995). Detection of serotonergic and noradrenergic antidepressants in the rat forced swimming test: the effects of water depth. Behav. Brain Res. 73, 43–46. doi: 10.1016/0166-4328(96)00067-8
Dewar, A. D. (1964). The nature of the weight gain induced by progesterone in mice. Q. J. Exp. Physiol. Cogn. Med. Sci. 49, 151–161. doi: 10.1113/expphysiol.1964.sp001715
Dingle, K., Alati, R., Clavarino, A., Najman, J. M., and Williams, G. M. (2008). Pregnancy loss and psychiatric disorders in young women: an Australian birth cohort study. Br. J. Psychiatry 193, 455–460. doi: 10.1192/bjp.bp.108.055079
Du, K., Ramachandran, A., and Jaeschke, H. (2016). Oxidative stress during acetaminophen hepatotoxicity: sources, pathophysiological role and therapeutic potential. Redox Biol. 10, 148–156. doi: 10.1016/j.redox.2016.10.001
Duthie, L., and Reynolds, R. M. (2013). Changes in the maternal hypothalamic-pituitary-adrenal axis in pregnancy and postpartum: influences on maternal and fetal outcomes. Neuroendocrinology 98, 106–115. doi: 10.1159/000354702
Fergusson, D. M., Horwood, L. J., and Boden, J. M. (2008). Abortion and mental health disorders: evidence from a 30-year longitudinal study. Br. J. Psychiatry 193, 444–451. doi: 10.1192/bjp.bp.108.056499
Fergusson, D. M., John Horwood, L., and Ridder, E. M. (2006). Abortion in young women and subsequent mental health. J. Child Psychol. Psychiatry 47, 16–24. doi: 10.1111/j.1469-7610.2005.01538.x
Fiala, C., and Danielsson, K.-G. (2006). Review of medical abortion using mifepristone in combination with a prostaglandin analogue. Contraception 74, 66–86. doi: 10.1016/j.contraception.2006.03.018
Field, K. J., White, W. J., and Lang, C. M. (1993). Anaesthetic effects of chloral hydrate, pentobarbitone and urethane in adult male rats. Lab. Anim. 27, 258–269. doi: 10.1258/002367793780745471
Finer, L. B., and Zolna, M. R. (2016). Declines in unintended pregnancy in the united states, 2008-2011. N. Engl. J. Med. 374, 843–852. doi: 10.1056/NEJMsa1506575
Foster, D. G., Steinberg, J. R., Roberts, S. C., Neuhaus, J., and Biggs, M. A. (2015). A comparison of depression and anxiety symptom trajectories between women who had an abortion and women denied one. Psychol. Med. 45, 2073–2082. doi: 10.1017/S0033291714003213
Gorka, Z., Moryl, E., and Papp, M. (1996). Effect of chronic mild stress on circadian rhythms in the locomotor activity in rats. Pharmacol. Biochem. Behav. 54, 229–234. doi: 10.1016/0091-3057(95)02173-6
Griffith, O. W. (1980). Determination of glutathione and glutathione disulfide using glutathione reductase and 2-vinylpyridine. Anal. Biochem. 106, 207–212. doi: 10.1016/0003-2697(80)90139-6
Grippo, A. J., Beltz, T. G., and Johnson, A. K. (2003). Behavioral and cardiovascular changes in the chronic mild stress model of depression. Physiol. Behav. 78, 703–710. doi: 10.1016/s0031-9384(03)00050-7
Grippo, A. J., Beltz, T. G., Weiss, R. M., and Johnson, A. K. (2006). The effects of chronic fluoxetine treatment on chronic mild stress-induced cardiovascular changes and anhedonia. Biol. Psychiatry 59, 309–316. doi: 10.1016/j.biopsych.2005.07.010
Grønli, J., Murison, R., Fiske, E., Bjorvatn, B., Sørensen, E., Portas, C. M., et al. (2005). Effects of chronic mild stress on sexual behavior, locomotor activity and consumption of sucrose and saccharine solutions. Physiol. Behav. 84, 571–577. doi: 10.1016/j.physbeh.2005.02.007
Habig, W. H., Pabst, M. J., and Jakoby, W. B. (1974). Glutathione S-transferases. The first enzymatic step in mercapturic acid formation. J. Biol. Chem. 249, 7130–7139.
