- 1Department of Psychology, University of Greifswald, Greifswald, Germany
- 2Department of Psychology, The Ohio State University, Columbus, OH, United States
- 3Section for Translational Psychobiology in Child and Adolescent Psychiatry, Department of Child and Adolescent Psychiatry, Centre for Psychosocial Medicine, University of Heidelberg, Heidelberg, Germany
- 4University Hospital of Child and Adolescent Psychiatry and Psychotherapy, University of Bern, Bern, Switzerland
Resting state vagally mediated heart rate variability (vmHRV) is related to difficulties in emotion regulation (ER). The prefrontal cortex (PFC) provides inhibitory control over the amygdala during ER. Previous studies linked vmHRV with activity in the ventromedial PFC (vmPFC) during implicit ER. To date no study examined the relation between vmHRV and brain activity during explicit ER. vmHRV was measured during a 7 min baseline at T1 2–5 days preceding T2. At T2 n = 24 participants (50% female, Mage = 24.6 years) viewed neutral or emotional pictures of pleasant or unpleasant valence and were instructed to intensify or to reduce their present emotion using two ER strategies (reappraisal and response modulation) or to passively view the picture. Participants rated the valence of their emotional state from pleasant to unpleasant after ER. Whole-brain fMRI data were collected using a 1.5-T-scanner. We observed an association between resting state vmHRV and brain activation in the PFC and the amygdala during ER of unpleasant emotions. Groups based on vmHRV showed significant differences in the modulation of amygdala activity as a function of ER strategy. In participants with high vmHRV amygdala activity was modulated only when using reappraisal and for low vmHRV participants only when using response modulation. Similar, dorsomedial PFC activity in high vmHRV participants was increased when using reappraisal and in low vmHRV participants when using response modulation to regulate unpleasant emotions. These results suggest that individuals with low vmHRV might have difficulties in recruiting prefrontal brain areas necessary for the modulation of amygdala activity during explicit ER.
Introduction
Emotion regulation (ER) can be defined as “the evocation of thoughts or behaviors that influence which emotions people have, when people have them, and how people experience or express these emotions” (Gross, 1998b). A host of brain imaging studies suggest that increased activation of the prefrontal cortex (PFC) is essential for ER (Beauregard et al., 2001; Ochsner and Gross, 2005; Wager et al., 2008). The PFC is theorized to have an inhibitory top down control over the amygdala during explicit (intentional) ER (Ochsner et al., 2002; Urry et al., 2006).
Resting state vagally-mediated heart rate variability (vmHRV), reflecting the potential for fast parasympathetic modulation of autonomic control of the heart, is inversely related to affective instability in daily life (Koval et al., 2013), and inversely correlated with self-reports on difficulties in ER (Berna et al., 2014; Beauchaine, 2015; Williams et al., 2015). Recent research suggests a relationship between brain activity and vmHRV, as higher resting state vmHRV is associated with stronger functional connectivity between the amygdala and the medial PFC (mPFC) across younger and older adults (Sakaki et al., 2016).
Importantly, in a series of studies on implicit (non-intentional) ER we have recently shown that (1) activity in ER related areas of the brain was positively correlated with coincident vmHRV when processing stimuli with its emotional significance in the attentional background (Lane et al., 2013); (2) that these relationships were absent in depression but increased and were similar to non-depressed controls after 12 week treatment with sertraline (Smith et al., 2014); and (3) that mPFC connectivity with the pons was negatively associated with depressive symptoms and positivity associated with coincident vmHRV when processing emotional stimuli with a non-emotional focus (Smith et al., 2015). These studies suggest that vmHRV and brain activity co-vary during implicit ER and that individual differences exist such that these associations are reduced or absent in persons with low vmHRV such as depressed patients (Kemp et al., 2010; Koenig et al., 2016).
While previous neuroimaging studies (e.g., Lane et al., 2013) demonstrated activity in the ventromedial PFC (vmPFC) during implicit (non-intentional) ER to be related to resting state vmHRV, the majority of neuroimaging studies on ER focused on explicit ER (i.e., intentional changes in affective state). However, surprisingly to date no study has examined the relationship between individual differences in resting vmHRV and brain activity during explicit ER.
Thus, the present study aimed to investigate the neural concomitants of explicit ER as a function of resting state vmHRV. Participants were trained in two ER strategies: reappraisal and response modulation and the neural activation during up- and down-regulation of emotions were measured. Resting state vmHRV was used to stratify participants into two groups. Based on previous findings it was hypothesized that individuals with high resting state vmHRV would exhibit differentiated activation of prefrontal brain regions and the amygdala according to the ER direction and that low resting state vmHRV would be related to less involvement of PFC structures and less modulation of the activation of the amygdala.
Materials and Methods1
Participants and General Procedures
Twenty-seven students participated in this investigation. Participants were selected if they were right-handed, had no current or prior mental disorders, and did not meet any MRI exclusion criteria, including metal in the body, claustrophobia, or pregnancy. Two participants did not complete the study due to schedule difficulties and one examination was discontinued due to technical problems. The final sample (n = 24) consisted of 12 female and 12 male participants, with a mean age of 24.6 years (range: 21–33). Participants received course credits or were paid an expense allowance of seven Euro. All participants gave written informed consent to the experiment approved by the University of Greifswald ethics committee. All procedures complied with the Declaration of Helsinki.
The investigation consisted of two parts: (1) a training session in the psychophysiological laboratory of the Institute of Psychology, 2–5 days prior to the experiment, and (2) the fMRI-experiment in the university clinic. During the training session participants practiced the two ER strategies and got familiar with the design of the study. Furthermore, their vmHRV was measured for 7 min at 1000 Hz in silence with a link belt, the Polar F5 Heart Rate Monitor Watch (Polar Electro Oy, Finland).
The fMRI-Session started with an introduction of the MRI-scanner and a medical check for participation by the clinic staff. The instructions for the ER strategies were repeated for each regulation direction and participants were asked to view all pictures attentively the whole time and not to close their eyes or move their head away from the picture. Pictures were projected on a tilted mirror mounted on the head coil.
Stimulus Material
Based on normative valence and arousal ratings and the general content, two comparable sets of 36 pleasant and 36 unpleasant pictures were selected from the International Affective Picture System (IAPS; Lang et al., 2005). Arousal ratings were balanced for pleasant (M = 5.60, SD = 0.99) and unpleasant (M = 5.83, SD = 0.69) stimuli. Two comparable sets of 12 neutral pictures were used as reference stimuli. For each participant, the two picture sets were randomly assigned to the two ER strategies and were presented in six pseudo-randomized orders across participants2.
To standardize the regulation of emotions, specific instructions were given for each strategy. Generally, participants were instructed not to replace one emotion by another but to intensify (increase) or to reduce (decrease) their present emotion or to just view the picture passively (maintain; cf. Ochsner and Gross, 2005). Participants were instructed to apply two ER strategies: Reappraisal and response modulation. Reappraisal involved the cognitive variation of the dimension distance-intimacy. To increase the emotion participants were instructed to reduce the distance to the depicted content by imagining to be either personally involved in the scene or indirectly via persons to whom they have a close relationship (e.g., friends, family, etc.). To decrease the emotion, distance was enhanced by imagining the picture as a simulation or by imagining being a casual bystander. Response modulation focused on changes of respiration, body tension and facial expressions, which were intensified to increase and reduced to decrease the emotion. Participants were also instructed to modulate the visibility of their emotions according to the regulation goal. In the increase condition a possible spectator should recognize the experienced emotion, whereas in the decrease condition a possible spectator should not be able to recognize which emotion is experienced.
