- 1Department of Pharmaceutical Sciences, North South University, Dhaka, Bangladesh
- 2Department of Pharmaceutics, College of Pharmacy, King Saud University, Riyadh, Saudi Arabia
- 3Department of Clinical Pharmacy, College of Pharmacy, King Saud University, Riyadh, Saudi Arabia
Background: Aluminum, a neurotoxic substance, causes oxidative stress induced-neurodegenerative diseases. Several lines of evidence suggest that levocarnitine has an antioxidant effect and also plays an important role in beta-oxidation of fatty acids. However, the role of levocarnitine in aluminum-induced neurotoxicity has not been well documented. Here we aimed to investigate the effect of levocarnitine on aluminum chloride (AlCl3)-induced oxidative stress and memory dysfunction.
Methods: Male Swiss albino mice (n = 30) were treated with either control (saline) or AlCl3 or AlCl3 plus levocarnitine or levocarnitine or astaxanthin plus AlCl3 or astaxanthin alone. The spatial working memory was determined by radial arm maze (RAM). In addition, we measured the lipid peroxidation (MDA), glutathione (GSH), advanced oxidation of protein products (AOPP), nitric oxide (NO) and activity of superoxide dismutase (SOD) in the various brain regions including prefrontal cortex (PFC), striatum (ST), parietal cortex (PC), hippocampus (HIP) hypothalamus (HT) and cerebellum (CB). We used astaxanthin as a standard antioxidant to compare the antioxidant activity of levocarnitine.
Results: The RAM data showed that AlCl3 treatment (50 mg/kg) for 2 weeks resulted in a significant deficit in spatial learning in mice. Moreover, aluminum exposure significantly (p < 0.05) increased the level of oxidative stress markers such as MDA, GSH, AOPP and NO in the various brain regions compared to the controls. In addition, combined administration of levocarnitine and AlCl3 significantly (p < 0.05) lowered the MDA, AOPP, GSH and NO levels in mice.
Conclusion: Our results demonstrate that levocarnitine could serve as a potential therapeutic agent in the treatment of oxidative stress associated diseases as well as in memory impairment.
Introduction
Homeostasis of metal ions is highly important for normal cognitive function. The homeostatic dysregulation has been considered as the key factor in the progression of neurodegenerative diseases (Nelson, 1999). Aluminum is widely used as a household product, and excessive use of aluminum has been found to be associated with bone, blood and brain disorders (Han et al., 2013). Earlier investigations revealed aluminum as a major risk factor in the development of amyotrophic lateral sclerosis, dementia, Parkinsonism and Alzheimer’s diseases (Kawahara, 2005; Singla and Dhawan, 2014). Aluminum exposure causes alternation of monoamine imbalance especially in cholinergic and noradrenergic neurotransmission and also leads to the generation of free radical species through disruption of glucose metabolism (LeBel and Bondy, 1991; Kawahara, 2005).
Aluminum has been shown to cause oxidative stress (OS)-induced neurodegeneration through iron accumulation and reactive oxygen species (ROS) formation (Wu et al., 2012). ROS alters the level of antioxidant enzymes such as catalase (CAT) and superoxide dismutase (SOD), and it was reported that the activity of SOD decreased in the hippocampus and cerebral cortex of the brain in response to the oxidative stress (Sitta et al., 2009). Different doses of aluminum lead to region-specific oxidative DNA damage in rats (Bodea et al., 2010) and mice (Augustyniak and Skrzydlewska, 2009). Aluminum exposure has also been reported to increase lipid peroxidation (Lee et al., 2014; Al-Amin et al., 2016) and decrease the level of glutathione (Dutta et al., 2008).
Several compounds with antioxidant properties are used for the treatment of oxidative stress- induced neurodegenerative disorders including cognitive dysfunction. Levocarnitine (4-N-trimethylammonium-3-hydroxybutyric acid) is a natural nutrient which prevents toxic accumulation of long chain fatty acids (LCFA) by transporting them into mitochondria (Sinclair et al., 2005). Levocarnitine supplementation had shown antioxidant effects in patients with coronary artery disease by improving malondialdehyde (MDA), CAT, SOD and glutathione (GSH) levels (Lee et al., 2014). It has been reported earlier that levocarnitine decreases oxidative stress by improving metabolic function (Hagen et al., 2002). We hypothesized that levocarnitine would exhibit the antioxidant effect in the brain tissues as well. In this study, we aimed to combinedly administer levocarnitine and aluminum chloride to understand whether levocarnitine could prevent aluminum chloride induced spatial working memory deficits and oxidative stress in mice. The experimental results were compared with a standard antioxidant drug, astaxanthin which is more potent than conventional antioxidants α-tocopherol and β-carotene (Miki, 1991). In addition, we previously showed that astaxanthin improved aluminum chloride induced oxidative stress in several brain regions including, prefrontal cortex, striatum, hypothalamus, hippocampus and cerebellum (Al-Amin et al., 2016).
Materials and Methods
Experimental Animals
Swiss albino mice (30–35 g and 8 weeks old) were used in this study. They were housed at 25°C under adequate light condition with standard mice pellets and water. Mice were randomly divided into four groups. Control groups were treated with saline while test groups were treated with levocarnitine (LC) (50 mg/kg) for 6 weeks. All the experimental procedures were approved by the local ethical committee of the North South University for animal experiments (Ref: ERC-IACUC/NSU/026-15).
Experimental Design
The drugs were administered orally once daily for 6 weeks. Animals were divided into the following six groups:
(1) Control (n = 6): Control groups received 1,000 μl of 0.9% saline.
(2) AlCl3 (n = 6): Aluminum chloride was given orally at a dose of 50 mg/kg body weight per day (Al-Amin et al., 2016). Aluminum chloride was dissolved in distilled water (50 mg/20 ml). The mice were given 1,200- 1,500 μl of aluminum chloride solution depending on body weight.
(3) LC (n = 6): Levocarnitine at a dose of 50 mg/kg body weight was given (Akisu et al., 2002). Levocarnitine was dissolved in distilled water (50 mg/20 ml). The mice were administered 1,200, –1,500 μl of levocarnitine solution depending on body weight.
(4) AlCl3-LC (n = 6): Levocarnitine was given orally at a dose of 50 mg/kg body weight (Akisu et al., 2002) together with aluminum chloride at a dose 50 mg/kg body weight (Singh and Goel, 2015).
(5) AST (n = 3): Astaxanthin at a dose of 20 mg/kg body weight was given (Pei et al., 2008; Al-Amin et al., 2016). Astaxanthin powder was dissolved in distilled water (20 mg/20 ml). The mice were given 500–600 μl of astaxanthin solution depending on body weight.
(6) AlCl3-AST (n = 3): Astaxanthin was given orally at a dose of 20 mg/kg body weight (Pei et al., 2008; Al-Amin et al., 2016) together with aluminum chloride at a dose 50 mg/kg body weight (Singh and Goel, 2015).
After 6 weeks of treatment, we measured spatial working memory using the 8-arm radial arm maze. The animals were then euthanized and brain tissues were collected to measure a range of oxidative stress markers.
Radial Arm Maze Test
Eight-arm radial maze was constructed following the measurements as described by Terragon et al. (2012).
Training
We used food pellets as a target to train the animals. The task of the mice was to find the target making fewer mistakes during the training session. Each mouse was placed in the center of the RAM device to find out the target for 10 min. Initially, all the arms contained the target. The target was placed at the end of the arm within a well. However, the target-containing arms were reduced gradually. The training sessions consisted of 8 consecutive days; Day 1,2: all arms contain target; Day 3: 4 arms; Day 4,5: 3 arms; Day 6: 2 arms; Day 7,8: 1 arm. The training was stopped once the animals visited all of the arms or spent 10 min. The successful performers (mice) were finally included for the experiments.
Testing Session
On the day of the experiment, only one arm contained food and all the animals were kept on overnight fasting (except water). Mice were released at the center of the maze and allowed to explore the maze. Parameters were followed as stated by Terragon et al. (2012) and three trials were run for each mouse. Each trial was recorded using the webcam for manual analysis later. After each run, the apparatus was cleaned with 70% ethanol. The spatial working memory data analysis was performed based on our previously established protocol (Al-Amin et al., 2014, 2016).
Tissue Processing
Mice were anesthetized using 200 μl of ketamine (50 mg/ml, ACI pharmaceuticals Ltd., Bangladesh). The animals were sacrificed by decapitation. The brain was immediately removed from the skull and immediately transferred to a petri dish placed over ice. The hippocampal, striatum, prefrontal cortex, parietal cortex, hypothalamus and cerebellum tissues were microdissected and preserved in –20°C.
The homogenates of brain tissues 10% (w/v) were prepared in sodium phosphate buffer (1× PBS pH 7.0) using Ultra-Turrax T25 (United States) homogenizer. Homogenized tissue samples were sonicated at 5-s cycle for 150 s using an ultrasonic processor. The homogenized samples were centrifuged at 10,000 rpm (7960 g) for 10 min at 4°C. The clear supernatants were collected and stored at –20°C for the biochemical analysis.