Haidkind, R., Eller, M., Harro, M., Kask, A., Rinken, A., Oreland, L., et al. (2003). Effects of partial locus coeruleus denervation and chronic mild stress on behaviour and monoamine neurochemistry in the rat. Eur. Neuropsychopharmacol. 13, 19–28. doi: 10.1016/s0924-977x(02)00076-7
Halliwell, B., and Chirico, S. (1993). Lipid peroxidation: its mechanism, measurement, and significance. Am. J. Clin. Nutr. 57, 715S–724S. doi: 10.1093/ajcn/57.5.715S
Hamoda, H., and Templeton, A. (2010). Medical and surgical options for induced abortion in first trimester. Best Pract. Res. Clin. Obstet. Gynaecol. 24, 503–516. doi: 10.1016/j.bpobgyn.2010.02.006
Harris, R. B. (2015). Chronic and acute effects of stress on energy balance: are there appropriate animal models? Am. J. Physiol. Regul. Integr. Comp. Physiol. 308, R250–R265. doi: 10.1152/ajpregu.00361.2014
Harris, R. B., Mitchell, T. D., Simpson, J., Redmann, S. M., Youngblood, B. D., and Ryan, D. H. (2002). Weight loss in rats exposed to repeated acute restraint stress is independent of energy or leptin status. Am. J. Physiol. Regul. Integr. Comp. Physiol. 282, R77–R88. doi: 10.1152/ajpregu.2002.282.1.R77
Harro, J., Tonissaar, M., Eller, M., Kask, A., and Oreland, L. (2001). Chronic variable stress and partial 5-HT denervation by parachloroamphetamine treatment in the rat: effects on behavior and monoamine neurochemistry. Brain Res. 899, 227–239. doi: 10.1016/s0006-8993(01)02256-9
Hayase, T. (2011). Depression-related anhedonic behaviors caused by immobilization stress: a comparison with nicotine-induced depression-like behavioral alterations and effects of nicotine and/or “antidepressant” drugs. J. Toxicol. Sci. 36, 31–41. doi: 10.2131/jts.36.31
He, Q.-J., Yang, B., Wang, W.-F., Wu, H.-H., and Fang, R.-Y. (2003). Synergistic effects of DL111-IT in combination with mifepristone and misoprostol on termination of early pregnancy in preclinical studies. Contraception 68, 289–295. doi: 10.1016/s0010-7824(03)00179-3
Hill, C. C., and Pickinpaugh, J. (2008). Physiologic changes in pregnancy. Surg. Clin. N. Am. 88, 391–401. doi: 10.1016/j.suc.2007.12.005
Hill, M. A. (2019). Embryology Carnegie Stage Comparison. Available at: https://embryology.med.unsw.edu.au/embryology/index.php/Carnegie_Stage_Comparison (accessed April 24, 2019).
Hockey, C. D., Norman, S. T., Morton, J. M., Boothby, D., Phillips, N. J., and McGowan, M. R. (2010). Use of vaginal electrical resistance to diagnose oestrus, dioestrus and early pregnancy in synchronized tropically adapted beef heifers. Reprod. Domest. Anim. 45, 629–636. doi: 10.1111/j.1439-0531.2008.01320.x
Hooks, M. S., Jones, G. H., Smith, A. D., Neill, D. B., and Justice, J. B. (1991). Individual differences in locomotor activity and sensitization. Pharmacol. Biochem. Behav. 38, 467–470. doi: 10.1016/0091-3057(91)90308-o
Horowitz, J. M., Kristal, M. B., and Torres, G. (1997). Differential behavioral responses to cocaethylene of long-evans and sprague-dawley rats: role of serotonin. Synapse 26, 11–21. doi: 10.1002/(sici)1098-2396(199705)26:1<11::aid-syn2>3.0.co;2-h
Hu, P., Oomen, C., van Dam, A. M., Wester, J., Zhou, J. N., Joels, M., et al. (2012). A single-day treatment with mifepristone is sufficient to normalize chronic glucocorticoid induced suppression of hippocampal cell proliferation. PLoS One 7:e46224. doi: 10.1371/journal.pone.0046224
Huang, Y., Zhang, X., Li, W., Song, F., Dai, H., Wang, J., et al. (2013). A meta-analysis of the association between induced abortion and breast cancer risk among Chinese females. Cancer Causes Control 25, 227–236. doi: 10.1007/s10552-013-0325-7
Huang, Z., Hao, J., Su, P., Huang, K., Xing, X., Cheng, D., et al. (2012). The impact of prior abortion on anxiety and depression symptoms during a subsequent pregnancy: data from a population-based cohort study in China. Bull. Clin. Psychopharmacol. 22, 51–58. doi: 10.5455/bcp.20111102040509
Ishikawa, I., Kitamura, H., Kimura, K., and Saito, M. (2001). Brain interleukin-1 is involved in blood interleukin-6 response to immobilization stress in rats. Jpn. J. Vet. Res. 49, 19–25.