Participants rated the valence of their emotional state from pleasant to unpleasant (range 1–5) after regulating their emotions using the Self-Assessment Manikin (SAM; Bradley and Lang, 1994). Since these ratings were recorded after each ER period, they can be considered as indicators of regulation success.
Experimental Paradigm
The experimental paradigm was a modified version from Eippert and co-workers (Eippert et al., 2007). It consisted of two experimental runs, one for each ER strategy. The strategy to be used first was balanced between participants. In each vmHRV group half (n = 6) of the participants started with reappraisal and the other half with response modulation. Every run was composed of 84 trials, 12 for each condition. The seven conditions reflected the seven regulation directions: pleasant-increase, pleasant-maintain, pleasant-decrease, neutral-maintain, unpleasant-increase, unpleasant-maintain, and unpleasant-decrease. All trials consisted of the following phases: induction, instruction, regulation and rating. Each trial began with the presentation of a picture for 2.5 s, which participants were instructed to view and to allow their emotional reactions to occur (induction phase). Then the instruction – equal sign (maintain), arrow up (increase), or arrow down (decrease) – appeared in the center of the picture, signaling the participants to regulate their emotions according to the practiced ER strategies during the training session. After 500 ms the instruction disappeared and the following 6 s were given for regulation with the picture still present. After the regulation phase SAM valence and arousal rating scales were presented for 3 s each and participants rated their current emotional experience. During an inter-trial-interval (8–10 s) a fixation cross was presented that signaled the participants to rest.
fMRI-Data Acquisition
Whole-brain fMRI data were collected using a 1.5 T scanner Magnetom Symphony system (Siemens) equipped with an 8-channel head coil. During the two regulation runs 506 functional T2∗-weighted images were acquired in transversal direction using echo-planar imaging (EPI) with a repetition time (TR) of 4 s, Field of view (FoV) = 192 mm, matrix = 128 × 128, flip angle = 90°, echo time (TE) = 38 ms). Each functional volume comprised 33 slices (voxel sixe: 3 × 1.5 × 1.5 mm). Between the two functional runs, a high-resolution anatomical T1-weighted scan was acquired with a TR of 11 ms (FoV = 256 mm, matrix = 256 × 256, TE = 5.2 ms, voxel size: 1 × 1 × 1 mm).
Data Analysis
The central 5 min of the recorded 7 min HR data were analyzed with HRV Analysis (Niskanen et al., 2004). The root mean square of successive differences (RMSSD) is thought to represent vmHRV and was used as time-domain measure of vmHRV (Task Force of the European Society of Cardiology and Task Force of the European Society of Cardiology, 1996). The RMSSD in milliseconds (ms) was extracted for each participant and further included in analysis with SPSS (SPSS IBM Statistics 22). High and low vmHRV groups were separated on basis of the median split (MD = 56.05; low vmHRV M = 37.49; high vmHRV M = 79.08), as illustrated in Figure 1. Groups did not differ on age [t(22) = 0.472, p = 0.642] or sex [χ(1) = 2.667, p = 0.102]. Further, there was no significant difference between groups on mean heart rate [low vmHRV: M = 74.26 bpm (11.11); high vmHRV: M = 67.89 bpm (7.93): t(22) = 1.62, p = 0.120]. Mean age (SD) was 25.3 (3.2) years in the low vmHRV group and 24.8 (1.9) in the high vmHRV group. Sex distribution was 4 women, 8 men in the low vmHRV and 8 women, 4 men in the high vmHRV group.
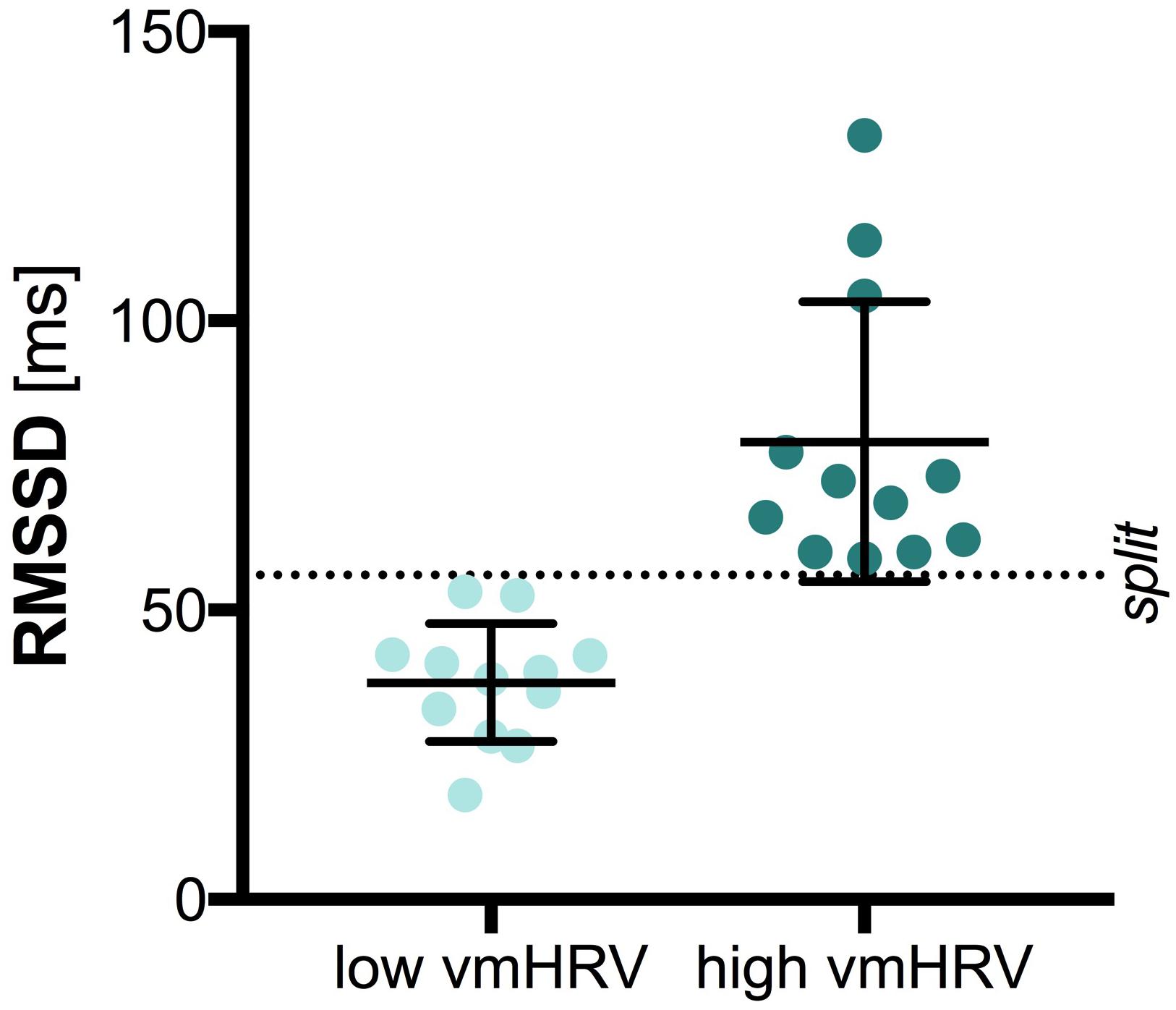
FIGURE 1. Vagally-mediated Heart Rate Variability by Group. Bars represent the mean and standard deviation. Dots represent individual participant data on the root mean square of successive differences (RMSSD) in milliseconds (ms); split: median split.
