- 1The Swedish School of Sport and Health Sciences (GIH), Stockholm, Sweden
- 2School of Health Sciences and Priority Research Centre for Stroke and Brain Injury, University of Newcastle, Newcastle, NSW, Australia
Introduction: Extended periods of sitting may have detrimental effects on brain health. However, the effects of breaking up prolonged sedentary periods with frequent, short physical activity bouts on mechanisms to improve brain health remain unclear. Therefore, this study aims to investigate the immediate effects of uninterrupted sitting and frequent, short bouts of physical activity on cerebral blood flow and cognitive function in the prefrontal cortex in middle-aged adults.
Methods: This is a protocol article to describe a randomized crossover study. We will collect data from 13 healthy adults, aged between 40 and 60 years old, with a body mass index <35 kg/m2. Participants will be required to come into the laboratory on three occasions, sit for 3 h, and perform a different type of break for 3 min every 30 min at each visit in a random order, being either: (1) a social break; (2) brisk walk on a treadmill; or (3) simple resistance activities. Before and after each experimental condition, cerebral blood flow (primary outcome) will be measured using functional near-infrared spectroscopy (fNIRS), with short-separation channels, and working memory (1-, 2-, and 3-back on the computer) will be assessed. The following additional secondary outcomes will be collected: psychological factors (questionnaires); arterial stiffness; salivary cortisol levels; and blood glucose levels.
Conclusion: The results from this randomized crossover study will determine the effects of uninterrupted sitting and frequent, short bouts of physical activity on cerebral blood flow and cognitive performance. Publication of this study protocol emphasizes the importance of registration and publication of protocols in the field of sedentary behavior research.
Introduction
Sedentary behavior is gaining more attention owing to its association with increased risk of cardiovascular diseases and early mortality (Biswas et al., 2015; Patterson et al., 2018; Ekelund et al., 2019), and potential adverse effects on cognitive and mental health (Voss et al., 2014; Wheeler et al., 2017). Understanding the neurophysiological consequences of sedentary behavior is important considering that adults spend about two-thirds of their waking time and the majority of working hours in long periods of uninterrupted sitting (Parry and Straker, 2013).
Physical activity is known to promote healthy brain functions (i.e., cognitive and mental health; Angevaren et al., 2008; Hillman et al., 2008; Gomez-Pinilla and Hillman, 2013); and a candidate mechanism by which this can occur is through increased cerebral blood flow (Ogoh and Ainslie, 2009), particularly in the prefrontal cortex (Herold et al., 2018).
In light of the negative effects of sedentary behavior on vascular outcomes, it is possible that prolonged sitting may have adverse effects on brain health by reducing cerebral blood flow. However, sedentary behavior’s effects on cerebral blood flow, the mitigating effects of physical activity breaks, and what types of physical activity breaks remain unknown. A few studies, in younger- or older-aged populations, have demonstrated immediate improvements in cerebral blood flow from a single exercise session (Chang et al., 2012; Herold et al., 2018; Ji et al., 2019), whereas the influence of breaking up a sitting day with frequent (e.g., 30 min), short (e.g., 3 min) bouts of physical activity on increasing cerebral blood flow is less clear. Using a surrogate measure of cerebral blood flow (middle cerebral artery blood flow velocity), one study of healthy desk workers found immediate increases in blood flow velocity after short, frequent bouts of walking compared with uninterrupted sitting for 4 h (Carter et al., 2018). Also, in a study of older adults, middle cerebral artery blood flow velocity increased over a day of interrupted sitting with light-intensity walking breaks for 3 min every 30 min, after performing a 30-min moderate-intensity walk in the morning before the prolonged sitting time (Wheeler et al., 2019). However, another study of young adults found a reduction in cerebral blood flow and cognition after short, frequent bouts of calf raises over 3 h, compared to uninterrupted sitting for 3 h (Stoner et al., 2019). Finally, a study of 19 overweight and obese adults demonstrated improved fatigue after short, frequent bouts of light-intensity walking, which may be explained by improved cerebrovascular regulation, although this was not directly measured (Wennberg et al., 2016). Thus, results from previous studies are inconsistent and emphasize the need for further studies. Middle-aged adults may be at particular risk as low cerebral blood flow may contribute to future cognitive decline (Bertsch et al., 2009).
Moderate-to-vigorous intensity physical activity levels are often not attainable by most people and knowing the negative health effects of sedentary behavior, strategies to increase physical activity are needed that are manageable for long term (Dunstan et al., 2018). Earlier studies have demonstrated positive effects of frequent (e.g., 30 min), short (e.g., 3 min) breaks of physical activity on glycemic control (Dunstan et al., 2012; Dempsey et al., 2017), arterial health (Carter et al., 2019), middle cerebral artery velocity (Wheeler et al., 2019), fatigue (Wennberg et al., 2016), and cognitive performance (Wheeler et al., 2020). However, these feasible breaks have yet to be studied concerning simultaneously measured cerebral blood flow and cognitive performance.
