- 1Department of Neurology, Faculty of Medicine, Albert Szent-Györgyi Health Center, University of Szeged, Szeged, Hungary
- 2MTA-SZTE Neuroscience Research Group, Szeged, Hungary
- 3Interdisciplinary Centre of Excellence, University of Szeged, Szeged, Hungary
- 4Faculty of Arts, Institute of Psychology, University of Szeged, Szeged, Hungary
Background: Transcranial magnetic stimulation (TMS) and transcranial direct current stimulation (tDCS) have been proposed as a new therapeutic way to enhance the cognition of patients with dementia. However, serious methodological limitations appear to affect the estimates of their efficacy. We reviewed the stimulation parameters and methods of studies that used TMS or tDCS to alleviate the cognitive symptoms of patients with Alzheimer's disease (AD) and mild cognitive impairment (MCI). Moreover, we evaluated the risk of bias in these studies. Our aim was to highlight the current vulnerabilities of the field and to formulate recommendations on how to manage these issues when designing studies.
Methods: Electronic databases and citation searching were used to identify studies administering TMS or tDCS on patients with AD or MCI to enhance cognitive function. Data were extracted by one review author into summary tables with the supervision of the authors. The risk of bias analysis of randomized-controlled trials was conducted by two independent assessors with version 2 of the Cochrane risk-of-bias tool for randomized trials.
Results: Overall, 36 trials were identified of which 23 randomized-controlled trials underwent a risk of bias assessment. More than 75% of randomized-controlled trials involved some levels of bias in at least one domain. Stimulation parameters were highly variable with some ranges of effectiveness emerging. Studies with low risk of bias indicated TMS to be potentially effective for patients with AD or MCI while questioned the efficacy of tDCS.
Conclusions: The presence and extent of methodical issues affecting TMS and tDCS research involving patients with AD and MCI were examined for the first time. The risk of bias frequently affected the domains of the randomization process and selection of the reported data while missing outcome was rare. Unclear reporting was present involving randomization, allocation concealment, and blinding. Methodological awareness can potentially reduce the high variability of the estimates regarding the effectiveness of TMS and tDCS. Studies with low risk of bias delineate a range within TMS parameters seem to be effective but question the efficacy of tDCS.
Introduction
Non-invasive brain stimulation (NIBS) has been tested to modify the cognition of healthy participants, as well as to mitigate cognitive symptoms in neurodegenerative disorders (Guse et al., 2010; Vacas et al., 2019). The two most common forms of NIBS, namely transcranial magnetic stimulation (TMS) and transcranial direct current stimulation (tDCS) have both been characterized by a great variability of application and diverse stimulation parameters. Accordingly, the results of NIBS studies are characterized by a large amount of inter- and intra-individual variability. This issue has led to the point that some reviews and meta-analyses have even questioned the efficacy of certain NIBS methods, especially tDCS, in modulating the cognitive performance of either healthy or demented participants (Jacobson et al., 2012; Horvath et al., 2015). Although accumulating evidence supports the efficacy of TMS in modulating cognition, not only the determination of the effectiveness, but also the estimation of the effect size is crucial which likewise needs to be based on reliable data. Reviews indicating positive cognitive effects of NIBS in neurodegenerative disorders have reported serious limitations of the analyzed studies (Freitas et al., 2011; Elder and Taylor, 2014; Hsu et al., 2015; Vacas et al., 2019). The limitations included high heterogeneity among the applied measurements and stimulation parameters, increased variability due to specific characteristics among demented samples, and low statistical power resulting from small sample sizes. All these factors might contribute to the high variability and hinder the accurate estimation of NIBS efficacy; however, the extent to which each of these factors is present has not been systematically reviewed. Moreover, the reporting of methods is often suboptimal regarding several important design aspects of clinical trials (e.g., allocation concealment, randomization, statistical analyses, and sample characteristics) (Gluud, 2006). Inadequate reporting, as well as the selection of trial design and applied methods, may affect the estimates of NIBS effects (Savović et al., 2012; Weuve et al., 2015; Polanía et al., 2018) with a more definite influence on subjectively assessed outcomes, such as cognitive status (Savović et al., 2012). Differences in stimulation parameters may result in the altered efficacy of stimulation. Moreover, some settings of stimulation parameters are designed to achieve different goals e.g., more focal stimulation or the modulation of subcortical structures. Consequently, clear and detailed reporting of NIBS protocols is crucial to allow the consideration of these differences (Polanía et al., 2018). An overview of the recommended methodological characteristics and stimulation parameters pointing toward fully developed methodology guidelines and consensus regarding the elements of NIBS is needed (Weuve et al., 2015; Polanía et al., 2018).
The current review aims to examine the presence and extent of methodological issues confounding NIBS studies attempting to alleviate the cognitive symptoms of demented patients. The term cognition covers multiple domains (e.g., attention, memory, language, decision-making, etc.), and each domain can be assessed by numerous types of measurement. However, pooling disparate measures that assess different constructs (i.e., different cognitive subdomains) is generally not recommended, especially in the presence of high heterogeneity of the intervention (Greenfield et al., 2007). By extracting the design characteristics and stimulation parameters of previous studies, we aim to highlight the current vulnerabilities of the field and to formulate recommendations on how to manage these issues when designing studies. We focused on original research articles that applied repetitive transcranial magnetic stimulation (rTMS) or tDCS, i.e., the two most frequent NIBS techniques. We included studies involving patients with mild cognitive impairment (MCI) and Alzheimer's disease (AD). AD is the most frequent form of dementia that accounts for 50–70% of all dementia cases (Hugo and Ganguli, 2014). Patients with MCI are in an intermediate cognitive state, with a remarkably increased risk of conversion to dementia compared to healthy elderly (Petersen et al., 1999). The treatment of cognitive symptoms in AD and MCI has become an area of major interest considering our aging population, which increased the need for testing alternative therapeutic solutions, such as NIBS. We argue that methodological awareness and effort to increase the experimental control over some sources of variability and bias would contribute to more accurate estimations of the real effects of NIBS on cognition in dementia.
Methods
Literature Search Strategy
Based on a recent analysis, literature search in PubMed/MEDLINE in combination with Web of Science leads to the recall of almost 80% of the relevant literature in at least 80% of the reviews (Bramer et al., 2017). To further improve this recall ratio, we searched for relevant articles also in ScienceDirect. Therefore, the literature search of three databases was conducted involving PubMed/MEDLINE, Web of Science, and ScienceDirect. Furthermore, bibliographies of the retrieved articles and the relevant reviews were hand-searched as well. The literature search was carried out by A.H., the result of which was confirmed by the co-authors. No review protocol or registration details are available.
The keywords were determined according to the PICO (population, intervention, comparison, outcome) framework (Schardt et al., 2007) and were searched in the full text of the articles to increase the recall of relevant publications (Kostoff, 2010). The following keywords were applied: Alzheimer* disease OR Alzheimer* dementia when searching for papers involving AD patients. Mild cognitive impairment was used to identify MCI research. For the intervention methods, the MESH terms, transcranial magnetic stimulation OR transcranial direct current stimulation were used. Finally, the following keywords were applied to define outcomes: cognition OR executive function* OR memory OR language OR attention. These elements were appended using AND operators (Table 1).
Eligibility Criteria
We aimed to identify original research articles examining the effects of two NIBS techniques (either TMS or tDCS) on any measures of cognitive function in AD or MCI patients. Correspondingly, the following inclusion criteria were determined prior to the literature search: (1) original research articles; (2) written in English; (3) involving human subjects diagnosed with AD or MCI; (4) using TMS or tDCS as an intervention to enhance cognition and; (5) applying any measures of cognitive function. We included clinical trials from the start dates of the databases published until 31 December 2018. As MCI can originate from a wide range of etiological backgrounds, we decided only to include studies that examined MCI with no specified subgroups or MCI due to AD. We decided not to exclude the articles that combined NIBS with other interventions such as cognitive training or ongoing medication, even without the presence of a NIBS-only condition. We argue that the inclusion of studies with combined therapies does not hinder the evaluation of the articles from a methodological point of view. No criteria regarding the design of the studies were determined. We excluded articles for (1) not reporting empirical research; (2) not being written in English; (3) involving animal models of dementia and; (4) not applying NIBS as an intervention aiming to enhance cognition. Conference abstracts and supplementary reports that were not peer-reviewed were excluded due to their nature of limited methodological reporting.
Risk of Bias Assessment
As randomized-controlled trials (RCTs) are reported to be particularly common in the field of NIBS (Lange et al., 2017), we decided in advance to perform risk of bias assessment of the identified RCTs. To assess the risk of bias in parallel-group and crossover design RCTs, we administered Version 2 of the Cochrane risk-of-bias tool for randomized trials (RoB 2) recommended by the Cochrane Collaboration (Higgins et al., 2019; Sterne et al., 2019). This tool involves more domains than other widely used scales, thus more effectively evaluating the trials' internal validity (Hartling et al., 2009). The five domains of RoB 2 are (1) randomization process (selection bias), (2) deviations from intended interventions (performance bias), (3) missing outcome data (attrition bias), (4) measurement of the outcome (detection bias), and (5) selection of the reported result (reporting bias). All domains were evaluated separately and ranked as presenting a low risk of bias, some concerns, or high risk of bias. Three levels regarding the overall risk of bias were possible: “Low,” containing no concerns on any of the examined domains; “Some Concerns” involving some concerns in at least one but less than three domains, and “High” if any of the domains involved a high risk of bias or more than three domains contained some concerns. The evaluation of the studies was conducted by two authors (AH and VLN). Any discrepancy was solved by discussion and the consensus results are presented.
Data Extraction
Single data extraction has been found comparable with the results of two independent data extractors in the direction, magnitude, and precision of estimates for a great number of outcomes (Buscemi et al., 2006); therefore, AH was responsible for the data extraction. Data were extracted from each eligible article regarding (1) the main characteristics of the study design and the sample; (2) information regarding the NIBS stimulation (Table 2) and; (3) steps to prevent bias (Table 3).
Study Characteristics, Methods, and Outcomes
We extracted information on the study design including the intervention model and relevant study methods. The sample size and the mean age were collected to describe the sample characteristics. The use of the Mini-Mental State Examination (MMSE) as a screening test was found to be a common practice, thus we report its mean score indicating the severity of the cognitive symptoms in the examined samples. Regarding the outcomes, we examined the targeted cognitive domains and the specific tests that were used to measure the given function. The concluded results of the studies were also collected. We examined the most important methodological characteristics of the identified studies most of which were also evaluated during the risk of bias assessment. We also extracted additional data from the retrieved studies, such as the applied diagnostic criteria for AD/MCI, as well as the time points of the applied cognitive assessment and other aspects affecting the effect estimates (e.g., the use of sample size estimation). In the case of repeated testing, the management of possible practice effects was also examined (Table 3).
Stimulation Parameters
We extracted the type of the applied NIBS method (rTMS or tDCS). We also collected the type of stimulation (HF or LF-TMS; anodal or cathodal tDCS or a combination of NIBS with cognitive training). For tDCS studies, intensity and duration of the stimulation, target region, and the location of the coil/electrode and the positioning method. For rTMS studies, the same data were extracted in addition to the frequency of the stimulation and the type of the coil. In sham-controlled studies, the method of sham was also identified.
Results
Search Results
After removing the duplicates, we identified 962 articles that underwent a thorough screening procedure (Figure 1). After the screening of the titles, 651 records (68%) were excluded due to not meeting the inclusion criteria. The remaining 311 records' abstracts were screened yielding 42 studies (13%) eligible for full-text search. At this stage, 4 studies were excluded as they involved mixed samples of dementia patients with AD or MCI patients not being evaluated as a separate group. Additionally, in 2 studies only cognitive screening was administered instead of measuring intervention-related changes, and one study used NIBS as a diagnostic method rather than as a tool to enhance cognition. The manual search of the included studies' and relevant reviews' references did not yield any additional results. Overall, we found 36 eligible articles that were included in the qualitative analysis.
