- 1Department of Rehabilitation Sciences, The Hong Kong Polytechnic University, Kowloon, Hong Kong
- 2University Research Facility in Behavioral and Systems Neuroscience, The Hong Kong Polytechnic University, Kowloon, Hong Kong
- 3School of Medical and Health Sciences, Tung Wah College, Kowloon, Hong Kong
- 4Department of Occupational Therapy, Monash University, Melbourne, VIC, Australia
Background: Transcranial direct-current stimulation (tDCS) facilitates cognitive improvement in healthy and pathological populations. It has been increasingly used in cases of mild cognitive impairment (MCI) and dementia. Our research question is: Can tDCS serve as a clinical intervention for improving the cognitive functions of persons with MCI (PwMCI) and dementia (PwD)?
Objective: This systematic review evaluated the evidence to determine the efficacy of tDCS in improving cognitive outcomes in PwD and PwMCI.
Methods: A systematic review was conducted of studies published up to November 2017 involving tDCS in cases of MCI and dementia. Studies were ranked according to the level of evidence (Oxford Center for Evidence-Based Medicine) and assessed for methodological quality (Risk of Bias Tool in the Cochrane Handbook for Systematic Reviews of Interventions). Data was extracted on all protocol variables to establish a reference framework for clinical interventions. Different modalities, tDCS alone or combined with cognitive training, compared with sham tDCS were examined in both short and long-term effects. Four randomized control trials (RCTs) with memory outcomes were pooled using the fixed-effect model for the meta-analysis.
Results: Twelve studies with 195 PwD and four with 53 PwMCI met the inclusion criteria. Eleven articles were ranked as Level 1b. The results on the meta-analysis on pooled effects of memory indicated a statistically significant medium effect size of 0.39 (p = 0.04) for immediate effects. This improvement was not maintained in the long term 0.15 (p = 0.44).
Conclusion: tDCS improves memory in PwD in the short term, it also seems to have a mild positive effect on memory and language in PwMCI. However, there is no conclusive advantage in coupling tDCS with cognitive training. More rigorous evidence is needed to establish whether tDCS can serve as an evidence-based intervention for both populations.
Introduction
Transcranial direct-current stimulation (tDCS) is a type of non-invasive brain stimulation (NIBS). tDCS delivers weak direct currents to the brain that can alter spontaneous firing rates on neural activity, which subsequently translates into behavioral changes (Nitsche et al., 2008). It is a process that has been described as “portable, painless, inexpensive and safe” (Kadosh et al., 2012). During the administration of tDCS, depolarization or hyperpolarization of the neuronal membrane of target neurons may be induced, even though the small electric fields of tDCS are considered to be below the intensity required to evoke action potentials (Nitsche et al., 2003; Miniussi et al., 2013; Tatti et al., 2016). In other words, tDCS causes a shift in the membrane potential threshold which is likely to change the probability that an incoming action potential will result in post-synaptic firing during and after its administration (Prehn and Flöel, 2015). Such changes in neuronal excitability modulates the cognitive processes and tDCS can induce physiological processes. Due to the proposed resemblance of the effects of tDCS and cognitive processes on cerebral physiology, researchers have been using NIBS to alter cognition (Kuo and Nitsche, 2012; Prehn and Flöel, 2015).
Mild Cognitive Impairment (MCI) is defined as the stage between normal and dementia-type pathological aging. MCI is a syndrome of cognitive decline in non-demented persons that does not affect the capacity to be independent in activities of daily living (ADLs; Portet et al., 2006). In contrast, people who suffer from dementia present a more severe cognitive decline and do not preserve independence in functional abilities and ADLs (Langa and Levine, 2014). Epidemiological investigations suggest a range of prevalence for MCI of 7–24% among adults aged over 65, and the manifestation of MCI is consistently shown to have a high risk of progression to dementia (Langa and Levine, 2014; Petersen et al., 2014). To date, there is no pharmaceutical treatment shown to be effective in improving cognitive functioning in MCI and dementia (Langa and Levine, 2014), although cognitive training interventions show promise for improving targeted cognitive functions in elderly persons without cognitive impairments (Ball et al., 2002). Cognitive Rehabilitation (CR) is defined as “the therapeutic process of increasing or improving an individual's capacity to process and use incoming information so as to allow increased functioning in everyday life.” This includes methods to train and restore cognitive functioning as well as compensatory techniques (Sohlberg and Mateer, 1989, p. 871).