Functional-near infrared spectroscopy (fNIRS) is an emerging neuroimaging technique that allows for accurate measurement of cerebral blood flow, such as in the prefrontal cortex (Herold et al., 2018). Other neuroimaging methods to measure cerebral blood flow, such as positron emission tomography and functional magnetic resonance imaging (fMRI) are cumbersome, due to the size and expense of the equipment, the need for participants to be supine, and high sensitivity to movement artifacts. By contrast, fNIRS is a more ecologically valid method to study cerebral blood flow, as measurement can be performed in various postures and settings. It is portable, non-invasive, cost-effective, and accurate when compared with fMRI measures (Huppert et al., 2006). One concern with fNIRS is that changes in superficial (i.e., extra-cerebral) blood flow may confound measures. Including short-separation channels in fNIRS systems allows the direct measurement of extra-cerebral blood flow thereby eliminating this as a confounder (Yücel et al., 2015), yet has rarely been used to date, but will be used in this study. In middle- and older-aged adults, vascular health and in particular arterial stiffness may moderate the effect of physical activity on cerebral blood flow (Elias et al., 2009; Tarumi et al., 2013) and is therefore also important to investigate.
The overall aim of this study is to explore the effect of uninterrupted sitting and frequent, short bouts of physical activity during a prolonged period of sitting on cerebral blood flow and cognitive function.
Our primary research question is:
1 In middle-aged adults, what are the acute (immediate) effects of uninterrupted sitting and frequent, short activity breaks during 3 h of sitting on cerebral blood flow in the prefrontal cortex?
Our secondary research questions are:
2 In middle-aged adults, what are the acute (immediate) effects of uninterrupted sitting and frequent short activity breaks during 3 h of sitting on:
(a) Cognitive performance?
(b) Psychological factors (stress, mood, alertness, and sleepiness)?
(c) Post-prandial blood glucose?
3 Does vascular health (arterial stiffness) attenuate the effects of activity breaks on cerebral blood flow?
Based on previous research, we hypothesize that:
(a) Changes in neural activity-related prefrontal cortex cerebral blood flow (hereafter termed cerebral blood flow) from pre-test to post-test will be greater following the conditions with frequent, short activity breaks compared with the uninterrupted sitting condition;
(b) Cerebral blood flow will be greater post-intervention compared to pre-intervention for the frequent, short activity breaks conditions, but not for the uninterrupted sitting condition;
(c) Improvements in cognitive performance will be larger in magnitude in the frequent, short activity breaks conditions compared with the uninterrupted sitting condition;
(d) The frequent, short activity break conditions will lead to improvements in psychological factors, compared with the uninterrupted sitting condition;
(e) Glucose levels will not differ between the conditions;
(f) Arterial stiffness will temporarily decrease immediately after the frequent, short activity break conditions (compared to pre-test levels), but will not change after the uninterrupted sitting condition; and
(g) Arterial stiffness will moderate the effect of frequent, short activity breaks on cerebral blood flow, such that participants with lower arterial stiffness at baseline will have greater cerebral blood flow after the frequent, short activity break conditions compared with participants with higher arterial stiffness.
Materials and Methods
Design
A crossover randomized 3-condition experimental trial will be carried out with a target sample size of 13 participants. All study procedures will occur at the Swedish School of Sport and Health Sciences (GIH) in Stockholm, Sweden. Participants will be recruited from the general Swedish population using social media, GIH’s homepage, and newspaper advertisements. As well, through the Swedish Armed Forces’ personnel magazine and word of mouth. Participants will initially attend a familiarization session for the collection of demographic data, fitness testing, and acquaintance with experimental procedures. Each participant will undergo 3-h experimental conditions in random order, with a minimum washout period of 4 days between each of the three visits (Bell et al., 2018). Physical activity, sleep, and glucose levels will be measured for the 24 h before each condition. A computer-generated randomization schedule will be created before study commencement, and participants will be randomized to a condition order at the end of the familiarization session. This trial obtained ethical approval by the Swedish Ethical Review Authority, Stockholm, Sweden (Dnr 2019-00998). Trial registration for experimental studies of this nature is not required but is increasingly being recognized as good practice (English et al., 2020). Therefore, we registered this trial at www.clinicaltrials.gov (NCT04137211) on October 23, 2019, after recruitment began on May 13, 2019.
Participants
We will include adults 40–60 years old, with a body mass index <35 kg/m2. People who have been diagnosed with diabetes, epilepsy, heart failure, stroke, or myocardial infarction, or other circulatory conditions will be excluded. Also, persons receiving current treatment for high blood pressure, sleep disorders, depression, or psychosis.