From the retrieved 36 studies, most involved participants with AD (28 trials; 76%), while 8 involved MCI patients (24%). Overall, 498 and 138 participants were recruited, respectively. Out of the AD studies, 13 applied TMS (46%), 5 combined TMS and cognitive training (TMS-Cog) (18%), 9 used tDCS (32%), and one performed a combination of tDCS and cognitive training (4%) to investigate the effects of NIBS on a wide range of cognitive functions. Of the MCI studies, 4 applied TMS (50%), 3 administered tDCS (38%), and one supplemented tDCS with cognitive training (12%). Furthermore, 3 research proposals were identified but will be detailed in the discussion only.
Trial Designs
Twenty-three of the retrieved studies (64%) had an RCT design, while 8 studies were non-RCTs (22%) and 5 were case studies (14%). Out of the RCTs, 11 had a parallel-group (48%) and 12 involved a crossover design (52%). Out of all studies, 3 had a prospective design, i.e., previously recruited data was analyzed.
Risk of Bias and Research Results
The risk of bias was typically present in at least one domain in 18 of the 23 RCTs (78%). In 9 studies, some concerns arose but less than 3 domains were affected, while 9 studies were ranked as having an overall high risk of bias since more than 3 domains were affected with bias. No study implied a high risk of bias in any domain (Figure 2). Interestingly, studies ranked as demonstrating a low risk of bias concluded promising clinical efficacy of TMS in both AD and MCI (Wu et al., 2015; Padala et al., 2018) in line with those studies with some risk of bias (for the overall assessment of studies see Figure 3). Mixed but mostly negative results have been found regarding the efficacy of tDCS in AD (Khedr et al., 2014; Suemoto et al., 2014; Bystad et al., 2016) while all other studies reported selective or overall improvement of cognition after tDCS.
Sample Characteristics
The diagnosis of AD patients was based on the NINCDS-ADRDA in 16 of the 28 AD studies (58%). DSM criteria were applied in 7 studies (27%) alone or in addition to other criteria. Three of the studies reported only that the diagnostic decision was made by an expert (Penolazzi et al., 2015; Rutherford et al., 2015; Avirame et al., 2016). To define MCI, various criteria were used, including the original criteria of Petersen et al. (1999) and its different revisions (Petersen et al., 1999; Portet et al., 2006; Albert et al., 2011). Overall, we identified 6 studies that did not specify how the diagnosis was established (18%) (Eliasova et al., 2014; Drumond Marra et al., 2015; Rutherford et al., 2015; Avirame et al., 2016; Rabey and Dobronevsky, 2016; Nguyen et al., 2017). Additional exclusion criteria were listed in almost every study. Partly, necessary restrictions were made inherent to the application of NIBS (e.g., no metals or stimulators in the body) but mostly aiming to obtain a more homogeneous sample. Patients with severe depression and other major neurological or psychiatric disorders were often excluded to limit the potential sources of the observed cognitive changes. Exclusions were also made based on the scores of cognitive screening tools to achieve the predefined severity profile of the sample (Bentwich et al., 2011). Strikingly, the determination of sample size was reasoned in only 3 studies (13%) (Suemoto et al., 2014; Bystad et al., 2016; Alcalá-Lozano et al., 2018), of which 2 conducted an a priori sample size calculation (Suemoto et al., 2014; Alcalá-Lozano et al., 2018). In contrast, 7 studies noted the sample size as a limitation to their findings (30%) (Ahmed et al., 2012; Devi et al., 2014; Eliasova et al., 2014; Murugaraja et al., 2017; Zhao et al., 2017; Koch et al., 2018; Padala et al., 2018).
Randomization, Allocation Concealment, and Blinding
The exact method of randomization was highly underreported with only 8 RCTs (34%) describing the process of random-sequence generation. Different methods were identified, such as computer- (Suemoto et al., 2014; Drumond Marra et al., 2015) or tDCS device-generated (Bystad et al., 2016) randomization, the use of a table of random numbers (Khedr et al., 2014; Wu et al., 2015) or randomized block design (Padala et al., 2018). One study allocated patients to groups in the order of assignment (Devi et al., 2014). Allocation concealment was reported in 7 studies, i.e., it was unclear in 70% of all RCTs. In 3 studies, opaque envelopes were used (Ahmed et al., 2012; Khedr et al., 2014; Suemoto et al., 2014). The DC stimulators' built-in function, used in one study, consists of a computer-generated list of 5-digit codes which meant to be decrypted only after the closure of the study, therefore, randomization and blinding are both realized (Bystad et al., 2016). In addition, 2 studies had an independent staff member to manage the allocation without informing the investigators and outcome assessors (Drumond Marra et al., 2015; Padala et al., 2018) and 2 studies stated that the allocation was concealed without specifying its method (Wu et al., 2015; Bystad et al., 2016). We identified 17 RCTs that were double-blind (74%) and 2 additional studies that were single-blind to the type of stimulation (8%). The latter usually refers to the blinding of the participants, while double-blinding means that both the participants and the outcome assessors are blinded. The blinding state of the person delivering the stimulation was mentioned in 17% of the RCTs.
Cognitive Measurement and Effects of Repeated Testing
For the evaluation of the general cognition of patients, the cognitive subsection of the Alzheimer Disease Assessment Scale (ADAS-Cog) and the MMSE was administered in 11 and 18 of all 28 studies (39 and 64%), respectively. The Addenbrooke's Cognitive Examination (ACE) was performed in two additional studies (7%). In MCI, it was less frequent to measure global cognition. Separate cognitive domains (language, verbal learning, attention, working memory, executive function, visuospatial skills, and psychomotor speed) were assessed by various neurocognitive tests (see Table 3). Since measurements were repeated at least once in every study, practice effects needed to be considered. In several cases, the alternate versions of the applied tests were performed to reduce practice effects. Two studies failed to explicitly mention whether alternate forms of ADAS-Cog have been used or not (Bentwich et al., 2011; Lee et al., 2016). Additionally, in one study double baseline was measured (Cotelli et al., 2012) and three studies measured the baseline weeks before the commencement of NIBS (Bentwich et al., 2011; Haffen et al., 2012; Andrade et al., 2016). Retesting usually occurred immediately after the last session of stimulation meaning that the interval between baseline and the first retest varied between 5 days and 5 months in the reviewed studies.
Statistical Analysis of Results
A predefined analysis protocol was available for 7 studies (30%) (Khedr et al., 2014; Suemoto et al., 2014; Drumond Marra et al., 2015; Meinzer et al., 2015; Wu et al., 2015; Bystad et al., 2016; Padala et al., 2018) and predefined cutoff scores indicating a meaningful change were uncommon. Moreover, whether the statistical analysis was conducted blindly or not remained unclear in 85% of all studies.
Stimulation Parameters
Stimulation Parameters of TMS Studies
Number of sessions
Of the identified TMS studies, 5 had a single-session paradigm (22%), and 18 contained multiple stimulation sessions of TMS (78%). Single-session studies often compared an active protocol with a sham condition in an online (Cotelli et al., 2006, 2008) or offline setting (Sole-Padulles et al., 2006; Turriziani et al., 2012; Eliasova et al., 2014). Online single-session rTMS was performed in two studies of Cotelli et al. (2006, 2008) to investigate its effect on object and action naming in AD, while Eliasova et al. (2014) examined the effect of offline TMS on a broader scale of cognitive tasks (Cotelli et al., 2006, 2008; Eliasova et al., 2014). Two TMS studies administered one session of either facilitatory or inhibitory TMS in MCI patients to modulate memory performance (Sole-Padulles et al., 2006; Turriziani et al., 2012). Multiple-session paradigms varied in length ranging from 5 to 30 sessions. TMS treatment lasted generally longer than tDCS with 20 to 30 sessions being the most common in AD and 10 sessions in MCI. The average length of multiple-session TMS and tDCS was 16 and 7.5 sessions, respectively. No studies administered more than 10 sessions of NIBS on MCI patients, while 8 administered more than 10 sessions of NIBS in AD.
Target Region, Localization Methods, and Type of the Coil
Eight different cortical areas were targeted with NIBS of which the DLPFC appeared to be the most favored region. DLPFC was stimulated either unilaterally or bilaterally in 21 out of 36 studies (58%). Based on the paradigm of Bentwich et al. (2011), several further studies involving AD participants stimulated six brain regions, including Broca's area, Wernicke's area, the left and right DLPFC (LDLPFC and RDLPFC), and the right and left parietal somatosensory association cortices (R-pSAC and L-pSAC) (Boggio et al., 2012; Rabey et al., 2013; Lee et al., 2016; Rabey and Dobronevsky, 2016; Nguyen et al., 2017; Alcalá-Lozano et al., 2018). Since the temporal cortex is one of the first areas affected in AD (Toepper, 2017), it was targeted by 5 studies (Boggio et al., 2009, 2012; Bystad et al., 2016, 2017; Zhao et al., 2017). The precuneus and the inferior frontal gyrus (IFG) were also stimulated (Eliasova et al., 2014; Meinzer et al., 2015; Koch et al., 2018). Regarding MCI, only two studies deviated from targeting the DLPFC, one of which aimed to stimulate the left IFG, while the other stimulated the left inferior parietal lobule, both sides being targeted in AD as well (Cotelli et al., 2012; Meinzer et al., 2015). TMS and tDCS studies did not differ significantly in the choice of stimulation sites.
Of all TMS studies, figure-of-eight coil was used the most (15 studies, 77%). One study used a double-cone coil (Sole-Padulles et al., 2006) and an H2 coil was equipped in another (Avirame et al., 2016). The shape or type of the coil was not mentioned in four studies (Rutherford et al., 2015; Lee et al., 2016; Zhao et al., 2017). Likewise, the manufacturer and the type of the TMS device was not specified in 4 studies (14%).
Neuronavigation was used in 10 TMS studies. All tDCS studies with reported electrode localization method and 3 additional TMS studies positioned the coil/electrodes based on the international 10–20 electroencephalography (EEG) system. In 6 TMS studies, the coil position was calculated based on the location of the motor cortex. When defining the DLPFC concerning the motor hotspot, the optimal localization of the motor cortex also varied across studies. One study named resting motor threshold as the reference, while others used the first dorsal interosseous, and two did not specify the exact procedure (Ahmed et al., 2012; Haffen et al., 2012; Devi et al., 2014; Avirame et al., 2016).
Frequency and Intensity of TMS
In the reviewed studies, TMS frequency was set at 1 Hz for LF stimulation (Ahmed et al., 2012; Turriziani et al., 2012), while HF-TMS ranged from 5 to 20 Hz. Ten and twenty hertz were the most applied for HF-stimulation which was performed in 11 and 10 studies, respectively. Five hertz stimulation was administered in 2 studies (Sole-Padulles et al., 2006; Alcalá-Lozano et al., 2018), while 15 Hz was used in 1 study (Devi et al., 2014). TMS intensity varied between 80 and 120% of the resting motor threshold (RMT). LF-TMS was performed at 90% of RMT in both studies. Suprathreshold stimulation (at 110 or 120% of RMT) was administered in two HF-TMS studies. Stimulation at the intensity of the motor threshold was performed in 7 studies. The remaining 8 studies applied subthreshold stimulation with the internsity of 80% or 90% of the RMT. Only one study applied a fixed intensity, setting it to 60% of the maximum stimulation output (Avirame et al., 2016). The number of pulses ranged from 600 to 3,000 pulses/session.
Sham Stimulation
Sham stimulation was administered in 24 studies. In TMS studies, sham coil or other instruments to increase the distance of the real TMS coil from the scalp were used in 6 cases (Cotelli et al., 2011; Rabey et al., 2013; Drumond Marra et al., 2015; Rutherford et al., 2015; Koch et al., 2018; Padala et al., 2018). Two studies placed the real coil over the targeted area but did not apply magnetic stimulation and prerecorded clicking sounds of the TMS device were played instead (Lee et al., 2016; Zhao et al., 2017). Changing the coil position such as elevating or tilting it was chosen in 4 studies (Sole-Padulles et al., 2006; Ahmed et al., 2012; Turriziani et al., 2012; Wu et al., 2015). Another possible method is the stimulation of an unrelated control site, e.g., the vertex, indeed applied by 3 studies as the sham procedure (Cotelli et al., 2006, 2008; Eliasova et al., 2014). Two of these studies performed vertex stimulation with the coil held perpendicularly, thus actually not administering active stimulation over the vertex (Cotelli et al., 2006, 2008).
