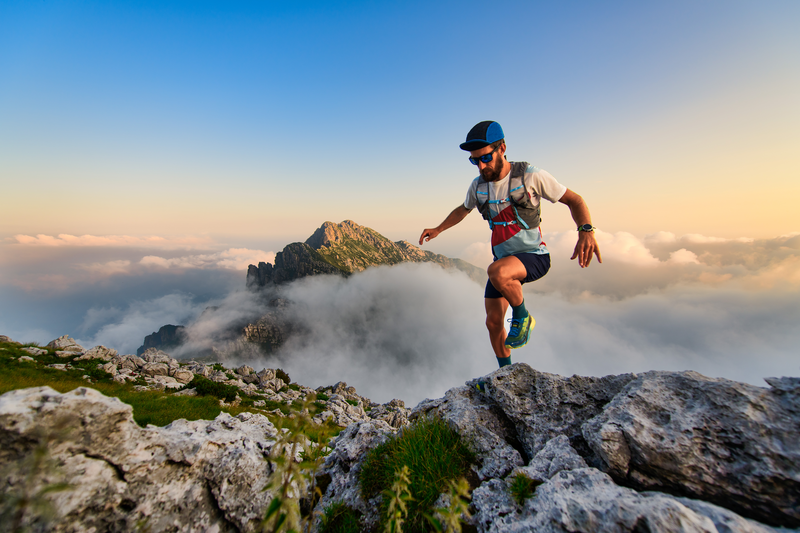
94% of researchers rate our articles as excellent or good
Learn more about the work of our research integrity team to safeguard the quality of each article we publish.
Find out more
OPINION article
Front. Neurosci. , 21 August 2018
Sec. Perception Science
Volume 12 - 2018 | https://doi.org/10.3389/fnins.2018.00578
Cross-modal interactions are common in sensory processing, and phenomena reach from changed perception within one modality due to input from another like in the well-known McGurk effect (McGurk and MacDonald, 1976) to misperceptions like synesthesia (Marks, 1975). A recent study demonstrated that electro-tactile stimulation applied to the index finger significantly improves speech perception thresholds (Huang et al., 2017). Here we argue that such cross-modal enhancement can be explained in terms of stochastic resonance (Benzi et al., 1981), a phenomenon that is ubiquitous in nature (Wiesenfeld and Moss, 1995), and especially within the context of neuroscience, receives increasing attention (Douglass et al., 1993; Moss et al., 2004; Faisal et al., 2008; Mino, 2014).
Stochastic resonance refers to a processing principle where signals otherwise sub-threshold for a given sensor can, at least partially, be detected anyway by adding noise of a suitable intensity to the sensor input (Benzi et al., 1981; Collins et al., 1996; Levin and Miller, 1996; Gammaitoni et al., 1998).
In self-adaptive signal detection systems based on stochastic resonance, the optimum noise level is continuously adjusted via a feed-back loop, so that the system response in terms of information throughput remains optimal, even if the properties of the input signal change. For this processing principle the term adaptive stochastic resonance has been coined (Mitaim and Kosko, 1998, 2004; Wenning and Obermayer, 2003). Most objective functions to quantify such information transmission, e.g., mutual information, require knowledge of the signal to be detected (Levin and Miller, 1996; Mitaim and Kosko, 2004; Moss et al., 2004). In a previous study we demonstrated that the autocorrelation of the sensor output, a quantity always accessible and easy to analyze by neuronal nets, can be used to quantify and hence maximize information transmission even for unknown and variable input signals (Krauss et al., 2017). In a further study we demonstrated by implementing a phenomenological computational model that adaptive stochastic resonance based on output autocorrelations might be a major processing principle of the auditory system (Krauss et al., 2016) that serves to partially compensate for acute or chronic hearing loss, e.g., due to cochlear damage (Krauss et al., 2016; Gollnast et al., 2017). In that view, the noise necessary for stochastic resonance to work corresponds to increased spontaneous neuronal firing rates in early processing stages of the auditory brainstem and cortex, a phenomenon that has been observed in animal models and human subjects with central tinnitus (Wang et al., 1997; Ahlf et al., 2012; Tziridis et al., 2015; Wu et al., 2015). In fact we have proposed earlier that the noise necessary for stochastic resonance is the neurophysiological correlate of tinnitus-related enhanced neuronal activity, and that in this view tinnitus is a side effect of an adaptive mechanism within the auditory system whose main purpose is to compensate for hearing loss by constantly optimizing information transmission (Krauss et al., 2016).