Familiarization
Before randomization, participants will come to the laboratory at GIH for a familiarization visit where they will be introduced to all procedures of the study, including a practice of the cognitive tests. Demographic and other data to describe the sample will be collected including age, sex, height, weight, head circumference (to determine the optimal fNIRS cap-size), and a health screening questionnaire. Participants will also undergo an incremental treadmill test to establish their maximal oxygen uptake (VO2max) using a standard protocol and the OxyCon Pro metabolic system (Erich Jaeger GmbH, Hochenberg, Germany). The results of the fitness test will be used to determine the intensity of the walking break condition.
Pre-experimental Day Monitoring
To standardize the 24 h before each test day, participants will be advised to abstain from any physical activity and alcohol intake and will be asked to record details about their physical activity and sleep in a standardized diary. Participants will also wear activity monitors during this period to collect objective data about physical activity (hip-worn actigraph GT3X+ and activPAL micro) and sleep (wrist-worn actigraph GT3X+). Physical activity variables from the actigraph will include total physical activity and percentages of wear time spent in sedentary, light, and moderate-to-vigorous physical activity, according to standardized cut-points (Sasaki et al., 2011; Aguilar-Farias et al., 2014). Physical activity variables from the activPAL micro monitor will include time spent sitting including in prolonged bouts (>20 min), number of breaks from sitting each hour and time spent standing, stepping, and intense stepping. During night time, participants will wear the actigraph GT3X+ on the non-dominant wrist (Cellini et al., 2013). Using the existing Cole-Kripke algorithm (Cole et al., 1992), the following key aspects of sleep will be calculated: time to sleep onset, sleep efficiency, times waking up after sleep onset, and total sleep.
To standardize dietary intake and blood glucose levels, participants will be asked to record their dietary intake for the 24 h before each condition in a standardized food diary and closely match their diet for each pre-condition day. They will also be asked to fast and abstain from caffeine after dinner the night before. Additionally, participants will wear a continuous blood glucose monitor (Abbott FreeStyle Libre sensor) during the 24 h before, and during each experimental condition.
Experimental Conditions
To minimize physical activity the morning of the visit participants will be transported to the laboratory via taxi. On the morning of testing, participants will be asked to provide four saliva samples (for cortisol testing); immediately after waking and at 30, 45, and 60 min thereafter. Once they arrive at the laboratory, pre-condition outcome measures will be collected, followed by an individually standardized breakfast (consisting of maximum two slices of bread with butter and cheese, a bowl of yogurt, a banana, and a glass of water), with no specific time restriction for eating. Participants at their initial visit will be advised to eat their preferred amount, which will be subsequently weighed and recorded. The portion sizes will be then replicated at each visit. The activPAL activity monitor and glucose monitor will be worn during the experimental conditions to monitor sitting time during the test day.
The three experimental conditions (summarized in Figure 1) are: (A) uninterrupted sitting for 3 h with a 3-min social break every 30 min (SOCIAL); (B) sitting for 3 h with a 3-min bout of moderate-intensity walking on a treadmill every 30 min (WALK), or (C) sitting for 3 h with a 3-min bout of simple resistance activities every 30 min (SRA). During the sitting, participants will be permitted to read a book and drink water, but not use any technological devices or doze off. The SOCIAL break (condition A) will consist of a 3-min chat between the research staff and the participant. The WALK break (condition B) will be performed at moderate intensity (75–80% of maximal heart rate), normalized to individual participants’ VO2max (see Supplementary Material). For the SRA break (condition C), participants will be asked to complete 20-s bodyweight half-squats, 20-s calf raises, and 20-s gluteal contractions and knee raises repeated three times in sequential order by following standardized video instructions (as per Dempsey et al., 2017). At 135 min after the experimental condition commences, participants will be offered a toilet break.
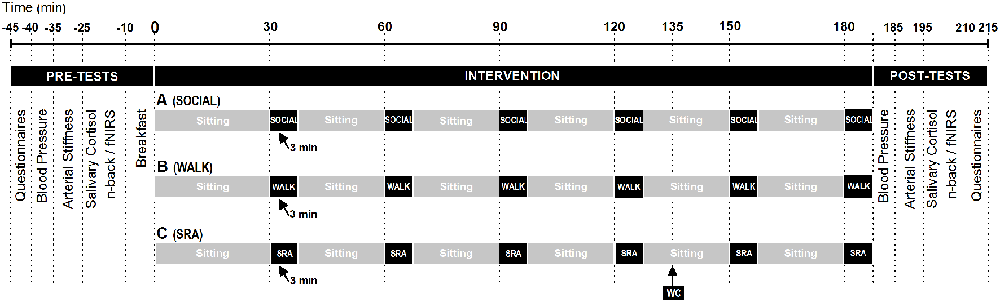
Figure 1. Experimental day procedures of the three conditions after a standardized breakfast. Condition A: 3 h of sitting with 3-min social breaks every 30 min (SOCIAL); Condition B: sitting 3 h with a 3-min bout of brisk walking on a treadmill every 30 min (WALK); Condition C: sitting for 3 h with a 3-min bout of simple resistance activities every 30 min (SRA). WC: toilet break; fNIRS, functional near-infrared spectroscopy.
