- 1Department of Psychiatry, Faculty of Medicine, University of Szeged, Szeged, Hungary
- 2Department of Physiology, University of Szeged, Szeged, Hungary
- 3Department of Surgery and Cancer, Faculty of Medicine, Imperial College London, London, United Kingdom
Apart from its pivotal role in the regulation of carbohydrate metabolism, insulin exerts important neurotrophic and neuromodulator effects on dorsal root ganglion (DRG) neurons. The neurite outgrowth-promoting effect is one of the salient features of insulin’s action on cultured DRG neurons. Although it has been established that a significant population of DRG neurons express the insulin receptor (InsR), the significance of InsR expression and the chemical phenotype of DRG neurons in relation to the neurite outgrowth-promoting effect of insulin has not been studied. Therefore, in this study by using immunohistochemical and quantitative stereological methods we evaluated the effect of insulin on neurite outgrowth of DRG neurons of different chemical phenotypes which express or lack the InsR. Insulin, at a concentration of 10 nM, significantly increased total neurite length, the length of the longest neurite and the number of branch points of cultured DRG neurons as compared to neurons cultured in control medium or in the presence of 1 μM insulin. In both the control and the insulin exposed cultures, ∼43% of neurons displayed InsR-immunoreactivity. The proportions of transient receptor potential vanilloid type 1 receptor (TRPV1)-immunoreactive (IR), calcitonin gene-related peptide (CGRP)-IR and Bandeiraea simplicifolia isolectin B4 (IB4)-binding neurons amounted to ∼61%, ∼57%, and ∼31% of DRG neurons IR for the InsR. Of the IB4-positive population only neurons expressing the InsR were responsive to insulin. In contrast, TRPV1-IR nociceptive and CGRP-IR peptidergic neurons showed increased tendency for neurite outgrowth which was further enhanced by insulin. However, the responsiveness of DRG neurons expressing the InsR was superior to populations of DRG neurons which lack this receptor. The findings also revealed that besides the expression of the InsR, inherent properties of peptidergic, but not non-peptidergic nociceptive neurons may also significantly contribute to the mechanisms of neurite outgrowth of DRG neurons. These observations suggest distinct regenerative propensity for differing populations of DRG neurons which is significantly affected through insulin receptor signaling.
Introduction
Insulin, besides being essential in the regulation of glucose homeostasis, is involved in several neuronal processes such as neural survival and neurite outgrowth in both the central and the peripheral nervous system (Fernyhough et al., 1993; Wan et al., 1997; Barber et al., 2001; Stella et al., 2001; Gerozissis, 2003; Singh et al., 2012). Insulin exerts its neural actions through the insulin receptor (InsR), a member of the receptor tyrosine kinases family (Ward and Lawrence, 2009) present in nerve tissue (Havrankova et al., 1978; Unger et al., 1989). Further, it has also been revealed that a relatively high proportion of dorsal root ganglion (DRG) neurons express the InsR (Baiou et al., 2007; Lázár et al., 2018a,b).
Previous studies demonstrated that a unique population of C-fiber DRG neurons is sensitive to capsaicin and express the transient receptor potential vanilloid type 1 receptor (TRPV1) (Jancsó et al., 1977; Buck and Burks, 1986; Holzer, 1991; Caterina et al., 1997; Guo et al., 2001). These neurons are involved in pain sensation and, in a variety of organs, local regulatory functions, including neurogenic inflammation brought about by the release of sensory neuropeptides such as substance P (SP) and calcitonin gene-related peptide (CGRP) (Jancsó, 1960; Jancso et al., 1968, Jancsó et al., 1977, 2009; Maggi and Meli, 1988; Holzer, 1991; Nagy et al., 2004). These TRPV1-immunoreactive (IR) nociceptive DRG neurons comprise two major subpopulations: CGRP-containing peptidergic and Bandeiraea simplicifolia isolectin B4 (IB4)-binding non-peptidergic neurons (Silos-Santiago et al., 1995; Santha et al., 2010). It has also been shown that peptidergic and non-peptidergic sensory neurons have distinct sensitivities to neurotrophic factors such as nerve growth factor (NGF) and glial cell derived neurotrophic factor (GDNF) (Molliver et al., 1997).
Recent findings indicated that insulin may modulate the activation of nociceptive sensory neurons through indirectly opening the TRPV1 (Sathianathan et al., 2003; Van Buren et al., 2005; Lilja et al., 2007). Immunohistochemical studies have also revealed that a high proportion of InsR-IR DRG neurons show colocalization with TRPV1 and CGRP in rat and mouse DRG neurons (Baiou et al., 2007; Lázár et al., 2018a,b). It has also been suggested that an interplay among insulin, InsR and TRPV1 in nociceptive DRG neurons may contribute to the mechanisms of inflammatory processes (Razavi et al., 2006; Tsui et al., 2007; Lázár et al., 2018a,b).
Additionally, InsR signaling has been shown to affect neurite outgrowth in cultured sympathetic and sensory neurons, but the nature of change, an increase or decrease in neurite outgrowth is concentration dependent (Recio-Pinto et al., 1986; Fernyhough et al., 1993; Singh et al., 2012). Insulin signaling in sensory neurons has also been suggested to participate in pathophysiological alterations induced by diabetes. Available experimental evidence indicates that insulin not only promotes peripheral nerve regeneration in vivo (Xu et al., 2004; Toth et al., 2006), but may prevent diabetes-induced functional deficits, too (Singhal et al., 1997). Although these studies furnished evidence for a pro-regenerative effect of insulin on sensory neurons, a possible association of the neuronal phenotype with the neurite outgrowth-promoting effect of insulin has not been evaluated.
Therefore, the present study was initiated in an attempt to characterize the phenotypic traits of DRG neurons responsive to insulin’s neurite outgrowth-promoting action. To achieve this goal, selected parameters of neurite outgrowth of cultured DRG neurons which expressed the InsR, TRPV1, CGRP and/or bound the IB4 were assessed in the presence or absence of insulin with immunohistochemical and quantitative stereological methods.
Materials and Methods
All experiments were approved by the Ethics Committee for Animal Care of the University of Szeged and were carried out in full accordance with the Directive 2010/63/EU of the European Parliament and of the Council on the Protection of Animals Used for Scientific Purposes and the guidelines of the Committee for Research. All efforts were made to minimize animal suffering. The number of experimental animals was kept as low as possible.
Preparation of Cultures of DRG Neurons
Adult male Wistar rats weighing 300–350 g (n = 12) were terminally anesthetized and DRGs were removed under aseptic conditions. DRGs were collected in a culture medium (Dulbecco’s Modified Eagle’s Medium/Nutrient mixture F-12 HAM; Sigma-Aldrich, Gillingham, United Kingdom) containing 1 mM l-glutamine, 50 IU/ml penicillin, 50 μg/ml streptomycin, and 300 mg/dL glucose (all from Sigma-Aldrich) and 4% Ultroser G (BioSepra SA, Cergy, France) without insulin. DRG neuron cultures were prepared as described previously (Winter et al., 1988; Santha et al., 2010). Briefly, DRGs were incubated with collagenase type IV (2000 U/ml, Sigma-Aldrich) for 3 h at 37°C in a 5% CO2 incubator. DRGs were triturated with a fire-polished Pasteur pipette. The cell suspension was spun through 21% Percoll® (Sigma-Aldrich). Following re-suspension in the supplemented culture medium, DRG cells were plated on poly-DL-ornithine (Sigma-Aldrich) coated glass coverslips and kept at 37°C in a 5% CO2 atmosphere. Insulin (Sigma-Aldrich) was administered to the culture medium at concentrations of 10 nM and 1 μM for 48 h. The concentrations of insulin applied in the present experiments are similar to those found in commonly used serum-free media (cf. Chen et al., 2008), importantly, these concentrations of insulin were applied in previous studies as well (Recio-Pinto et al., 1986; Fernyhough et al., 1993; Singh et al., 2012). To determine the survival ratios of DRG neurons among each experimental group, the ethidium homodimer-1 assay for cell viability (LIVE/DEADTM Viability Kit; Invitrogen, Carlsbad, CA, United States) was performed.
Immunohistochemistry
DRG cultures were fixed with 4% paraformaldehyde in 0.1 M phosphate buffer (pH 7.4) for 10 min and then washed twice in phosphate-buffered isotonic NaCl solution (phosphate-buffered saline, PBS). In single and multiple labeling experiments, the following primary antibodies and lectins were used: mouse anti-β3-tubulin (Santa Cruz Biotechnologies, Dallas, TX, United States; 1:1500), rabbit polyclonal anti-InsRα subunit IgG (Santa Cruz Biotechnologies, 1:1500), polyclonal guinea pig anti-TRPV1 IgG (Neuromics, Edina, MN, United States; 1:1500), polyclonal goat anti-CGRP IgG (Santa Cruz Biotechnologies; 1:5000) and biotin-conjugated IB4 (Sigma-Aldrich, 1:1000). Primary antibodies were dissolved in PBS containing 0.3% Triton X-100 and applied to the cultures overnight at 4°C. After rinsing in PBS, the following secondary antibodies were applied: donkey anti-rabbit IgG labeled with DL488 (1:500), donkey anti-guinea pig IgG labeled with Cy3 (1:500) and AMCA-avidin conjugates were used as secondary antibodies (all from Jackson Immunoresearch Laboratories, West Grove, PA, United States). After incubation for 2 h, the specimens were rinsed in PBS and covered with Prolong Gold antifade mounting medium (Invitrogen). The selectivity and specificity of the polyclonal rabbit anti-TRPV1 and anti-InsR antisera (Santa Cruz Biotechnologies) were assessed in DRG sections by the lack of staining with the TRPV1 antibody in specimens obtained from TRPV1-/- mice and by the failure of staining with the InsR antibody after preincubation with the immunizing peptide supplied by the manufacturer (Baiou et al., 2007). Control procedures for CGRP immunolabeling was performed by replacing the primary antiserum with normal donkey serum. No immunostaining was observed in control experiments.