CR is therefore essential and research has indicated that NIBS can positively affect the cognitive performance of populations affected by cognitive disorders (Miniussi et al., 2008). Differences in tDCS experimental protocols regarding the parameters employed such as the montage, the current, the intensity or the size of the electrodes can affect the electric field strength. All of these variables contribute to increase the heterogeneity of the electric field's properties among studies thus producing different outcomes (Woods et al., 2016). Furthermore, targeting a neural network with tDCS while it is engaged by a cognitive stimulation activity, during or after the administration of tDCS, may yield better therapeutic effects than stimulating the same cortical region lacking cognitive stimuli (Cruz Gonzalez et al., 2018). tDCS may increase the strength of transmission across synaptic circuits in pathways that are stimulated by cognitive practice. Thus, coupling both techniques could create a synergistic positive effect on behavior (Miniussi et al., 2013; Birba et al., 2017; Cruz Gonzalez et al., 2018). The effectiveness of tDCS in CR targeting people with MCI or dementia must therefore be established. It is fundamentally important to learn about all the different configurations and protocols in which tDCS has been employed to assess its utility.
We systematically reviewed the literature regarding effects of tDCS on persons with MCI and dementia to address the following questions: (1) Does tDCS alone improve cognitive functioning in persons with MCI and dementia? (2) Does tDCS coupled with cognitive training, or as a priming to other cognitive interventions yield greater benefits in cognitive functioning than the administration of tDCS alone? (3) Are the effects of tDCS on the cognitive functions able to maintain across time?
In this study, we reviewed and evaluated the effects of tDCS on cognitive functions in people with MCI or dementia from all the available clinical trials. A systematic review of the available information up to the present will enable researchers to better understand the potential of tDCS to offer solutions for cognitive deterioration, with the aim of outlining more robust interventions in the future for people with MCI and dementia. Other reviews involving the use of different NIBS on healthy aging (Prehn and Flöel, 2015), dementia (Freitas et al., 2011; Hsu et al., 2015), MCI (Birba et al., 2017) have been carried out since 2011, but we provide an update and meta-analysis of recent trials to focus exclusively on the use of tDCS in MCI and dementia populations.
Methods
Eligibility Criteria
We performed a systematic review and meta-analysis following the PRISMA guidelines (Liberati et al., 2009). Studies were selected based on the following criteria:
- Participants: Participants included in the study were older adults with MCI and persons with a diagnosis of dementia. The criteria for MCI includes (a) subjective memory complaint; (b) objective cognitive decline; (c) preserved ADLs, and (d) not demented (Petersen et al., 1999). The diagnosis of dementia followed the criteria of the NINCDS-ADRDA (McKhann et al., 1984) and the DSM-IV (American Psychiatric Association, 2000). Participants with any other neurological disease that was not dementia, such as only the Parkinson's type, were excluded.
- Interventions: tDCS alone (anodal, cathodal, or sham), or a combination of tDCS (online or offline) with an additional cognitive task (CT).
- Comparisons: The comparison group could be a placebo with sham tDCS, sham tDCS in combination with a CT, or a control group performing a cognitive intervention. In order to establish evidence on tDCS protocols for people with MCI or dementia, studies without sham tDCS were included.
- Outcome measurements: The outcomes were measurements of cognitive functions and neuroimaging techniques.
- Study design: All clinical trials published in English from January 2007 to November 2017 were included.
Search Strategy
Studies were identified by a systematic literature search in the following databases: PubMed, Web of Science, Science Direct, MEDLINE, and PsycINFO. A search was performed combining all the chosen keywords across the above databases. The keywords and the search strategy are presented in Table 1. A hand search was also performed to identify relevant studies.
Selection Criteria
After removing duplicates, the abstracts of the articles retrieved were screened to make a final decision for further review. Two investigators realized the search and the selection of studies to be included. Any disagreements were resolved by a third reviewer.