Within the auditory system, the dorsal cochlear nucleus (DCN) is the earliest processing stage in the auditory pathway in which acoustic trauma leads to tinnitus-related changes and increased spontaneous firing rates (Kaltenbach et al., 1998, 2004; Kaltenbach and Afman, 2000; Brozoski et al., 2002; Zacharek et al., 2002; Wu et al., 2015). The amount of this increase in spontaneous activity in the DCN has been shown to be correlated with the strength of the behavioral signs of tinnitus (Kaltenbach et al., 2004). Furthermore, this hyperactivity is only found in regions innervated by the damaged parts of the cochlear receptor epithelium (Kaltenbach et al., 2002). Gao and colleagues recently described changes in DCN fusiform cell spontaneous activity after noise exposure that supports our proposed stochastic resonance mechanism. In particular, the time course of spontaneous rate changes shows an almost complete loss of spontaneous activity immediately after loud sound exposure (as no stochastic resonance is needed due to stimulation that is well above threshold), followed by an overcompensation of spontaneous rates to levels well above pre-exposition rates where stochastic resonance is now needed to compensate for acute hearing loss (Gao et al., 2016).
It is well-known that the DCN receives not only input from the cochlea, but also from the somatosensory system (Ryugo et al., 2003; Shore and Zhou, 2006; Dehmel et al., 2012; Zeng et al., 2012). Therefore, in a previous paper we proposed the possibility that the neuronal noise which is crucial for stochastic resonance to work may be injected into the auditory system via somatosensory projections (Krauss et al., 2016). This idea is supported by a number of papers.
For example, it is well-known that jaw movements lead to a modulation of tinnitus sensation in patients (Pinchoff et al., 1998). This may easily be explained within our model, as jaw movements alter somatosensory input to the DCN. Since this somatosensory input would correspond to the noise which is crucial for stochastic resonance, auditory input to the DCN is modulated through this mechanism and the altered noise level would be perceived as modulated tinnitus (Krauss et al., 2016). Tang et al. demonstrated that somatosensory (noise) input and hence tinnitus sensation may also be modified by serotonergic regulation of excitability of principal cells of the DCN (Tang and Trussell, 2015, 2017).
In addition, the finding that DCN responses to somatosensory stimulation are enhanced after noise-induced hearing loss (Shore et al., 2008) supports our idea that stochastic resonance plays a key role in auditory processing and actually takes place in the DCN. After hearing-loss, the auditory input to the DCN is decreased. By that, information transmission is reduced leading to an increase of internally generated neuronal noise which is crucial for adaptive stochastic resonance to work and partially restore hearing thresholds.
Finally, the finding that electro-tactile stimulation applied to the index finger significantly improves speech perception thresholds (Huang et al., 2017) further supports our interpretation that somatosensory input to the DCN corresponds to the noise input for stochastic resonance. Again, electro-tactile stimulation increases somatosensory input which is equivalent to increased neuronal noise, which in turn improves detection thresholds for auditory stimuli via stochastic resonance.
In a previous paper we already discussed the possibility of superseding the internally generated neuronal noise by adding external acoustic noise, thereby suppressing the tinnitus percept (Krauss et al., 2016). Remarkably, a recent publication demonstrated that exposure to moderate levels of white noise after acoustic trauma prevented most individuals from developing tinnitus in a mouse model (Sturm et al., 2017). Along the same line, Marks and coworkers found that auditory-somatosensory bimodal stimulation reduces tinnitus in guinea pigs and humans (Marks et al., 2018). Within the framework of our modal one can argue that simultaneous auditory and somatosensory stimulation corresponds to a combination of the two aforementioned studies (Huang et al., 2017; Sturm et al., 2017).