Quantitative Assessment of Neurite Outgrowth of Cultured DRG Neurons
Photomicrographs were taken with a Leica DMLB fluorescence microscope (Wetzlar, Germany) equipped with a Retiga 2000R digital camera (QImaging, Surrx, BC, Canada). In control and insulin-treated cultures, at least 30 neurons per culture were counted and analyzed. Data collection was performed blinded to the experimental conditions. Each image was analyzed using ImagePro Plus 7 image processing and analysis software (Media Cybernetics, Bethesda, MD, United States) and ImageJ image analysis software (NIH, Bethesda, MD, United States). Neurons exhibiting IB4 binding and immunoreactivity for the InsR, TRPV1, and CGRP or a combination of these molecular markers were counted. To assess the effect of insulin on neurite outgrowth, first, we examined the percentages of neurite-bearing DRG neurons in the control and the insulin-treated cultures. Thereafter, by using the ImageJ (NIH) simple neurite tracer program, we measured three quantified parameters of neurons with neurites longer than the soma diameter and clearly identifiable axonal arborization: total neurite length (sum of lengths of all neurites), maximum neurite length (length of longest neurite) and neurite branch points (number of neurite branch points).
Statistical Analysis
Data were collected from three to nine independent experiments. All data are presented as the mean ± standard error of the mean (SEM). To test the normality of the distribution of data in each experimental group, the Shapiro–Wilk test was applied. Statistical comparisons of data were performed with Fisher’s exact probability test or a one-way ANOVA with post hoc Tukey’s multiple comparison test, as appropriate. A p-value of less than 0.05 was considered to be statistically significant for all tests. Statistical analysis was made by using the Dell Statistica software (Dell Inc., Tulsa, OK, United States).
Results
Insulin Increases Neurite Outgrowth of Cultured Adult Rat DRG Neurons at Nanomolar Concentration
The morphology of cultured DRG neurons was studied in specimens stained with the mouse anti-β3-tubulin antibody. The survival ratios of DRG neurons were not significantly altered by insulin and the high glucose concentration of the medium as assessed with the ethidium homodimer-1 assay. The percentages of dead cells amounted to 3.01 ± 0.34%, 2.47 ± 0.21%, and 4.13 ± 0.18% in the cultures exposed to culture medium and insulin at a concentrations of 10 nM and 1 μM. The differences between each experimental groups were not significant (p < 0.05). These observations are in accord with previous findings showing the lack of a significant effect of insulin on neuronal counts in DRG cultures (Fernyhough et al., 1993). Similarly, the high concentration of glucose in the DMEM/F12 medium has been shown to induce oxidative stress and neuronal degeneration in cultured DRG neurons of streptozotocin diabetic but not control rats (Zherebitskaya et al., 2009).
Administration of insulin at concentrations of 10 nM and 1 μM significantly increased the percentages of neurite-bearing DRG neurons by 50.74 ± 2.52% and 26.86 ± 1.78%, respectively, as compared to controls (p < 0.05). The difference between the cultures exposed to 10 nM and 1 μM were also significant (p < 0.05). Neurite outgrowth was examined by analyzing three quantified parameters of neurons with neurites longer than the soma diameter and clearly identifiable axonal arborization: total neurite length (sum of lengths of all neurites), maximum neurite length (length of the longest neurite), and neurite branch points (number of neurite branch points). The results showed that administration of insulin at a concentration of 10 nM for 48 h increased total neurite length by 2.08-fold, maximum neurite length by 1.88-fold and neurite branch points by 2.35-fold as compared to control cultures (for all comparisons p < 0.05; Figures 1A–I). Administration of insulin, at a concentration of 1 μM, increased maximum neurite length by 1.64-fold (p < 0.05), whereas no significant changes were observed in total neurite length and neurite branch points (Figures 1A–I). Therefore, only the effects of insulin applied at a concentration of 10 nM were analyzed in detail in this study.
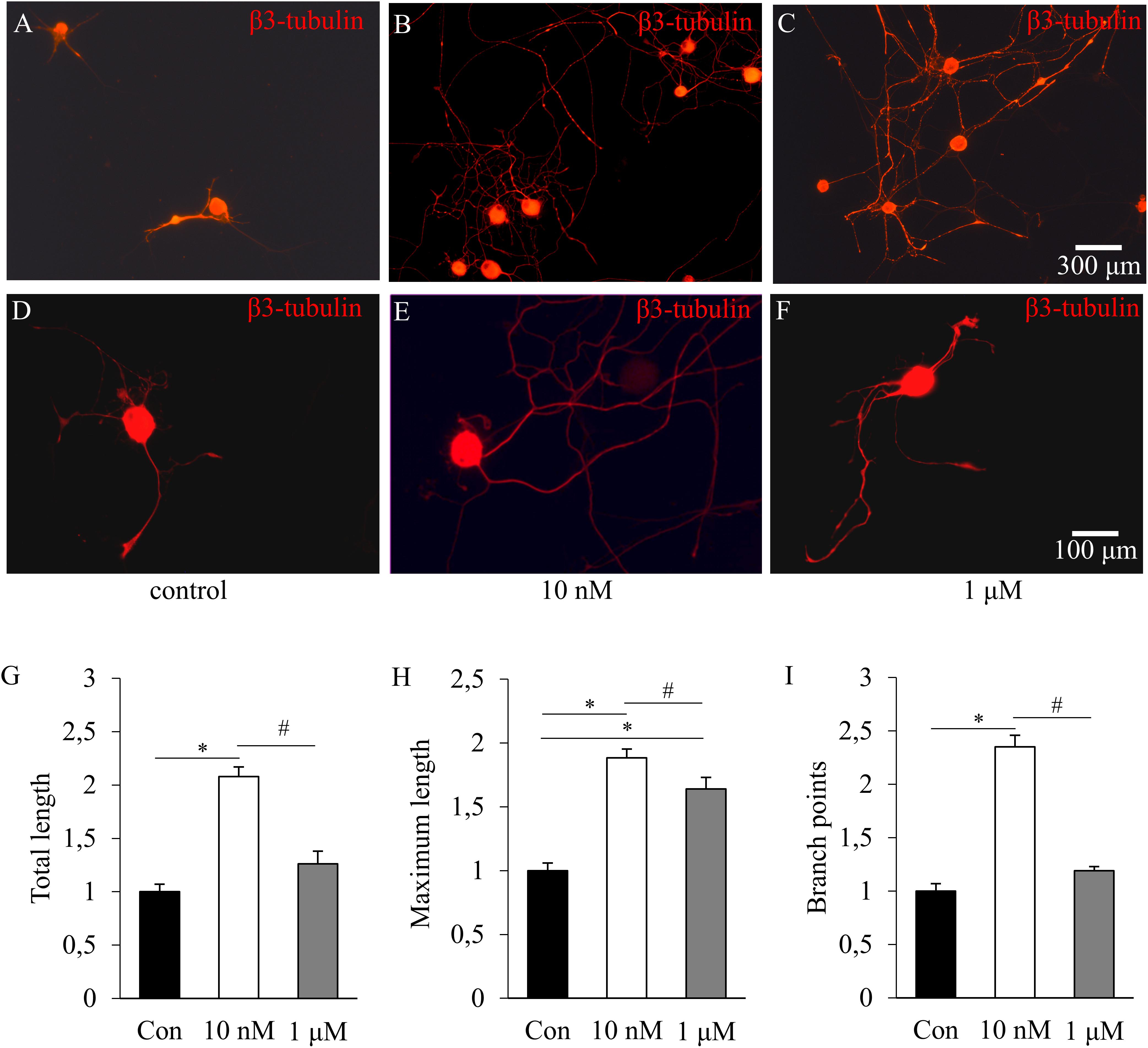
FIGURE 1. The effects of insulin on neurite outgrowth of cultured adult rat dorsal root ganglion (DRG) neurons. (A–F) Fluorescence photomicrographs of β3-tubulin-stained DRG neurons illustrating the effects of insulin applied at concentrations of 10 nM (B) and 1 μM (C). The scale bar in (C) indicates 300 μm and applies to photomicrographs (A–C), the scale bar in (F) indicates 100 μm and applies to photomicrographs (D–F). (G–I) Quantitative morphometric evaluation of the effects of insulin on neurite outgrowth. Changes in total neurite length (G), maximum neurite length (H), and number of branch points (I) are shown as fold increase of the control. Values are expressed as mean ± standard error of the mean (SEM). ∗Statistically significantly different from the control (p < 0.05). #Parameters of DRG neurons treated with 10 nM and 1 μM insulin, respectively, are significantly different (p < 0.05).
Distinct Neurite Growth-Promoting Effect of Insulin on Neurochemically Differing Subpopulations of DRG Neurons
Neurochemically distinct subpopulations of cultured DRG neurons were assessed by counting DRG neurons which showed immunoreactivity for the InsR, TRPV1, CGRP, or IB4 binding, respectively. In control cultures, 43.68 ± 1.71% of DRG neurons were IR for the InsR (Figure 2). The size-frequency distribution of the InsR-IR neurons revealed that InsR-positive DRG neurons are small-medium sized neurons. The mean cross-sectional area of the InsR-IR and InsR-immunonegative DRG neurons amounted to 321.11 ± 11.2 μm2 and 526.39 ± 15.22 μm2, respectively (Figure 3). Of the TRPV1-, CGRP-, and IB4-positive DRG neurons 63.09 ± 2.42%, 48.13 ± 2.37%, and 49.58 ± 3.7%, respectively, showed InsR-IR (Figure 2). Administration of insulin at concentrations of 10 nM and 1 μM did not significantly alter the percentage distribution of InsR-, TRPV1-, and CGRP-IR, and IB4-binding DRG neurons (p < 0.05). Analysis of the TRPV1- and the CGRP-IR and the IB4-binding populations revealed that 60.92 ± 3.38%, 56.98 ± 2.81%, and 31.11 ± 3.7% of the InsR-IR neurons were TRPV1-, CGRP-, and IB4-positive, respectively (Figure 2). The proportions of TRPV1-IR, CGRP-IR, and IB4-binding neurons expressing the InsR were significantly different (p < 0.05). The InsR was expressed in 42.06 ± 3.39%, 51.04 ± 4.26%, and 28.29 ± 2.4% of the TRPV1-IR, CGRP-IR, and IB4-binding neurons, respectively (Figure 2). The percentages of neurons which colocalized the InsR with TRPV1, CGRP, and IB4 amounted to 26.5 ± 2.17%, 24.33 ± 3.25%, and 13.82 ± 2.37%, respectively (Figure 2).