Stimulation Parameters of tDCS Studies
Number of sessions
Of the identified tDCS studies, 4 had a single-session paradigm (31%), and 9 contained multiple stimulation sessions (69%). In single-session studies, an active tDCS protocol was often compared to a sham condition in an online (Boggio et al., 2009; Meinzer et al., 2015) or offline setting (Ferrucci et al., 2008). Single-session anodal, cathodal, and sham tDCS were also tested to examine their effects on a word recognition task (Ferrucci et al., 2008; Marceglia et al., 2016). Anodal tDCS (atDCS) over two cortical regions were compared to sham stimulation attempting to reduce the cognitive symptoms of AD patients (Ferrucci et al., 2008; Boggio et al., 2009, 2012). In addition, single-session atDCS was performed on MCI patients to examine its effects on a range of cognitive functions (Meinzer et al., 2015). One study compared single sessions of two active tDCS protocols (Marceglia et al., 2016).
The duration of tDCS appeared to be shorter than TMS treatment ranging from 1 to 10 sessions in the case of both patient populations. The average length of multiple-session tDCS 7.5 sessions. A notable exception was a case study in which atDCS was applied every day for 8 months (Bystad et al., 2017).
Target region and localization methods of tDCS studies
TMS and tDCS studies did not differ significantly in the choice of stimulation sites. For summarization of target regions and localization methods see the subsection Target Region, Localization Methods, and Type of the Coil. The manufacturer of the tDCS device was not recorded in more than half of the tDCS studies (6 of 14, 43%).
Frequency and intensity of tDCS
Intensity appears more unified in tDCS research than in TMS studies as it was set to 2 mA in 9 of 12 studies (75%), and to 1 mA in the remaining 3 studies (25%). The duration of one session ranged from 10 to 30 min with the longer stimulation periods being more frequent.
Sham stimulation
The most frequently used sham condition involves a short stimulation period (usually 30 s or less). Among the articles reviewed here, 6 mentioned the use of 30 s of stimulation (Boggio et al., 2009, 2012; Khedr et al., 2014; Meinzer et al., 2015; Bystad et al., 2016; Cruz Gonzalez et al., 2018), while 3 studies chose shorter intervals, 10 or 20 s as sham stimulation (Ferrucci et al., 2008; Suemoto et al., 2014; Penolazzi et al., 2015). None of the reviewed studies applied active tDCS over a control site.
Discussion
In the current paper, we proposed to systematically review the current methods, quality and stimulation parameters of research, which aims to enhance cognition in AD and MCI patients. We included data from 36 clinical trials. Several reviews and meta-analyses have lately concluded the positive effect of NIBS in neurodegenerative disorders (Freitas et al., 2011; Elder and Taylor, 2014; Hsu et al., 2015; Vacas et al., 2019); however, important limitations have been overlooked involving the methodology and the stimulation parameters. Our goal was to examine the extent to which these methodological issues are present in the field, and to provide objective recommendations on how to improve future research. The common major aim is to gain more reliable evidence on the effectiveness of NIBS to mitigate the cognitive symptoms in MCI or AD dementia.
Most studies seemed to support the cognitive enhancing effect of NIBS in dementia, regardless of the risk of bias ranking. Interestingly, examining those RCTs with a low risk of bias offered a more elaborate picture (for the summarization of the methods and stimulation parameters of these studies see Table 4). Three high-quality studies performing HF-TMS with a figure-of-eight coil over the LDLPFC supported the enhancing effect of TMS on cognition in AD and MCI. It is noteworthy that parameters such as the number of sessions, the intensity and the frequency of the stimulation differed across these studies to some extent. Suprathreshold stimulation on 10 Hz was administered to MCI patients, while the stimulation of AD patients was conducted at 80% of RMT with a frequency of 20 Hz. Since systematic comparisons are lacking regarding these parameters, it is hard to reason which should be preferred. Some evidence indicates that the prefrontal cortices might require higher stimulation intensity than the motor cortex (Thomson et al., 2011). However, cognitive improvements in dementia were observed when applying a range of parameters covering subthreshold and suprathreshold intensities as well.
While the beneficial effects of TMS were further supported, mixed results were found regarding the efficacy of tDCS. Albeit all studies with a moderate or high risk of bias were optimistic regarding the efficacy of tDCS, Khedr et al. (2014) have found the facilitatory effect of both anodal and cathodal tDCS, whereas two high-quality studies have not found any effect of tDCS on cognition in dementia (Suemoto et al., 2014; Bystad et al., 2016). Although all three studies stimulated AD patients recruited based on similar criteria and each used tDCS on 2 mA intensity, two different brain areas (LDLPFC and left temporal cortex) were stimulated. In addition, the duration of the stimulation and the overall number of sessions was different as well. The only study with a low risk of bias that detected a cognitive change applied the highest number of sessions (10 sessions) and a relatively long session duration (25 min/session) compared to the other high-quality studies (for a summarization see Table 4). Despite the evidence available on the effects of intensity and duration on the excitability of the motor cortex (Agboada et al., 2019), optimal parameters for stimulating cognition are currently lacking. However, tDCS studies with low risk of bias featured deviations of effects from the hypothesized direction and null results. It indicates that NIBS effect estimates might be prone to the confounding factors in studies with less experimental rigidity.
More than 75% of RCTs involved some levels of bias in at least one domain, according to our risk of bias assessment. The most affected domains were the randomization process and the selection of the reported data. Unclear reporting was also observable which involved the allocation concealment, the randomization, the method of blinding, and the managing of drop-outs. Although the risk of bias in non-RCTs was not assessed systematically, most of them explicitly set the goal of measuring the efficacy of NIBS. In this case, the lack of sham-control and blinding is a major methodical drawback that confounds the results. On the other hand, case studies allow investigating new and more unique protocols, such as the strikingly long stimulation period of 8 months of Bystad et al. (2017).
A considerable amount of variance was detected between studies applying either TMS or tDCS present concerning the number of sessions, the stimulation duration and intensity, the choice and location of target regions, and the type of sham stimulation. It has been emphasized that due to the diversity of protocols, studies are less comparable, and it is more difficult to evaluate the underlying causes of the results (Chang et al., 2018). We attempted to synthesize these studies to determine a range of stimulation parameters that seem to be effective in treating cognition of AD and MCI patients. Also, we introduced some options that might guide the design of new research.
Recommendations on Design and Methodology
The design should always be chosen depending on the research question and considering its specific advantages and disadvantages. Non-RCTs may be less optimal to evaluate the effectiveness of a stimulation protocol compared to RCTs; however, they can help in understanding the feasibility of new paradigms. RCTs are considered the gold standard of study designs. Some drawbacks of them are the ethical considerations of the formation of some groups (e.g., a control group of demented patients left without rehabilitation is unacceptable) and the under-representativeness of specific comorbidities, aggressive behavior and minorities of the target population (Cohen-Mansfield et al., 2014). On the other hand, homogenous sampling reduces the variability of the studied factors, thus introduces higher statistical power. Parallel-group RCTs require a higher sample size than crossover-design studies; although, the blinding of NIBS condition in the latter design is more vulnerable.
Clear reporting is essential and should involve: (1) the method of randomization, (2) the allocation concealment, (3) whether the participants, their caregivers, the staff delivering the stimulation, the outcome assessors and the person conducting the statistical analysis were blind to the type of NIBS, (4) the occurrence, reason and management of missing data points or drop-outs, and (5) whether statistical analysis plan was predefined and what tests were conducted. While different guidelines repeatedly urge the improvement of reporting, it remains a serious issue in clinical trials (MacPherson et al., 2010; Schulz et al., 2010).
Randomization, blinding, and allocation concealment are all feasible methods to reduce information bias in studies with the appropriate design. Allocation concealment was found strongly underreported here and in other reports; due to which its effect on the results is hard to estimate (Savović et al., 2012). The lack of proper blinding seems to be one of the most influential sources of information bias, leading to the overestimation of the intervention by 13% on average (Savović et al., 2012). To avoid information bias, a viable solution is to have an independent staff member delivering the intervention who is not involved with other stages of the research. The built-in function of tDCS is also a great option for randomization and blinding.
It must be stated that blinding is not as straightforward as it may seem in NIBS trials (Kessler et al., 2012; Fonteneau et al., 2019). Skin redness or on the contrary, the lack of skin sensations during NIBS might alleviate the effective blinding of patients and assessors as well, to some extent. Reflecting on this issue, some sham TMS methods incorporate prerecorded sounds to mimic TMS pulses (Zhao et al., 2017), or weak electric stimulation of the scalp to reproduce skin sensations; although, participants with previous experience with TMS might be hard to blind even with these methods (Mennemeier et al., 2009). Vertex stimulation has been proposed as another solution that has been supported by a recent study (Jung et al., 2016). According to functional neuroimaging results, vertex stimulation does not result in elevated activation of the stimulated site; however, a widespread decrease of brain areas related to the default brain network has been observed. This effect might be reduced by tilting the coil; thus, reducing the effectiveness (but also the induced skin sensations) of the stimulation. This approach has been chosen by some of the reviewed studies too (Cotelli et al., 2006, 2008). To provide insight into the mechanism of how active TMS over a given brain area affects cognitive function, the use of multiple control methods including sham NIBS and the active stimulation of a control site has been strongly recommended (Duecker and Sack, 2015).
Some evidence suggests that participants can distinguish the active tDCS condition from the sham trials above chance-level, which might be an important limitation of crossover-designed studies (Wallace et al., 2016; Turi et al., 2019). Moreover, short-interval active stimulation applied as a sham condition can result in exaggerated placebo responses and has the potential to even modulate relevant brain areas (Fonteneau et al., 2019). This might be of interest since the sham condition in every examined tDCS study consisted of a short duration of active stimulation. A novel sham method involving 30–30 s of active tDCS at the beginning and at the end of the sham stimulation to provide more convincing sensory experiences has been described in the protocol of Hampstead and Hartley (2015). This might be an interesting solution assuming that 1 min of stimulation does not result in major neuronal effects. To sum up, the blinding of NIBS is not completely without unresolved issues. Consequently, it is strongly advised to ask participants what they think which type of stimulation they received. Inserting this simple procedure into the research process may validate the blinding and in the long term, it can enhance the comparison of different procedures.
Careful consideration is recommended prior to the selection of the optimal testing instrument or battery. The cognitive subsection of the Alzheimer Disease Assessment Scale (ADAS-Cog) and the MMSE, the two most common tests we identified, are recognized as standard instruments for assessing global cognition in AD. The ADAS-Cog takes around 40 min to administer, while the MMSE is a substantially shorter and simpler tool (Hannesdóttir and Snædal, 2002). Additionally, outstanding reliability and validity properties and reliable change indices are available for both batteries (ADAS-Cog: 3 points and MMSE: 2–4 points of improvement) (Hensel et al., 2007; Bossers et al., 2012). Notably, some deficiencies have been emphasized regarding the accuracy of both tests. Most importantly, their sensitivity to change has found to be low (Bossers et al., 2012), while this would be essential to capture the NIBS treatment-related effects. Secondly, floor and ceiling effects are present in the case of both batteries (Cano et al., 2010; Edgar et al., 2015), and might cause problems particularly in MCI. ADAS-Cog has been further criticized since cognitive decline indicated by this tool cannot be considered as clinically relevant in the elderly (Rockwood et al., 2007). To overcome these drawbacks, alternative scoring methods have been recommended; however, none of the identified articles mentioned or applied them (Verma and Howard, 2012; Philipps et al., 2014; Kueper et al., 2018).