Data Extraction
The data extracted from the selected studies were conducted by two investigators using a standardized data extraction sheet which included study design, study population, number of participants, mean participant age, gender ratio, general cognitive level, number of intervention sessions, experimental/sham tDCS parameters, combination of tDCS with other interventions, outcome measures, neuroimaging techniques, assessment sequence, follow-up, effect(s) of the intervention, and intervention safety reports.
Methodological Quality
The studies selected for review were categorized and leveled according to their design based on the hierarchy level of evidence [Oxford Center for Evidence-based Medicine—Levels of Evidence (March 2009)—CEBM1]. All randomized control trials (RCTs) were then rated by the first two authors using the Risk of Bias Tool in the Cochrane Handbook for Systematic Reviews of Interventions (Higgins and Green, 2008).
Data Analysis
Only RCTs, excluding crossover designs, were considered for meta-analysis. In some cases, authors were contacted to obtain data from their studies. After the review of the clinical methodology's heterogeneity of each study (Table 2), the selected papers were further assessed for statistical heterogeneity, using the I-squared and Chi-squared statistics of the outcome measures.
Data of pooled memory outcomes comparing: (1) Short-term effects of tDCS treatments vs. sham tDCS that targeted the dorsolateral prefrontal cortex (DLPFC) were calculated based on the differences between post-intervention evaluations relative to the baseline to assess the immediate effects of tDCS; (2) Long-term effects of tDCS treatments vs. sham tDCS that targeted the DLPFC; were assessed according to the differences between follow-up evaluations relative to the baseline.
All outcomes were analyzed as continuous variables with the mean change, the largest standard deviation, and the sample size in each group. The standardized mean difference and 95% confidence intervals were calculated for all meta-analyses using the fixed-effect model. The effect size was considered to be small between 0.2–0.49, moderate (0.5–0.79), and a value of 0.8 or above was considered to be large (Cohen, 1992). If I2 was below 40%, it was considered to not represent statistical heterogeneity. Otherwise, the random-effect model was used instead. Significance was set at p = 0.05 and both meta-analyses were conducted using Review Manager Software 5.3.
Results
Study Selection
The search strategy identified 1,198 published articles from the selected databases: PubMed (n = 434), Web of Science (n = 317), Science Direct (n = 31), Medline (n = 235), and PsycINFO (n = 181) (Table 1). Sixteen articles met the eligibility criteria (Figure 1).
Study Characteristics
Eleven studies (Ferrucci et al., 2008; Boggio et al., 2009, 2012; Cotelli et al., 2014; Khedr et al., 2014; Suemoto et al., 2014; Penolazzi et al., 2015; André et al., 2016; Bystad et al., 2016a,b, 2017; Costa et al., 2017) involved the application of tDCS on persons with dementia (PwD). These articles included three randomized crossover studies (Ferrucci et al., 2008; Boggio et al., 2009, 2012), five RCTs (Cotelli et al., 2014; Khedr et al., 2014; Suemoto et al., 2014; André et al., 2016; Bystad et al., 2016a), two single-subject pretest-post-test case studies (Bystad et al., 2016b, 2017), and two single-subject crossover-design studies (Penolazzi et al., 2015; Costa et al., 2017). Four articles (Meinzer et al., 2015; Yun et al., 2016; Ladenbauer et al., 2017; Murugaraja et al., 2017) exposed persons with MCI (PwMCI) to the application of tDCS. These four studies each used a different design: a randomized crossover (Meinzer et al., 2015), an RCT (Yun et al., 2016), a group pretest-post-test (Murugaraja et al., 2017), and a balanced crossover (Ladenbauer et al., 2017).