Outcome Measures
Outcome measures will be collected immediately before and immediately after each experimental condition.
Cerebral Blood Flow
The primary outcome measure will be the changes in oxygenated hemoglobin (oxy-Hb) using a non-invasive multi-channel continuous-wave fNIRS instrument (portable NIRSport, 8–8 system, with short-separation channels, NIRx Medizintechnik GmbH, Berlin, Germany). The optodes will be positioned over the prefrontal cortex, with data sampled at 7.81 Hz at wavelengths 750 nm and 820 nm. The fNIRS cap has 16 channels created by eight LED light sources and 8 detectors placed according to the standard 10–20 system, with a source-detector separation distance of 3 cm. Additional short-separation optodes with a distance of 0.8 cm (NIRx Medizintechnik GmbH, Berlin, Germany) to account for superficial blood flow (Yücel et al., 2015) will also be used. We chose to measure cerebral blood flow in the prefrontal cortex region as working memory tasks (such as the n-back) predominately activate this region (Smith and Jonides, 1997; Owen et al., 2005). System calibration will be performed before each assessment using NIRStar 15.2 software and using the predefined montage, and the fNIRS signals will be visually quality checked during data collection for motion artifacts. Cerebral blood flow measures will be taken simultaneously while the participant performs three cognitive tasks of increasing workload (numerical 1-, 2-, and 3-back tests). Our primary outcome variables will be changes in oxy-Hb between the 1-back and 2-back and between the 1-back and 3-back tests (expressed in ΔμM). We will also record deoxygenated (deoxy-Hb) concentrations. Further details about system calibration and data processing are included in Supplementary Material.
Cognitive Performance
Cognitive performance in working memory will be assessed using a computerized n-back test (Kirchner, 1958), consisting of the 1-, 2-, and 3-back tests administered simultaneously with fNIRS measures. We will use a computerized, numerical version of the n-back, whereby participants will be required to indicate (via a keypress within 2 s from stimulus onset) whether the digit presented on the screen was the same as the digit presented 1 stimulus previously (1-back), 2 stimuli previously (2-back), or 3 stimuli previously (3-back). Each digit will be presented for 1.5 s at an interstimulus interval of 500 ms. The n-back tests were created using E-Prime 2.0 (Psychology Software Tools). To reduce practice effects, participants will perform practice tests before data collection on each experimental day. The outcome variables for cognitive performance will include average reaction time (ms) and accuracy (average number of correct responses) for each test across two blocks of 20 digit sequences (1-back) or four blocks of 20 digit sequences (2- and 3-back).
Arterial Stiffness
Arterial stiffness will be measured using SphygmoCor Technology (Butlin and Qasem, 2017). The technology is a non-invasive, reproducible, and accurate method that uses waveform shape parameters from the radial artery to acquire central aortic blood pressure waveform. The aortic pressure waveform will be derived from the radial waveform by a validated transfer function (Pauca et al., 2001). Briefly, after 5 min of supine rest (only at pre-test), high fidelity pressure waveforms will be recorded (three times). The measurements will be ended when all quality parameters (pulse height variation, pulse length variation, diastolic variation, and shape deviation) are fulfilled in the SphygmoCor equipment (SphygmoCor, AtcorMedical, Sydney, Australia; Nelson et al., 2010). The variable for arterial stiffness will be the average of the augmentation index (AIx) across the three measures.
Other Measures
Stress will be measured using salivary cortisol concentrations. Saliva samples (collected immediately on waking on testing days and immediately pre- and post-testing) will be centrifuged and frozen at −80° Celsius and concentrations measured using the ELISA kit Abcam, ab154996. Mood will be assessed using the Positive and Negative Affect Scale (PANAS; Crawford and Henry, 2004). Alertness will be measured using a simple visual analog scale (VAS; Monk, 1989). Sleepiness will be measured using the Karolinska Sleepiness Questionnaire (Putilov and Donskaya, 2013). Blood glucose will be collected continuously using an Abbott FreeStyle Libre system (Abbott Scandinavia AB, Abbott Diabetes Care, Solna), which records glucose concentration in the interstitial fluid every minute.
Sample Size
Several studies (Hyodo et al., 2012; Endo et al., 2013; Byun et al., 2014; Bediz et al., 2016; Carter et al., 2018; Kujach et al., 2018; Ichinose et al., 2020) on the acute effects of light, moderate, and intense physical activity on post-exercise cerebral blood flow have been performed on similar, but not identical, designs and interventions as in our study, but have used comparable populations. Data from these studies were used to recalculate effect sizes using G*Power software (Franz Faul, Universität Kiel, Germany, v 3.1.9.2). Results showed effect sizes between 0.9 and 2.4, rendering a minimum sample size of between 6 and 13 individuals with an α = 0.05 and β = 0.8 assuming a two-tailed test. We chose to match our sample size to the largest found among the reviewed studies, namely 13 subjects.