Oxidative Stress Measurement
Estimation of Lipid Peroxidation (MDA)
Lipid peroxidation was estimated colorimetrically by measuring thiobarbituric acid reactive substances (TBARS) as described previously (Niehaus and Samuelsson, 1968). Briefly, 0.1 ml of tissue homogenate in Tris–HCl buffer (pH 7.5) was treated with 2 ml of TBA-TCA-HCl (1:1:1 ratio) reagent (thiobarbituric acid 0.37%, 0.25 N HCl and 15% TCA) and placed in water bath for 15 min and cooled. The absorbance of the clear supernatant was measured against reference blank at 535 nm (Al-Amin et al., 2014). The level of MDA was measured by using standard curve and expressed as nmol/ml.
Advanced Oxidation of Protein Products (AOPP)
Advanced oxidation of protein products were determined spectrophotometrically (Witko-Sarsat et al., 1996; Al-Amin et al., 2015a). Briefly, 50 μl of plasma diluted with phosphate-buffered saline (PBS) at a ratio of 1:2 and chloramine T (0–100 mmol/L) were used for the preparation of the calibration curve. PBS was used as a blank. 100 μl of 1.16 M potassium iodide and 50 μl of acetic acid were added to each well and absorbance at 340 nm was measured immediately. Concentrations of APOPs were expressed in chloramine units (μmol/ml).
Glutathione (GSH) Level
Glutathione in the brain was assayed according to a previously described method (Ellman, 1959; Al-Amin et al., 2015b). Briefly, 2.7 ml of phosphate buffer (0.1 M, pH 8) and 0.2 ml of 5, 5-dithio-bis (2-nitrobenzoic acid) were added with 1 ml of plasma. The color developed was measured immediately at 412 nm. Results were expressed as μmol/mg protein.
Nitric Oxide (NO) Level
The levels of Nitric Oxide (NO) was measured based on a previous protocol (Tracey et al., 1995) using the Griess-Illosvoy reagent. The tissue homogenates were diluted with PBS (2:8 ratio) and incubated at 25°C for 15 min in a 96-well plate (Al-Amin et al., 2015b). The absorbances were then measured at a wavelength of 540 nm against the blank readings of the spectrophotometer.
Assay of Superoxide Dismutase (SOD) Activity
The activity of SOD was assayed by a modified procedure as described previously (Ma et al., 2010; Al-Amin et al., 2018a). Briefly, the reaction mixture contained 50 mM sodium phosphate (pH 7.8), 13 mM methionine, 75 mM nitroblue tetrazolium (NBT), 2 mM riboflavin, 100 mM EDTA and 2 mL of plasma. The change in absorbance of each sample was then recorded at 560 nm after the formation of the blue formazan.
Statistical Analysis
One-way ANOVA was conducted to measure the main effect of the treatment groups. Post hoc test namely, “Newman-Keuls” was used to compare the difference between groups. All analyses were carried out with the Graph pad prism (version 6.0) software. The difference was considered significant when the p-value was found less than 0.05. Data are represented as mean ± SEM (Standard Error of the Mean).
Results
Effect of Various Treatments on Working Memory Performance in Radial Arm Maze Test
There was a significant main effect of treatment on the working memory correct [F(5,24) = 4.58; p = 0.004] (Figure 1A) and working memory incorrect [F(5,24) = 7.90; p < 0.001] (Figure 1B). However, student t-test showed that the number of “working memory correct” was significantly (p < 0.05) decreased in the AlCl3-treated mice compared to control mice. In addition, the number of “working memory incorrect” was also significantly (p < 0.05) increased in AlCl3 treated mice compared to the control mice. Surprisingly, AlCl3+LC-treated mice showed increased entry to the target arm than AlCl3-treated mice, indicating an improvement of working memory. We also observed a significant main effect of treatment on the “percentage entry to the target arm” [F(5,24) = 3.93; p < 0.001] (Figure 1C) and “time spent in the target arm” [F(5,24) = 8.50 p < 0.001] (Figure 1D). The results of this RAM test suggest that levocarnitine intake may facilitate the finding of the target, suggesting an improvement of the spatial working memory performance. The result has been compared with the standard drug AST.
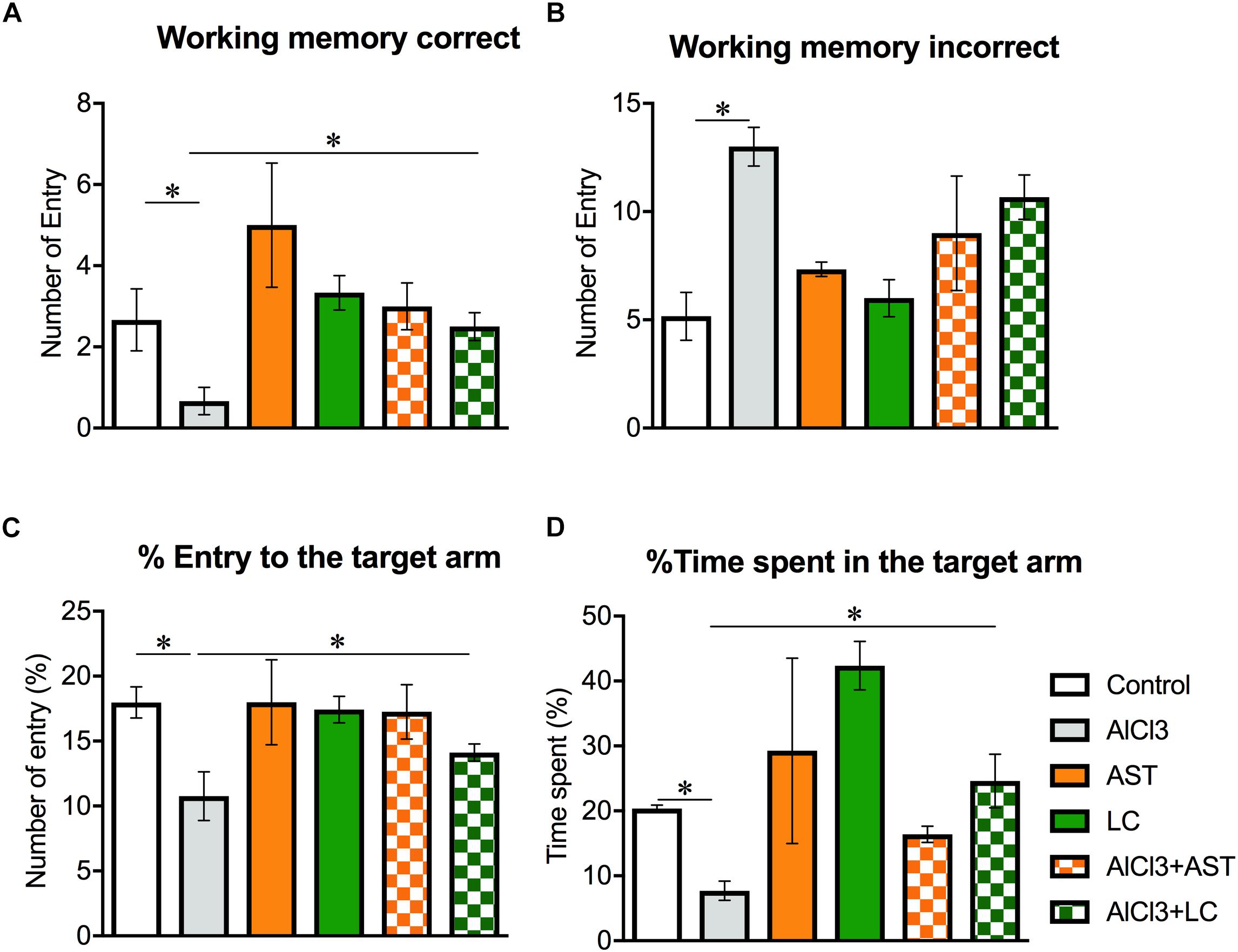
Figure 1. Effect of levocarnitine and aluminum chloride on spatial working memory. The aluminum treated mice showed a lower working memory (A); a higher incorrect working memory (B); a lower percentage of entry to the target arm (C); and a higher number of arm entry (D). The combined (levocarnitine plus aluminum) treatment improved these parameters. The groups were control, AlCl3 (aluminum chloride) and AlCl3+LC (aluminum chloride and levocarnitine) and LC (levocarnitine). Data presented as mean ± SEM. n = 6 per group except AST (n = 3); and AlCL3+AST (n = 3); ∗∗p < 0.01, ∗p < 0.05.
Biochemical Estimation
Lipid Peroxidation (MDA Levels)
Our results showed a significant main effect of treatments on the MDA levels in the prefrontal cortex (PFC) [F(5,16) = 49.58, p < 0.001] (Figure 2A); striatum (ST) [F(5,21) = 33.82, p < 0.001] (Figure 2B); parietal cortex (PC) [F(5,21) = 76.39, p < 0.001] (Figure 2C); hypothalamus (HT) [F(5,22) = 240.0, p < 0.001] (Figure 2D); hippocampus (HIP) [F(5,22) = 90.0, p < 0.001] (Figure 2E) and cerebellum (CB) [F(5,17) = 19.30, p < 0.001] (Figure 2F). The post hoc test indicated that the level of MDA was noticeably (p < 0.05) increased in the aluminum-exposed group in the PFC, ST, PC, HT, HIP and CB. On the other hand, levocarnitine treatment in aluminum exposed group improved the levels of MDA in these brain regions. AST has been used as reference standard.