Jenkins, C., Wilson, R., Roberts, J., Miller, H., McKillop, J. H., and Walker, J. J. (2000). Antioxidants: their role in pregnancy and miscarriage. Antioxid. Redox Signal. 2, 623–628. doi: 10.1089/15230860050192369
Jones, R. K., and Kooistra, K. (2011). Abortion incidence and access to services in the United States, 2008. Perspect. Sex. Reprod. health 43, 41–50. doi: 10.1363/4304111
Juruena, M. F. (2014). Early-life stress and HPA axis trigger recurrent adulthood depression. Epilepsy Behav. 38, 148–159. doi: 10.1016/j.yebeh.2013.10.020
Katz, R. J. (1981). Animal models and human depressive disorders. Neurosci. Biobehav. Rev. 5, 231–246. doi: 10.1016/0149-7634(81)90004-x
Kennedy, G. C., and Mitra, J. (1963). Spontaneous pseudopregnancy and obesity in the rat. J. Physiol. 166, 419–424. doi: 10.1113/jphysiol.1963.sp007113
Kivimaki, M., Head, J., Ferrie, J. E., Shipley, M. J., Brunner, E., Vahtera, J., et al. (2006). Work stress, weight gain and weight loss: evidence for bidirectional effects of job strain on body mass index in the whitehall II study. Int. J. Obes. 30, 982–987. doi: 10.1038/sj.ijo.0803229
Konttinen, H., Mannisto, S., Sarlio-Lahteenkorva, S., Silventoinen, K., and Haukkala, A. (2010). Emotional eating, depressive symptoms and self-reported food consumption. A population-based study. Appetite 54, 473–479. doi: 10.1016/j.appet.2010.01.014
Korkeila, M., Kaprio, J., Rissanen, A., Koskenvuo, M., and Sörensen, T. I. A. (1998). Predictors of major weight gain in adult finns: stress, life satisfaction and personality traits. Int. J. Obes. 22, 949–957. doi: 10.1038/sj.ijo.0800694
Krebs, H., Macht, M., Weyers, P., Weijers, H. G., and Janke, W. (1996). Effects of stressful noise on eating and non-eating behavior in rats. Appetite 26, 193–202. doi: 10.1006/appe.1996.0015
Kumar, B., Kuhad, A., and Chopra, K. (2011). Neuropsychopharmacological effect of sesamol in unpredictable chronic mild stress model of depression: behavioral and biochemical evidences. Psychopharmacology 214, 819–828. doi: 10.1007/s00213-010-2094-2
Kupfer, D. J., Frank, E., and Phillips, M. L. (2012). Major depressive disorder: new clinical, neurobiological, and treatment perspectives. Lancet 379, 1045–1055. doi: 10.1016/S0140-6736(11)60602-8
Laakso, M. L., Leinonen, L., Joutsiniemi, S. L., Porkka-Heiskanen, T., and Alila, A. (1995). Locomotor activity and melatonin rhythms in rats under non-24-h lighting cycles. Physiol. Behav. 57, 849–856. doi: 10.1016/0031-9384(94)00311-r
Lanfranchi, A. (2005). The science, studies and sociology of the abortion breast cancer link. Issues Law Med. 21, 95–108.
Lanfranchi, A. (2014). Normal breast physiology: the reasons hormonal contraceptives and induced abortion increase breast-cancer risk. Issues Law Med. 29, 135–146.
Lanfranchi, A., and Fagan, P. (2014a). Induced Abortion and Breast Cancer. Washington, D.C: Marriage & Religion Research Institute.
Lanfranchi, A. E., and Fagan, P. (2014b). Breast cancer and induced abortion: a comprehensive review of breast development and pathophysiology, the epidemiologic literature, and proposal for creation of databanks to elucidate all breast cancer risk factors. Issues Law Med. 29, 3–133.