These studies included a total of 195 participants with dementia and 53 participants with MCI. Eleven studies applied tDCS “alone” (Ferrucci et al., 2008; Boggio et al., 2012; Khedr et al., 2014; Suemoto et al., 2014; André et al., 2016; Bystad et al., 2016a,b, 2017; Yun et al., 2016; Ladenbauer et al., 2017; Murugaraja et al., 2017) and five paired tDCS with CT (Boggio et al., 2009; Cotelli et al., 2014; Meinzer et al., 2015; Penolazzi et al., 2015; Costa et al., 2017). The details of the studies' characteristics and protocols are set out in Table 3.
tDCS Parameters
Two studies randomly assigned participants to anodal, cathodal, and sham groups (Ferrucci et al., 2008; Khedr et al., 2014). The majority of the studies involved anodal and sham groups (Boggio et al., 2009, 2012; Cotelli et al., 2014; Suemoto et al., 2014; Meinzer et al., 2015; Penolazzi et al., 2015; André et al., 2016; Bystad et al., 2016a; Yun et al., 2016; Costa et al., 2017; Ladenbauer et al., 2017; Murugaraja et al., 2017). In contrast, three studies focused on anodal stimulation lacking sham tDCS (Bystad et al., 2016b, 2017; Murugaraja et al., 2017). Regarding the dose, we found a high level of heterogeneity among experiments. Only four studies were single-session (Ferrucci et al., 2008; Boggio et al., 2009; Meinzer et al., 2015; Ladenbauer et al., 2017) whereas the number of sessions for the rest of studies ranged from 4 to 10 (Cotelli et al., 2014; Khedr et al., 2014; Suemoto et al., 2014; Penolazzi et al., 2015; André et al., 2016; Bystad et al., 2016a; Yun et al., 2016). Bystad carried out two case studies adopting unusual approaches, the first study with a daily dose of tDCS for a duration of 8 months (Bystad et al., 2017) and the second study using tDCS twice daily consecutively for 6 days (Bystad et al., 2016b). With respect to the electric fields, more homogeneous parameters were chosen among studies. The majority of the studies applied 2 mA of intensity and the targeted region for the active electrode was the DLFPC and the right supraorbital region for the cathode (Figure 2).
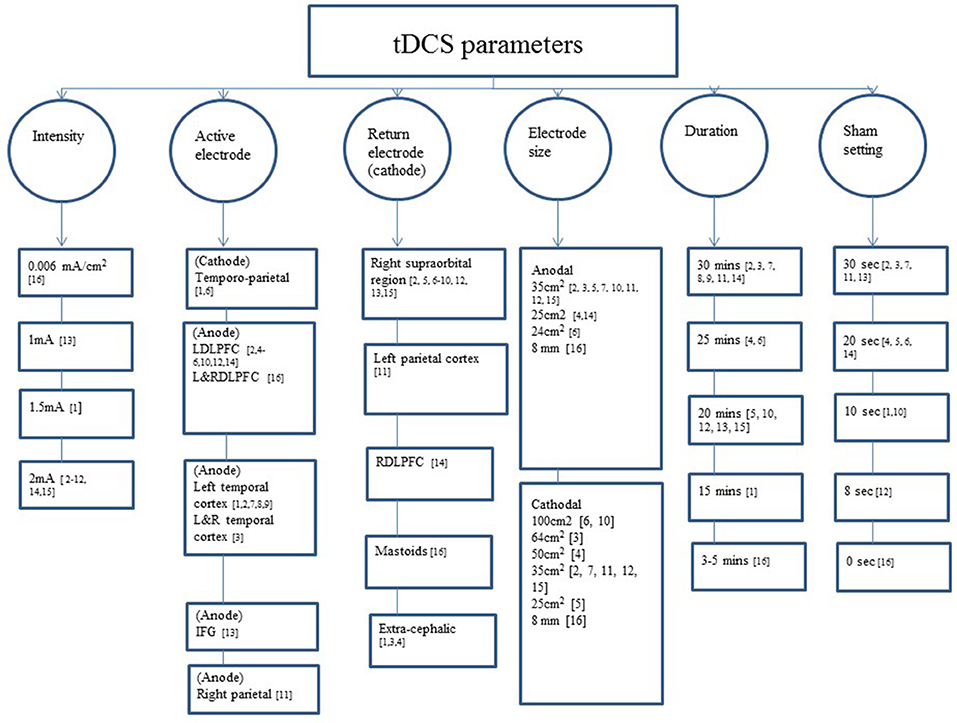
Figure 2. tDCS parameters used across the studies included. IFG: 1, (Ferrucci et al., 2008); 2, (Boggio et al., 2009); 3, (Boggio et al., 2012); 4, (Cotelli et al., 2014); 5, (Suemoto et al., 2014); 6, (Khedr et al., 2014); 7, (Bystad et al., 2016a); 8, (Bystad et al., 2016b); 9, (Bystad et al., 2017); 10, (Penolazzi et al., 2015); 11, (Costa et al., 2017); 12, (André et al., 2016); 13, (Meinzer et al., 2015); 14, (Yun et al., 2016); 15, (Murugaraja et al., 2017); 16, (Ladenbauer et al., 2017); IFG, inferior frontal gyrus; L/DLPFCT, left/right dorsolateral prefrontal cortex; L&R, left and right.