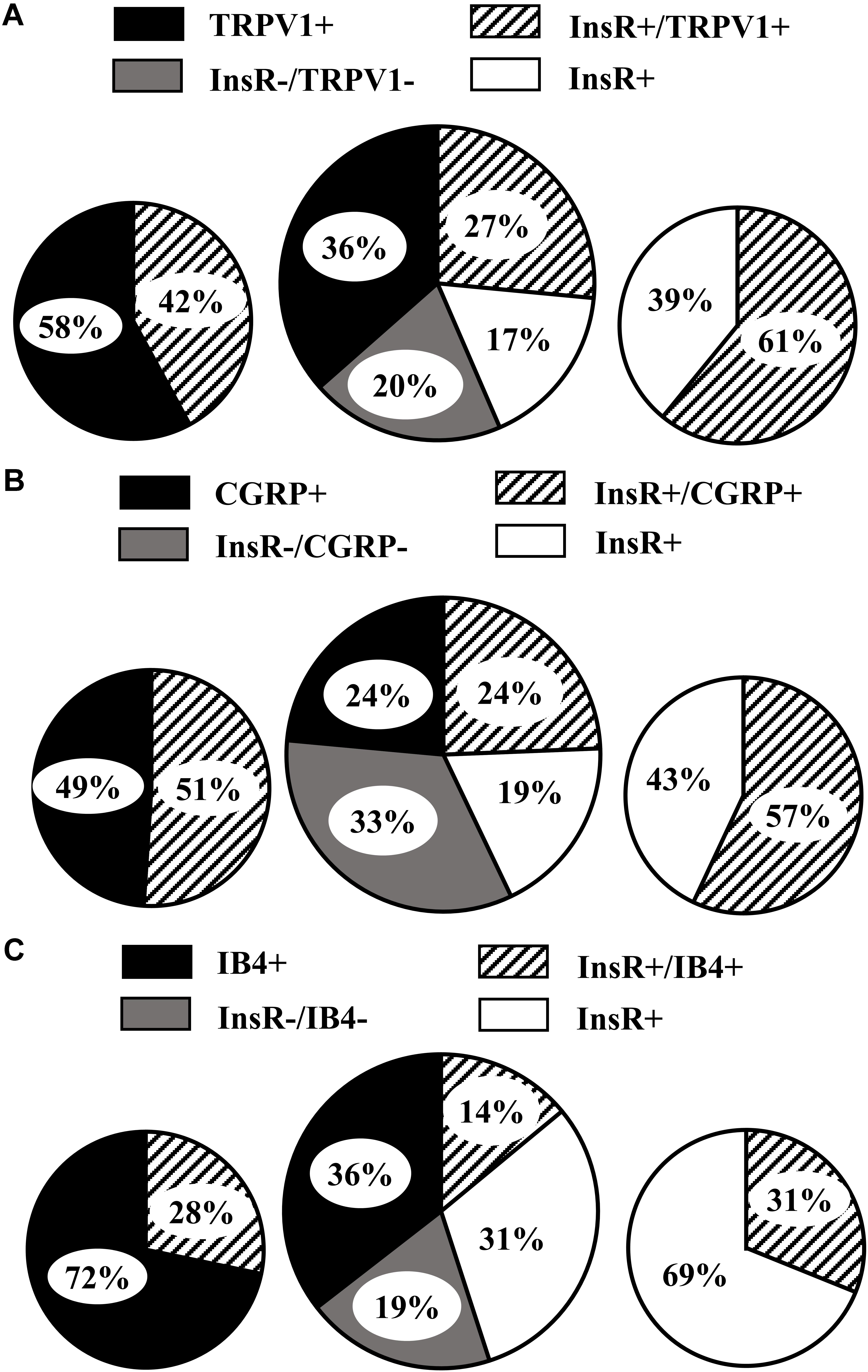
FIGURE 2. Neurochemical phenotypes of cultured adult rat dorsal root ganglion (DRG) neurons examined. (A) Large pie chart shows the percentage distribution of populations of DRG neurons showing immunoreactivities for the insulin receptor (InsR) and the transient receptor potential vanilloid type 1 receptor (TRPV1). Small pie charts show the relative proportions of TRPV1-immunoreactive (IR) neurons which display colocalization with the InsR and, conversely, the relative proportions of InsR-IR DRG neurons which display colocalization with TRPV1. (B) Large pie chart shows the percentage distribution of populations of DRG neurons showing immunoreactivities for the InsR and calcitonin gene-related peptide (CGRP). Small pie charts show the relative proportions of CGRP-IR DRG neurons which display colocalization with the InsR and, conversely, the relative proportions of InsR-IR DRG neurons which display colocalization with CGRP. (C) Large pie chart shows the percentage distribution of populations of DRG neurons showing immunoreactivity for the InsR and binding of the isolectin B4 (IB4). Small pie charts show the relative proportions of IB4-binding neurons which display colocalization with the InsR and, conversely, the relative proportions of InsR-IR DRG neurons which display colocalization with IB4.
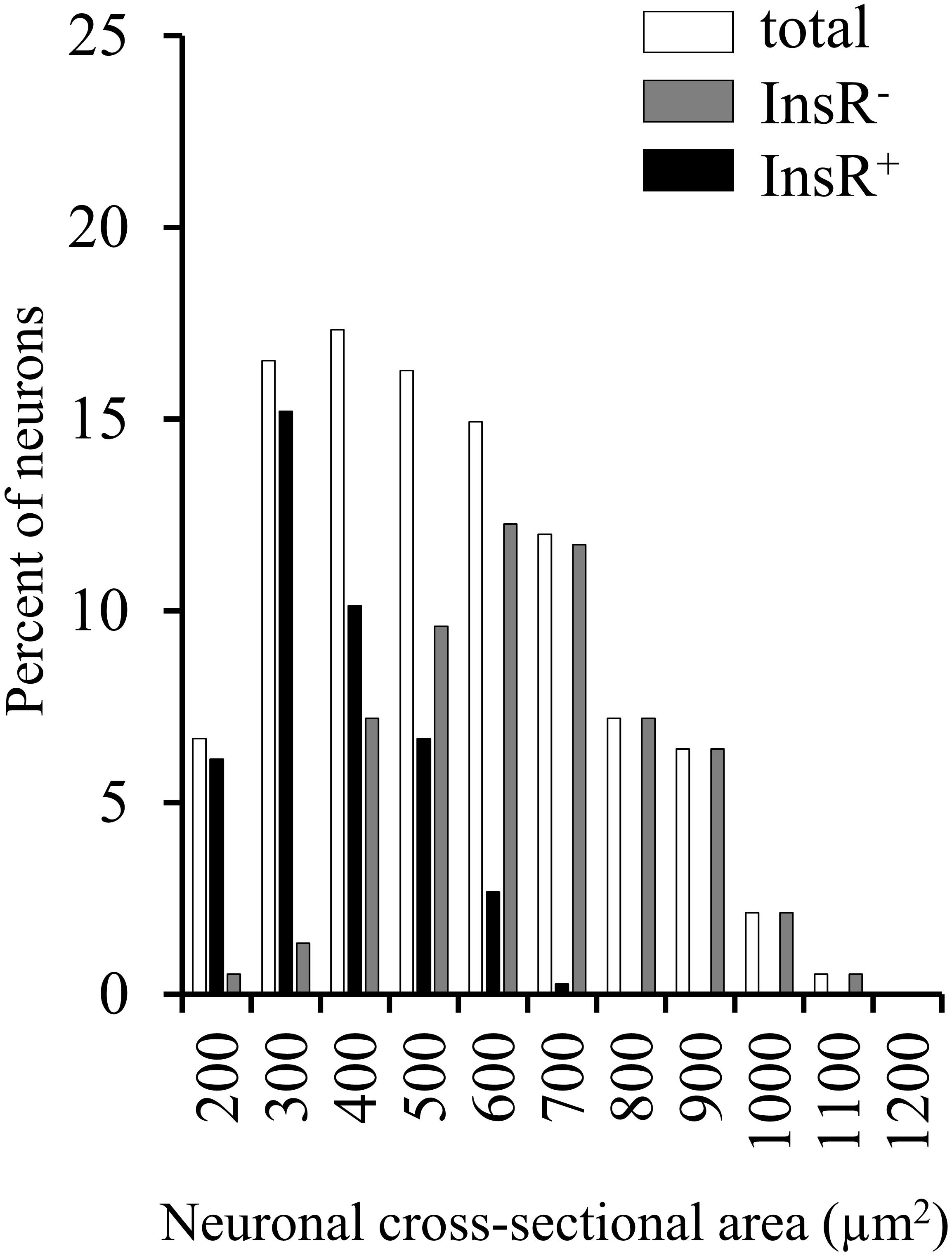
FIGURE 3. Size-frequency distribution histogram of the total and the insulin receptor (InsR)-immunopositive and -negative populations of adult rat cultured dorsal root ganglion (DRG) neurons. Size-frequency distribution histogram shows the cross-sectional areas of the total DRG neuronal population (white bars), the InsR-immunopositive (black bars) and the InsR-immunonegative (gray bars) populations in the control cultures.