Data Analysis
To answer our primary research question, linear mixed-effects models with subject as the random effect will be used to assess within condition differences in the changes in cerebral blood flow (oxy-Hb) between pre- and post-tests correcting for multiple comparisons from estimation over many channels, using Benjamini–Hochberg. Time and condition interactions will be used to estimate differences between conditions. Baseline arterial stiffness will be assessed as a moderator in the models. Linear mixed models will also be used to assess secondary outcomes, to investigate within-person changes from pre- to post-test, and between conditions. Stata version 15 (StataCorp, College Station, TX, USA) and NIRSToolboxAnalyzer (Santosa et al., 2018) will be used for statistical analyses.
Discussion
Short, frequent bouts of physical activity may be a promising strategy to increase cerebral blood flow to counteract the negative effects of extended periods of sitting. This crossover randomized trial will determine the effects of frequent, short bouts of physical activity on cerebral blood flow and cognitive performance, as well as psychological factors, and blood glucose levels. This topic is important considering that people are spending increasingly longer periods sedentary each day, particularly in their workplaces (Parry and Straker, 2013), which could potentially lead to long-term unfavorable effects on cognitive function (Voss et al., 2014; Wheeler et al., 2017).
This trial has many strengths. The randomized crossover design reduces contamination and the trial is powered to detect meaningful differences in cerebral blood flow. Importantly, our fNIRS protocol includes short-separation channels to remove superficial blood flow from measures of cerebral blood flow. The use of device-based measures of physical activity, sedentary behavior, and sleep and the use of a VO2max fitness test to personalize the physical activity walking bout are further strengths. The 3-h duration of uninterrupted sitting was chosen as it has high ecological validity; in a cross-sectional study of 334 adults of mean age 42 years, participants rarely sat for longer than 3 h at a time (Bojsen-Moller et al., 2019).
The two exercise paradigms we selected are readily applicable to the workplace setting. If we find immediate effects of physical activity on cerebral blood flow, the next steps would be to develop and test workplace interventions to examine the long-term effects of these paradigms and determine the clinical significance of cerebral blood flow changes on other factors such as productivity, concentration, and cognitive decline.
Finally, this protocol article will contribute to increasing scientific rigor in understanding how immediate, frequent, short activity bouts can affect cerebral blood flow since only one of the previous trials with similar aims has been registered or has published their protocols (Wennberg et al., 2016). Future studies should consider registering and publishing protocols to reduce negative findings not being published and to avoid potential bias of future reviews.
Author Contributions
ME and ÖE are project leaders. EH, ÖE, OT, MF, and ME were involved in the discussions in designing the protocol and planning the study. EH and CE drafted the manuscript. All authors contributed to reviewing, revising, and approving the final manuscript.
Funding
This project was supported by the Knowledge Foundation (20160040), the Swedish Armed Forces (AF9220915), ICA-Gruppen, Intrum, SATS Elixia, Monark Exercise, and Itrim Sweden. Professor English was supported by a National Heart Foundation Future Leaders Fellowship (101177).
Conflict of Interest
The authors declare that the research was conducted in the absence of any commercial or financial relationships that could be construed as a potential conflict of interest.
Supplementary Material
The Supplementary Material for this article can be found online at: https://www.frontiersin.org/articles/10.3389/fnhum.2020.00273/full#supplementary-material.