Based on these observations we here speculate that stochastic resonance in one sensory modality driven by input from another modality may be a general principle, namely multisensory integration causing stochastic resonance like cross-modal enhancement. For example, it is known that the concept of stochastic resonance via internal noise applies also to visual perception (Aihara et al., 2008). Furthermore, analogous to the above discussed somatosensory enhancement of auditory perception, visual perception at threshold can be enhanced by auditory stimulation (Caclin et al., 2011) and that even visual below threshold stimuli may be perceived through spatially converging audiovisual inputs (Bolognini et al., 2005). Such audiovisual cross-modal improvement of detection thresholds has been demonstrated in a broad range of different species, e.g., the ferret (Hollensteiner et al., 2015) or the chicken (Verhaal and Luksch, 2016).
In addition to the here proposed neuronal mechanism of somatosensory-driven stochastic resonance in the auditory system explaining the better hearing in the CI patients during finger tapping (Huang et al., 2017), alternative explanations are discussed: First, an attentional effect modulating the sensory threshold has been proposed (for Review see Sarter et al., 2005). Second, due to the fact that patients are dealing with a multimodal integration task, the interaction of both stimuli can mathematically be explained by the “optimal observer” model of sensory integration based on maximum-likelihood estimation theory as already shown in visual and auditory (e.g., Battaglia et al., 2003) and visual and somatosensory interaction (Hollensteiner et al., 2015). Nevertheless, both alternative interpretations would most likely interact on the level of cortical representations of the sensory modalities. Hence, to proof or falsify our hypothesis proposed here that auditory-somatosensory interaction takes place at the level of the dorsal cochlear nucleus, neuronal recordings in the dorsal cochlear nucleus should be performed in search of a somatosensory driven modulation of auditory neuron spontaneous activity in combination with lowered neuronal and/or behavioral thresholds, Nevertheless, finding such interaction within the DCN would not disproof that cross-modal interactions between other modalities and/or in other contexts might take place at the level of sensory cortex.
Even though multisensory integration leading to improved perception has been reported earlier (Stein and Stanford, 2008), we here provide a new mechanistic explanation of how this enhancement might work. We hypothesize that this mechanism represents a universal principle of neural computation.
PK and HS wrote the manuscript. PK, HS, KT, and AS discussed possible interpretations of the cited studies and developed the hypothesis presented in this paper.
The authors declare that the research was conducted in the absence of any commercial or financial relationships that could be construed as a potential conflict of interest.
The reviewer SV and the handling editor declared their shared affiliation.
This work was supported by the Deutsche Forschungsgemeinschaft (DFG-grant SCHU 1272/12-1).
Ahlf, S., Tziridis, K., Korn, S., Strohmeyer, I., and Schulze, H. (2012). Predisposition for and prevention of subjective tinnitus development. PLoS ONE 7:e44519. doi: 10.1371/journal.pone.0044519
Aihara, T., Kitajo, K., Nozaki, D., and Yamamoto, Y. (2008). Internal noise determines external stochastic resonance in visual perception. Vis. Res. 48, 1569–1573. doi: 10.1016/j.visres.2008.04.022
Battaglia, P. W., Jacobs, R. A., and Aslin, R. N. (2003). Bayesian integration of visual and auditory signals for spatial localization. J. Opt. Soc. Am. A. Opt. Image Sci. Vis. 20, 1391–1397. doi: 10.1364/JOSAA.20.001391
Benzi, R., Sutera, A., and Vulpiani, A. (1981). The mechanism of stochastic resonance. J. Phys. A Math. Gen. 14:L453.