Valence ratings obtained after the regulation process were analyzed with a repeated measures analysis of variance (ANOVA) with the within-factors Strategy (reappraisal vs. response modulation), Valence (pleasant vs. unpleasant), and Direction (increase vs. maintain vs. decrease), and the between-factor vmHRV (low vs. high). Reporting of findings focusses on model fits and planned contrasts, adjusting for multiple testing using Bonferroni correction. Full reporting is provided in the Supplementary Table S1. All data were analyzed with SPSS (SPSS IBM Statistics 22). All results reported met a significance level of p < 0.05, unless otherwise noted. In case of violation of sphericity Greenhouse-Geisser corrected p-values are reported. Partial eta-squared () was used as measure of effect size, indicating the proportion of the total variance in a dependent variable explained by an independent variable while the effects of other independent variables and interactions are partialled out.
MRI-data pre-processing and statistical data analysis were performed with Statistical Parametric Mapping software (SPM8, Functional Imaging Laboratory, Wellcome Trust Centre for Neuroimaging, London, United Kingdom). The functional images of each subject were acquisition time corrected to the middle slice, realigned, co-registered to the anatomical image, segmented, and spatially normalized to a standard template of the Montreal Neurological Institute (MNI) and smoothed (FWHM 8 mm).
During first level analyses, for each participant a general linear model, as implemented in SPM8, was applied to the time-course of each voxel. The induction and regulation phase were modeled together using a boxcar function with a length of 9 s convolved with the hemodynamic responses function. The six movement parameters estimated during the realignment procedure were introduced as covariates into the model to control for variance caused by head movements (Johnstone et al., 2006). The resulting beta images were further analyzed on the second level in a full factorial model with the factors Strategy (reappraisal vs. response modulation), Valence (pleasant vs. unpleasant), and Direction (increase vs. maintain vs. decrease). The amygdala response to the experimental manipulation was quantified by means of the main effect of regulation direction. The prefrontal regions involved in ER were identified by means of the contrast ‘regulate (increase and decrease) vs. maintain.’
The time course of amygdala and prefrontal clusters exceeding a significance threshold of p < 0.001 (uncorrected) and an extend threshold of k = 5 were extracted using rfxplot (Gläscher, 2009) with spheres of a 3 mm radius centered around the individual peak activation within that cluster. Extracted scores were averaged for the 6 s of instructed ER and analyzed with SPSS (SPSS IBM Statistics 22) as described for valence ratings.
Results
Valence Ratings
As indicated by a significant 4-way interaction [Strategy × Valence × Direction × vmHRV: F(2,44): 4.77, p = 0.021, η2p = 0.18], the valence ratings of the participants current emotional state when using different ER strategies were influenced by vmHRV only when down-regulating unpleasant emotions. Compared to the corresponding maintain condition, participants with high vmHRV reported less unpleasant feelings after down-regulating their emotions using reappraisal [t(11) = 5.22, p < 0.001 for reappraisal; t(11) = 2.51, ns after bonferroni correction for response modulation]. In contrast, participants with low vmHRV reported a greater subjective reduction in unpleasantness when using response modulation [t(11) = 3.28, p = 0.007 for response modulation; t(11) = 2.25, ns after bonferroni correction for reappraisal; Strategy × Direction x vmHRV (unpleasant): F(2,44) = 5.49, p = 0.007, 0.20; see Figure 2]. No such effect of vmHRV was found for valence ratings after regulating emotions evoked by pleasant pictures (Strategy × Direction × HRV (pleasant): F < 1).
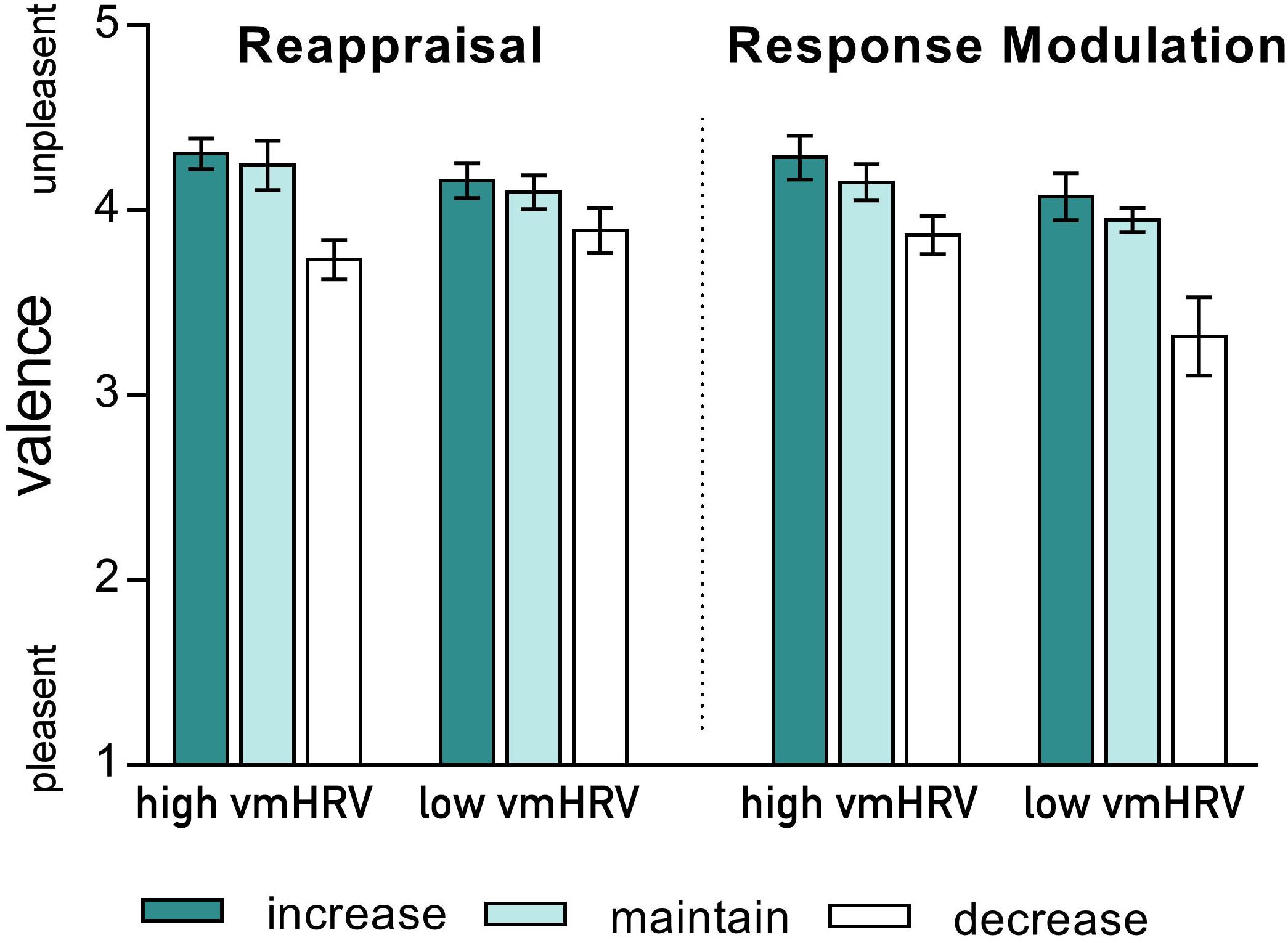
FIGURE 2. Valence ratings of the current emotional state after regulating emotions evoked by unpleasant pictures using either reappraisal (left) or response modulation (right) in participants with high and low resting state vagally mediated heart rate variability (vmHRV). Bars represent group means with standard errors.