When repeated testing occurs, practice effects should not be ignored. Alternate versions of the tasks proven not to differ from each other in difficulty can be applied. Since it can be assumed that practice effects decrease over time, baseline measurement might be recorded weeks or months prior to the commencement of NIBS therapy. However, it is not clear how long the ideal period would be between two sessions, as practice effects seem to persist for years in healthy adults, and remarkable practice effects have been found in AD and in a subgroup of MCI patients as well (Galasko et al., 1993; Gross et al., 2018). Moreover, sudden changes in cognitive state cannot be ruled out; thus, the risk of drop-outs might increase. Averaging two baseline measurements might be more viable since the strongest association appears to be between the first two administrations of a task. Also, this method can reduce the confounding of the fluctuations of the cognitive state. Otherwise, practice effects may carry clinically useful information about the prognosis of the disease in the elderly with cognitive impairment and may be used as an indicator of the successfulness of brain stimulation (Verma and Howard, 2012; Weuve et al., 2015). Theta-burst stimulation (TBS), a patterned version of rTMS, has been found to modulate practice effects in healthy subjects (Vékony et al., 2018), and an effect of NIBS on practice effects might be speculated in demented samples as well.
The way of handling missing data points or drop-outs should be conducted following the available guidelines (Altman, 2009). Moreover, researchers should predefine how missing values will be handled beforehand. Imputation methods are encouraged to be used; however, the last observation carried forward (LOFT) as a sole form of analysis has been criticized and not recommended by statisticians (Altman, 2009). Rabey et al. (2013) applied sensitivity analysis for the observed data only and for worst-case analysis, which is a highly suggested procedure (Carpenter et al., 2007). Also, the results of both analyses have been reported and evaluated, considered as the optimal way of managing missing data according to the guide steps of Altman (2009).
The statistical analysis of the gathered data should also gain more attention. Firstly, blinding should be maintained throughout the statistical evaluation of the data to minimize information bias. Secondly, clinical researchers should follow the trends in statistics and evaluate their applicability in their area. For instance, the “Bayesian revolution” can add meaningful tools to revisit the results (Etz and Vandekerckhove, 2016). Null effects (when statistically no significant difference has been observed) should be further investigated by measuring the strength of evidence using the Bayes factor or equivalence testing (Lakens et al., 2018). Bayesian statistics can reinforce the findings gathered by traditional statistical methods and support the strength of non-significant results. Also, the results should be made available in order to reduce publication bias and selective reporting. Reporting null effects is especially crucial in research involving patient populations as publication bias can lead to the overestimation of the effect of NIBS. This might even lead to the advancement of a less effective treatment over a more effective one.
Recommendations on Stimulation Parameters
Clear reporting of stimulation parameters is equally essential as of the research methods. Little research is available comparing different stimulation parameters; moreover, their results might not be generally applicable (e.g., in different populations, over different brain areas). Considering TMS, when stimulating the motor cortex, 10 Hz stimulation failed to have an effect on motor evoked potentials (Maeda et al., 2000), while 10 Hz as compared to 15 Hz TMS similarly improved the cognitive function of AD patients (Devi et al., 2014). In addition, some stimulation methods are developed to achieve a specific result. Different types of coil induce electric fields that are distinctive from one another regarding the focality and the depth of the stimulation (Lu and Ueno, 2017) which highlights the importance of detailed reporting.
Similarly, the position, number, and size of tDCS electrodes might affect the focality and the target of the stimulation to an extent (Bai et al., 2014). Extracephalic reference electrode placement as compared to cathode placement over a cephalic region results in higher current density in deeper brain regions and white matter at the cost of stimulating in a more diffuse way (Noetscher et al., 2014). Therefore, a detailed description of the stimulation methods is essential as it provides an opportunity to determine which brain regions might have been stimulated and whether the stimulation was more focal, or it extended to other brain sites. The comparison of studies with different or unknown parameters might introduce bias to the estimates of efficacy and the outcomes of the results.
Based on the results of the recruited studies with low or moderate risk of bias, the following TMS parameters are most likely within the range of effectiveness when targeting the cognitive function of AD or MCI patients: 10 or more sessions with 1,200–2,000 pulses per session, a frequency of 10–20 Hz for HF-TMS and 1 Hz for LF-TMS, an intensity of 80–120% of the RMT (see Figure 4). To address the heterogeneity of the aim and parameters of these studies, a subgroup of RCTs that administered HF-TMS with a figure-of-eight coil were tabulated (Table 5). This set of studies got selected because of the overwhelming popularity of facilitatory stimulation not only in this specific field but in all fields of TMS research where the therapeutic effects of the device are being investigated. The risk of bias and the reported outcomes of these studies are also indicated to enhance comparison. When the parameters of these studies are taken into consideration, a similar optimum as previously described seems to emerge: the most frequent settings were 10 or more sessions with a mean of ~2,000 pulses given on the 90–100% of the RMT (Figure 5 depicts the stimulation parameters of the studies in Table 5). Setting fixed intensity has also been proposed (Kaminski et al., 2011) referring to the fact that individual adaptation of TMS intensities has not yet been proven to achieve more reliable behavioral effects. This approach was only present in one study, which nonetheless found TMS to improve global cognition in AD (Avirame et al., 2016). Additionally, combining facilitatory NIBS with cognitive stimulation seems to be a promising approach as all studies applying this approach have reported the enhancement of cognition (Bentwich et al., 2011; Rabey et al., 2013; Lee et al., 2016; Rabey and Dobronevsky, 2016; Nguyen et al., 2017). It should be noted that LF stimulation was underrepresented with only 2 out of 34 studies applying it (Ahmed et al., 2012; Turriziani et al., 2012); thus, its effects should be further investigated.
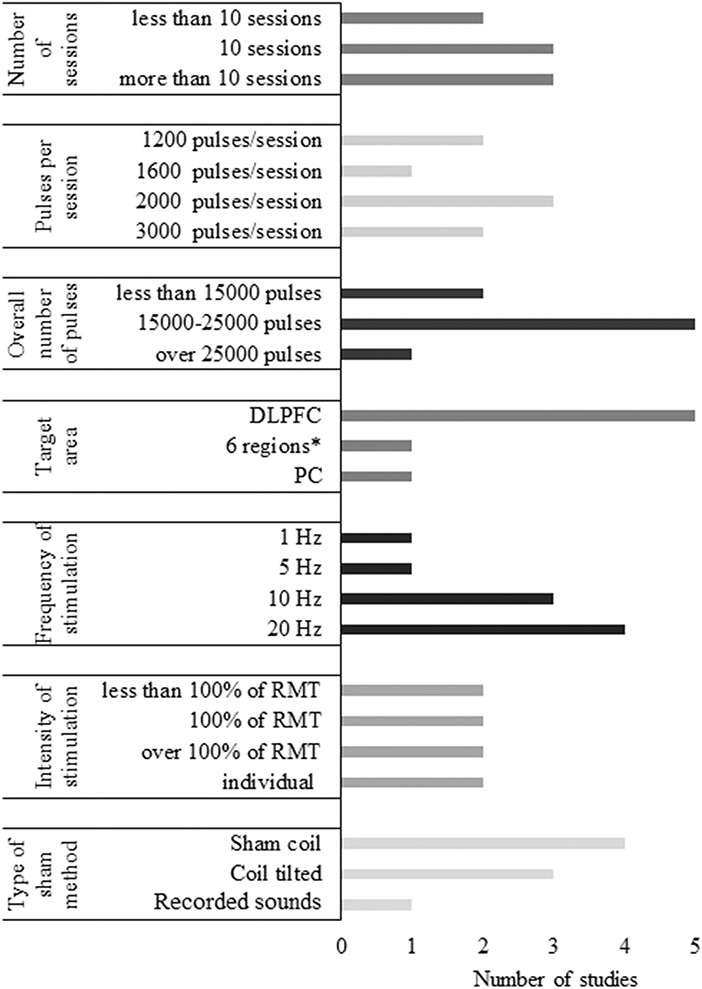
Figure 4. Summary of the stimulation parameters of TMS studies with low or moderate risk of bias. *6 brain regions: Broca's area, Wernicke's area, LDLPFC, RDLPFC, R-pSAP, and L-pSAC (as in Table 2).
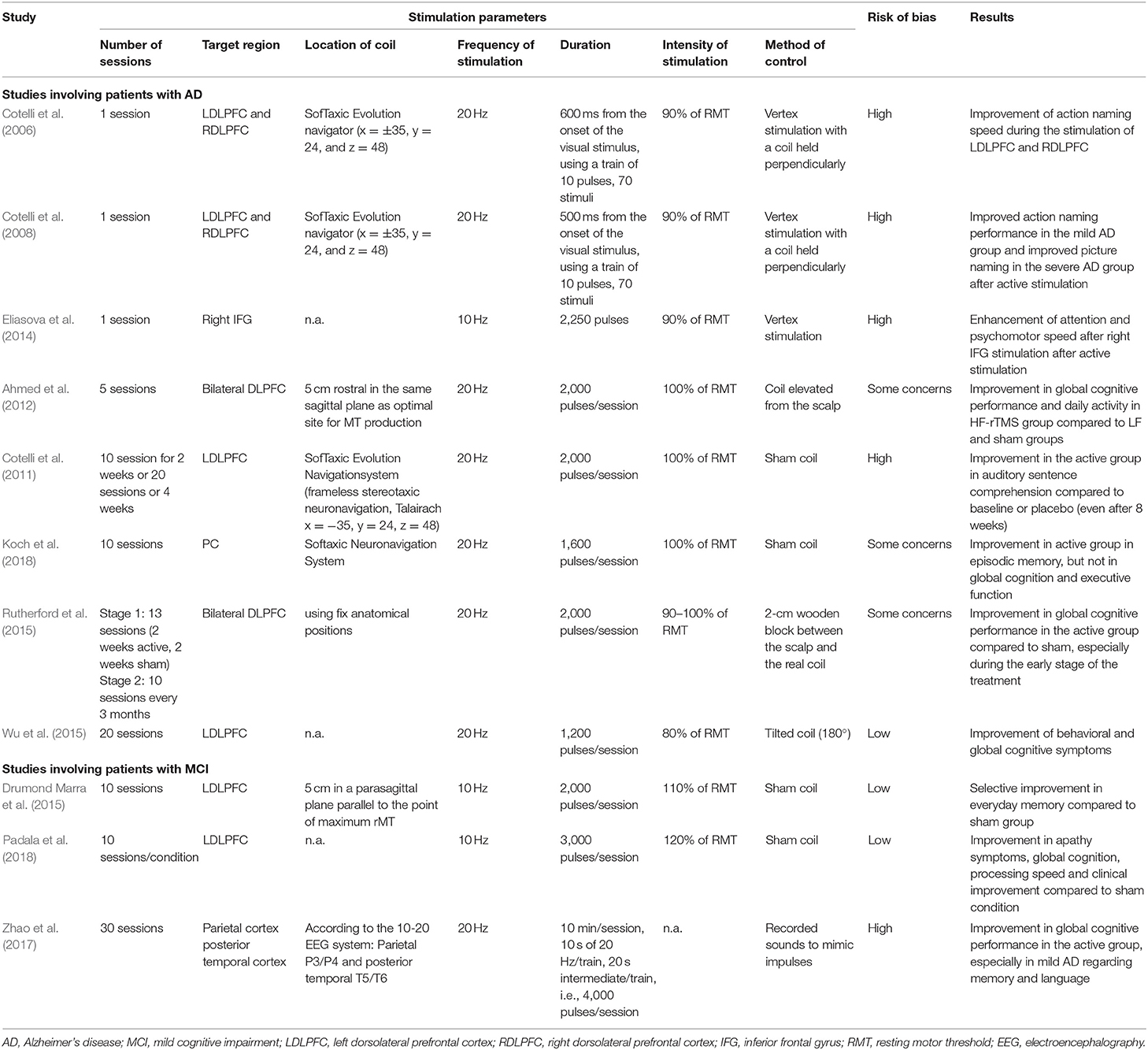
Table 5. Stimulation paramterers and findings of randomized-controlled trials applying high-frequency TMS using a figure-of-eight coil.
Regarding tDCS, stimulation parameters are hard to recommend since studies with the highest reliability questioned the efficacy of the most common paradigm involving multiple-session anodal (and cathodal) stimulation on 2 mA intensity (Khedr et al., 2014; Suemoto et al., 2014; Bystad et al., 2016). Further high-quality research is needed to explore under what circumstances may tDCS be beneficial in dementia (for a summarization of the stimulation parameters of tDCS studies with low or moderate risk of bias see Figure 6).