Lau, E. X., and Rapee, R. M. (2011). Prevention of anxiety disorders. Curr. Psychiatr. Rep. 13, 258–266. doi: 10.1007/s11920-011-0199-x
Laue, L., Lotze, M. T., Chrousos, G. P., Barnes, K., Loriaux, D. L., and Fleisher, T. A. (1990). Effect of chronic treatment with the glucocorticoid antagonist RU 486 in man: toxicity, immunological, and hormonal aspects. J. Clin. Endocrinol. Metab. 71, 1474–1480. doi: 10.1210/jcem-71-6-1474
Lee, V. C. Y., Ng, E. H. Y., and Ho, P. C. (2010). Issues in second trimester induced abortion (medical/surgical methods). Best Pract. Res. Clin. Obstet. Gynaecol. 24, 517–527. doi: 10.1016/j.bpobgyn.2010.02.008
Li, H. Z., Sun, X., Stavreus-Evers, A., and Gemzell-Danielsson, K. (2004). Effect of mifepristone on the expression of cytokines in the human Fallopian tube. Mol. Hum. Reprod. 10, 489–493. doi: 10.1093/molehr/gah063
Lindsay, J. R., and Nieman, L. K. (2005). The hypothalamic-pituitary-adrenal axis in pregnancy: challenges in disease detection and treatment. Endocrine Rev. 26, 775–799. doi: 10.1210/er.2004-0025
Liu, T., Zhong, S., Liao, X., Chen, J., He, T., Lai, S., et al. (2015). A meta-analysis of oxidative stress markers in depression. PLoS One 10:e0138904. doi: 10.1371/journal.pone.0138904
Luno, V., Gil, L., Jerez, R. A., Malo, C., Gonzalez, N., Grandia, J., et al. (2013). Determination of ovulation time in sows based on skin temperature and genital electrical resistance changes. Vet. Rec. 172:579. doi: 10.1136/vr.101221
Luo, M., Jiang, X., Wang, Y., Wang, Z., Shen, Q., Li, R., et al. (2018). Association between induced abortion and suicidal ideation among unmarried female migrant workers in three metropolitan cities in China: a cross-sectional study. BMC Public Health 18:625. doi: 10.1186/s12889-018-5527-1
Lushchak, V. I. (2012). Glutathione homeostasis and functions: potential targets for medical interventions. J. Amino Acids 2012:736837. doi: 10.1155/2012/736837
Lv, F., Xu, X., Zhang, S., Wang, L., Wang, N., He, B., et al. (2012). Repeated abortion affects subsequent pregnancy outcomes in BALB/c mice. PLoS One 7:e48384. doi: 10.1371/journal.pone.0048384
Maddalena, A., Tereshchenko, J., Bahr, M., and Kugler, S. (2013). Adeno-associated virus-mediated, mifepristone-regulated transgene expression in the brain. Mol. Ther. Nucleic Acids 2:e106. doi: 10.1038/mtna.2013.35
Maes, M., Galecki, P., Chang, Y. S., and Berk, M. (2011). A review on the oxidative and nitrosative stress (O&NS) pathways in major depression and their possible contribution to the (neuro)degenerative processes in that illness. Prog. Neuropsychopharmacol. Biol. Psychiatry 35, 676–692. doi: 10.1016/j.pnpbp.2010.05.004
Major, B., Appelbaum, M., Beckman, L., Dutton, M. A., Russo, N. F., and West, C. (2009). Abortion and mental health: evaluating the evidence. Am. Psychol. 64, 863–890. doi: 10.1037/a0017497
Mannaerts, D., Faes, E., Cos, P., Briede, J. J., Gyselaers, W., Cornette, J., et al. (2018). Oxidative stress in healthy pregnancy and preeclampsia is linked to chronic inflammation, iron status and vascular function. PLoS One 13:e0202919. doi: 10.1371/journal.pone.0202919
Marti, O., Marti, J., and Armario, A. (1994). Effects of chronic stress on food intake in rats: influence of stressor intensity and duration of daily exposure. Physiol. Behav. 55, 747–753. doi: 10.1016/0031-9384(94)90055-8
McGill, M. R., Du, K., Weemhoff, J. L., and Jaeschke, H. (2015). Critical review of resveratrol in xenobiotic-induced hepatotoxicity. Food Chem. Toxicol. 86, 309–318. doi: 10.1016/j.fct.2015.11.003
McIntosh, L. J., Hong, K. E., and Sapolsky, R. M. (1998). Glucocorticoids may alter antioxidant enzyme capacity in the brain: baseline studies. Brain Res. 791, 209–214. doi: 10.1016/s0006-8993(98)00115-2
Michel, C. L., and Bonnet, X. (2014). Effect of a brief stress on progesterone plasma levels in pregnant and non-pregnant guinea pigs. Animal Biol. 64, 19–29. doi: 10.1163/15707563-00002428
Mikolajczyk, R. T., El Ansari, W., and Maxwell, A. E. (2009). Food consumption frequency and perceived stress and depressive symptoms among students in three European countries. Nutr. J. 8:31. doi: 10.1186/1475-2891-8-31
Monteiro, F., Abraham, M. E., Sahakari, S. D., and Mascarenhas, J. F. (1989). Effect of immobilization stress on food intake, body weight and weights of various organs in rat. Ind. J. Physiol. Pharmac. 33, 186–190.
Mota, N. P., Burnett, M., and Sareen, J. (2010). Associations between abortion, mental disorders, and suicidal behaviour in a nationally representative sample. Can. J. Psychiatry 55, 239–247. doi: 10.1177/070674371005500407
Munk-Olsen, T., Laursen, T. M., Pedersen, C. B., Lidegaard,Ø, and Mortensen, P. B. (2011). Induced first-trimester abortion and risk of mental disorder. N. Engl. J. Med. 364, 332–339. doi: 10.1056/NEJMoa0905882
Muscat, R., and Willner, P. (1992). Suppression of sucrose drinking by chronic mild unpredictable stress: a methodological analysis. Neurosci. Biobehav. Rev. 16, 507–517. doi: 10.1016/s0149-7634(05)80192-7
Naieni, K. H., Ardalan, A., Mahmoodi, M., Motevalian, A., Yahyapoor, Y., and Yazdizadeh, B. (2007). Risk factors of breast cancer in north of iran: a case-control in mazandaran province. Asian Pac. J. Cancer Prev. 8, 395–398.