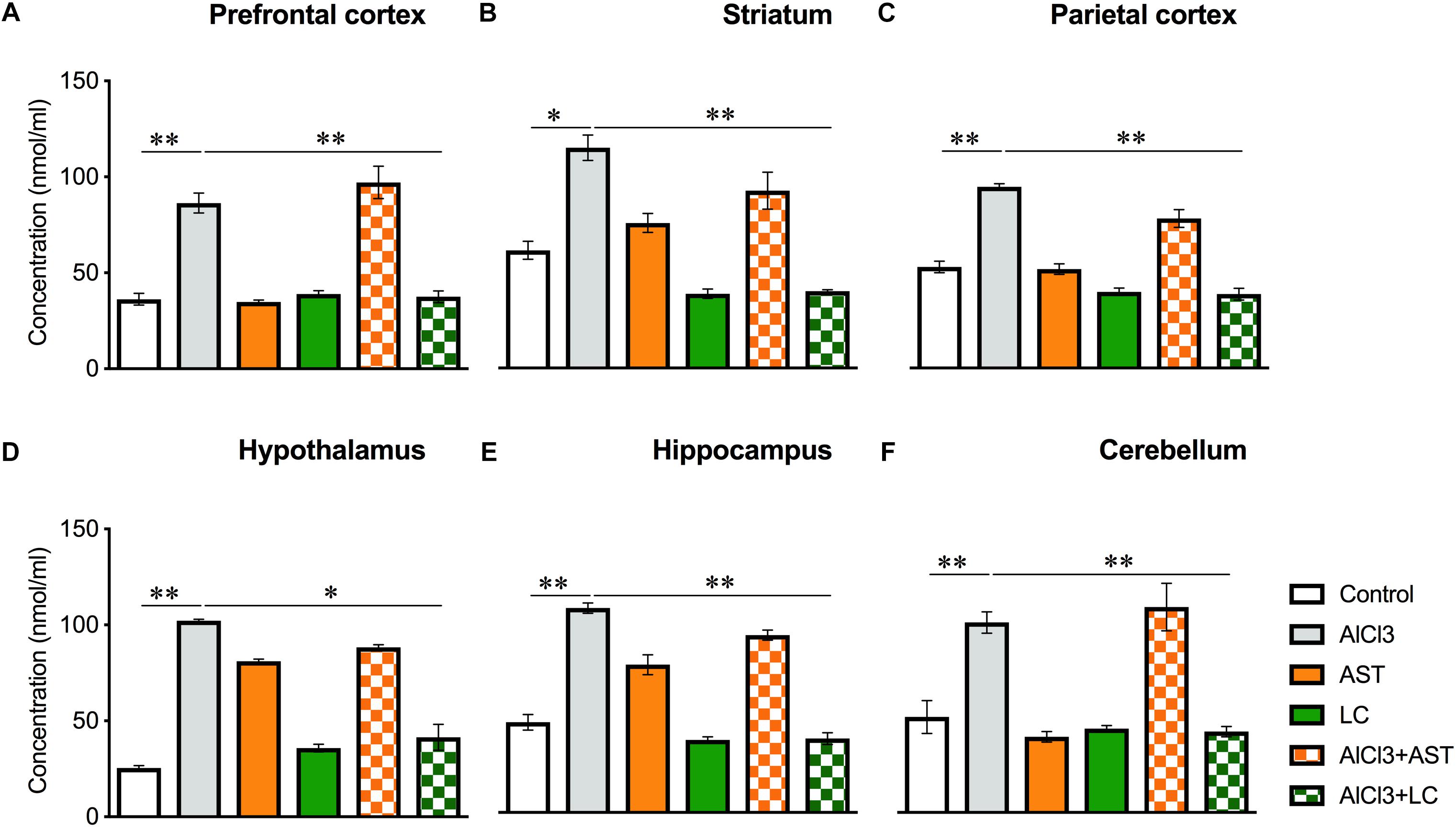
Figure 2. Treatment of levocarnitine and aluminum chloride on MDA (lipid peroxidation) (A–F); in the prefrontal cortex (PFC) (A), striatum (ST) (B), parietal cortex (PC) (C), hypothalamus (HT) (D), hippocampus (HP) (E) and cerebellum (CB) (F). The groups were control, AlCl3 (aluminum chloride), AST (astaxanthin), AST+AlCl3 (aluminum chloride and astaxanthin) and AlCl3+LC (aluminum chloride and levocarnitine) and LC (levocarnitine). Data presented as mean ± SEM. n = 6 per group except AST (n = 3); and AlCL3+AST (n = 3); ∗∗∗p < 0.001, ∗∗p < 0.01, ∗p < 0.05.
Advanced Oxidation of Protein Products (AOPP)
Our results showed a significant main effect of treatment on the AOPP levels in the PFC [F(5,22) = 177.2, p < 0.001] (Figure 3A); ST [F(5,21) = 412.2, p < 0.001] (Figure 3B); PC [F(5,21) = 50.26, p < 0.001] (Figure 3C); HT [F(5,21) = 643, p < 0.001] (Figure 3D); HIP [F(5,21) = 138.4, p < 0.001] (Figure 3E) and in the CB [F(5,) = 138.4, p < 0.001] (Figure 3F). The post hoc test indicated that the level of AOPP was markedly (p < 0.05) elevated in the PFC, ST, PC, HT, HIP of the aluminum-exposed group except for CB. Interestingly, exposure with aluminum plus levocarnitine improved the levels of AOPP in these brain regions. The standard drug AST has been used to compare the result.
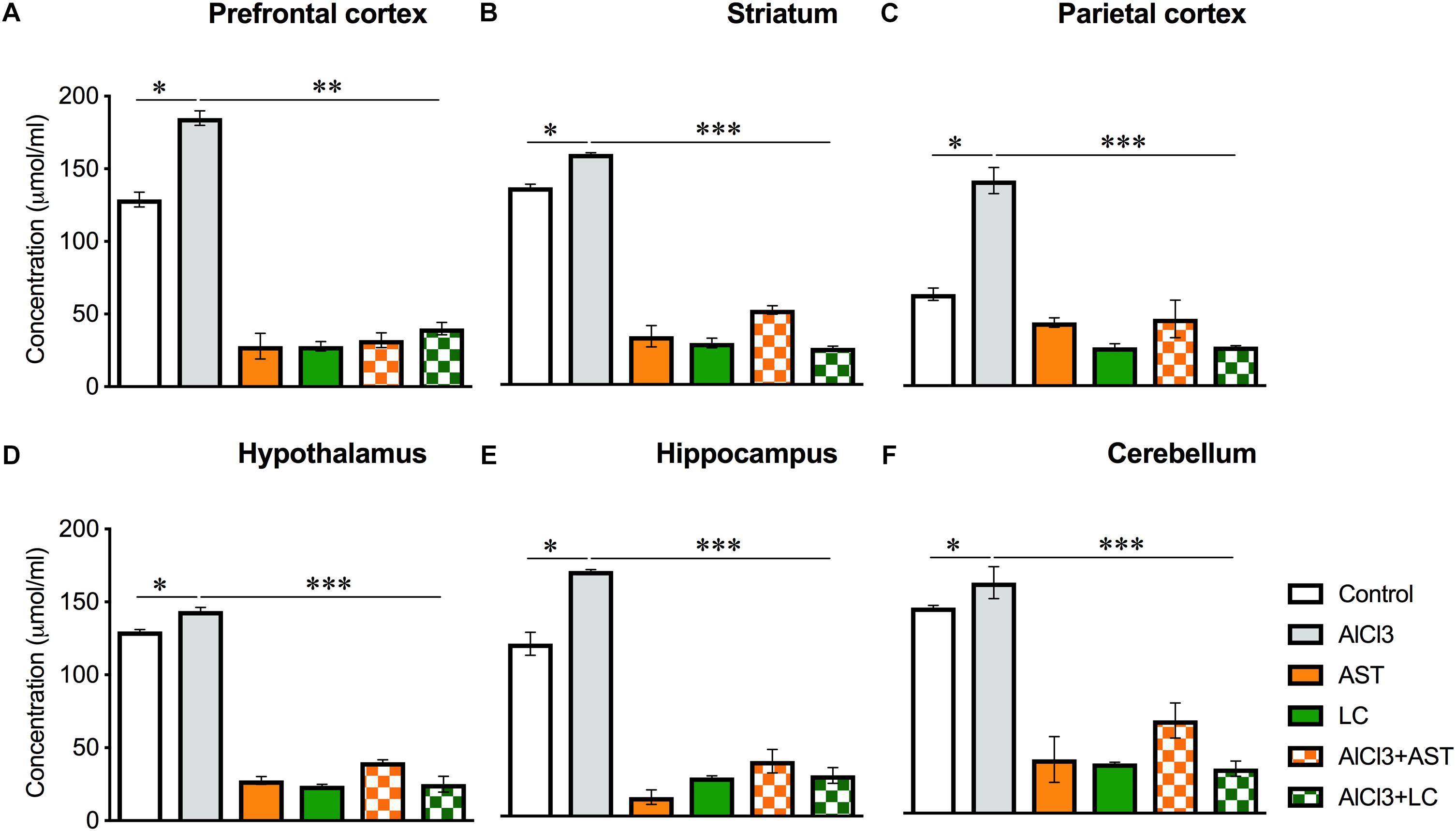
Figure 3. Treatment of levocarnitine and aluminum chloride on advanced oxidation of protein produces (AOPP) (A–F); in the prefrontal cortex (PFC) (A), striatum (ST) (B), parietal cortex (PC) (C), hypothalamus (HT) (D), hippocampus (HP) (E) and cerebellum (CB) (F). The groups were control, AlCl3 (aluminum chloride), AST (astaxanthin), AST+AlCl3 (aluminum chloride and astaxanthin) and AlCl3+LC (aluminum chloride and levocarnitine) and LC (levocarnitine). Data presented as mean ± SEM. n = 6 per group except AST (n = 3); and AlCL3+AST (n = 3); ∗∗∗p < 0.001, ∗∗p < 0.01, ∗p < 0.05.