Amygdala
Whole-brain analysis revealed a main effect of ER direction in clusters in both the right (MNI: x = 24, y = 4, z = -16; F = 7.36, puncorr < 0.001, kE = 5) and the left (MNI: x = -24, y = 2, z = -18; F = 12.51, puncorr < 0.001, kE = 15) amygdala.
As for valence ratings, resting vmHRV levels influenced right amygdala responses as a function of valence and regulation strategy (Strategy x Valence x Direction x vmHRV: F(2,44): 3.11, p = 0.06, = 0.12). That is, the differentiation, between increasing and decreasing their negative states was more pronounced for high vmHRV participants when using reappraisal (increase > decrease; t(11) = 3.95, p = 0.002) but not when using response modulation (t(11) = 0.21, ns) and for low vmHRV participants when using response modulation (t(11) = 4.57, p = 0.001) but not when using reappraisal (t(11) = 0.95, ns). No effects of resting vmHRV were found on right amygdala response during the regulation of emotions evoked by pleasant pictures (Direction x vmHRV: F(2,44) = 1.58, n.s.; Strategy x Direction x vmHRV: F < 1).
Resting vmHRV did not affect left amygdala responses during emotion regulation (Direction x vmHRV: F < 1), neither as a function of stimulus valence (Valence x Direction x vmHRV: F(2,44) = 2.29, ns) nor as a function of regulation strategy (Strategy x Direction x vmHRV: F(2,44) = 1.01, ns) or both (Strategy × Valence × Direction × vmHRV: F < 1).
Prefrontal Cortex
Whole-brain analysis revealed five clusters in the dorsolateral PFC and one cluster in the dorsomedial PFC showing a more pronounced response to conditions in which participants were instructed to regulate their emotions (Regulate > Maintain; see Table 1).
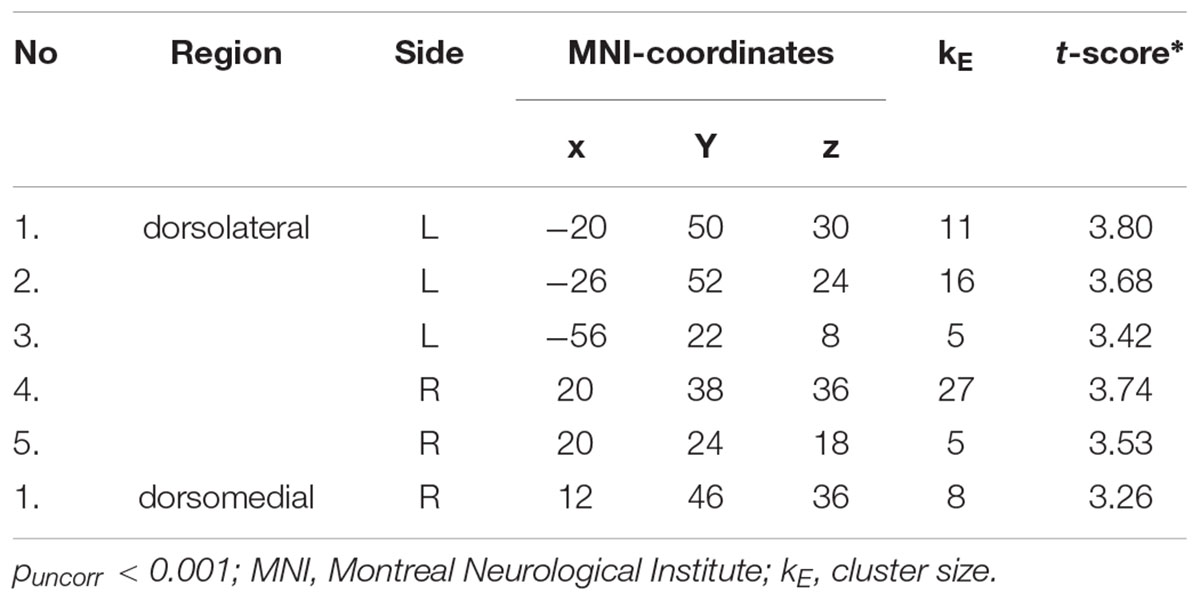
TABLE 1. Prefrontal clusters showing more pronounced activity during regulation conditions (increasing and decreasing emotions) compared to maintain conditions.
Since an influence of resting vmHRV on amygdala activity was only found during regulating emotions evoked by unpleasant pictures, the subsequent analyses of prefrontal regions were conducted for the negative valence category only. Resting vmHRV did not affect responses during ER in the five dorsolateral PFC clusters. In contrast, alike valence ratings and right amygdala responses, responses in the dorsomedial cluster (MNI: x = 12, y = 46, z = 36) during regulating emotions evoked by unpleasant pictures were influenced by resting vmHRV levels as a function of the used regulation strategy (Strategy x Direction x vmHRV: F(2,44): 3.59, p = .036, η2p = 0.14; see Figure 3). Compared to the corresponding maintain condition, participants with high vmHRV showed enhanced dorsomedial PFC activity while up-regulating unpleasant emotions using reappraisal [t(11) = 3.44, p = 0.005] but not when using response modulation [t(11) = 1.53, ns], whereas participants with low vmHRV showed no significant difference during reappraisal [t(11) = 1.66, ns] or response modulation [t(11) = 1.78, ns]. In contrast, low vmHRV participants showed more pronounced dorsomedial PFC activity while down-regulating unpleasant emotions using response modulation [t(11) = 2.97, p = 0.013] but not while using reappraisal [t(11) = 0.39, ns], whereas participants with high vmHRV showed no significant difference during response modulation [t(11) = -0.79, ns] or reappraisal [t(11) = 1.44, ns]. In direct comparison, low vmHRV participants showed more pronounced dorsomedial PFC activity while down-regulating unpleasant emotions using response modulation compared to high vmHRV participants [t(22) = 3.13, p = 0.005]. In sum, when regulating unpleasant emotions (mean of dorsomedial activity while up- and down-regulating) compared to maintaining emotions, high vmHRV participants showed significantly increased dorsomedial PFC activity when using reappraisal [t(11) = 2.38, p = 0.037] but not when using response modulation [t(11) = 0.70, ns]. In contrast, participants with low vmHRV showed significantly increased dorsomedial PFC activity when using response modulation [t(11) = 2.56, p = 0.026] but not when using reappraisal [t(11) = 1.10, ns] as ER strategy.