Targeting the DLPFC is not only widely frequent but leads to satisfactory results. However, its localization should be carefully implemented. TMS-based definition of the DLPFC with respect to the motor hotspot did not overlay with the anatomical location in healthy subjects (Ahdab et al., 2016) which may cause differences between studies even if the same brain region was originally intended to be targeted. Localization according to the international EEG system, on the other hand, seems to offer a relatively sufficient approximation (Fitzgerald et al., 2009). This method is already frequently used in tDCS studies and might be a non-neuronavigated alternative for TMS studies as well. Neuronavigation is common in TMS research and is usually based on structural images of the participants' brains. Nonetheless, stimulation based on the functional connections of the individual brain might be an even better approach considering its high accuracy (Sparing and Mottaghy, 2008). Similarly, the use of group-based as compared to individual coordinates to establish target location is also an aspect to be considered, as it raises further questions about stimulation efficacy (Sparing et al., 2009).
The stimulation of multiple sites may not enhance NIBS effectiveness as compared to targeting a more focal area. This has been supported by the findings of Alcalá-Lozano et al. (2018) reporting the effects of stimulation over six regions of interest and a simple protocol over the LDLPFC similarly effective in AD (Alcalá-Lozano et al., 2018). On the other hand, more studies should explore the potential of stimulating other brain areas considering the promising results of the few available studied targeting different brain sites, and the fact that other cortical regions are also affected in dementia (Ruan et al., 2016).
Another important aspect that needs to be considered is that NIBS not only modulates the brain tissue underlying the coil/electrode. Even paradigms believed to be relatively focal such as the application of TMS using a figure-of-eight coil might induce activation in functionally or structurally connected brain areas (Nahas et al., 2001; Siebner et al., 2009; Hanlon et al., 2013). Brain regions organize into brain networks to implement various cognitive and other operations (Pessoa, 2014). Both TMS and tDCS can modulate functional networks of the brain which capability can be utilized for studying and treating brain disorders (To et al., 2018). In stroke patients, LF-TMS over the contralesional primary motor cortex changed the functional connectivity of the related brain network and resulted in behavioral improvement of motor functions (Grefkes et al., 2010). Prefrontal tDCS of healthy adult also resulted in the connectivity changes of distinct functional networks close to the stimulation site and its connected regions (Keeser et al., 2011). Targeting brain hubs of those networks that are affected in dementia might lead to new (and maybe more personalized) treatment solutions. The idea of targeting brain hubs was supported by one of the identified studies where atDCS of the IFG has been found to reverse the abnormal activity of several networks and to improve the overall cognitive performance in MCI (Meinzer et al., 2015).
It is poorly understood how different stimulation parameters contribute to the outcome of the stimulation. When frequency was kept constant, 3.125 Hz stimulation over the left motor cortex at either a subthreshold (at 90% of RTM) or a suprathreshold (at 110% of RTM) intensity enhanced the activation of cortical and subcortical regions of the motor (and the auditory) system (Bestmann et al., 2004). However, when subthreshold stimulation was administered, the magnitude of activation was lower in the remote sites and the effect on the target area could not be distinguished from the physiological level. Similarly, subthreshold (at 80% of RMT) stimulation during LF-TMS has been found to cause the drop of oxygenation level; however, to a shorter time period than suprathreshold (at 120% of RMT) stimulation (Thomson et al., 2012). On the contrary, different connectivity patterns emerged when facilitatory TBS over the LDLPFC at 90% of the RMT was compared with suprathreshold TBS (120% of RMT) (Alkhasli et al., 2019). When the dose of TMS was kept constant at 120% of the RMT, the effectiveness of 10 and 20 Hz rTMS over the LDLPFC was comparable in treating the affective symptoms of patients with major depression (DeBlasio and Tendler, 2012). These studies not only reveal that different methods might act through different mechanisms, but they also shed light on the diversity of how brain activity can be operationalized. More systematic comparisons on how the different parameters and their combinations modify the outcome might pave the way for TMS therapies tailored to the patient. Accordingly, it has been suggested that individualized, connectivity-based stimulation might serve as a means to optimize TMS efficacy (Fox et al., 2012).
Combining brain imaging and electrophysiological techniques with NIBS methods might offer deeper insight into the underlying mechanisms of brain stimulation. To date, only a few studies of such are available and they have suggested the reversion of abnormal brain mechanisms, observed by both EEG and fMRI (Meinzer et al., 2015; Marceglia et al., 2016). Additionally, new NIBS methods such as TBS, deep TMS (dTMS), accelerated or spaced TMS and high-definition tDCS (HD-tDCS) might be also considered to apply in future studies. Deep cortical regions might be stimulated by applying dTMS, with the use of specified coil configurations such as an H-shaped coil (Bersani et al., 2013). It has been administered in AD patients and found to be effective in improving global cognition to a great extent and is associated with similar effects as traditional rTMS protocols (Zafar et al., 2008; Blumberger et al., 2018). Strikingly, only one research proposal was found aiming to measure its effectiveness on the cognition of demented patients. The utilization of specialized small electrodes (i.e., high-definition tDCS, HD-tDCS) appears to be promising as well and is currently tested on healthy individuals (Hampstead and Hartley, 2015; Turski et al., 2017).
Prospects and Limitations of the Present Review
Limitations of this review include the lack of quality assessment of non-RCTs. However, as previous analyses have indicated (Lange et al., 2017), the majority of the recruited studies had an RCT design. Also, most of the identified non-RCTs aimed to measure the cognitive effects of NIBS which is biased by the nature of the design. Non-RCTs are more suitable to examine the feasibility and acceptability of new protocols, and indeed some of the studies have investigated new methods. The lack of quantitative analysis may also be considered as a limitation of this review. In order to quantify the results, reliable studies more similar to each other regarding the intervention, measurements, and the sample should be available (Greenfield et al., 2007). The qualitative summarization presented here aims to increase the number of such articles and to pave the way for future quantitative meta-analyses. Some articles might not be identified as restrictions were made regarding the language and due to not including a gray literature search.
Our current findings on the narrower sample of TMS and tDCS studies in AD and MCI can be expanded to other brain stimulation methods and different types of dementias. However, this requires the consideration of the specificities of the given method and population. While AD and MCI mostly differ in the severity and number of cognitive symptoms, the cognitive profile and the trajectory of decline show significant differences in other dementias (Smits et al., 2015). While episodic learning is impaired in AD affecting immediate and delayed recall and the deficit of working memory and executive functioning is present, language skills are more preserved as compared to frontotemporal dementia. Attention and visuospatial dysfunctions are more characteristic to dementia with Lewy bodies (Sparing et al., 2009). Therefore, researchers should consider which cognitive function to assess and train if cognitive training is included. Also, the double baseline approach might be considered in order to reduce the effects of fluctuating performance which is often observable in patients with frontotemporal dementia (Smeding and de Koning, 2000; Lange et al., 2017). Due to these fluctuations, the number of missing data may also increase during cognitive assessments (Smeding and de Koning, 2000; Lange et al., 2017). As recommended above, the management and the statistical methods to assess their effects should be predetermined and reported.
In the present review, we considered measurements of the cognitive domain only; however, neuropsychiatric symptoms are considered to be closely linked with cognitive disturbances causing reduced quality of life in neurodegenerative disorders (Rog et al., 2014; van der Linde et al., 2016). Different scales are used to measure neuropsychiatric symptoms in patients, mostly applying self-report questionnaires, which introduces new sources of bias (Althubaiti, 2016). Scales that collect information from different sources, such as from the caregivers and/or clinicians should be preferred (Sheehan, 2012). Moreover, these seem to be more reliable in the presence of unclear blinding efficacy (Wood et al., 2008).
Conclusion
In the present review, we described and examined for the first time the actual presence of methodical factors that can obscure the results when investigating the effects of NIBS in MCI and AD. Great diversity among stimulation parameters was found, a common characteristic of all NIBS studies in a general sense. The risk of bias affects most of the identified studies to a various extent. We revealed that the conclusion of studies with low risk of bias differs from the others regarding the efficacy of NIBS. This indicates that potential sources of bias can lead to further distortions of the estimated effects of NIBS. Therefore, cautious planning and rigorous implementation are highly advised with the consideration of the aspects we collected.
At this point, based on the currently available literature, it is difficult to conclude the effectiveness of NIBS methods in dementia research. Nonetheless, some arguments can be made. Our results indicate that TMS exerts more prominent and reliable behavioral effects. Moreover, we identified a range of TMS parameters that seem to effectively achieve behavioral improvements based on the reviewed articles and further evidence. Also, the combination of NIBS with cognitive stimulation appears to be a promising approach in MCI and AD. We argue that, with the elimination of the identified methodological issues, the variability of results would be reduced, their interpretation improved, and stronger conclusions could be drawn for the future implementation of NIBS.
Author Contributions
AH, PK, LV, and AM contributed conception and design of the study. AH conducted the literature search and data extraction, VN, TV, and AM supervised the literature search and data extraction. AH and VN conducted the risk of bias assessment. AH wrote the draft of the manuscript. All authors contributed to manuscript revision, read, and approved the submitted version.
Funding
This study was supported by the University of Szeged Open Access Fund (grant number: 4534). AH was supported by the grant EFOP 3.6.3-VEKOP-16-2017-00009. LV received support from the grants GINOP-2.3.2-15-2016-00034 and GINOP 2.3.2-15-2016-00048. PK was supported by the Hungarian Brain Research program: 2017-1.2.1-NKP-2017-00002. AM received support from the Bolyai Scholarship Programme of the Hungarian Academy of Sciences and from the EU-funded Hungarian grant EFOP-3.6.1-16-2016-00008.
Conflict of Interest
The authors declare that the research was conducted in the absence of any commercial or financial relationships that could be construed as a potential conflict of interest.
Acknowledgments
We are grateful to Mihály Racsmány for his insightful comments on the drafts of the manuscript.
References
Agboada, D., Mosayebi Samani, M., Jamil, A., Kuo, M.-F., and Nitsche, M. A. (2019). Expanding the parameter space of anodal transcranial direct current stimulation of the primary motor cortex. Sci. Rep. 9, 1–11. doi: 10.1038/s41598-019-54621-0
Ahdab, R., Ayache, S. S., Brugières, P., Farhat, W. H., and Lefaucheur, J.-P. (2016). The hand motor hotspot is not always located in the hand knob: a neuronavigated transcranial magnetic stimulation study. Brain Topogr. 29, 590–597. doi: 10.1007/s10548-016-0486-2
Ahmed, M. A., Darwish, E. S., Khedr, E. M., El Serogy, Y. M., and Ali, A. M. (2012). Effects of low versus high frequencies of repetitive transcranial magnetic stimulation on cognitive function and cortical excitability in Alzheimer's dementia. J. Neurol. 259, 83–92. doi: 10.1007/s00415-011-6128-4
Albert, M. S., DeKosky, S. T., Dickson, D., Dubois, B., Feldman, H. H., Fox, N. C., et al. (2011). The diagnosis of mild cognitive impairment due to Alzheimer's disease: recommendations from the National Institute on Aging-Alzheimer's Association workgroups on diagnostic guidelines for Alzheimer's disease. Alzheimers Dement. 7, 270–279. doi: 10.1016/j.jalz.2011.03.008
Alcalá-Lozano, R., Morelos-Santana, E., Cortés-Sotres, J. F., Garza-Villarreal, E. A., Sosa-Ortiz, A. L., and González-Olvera, J. J. (2018). Similar clinical improvement and maintenance after rTMS at 5 Hz using a simple vs. complex protocol in Alzheimer's disease. Brain Stimul. 11, 625–627. doi: 10.1016/j.brs.2017.12.011
Alkhasli, I., Sakreida, K., Mottaghy, F. M., and Binkofski, F. (2019). Modulation of fronto-striatal functional connectivity using transcranial magnetic stimulation. Front. Hum. Neurosci. 13:190. doi: 10.3389/fnhum.2019.00190
Althubaiti, A. (2016). Information bias in health research: definition, pitfalls, and adjustment methods. J. Multidiscipl. Healthc. 9, 211–217. doi: 10.2147/JMDH.S104807
Altman, D. G. (2009). Missing outcomes in randomized trials: addressing the dilemma. Open Med. 3, e51–e53.