Glutathione (GSH)
A significant main effect of various treatments was observed on PFC [F(5,21) = 102.9, p < 0.001] (Figure 4A); ST [F(5,22) = 340.7, p < 0.001] (Figure 4B); PC [F(5,21) = 104.5, p < 0.001] (Figure 4C); HT [F(5,22) = 278.2, p < 0.001] (Figure 4D); HIP [F(5,21) = 73.41, p < 0.001] (Figure 4E) and CB [F(5,20) = 28.74, p < 0.01] (Figure 4F). The post hoc test indicated that the levels of GSH in the PFC, ST, PC, HT, HIP and CB were markedly (p < 0.05) higher in the aluminum-exposed group than the control group. However, exposure with aluminum plus levocarnitine reversed the levels of GSH in these brain regions. The result has been compared with the standard drug AST.
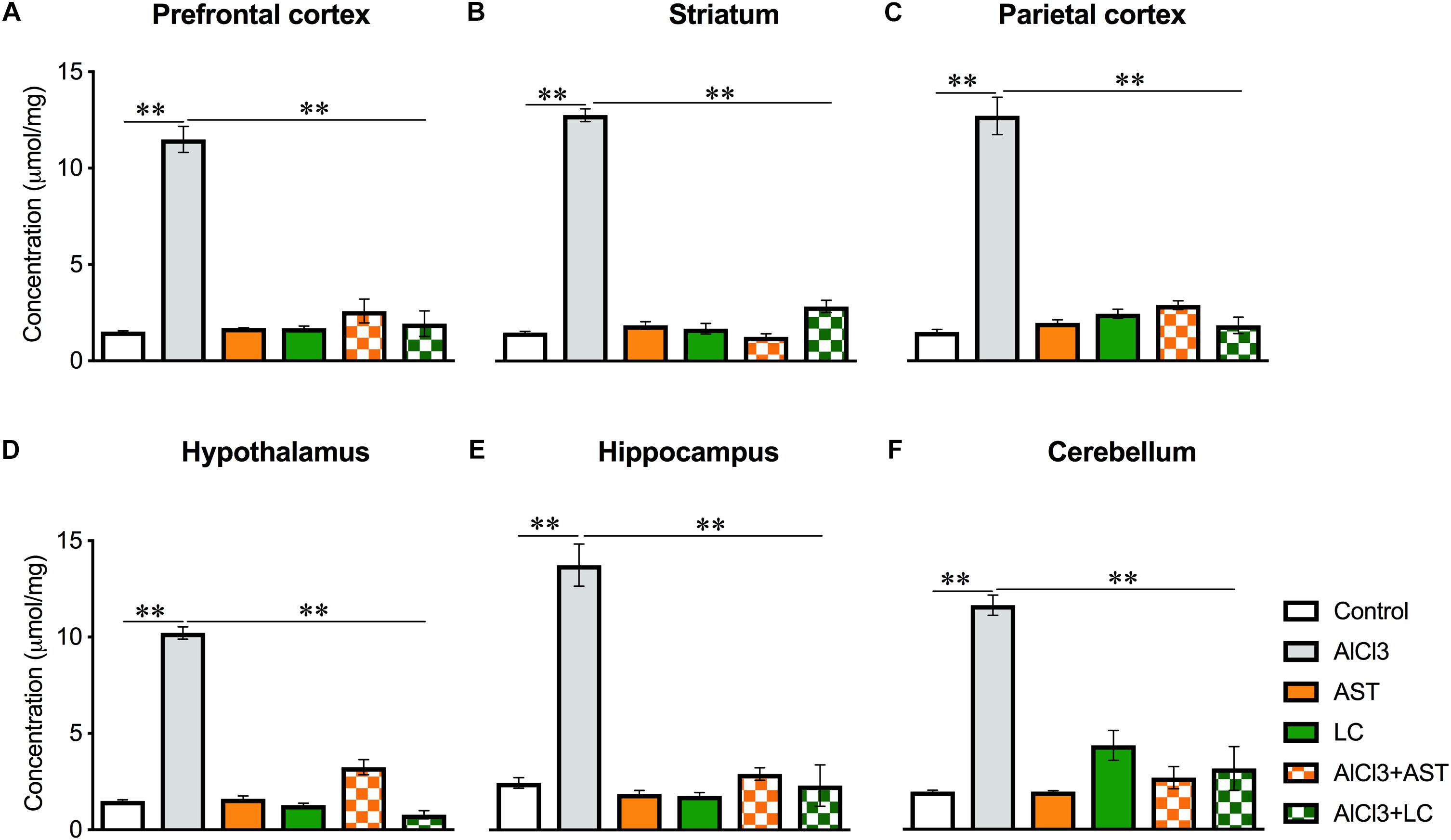
Figure 4. Treatment of levocarnitine and aluminum chloride on glutathione (GSH) (A–F); in the prefrontal cortex (PFC) (A), striatum (ST) (B), parietal cortex (PC) (C), hypothalamus (HT) (D), hippocampus (HP) (E) and cerebellum (CB) (F). The groups were control, AlCl3 (aluminum chloride), AST (astaxanthin), AST+AlCl3 (aluminum chloride and astaxanthin) and AlCl3+LC (aluminum chloride and levocarnitine) and LC (levocarnitine). Data presented as mean ± SEM. n = 6 per group except AST (n = 3); and AlCL3+AST (n = 3); ∗∗∗p < 0.001, ∗∗p < 0.01, ∗p < 0.05.
Nitric Oxide (NO)
Our results showed a significant main effect of treatments on the NO levels in the PFC [F(5,24) = 25.2, p < 0.001] (Figure 5A); ST [F(5,24) = 99.65, p < 0.001] (Figure 5B); PC [F(5,24) = 71.80, p < 0.001] (Figure 5C); HT [F(5,24) = 43.86, p < 0.001] (Figure 5D); HIP [F(5,24) = 40.51, p < 0.001] (Figure 5E) and in the CB [F(5,24) = 115.4, p < 0.001] (Figure 5F). The post hoc test indicated that the level of NO was markedly (p < 0.05) raised in the PFC, ST, PC, HT, HIP and CB of the aluminum-exposed group. On the other hand, exposure with aluminum plus levocarnitine improved the levels of NO in these brain regions. The result has been compared with the standard drug AST.