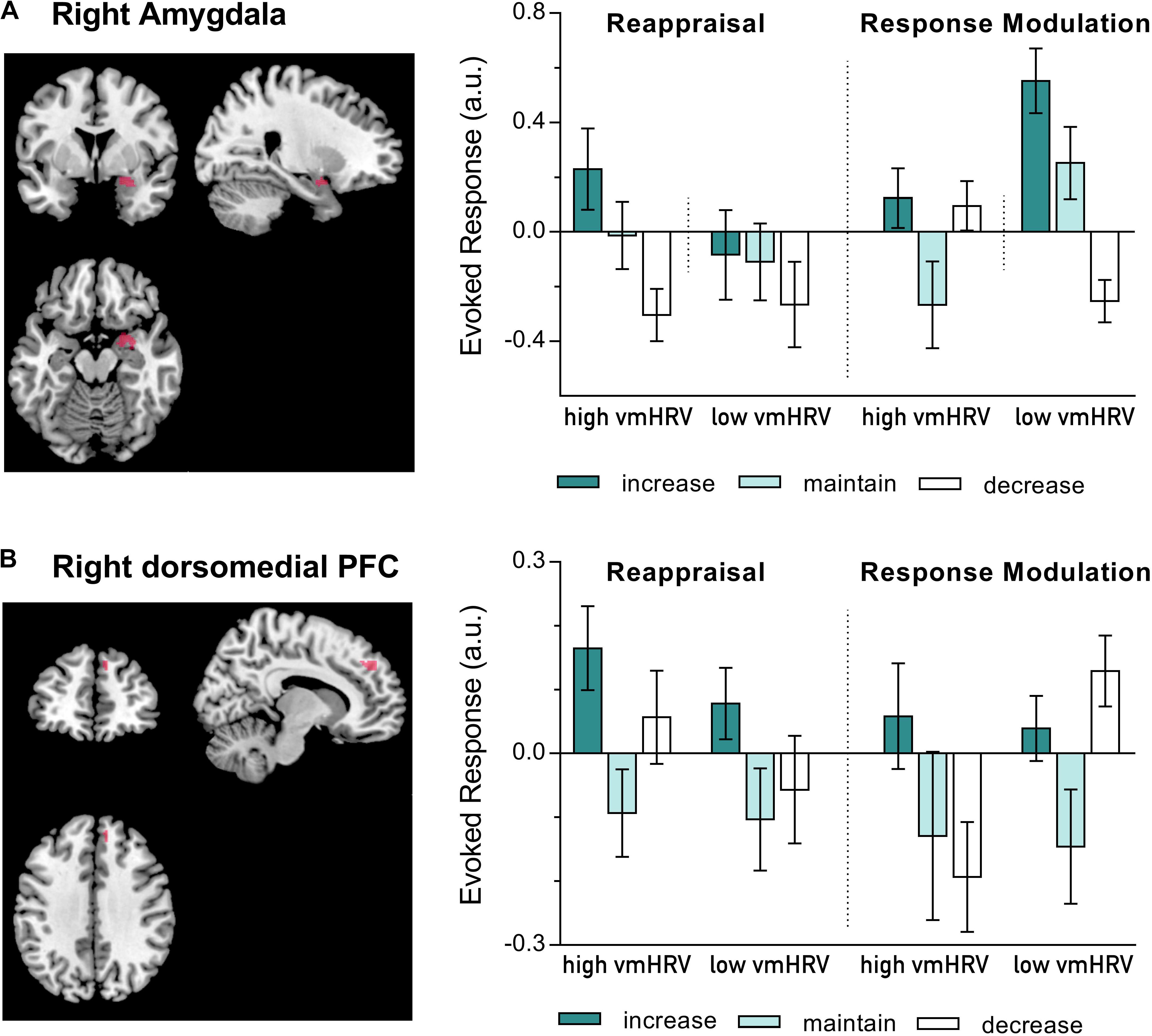
FIGURE 3. BOLD activation during the regulation of emotions evoked by unpleasant pictures using either reappraisal or response modulation in participants with high and low resting state vagally mediated heart rate variability (vmHRV). On the left side is the BOLD activity at puncorr = 0.001, k = 5, overlayed on a standard template (ch2better.nii.gz) using MRIcron (www.cabiatl.com/mricro/mricron). On the right side is the extracted data (individual spheres with a radius of 3 mm). Bars represent group means (arbitrary units) with standard errors. (A) Right amygdala activation (y = 2); (B) Right dorsomedial prefrontal cortex (PFC) activation (MNI: x = 10–12, y = 44–46, z = 36–38).
Discussion
This is the first study to combine functional imaging data of the explicit ER process and habitual resting state vmHRV data. We observed a differentiated modulation of the activation of the dorsomedial PFC and the amygdala according to the participants’ vmHRV. Thus these results further support recent findings on the relationship between resting state amygdala-PFC functional connectivity and vmHRV, and increase our understanding of the neural concomitants of vmHRV and its role in ER.
We observed that in participants with high vmHRV right amygdala activity was modulated according to the regulation direction (i.e., enhanced when instructed to increase and diminished when instructed to decrease) only when reappraisal was used to regulate unpleasant emotions. In contrast, in participants with low vmHRV the same modulation of amygdala activity was observed when response modulation was used to regulate unpleasant emotions. Similarly, only in participants with high vmHRV right dorsomedial PFC activity was increased when using reappraisal to regulate unpleasant emotions whereas participants with low vmHRV showed increased activation of the right dorsomedial PFC using response modulation to regulate unpleasant emotions.
These findings add to the existing findings on the neural concomitants of vmHRV during implicit ER (e.g., Lane et al., 2013), and provide further support for the Neurovisceral Integration Model (Thayer and Lane, 2000, 2009), which proposes that individuals with high resting state vmHRV are better able to inhibit prepotent emotional responses in the service of more desirable and appropriate ones in accordance with contextual factors. In line with this view, Geisler et al. (2010) have shown that vmHRV is positively associated with subjective well-being, and that this relationship is mediated by the habitual use of executive (i.e., conscious cognitive) ER strategies such as reappraisal. Therefore, our data support the findings by Geisler et al. (2010) showing that high vmHRV might be beneficial for the explicit cognitive regulation of emotions.
Most interestingly, participants with low vmHRV showed modulated activation of the right amygdala during the use of response modulation. Response modulation focuses on changes of respiration, body tension and facial expressions that do not rely on executive (prefrontal) recruitment to the same degree as reappraisal, which involves cognitive variation of the dimension distance-intimacy to the depicted content. Differences in the executive recruitment between those with low and high vmHRV might explain modulated activation of the amygdala only during the use of response modulation in those with low vmHRV, while those with high vmHRV showed modulated activation of the amygdala during reappraisal.
Indeed, our data show that the dorsomedial PFC seems to play an important role in the top-down modulation of the amygdala activation. Whereas only participants with high vmHRV showed enhanced activation of the right dorsomedial PFC using reappraisal to regulate unpleasant emotions and enhanced modulated activation of the amygdala; participants with low vmHRV showed enhanced activation of the right dorsomedial PFC using response modulation to regulate unpleasant emotions and enhanced modulated activation of the amgydala. Differences with respect to the ER-direction between individuals with high and low vmHRV, are potentially related to habitual experiences. Given that lower levels of vmHRV are associated with habitually increased experiences of negative affect (Sloan et al., 2017), the prefrontal cortex results may reflect the greater effort needed to act against one’s habits, i.e., for low vmHRV participants to decrease and for high vmHRV to increase experiences of unpleasant states. Taken together these findings suggest, that those with low vmHRV have particular difficulties in conscious, cognitive ER (i.e., reappraisal), that involve the recruitment of executive brain areas necessary for the top-down modulation of the amygdala. These findings support the hypothesis that greater resting vmHRV may reflect the efficiency of the prefrontal cortex to regulate amygdala activity in the service of ER.
In line with these observations, we have recently shown that higher vmHRV is associated with stronger resting state functional connectivity between the amygdala and the mPFC (Sakaki et al., 2016). Whereas this association was present independent of age, we also found age-related differences in the amygdala’s functional connectivity associated with vmHRV. Compared to older adults, we found that the functional connectivity between the amygdala and the ventrolateral PFC (vlPFC) was more strongly correlated with HRV in younger adults (Sakaki et al., 2016). In line with these findings it has previously been shown that during (implicit) ER older and younger adults spontaneously recruit the mPFC (Nashiro et al., 2012), while in addition to the mPFC younger adults also recruit the vlPFC during (explicit) ER (Winecoff et al., 2011). Clearly, future studies addressing age related differences in the neural concomitants of implicit and explicit ER as a function of resting state vmHRV are needed.