To reveal the neurite outgrowth-promoting effect of insulin on different subpopulations of DRG neurons, the three quantified parameters of InsR-, TRPV1-, and CGRP-IR and IB4-binding DRG neurons were analyzed in control and insulin-treated cultures. Photomicrographs in Figures 5A–C, 6A–C, 7A–C illustrate examples of analyzed InsR and TRPV1-IR, InsR- and CGRP-IR, and InsR-IR and IB4-binding populations of cultured DRG neurons exposed to insulin (10 nM).
Our data revealed that insulin significantly increased the total neurite length from 513.21 ± 25.86 μm to 853.95 ± 52.65 μm, the maximum neurite length from 66.57 ± 12.9 μm to 127.88 ± 11.21 μm, and the neurite branch points from 25.38 ± 4.32 to 65.38 ± 9.02 in the InsR-immunonegative neuronal population (p < 0.05). Our data also demonstrated that in InsR-IR neurons, insulin significantly increased the total neurite length from 536.61 ± 20.29 μm to 1390.21 ± 27.17 μm, the maximum neurite length from 74.42 ± 7.74 μm to 174.33 ± 8.36 μm, and the neurite branch points from 22.56 ± 5.14 to 123.15 ± 4.0, respectively (for all comparisons p < 0.05; Figures 4A–F). Statistical analysis revealed that differences between each quantified parameter of insulin-treated neuronal populations which express or lack the InsR were significant (p < 0.05).
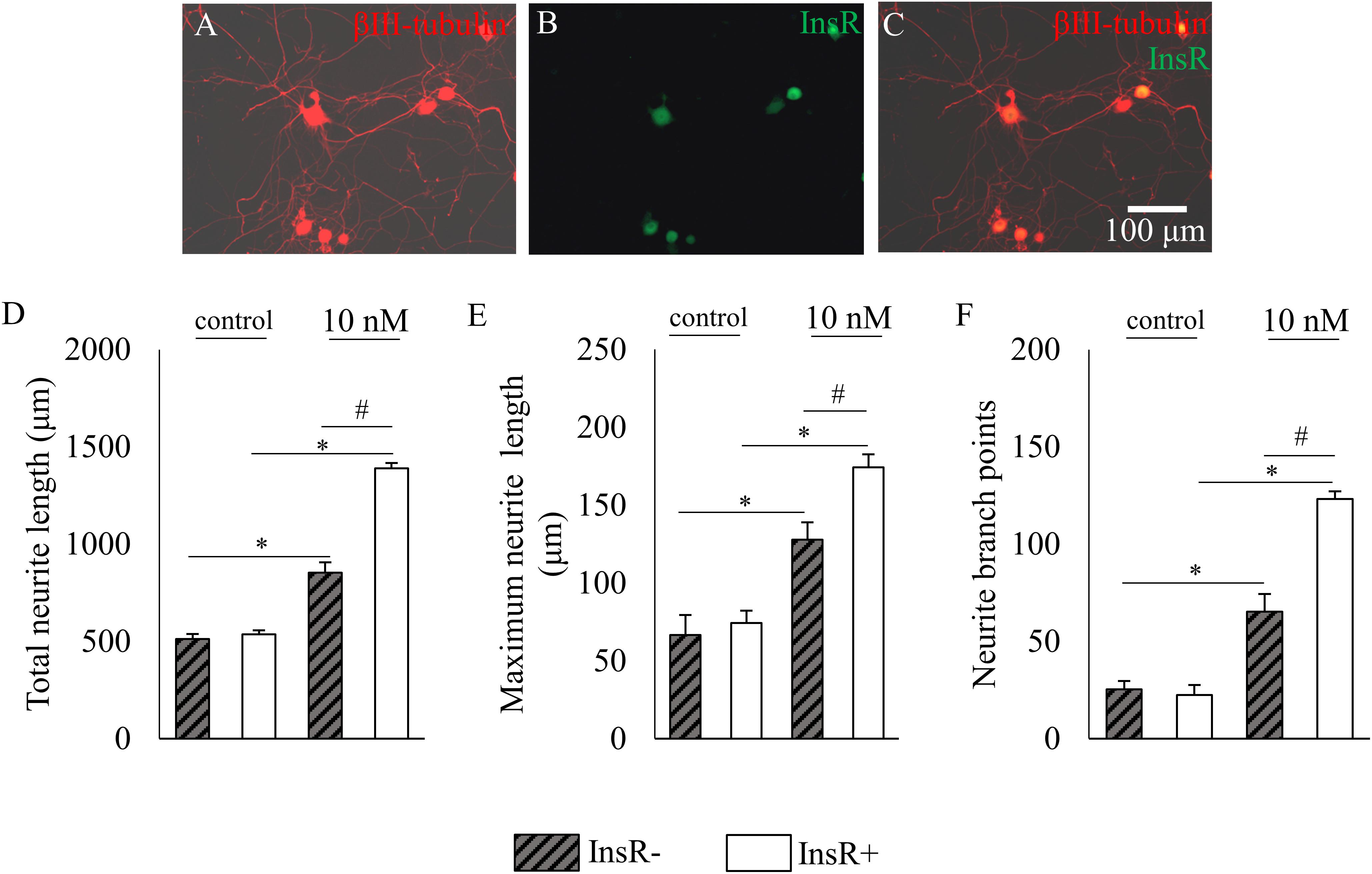
FIGURE 4. The effects of insulin (10 nM, 48 h) on neurite outgrowth of insulin receptor (InsR)-positive and InsR-negative cultured adult rat dorsal root ganglion (DRG) neurons. (A–C) Fluorescence photomicrographs of cultured adult rat dorsal root ganglion (DRG) neurons double-stained for β3-tubulin- and InsR-immunoreactivities. The scale bar in (C) indicates 100 μm and applies to all photomicrographs. (D–F) Quantitative morphometric evaluation of the effects of insulin on neurite outgrowth of InsR-positive and InsR-negative populations of DRG neurons. Changes in total neurite length (D), maximum neurite length (E) and number of branch points (F) are shown. Values are expressed as mean ± standard error of the mean (SEM). ∗Statistically significantly different from the control (p < 0.05). #Parameters of InsR-positive and InsR-negative DRG neurons are significantly different (p < 0.05).
Our morphological analysis also revealed that insulin significantly increased total neurite length from 539.02 ± 27.91 μm to 969.45 ± 25.62 μm, the maximum neurite length from 65.85 ± 8.01 μm to 125.59 ± 5.04 μm, and the neurite branch points from 22.91 ± 5.32 to 48.04 ± 3.65 of neurons which were IR for TRPV1, but not for the InsR (p < 0.05; Figures 5A–G). Conversely, in neurons which displayed the InsR, but not the TRPV1, insulin significantly increased the total neurite length from 522.98 ± 26.44 μm to 1150.89 ± 21.01 μm, the maximum neurite length from 74.426 ± 7.22 μm to 160.36 ± 4.77 μm, and the neurite branch points from 22.56 ± 6.2 to 90.38 ± 2.99, respectively (p < 0.05; Figures 5A–G). Statistical analysis revealed that differences between each quantified parameter of insulin-treated neuronal populations which express or lack the InsR were significant (p < 0.05). In DRG neurons which showed colocalization of the InsR and TRPV1, insulin significantly increased the total neurite length from 542.08 ± 25.17 μm to 1326.97 ± 22.77 μm, the maximum neurite length from 85.84 ± 9.02 μm to 188.93 ± 5.2 μm, and the neurite branch points from 24.56 ± 4.88 to 124.05 ± 3.84, respectively (for all comparisons p < 0.05; Figures 5A–G). Statistical analysis revealed that differences between each quantified parameter of insulin-treated InsR-IR neuronal populations which express or lack the TRPV1 were significant (p < 0.05).