Six studies reported mild adverse reactions such as itchy and tingling sensations, redness in the area of electrode application, burning scalp, headache, dizziness, and pricking (Ferrucci et al., 2008; Khedr et al., 2014; Suemoto et al., 2014; Bystad et al., 2017; Ladenbauer et al., 2017; Murugaraja et al., 2017).
Effectiveness of tDCS “Alone”
Seven studies on the dementia population reported positive effects of anodal (Ferrucci et al., 2008; Boggio et al., 2012; Khedr et al., 2014; André et al., 2016; Bystad et al., 2016b, 2017) and cathodal tDCS (Khedr et al., 2014) on cognition. All these cognitive improvements were associated with memory and global cognition. All outcomes but two (Boggio et al., 2012; Bystad et al., 2017) were statistically significant. However, two of these studies failed to report positive effects in the attention domain (Ferrucci et al., 2008; Boggio et al., 2012). Two others did not report any positive effects of anodal tDCS on cognition (Suemoto et al., 2014; Bystad et al., 2016a).
Four studies (Boggio et al., 2012; Cotelli et al., 2014; Khedr et al., 2014; Bystad et al., 2016b) assessed the long-term effects of tDCS. Three of these reported significant changes: one showed that the improvement caused by anodal tDCS persisted 4 weeks after the end of stimulation (Boggio et al., 2012), another indicated that either anodal or cathodal tDCS improved mean MMSE score at 1- and 2-month follow-up (Khedr et al., 2014), and the third study revealed that 2 months after the end of the intervention, anodal tDCS was clinically significant (Bystad et al., 2016b).
Only two studies performed neuroimaging tests. In the first, an ERP experiment confirmed significant effects reducing P300 latency after both anodal and cathodal tDCS (Khedr et al., 2014). The second used EEG, although it did not prove changes from baseline (Bystad et al., 2016b).
Three studies evaluated the efficacy of anodal tDCS on PwMCI. Overall, anodal tDCS achieved significant improvement in memory (Yun et al., 2016; Murugaraja et al., 2017). Furthermore, two of these studies investigated the neural effects of anodal tDCS. Yun et al. (2016) utilized PET to demonstrate a significantly increased metabolism in cortical regions. In the same way, the work of Ladenbauer et al. (2017) made clear, through the use of concurrent EEG, that slow oscillatory tDCS significantly increased overall slow oscillations (SO) and spindle power (Ladenbauer et al., 2017).
Effectiveness of tDCS Combined With CT
Details and methods about the CT operated among studies are shown in Table 3. All the studies involving PwD showed significant benefits after receipt of anodal tDCS paired with a CT. Boggio et al. (2009)applied tDCS while participants completed cognitive assessments, enhancing memory in a visual recognition memory task, but there were no effects on attention. The work of Cotelli et al. (2014) combining memory training with tDCS and sham tDCS resulted in improved memory performance illustrated in a face-name association memory task, as compared to a group which received tDCS paired with motor training; this improvement persisted significantly after 12 weeks. However, it failed to produce significant effects on standardized cognitive tests. In one single-subject crossover study, the cognitive training associated with memory components was started right after the end of tDCS administration and the findings revealed a significant accuracy improvement in a verbal working memory task. In contrast, there is no indication of amelioration in other cognitive assessments (Penolazzi et al., 2015). Alternatively, one case study that focused on stimulating the production and comprehension of language through a combination of anodal tDCS and linguistic training found a significant effect in an auditory comprehension task (Costa et al., 2017).