Bolognini, N., Frassinetti, F., Serino, A., and Làdavas, E. (2005). “Acoustical vision” of below threshold stimuli: interaction among spatially converging audiovisual inputs. Exp. Brain Res. 160, 273–282. doi: 10.1007/s00221-004-2005-z
Brozoski, T. J., Bauer, C. A., and Caspary, D. M. (2002). Elevated fusiform cell activity in the dorsal cochlear nucleus of chinchillas with psychophysical evidence of tinnitus. J. Neurosci. 22, 2383–2390. doi: 10.1523/JNEUROSCI.22-06-02383.2002
Caclin, A., Bouchet, P., Djoulah, F., Pirat, E., Pernier, J., and Giard, M. H. (2011). Auditory enhancement of visual perception at threshold depends on visual abilities. Brain Res. 1396, 35–44. doi: 10.1016/j.brainres.2011.04.016
Collins, J. J., Imhoff, T. T., and Grigg, P. (1996). Noise-enhanced information transmission in rat SA1 cutaneous mechanoreceptors via aperiodic stochastic resonance. J. Neurophysiol. 76, 642–645. doi: 10.1152/jn.1996.76.1.642
Dehmel, S., Pradhan, S., Koehler, S., Bledsoe, S., and Shore, S. (2012). Noise overexposure alters long-term somatosensory-auditory processing in the dorsal cochlear nucleus–possible basis for tinnitus-related hyperactivity? J. Neurosci. 32, 1660–1671. doi: 10.1523/JNEUROSCI.4608-11.2012
Douglass, J. K., Wilkens, L., Pantazelou, E., and Moss, F. (1993). Noise enhancement of information transfer in crayfish mechanoreceptors by stochastic resonance. Nature 365, 337–340. doi: 10.1038/365337a0
Faisal, A. A., Selen, L. P., and Wolpert, D. M. (2008). Noise in the nervous system. Nat. Rev. Neurosci. 9, 292–303. doi: 10.1038/nrn2258
Gammaitoni, L., Hänggi, P., Jung, P., and Marchesoni, F. (1998). Stochastic resonance. Rev. Mod. Phys. 70:223. doi: 10.1103/RevModPhys.70.223
Gao, Y., Manzoor, N., and Kaltenbach, J. A. (2016). Evidence of activity-dependent plasticity in the dorsal cochlear nucleus, in vivo, induced by brief sound exposure. Hear. Res. 341, 31–42. doi: 10.1016/j.heares.2016.07.011
Gollnast, D., Tziridis, K., Krauss, P., Schilling, A., Hoppe, U., and Schulze, H. (2017). Analysis of audiometric differences of patients with and without Tinnitus in a large clinical database. Front. Neurol. 8:31. doi: 10.3389/fneur.2017.00031
Hollensteiner, K. J., Pieper, F., Engler, G., König, P., and Engel, A. K. (2015). Crossmodal integration improves sensory detection thresholds in the ferret. PLoS ONE 10:e0124952. doi: 10.1371/journal.pone.0124952
Huang, J., Sheffield, B., Lin, P., and Zeng, F. G. (2017). Electro-tactile stimulation enhances cochlear implant speech recognition in noise. Sci. Rep. 7:2196. doi: 10.1038/s41598-017-02429-1
Kaltenbach, J. A., and Afman, C. E. (2000). Hyperactivity in the dorsal cochlear nucleus after intense sound exposure and its resemblance to tone-evoked activity: a physiological model for tinnitus. Hear. Res. 140, 165–172. doi: 10.1016/S0378-5955(99)00197-5
Kaltenbach, J. A., Godfrey, D. A., Neumann, J. B., McCaslin, D. L., Afman, C. E., and Zhang, J. (1998). Changes in spontaneous neural activity in the dorsal cochlear nucleus following exposure to intense sound: relation to threshold shift. Hear. Res. 124, 78–84. doi: 10.1016/S0378-5955(98)00119-1
Kaltenbach, J. A., Rachel, J. D., Mathog, T. A., Zhang, J., Falzarano, P. R., and Lewandowski, M. (2002). Cisplatin-induced hyperactivity in the dorsal cochlear nucleus and its relation to outer hair cell loss: relevance to tinnitus. J. Neurophysiol. 88, 699–714. doi: 10.1152/jn.2002.88.2.699
Kaltenbach, J. A., Zacharek, M. A., Zhang, J., and Frederick, S. (2004). Activity in the dorsal cochlear nucleus of hamsters previously tested for tinnitus following intense tone exposure. Neurosci. Lett. 355, 121–125. doi: 10.1016/j.neulet.2003.10.038