References
Aguilar-Farias, N., Brown, W. J., and Peeters, G. M. (2014). ActiGraph GT3X+ cut-points for identifying sedentary behaviour in older adults in free-living environments. J. Sci. Med. Sport 17, 293–299. doi: 10.1016/j.jsams.2013.07.002
Angevaren, M., Aufdemkampe, G., Verhaar, H. J. J., Aleman, A., and Vanhees, L. (2008). Physical activity and enhanced fitness to improve cognitive function in older people without known cognitive impairment. Cochrane Database Syst. Rev. 3:CD005381. doi: 10.1002/14651858.cd005381.pub3
Bediz, C. S., Oniz, A., Guducu, C., Ural Demirci, E., Ogut, H., Gunay, E., et al. (2016). Acute supramaximal exercise increases the brain oxygenation in relation to cognitive workload. Front. Hum. Neurosci. 10:174. doi: 10.3389/fnhum.2016.00174
Bell, L., Lamport, D. J., Field, D. T., Butler, L. T., and Williams, C. M. (2018). Practice effects in nutrition intervention studies with repeated cognitive testing. Nutr. Healthy Aging 4, 309–322. doi: 10.3233/nha-170038
Bertsch, K., Hagemann, D., Hermes, M., Walter, C., Khan, R., and Naumann, E. (2009). Resting cerebral blood flow, attention, and aging. Brain Res. 1267, 77–88. doi: 10.1016/j.brainres.2009.02.053
Biswas, A., Oh, P. I., Faulkner, G. E., Bajaj, R. R., Silver, M. A., Mitchell, M. S., et al. (2015). Sedentary time and its association with risk for disease incidence, mortality and hospitalization in adults: a systematic review and meta-analysis. Ann. Intern. Med. 162, 123–132. doi: 10.7326/m14-1651
Bojsen-Moller, E., Boraxbekk, C. J., Ekblom, O., Blom, V., and Ekblom, M. M. (2019). Relationships between physical activity, sedentary behaviour and cognitive functions in office workers. Int. J. Environ. Res. Public Health 16:4721. doi: 10.3390/ijerph16234721
Butlin, M., and Qasem, A. (2017). Large artery stiffness assessment using sphygmocor technology. Pulse 4, 180–192. doi: 10.1159/000452448
Byun, K., Hyodo, K., Suwabe, K., Ochi, G., Sakairi, Y., Kato, M., et al. (2014). Positive effect of acute mild exercise on executive function via arousal-related prefrontal activations: an fNIRS study. NeuroImage 98, 336–345. doi: 10.1016/j.neuroimage.2014.04.067
Carter, S. E., Draijer, R., Holder, S. M., Brown, L., Thijssen, D. H. J., and Hopkins, N. D. (2018). Regular walking breaks prevent the decline in cerebral blood flow associated with prolonged sitting. J. Appl. Physiol. 125, 790–798. doi: 10.1152/japplphysiol.00310.2018
Carter, S. E., Draijer, R., Holder, S. M., Brown, L., Thijssen, D. H. J., and Hopkins, N. D. (2019). Effect of different walking break strategies on superficial femoral artery endothelial function. Physiol. Rep. 7:e14190. doi: 10.14814/phy2.14190
Cellini, N., Buman, M. P., McDevitt, E. A., Ricker, A. A., and Mednick, S. C. (2013). Direct comparison of two actigraphy devices with polysomnographically recorded naps in healthy young adults. Chronobiol. Int. 30, 691–698. doi: 10.3109/07420528.2013.782312
Chang, Y. K., Labban, J. D., Gapin, J. I., and Etnier, J. L. (2012). The effects of acute exercise on cognitive performance: a meta-analysis. Brain Res. 1453, 87–101. doi: 10.1016/j.brainres.2012.02.068
Cole, R. J., Kripke, D. F., Gruen, W., Mullaney, D. J., and Gillin, J. C. (1992). Automatic sleep/wake identification from wrist activity. Sleep 15, 461–469. doi: 10.1093/sleep/15.5.461
Crawford, J. R., and Henry, J. D. (2004). The positive and negative affect schedule (PANAS): construct validity, measurement properties and normative data in a large non-clinical sample. Br. J. Clin. Psychol. 43, 245–265. doi: 10.1348/0144665031752934
Dempsey, P. C., Blankenship, J. M., Larsen, R. N., Sacre, J. W., Sethi, P., Straznicky, N. E., et al. (2017). Interrupting prolonged sitting in type 2 diabetes: nocturnal persistence of improved glycaemic control. Diabetologia 60, 499–507. doi: 10.1007/s00125-016-4169-z
Dunstan, D. W., Kingwell, B. A., Larsen, R., Healy, G. N., Cerin, E., Hamilton, M. T., et al. (2012). Breaking up prolonged sitting reduces postprandial glucose and insulin responses. Diabetes Care 35, 976–983. doi: 10.2337/dc11-1931
Dunstan, D. W., Wheeler, M. J., Ellis, K. A., Cerin, E., and Green, D. J. (2018). Interacting effects of exercise with breaks in sitting time on cognitive and metabolic function in older adults: rationale and design of a randomised crossover trial. Ment. Health Phys. Act. 15, 11–16. doi: 10.1016/j.mhpa.2018.05.003
Ekelund, U., Tarp, J., Steene-Johannessen, J., Hansen, B. H., Jefferis, B., Fagerland, M. W., et al. (2019). Dose-response associations between accelerometry measured physical activity and sedentary time and all cause mortality: systematic review and harmonised meta-analysis. BMJ 366:l4570. doi: 10.1136/bmj.l4570
Elias, M. F., Robbins, M. A., Budge, M. M., Abhayaratna, W. P., Dore, G. A., and Elias, P. K. (2009). Arterial pulse wave velocity and cognition with advancing age. Hypertension 53, 668–673. doi: 10.1161/hypertensionaha.108.126342
Endo, K., Matsukawa, K., Liang, N., Nakatsuka, C., Tsuchimochi, H., Okamura, H., et al. (2013). Dynamic exercise improves cognitive function in association with increased prefrontal oxygenation. J. Physiol. Sci. 63, 287–298. doi: 10.1007/s12576-013-0267-6
English, C., Weerasekara, I., Carlos, A., Chastin, S., Crowfoot, G., Fitzsimons, C., et al. (2020). Investigating the rigour of research findings in experimental studies assessing the effects of breaking up prolonged sitting—extended scoping review. Braz. J. Phys. Ther. doi: 10.1016/j.bjpt.2020.04.007 [Epub ahead of print].