Andrade, S. M., de Mendonça, C. T. P. L., Pereira, T. C. L., Fernandez-Calvo, B., Araújo, R. C. N., and Alves, N. T. (2016). Adjuvant transcranial direct current stimulation for treating Alzheimer's disease: a case study. Dement. Neuropsychol. 10, 156–159. doi: 10.1590/S1980-5764-2016DN1002013
Avirame, K., Stehberg, J., and Todder, D. (2016). Benefits of deep transcranial magnetic stimulation in Alzheimer disease: case series. J. ECT 32, 127–133. doi: 10.1097/YCT.0000000000000286
Bai, S., Dokos, S., Ho, K.-A., and Loo, C. (2014). A computational modelling study of transcranial direct current stimulation montages used in depression. Neuroimage 87, 332–344. doi: 10.1016/j.neuroimage.2013.11.015
Bentwich, J., Dobronevsky, E., Aichenbaum, S., Shorer, R., Peretz, R., Khaigrekht, M., et al. (2011). Beneficial effect of repetitive transcranial magnetic stimulation combined with cognitive training for the treatment of Alzheimer's disease: a proof of concept study. J. Neural Transm. 118, 463–471. doi: 10.1007/s00702-010-0578-1
Bersani, F. S., Minichino, A., Enticott, P. G., Mazzarini, L., Khan, N., Antonacci, G., et al. (2013). Deep transcranial magnetic stimulation as a treatment for psychiatric disorders: a comprehensive review. Eur. Psychiatry 28, 30–39. doi: 10.1016/j.eurpsy.2012.02.006
Bestmann, S., Baudewig, J., Siebner, H. R., Rothwell, J. C., and Frahm, J. (2004). Functional MRI of the immediate impact of transcranial magnetic stimulation on cortical and subcortical motor circuits. Eur. J. Neurosci. 19, 1950–1962. doi: 10.1111/j.1460-9568.2004.03277.x
Blumberger, D. M., Vila-Rodriguez, F., Thorpe, K. E., Feffer, K., Noda, Y., Giacobbe, P., et al. (2018). Effectiveness of theta burst versus high-frequency repetitive transcranial magnetic stimulation in patients with depression (THREE-D): a randomised non-inferiority trial. Lancet 391, 1683–1692. doi: 10.1016/S0140-6736(18)30295-2
Boggio, P. S., Ferrucci, R., Mameli, F., Martins, D., Martins, O., Vergari, M., et al. (2012). Prolonged visual memory enhancement after direct current stimulation in Alzheimer's disease. Brain Stimul. 5, 223–230. doi: 10.1016/j.brs.2011.06.006
Boggio, P. S., Khoury, L. P., Martins, D. C. S., Martins, O. E. M. S., de Macedo, E. C., and Fregni, F. (2009). Temporal cortex direct current stimulation enhances performance on a visual recognition memory task in Alzheimer disease. J. Neurol. Neurosurg. Psychiatry 80, 444–447. doi: 10.1136/jnnp.2007.141853
Bossers, W. J. R., van der Woude, L. H. V., Boersma, F., Scherder, E. J. A., and van Heuvelen, M. J. G. (2012). Recommended measures for the assessment of cognitive and physical performance in older patients with dementia: a systematic review. Dement. Geriatr. Cogn. Disord. 2, 589–609. doi: 10.1159/000345038
Bramer, W. M., Rethlefsen, M. L., Kleijnen, J., and Franco, O. H. (2017). Optimal database combinations for literature searches in systematic reviews: a prospective exploratory study. Syst. Rev. 6:245. doi: 10.1186/s13643-017-0644-y
Buscemi, N., Hartling, L., Vandermeer, B., Tjosvold, L., and Klassen, T. P. (2006). Single data extraction generated more errors than double data extraction in systematic reviews. J. Clin. Epidemiol. 59, 697–703. doi: 10.1016/j.jclinepi.2005.11.010
Bystad, M., Grønli, O., Rasmussen, I. D., Gundersen, N., Nordvang, L., Wang-Iversen, H., et al. (2016). Transcranial direct current stimulation as a memory enhancer in patients with Alzheimer's disease: a randomized, placebo-controlled trial. Alzheimers Res. Ther. 8:13. doi: 10.1186/s13195-016-0180-3
Bystad, M., Rasmussen, I. D., Grønli, O., and Aslaksen, P. M. (2017). Can 8 months of daily tDCS application slow the cognitive decline in Alzheimer's disease? A case study. Neurocase 23, 146–148. doi: 10.1080/13554794.2017.1325911
Cano, S. J., Posner, H. B., Moline, M. L., Hurt, S. W., Swartz, J., Hsu, T., et al. (2010). The ADAS-cog in Alzheimer's disease clinical trials: psychometric evaluation of the sum and its parts. J. Neurol. Neurosurg. Psychiatry 81, 1363–1368. doi: 10.1136/jnnp.2009.204008
Carpenter, J. R., Kenward, M. G., and White, I. R. (2007). Sensitivity analysis after multiple imputation under missing at random: a weighting approach. Stat. Methods Med. Res. 16, 259–275. doi: 10.1177/0962280206075303
Chang, C.-H., Lane, H.-Y., and Lin, C.-H. (2018). Brain stimulation in Alzheimer's disease. Front. Psychiatry 9:201. doi: 10.3389/fpsyt.2018.00201
Cohen-Mansfield, J., Buckwalter, K., Beattie, E., Rose, K., Neville, C., and Kolanowski, A. (2014). Expanded review criteria: the case of nonpharmacological interventions in dementia. J. Alzheimers Dis. 41, 15–28. doi: 10.3233/JAD-132357
Cotelli, M., Calabria, M., Manenti, R., Rosini, S., Maioli, C., Zanetti, O., et al. (2012). Brain stimulation improves associative memory in an individual with amnestic mild cognitive impairment. Neurocase 18, 217–223. doi: 10.1080/13554794.2011.588176
Cotelli, M., Calabria, M., Manenti, R., Rosini, S., Zanetti, O., Cappa, S. F., et al. (2011). Improved language performance in Alzheimer disease following brain stimulation. J. Neurol. Neurosurg. Psychiatry 82, 794–797. doi: 10.1136/jnnp.2009.197848
Cotelli, M., Manenti, R., Cappa, S. F., Geroldi, C., Zanetti, O., Rossini, P. M., et al. (2006). Effect of transcranial magnetic stimulation on action naming in patients with Alzheimer disease. Arch. Neurol. 63, 1602–1604. doi: 10.1001/archneur.63.11.1602
Cotelli, M., Manenti, R., Cappa, S. F., Zanetti, O., and Miniussi, C. (2008). Transcranial magnetic stimulation improves naming in Alzheimer disease patients at different stages of cognitive decline. Eur. J. Neurol. 15, 1286–1292. doi: 10.1111/j.1468-1331.2008.02202.x
Cruz Gonzalez, P., Fong, K. N. K., and Brown, T. (2018). The effects of transcranial direct current stimulation on the cognitive functions in older adults with mild cognitive impairment: a pilot study. Behav. Neurol. 2018:5971385. doi: 10.1155/2018/5971385
DeBlasio, K. L., and Tendler, A. (2012). A retrospective chart review of 10 Hz versus 20 Hz repetitive transcranial magnetic stimulation for depression. SAGE Open 2:2158244012470109. doi: 10.1177/2158244012470109
Devi, G., Voss, H. U., Levine, D., Abrassart, D., Heier, L., Halper, J., et al. (2014). Open-label, short-term, repetitive transcranial magnetic stimulation in patients with Alzheimer's disease with functional imaging correlates and literature review. Am. J. Alzheimers Dis. Other Dement. 29, 248–255. doi: 10.1177/1533317513517047
Drumond Marra, H. L., Myczkowski, M. L., Maia Memória, C., Arnaut, D., Leite Ribeiro, P., Sardinha Mansur, C. G., et al. (2015). Transcranial magnetic stimulation to address mild cognitive impairment in the elderly: a randomized controlled study. Behav. Neurol. 2015:287843. doi: 10.1155/2015/287843
Dubois, B., Hampel, H., Feldman, H. H., Scheltens, P., Aisen, P., Andrieu, S., et al. (2016). Preclinical Alzheimer's disease: definition, natural history, and diagnostic criteria. Alzheimer Dementia 12, 292–323. doi: 10.1016/j.jalz.2016.02.002
Duecker, F., and Sack, A. T. (2015). Rethinking the role of sham TMS. Front. Psychol. 6:210. doi: 10.3389/fpsyg.2015.00210
Edgar, C., Rylands, A. J., Volz, D., Mertes, M., Gruendl, E., Fontoura, P., et al. (2015). Comparative traditional psychometrics of cognitive and functional endpoints in a prodromal Alzheimer's disease population. in Ostrowitzki, S., Lasser, R. A., Dorflinger, E., Scheltens, P., Barkhof, F., Nikolcheva, T., et al. (2017). A phase III randomized trial of gantenerumab in prodromal Alzheimer's disease. Alzheimers Res. Ther. 9:95. doi: 10.1186/s13195-017-0318-y
Elder, G. J., and Taylor, J.-P. (2014). Transcranial magnetic stimulation and transcranial direct current stimulation: treatments for cognitive and neuropsychiatric symptoms in the neurodegenerative dementias? Alzheimers Res. Ther. 6:74. doi: 10.1186/s13195-014-0074-1
Eliasova, I., Anderkova, L., Marecek, R., and Rektorova, I. (2014). Non-invasive brain stimulation of the right inferior frontal gyrus may improve attention in early Alzheimer's disease: a pilot study. J. Neurol. Sci. 346, 318–322. doi: 10.1016/j.jns.2014.08.036
Etz, A., and Vandekerckhove, J. (2016). A Bayesian perspective on the reproducibility project: psychology. PLoS ONE 11:e0149794. doi: 10.1371/journal.pone.0149794
Ferrucci, R., Mameli, F., Guidi, I., Mrakic-Sposta, S., Vergari, M., Marceglia, S., et al. (2008). Transcranial direct current stimulation improves recognition memory in Alzheimer disease. Neurology 71, 493–498. doi: 10.1212/01.wnl.0000317060.43722.a3
Fitzgerald, P. B., Maller, J. J., Hoy, K. E., Thomson, R., and Daskalakis, Z. J. (2009). Exploring the optimal site for the localization of dorsolateral prefrontal cortex in brain stimulation experiments. Brain Stimul. 2, 234–237. doi: 10.1016/j.brs.2009.03.002
Fonteneau, C., Mondino, M., Arns, M., Baeken, C., Bikson, M., Brunoni, A. R., et al. (2019). Sham tDCS: a hidden source of variability? Reflections for further blinded, controlled trials. Brain Stimul. 12:668–673. doi: 10.1016/j.brs.2018.12.977
Fox, M. D., Buckner, R. L., White, M. P., Greicius, M. D., and Pascual-Leone, A. (2012). Efficacy of transcranial magnetic stimulation targets for depression is related to intrinsic functional connectivity with the subgenual cingulate. Biol. Psychiatry 72, 595–603. doi: 10.1016/j.biopsych.2012.04.028
Freitas, C., Mondragón-Llorca, H., and Pascual-Leone, A. (2011). Noninvasive brain stimulation in Alzheimer's disease: systematic review and perspectives for the future. Exp. Gerontol. 46, 611–627. doi: 10.1016/j.exger.2011.04.001
Galasko, D., Abramson, I., Corey-Bloom, J., and Thal, L. J. (1993). Repeated exposure to the Mini-Mental State Examination and the Information-Memory-Concentration Test results in a practice effect in Alzheimer's disease. Neurology 43, 1559–1563.