Existing research implicates dlPFC regions in voluntary ER and vmPFC regions (including the anterior cingulate cortex, ACC) in automatic – more habitual – ER (Phillips et al., 2008; Silvers et al., 2015). Therefore, it is suggested that explicit ER involves greater lateral PFC recruitment (Gyurak et al., 2011), a brain region that shows thinning with increasing age (Fjell et al., 2009). Greater connectivity between vmPFC and amygdala has been shown in older adults even when not told to use explicit ER, suggesting that explicit ER strategies have become habitual and automatic (Ochsner et al., 2012). Here we have shown in line with a large body of research (Ochsner et al., 2012), that explicit ER leads to enhanced activation of the dlPFC. The lack of association of vmHRV with activity of the dlPFC suggests a particular association with vmHRV and implicit – automatic – ER. However, we found vmHRV related to activation of the dorsomedial PFC during explicit ER. As noted above, this finding suggests that explicit ER strategies that require enhanced recruitment of PFC regions are related to vmHRV. In particular, the present results show that activation of the right dorsomedial PFC significantly varied between participants with low and high vmHRV according to the ER direction and strategy. Future research is needed to fully explicate the associations of vmHRV with both implicit and explicit ER.
Differences might also arise as a result of the use of different explicit ER strategies. Most fMRI studies on explicit ER focused on the down-regulation of unpleasant emotions with reappraisal (i.e., the cognitive change of the meaning of a picture; e.g., Ochsner et al., 2002; Eippert et al., 2007; Goldin et al., 2008), or suppression (i.e., the inhibition of an ongoing emotion-expressive behavior; e.g., Gross, 1998a,b; Goldin et al., 2008). While our implementation of reappraisal is consistent with these prior studies, our implementation of response modulation is much broader than that of suppression. Here we instructed participants to suppress or enhance their facial expressions or to influence their physiological responses by increasing or decreasing their respiration or their muscle tension. Particularly the regulation of respiration might account for the effectiveness of response modulation in participants with low vmHRV. Respiration patterns have been shown to reflect the general dimensions of the emotional response (Boiten et al., 1994), in particular the arousal dimension of self-reported emotions (Nyklíček et al., 1997). Previous studies comparing the two ER strategies of physiological suppression and expressive suppression (Dan-Glauser and Gross, 2011) found that both ER strategies, although targeting different responses, have very similar effects. Therefore, it might be possible that response modulation in our study was a multidimensional explicit ER strategy, which encompassed the manipulation of one’s facial expression, breathing frequency, and body tension. Future research is needed to clarify whether participants with low vmHRV are indeed more effective in non-cognitive ER strategies focusing on manipulations of the breathing frequency.
Finally, the study has several limitations that need to be addressed. vmHRV was recorded using an ambulatory HR monitor (Polar F5 Heart Rate Monitor Watch). Although devices from the respective manufacturer (Polar Electro Oy, Finland) are widely applied for recordings of HRV and show good reliability (e.g., Gamelin et al., 2006; Porto and Junqueira, 2009; Giles et al., 2016), multiple-lead ECG recordings are of better quality and more robust to potential artifacts (i.e., movement). Further, we didn’t implement restrictions regarding physical activity, caffeine intake and smoking prior to recordings of HRV and didn’t control for time of day of the recordings. Future studies, aiming to replicate the present findings, should implement ECG recordings with a more restrictive protocol in the assessment of HRV. We limited our analyses to a time-domain measure of vmHRV (RMSSD), that has been shown to be less affected by respiration and shows greater trait specificity under spontaneous breathing conditions compared to other HRV indices such as spectrally derived high-frequency HRV (Penttilä et al., 2001; Hill et al., 2009; Bertsch et al., 2012). Further, we didn’t control or adjust for levels of mean HR (for a review of the ongoing discussion see de Geus et al., 2018).
Finally, the present analyses focused on group differences between individuals with high and low vmHRV based on a median split. Future studies in larger samples would do well to address a (potential) continuous association between vmHRV and brain function during ER. Such analyses might further address if there are particular levels of vmHRV that predict differences in brain activity during ER. The present approach is justified to a certain degree, given that evidence on altered HRV in individuals with difficulties in ER (i.e., patients with depression), is mainly based on case-control studies – illustrating HRV differences on a group level. It was beyond the scope of the present study to address actual heart-brain interaction during explicit ER. Beyond the present approach, linking resting state vmHRV to brain function during explicit ER, future studies should address how resting vmHRV in turn relates to changes in cardiac activity during ER and how these are related to brain function drawing on recent linear and non-linear techniques for the assessment of heart-brain coupling (e.g., Valenza et al., 2016).
With respect to the fMRI assessments, scanner noise may impact affective states (i.e., Jacob et al., 2015). Similar to other research in the domain, the present study faces these challenges. However, experimental conditions between groups based on vmHRV were identical. Thus, it is unlikely that scanner noise had an impact on the present findings. vmHRV differs by sex (Koenig and Thayer, 2016), such that females show greater vmHRV compared to males. Future studies should address potential sex differences in the association between vmHRV and neural activity during ER, as the present sample was too small to investigate this factor.
Summary
In conclusion, our results replicate and extend findings from neuroimaging studies on ER and significantly add to the literature by showing that different levels of resting state vmHRV predict different patterns of neural activity during explicit ER. In line with the process model of ER (Gross, 1998b), we observed enhanced activation of prefrontal brain structures associated with emotional control whereas activation of the amygdala was modulated according to the ER direction. Our data provide first evidence for neural differences during explicit ER using different ER strategies as a function of resting state vmHRV. Participants with high vmHRV only show modulated activation of the right amygdala during the use of reappraisal whereas participants with low vmHRV show modulated activation of the right amygdala only during the use of response modulation. Further, we found increased activation of the dorsomedial PFC in participants with high vmHRV when regulating unpleasant emotions with reappraisal and in participants with low vmHRV when regulating unpleasant emotions when using response modulation. Future studies need to address age related differences in the neural concomitants of vmHRV during explicit ER and should aim to replicate these findings in samples with difficulties in ER (i.e., depressed patients). As habitual ER strategies have been shown to be related to vmHRV (Geisler et al., 2010) and brain activity when viewing emotional stimuli (e.g., Abler et al., 2010), future studies should also address how habitual ER strategies relate to the present findings. Adding to existing findings on the neural concomitants of vmHRV during implicit ER, the present findings support the Neurovisceral Integration Model (Thayer and Lane, 2000, 2009), suggesting that higher vmHRV is associated with neural mechanisms that support successful ER.
Author Contributions
ES, JW, and FG were involved in data collection, analysis and writing of the manuscript. AH and JT contributed to the critical design of the study. JK and JW wrote the first draft of the manuscript. All authors provided important intellectual content in revising the manuscript and approved the final version before submission.
Funding
JK was supported by a Physician-Scientist Fellowship provided by the Medical Faculty, Heidelberg University, Heidelberg, Germany, and a Post-Doctoral Scholarship provided by the Daimler and Benz Foundation (Ladenburg, Germany). ES was supported by a Doctoral Scholarship provided by the federal state of Mecklenburg-Vorpommern, Germany. The paper was previously published as part of the first authors PhD thesis (Steinfurth, 2017, Neurobiological Correlates of Emotion Regulation, Greifswald, available at https://epub.ub.uni-greifswald.de/home).
Conflict of Interest Statement
The authors declare that the research was conducted in the absence of any commercial or financial relationships that could be construed as a potential conflict of interest.
Supplementary Material
The Supplementary Material for this article can be found online at: https://www.frontiersin.org/articles/10.3389/fnins.2018.00794/full#supplementary-material
Footnotes
- ^The overall results of this analysis are reported in Steinfurth et al. (2013).