Gomez-Pinilla, F., and Hillman, C. (2013). The influence of exercise on cognitive abilities. Comp. Physiol. 3, 403–428. doi: 10.1002/cphy.c110063
Herold, F., Wiegel, P., Scholkmann, F., and Müller, N. G. (2018). Applications of functional near-infrared spectroscopy (fNIRS) neuroimaging in exercise-cognition science: a systematic, methodology-focused review. J. Clin. Med. 7:466. doi: 10.3390/jcm7120466
Hillman, C. H., Erickson, K. I., and Kramer, A. F. (2008). Be smart, exercise your heart: exercise effects on brain and cognition. Nat. Rev. Neurosci. 9, 58–65. doi: 10.1038/nrn2298
Huppert, T. J., Hoge, R. D., Diamond, S. G., Franceschini, M. A., and Boas, D. A. (2006). A temporal comparison of BOLD, ASL, and NIRS hemodynamic responses to motor stimuli in adult humans. NeuroImage 29, 368–382. doi: 10.1016/j.neuroimage.2005.08.065
Hyodo, K., Dan, I., Suwabe, K., Kyutoku, Y., Yamada, Y., Akahori, M., et al. (2012). Acute moderate exercise enhances compensatory brain activation in older adults. Neurobiol. Aging 33, 2621–2632. doi: 10.1016/j.neurobiolaging.2011.12.022
Ichinose, Y., Morishita, S., Suzuki, R., Endo, G., and Tsubaki, A. (2020). Comparison of the effects of continuous and intermittent exercise on cerebral oxygenation and cognitive function. Adv. Exp. Med. Biol. 1232, 209–214. doi: 10.1007/978-3-030-34461-0_26
Ji, Z., Feng, T., Mei, L., Li, A., and Zhang, C. (2019). Influence of acute combined physical and cognitive exercise on cognitive function: an NIRS study. PeerJ 7:e7418. doi: 10.7717/peerj.7418
Kirchner, W. K. (1958). Age differences in short-term retention of rapidly changing information. J. Exp. Psychol. 55, 352–358. doi: 10.1037/h0043688
Kujach, S., Byun, K., Hyodo, K., Suwabe, K., Fukuie, T., Laskowski, R., et al. (2018). A transferable high-intensity intermittent exercise improves executive performance in association with dorsolateral prefrontal activation in young adults. NeuroImage 169, 117–125. doi: 10.1016/j.neuroimage.2017.12.003
Monk, T. H. (1989). A visual analogue scale technique to measure global vigor and affect. Psychiatry Res. 27, 89–99. doi: 10.1016/0165-1781(89)90013-9
Nelson, M. R., Stepanek, J., Cevette, M., Covalciuc, M., Hurst, R. T., and Tajik, A. J. (2010). Noninvasive measurement of central vascular pressures with arterial tonometry: clinical revival of the pulse pressure waveform? Mayo Clin. Proc. 85, 460–472. doi: 10.4065/mcp.2009.0336
Ogoh, S., and Ainslie, P. N. (2009). Cerebral blood flow during exercise: mechanisms of regulation. J. Appl. Physiol. 107, 1370–1380. doi: 10.1152/japplphysiol.00573.2009
Owen, A. M., McMillan, K. M., Laird, A. R., and Bullmore, E. (2005). N-back working memory paradigm: a meta-analysis of normative functional neuroimaging studies. Hum. Brain Mapp. 25, 46–59. doi: 10.1002/hbm.20131
Parry, S., and Straker, L. (2013). The contribution of office work to sedentary behaviour associated risk. BMC Public Health 13:296. doi: 10.1186/1471-2458-13-296
Patterson, R., McNamara, E., Tainio, M., de Sá, T. H., Smith, A. D., Sharp, S. J., et al. (2018). Sedentary behaviour and risk of all-cause, cardiovascular and cancer mortality and incident type 2 diabetes: a systematic review and dose response meta-analysis. Eur. J. Epidemiol. 33, 811–829. doi: 10.1007/s10654-018-0380-1
Pauca, A. L., O’Rourke, M. F., and Kon, N. D. (2001). Prospective evaluation of a method for estimating ascending aortic pressure from the radial artery pressure waveform. Hypertension 38, 932–937. doi: 10.1161/hy1001.096106
Putilov, A. A., and Donskaya, O. G. (2013). Construction and validation of the EEG analogues of the Karolinska sleepiness scale based on the Karolinska drowsiness test. Clin. Neurophysiol. 124, 1346–1352. doi: 10.1016/j.clinph.2013.01.018
Santosa, H., Zhai, X., Fishburn, F., and Huppert, T. (2018). The NIRS brain AnalyzIR toolbox. Algorithms 11:73. doi: 10.3390/a11050073