Navarre, B. M., Laggart, J. D., and Craft, R. M. (2010). Anhedonia in postpartum rats. Physiol. Behav. 99, 59–66. doi: 10.1016/j.physbeh.2009.10.011
Nguyen, K. T., Deak, T., Owens, S. M., Kohno, T., Fleshner, M., Watkins, L. R., et al. (1998). Exposure to acute stress induces brain interleukin-1beta protein in the rat. J. Neurosci. 18, 2239–2246. doi: 10.1523/jneurosci.18-06-02239.1998
Ohkawa, H., Ohishi, N., and Yagi, K. (1979). Assay for lipid peroxides in animal tissues by thiobarbituric acid reaction. Anal. Biochem. 95, 351–358. doi: 10.1016/0003-2697(79)90738-3
O’Rahilly, R. (1979). Early human development and the chief sources of information on staged human embryos. Eur. J. Obstet. Gynecol. Reprod. Biol. 9, 273–280. doi: 10.1016/0028-2243(79)90068-6
Papp, M., Willner, P., and Muscat, R. (1991). An animal model of anhedonia: attenuation of sucrose consumption and place preference conditioning by chronic unpredictable mild stress. Psychopharmacology 104, 255–259. doi: 10.1007/bf02244188
Pariante, C. M., and Lightman, S. L. (2008). The HPA axis in major depression: classical theories and new developments. Trends Neurosci. 31, 464–468. doi: 10.1016/j.tins.2008.06.006
Patel, R., McIntosh, L., McLaughlin, J., Brooke, S., Nimon, V., and Sapolsky, R. (2002). Disruptive effects of glucocorticoids on glutathione peroxidase biochemistry in hippocampal cultures. J. Neurochem. 82, 118–125. doi: 10.1046/j.1471-4159.2002.00948.x
Patil, S. B., Kodliwadmath, M. V., and Kodliwadmath, S. M. (2007). Study of oxidative stress and enzymatic antioxidants in normal pregnancy. Indian J. Clin. Biochem. 22, 135–137. doi: 10.1007/BF02912897
Pedersen, W. (2008). Abortion and depression: a population-based longitudinal study of young women. Scand. J. Public Health 36, 424–428. doi: 10.1177/1403494807088449
Pereira, R. D., De Long, N. E., Wang, R. C., Yazdi, F. T., Holloway, A. C., and Raha, S. (2015). Angiogenesis in the placenta: the role of reactive oxygen species signaling. Biomed. Res. Int. 2015:814543. doi: 10.1155/2015/814543
Porsolt, R. D., Anton, G., Blavet, N., and Jalfre, M. (1978a). Behavioural despair in rats: a new model sensitive to antidepressant treatments. Eur. J. Pharmacol. 47, 379–391. doi: 10.1016/0014-2999(78)90118-8
Porsolt, R. D., Bertin, A., and Jalfre, M. (1978b). “Behavioural despair” in rats and mice: strain differences and the effects of imipramine. Eur. J. Pharmacol. 51, 291–294. doi: 10.1016/0014-2999(78)90414-4
Porsolt, R. D., Le Pichon, M., and Jalfre, M. (1977). Depression: a new animal model sensitive to antidepressant treatments. Nature 266, 730–732. doi: 10.1038/266730a0
Radestad, A., Bygdeman, M., and Green, K. (1990). Induced cervical ripening with mifepristone (RU 486) and bioconversion of arachidonic acid in human pregnant uterine cervix in the first trimester. A double-blind, randomized, biomechanical and biochemical study. Contraception 41, 283–292. doi: 10.1016/0010-7824(90)90069-8
Rajkumar, L., Guzman, R. C., Yang, J., Thordarson, G., Talamantes, F., and Nandi, S. (2001). Short-term exposure to pregnancy levels of estrogen prevents mammary carcinogenesis. Proc. Natl. Acad. Sci. U.S.A. 98, 11755–11759. doi: 10.1073/pnas.201393798
Ramiro-Cortijo, D., Herrera, T., Rodriguez-Rodriguez, P., Lopez De Pablo, A. L., De La Calle, M., Lopez-Gimenez, M. R., et al. (2016). Maternal plasma antioxidant status in the first trimester of pregnancy and development of obstetric complications. Placenta 47, 37–45. doi: 10.1016/j.placenta.2016.08.090
Reagan-Shaw, S., Nihal, M., and Ahmad, N. (2008). Dose translation from animal to human studies revisited. FASEB J. 22, 659–661. doi: 10.1096/fj.07-9574LSF
Reardon, D. C. (2014). Abortion and mental health: findings from the national comorbidity survey-replication. Obstet. Gynecol. 123, 1354–1355. doi: 10.1097/AOG.0000000000000306
Reardon, D. C. (2018). The abortion and mental health controversy: a comprehensive literature review of common ground agreements, disagreements, actionable recommendations, and research opportunities. SAGE Open Med. 6:2050312118807624. doi: 10.1177/2050312118807624
Reardon, D. C., Coleman, P. K., and Cougle, J. R. (2004a). Substance use associated with unintended pregnancy outcomes in the national longitudinal survey of youth. Am. J. Drug Alcohol Abuse 30, 369–383. doi: 10.1081/Ada-120037383
Reardon, D. C., Strahan, T. W., Thorp, J. M., and Shuping, M. W. (2004b). Deaths associated with abortion compared to childbirth–a review of new and old data and the medical and legal implications. J. Contemp. Health Law Policy 20, 279–327.