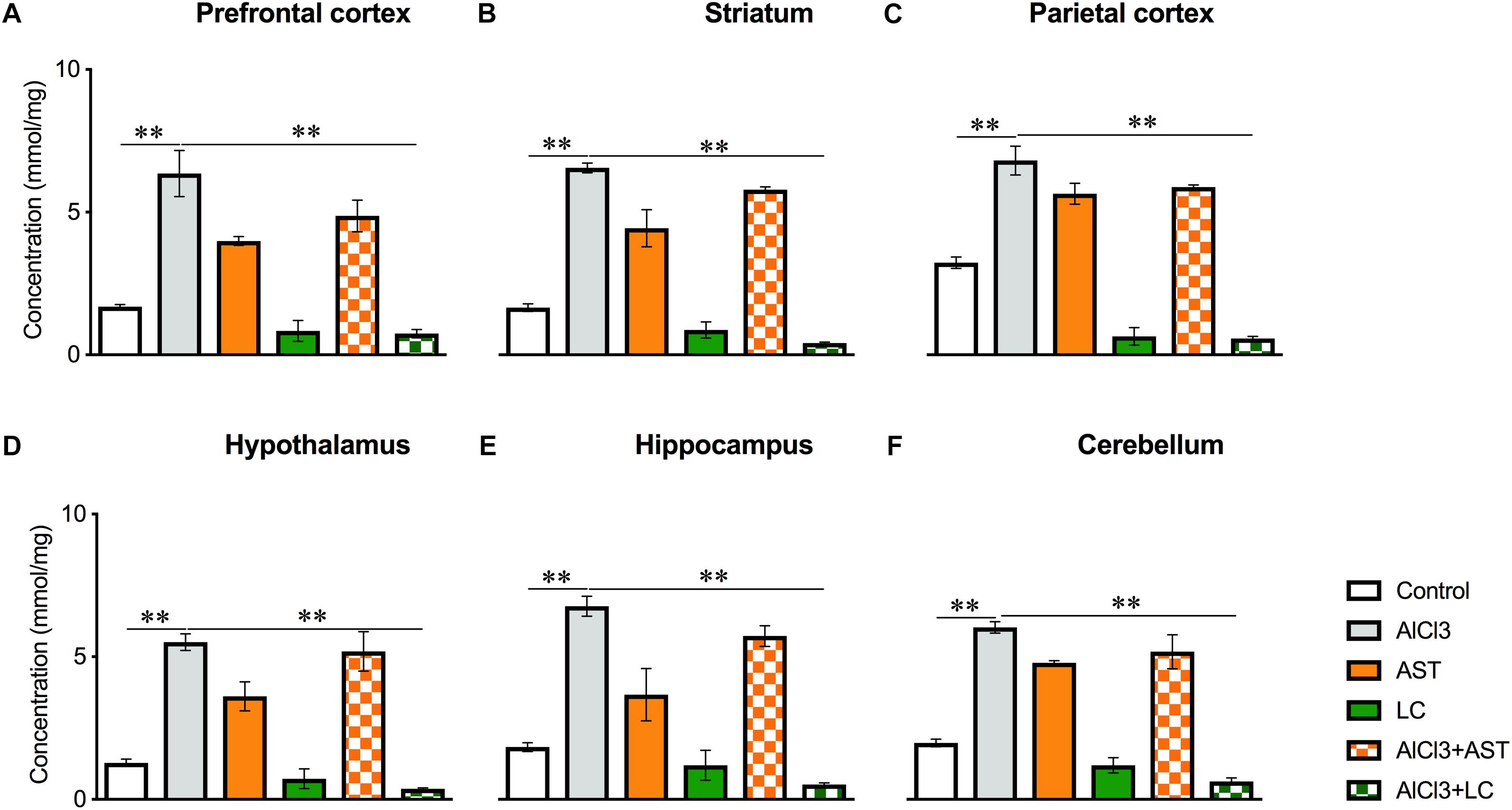
Figure 5. Treatment of levocarnitine and aluminum chloride on nitric oxide (NO) (A–F); in the prefrontal cortex (PFC) (A), striatum (ST) (B), parietal cortex (PC) (C), hypothalamus (HT) (D), hippocampus (HP) (E) and cerebellum (CB) (F). The groups were control, AlCl3 (aluminum chloride), AST (astaxanthin), AST+AlCl3 (aluminum chloride and astaxanthin) and AlCl3+LC (aluminum chloride and levocarnitine) and LC (levocarnitine). Data presented as mean ± SEM. n = 6 per group except AST (n = 3); and AlCL3+AST (n = 3); ∗∗∗p < 0.001, ∗∗p < 0.01, ∗p < 0.05.
Superoxide Dismutase (SOD)
Results of one-way ANOVA analysis showed a significant main effect of treatments on PFC [F(5,22) = 8.14, p < 0.001] (Figure 6A); PC [F(5,21) = 8.41, p < 0.01)] (Figure 6C) and HIP [F(5,24) = 3.06, p < 0.02] (Figure 6E); but not ST [F(5,24) = 2.45, p = 0.06] (Figure 6B); HT [F(5,19) = 1.73, p = 0.17] (Figure 6D); and CB [F(5,24) = 0.83, p = 0.53] (Figure 6F). The post hoc test indicated that the level of SOD in the PC was markedly (p < 0.05) lowered in the aluminum-exposed group as compared to the control group. Interestingly, exposure to aluminum plus levocarnitine reversed the levels of SOD in PC. AST has been used as reference standard.
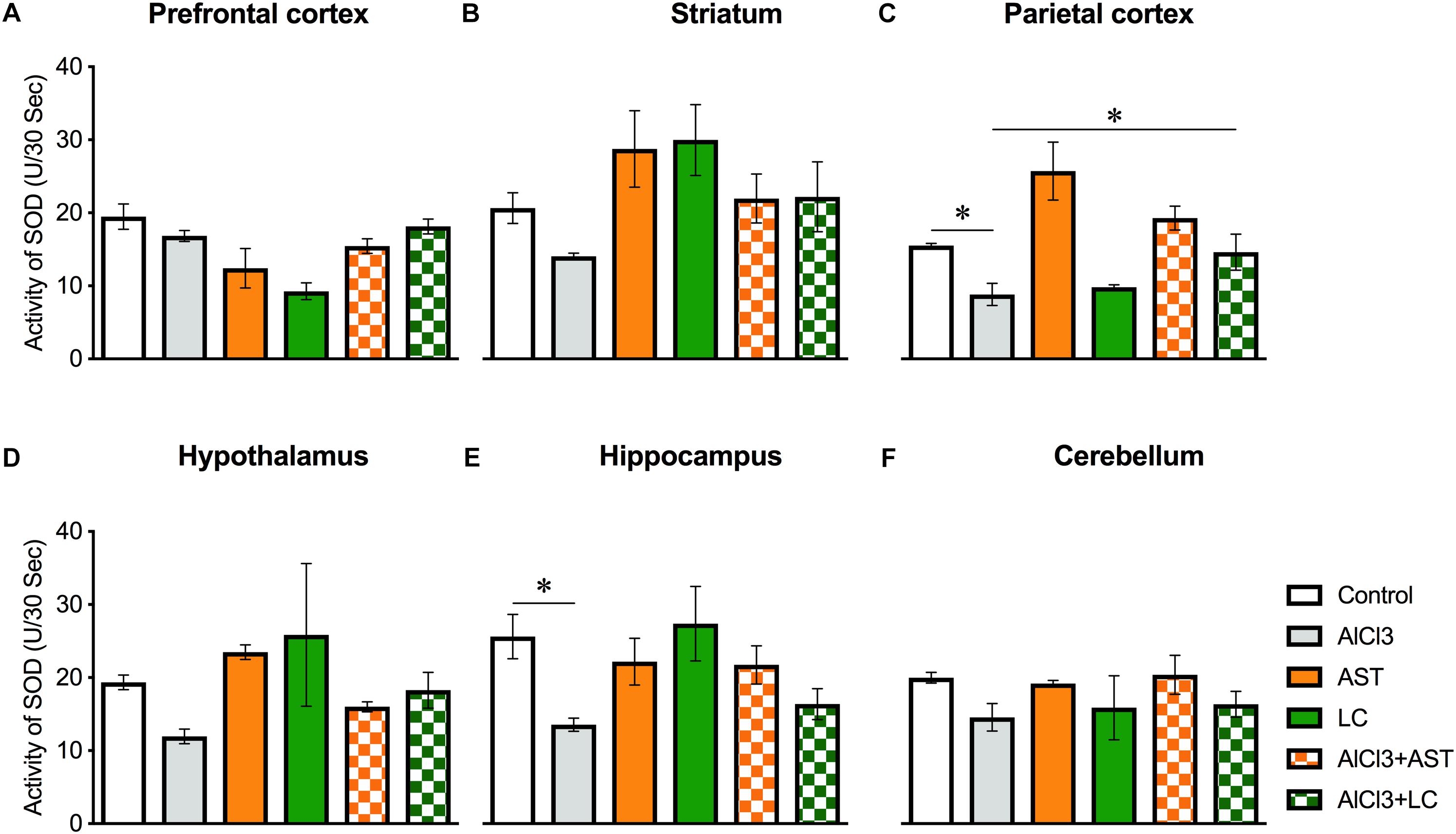
Figure 6. Treatment of levocarnitine and aluminum chloride on superoxide dismutase (SOD) (A–F); in the prefrontal cortex (PFC) (A), striatum (ST) (B), parietal cortex (PC) (C), hypothalamus (HT) (D), hippocampus (HP) (E) and cerebellum (CB) (F). The groups were control, AlCl3 (aluminum chloride), AST (astaxanthin), AST+AlCl3 (aluminum chloride and astaxanthin) and AlCl3+LC (aluminum chloride and levocarnitine) and LC (levocarnitine). Data presented as mean ± SEM. n = 6 per group except AST (n = 3); and AlCL3+AST (n = 3); ∗∗∗p < 0.001, ∗∗p < 0.01, ∗p < 0.05.