Krauss, P., Metzner, C., Schilling, A., Schütz, C., Tziridis, K., Fabry, B., et al. (2017). Adaptive stochastic resonance for unknown and variable input signals. Sci. Rep. 7:2450. doi: 10.1038/s41598-017-02644-w
Krauss, P., Tziridis, K., Metzner, C., Schilling, A., Hoppe, U., and Schulze, H. (2016). Stochastic resonance controlled upregulation of internal noise after hearing loss as a putative cause of tinnitus-related neuronal hyperactivity. Front. Neurosci. 10:597. doi: 10.3389/fnins.2016.00597
Levin, J. E., and Miller, J. P. (1996). Broadband neural encoding in the cricket cercal sensory system enhanced by stochastic resonance. Nature 380, 165–168. doi: 10.1038/380165a0
Marks, K. L., Martel, D. T., Wu, C., Basura, G. J., Roberts, L. E., Schvartz-Leyzac, K. C., et al. (2018). Auditory-somatosensory bimodal stimulation desynchronizes brain circuitry to reduce tinnitus in guinea pigs and humans. Sci. Transl. Med. 10:eaal3175. doi: 10.1126/scitranslmed.aal3175
Marks, L. E. (1975). On colored-hearing synesthesia: cross-modal translations of sensory dimensions. Psychol. Bull. 82:303. doi: 10.1037/0033-2909.82.3.303
McGurk, H., and MacDonald, J. (1976). Hearing lips and seeing voices. Nature 264, 746–748. doi: 10.1038/264746a0
Mino, H. (2014). The effects of spontaneous random activity on information transmission in an auditory brain stem neuron model. Entropy 16, 6654–6666. doi: 10.3390/e16126654
Mitaim, S., and Kosko, B. (1998). Adaptive stochastic resonance. Proc. IEEE 86, 2152–2183. doi: 10.1109/5.726785
Mitaim, S., and Kosko, B. (2004). Adaptive stochastic resonance in noisy neurons based on mutual information. IEEE Trans. Neural Netw. 15, 1526–1540. doi: 10.1109/TNN.2004.826218
Moss, F., Ward, L. M., and Sannita, W. G. (2004). Stochastic resonance and sensory information processing: a tutorial and review of application. Clin. Neurophysiol. 115, 267–281. doi: 10.1016/j.clinph.2003.09.014
Pinchoff, R. J., Burkard, R. F., Salvi, R. J., Coad, M. L., and Lockwood, A. H. (1998). Modulation of tinnitus by voluntary jaw movements. Otol. Neurotol. 19, 785–789.
Ryugo, D. K., Haenggeli, C. A., and Doucet, J. R. (2003). Multimodal inputs to the granule cell domain of the cochlear nucleus. Exp. Brain Res. 153, 477–485. doi: 10.1007/s00221-003-1605-3
Sarter, M., Hasselmo, M. E., Bruno, J. P., and Givens, B. (2005). Unraveling the attentional functions of cortical cholinergic inputs: interactions between signal-driven and cognitive modulation of signal detection. Brain Res. Rev. 48, 98–111. doi: 10.1016/j.brainresrev.2004.08.006
Shore, S. E., Koehler, S., Oldakowski, M., Hughes, L. F., and Syed, S. (2008). Dorsal cochlear nucleus responses to somatosensory stimulation are enhanced after noise-induced hearing loss. Eur. J. Neurosci. 27, 155–168. doi: 10.1111/j.1460-9568.2007.05983.x
Shore, S. E., and Zhou, J. (2006). Somatosensory influence on the cochlear nucleus and beyond. Hear. Res. 216, 90–99. doi: 10.1016/j.heares.2006.01.006
Stein, B. E., and Stanford, T. R. (2008). Multisensory integration: current issues from the perspective of the single neuron. Nat. Rev. Neurosci. 9, 255–266. doi: 10.1038/nrn2331
Sturm, J. J., Zhang-Hooks, Y. X., Roos, H., Nguyen, T., and Kandler, K. (2017). Noise trauma-induced behavioral gap detection deficits correlate with reorganization of excitatory and inhibitory local circuits in the inferior colliculus and are prevented by acoustic enrichment. J. Neurosci. 37, 6314–6330. doi: 10.1523/JNEUROSCI.0602-17.2017