Gluud, L. L. (2006). Bias in clinical intervention research. Am. J. Epidemiol. 163, 493–501. doi: 10.1093/aje/kwj069
Greenfield, S., Kravitz, R., Duan, N., and Kaplan, S. H. (2007). Heterogeneity of treatment effects: implications for guidelines, payment, and quality assessment. Am. J. Med. 120, S3–S9. doi: 10.1016/j.amjmed.2007.02.002
Grefkes, C., Nowak, D. A., Wang, L. E., Dafotakis, M., Eickhoff, S. B., and Fink, G. R. (2010). Modulating cortical connectivity in stroke patients by rTMS assessed with fMRI and dynamic causal modeling. Neuroimage 50, 233–242. doi: 10.1016/j.neuroimage.2009.12.029
Gross, A. L., Chu, N., Anderson, L., Glymour, M. M., and Jones, R. N. (2018). Do people with Alzheimer's disease improve with repeated testing? Unpacking the role of content and context in retest effects. Age Ageing 47, 866–871. doi: 10.1093/ageing/afy136
Guse, B., Falkai, P., and Wobrock, T. (2010). Cognitive effects of high-frequency repetitive transcranial magnetic stimulation: a systematic review. J. Neural Transm. 117, 105–122. doi: 10.1007/s00702-009-0333-7
Haffen, E., Chopard, G., Pretalli, J.-B., Magnin, E., Nicolier, M., Monnin, J., et al. (2012). A case report of daily left prefrontal repetitive transcranial magnetic stimulation (rTMS) as an adjunctive treatment for Alzheimer disease. Brain Stimul. 5, 264–266. doi: 10.1016/j.brs.2011.03.003
Hampstead, B. M., and Hartley, J. F. (2015). High-definition tDCS modulates activation during spatial navigation in healthy older adults. Brain Stimul. 8:314. doi: 10.1016/j.brs.2015.01.021
Hanlon, C. A., Canterberry, M., Taylor, J. J., DeVries, W., Li, X., Brown, T. R., et al. (2013). Probing the frontostriatal loops involved in executive and limbic processing via interleaved TMS and functional MRI at two prefrontal locations: a pilot study. PLoS ONE 8:e67917. doi: 10.1371/journal.pone.0067917
Hannesdóttir, K., and Snædal, J. (2002). A study of the Alzheimer's Disease Assessment Scale-Cognitive (ADAS-Cog) in an Icelandic elderly population. Nord. J. Psychiatry 56, 201–206. doi: 10.1080/080394802317607183
Hartling, L., Ospina, M., Liang, Y., Dryden, D. M., Hooton, N., Seida, J. K., et al. (2009). Risk of bias versus quality assessment of randomised controlled trials: cross sectional study. BMJ 339:b4012. doi: 10.1136/bmj.b4012
Hensel, A., Angermeyer, M. C., and Riedel-Heller, S. G. (2007). Measuring cognitive change in older adults: reliable change indices for the Mini-Mental State Examination. J. Neurol. Neurosurg. Psychiatry 78, 1298–1303. doi: 10.1136/jnnp.2006.109074
Higgins, J. P., Savović, J., Page, M. J., Elbers, R. G., and Sterne, J. A. (2019). Assessing risk of bias in a randomized trial,” in Cochrane Handbook for Systematic Reviews of Interventions, eds J. P. T. Higgins, J. Thomas, J. Chandler, M. Cumpston, T. Li, M. J. Page, and V. A. Welch (Chichester, UK: John Wiley & Sons Ltd), 205–228. doi: 10.1002/9781119536604.ch8
Horvath, J. C., Forte, J. D., and Carter, O. (2015). Quantitative review finds no evidence of cognitive effects in healthy populations from single-session transcranial direct current stimulation (tDCS). Brain Stimul. 8, 535–550. doi: 10.1016/j.brs.2015.01.400
Hsu, W.-Y., Ku, Y., Zanto, T. P., and Gazzaley, A. (2015). Effects of noninvasive brain stimulation on cognitive function in healthy aging and Alzheimer's disease: a systematic review and meta-analysis. Neurobiol. Aging 36, 2348–2359. doi: 10.1016/j.neurobiolaging.2015.04.016
Hugo, J., and Ganguli, M. (2014). Dementia and cognitive impairment: epidemiology, diagnosis, and treatment. Clin. Geriatr. Med. 30, 421–442. doi: 10.1016/j.cger.2014.04.001
Jacobson, L., Koslowsky, M., and Lavidor, M. (2012). tDCS polarity effects in motor and cognitive domains: a meta-analytical review. Exp. Brain Res. 216, 1–10. doi: 10.1007/s00221-011-2891-9
Jung, J., Bungert, A., Bowtell, R., and Jackson, S. R. (2016). Vertex stimulation as a control site for transcranial magnetic stimulation: a concurrent TMS/fMRI study. Brain Stimul. 9, 58–64. doi: 10.1016/j.brs.2015.09.008
Kaminski, J. A., Korb, F. M., Villringer, A., and Ott, D. V. M. (2011). Transcranial magnetic stimulation intensities in cognitive paradigms. PLoS ONE 6:e24836. doi: 10.1371/journal.pone.0024836
Keeser, D., Meindl, T., Bor, J., Palm, U., Pogarell, O., Mulert, C., et al. (2011). Prefrontal transcranial direct current stimulation changes connectivity of resting-state networks during fMRI. J. Neurosci. 31, 15284–15293. doi: 10.1523/JNEUROSCI.0542-11.2011
Kessler, S. K., Turkeltaub, P. E., Benson, J. G., and Hamilton, R. H. (2012). Differences in the experience of active and sham transcranial direct current stimulation. Brain Stimul. 5:155. doi: 10.1016/j.brs.2011.02.007
Khedr, E. M., Gamal, N. F. E., El-Fetoh, N. A., Khalifa, H., Ahmed, E. M., Ali, A. M., et al. (2014). A double-blind randomized clinical trial on the efficacy of cortical direct current stimulation for the treatment of Alzheimer's disease. Front. Aging Neurosci. 6:275. doi: 10.3389/fnagi.2014.00275
Koch, G., Bonnì, S., Pellicciari, M. C., Casula, E. P., Mancini, M., Esposito, R., et al. (2018). Transcranial magnetic stimulation of the precuneus enhances memory and neural activity in prodromal Alzheimer's disease. Neuroimage 169, 302–311. doi: 10.1016/j.neuroimage.2017.12.048
Kostoff, R. N. (2010). Expanded information retrieval using full-text searching. J. Inform. Sci. 36, 104–113. doi: 10.1177/0165551509353250
Kueper, J. K., Speechley, M., and Montero-Odasso, M. (2018). The Alzheimer's Disease Assessment Scale–Cognitive Subscale (ADAS-Cog): modifications and responsiveness in pre-dementia populations. A narrative review. J. Alzheimers Dis. 63, 423–444. doi: 10.3233/JAD-170991
Lakens, D., McLatchie, N., Isager, P. M., Scheel, A. M., and Dienes, Z. (2018). Improving inferences about null effects with Bayes factors and equivalence tests. J. Gerontol. B Psychol. 75, 45–57. doi: 10.1093/geronb/gby065
Lange, S., Sauerland, S., Lauterberg, J., and Windeler, J. (2017). The range and scientific value of randomized trials. Dtsch. Ärztebl. Int. 114, 635–640. doi: 10.3238/arztebl.2017.0635
Lee, J., Choi, B. H., Oh, E., Sohn, E. H., and Lee, A. Y. (2016). Treatment of Alzheimer's disease with repetitive transcranial magnetic stimulation combined with cognitive training: a prospective, randomized, double-blind, placebo-controlled study. J. Clin. Neurol. 12, 57–64. doi: 10.3988/jcn.2016.12.1.57
Lu, M., and Ueno, S. (2017). Comparison of the induced fields using different coil configurations during deep transcranial magnetic stimulation. PLoS ONE 12:e0178422. doi: 10.1371/journal.pone.0178422
MacPherson, H., Altman, D. G., Hammerschlag, R., Youping, L., Taixiang, W., White, A., et al. (2010). Revised Standards for Reporting Interventions in Clinical Trials of Acupuncture (STRICTA): extending the CONSORT statement. PLoS Med. 7:e1000261. doi: 10.1371/journal.pmed.1000261
Maeda, F., Keenan, J. P., Tormos, J. M., Topka, H., and Pascual-Leone, A. (2000). Modulation of corticospinal excitability by repetitive transcranial magnetic stimulation. Clin. Neurophysiol. 111, 800–805. doi: 10.1016/s1388-2457(99)00323-5
Marceglia, S., Mrakic-Sposta, S., Rosa, M., Ferrucci, R., Mameli, F., Vergari, M., et al. (2016). Transcranial direct current stimulation modulates cortical neuronal activity in Alzheimer's disease. Front. Neurosci. 10:134. doi: 10.3389/fnins.2016.00134
Meinzer, M., Lindenberg, R., Phan, M. T., Ulm, L., Volk, C., and Flöel, A. (2015). Transcranial direct current stimulation in mild cognitive impairment: behavioral effects and neural mechanisms. Alzheimers Dement. 11, 1032–1040. doi: 10.1016/j.jalz.2014.07.159
Mennemeier, M., Triggs, W., Chelette, K., Woods, A., Kimbrell, T., and Dornhoffer, J. (2009). Sham transcranial magnetic stimulation using electrical stimulation of the scalp. Brain Stimul. 2, 168–173. doi: 10.1016/j.brs.2009.02.002
Murugaraja, V., Shivakumar, V., Sivakumar, P. T., Sinha, P., and Venkatasubramanian, G. (2017). Clinical utility and tolerability of transcranial direct current stimulation in mild cognitive impairment. Asian J. Psychiatry 30, 135–140. doi: 10.1016/j.ajp.2017.09.001
Nahas, Z., Lomarev, M., Roberts, D. R., Shastri, A., Lorberbaum, J. P., Teneback, C., et al. (2001). Unilateral left prefrontal transcranial magnetic stimulation (TMS) produces intensity-dependent bilateral effects as measured by interleaved BOLD fMRI. Biol. Psychiatry 50, 712–720. doi: 10.1016/S0006-3223(01)01199-4
Nguyen, J.-P., Suarez, A., Kemoun, G., Meignier, M., Le Saout, E., Damier, P., et al. (2017). Repetitive transcranial magnetic stimulation combined with cognitive training for the treatment of Alzheimer's disease. Neurophysiol. Clin. 47, 47–53. doi: 10.1016/j.neucli.2017.01.001
Noetscher, G. M., Yanamadala, J., Makarov, S. N., and Pascual-Leone, A. (2014). Comparison of cephalic and extracephalic montages for transcranial direct current stimulation—a numerical study. IEEE Trans. Biomed. Eng. 61, 2488–2498. doi: 10.1109/TBME.2014.2322774
Padala, P. R., Padala, K. P., Lensing, S. Y., Jackson, A. N., Hunter, C. R., Parkes, C. M., et al. (2018). Repetitive transcranial magnetic stimulation for apathy in mild cognitive impairment: a double-blind, randomized, sham-controlled, cross-over pilot study. Psychiatry Res. 261, 312–318. doi: 10.1016/j.psychres.2017.12.063
Penolazzi, B., Bergamaschi, S., Pastore, M., Villani, D., Sartori, G., and Mondini, S. (2015). Transcranial direct current stimulation and cognitive training in the rehabilitation of Alzheimer disease: a case study. Neuropsychol. Rehabil. 25, 799–817. doi: 10.1080/09602011.2014.977301
Pessoa, L. (2014). Understanding brain networks and brain organization. Phys. Life Rev. 11, 400–435. doi: 10.1016/j.plrev.2014.03.005
Petersen, R. C., Smith, G. E., Waring, S. C., Ivnik, R. J., Tangalos, E. G., and Kokmen, E. (1999). Mild cognitive impairment: clinical characterization and outcome. Arch. Neurol. 56, 303–308.