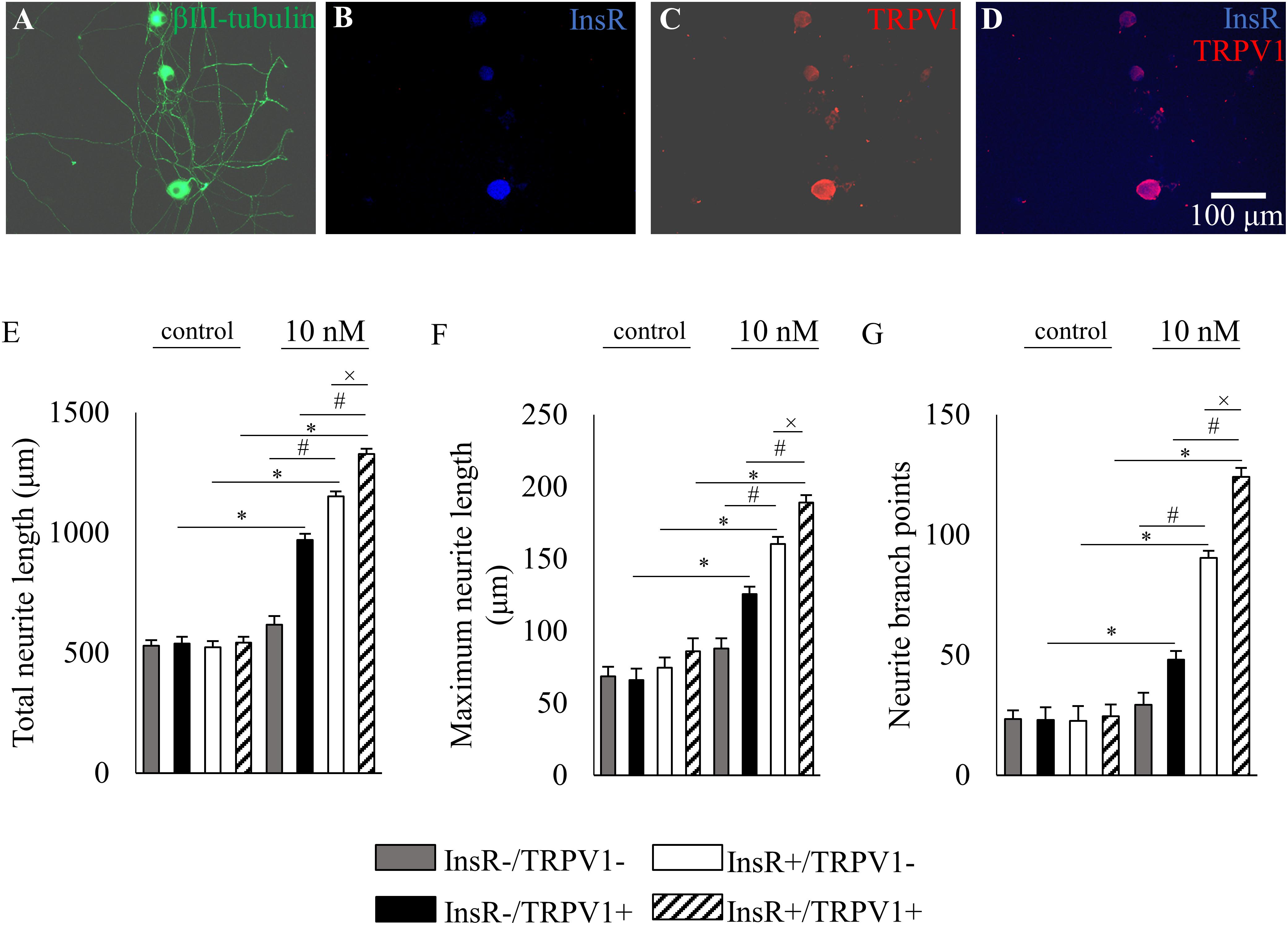
FIGURE 5. The effects of insulin (10 nM, 48 h) on neurite outgrowth of cultured adult rat dorsal root ganglion (DRG) neurons. (A–D) Fluorescence photomicrographs of cultured adult rat dorsal root ganglion (DRG) neurons triple-stained for β3-tubulin, insulin receptor (InsR) and transient receptor potential vanilloid type 1 receptor (TRPV1). The scale bar in (D) indicates 100 μm and applies to all photomicrographs. (E–G) Quantitative morphometric evaluation of the effects of insulin on neurite outgrowth of InsR-positive and InsR-negative populations of TRPV1-positive and TRPV1-negative DRG neurons. Changes in total neurite length (E), maximum neurite length (F), and number of branch points (G) are shown. Values are expressed as mean ± standard error of the mean (SEM). ∗, #, and x: Statistically significantly different from the corresponding control and/or insulin-treated DRG neuron populations (p < 0.05).
Our data also showed that in the CGRP-IR and InsR-immunonegative DRG neuronal population, insulin significantly increased the total neurite length from 565.47 ± 3.94 μm to 984.33 ± 24.03 μm, the maximum neurite length from 57.4 ± 9.06 μm to 110.81 ± 4.54 μm, and the neurite branch points from 21.47 ± 4.73 to 60.5 ± 4.99, respectively (for all comparisons p < 0.05; Figures 6A–G). In the InsR-IR and CGRP-immunonegative DRG neurons, insulin significantly increased the total neurite length from 522.99 ± 26.59 μm to 1099.66 ± 19.77 μm, the maximum neurite length from 54.99 ± 8.01 μm to 140.67 ± 3.99 μm, and the neurite branch points from 24.97 ± 3.66 to 106.37 ± 3.01, respectively (for all comparisons p < 0.05; Figures 6A–G). Statistical analysis revealed that differences between each quantified parameter of insulin-treated neuronal populations which express or lack the InsR were significant (p < 0.05). In InsR- and CGRP-IR neurons insulin significantly increased all the three quantified parameters: the total neurite length from 535.62 ± 30.6 μm to 1292.86 ± 26.88 μm, the maximum neurite length from 59.67 ± 6.44 μm to 182.55 ± 5.8 μm, and the neurite branch points from 26.55 ± 5.89 to 131.55 ± 3.99 (for all comparisons p < 0.05; Figures 6E–G). Statistical analysis revealed that differences between each quantified parameter of insulin-treated InsR-IR neuronal populations which express, or lack CGRP-IR were significant (p < 0.05). The statistical analysis revealed that the differences between the treated populations were also significant (p < 0.05).
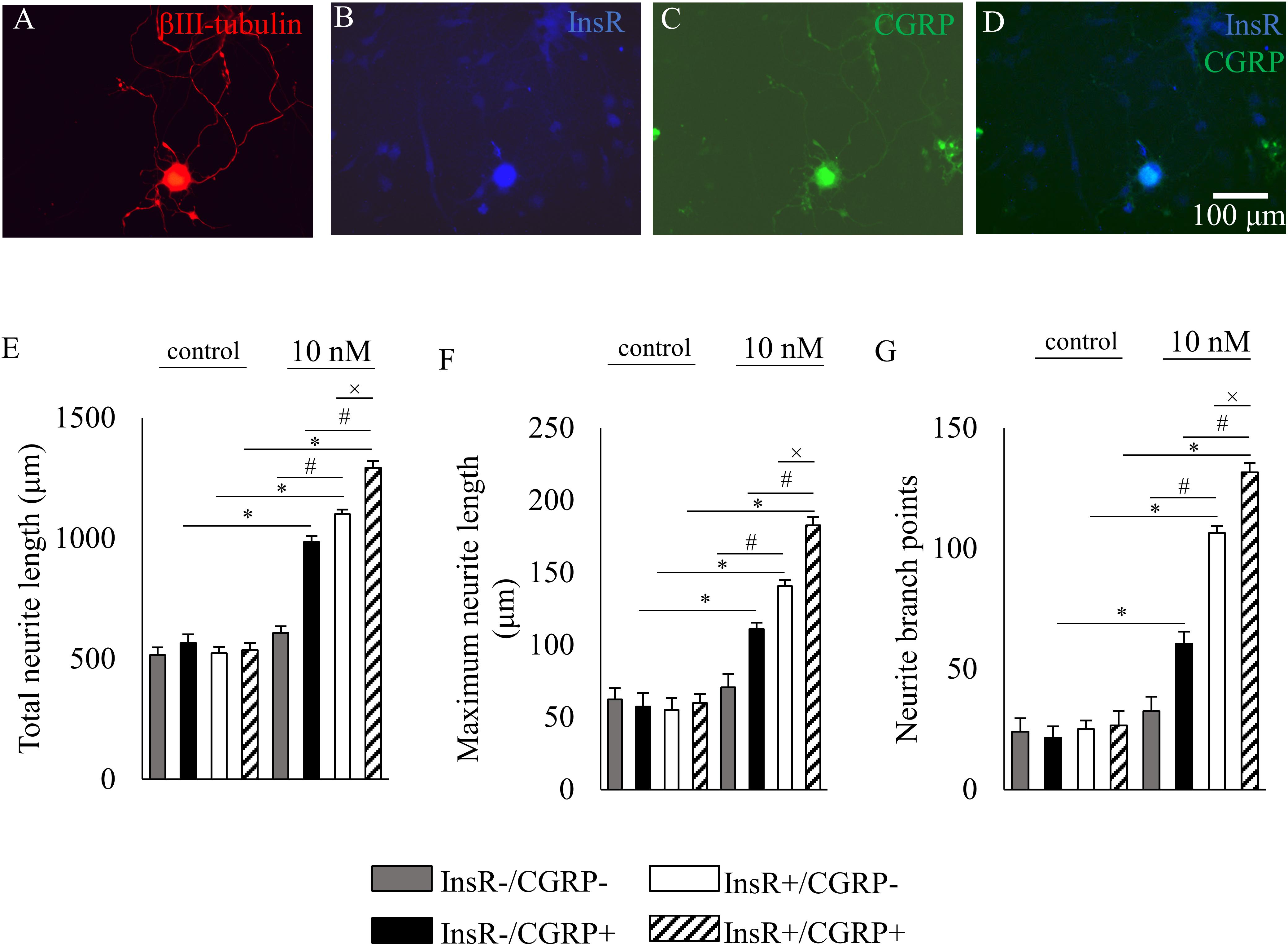
FIGURE 6. The effects of insulin (10 nM, 48 h) on neurite outgrowth of cultured adult rat dorsal root ganglion (DRG) neurons. (A–D) Fluorescence photomicrographs of cultured adult rat dorsal root ganglion (DRG) neurons triple-stained for β3-tubulin, insulin receptor (InsR) and calcitonin gene-related peptide (CGRP). The scale bar in (D) indicates 100 μm and applies to all photomicrographs. (E–G) Quantitative morphometric evaluation of the effects of insulin on neurite outgrowth of InsR-positive and InsR-negative populations of CGRP-positive and CGRP-negative DRG neurons. Changes in total neurite length (E), maximum neurite length (F) and number of branch points (G) are shown. Values are expressed as mean ± standard error of the mean (SEM). ∗, #, and x: Statistically significantly different from the corresponding control and/or insulin-treated DRG neuron populations (p < 0.05).
Analysis of the insulin responsiveness of the IB4-positive DRG neurons revealed that neurons which lack the InsR failed to show changes in any of the quantified parameters examined (Figures 7A–G). In contrast, in IB4-positive neurons displaying the InsR, insulin treatment significantly increased total neurite length from 543.56 ± 30.96 μm to 1210.81 ± 20.08 μm, the maximum neurite length from 70.3 ± 7.99 μm to 156.81 ± 10.1 μm, and neurite branch points from 28.67 ± 7.99 to 119.21 ± 4.7, respectively (for all comparisons p < 0.05; Figures 7A–G).