- ^The following randomized orders were generated: Order one started with a pleasant picture and the instruction to increase, order two started with a pleasant picture and the instruction to just view passively, order three started with a pleasant picture and the instruction to decrease the emotion, order four started with an unpleasant picture and the instruction to just view passively, order five started with an unpleasant picture and the instruction to decrease the appearing emotion and order six started with an unpleasant picture and the instruction to increase the emotion.
References
Abler, B., Hofer, C., Walter, H., Erk, S., Hoffmann, H., Traue, H. C., et al. (2010). Habitual emotion regulation strategies and depressive symptoms in healthy subjects predict fMRI brain activation patterns related to major depression. Psychiatry Res. 183, 105–113. doi: 10.1016/j.pscychresns.2010.05.010
Beauchaine, T. P. (2015). Future directions in emotion dysregulation and youth psychopathology. J. Clin. Child Adolesc. Psychol. 53, 875–896. doi: 10.1080/15374416.2015.1038827
Beauregard, M., Lévesque, J., and Bourgouin, P. (2001). Neural correlates of conscious self-regulation of emotion. J. Neurosci. 21:RC165.
Berna, G., Ott, L., and Nandrino, J.-L. (2014). Effects of emotion regulation difficulties on the tonic and phasic cardiac autonomic response. PLoS One 9:e102971. doi: 10.1371/journal.pone.0102971
Bertsch, K., Hagemann, D., Naumann, E., Schächinger, H., and Schulz, A. (2012). Stability of heart rate variability indices reflecting parasympathetic activity. Psychophysiology 49, 672–682. doi: 10.1111/j.1469-8986.2011.01341.x
Boiten, F. A., Frijda, N. H., and Wientjes, C. J. (1994). Emotions and respiratory patterns: review and critical analysis. Int. J. Psychophysiol. 17, 103–128. doi: 10.1016/0167-8760(94)90027-2
Bradley, M. M., and Lang, P. J. (1994). Measuring emotion: the self-assessment manikin and the semantic differential. J. Behav. Ther. Exp. Psychiatry 25, 49–59. doi: 10.1016/0005-7916(94)90063-9
Dan-Glauser, E. S., and Gross, J. J. (2011). The temporal dynamics of two response-focused forms of emotion regulation: experiential, expressive, and autonomic consequences. Psychophysiology 48, 1309–1322. doi: 10.1111/j.1469-8986.2011.01191.x
de Geus, E. J. C., Giannaros, P. J., Brindle, R. C., Jennings, R. J., and Berntson, G. G. (2018). Should heart rate variability be “corrected” for heart rate? Biological, quantitative, and interpretive considerations. Psychophysiology (in press).
Eippert, F., Veit, R., Weiskopf, N., Erb, M., Birbaumer, N., and Anders, S. (2007). Regulation of emotional responses elicited by threat-related stimuli. Hum. Brain Mapp. 28, 409–423. doi: 10.1002/hbm.20291
Fjell, A. M., Westlye, L. T., Amlien, I., Espeseth, T., Reinvang, I., Raz, N., et al. (2009). High consistency of regional cortical thinning in aging across multiple samples. Cereb. Cortex 19, 2001–2012. doi: 10.1093/cercor/bhn232
Gamelin, F. X., Berthoin, S., and Bosquet, L. (2006). Validity of the polar S810 heart rate monitor to measure R-R intervals at rest. Med. Sci. Sports Exerc. 38, 887–893. doi: 10.1249/01.mss.0000218135.79476.9c
Geisler, F. C. M., Vennewald, N., Kubiak, T., and Weber, H. (2010). The impact of heart rate variability on subjective well-being is mediated by emotion regulation. Pers. Individ. Dif. 49, 723–728. doi: 10.1016/j.paid.2010.06.015
Giles, D., Draper, N., and Neil, W. (2016). Validity of the Polar V800 heart rate monitor to measure RR intervals at rest. Eur. J. Appl. Physiol. 116, 563–571. doi: 10.1007/s00421-015-3303-9
Gläscher, J. (2009). Visualization of group inference data in functional neuroimaging. Neuroinformatics 7, 73–82. doi: 10.1007/s12021-008-9042-x
Goldin, P. R., McRae, K., Ramel, W., and Gross, J. J. (2008). The neural bases of emotion regulation: reappraisal and suppression of negative emotion. Biol. Psychiatry 63, 577–586. doi: 10.1016/j.biopsych.2007.05.031
Gross, J. J. (1998a). Antecedent- and response-focused emotion regulation: divergent consequences for experience, expression, and physiology. J. Pers. Soc. Psychol. 74, 224–237. doi: 10.1037/0022-3514.74.1.224
Gross, J. J. (1998b). The emerging field of emotion regulation: an integrative review. Rev. Gen. Psychol. 2, 271–299. doi: 10.1037/1089-2680.2.3.271
Gyurak, A., Gross, J. J., and Etkin, A. (2011). Explicit and implicit emotion regulation: a dual-process framework. Cogn. Emot. 25, 400–412. doi: 10.1080/02699931.2010.544160
Hill, L. K., Siebenbrock, A., Sollers, J. J. III, and Thayer, J. F. (2009). Are all measures created equal? Heart rate variability and respiration. Biomed. Sci. Instrum. 45, 71–76.
Jacob, S. N., Shear, P. K., Norris, M., Smith, M., Osterhage, J., Strakowski, S. M., et al. (2015). Impact of fMRI scanner noise on affective state and attentional performance. J. Clin. Exp. Neuropsychol. 37, 563–570. doi: 10.1080/13803395.2015.1029440
Johnstone, T., Ores Walsh, K. S., Greischar, L. L., Alexander, A. L., Fox, A. S., Davidson, R. J., et al. (2006). Motion correction and the use of motion covariates in multiple-subject fMRI analysis. Hum. Brain Mapp. 27, 779–788. doi: 10.1002/hbm.20219
Kemp, A. H., Quintana, D. S., Gray, M. A., Felmingham, K. L., Brown, K., and Gatt, J. M. (2010). Impact of depression and antidepressant treatment on heart rate variability: a review and meta-analysis. Biol. Psychiatry 67, 1067–1074. doi: 10.1016/j.biopsych.2009.12.012
Koenig, J., Kemp, A. H., Beauchaine, T. P., Thayer, J. F., and Kaess, M. (2016). Depression and resting state heart rate variability in children and adolescents - A systematic review and meta-analysis. Clin. Psychol. Rev. 46, 136–150. doi: 10.1016/j.cpr.2016.04.013
Koenig, J., and Thayer, J. F. (2016). Sex differences in healthy human heart rate variability: a meta-analysis. Neurosci. Biobehav. Rev. 64, 288–310. doi: 10.1016/j.neubiorev.2016.03.007
Koval, P., Ogrinz, B., Kuppens, P., Van den Bergh, O., Tuerlinckx, F., and Sütterlin, S. (2013). Affective instability in daily life is predicted by resting heart rate variability. PLoS One 8:e81536. doi: 10.1371/journal.pone.0081536
Lane, R. D., Weidenbacher, H., Smith, R., Fort, C., Thayer, J. F., and Allen, J. J. B. (2013). Subgenual anterior cingulate cortex activity covariation with cardiac vagal control is altered in depression. J. Affect. Disord. 150, 565–570. doi: 10.1016/j.jad.2013.02.005
Lang, P. J., Bradley, M. M., and Cuthbert, B. N. (2005). International Affective Picture System (IAPS): Affective Ratings of Pictures and Instruction Manual. Gainesville: University of Florida.