Tang, Z. Q., and Trussell, L. O. (2015). Serotonergic regulation of excitability of principal cells of the dorsal cochlear nucleus. J. Neurosci. 35, 4540–4551. doi: 10.1523/JNEUROSCI.4825-14.2015
Tang, Z. Q., and Trussell, L. O. (2017). Serotonergic modulation of sensory representation in a central multisensory circuit is pathway specific. Cell Rep. 20, 1844–1854. doi: 10.1016/j.celrep.2017.07.079
Tziridis, K., Ahlf, S., Jeschke, M., Happel, M. F., Ohl, F. W., and Schulze, H. (2015). Noise trauma induced neural plasticity throughout the auditory system of Mongolian gerbils: differences between tinnitus developing and non-developing animals. Front. Neurol. 6:22. doi: 10.3389/fneur.2015.00022
Verhaal, J., and Luksch, H. (2016). Multimodal integration in the chicken. J. Exp. Biol. 219, 90–95. doi: 10.1242/jeb.129387
Wang, J., Powers, N. L., Hofstetter, P., Trautwein, P., Ding, D., and Salvi, R. (1997). Effects of selective inner hair cell loss on auditory nerve fiber threshold, tuning and spontaneous and driven discharge rate. Hear. Res. 107, 67–82. doi: 10.1016/S0378-5955(97)00020-8
Wenning, G., and Obermayer, K. (2003). Activity driven adaptive stochastic resonance. Phys. Rev. Lett. 90:120602. doi: 10.1103/PhysRevLett.90.120602
Wiesenfeld, K., and Moss, F. (1995). Stochastic resonance and the benefits of noise: from ice ages to crayfish and SQUIDs. Nature 373, 33–36. doi: 10.1038/373033a0
Wu, C., Stefanescu, R. A., Martel, D. T., and Shore, S. E. (2015). Tinnitus: maladaptive auditory–somatosensory plasticity. Hear. Res. 334, 20–29. doi: 10.1016/j.heares.2015.06.005
Zacharek, M. A., Kaltenbach, J. A., Mathog, T. A., and Zhang, J. (2002). Effects of cochlear ablation on noise induced hyperactivity in the hamster dorsal cochlear nucleus: implications for the origin of noise induced tinnitus. Hear. Res. 172, 137–144. doi: 10.1016/S0378-5955(02)00575-0
Keywords: stochastic resonance, cross-modal perception, neural processing, dorsal cochlear nucleus, tinnitus
Citation: Krauss P, Tziridis K, Schilling A and Schulze H (2018) Cross-Modal Stochastic Resonance as a Universal Principle to Enhance Sensory Processing. Front. Neurosci. 12:578. doi: 10.3389/fnins.2018.00578
Received: 13 March 2018; Accepted: 30 July 2018;
Published: 21 August 2018.
Edited by:
Kathrin Ohla, Institut für Neurowissenschaften und Medizin, Forschungszentrum Jülich, GermanyReviewed by:
Manfred Kössl, Institut für Zellbiologie & Neurowissenschaft, Goethe-Universität Frankfurt am Main, GermanyCopyright © 2018 Krauss, Tziridis, Schilling and Schulze. This is an open-access article distributed under the terms of the Creative Commons Attribution License (CC BY). The use, distribution or reproduction in other forums is permitted, provided the original author(s) and the copyright owner(s) are credited and that the original publication in this journal is cited, in accordance with accepted academic practice. No use, distribution or reproduction is permitted which does not comply with these terms.
*Correspondence: Holger Schulze, aG9sZ2VyLnNjaHVsemVAdWstZXJsYW5nZW4uZGU=
Disclaimer: All claims expressed in this article are solely those of the authors and do not necessarily represent those of their affiliated organizations, or those of the publisher, the editors and the reviewers. Any product that may be evaluated in this article or claim that may be made by its manufacturer is not guaranteed or endorsed by the publisher.
Research integrity at Frontiers
Learn more about the work of our research integrity team to safeguard the quality of each article we publish.