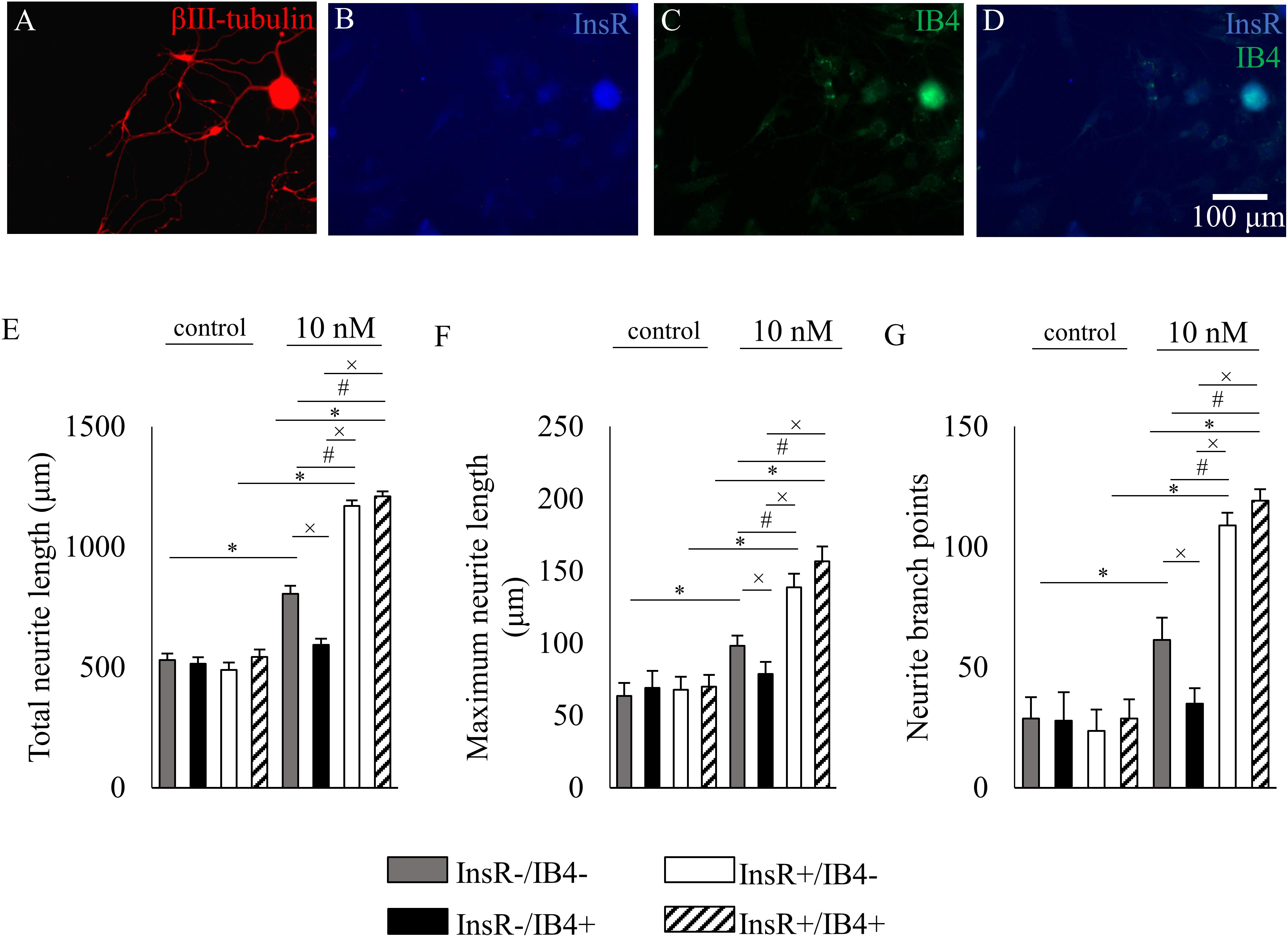
FIGURE 7. The effects of insulin (10 nM, 48 h) on neurite outgrowth of cultured adult rat dorsal root ganglion (DRG) neurons. (A–D) Fluorescence photomicrographs of cultured adult rat dorsal root ganglion (DRG) neurons triple-stained for β3-tubulin, insulin receptor (InsR) and isolectin B4 (IB4). The scale bar in (D) indicates 100 μm and applies to all photomicrographs. (E–G) Quantitative morphometric evaluation of the effects of insulin on neurite outgrowth of InsR-positive and InsR-negative populations of IB4-positive and IB4-negative DRG neurons. Changes in total neurite length (E), maximum neurite length (F) and number of branch points (G) are shown. Values are expressed as mean ± standard error of the mean (SEM). ∗, #, and x: Statistically significantly different from the corresponding control and/or insulin-treated DRG neuron populations (p < 0.05).
Discussion
Insulin exerts neurotrophic and neuromodulatory effects in a variety of central and peripheral neuronal systems (Recio-Pinto et al., 1986; Fernyhough et al., 1993; Wan et al., 1997; Barber et al., 2001; Stella et al., 2001; Gerozissis, 2003; Sathianathan et al., 2003; Singh et al., 2012). The present data support and extend previous observations by showing that nanomolar concentrations of insulin significantly increase neurite outgrowth of cultured adult rat DRG neurons (Recio-Pinto et al., 1986; Fernyhough et al., 1993; Singh et al., 2012). In accord with earlier studies, the present findings also demonstrate the disparate effects of low (nM) and high (μm) concentrations of insulin on neurite outgrowth (Recio-Pinto et al., 1986; Fernyhough et al., 1993; Singh et al., 2012). However, the most important finding of the present study is that neurochemically distinct populations of DRG neurons display differing responsiveness to the neurite outgrowth-promoting effect of insulin.
Adult rat DRG neurons can be classified on the basis of their sensitivity to and dependence on neurotrophins. During the postnatal period both peptidergic and non-peptidergic DRG neurons are responsive to NGF and express the tropomyosin receptor kinase A (TrkA). However, in the course of their development, expression of TrkA is downregulated and non-peptidergic, IB4-binding neurons lose their NGF-sensitivity and become sensitive to GDNF (Molliver et al., 1997). Several studies demonstrated significant changes in the expression of proteins and peptides in DRG neurons following axonal injuries or culturing (cf. Jancso, 1992; Hokfelt et al., 1994). However, in the present study morphometric analysis of CGRP- and IB4-positive neurons in control and insulin-treated cultures revealed percentage distributions similar to control ganglia in vivo (e.g., Lindsay et al., 1989; Hjerling-Leffler et al., 2007). This is in agreement with previous findings showing that the time frame of changes in the expression pattern of DRG neurons which commence in response to injury or exposure to trophic factors is longer than that in the present experiments (cf. Lindsay et al., 1989; Hokfelt et al., 1994).
The expression of the InsR has been demonstrated in a relatively high proportion of DRG neurons in both rats and mice (Baiou et al., 2007; Lázár et al., 2018a,b). The present findings confirm these observations by showing that up to 45% of cultured adult rat DRG neurons, which comprise both peptidergic and IB4-binding DRG neurons, display InsR-immunoreactivity. Further, most TRPV1-expressing neurons, which involve both peptidergic and non-peptidergic populations, also express the InsR. The present results are in agreement with previous in vitro and in vivo findings by showing that InsR-IR DRG neurons are small-medium sized (Baiou et al., 2007; Lázár et al., 2018a,b).
The results demonstrate that populations of DRG neurons which express the InsR show an increased propensity for neurite outgrowth as compared to populations which lack this receptor. This is especially evident in the IB4-binding population of neurons; only those neurons which express the InsR exhibit an increase in neurite outgrowth upon insulin exposure. The exact mechanism of insulin-induced increased neurite outgrowth is not clearly established, but the role of the InsR has been implicated (Singh et al., 2012; Grote et al., 2013; Grote and Wright, 2016). It has been proposed that common signaling mechanisms may contribute to the activation of both the InsR and some classic neurotrophins such as NGF (Brewster et al., 1994; Grote et al., 2013; Grote and Wright, 2016). Possible molecular mechanisms of insulin’s neurite outgrowth promoting action involve stabilization of tubulin, an essential component of axon and dendrite growth (Fernyhough et al., 1989). The role of insulin at low (nM) concentrations in the activation of the phosphoinositide 3-kinase/protein kinase B (PI3K-Akt) signaling pathway (Huang et al., 2005), which is critically involved in the mechanisms of axonal growth (Goldberg, 2003), has also been revealed. Previous observations have also shown that high (μM) concentrations of insulin, through the desensitization of the InsRs, render DRG neurons less sensitive to the neurotrophic effects of insulin by the inhibition of the PI3K-Akt pathway (Singh et al., 2012). In addition, increase in GSK-3β levels may also significantly contribute to the decreased sensitivity and, consequently impaired growth cone advancement (Eickholt et al., 2002; Singh et al., 2012).
However, other specific traits, such as the expression of TRPV1 and/or CGRP also significantly modulate neurite outgrowth. Hence, although the results suggest that the expression of the InsR enhances neurite outgrowth in all populations of DRG neurons examined, the expression of TRPV1 and/or CGRP per se appear to promote neurite outgrowth. Signaling mechanisms involved in the mediation of the neurite outgrowth-promoting effect of insulin may share common pathways contributing to the expression and sensitization of the TRPV1 by NGF (Winter et al., 1988; Brewster et al., 1994; Shu and Mendell, 1999; Nicol and Vasko, 2007; Grote et al., 2013; Grote and Wright, 2016). It has been demonstrated that the NGF receptor, TrkA plays an important role in insulin signaling in PC12 cells which share characteristics with sensory neurons (Geetha et al., 2013). Hence, it has been shown that insulin can induce the phosphorylation of TrkA which is essential in the activation of the InsR in PC12 cells (Geetha et al., 2013). CGRP-IR neurons are also sensitive to and dependent on NGF: it plays a critical role in the survival of CGRP-IR neurons in early development and regulates peptide expression and neurite outgrowth in the adult (Lindsay et al., 1989; Molliver et al., 1997). It can be hypothesized that in CGRP-IR neurons which lack the InsR, insulin promotes neurite outgrowth through TrkA signaling, instead of insulin signaling via the InsR. Further, CGRP-IR DRG neurons have been shown to be increasingly sensitive to high concentrations of both insulin and NGF under pathological conditions (Takatori et al., 2008; Zamami et al., 2011; Hashikawa-Hobara et al., 2012). Taken together, these findings may suggest that NGF sensitivity of the TRPV1- and CGRP-IR DRG neurons could explain their inherent propensity for increased neurite outgrowth. This assumption is supported by the apparent insensitivity to insulin’s neurite outgrowth promoting effect of the IB4-positive neurons which lack both the InsR and sensitivity to NGF. Furthermore, novel findings in sensory neuron InsR knockout mice suggested that not the InsR itself, but an interaction between insulin, TRPV1 and/or neuropeptides, such as CGRP is crucial in the development of dysfunctions of insulin signaling in DRG neurons (Grote et al., 2018). However, the results demonstrate a significantly higher expression rate of the InsR in TRPV1- and CGRP-IR DRG neurons as compared to the IB4-positive population. Importantly, the responsiveness of DRG neurons expressing the InsR was superior to populations of DRG neurons which lack this receptor, suggesting a pivotal role of InsR signaling in the mechanisms of neurite outgrowth.