Nashiro, K., Sakaki, M., and Mather, M. (2012). Age differences in brain activity during emotion processing: reflections of age-related decline or increased emotion regulation? Gerontology 58, 156–163. doi: 10.1159/000328465
Niskanen, J. P., Tarvainen, M. P., Ranta-Aho, P. O., and Karjalainen, P. A. (2004). Software for advanced HRV analysis. Comput. Methods Programs Biomed. 76, 73–81. doi: 10.1016/j.cmpb.2004.03.004
Nyklíček, I., Thayer, J. F., and Van Doornen, L. J. P. (1997). Cardiorespiratory differentiation of musically-induced emotions. J. Psychophysiol. 11, 304–321.
Ochsner, K. N., Bunge, S. A., Gross, J. J., and Gabrieli, J. D. E. (2002). Rethinking feelings: an FMRI study of the cognitive regulation of emotion. J. Cogn. Neurosci. 14, 1215–1229. doi: 10.1162/089892902760807212
Ochsner, K. N., and Gross, J. J. (2005). The cognitive control of emotion. Trends Cogn. Sci. 9, 242–249. doi: 10.1016/j.tics.2005.03.010
Ochsner, K. N., Silvers, J. A., and Buhle, J. T. (2012). Functional imaging studies of emotion regulation: a synthetic review and evolving model of the cognitive control of emotion. Ann. N. Y. Acad. Sci. 1251, E1–E24. doi: 10.1111/j.1749-6632.2012.06751.x
Penttilä, J., Helminen, A., Jartti, T., Kuusela, T., Huikuri, H. V., Tulppo, M. P., et al. (2001). Time domain, geometrical and frequency domain analysis of cardiac vagal outflow: effects of various respiratory patterns. Clin. Physiol. 21, 365–376. doi: 10.1046/j.1365-2281.2001.00337.x
Phillips, M., Ladouceur, C., and Drevets, W. (2008). A neural model of voluntary and automatic emotion regulation: implications for understanding the pathophysiology and neurodevelopment of bipolar disorder. Mol. Psychiatry 13, 829–857. doi: 10.1038/mp.2008.65
Porto, L. G., and Junqueira, L. F. Jr. (2009). Comparison of time-domain short-term heart interval variability analysis using a wrist-worn heart rate monitor and the conventional electrocardiogram. Pacing Clin. Electrophysiol. 32, 43–51. doi: 10.1111/j.1540-8159.2009.02175.x
Sakaki, M., Yoo, H. J., Nga, L., Lee, T.-H., Thayer, J. F., and Mather, M. (2016). Heart rate variability is associated with amygdala functional connectivity with MPFC across younger and older adults. Neuroimage 139, 44–52. doi: 10.1016/j.neuroimage.2016.05.076
Silvers, J. A., Wager, T. D., Weber, J., and Ochsner, K. N. (2015). The neural bases of uninstructed negative emotion modulation. Soc. Cogn. Affect. Neurosci. 10, 10–18. doi: 10.1093/scan/nsu016
Sloan, R. P., Schwarz, E., McKinley, P. S., Weinstein, M., Love, G., Ryff, C., et al. (2017). Vagally-mediated heart rate variability and indices of well-being: results of a nationally representative study. Health Psychol. 36, 73–81. doi: 10.1037/hea0000397
Smith, R., Allen, J. J. B., Thayer, J. F., Fort, C., and Lane, R. D. (2014). Increased association over time between regional frontal lobe BOLD change magnitude and cardiac vagal control with sertraline treatment for major depression. Psychiatry Res. 224, 225–233. doi: 10.1016/j.pscychresns.2014.08.015
Smith, R., Allen, J. J. B., Thayer, J. F., and Lane, R. D. (2015). Altered functional connectivity between medial prefrontal cortex and the inferior brainstem in major depression during appraisal of subjective emotional responses: a preliminary study. Biol. Psychol. 108, 13–24. doi: 10.1016/j.biopsycho.2015.03.007
Steinfurth, E. C. K. (2017). Neurobiological Correlates of Emotion Regulation. Dissertation at the University of Greifswald, Greifswald. Available at: urn:nbn:de:gbv:9-opus-22471
Steinfurth, E. C. K., Wendt, J., and Hamm, A. O. (2013). Neurobiologische grundlagen der emotionsregulation. Psychol. Rundsch. 64, 208–216. doi: 10.1026/0033-3042/a000173
Task Force of the European Society of Cardiology and Task Force of the European Society of Cardiology (1996). Heart rate variability: standards of measurement, physiological interpretation and clinical use. Task force of the European society of cardiology and the North American society of pacing and electrophysiology. Circulation 93, 1043–1065. doi: 10.1161/01.CIR.93.5.1043
Thayer, J. F., and Lane, R. D. (2000). A model of neurovisceral integration in emotion regulation and dysregulation. J. Affect. Disord. 61, 201–216. doi: 10.1016/S0165-0327(00)00338-4
Thayer, J. F., and Lane, R. D. (2009). Claude bernard and the heart—brain connection: further elaboration of a model of neurovisceral integration. Neurosci. Biobehav. Rev. 33, 81–88. doi: 10.1016/j.neubiorev.2008.08.004
Urry, H. L., van Reekum, C. M., Johnstone, T., Kalin, N. H., Thurow, M. E., Schaefer, H. S., et al. (2006). Amygdala and ventromedial prefrontal cortex are inversely coupled during regulation of negative affect and predict the diurnal pattern of cortisol secretion among older adults. J. Neurosci. 26, 4415–4425. doi: 10.1523/JNEUROSCI.3215-05.2006
Valenza, G., Toschi, N., and Barbieri, R. (2016). Uncovering brain–heart information through advanced signal and image processing. Philos. Trans. A Math. Phys. Eng. Sci. 374:20160020. doi: 10.1098/rsta.2016.0020
Wager, T. D., Davidson, M. L., Hughes, B. L., Lindquist, M. A., and Ochsner, K. N. (2008). Neural mechanisms of emotion regulation: evidence for two independent prefrontal-subcortical pathways. Neuron 59, 1037–1050. doi: 10.1016/j.neuron.2008.09.006
Williams, D. P., Cash, C., Rankin, C., Bernardi, A., Koenig, J., and Thayer, J. F. (2015). Resting heart rate variability predicts self-reported difficulties in emotion regulation: a focus on different facets of emotion regulation. Front. Psychol. 6:261. doi: 10.3389/fpsyg.2015.00261
Keywords: prefrontal cortex, amygdala, heart rate variability, emotion regulation, vagal
Citation: Steinfurth ECK, Wendt J, Geisler F, Hamm AO, Thayer JF and Koenig J (2018) Resting State Vagally-Mediated Heart Rate Variability Is Associated With Neural Activity During Explicit Emotion Regulation. Front. Neurosci. 12:794. doi: 10.3389/fnins.2018.00794
Received: 01 August 2018; Accepted: 15 October 2018;
Published: 05 November 2018.
Edited by:
Alberto Porta, Università degli Studi di Milano, ItalyReviewed by:
Roberto Maestri, IRCCS Istituti Clinici Scientifici Maugeri (ICS Maugeri), ItalyPhyllis Kravet Stein, Washington University in St. Louis, United States
Michela Masè, Università degli Studi di Trento, Italy
Copyright © 2018 Steinfurth, Wendt, Geisler, Hamm, Thayer and Koenig. This is an open-access article distributed under the terms of the Creative Commons Attribution License (CC BY). The use, distribution or reproduction in other forums is permitted, provided the original author(s) and the copyright owner(s) are credited and that the original publication in this journal is cited, in accordance with accepted academic practice. No use, distribution or reproduction is permitted which does not comply with these terms.
*Correspondence: Julian F. Thayer, thayer.39@osu.edu