Philipps, V., Amieva, H., Andrieu, S., Dufouil, C., Berr, C., Dartigues, J.-F., et al. (2014). Normalized Mini-Mental State Examination for assessing cognitive change in population-based brain aging studies. Neuroepidemiology 43, 15–25. doi: 10.1159/000365637
Polanía, R., Nitsche, M. A., and Ruff, C. C. (2018). Studying and modifying brain function with non-invasive brain stimulation. Nat. Neurosci. 21:174. doi: 10.1038/s41593-017-0054-4
Portet, F., Ousset, P. J., Visser, P. J., Frisoni, G. B., Nobili, F., Scheltens, P., et al. (2006). Mild cognitive impairment (MCI) in medical practice: a critical review of the concept and new diagnostic procedure. Report of the MCI Working Group of the European Consortium on Alzheimer's Disease. J. Neurol. Neurosurg. Psychiatry 77, 714–718. doi: 10.1136/jnnp.2005.085332
Rabey, J. M., and Dobronevsky, E. (2016). Repetitive transcranial magnetic stimulation (rTMS) combined with cognitive training is a safe and effective modality for the treatment of Alzheimer's disease: clinical experience. J. Neural Transm. 123, 1449–1455. doi: 10.1007/s00702-016-1606-6
Rabey, J. M., Dobronevsky, E., Aichenbaum, S., Gonen, O., Marton, R. G., and Khaigrekht, M. (2013). Repetitive transcranial magnetic stimulation combined with cognitive training is a safe and effective modality for the treatment of Alzheimer's disease: a randomized, double-blind study. J. Neural Transm. 120, 813–819. doi: 10.1007/s00702-012-0902-z
Rockwood, K., Fay, S., Gorman, M., Carver, D., and Graham, J. E. (2007). The clinical meaningfulness of ADAS-Cog changes in Alzheimer's disease patients treated with donepezil in an open-label trial. BMC Neurol. 7:26. doi: 10.1186/1471-2377-7-26
Rog, L. A., Park, L. Q., Harvey, D. J., Huang, C.-J., Mackin, S., and Farias, S. T. (2014). The independent contributions of cognitive impairment and neuropsychiatric symptoms to everyday function in older adults. Clin. Neuropsychol. 28, 215–236. doi: 10.1080/13854046.2013.876101
Ruan, Q., D'Onofrio, G., Sancarlo, D., Bao, Z., Greco, A., and Yu, Z. (2016). Potential neuroimaging biomarkers of pathologic brain changes in Mild Cognitive Impairment and Alzheimer's disease: a systematic review. BMC Geriatr. 16:104. doi: 10.1186/s12877-016-0281-7
Rutherford, G., Lithgow, B., and Moussavi, Z. (2015). Short and long-term effects of rTMS treatment on Alzheimer's disease at different Stages: a Pilot Study. J. Exp. Neurosci. 9, 43–51. doi: 10.4137/JEN.S24004
Savović, J., Jones, H. E., Altman, D. G., Harris, R. J., Jüni, P., Pildal, J., et al. (2012). Influence of reported study design characteristics on intervention effect estimates from randomized, controlled trials. Ann. Intern. Med. 157, 429. doi: 10.7326/0003-4819-157-6-201209180-00537
Schardt, C., Adams, M. B., Owens, T., Keitz, S., and Fontelo, P. (2007). Utilization of the PICO framework to improve searching PubMed for clinical questions. BMC Med. 7:16. doi: 10.1186/1472-6947-7-16
Schulz, K. F., Altman, D. G., Moher, D., and the CONSORT Group (2010). CONSORT 2010 Statement: updated guidelines for reporting parallel group randomised trials. BMC Med. 8:18. doi: 10.1186/1741-7015-8-18
Sheehan, B. (2012). Assessment scales in dementia. Ther. Adv. Neurol. Disord. 5, 349–358. doi: 10.1177/1756285612455733
Siebner, H. R., Hartwigsen, G., Kassuba, T., and Rothwell, J. (2009). How does transcranial magnetic stimulation modify neuronal activity in the brain? - Implications for studies of cognition. Cortex 45, 1035–1042. doi: 10.1016/j.cortex.2009.02.007
Smeding, H. M., and de Koning, I. (2000). Frontotemporal dementia and neuropsychology: the value of missing values. J. Neurol. Neurosurg. Psychiatry 68, 726–730. doi: 10.1136/jnnp.68.6.726
Smits, L. L., Harten, A. C., van Pijnenburg, Y. L., Koedam, E. L. G. E., Bouwman, F. H., Sistermans, N., et al. (2015). Trajectories of cognitive decline in different types of dementia. Psychol. Med. 45, 1051–1059. doi: 10.1017/S0033291714002153
Sole-Padulles, C., Bartres-Faz, D., Junque, C., Clemente, I. C., Molinuevo, J. L., Bargallo, N., et al. (2006). Repetitive transcranial magnetic stimulation effects on brain function and cognition among elders with memory dysfunction. A randomized sham-controlled study. Cereb. Cortex 16, 1487–1493. doi: 10.1093/cercor/bhj083
Sparing, R., and Mottaghy, F. M. (2008). Noninvasive brain stimulation with transcranial magnetic or direct current stimulation (TMS/tDCS)-From insights into human memory to therapy of its dysfunction. Methods 44, 329–337. doi: 10.1016/j.ymeth.2007.02.001
Sparing, R., Thimm, M., Hesse, M. D., Küst, J., Karbe, H., and Fink, G. R. (2009). Bidirectional alterations of interhemispheric parietal balance by non-invasive cortical stimulation. Brain J. Neurol. 132, 3011–3020. doi: 10.1093/brain/awp154
Sterne, J. A. C., Savović, J., Page, M. J., Elbers, R. G., Blencowe, N. S., Boutron, I., et al. (2019). RoB 2: a revised tool for assessing risk of bias in randomised trials. BMJ 366:l4898. doi: 10.1136/bmj.l4898
Suemoto, C. K., Apolinario, D., Nakamura-Palacios, E. M., Lopes, L., Leite, R. E. P., Sales, M. C., et al. (2014). Effects of a non-focal plasticity protocol on apathy in moderate Alzheimer's disease: a randomized, double-blind, sham-controlled trial. Brain Stimul. 7, 308–313. doi: 10.1016/j.brs.2013.10.003
Thomson, R. H., Maller, J. J., Daskalakis, Z. J., and Fitzgerald, P. B. (2011). Blood oxygenation changes resulting from suprathreshold transcranial magnetic stimulation. Brain Stimul. 4, 165–168. doi: 10.1016/j.brs.2010.10.003
Thomson, R. H., Rogasch, N. C., Maller, J. J., Daskalakis, Z. J., and Fitzgerald, P. B. (2012). Intensity dependent repetitive transcranial magnetic stimulation modulation of blood oxygenation. J. Affect. Disord. 136, 1243–1246. doi: 10.1016/j.jad.2011.08.005
To, W. T., De Ridder, D., Hart, J. Jr., and Vanneste, S. (2018). Changing brain networks through non-invasive neuromodulation. Front. Hum. Neurosci. 12:128. doi: 10.3389/fnhum.2018.00128
Toepper, M. (2017). Dissociating normal aging from Alzheimer's disease: a view from cognitive neuroscience. J. Alzheimers Dis. 57, 331–352. doi: 10.3233/JAD-161099
Turi, Z., Csifcsák, G., Boayue, N. M., Aslaksen, P., Antal, A., Paulus, W., et al. (2019). Blinding is compromised for transcranial direct current stimulation at 1 mA for 20 min in young healthy adults. Eur. J. Neurosci. 50, 3261–3268. doi: 10.1111/ejn.14403
Turriziani, P., Smirni, R., Zappalá, G., Mangano, G. R., Oliveri, M., and Cipolotti, L. (2012). Enhancing memory performance with rTMS in healthy subjects and individuals with Mild Cognitive Impairment: the role of the right dorsolateral prefrontal cortex. Front. Hum. Neurosci. 6:62. doi: 10.3389/fnhum.2012.00062
Turski, C. A., Kessler-Jones, A., Chow, C., Hermann, B., Hsu, D., Jones, J., et al. (2017). Extended Multiple-Field High-Definition transcranial direct current stimulation (HD-tDCS) is well tolerated and safe in healthy adults. Restor. Neurol. Neurosci. 35, 631–642. doi: 10.3233/RNN-170757
Vacas, S. M., Stella, F., Loureiro, J. C., Simões do Couto, F., Oliveira-Maia, A. J., and Forlenza, O. V. (2019). Noninvasive brain stimulation for behavioural and psychological symptoms of dementia: a systematic review and meta-analysis. Int. J. Geriatr. Psychiatry 34, 1336–1345. doi: 10.1002/gps.5003
van der Linde, R. M., Dening, T., Stephan, B. C. M., Prina, A. M., Evans, E., and Brayne, C. (2016). Longitudinal course of behavioural and psychological symptoms of dementia: systematic review. Br. J. Psychiatry 209, 366–377. doi: 10.1192/bjp.bp.114.148403
Vékony, T., Németh, V. L., Holczer, A., Kocsis, K., Kincses, Z. T., Vécsei, L., et al. (2018). Continuous theta-burst stimulation over the dorsolateral prefrontal cortex inhibits improvement on a working memory task. Sci. Rep. 8:14835. doi: 10.1038/s41598-018-33187-3
Verma, M., and Howard, R. J. (2012). Semantic memory and language dysfunction in early Alzheimer's disease: a review. Int. J. Geriatr. Psychiatry 27, 1209–1217. doi: 10.1002/gps.3766
Wallace, D., Cooper, N. R., Paulmann, S., Fitzgerald, P. B., and Russo, R. (2016). Perceived comfort and blinding efficacy in randomised sham-controlled transcranial direct current stimulation (tDCS) trials at 2 mA in young and older healthy adults. PLoS ONE 11:e0149703. doi: 10.1371/journal.pone.0149703
Weuve, J., Proust-Lima, C., Power, M. C., Gross, A. L., Hofer, S. M., Thiébaut, R., et al. (2015). Guidelines for reporting methodological challenges and evaluating potential bias in dementia research. Alzheimers Dement. 11, 1098–1109. doi: 10.1016/j.jalz.2015.06.1885
Wood, L., Egger, M., Gluud, L. L., Schulz, K. F., Jüni, P., Altman, D. G., et al. (2008). Empirical evidence of bias in treatment effect estimates in controlled trials with different interventions and outcomes: meta-epidemiological study. BMJ 336, 601–605. doi: 10.1136/bmj.39465.451748.AD
Wu, Y., Xu, W., Liu, X., Xu, Q., Tang, L., and Wu, S. (2015). Adjunctive treatment with high frequency repetitive transcranial magnetic stimulation for the behavioral and psychological symptoms of patients with Alzheimer's disease: a randomized, double-blind, sham-controlled study. Arch. Psychiatry 27, 280–288. doi: 10.11919/j.issn.1002-0829.215107
Zafar, N., Paulus, W., and Sommer, M. (2008). Comparative assessment of best conventional with best theta burst repetitive transcranial magnetic stimulation protocols on human motor cortex excitability. Clin. Neurophysiol. 119, 1393–1399. doi: 10.1016/j.clinph.2008.02.006
Keywords: Alzheimer's disease, mild cognitive impairment, research methodology, transcranial magnetic stimulation, transcranial direct current stimulation
Citation: Holczer A, Németh VL, Vékony T, Vécsei L, Klivényi P and Must A (2020) Non-invasive Brain Stimulation in Alzheimer's Disease and Mild Cognitive Impairment—A State-of-the-Art Review on Methodological Characteristics and Stimulation Parameters. Front. Hum. Neurosci. 14:179. doi: 10.3389/fnhum.2020.00179
Received: 20 January 2020; Accepted: 21 April 2020;
Published: 25 May 2020.
Edited by:
Raffaella Franciotti, Università degli Studi G. d'Annunzio Chieti e Pescara, ItalyReviewed by:
Ravi L. Hadimani, Virginia Commonwealth University, United StatesUlrich Palm, Medical Park Chiemseeblick, Germany
Copyright © 2020 Holczer, Németh, Vékony, Vécsei, Klivényi and Must. This is an open-access article distributed under the terms of the Creative Commons Attribution License (CC BY). The use, distribution or reproduction in other forums is permitted, provided the original author(s) and the copyright owner(s) are credited and that the original publication in this journal is cited, in accordance with accepted academic practice. No use, distribution or reproduction is permitted which does not comply with these terms.
*Correspondence: Adrienn Holczer, holczer.adrienn@med.u-szeged.hu; Anita Must, must.anita@med.u-szeged.hu