Reardon, D. C., and Cougle, J. R. (2002). Depression and unintended pregnancy in the national longitudinal survey of youth: a cohort study. BMJ 324, 151–152. doi: 10.1136/bmj.324.7330.151
Rees, D. I., and Sabia, J. J. (2007). The Relationship Between Abortion and Depression : New Evidence from the Fragile Families and Child Wellbeing Study. Albertson, NY: International Scientific Literature.
Rezac, P. (2008). Potential applications of electrical impedance techniques in female mammalian reproduction. Theriogenology 70, 1–14. doi: 10.1016/j.theriogenology.2008.03.001
Robinson, G. E., Stotland, N. L., Russo, N. F., Lang, J. A., and Occhiogrosso, M. (2009). Is there an abortion trauma syndrome? Critiquing the evidence. Harv. Rev. Psychiatry 17, 268–290. doi: 10.1080/10673220903149119
Rossetti, A. C., Papp, M., Gruca, P., Paladini, M. S., Racagni, G., Riva, M. A., et al. (2016). Stress-induced anhedonia is associated with the activation of the inflammatory system in the rat brain: restorative effect of pharmacological intervention. Pharmacol. Res. 103, 1–12. doi: 10.1016/j.phrs.2015.10.022
Ruder, E. H., Hartman, T. J., and Goldman, M. B. (2009). Impact of oxidative stress on female fertility. Curr. Opin. Obstet. Gynecol. 21, 219–222. doi: 10.1097/gco.0b013e32832924ba
Russo, J., Moral, R., Balogh, G. A., Mailo, D., and Russo, I. H. (2005). The protective role of pregnancy in breast cancer. Breast Cancer Res. 7, 131–142. doi: 10.1186/bcr1029
Russo, J., and Russo, I. H. (1980). Susceptibility of the mammary gland to carcinogenesis. II. pregnancy interruption as a risk factor in tumor incidence. Am. J. Pathol. 100, 497–512.
Sarkaki, A., Farbood, Y., Badavi, M., Khalaj, L., Khodagholi, F., and Ashabi, G. (2015). Metformin improves anxiety-like behaviors through AMPK-dependent regulation of autophagy following transient forebrain ischemia. Metab. Brain Dis. 30, 1139–1150. doi: 10.1007/s11011-015-9677-x
Schafer, F. Q., and Buettner, G. R. (2001). Redox environment of the cell as viewed through the redox state of the glutathione disulfide/glutathione couple. Free Radic. Biol. Med. 30, 1191–1212. doi: 10.1016/S0891-5849(01)00480-4
Schiavone, S., Jaquet, V., Trabace, L., and Krause, K. H. (2013). Severe life stress and oxidative stress in the brain: from animal models to human pathology. Antioxid. Redox Signal. 18, 1475–1490. doi: 10.1089/ars.2012.4720
Sestakova, N., Puzserova, A., Kluknavsky, M., and Bernatova, I. (2013). Determination of motor activity and anxiety-related behaviour in rodents: methodological aspects and role of nitric oxide. Interdiscip. Toxicol. 6, 126–135. doi: 10.2478/intox-2013-0020
Shapira-Lichter, I., Beilin, B., Ofek, K., Bessler, H., Gruberger, M., Shavit, Y., et al. (2008). Cytokines and cholinergic signals co-modulate surgical stress-induced changes in mood and memory. Brain Behav. Immun. 22, 388–398. doi: 10.1016/j.bbi.2007.09.006
Singletary, S. J., Kirsch, A. J., Watson, J., Karim, B. O., Huso, D. L., Hurn, P. D., et al. (2005). Lack of correlation of vaginal impedance measurements with hormone levels in the rat. Contemp. Top. Lab. Anim. Sci. 44, 37–42.