Discussion
We aimed to investigate the impact of levocarnitine on memory performance and oxidative stress in the PFC, ST, PC, HT, HIP and CB of the AlCl3-treated mice. Our study revealed several important findings. First, we found that aluminum exposure leads to impairment of spatial working memory in mice. Second, the concurrent administration of levocarnitine and aluminum improves the spatial working memory performance, suggesting that levocarnitine may have a role in preventing aluminum-induced behavioral impairment. Third, the results of our oxidative stress associated study further support the behavioral data obtained, indicating that the spatial working memory deficit could be associated with the oxidative stress in the cortical and subcortical brain tissues. Fourth, the effect of LC on spatial working memory and oxidative stress was comparable to AST, a potent antioxidant, when concurrently administered with aluminum chloride (Table 1).
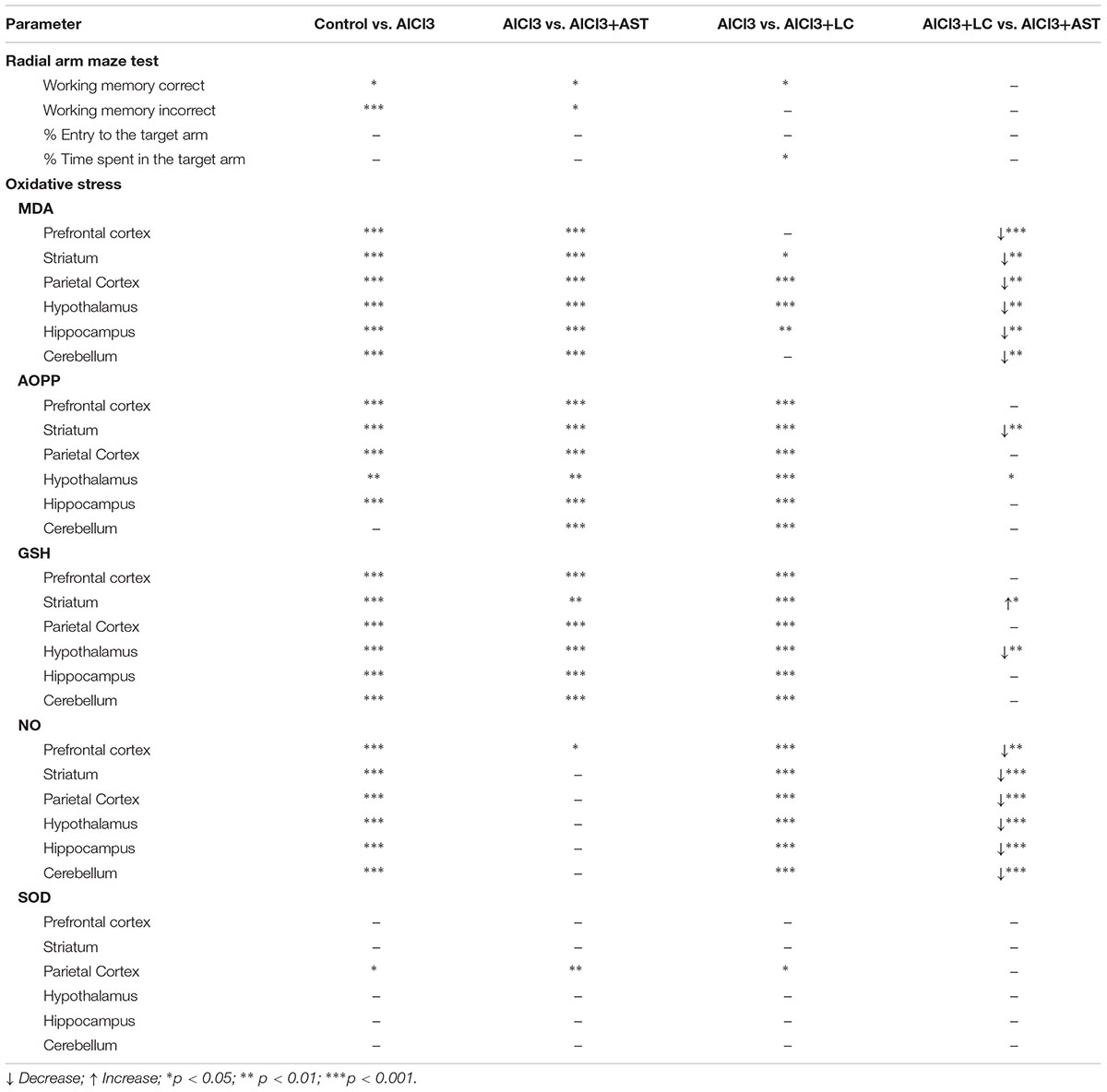
Table 1. Summary of the p values showing comparative effects of treatments between the groups of interest.
Radial arm maze test is purely a hippocampal-dependent test to measure the hippocampal-dependent spatial working memory (Zhang and O’Donnell, 2000; Sun et al., 2007; Hodges et al., 2009; Al-Amin et al., 2016). In this experiment, the task of the mice was to find out the target making fewer errors (incorrect entry). The fastest re-entry to the target arm indicates that mice encode the spatial information of the baited arm (arm containing pellet) as the target was not visible with the direct eyesight, and the animals had to depend on the hippocampal encoding and retrieval of spatial memory. In radial arm maze test, our results showed that aluminum-exposed animals made more mistakes in finding the target. On the other hand, aluminum-exposed animals visited arms that contained no target. This result suggests that aluminum treatment causes impaired spatial working memory formation. Administration of aluminum chloride also resulted in deterioration of the spatial memory as determined by the radial arm maze test. Our results are in line with a previous report that showed oral administration of aluminum impaired the spatial working memory formation in Swiss albino mice (Al-Amin et al., 2016). Also, an intracerebral administration of aluminum chloride caused the spatial learning deficit in the Morris water maze task in rabbits (Goo et al., 2012).
To be noted that in this study all animals were previously trained before administration of drugs. However, levocarnitine plus aluminum-treated mice remembered food containing arms efficiently. Radial-arm maze (RAM) test is a sensitive and reliable way to assess both learning and memory in a variety of species such as rats and mice (Levin, 2015). RAM test device has seven options to make a mistake to enter into the wrong arm. We observed that levocarnitine plus aluminum-treated animals picked up the food spending less time as compared to the aluminum group and also, levocarnitine plus aluminum-treated animals spent more time in the food containing arms. Our findings are also consistent with a previous study demonstrating the learning memory performance (Ritter and Cummings, 2015). Taken together, it is possible that aluminum could cause spatial working memory deficit in animals, while levocarnitine prevents the aluminum-induced decrement of spatial working memory, suggesting that this drug may have a beneficial effect on spatial cognition. However, we do not know the exact mechanism of the spatial working memory impairment caused by aluminum exposure. One possibility may be an increment of oxidative stress (Al-Amin et al., 2016). Since free radicals mediate oxidative damage, it is necessary to investigate the endogenous antioxidant enzymes like superoxide dismutase and glutathione which are the first line defense against free radical damage under oxidative stress.
When the impact of LC was compared with AST on aluminum-induced spatial working memory deficit, we did not find any significant difference. Previously, we reported that AST improves aluminum chloride- (Al-Amin et al., 2016) and scopolamine-induced (Al-Amin et al., 2018b) spatial working memory deficits in Swiss albino mice in RAM task. It is possible that the underlying basis of this behavioral finding is similar.
In our study, administration of aluminum chloride resulted in marked oxidation stress as indicated by an increase in lipid peroxidation and nitrite concentrations, and a decrease in glutathione and superoxide dismutase. These changes could have been due to the reduced axonal mitochondrial turnover and disruption of the Golgi induced by aluminum treatment, which resulted in the release of oxidative products like malondialdehyde, carbonyls and peroxynitrites within the neurons. Under oxidative stress, MDA is considered one of the key intermediates of free radical-induced damage. Increased level of MDA has been reported in the cortex, hippocampus, cerebellum in the neurons and astrocyte of aged rodents. MDA interferes with the brain homeostasis between an inhibitory and excitatory neuron (Vaishnav et al., 2010); impairs the function of the brain mitochondria (Li et al., 2012) which results in the disturbances of brain function. Our study showed a significantly increased MDA level in aluminum chloride-treated animals while lower MDA level in all the tested regions of the brain of the levocarnitine-treated mice. These results are consistent with the previous report showing similar findings (Harooni et al., 2009). In addition, levocarnitine treatment was found to reduce MDA level in humans (Atalay Guzel et al., 2015).
Advanced Oxidation of Protein Product is also used as a marker of oxidative stress which is formed when ROS oxidizes the protein products such as oxidized albumin. In this study aluminum chloride exposure significantly increased the level of AOPP, while treatment of levocarnitine strongly reversed this effect. This drastic lowering effect by levocarnitine may be due to its potent antioxidant property that scavenges the level of free radicals produced in the brain tissues. The current results are consistent with the previous report by Orihuela et al. (2005). Glutathione is the most abundant intracellular antioxidant found in reduced form and is involved in direct scavenging of free radicals or serving as a substrate for the glutathione peroxidase enzyme that catalyzes the detoxification of hydrogen peroxide. Our findings that aluminum chloride exposure significantly increased the level of GSH, while treatment of levocarnitine reversed this action are again consistent with a previous report (Kumar et al., 2011). Superoxide dismutase presents the first line of defense against superoxide as it dismutates the superoxide anion to hydrogen peroxide and oxygen. Catalase protects SOD by converting hydrogen peroxide to water and oxygen (Ighodaro and Akinloye, 2017). In this study, aluminum chloride exposure significantly decreased the level of SOD, while treatment with levocarnitine reversed this action. Another mechanism of spatial working memory performance impairment by aluminum may be due to its interference with downstream effector molecules such as cyclic GMP involved in long-term potentiation (Canales et al., 2001).