Importantly, it has also been reported that similar to NGF application, insulin treatment facilitates the formation of collateral sprouting of small diameter neurons (Diamond et al., 1992; Singhal et al., 1997; Guo et al., 2011). The present findings are in accord with this observation and, in addition, demonstrate that branch points were particularly increased by insulin in InsR-IR neurons. These observations may suggest that InsR signaling may bear of importance in the mechanism of collateral sprouting of sensory neurons as well.
Conclusion
The present experiments confirm and extend previous findings on the neurite outgrowth-promoting effect of insulin and the expression of the InsR in cultured adult rat DRG neurons. The most important new observation of this study is that neurons which express the InsR exhibit a significantly higher propensity for the neurite outgrowth-promoting effect of insulin as compared to neurons which lack this particular receptor. Further, the data indicate that peptidergic CGRP-IR and TRPV1-IR nociceptive neurons expressing the InsR may be identified as the major subpopulations of DRG neurons which exhibit insulin-induced increased neurite outgrowth. The significance of the InsR in promoting neurite outgrowth is further supported by the observation that insulin increased neurite outgrowth only in a subpopulation of IB4-positive neurons which express the InsR. These findings suggest distinct regenerative propensity of differing populations of DRG neurons which is significantly affected through InsR signaling. In addition, the different responsiveness of separate chemotypes of primary sensory neurons to insulin may interfere with the development of pathologies associated with diabetes mellitus.
Author Contributions
BL, PS, and GJ were responsible for the study concept and design. BL, LP, ID, and PS were responsible for the data collection and analysis. BL, GJ, IN, and PS were interpreted the data, revised for intellectual content. All authors were involved in manuscript editing and have approved the final version for submission.
Funding
This work was supported by GINOP-2.3.2-15-2016-00034 projects of the Hungarian National Research, Development and Innovation Office. BL was supported by the UNKP-17-3 New National Excellence Program of the Ministry if Human Capacities.
Conflict of Interest Statement
The authors declare that the research was conducted in the absence of any commercial or financial relationships that could be construed as a potential conflict of interest.
References
Baiou, D., Santha, P., Avelino, A., Charrua, A., Bacskai, T., Matesz, K., et al. (2007). Neurochemical characterization of insulin receptor-expressing primary sensory neurons in wild-type and vanilloid type 1 transient receptor potential receptor knockout mice. J. Comp. Neurol. 503, 334–347. doi: 10.1002/cne.21389
Barber, A. J., Nakamura, M., Wolpert, E. B., Reiter, C. E., Seigel, G. M., Antonetti, D. A., et al. (2001). Insulin rescues retinal neurons from apoptosis by a phosphatidylinositol 3-kinase/Akt-mediated mechanism that reduces the activation of caspase-3. J. Biol. Chem. 276, 32814–32821. doi: 10.1074/jbc.M104738200
Brewster, W. J., Fernyhough, P., Diemel, L. T., Mohiuddin, L., and Tomlinson, D. R. (1994). Diabetic neuropathy, nerve growth factor and other neurotrophic factors. Trends Neurosci. 17, 321–325. doi: 10.1016/0166-2236(94)90169-4
Buck, S. H., and Burks, T. F. (1986). The neuropharmacology of capsaicin: review of some recent observations. Pharmacol. Rev. 38, 179–226.
Caterina, M. J., Schumacher, M. A., Tominaga, M., Rosen, T. A., Levine, J. D., and Julius, D. (1997). The capsaicin receptor: a heat-activated ion channel in the pain pathway. Nature 389, 816–824. doi: 10.1038/39807
Chen, Y., Stevens, B., Chang, J., Milbrandt, J., Barres, B. A., and Hell, J. W. (2008). NS21: re-defined and modified supplement B27 for neuronal cultures. J. Neurosci. Methods 171, 239–247. doi: 10.1016/j.jneumeth.2008.03.013
Diamond, J., Holmes, M., and Coughlin, M. (1992). Endogenous NGF and nerve impulses regulate the collateral sprouting of sensory axons in the skin of the adult rat. J. Neurosci. 12, 1454–1466. doi: 10.1523/JNEUROSCI.12-04-01454.1992
Eickholt, B. J., Walsh, F. S., and Doherty, P. (2002). An inactive pool of GSK-3 at the leading edge of growth cones is implicated in Semaphorin 3A signaling. J. Cell Biol. 157, 211–217. doi: 10.1083/jcb.200201098
Fernyhough, P., Mill, J. F., Roberts, J. L., and Ishii, D. N. (1989). Stabilization of tubulin mRNAs by insulin and insulin-like growth factor I during neurite formation. Brain Res. Mol. Brain Res. 6, 109–120. doi: 10.1016/0169-328X(89)90044-2
Fernyhough, P., Willars, G. B., Lindsay, R. M., and Tomlinson, D. R. (1993). Insulin and insulin-like growth factor I enhance regeneration in cultured adult rat sensory neurones. Brain Res. 607, 117–124. doi: 10.1016/0006-8993(93)91496-F
Geetha, T., Rege, S. D., Mathews, S. E., Meakin, S. O., White, M. F., and Babu, J. R. (2013). Nerve growth factor receptor TrkA, a new receptor in insulin signaling pathway in PC12 cells. J. Biol. Chem. 288, 23807–23813. doi: 10.1074/jbc.M112.436279
Gerozissis, K. (2003). Brain insulin: regulation, mechanisms of action and functions. Cell. Mol. Neurobiol. 23, 1–25. doi: 10.1023/A:1022598900246
Grote, C. W., Ryals, J. M., and Wright, D. E. (2013). In vivo peripheral nervous system insulin signaling. J. Peripher. Nerv. Syst. 18, 209–219. doi: 10.1111/jns5.12033
Grote, C. W., Wilson, N. M., Katz, N. K., Guilford, B. L., Ryals, J. M., Novikova, L., et al. (2018). Deletion of the insulin receptor in sensory neurons increases pancreatic insulin levels. Exp. Neurol. 305, 97–107. doi: 10.1016/j.expneurol.2018.04.002
Grote, C. W., and Wright, D. E. (2016). A role for insulin in diabetic neuropathy. Front. Neurosci. 10:581. doi: 10.3389/fnins.2016.00581
Guo, A., Simone, D. A., Stone, L. S., Fairbanks, C. A., Wang, J., and Elde, R. (2001). Developmental shift of vanilloid receptor 1 (VR1) terminals into deeper regions of the superficial dorsal horn: correlation with a shift from TrkA to Ret expression by dorsal root ganglion neurons. Eur. J. Neurosci. 14, 293–304. doi: 10.1046/j.0953-816x.2001.01665.x
Guo, G., Kan, M., Martinez, J. A., and Zochodne, D. W. (2011). Local insulin and the rapid regrowth of diabetic epidermal axons. Neurobiol. Dis. 43, 414–421. doi: 10.1016/j.nbd.2011.04.012
Hashikawa-Hobara, N., Hashikawa, N., Zamami, Y., Takatori, S., and Kawasaki, H. (2012). The mechanism of calcitonin gene-related peptide-containing nerve innervation. J. Pharmacol. Sci. 119, 117–121. doi: 10.1254/jphs.12R02CP
Havrankova, J., Roth, J., and Brownstein, M. (1978). Insulin receptors are widely distributed in the central nervous system of the rat. Nature 272, 827–829. doi: 10.1038/272827a0
Hjerling-Leffler, J., Alqatari, M., Ernfors, P., and Koltzenburg, M. (2007). Emergence of functional sensory subtypes as defined by transient receptor potential channel expression. J. Neurosci. 27, 2435–2443. doi: 10.1523/JNEUROSCI.5614-06.2007
Hokfelt, T., Zhang, X., and Wiesenfeld-Hallin, Z. (1994). Messenger plasticity in primary sensory neurons following axotomy and its functional implications. Trends Neurosci. 17, 22–30. doi: 10.1016/0166-2236(94)90031-0
Holzer, P. (1991). Capsaicin: cellular targets, mechanisms of action, and selectivity for thin sensory neurons. Pharmacol. Rev. 43, 143–201.
Huang, T. -J., Verkhratsky, A., and Fernyhough, P. (2005). Insulin enhances mitochondrial inner membrane potential and increases ATP levels through phosphoinositide 3-kinase in adult sensory neurons. Mol. Cell. Neurosci. 28, 42–54. doi: 10.1016/j.mcn.2004.08.009
Jancso, G. (1992). Pathobiological reactions of C-fibre primary sensory neurones to peripheral nerve injury. Exp. Physiol. 77, 405–431. doi: 10.1113/expphysiol.1992.sp003603
Jancsó, G., Katona, M., Horváth, V., Sántha, P., and Nagy, I. (2009). “Sensory nerves as modulators of cutaneous inflammatory reactions in health and disease,” in Neurogenic Inflammation in Health and Disease, ed. G. Jancsó (Amsterdam: Elsevier), 3–36.