Sinha, D. K., Pazik, J. E., and Dao, T. L. (1988). Prevention of mammary carcinogenesis in rats by pregnancy: effect of full-term and interrupted pregnancy. Br. J. Cancer 57, 390–394. doi: 10.1038/bjc.1988.88
Slattery, D. A., and Cryan, J. F. (2012). Using the rat forced swim test to assess antidepressant-like activity in rodents. Nat. Protoc. 7, 1009–1014. doi: 10.1038/nprot.2012.044
Spitz, I. M., and Bardin, C. W. (1993). Mifepristone (RU 486)–a modulator of progestin and glucocorticoid action. N. Engl. J. Med. 329, 404–412. doi: 10.1056/nejm199308053290607
Steptoe, A., Hamer, M., and Chida, Y. (2007). The effects of acute psychological stress on circulating inflammatory factors in humans: a review and meta-analysis. Brain Behav. Immun. 21, 901–912. doi: 10.1016/j.bbi.2007.03.011
Stinson, E. J., Krakoff, J., and Gluck, M. E. (2018). Depressive symptoms and poorer performance on the stroop task are associated with weight gain. Physiol. Behav. 186, 25–30. doi: 10.1016/j.physbeh.2018.01.005
Taradach, C. (1982). Monitoring of the oestrus cycle in the rat by measurement of vaginal impedance. Arch. Toxicol. Suppl. 5, 184–186. doi: 10.1007/978-3-642-68511-8_32
Thorp, J. M., Hartmann, K. E., and Shadigan, E. (2005). Long-term physical and psychological health consequences of induced abortion: a review of the evidence. Linacre Q. 72, 44–69. doi: 10.1080/20508549.2005.11877742
Tietze, F. (1969). Enzymic method for quantitative determination of nanogram amounts of total and oxidized glutathione: applications to mammalian blood and other tissues. Anal. Biochem. 27, 502–522. doi: 10.1016/0003-2697(69)90064-5
Torres, S. J., and Nowson, C. A. (2007). Relationship between stress, eating behavior, and obesity. Nutrition 23, 887–894. doi: 10.1016/j.nut.2007.08.008
Tsoi, W. F., Cheng, M. C., Vengadasalam, D., and Seng, K. M. (1976). Psychological effects of abortion (a study of 1739 cases). Singapore Med. J. 17, 68–73.
Valles, A., Marti, O., Garcia, A., and Armario, A. (2000). Single exposure to stressors causes long-lasting, stress-dependent reduction of food intake in rats. Am. J. Physiol. Regul. Integr. Comp. Physiol. 279, R1138–R1144. doi: 10.1152/ajpregu.2000.279.3.R1138
van Ditzhuijzen, J., Ten Have, M., de Graaf, R., van Nijnatten, C., and Vollebergh, W. A. M. (2018). Long-term incidence and recurrence of common mental disorders after abortion. A dutch prospective cohort study. J. Psychiatr. Res. 102, 132–135. doi: 10.1016/j.jpsychires.2018.04.001
Walker, C. L., Cesen-Cummings, K., Houle, C., Baird, D., Barrett, J. C., and Davis, B. (2001). Protective effect of pregnancy for development of uterine leiomyoma. Carcinogenesis 22, 2049–2052. doi: 10.1093/carcin/22.12.2049
Wang, D., Lin, W., Pan, Y., Kuang, X., Qi, X., and Sun, H. (2011). Chronic blockade of glucocorticoid receptors by RU486 enhances lipopolysaccharide-induced depressive-like behaviour and cytokine production in rats. Brain Behav. Immun. 25, 706–714. doi: 10.1016/j.bbi.2011.01.011
Wang, S. H., Zhang, Z. J., Guo, Y. J., Zhou, H., Teng, G. J., and Chen, B. A. (2009). Anhedonia and activity deficits in rats: impact of post-stroke depression. J. Psychopharmacol. 23, 295–304. doi: 10.1177/0269881108089814
Warren, J. T., Harvey, S. M., and Henderson, J. T. (2010). Do depression and low self-esteem follow abortion among adolescents? Evidence from a national study. Perspect. Sex. Reprod. Health 42, 230–235. doi: 10.1363/4223010
Wiener-Megnazi, Z., Vardi, L., Lissak, A., Shnizer, S., Reznick, A. Z., Ishai, D., et al. (2004). Oxidative stress indices in follicular fluid as measured by the thermochemiluminescence assay correlate with outcome parameters in in vitro fertilization. Fertil. Steril. 82(Suppl. 3), 1171–1176. doi: 10.1016/j.fertnstert.2004.06.013
Willner, P. (1991). Animal models as simulations of depression. Trends Pharmacol. Sci. 12, 131–136. doi: 10.1016/0165-6147(91)90529-2
Willner, P. (1997). Validity, reliability and utility of the chronic mild stress model of depression: a 10-year review and evaluation. Psychopharmacology 134, 319–329. doi: 10.1007/s002130050456
Willner, P. (2017). The chronic mild stress (CMS) model of depression: history, evaluation and usage. Neurobiol. Stress 6, 78–93. doi: 10.1016/j.ynstr.2016.08.002
Willner, P., Muscat, R., and Papp, M. (1992). Chronic mild stress-induced anhedonia: a realistic animal model of depression. Neurosci. Biobehav. Rev. 16, 525–534. doi: 10.1016/s0149-7634(05)80194-0
Willner, P., Towell, A., Sampson, D., Sophokleous, S., and Muscat, R. (1987). Reduction of sucrose preference by chronic unpredictable mild stress, and its restoration by a tricyclic antidepressant. Psychopharmacology 93, 358–364. doi: 10.1007/bf00187257
Wing, D. A., Rahall, A., Jones, M. M., Goodwin, T. M., and Paul, R. H. (1995). Misoprostol: an effective agent for cervical ripening and labor induction. Am. J. Obstet. Gynecol. 172, 1811–1816.