Astaxanthin has been reported to improve spatial working memory in RAM test in Swiss albino mice (Al-Amin et al., 2015b, 2016, 2018b). Therefore, we compared the impact of LC with AST on aluminum-induced oxidative stress. Our findings showed that LC had a stronger effect than AST (Table 1) on most of the oxidative stress parameters. MDA and Nitric oxide levels were significantly downregulated with the combined treatment of AlCl3+LC than AlCl3+AST. This could be attributed to the role of levocarnitine in transfer of LCFA to the mitochondria resulting in a reduced level of MDA. However, we do not know why LC has shown a greater impact on reducing the level of nitric oxide. Future study will be required to reveal the mechanism of LC on nitric oxide level in brain.
Besides hippocampus, the performance of radial arm maze task also depends on a specific circuit that connects hippocampus with prefrontal and ventral striatal cortices (Floresco et al., 1997). Therefore, we measured the level of oxidative stress parameters in the prefrontal cortex, striatum, and hippocampus. Moreover, we included the hypothalamus, parietal cortex and cerebellum tissues since a previous study included these brain regions and showed that levocarnitine treatment showed the neuroprotective effect by reducing the lipid peroxidation (Rani and Panneerselvam, 2002). We acknowledge that we have some experimental limitations as we could not perform immunohistochemistry and measure signaling proteins, such as NOS, HO-1 and Nrf-2 in the brain tissues, which could add value to this study. Nevertheless, we have confirmed some previous findings of the neurotoxic effect of aluminum and here we first time demonstrate the compelling evidence in supporting the beneficial effect of levocarnitine in the improvement of the brain oxidative stress and memory function in aluminum chloride-treated mice.
Conclusion
This study aimed to investigate the effect of levocarnitine on brain oxidative stress induced by aluminum chloride. We focused on six specific brain regions namely frontal cortex, striatum, parietal cortex, hypothalamus, hippocampus, cerebellum that are distinguishable and responsible for vital brain functions. Four non-enzymatic and two enzymatic antioxidant markers were evaluated. The results obtained in this study demonstrate that treatment with levocarnitine alleviates oxidative stress as determined by evaluating oxidative stress markers in most of the brain regions and improves aluminum chloride-induced memory dysfunction.
Data Availability
All datasets generated for this study are included in the manuscript and/or the supplementary files.
Author Contributions
MA-A, MC, and AS performed behavioral experiments and carried out neuronal oxidative stress study. The manuscript was drafted by MA-A and MC. MA-A, AS, and MNA analyzed the data. HR, PJ, MH, MAA, and MA-A contributed to editing and critically revising the manuscript for important intellectual content. MK, AA, MR, AAh, and HR supported with chemical/lab facilities corrected drafts and obtained the funding. All co-authors approved the final version of the manuscript for submission.
Funding
This research was funded by RG#1435-017.
Conflict of Interest Statement
The authors declare that the research was conducted in the absence of any commercial or financial relationships that could be construed as a potential conflict of interest.
Acknowledgments
The authors would like to extend their sincere appreciation to the Deanship of Scientific Research at King Saud University for its funding through research group number (RG#1435-017). This work was partially supported by the research grant provided by the North South University 2015–16.
References
Akisu, M., Ozmen, D., Baka, M., Habif, S., Yalaz, M., Arslanoglu, S., et al. (2002). Protective effect of dietary supplementation with L-arginine and L-carnitine on hypoxia/reoxygenation-induced necrotizing enterocolitis in young mice. Biol. Neonate 81, 260–265. doi: 10.1159/000056757
Al-Amin, M. M., Akhter, S., Hasan, A. T., Alam, T., Nageeb Hasan, S. M., Saifullah, A. R., et al. (2015a). The antioxidant effect of astaxanthin is higher in young mice than aged: a region specific study on brain. Metab. Brain Dis. 30, 1237–1246. doi: 10.1007/s11011-015-9699-4
Al-Amin, M. M., Rahman, M. M., Khan, F. R., Zaman, F., and Reza, H. M. (2015b). Astaxanthin improves behavioral disorder and oxidative stress in prenatal valproic acid-induced mice model of autism. Behav. Brain Res. 286, 112–121. doi: 10.1016/j.bbr.2015.02.041
Al-Amin, M. M., Choudhury, M. F. R., Chowdhury, A. S., Chowdhury, T. R., Jain, P., Kazi, M., et al. (2018a). Pretreatment with risperidone ameliorates systemic LPS-induced oxidative stress in the cortex and hippocampus. Front. Neurosci. 12:384. doi: 10.3389/fnins.2018.00384
Al-Amin, M. M., Mahmud, W., Pervin, M. S., Islam, S. M. R., Rahman, M. A., and Zinchenko, A. (2018b). Astaxanthin ameliorates scopolamine-induced spatial memory via reduced cortical-striato-hippocampal oxidative stress. Brain Res. doi: 10.1016/j.brainres.2018.12.014 [Epub ahead of print].
Al-Amin, M. M., Hasan, S. M., Alam, T., Hasan, A. T., Hossain, I., Didar, R. R., et al. (2014). Tadalafil enhances working memory, and reduces hippocampal oxidative stress in both young and aged mice. Eur. J. Pharmacol. 745, 84–90. doi: 10.1016/j.ejphar.2014.10.026
Al-Amin, M. M., Reza, H. M., Saadi, H. M., Mahmud, W., Ibrahim, A. A., Alam, M. M., et al. (2016). Astaxanthin ameliorates aluminium chloride-induced spatial memory impairment and neuronal oxidative stress in mice. Eur. J. Pharmacol. 777, 60–69. doi: 10.1016/j.ejphar.2016.02.062
Atalay Guzel, N., Erikoglu Orer, G., Sezen Bircan, F., and Coskun Cevher, S. (2015). Effects of acute L-carnitine supplementation on nitric oxide production and oxidative stress after exhaustive exercise in young soccer players. J. Sports Med. Phys. Fitness 55, 9–15.
Augustyniak, A., and Skrzydlewska, E. (2009). L-Carnitine in the lipid and protein protection against ethanol-induced oxidative stress. Alcohol 43, 217–223. doi: 10.1016/j.alcohol.2008.12.005
Bodea, F., Bocea, A., and Decea, N. (2010). [L-carnitine decreases oxidative stress induced by experimental hypobaric hypoxia]. Pediatr. Endocrinol. Diabetes Metab. 16, 78–81.