Jancso, G., Kiraly, E., and Jancso-Gabor, A. (1977). Pharmacologically induced selective degeneration of chemosensitive primary sensory neurones. Nature 270, 741–743. doi: 10.1038/270741a0
Jancsó, N. (1960). Role of the nerve terminals in the mechanism of inflammatory reactions. Bull. Millard Film. Hosp. 7, 53–77.
Jancso, N., Jancso-Gabor, A., and Szolcsanyi, J. (1968). The role of sensory nerve endings in neurogenic inflammation induced in human skin and in the eye and paw of the rat. Br. J. Pharmacol. Chemother. 33, 32–41. doi: 10.1111/j.1476-5381.1968.tb00471.x
Lázár, B. A., Jancsó, G., Nagy, I., Horváth, V., and Sántha, P. (2018a). The insulin receptor is differentially expressed in somatic and visceral primary sensory neurons. Cell Tissue Res. doi: 10.1007/s00441-018-2868-0 [Epub ahead of print].
Lázár, B. A., Jancsó, G., Oszlács, O., Nagy, I., and Sántha, P. (2018b). The insulin receptor is colocalized with the TRPV1 nociceptive ion channel and neuropeptides in pancreatic spinal and vagal primary sensory neurons. Pancreas 47, 110–115. doi: 10.1097/MPA.0000000000000959
Lilja, J., Laulund, F., and Forsby, A. (2007). Insulin and insulin-like growth factor type-I up-regulate the vanilloid receptor-1 (TRPV1) in stably TRPV1-expressing SH-SY5Y neuroblastoma cells. J. Neurosci. Res. 85, 1413–1419. doi: 10.1002/jnr.21255
Lindsay, R. M., Lockett, C., Sternberg, J., and Winter, J. (1989). Neuropeptide expression in cultures of adult sensory neurons: modulation of substance P and calcitonin gene-related peptide levels by nerve growth factor. Neuroscience 33, 53–65. doi: 10.1016/0306-4522(89)90310-2
Maggi, C. A., and Meli, A. (1988). The sensory-efferent function of capsaicin-sensitive sensory neurons. Gen. Pharmacol. 19, 1–43. doi: 10.1016/0306-3623(88)90002-X
Molliver, D. C., Wright, D. E., Leitner, M. L., Parsadanian, A. S., Doster, K., Wen, D., et al. (1997). IB4-binding DRG neurons switch from NGF to GDNF dependence in early postnatal life. Neuron 19, 849–861. doi: 10.1016/S0896-6273(00)80966-6
Nagy, I., Santha, P., Jancso, G., and Urban, L. (2004). The role of the vanilloid (capsaicin) receptor (TRPV1) in physiology and pathology. Eur. J. Pharmacol. 500, 351–369. doi: 10.1016/j.ejphar.2004.07.037
Nicol, G. D., and Vasko, M. R. (2007). Unraveling the story of NGF-mediated sensitization of nociceptive sensory neurons: ON or OFF the Trks? Mol. Interv. 7, 26–41. doi: 10.1124/mi.7.1.6
Razavi, R., Chan, Y., Afifiyan, F. N., Liu, X. J., Wan, X., Yantha, J., et al. (2006). TRPV1+ sensory neurons control?beta cell stress and islet inflammation in autoimmune diabetes. Cell 127, 1123–1135. doi: 10.1016/j.cell.2006.10.038
Recio-Pinto, E., Rechler, M. M., and Ishii, D. N. (1986). Effects of insulin, insulin-like growth factor-II, and nerve growth factor on neurite formation and survival in cultured sympathetic and sensory neurons. J. Neurosci. 6, 1211–1219. doi: 10.1523/JNEUROSCI.06-05-01211.1986
Santha, P., Oszlacs, O., Dux, M., Dobos, I., and Jancso, G. (2010). Inhibition of glucosylceramide synthase reversibly decreases the capsaicin-induced activation and TRPV1 expression of cultured dorsal root ganglion neurons. Pain 150, 103–112. doi: 10.1016/j.pain.2010.04.006
Sathianathan, V., Avelino, A., Charrua, A., Santha, P., Matesz, K., Cruz, F., et al. (2003). Insulin induces cobalt uptake in a subpopulation of rat cultured primary sensory neurons. Eur. J. Neurosci. 18, 2477–2486. doi: 10.1046/j.1460-9568.2003.03004.x
Shu, X., and Mendell, L. M. (1999). Nerve growth factor acutely sensitizes the response of adult rat sensory neurons to capsaicin. Neurosci. Lett. 274, 159–162. doi: 10.1016/S0304-3940(99)00701-6
Silos-Santiago, I., Molliver, D. C., Ozaki, S., Smeyne, R. J., Fagan, A. M., Barbacid, M., et al. (1995). Non-TrkA-expressing small DRG neurons are lost in TrkA deficient mice. J. Neurosci. 15, 5929–5942. doi: 10.1523/JNEUROSCI.15-09-05929.1995
Singh, B., Xu, Y., McLaughlin, T., Singh, V., Martinez, J. A., Krishnan, A., et al. (2012). Resistance to trophic neurite outgrowth of sensory neurons exposed to insulin. J. Neurochem. 121, 263–276. doi: 10.1111/j.1471-4159.2012.07681.x
Singhal, A., Cheng, C., Sun, H., and Zochodne, D. W. (1997). Near nerve local insulin prevents conduction slowing in experimental diabetes. Brain Res. 763, 209–214. doi: 10.1016/S0006-8993(97)00412-5
Stella, S. L. J., Bryson, E. J., and Thoreson, W. B. (2001). Insulin inhibits voltage-dependent calcium influx into rod photoreceptors. Neuroreport 12, 947–951. doi: 10.1097/00001756-200104170-00017
Takatori, S., Zamami, Y., Yabumae, N., Hanafusa, N., Mio, M., Egawa, T., et al. (2008). Pioglitazone opposes neurogenic vascular dysfunction associated with chronic hyperinsulinaemia. Br. J. Pharmacol. 153, 1388–1398. doi: 10.1038/bjp.2008.8
Toth, C., Brussee, V., Martinez, J. A., McDonald, D., Cunningham, F. A., and Zochodne, D. W. (2006). Rescue and regeneration of injured peripheral nerve axons by intrathecal insulin. Neuroscience 139, 429–449. doi: 10.1016/j.neuroscience.2005.11.065
Tsui, H., Razavi, R., Chan, Y., Yantha, J., and Dosch, H. M. (2007). “Sensing” autoimmunity in type 1 diabetes. Trends Mol. Med. 13, 405–413. doi: 10.1016/j.molmed.2007.07.006
Unger, J., McNeill, T. H., Moxley, R. T. III, White, M., Moss, A., and Livingston, J. N. (1989). Distribution of insulin receptor-like immunoreactivity in the rat forebrain. Neuroscience 31, 143–157. doi: 10.1016/0306-4522(89)90036-5
Van Buren, J. J., Bhat, S., Rotello, R., Pauza, M. E., and Premkumar, L. S. (2005). Sensitization and translocation of TRPV1 by insulin and IGF-I. Mol. Pain 1:17. doi: 10.1186/1744-8069-1-17
Wan, Q., Xiong, Z. G., Man, H. Y., Ackerley, C. A., Braunton, J., Lu, W. Y., et al. (1997). Recruitment of functional GABA(A) receptors to postsynaptic domains by insulin. Nature 388, 686–690. doi: 10.1038/41792
Ward, C. W., and Lawrence, M. C. (2009). Ligand-induced activation of the insulin receptor: a multi-step process involving structural changes in both the ligand and the receptor. Bioessays 31, 422–434. doi: 10.1002/bies.200800210
Winter, J., Forbes, C. A., Sternberg, J., and Lindsay, R. M. (1988). Nerve growth factor (NGF) regulates adult rat cultured dorsal root ganglion neuron responses to the excitotoxin capsaicin. Neuron 1, 973–981. doi: 10.1016/0896-6273(88)90154-7
Xu, Q. G., Li, X. Q., Kotecha, S. A., Cheng, C., Sun, H. S., and Zochodne, D. W. (2004). Insulin as an in vivo growth factor. Exp. Neurol. 188, 43–51. doi: 10.1016/j.expneurol.2004.03.008
Zamami, Y., Takatori, S., Hobara, N., Yabumae, N., Tangsucharit, P., Jin, X., et al. (2011). Hyperinsulinemia induces hypertension associated with neurogenic vascular dysfunction resulting from abnormal perivascular innervations in rat mesenteric resistance arteries. Hypertens. Res. 34, 1190–1196. doi: 10.1038/hr.2011.97
Keywords: insulin, neurite outgrowth, dorsal root ganglion neurons, insulin receptor, transient receptor potential vanilloid type 1 receptor, calcitonin gene-related peptide, isolectin B4
Citation: Lázár BA, Jancsó G, Pálvölgyi L, Dobos I, Nagy I and Sántha P (2018) Insulin Confers Differing Effects on Neurite Outgrowth in Separate Populations of Cultured Dorsal Root Ganglion Neurons: The Role of the Insulin Receptor. Front. Neurosci. 12:732. doi: 10.3389/fnins.2018.00732
Received: 08 July 2018; Accepted: 24 September 2018;
Published: 10 October 2018.
Edited by:
Gustavo M. Somoza, Instituto de Investigaciones Biotecnológicas (IIB-INTECH), ArgentinaReviewed by:
Kazunori Sango, Tokyo Metropolitan Institute of Medical Science, JapanGang Chen, Nantong University, China
Copyright © 2018 Lázár, Jancsó, Pálvölgyi, Dobos, Nagy and Sántha. This is an open-access article distributed under the terms of the Creative Commons Attribution License (CC BY). The use, distribution or reproduction in other forums is permitted, provided the original author(s) and the copyright owner(s) are credited and that the original publication in this journal is cited, in accordance with accepted academic practice. No use, distribution or reproduction is permitted which does not comply with these terms.
*Correspondence: Bence András Lázár, b.a.lazar.md@gmail.com