Witschi, E. (1962). “Development: rat,” in Growth Including Reproduction and Morphological Development, eds P. L. Altman and D. D. Katz (Washington, DC: Federation of American Societies for Experimental Biology),304–314.
Wu, G., Fang, Y. Z., Yang, S., Lupton, J. R., and Turner, N. D. (2004). Glutathione metabolism and its implications for health. J. Nutr. 134, 489–492. doi: 10.1093/jn/134.3.489
Xing, P., Li, J., and Jin, F. (2010). A case–control study of reproductive factors associated with subtypes of breast cancer in northeast China. Med. Oncol. 27, 926–931. doi: 10.1007/s12032-009-9308-7
Yang, B., Zhou, H.-J., He, Q.-J., and Fang, R.-Y. (2000). Termination of early pregnancy in the mouse, rat and hamster with DL111-IT and RU486. Contraception 62, 211–216. doi: 10.1016/s0010-7824(00)00160-8
Yang, J., Yoshizawa, K., Nandi, S., and Tsubura, A. (1999). Protective effects of pregnancy and lactation against N-methyl-N-nitrosourea-induced mammary carcinomas in female lewis rats. Carcinogenesis 20, 623–628. doi: 10.1093/carcin/20.4.623
You, Z., Luo, C., Zhang, W., Chen, Y., He, J., Zhao, Q., et al. (2011). Pro- and anti-inflammatory cytokines expression in rat’s brain and spleen exposed to chronic mild stress: involvement in depression. Behav. Brain Res. 225, 135–141. doi: 10.1016/j.bbr.2011.07.006
Yuasa, S., and MacMahon, B. (1970). Lactation and reproductive histories of breast cancer patients in Tokyo, Japan. Bull. World Health Organ. 42, 195–204.
Zhu, X., Peng, S., Zhang, S., and Zhang, X. (2011). Stress-induced depressive behaviors are correlated with Par-4 and DRD2 expression in rat striatum. Behav. Brain Res. 223, 329–335. doi: 10.1016/j.bbr.2011.04.052
Keywords: animal models, locomotor behavior, anhedonia, vaginal impedance, pregnancy, mifepristone, misoprostol, medical abortion
Citation: Camilleri C, Beiter RM, Puentes L, Aracena-Sherck P and Sammut S (2019) Biological, Behavioral and Physiological Consequences of Drug-Induced Pregnancy Termination at First-Trimester Human Equivalent in an Animal Model. Front. Neurosci. 13:544. doi: 10.3389/fnins.2019.00544
Received: 15 March 2019; Accepted: 13 May 2019;
Published: 29 May 2019.
Edited by:
Tod Edward Kippin, University of California, Santa Barbara, United StatesReviewed by:
Genaro Alfonso Coria-Avila, Universidad Veracruzana, MexicoSherri Lee Jones, McGill University, Canada
Copyright © 2019 Camilleri, Beiter, Puentes, Aracena-Sherck and Sammut. This is an open-access article distributed under the terms of the Creative Commons Attribution License (CC BY). The use, distribution or reproduction in other forums is permitted, provided the original author(s) and the copyright owner(s) are credited and that the original publication in this journal is cited, in accordance with accepted academic practice. No use, distribution or reproduction is permitted which does not comply with these terms.
*Correspondence: Stephen Sammut, ssammut@franciscan.edu
†Present address: Rebecca M. Beiter, Department of Neuroscience, Center for Brain Immunology and Glia, University of Virginia School of Medicine, Charlottesville, VA, United States
Lisett Puentes, MELISA Institute, San Pedro de la Paz, Chile