Canales, J. J., Corbalan, R., Montoliu, C., Llansola, M., Monfort, P., Erceg, S., et al. (2001). Aluminium impairs the glutamate-nitric oxide-cGMP pathway in cultured neurons and in rat brain in vivo: molecular mechanisms and implications for neuropathology. J. Inorg. Biochem. 87, 63–69. doi: 10.1016/S0162-0134(01)00316-6
Dutta, A., Ray, K., Singh, V. K., Vats, P., Singh, S. N., and Singh, S. B. (2008). L-carnitine supplementation attenuates intermittent hypoxia-induced oxidative stress and delays muscle fatigue in rats. Exp. Physiol. 93, 1139–1146. doi: 10.1113/expphysiol.2008.042465
Ellman, G. L. (1959). Tissue sulfhydryl groups. Arch. Biochem. Biophys. 82, 70–77. doi: 10.1016/0003-9861(59)90090-6
Floresco, S. B., Seamans, J. K., and Phillips, A. G. (1997). Selective roles for hippocampal, prefrontal cortical, and ventral striatal circuits in radial-arm maze tasks with or without a delay. J. Neurosci. 17, 1880–1890. doi: 10.1523/JNEUROSCI.17-05-01880.1997
Goo, M. J., Choi, S. M., Kim, S. H., and Ahn, B. O. (2012). Protective effects of acetyl-L-carnitine on neurodegenarative changes in chronic cerebral ischemia models and learning-memory impairment in aged rats. Arch. Pharm. Res. 35, 145–154. doi: 10.1007/s12272-012-0116-9
Hagen, T. M., Liu, J., Lykkesfeldt, J., Wehr, C. M., Ingersoll, R. T., Vinarsky, V., et al. (2002). Feeding acetyl-L-carnitine and lipoic acid to old rats significantly improves metabolic function while decreasing oxidative stress. Proc. Natl. Acad. Sci. U.S.A. 99, 1870–1875. doi: 10.1073/pnas.261708898
Han, S., Lemire, J., Appanna, V. P., Auger, C., Castonguay, Z., and Appanna, V. D. (2013). How aluminium, an intracellular ROS generator promotes hepatic and neurological diseases: the metabolic tale. Cell Biol. Toxicol. 29, 75–84. doi: 10.1007/s10565-013-9239-0
Harooni, H. E., Naghdi, N., Sepehri, H., and Rohani, A. H. (2009). The role of hippocampal nitric oxide (NO) on learning and immediate, short- and long-term memory retrieval in inhibitory avoidance task in male adult rats. Behav. Brain Res. 201, 166–172. doi: 10.1016/j.bbr.2009.02.011
Hodges, D. B. Jr., Lindner, M. D., Hogan, J. B., Jones, K. M., and Markus, E. J. (2009). Scopolamine induced deficits in a battery of rat cognitive tests: comparisons of sensitivity and specificity. Behav. Pharmacol. 20, 237–251. doi: 10.1097/FBP.0b013e32832c70f5
Ighodaro, O. M., and Akinloye, O. A. (2017). First line defence antioxidants-superoxide dismutase (SOD), catalase (CAT) and glutathione peroxidase (GPX): their fundamental role in the entire antioxidant defence grid. Alexandria J. Med. 54, 287–293. doi: 10.1016/j.ajme.2017.09.001
Kawahara, M. (2005). Effects of aluminium on the nervous system and its possible link with neurodegenerative diseases. J. Alzheimers Dis. 8, 115–171. doi: 10.3233/JAD-2005-8210
Kumar, A., Prakash, A., and Dogra, S. (2011). Neuroprotective effect of carvedilol against aluminium induced toxicity: possible behavioral and biochemical alterations in rats. Pharmacol. Rep. 63, 915–923. doi: 10.1016/S1734-1140(11)70607-7
LeBel, C. P., and Bondy, S. C. (1991). Oxygen radicals: common mediators of neurotoxicity. Neurotoxicol. Teratol. 13, 341–346. doi: 10.1016/0892-0362(91)90081-7
Lee, B.-J., Lin, J.-S., Lin, Y.-C., and Lin, P.-T. (2014). Effects of L-carnitine supplementation on oxidative stress and antioxidant enzymes activities in patients with coronary artery disease: a randomized, placebo-controlled trial. Nutr. J. 13:79. doi: 10.1186/1475-2891-13-79
Levin, E. D. (2015). Learning about cognition risk with the radial-arm maze in the developmental neurotoxicology battery. Neurotoxicol. Teratol. 52, 88–92. doi: 10.1016/j.ntt.2015.05.007
Li, J.-L., Wang, Q.-Y., Luan, H.-Y., Kang, Z.-C., and Wang, C.-B. (2012). Effects of L-carnitine against oxidative stress in human hepatocytes: involvement of peroxisome proliferator-activated receptor alpha. J. Biomed. Sci. 19:32. doi: 10.1186/1423-0127-19-32
Ma, L., Liu, J., Li, N., Wang, J., Duan, Y., Yan, J., et al. (2010). Oxidative stress in the brain of mice caused by translocated nanoparticulate TiO2 delivered to the abdominal cavity. Biomaterials 31, 99–105. doi: 10.1016/j.biomaterials.2009.09.028
Miki, W. (1991). Biological functions and activities of animal carotenoids. Pure Appl. Chem. 63, 141–146. doi: 10.1351/pac199163010141
Nelson, N. (1999). Metal ion transporters and homeostasis. EMBO J. 18, 4361–4371. doi: 10.1093/emboj/18.16.4361
Niehaus, W. G. Jr., and Samuelsson, B. (1968). Formation of malonaldehyde from phospholipid arachidonate during microsomal lipid peroxidation. Eur. J. Biochem. 6, 126–130. doi: 10.1111/j.1432-1033.1968.tb00428.x
Orihuela, D., Meichtry, V., Pregi, N., and Pizarro, M. (2005). Short-term oral exposure to aluminium decreases glutathione intestinal levels and changes enzyme activities involved in its metabolism. J. Inorg. Biochem. 99, 1871–1878. doi: 10.1016/j.jinorgbio.2005.06.029
Pei, L. P., Dong, F. H., and Hui, B. D. (2008). Effects of astaxanthin on the damage of osteoblast induced by H2O2. Zhongguo Gu Shang 21, 187–189.
Rani, P. J., and Panneerselvam, C. (2002). Effect of L-carnitine on brain lipid peroxidation and antioxidant enzymes in old rats. J. Gerontol. A Biol. Sci. Med. Sci. 57, B134–B137. doi: 10.1093/gerona/57.4.B134
Ritter, A., and Cummings, J. (2015). Fluid biomarkers in clinical trials of Alzheimer’s disease therapeutics. Front. Neurol. 6:186. doi: 10.3389/fneur.2015.00186
Sinclair, C., Gilchrist, J. M., Hennessey, J. V., and Kandula, M. (2005). Muscle carnitine in hypo- and hyperthyroidism. Muscle Nerve 32, 357–359. doi: 10.1002/mus.20336
Singh, T., and Goel, R. K. (2015). Neuroprotective effect of Allium cepa L. in aluminium chloride induced neurotoxicity. Neurotoxicology 49, 1–7. doi: 10.1016/j.neuro.2015.04.007
Singla, N., and Dhawan, D. K. (2014). Zinc modulates aluminium-induced oxidative stress and cellular injury in rat brain. Metallomics 6, 1941–1950. doi: 10.1039/c4mt00097h
Sitta, A., Barschak, A. G., Deon, M., de Mari, J. F., Barden, A. T., Vanzin, C. S., et al. (2009). L-carnitine blood levels and oxidative stress in treated phenylketonuric patients. Cell. Mol. Neurobiol. 29, 211–218. doi: 10.1007/s10571-008-9313-y
Sun, X. L., Ito, H., Mssuoka, T., Kamei, C., and Hatano, T. (2007). Effect of Polygala tenuifolia root extract on scopolamine-induced impairment of rat spatial cognition in an eight-arm radial maze task. Biol. Pharm. Bull. 30, 1727–1731. doi: 10.1248/bpb.30.1727
Terragon, E., Ros-Bernal, F., Yuste, J. E., Ortiz-Cullera, V., Martin, E., Schenker, E., et al. (2012). The Radial Arm Maze (RAM) for the evaluation of working and reference memory deficits in the diurnal rodent Octodon degus. Proc. Meas. Behav. 98, 98–100.
Tracey, W. R., Tse, J., and Carter, G. (1995). Lipopolysaccharide-induced changes in plasma nitrite and nitrate concentrations in rats and mice: pharmacological evaluation of nitric oxide synthase inhibitors. J. Pharmacol. Exp. Ther. 272, 1011–1015.
Vaishnav, R. A., Singh, I. N., Miller, D. M., and Hall, E. D. (2010). Lipid peroxidation-derived reactive aldehydes directly and differentially impair spinal cord and brain mitochondrial function. J. Neurotrauma. 27, 1311–1320. doi: 10.1089/neu.2009.1172
Witko-Sarsat, V., Friedlander, M., Capeillere-Blandin, C., Nguyen-khoa, T., Nguyen, A. T., Zingraff, J., et al. (1996). Advanced oxidation protein products as a novel marker of oxidative stress in uremia. Kidney Int. 49, 1304–1313. doi: 10.1038/ki.1996.186
Wu, Z., Du, Y., Xue, H., Wu, Y., and Zhou, B. (2012). Aluminium induces neurodegeneration and its toxicity arises from increased iron accumulation and reactive oxygen species (ROS) production. Neurobiol. Aging 33:199.e1–12. doi: 10.1016/j.neurobiolaging.2010.06.018
Keywords: levocarnitine, Alzheimer’s disease, working memory, neurotoxicity, oxidative stress markers, antioxidants
Citation: Al-Amin MM, Chowdury MIA, Saifullah ARM, Alam MN, Jain P, Hossain M, Alam MA, Kazi M, Ahmad A, Raish M, Alqahtani A and Reza HM (2019) Levocarnitine Improves AlCl3-Induced Spatial Working Memory Impairment in Swiss albino Mice. Front. Neurosci. 13:278. doi: 10.3389/fnins.2019.00278
Received: 06 December 2018; Accepted: 08 March 2019;
Published: 26 March 2019.
Edited by:
Efthimios M. C. Skoulakis, Alexander Fleming Biomedical Sciences Research Center, GreeceReviewed by:
Sandeep Kumar Barodia, University of Alabama at Birmingham, United StatesGhulam Md Ashraf, King Abdulaziz University, Saudi Arabia
Copyright © 2019 Al-Amin, Chowdury, Saifullah, Alam, Jain, Hossain, Alam, Kazi, Ahmad, Raish, Alqahtani and Reza. This is an open-access article distributed under the terms of the Creative Commons Attribution License (CC BY). The use, distribution or reproduction in other forums is permitted, provided the original author(s) and the copyright owner(s) are credited and that the original publication in this journal is cited, in accordance with accepted academic practice. No use, distribution or reproduction is permitted which does not comply with these terms.
*Correspondence: Mohsin Kazi, bWthemlAa3N1LmVkdS5zYQ== Hasan Mahmud Reza, aGFzYW4ucmV6YUBub3J0aHNvdXRoLmVkdQ==