Sasaki, J. E., John, D., and Freedson, P. S. (2011). Validation and comparison of ActiGraph activity monitors. J. Sci. Med. Sport 14, 411–416. doi: 10.1016/j.jsams.2011.04.003
Smith, E. E., and Jonides, J. (1997). Working memory: a view from neuroimaging. Cogn. Psychol. 33, 5–42. doi: 10.1006/cogp.1997.0658
Stoner, L., Willey, Q., Evans, W. S., Burnet, K., Credeur, D. P., Fryer, S., et al. (2019). Effects of acute prolonged sitting on cerebral perfusion and executive function in young adults: a randomized cross-over trial. Psychophysiology 56:e13457. doi: 10.1111/psyp.13457
Tarumi, T., Gonzales, M. M., Fallow, B., Nualnim, N., Pyron, M., Tanaka, H., et al. (2013). Central artery stiffness, neuropsychological function, and cerebral perfusion in sedentary and endurance-trained middle-aged adults. J. Hypertens. 31, 2400–2409. doi: 10.1097/hjh.0b013e328364decc
Voss, M. W., Carr, L. J., Clark, R., and Weng, T. (2014). Revenge of the “sit” II: does lifestyle impact neuronal and cognitive health through distinct mechanisms associated with sedentary behavior and physical activity? Ment. Health Phys. Act. 7, 9–24. doi: 10.1016/j.mhpa.2014.01.001
Wennberg, P., Boraxbekk, C. J., Wheeler, M., Howard, B., Dempsey, P. C., Lambert, G., et al. (2016). Acute effects of breaking up prolonged sitting on fatigue and cognition: a pilot study. BMJ Open 6:e009630. doi: 10.1136/bmjopen-2015-009630
Wheeler, M. J., Dempsey, P. C., Grace, M. S., Ellis, K. A., Gardiner, P. A., Green, D. J., et al. (2017). Sedentary behavior as a risk factor for cognitive decline? A focus on the influence of glycemic control in brain health. Alzheimers Dement. 3, 291–300. doi: 10.1016/j.trci.2017.04.001
Wheeler, M. J., Dunstan, D. W., Smith, B., Smith, K. J., Scheer, A., Lewis, J., et al. (2019). Morning exercise mitigates the impact of prolonged sitting on cerebral blood flow in older adults. J. Appl. Physiol. 126, 1049–1055. doi: 10.1152/japplphysiol.00001.2019
Wheeler, M. J., Green, D. J., Ellis, K. A., Cerin, E., Heinonen, I., Naylor, L. H., et al. (2020). Distinct effects of acute exercise and breaks in sitting on working memory and executive function in older adults: a three-arm, randomised cross-over trial to evaluate the effects of exercise with and without breaks in sitting on cognition. Br. J. Sports Med. 54, 776–781. doi: 10.1136/bjsports-2018-100168
Yücel, M. A., Selb, J., Aasted, C. M., Petkov, M. P., Becerra, L., Borsook, D., et al. (2015). Short separation regression improves statistical significance and better localizes the hemodynamic response obtained by near-infrared spectroscopy for tasks with differing autonomic responses. Neurophotonics 2:035005. doi: 10.1117/1.nph.2.3.035005
Keywords: physical activity breaks, sedentary, cerebral blood flow, cognitive function, fNIRS
Citation: Heiland EG, Ekblom Ö, Tarassova O, Fernström M, English C and Ekblom MM (2020) ABBaH: Activity Breaks for Brain Health. A Protocol for a Randomized Crossover Trial. Front. Hum. Neurosci. 14:273. doi: 10.3389/fnhum.2020.00273
Received: 16 March 2020; Accepted: 17 June 2020;
Published: 14 July 2020.
Edited by:
Marcel Olde Rikkert, Radboud University Nijmegen Medical Centre, NetherlandsReviewed by:
Kirsten Dillon, University of Western Ontario, CanadaPaul Gardiner, The University of Queensland, Australia
Carlijn Maasakkers, Radboud University Nijmegen Medical Centre, Netherlands, in collaboration with reviewer PG
Copyright © 2020 Heiland, Ekblom, Tarassova, Fernström, English and Ekblom. This is an open-access article distributed under the terms of the Creative Commons Attribution License (CC BY). The use, distribution or reproduction in other forums is permitted, provided the original author(s) and the copyright owner(s) are credited and that the original publication in this journal is cited, in accordance with accepted academic practice. No use, distribution or reproduction is permitted which does not comply with these terms.
*Correspondence: Emerald G. Heiland, ZW1lcmFsZC5oZWlsYW5kQGdpaC5zZQ==