The work of Meinzer et al. (2015) targeting PwMCI revealed that during exposure to anodal tDCS, participants performed significantly better in a semantic word-retrieval task than those who received sham tDCS, achieving the level of healthy elderly subjects. Furthermore, the application of anodal tDCS led to reduced task-related prefrontal hyperactivity shown by resting-state fMRI.
Details of the CT
Study Quality
The level of evidence of all the trials is displayed in Figure 1. Details can be found in Table 4. Most of the studies reported a risk of bias describing the method used to conceal the allocation sequence (Ferrucci et al., 2008; Boggio et al., 2012; Meinzer et al., 2015; André et al., 2016; Bystad et al., 2016a; Yun et al., 2016; Ladenbauer et al., 2017). The most common methodological limitation of these studies was the issue of the blinding of the personnel due to the nature of most tDCS devices.
Meta-Analysis
Four studies (Cotelli et al., 2014; Khedr et al., 2014; Suemoto et al., 2014; André et al., 2016) involving 119 PwD in total were included in the meta-analysis. One RCT study was excluded because the region of stimulation was the temporal region (Bystad et al., 2016a). The results revealed a statistically significant mean effect size of 0.39 [95% CI, 0.02, 0.74] (p = 0.04) that favored real tDCS over sham stimulation for immediate effects. There was no evidence of heterogeneity across studies (Q = 4.73, I2 = 37%, p = 0.19). An overall small non-significant effect of 0.15 [95% CI, −0.023, 0.52] (p = 0.44) was noted in long-term effects of tDCS in comparison with sham tDCS. Heterogeneity was not found (Q = 2.18, I2 = 0%, p = 0.53; Figure 3).
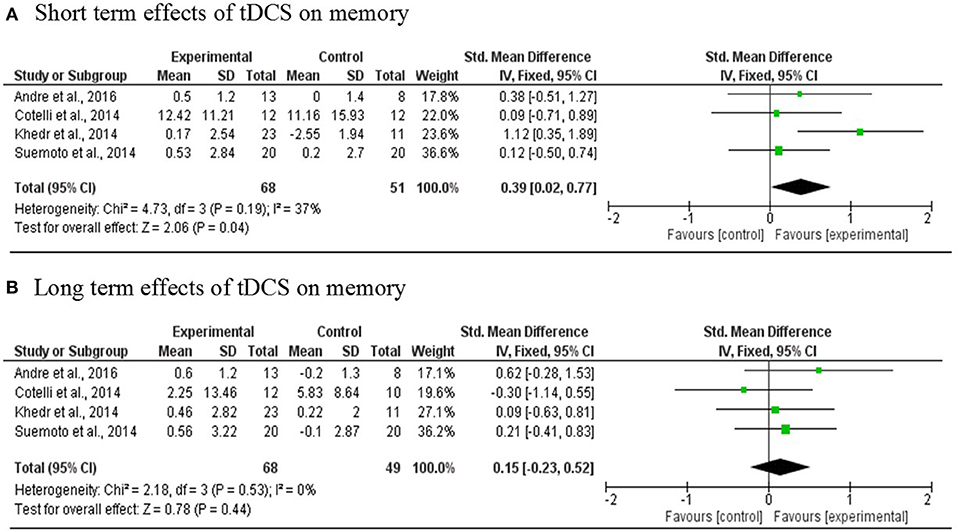
Figure 3. Meta-analyses forest plot. (A) Short term effects of tDCS on memory. Data derived from a fixed effect model. Each line represents an individual effect size of each study. The diamond at the bottom shows the standardized effect size (0.39). Relative weight for each trial is illustrated by the sized of the corresponding square. (B) Long term effects of tDCS on memory. Data derived from a fixed effect model. Each line represents an individual effect size of each study. The diamond at the bottom shows the standardized effect size (0.15). Relative weight for each trial is illustrated by the sized of the corresponding square.
Discussion
All the 11 articles (RCTs) whose evidence was ranked as level 1b presented a commendable methodological quality with a general presence of low risk of bias. From the MMSE admission scores in the AD studies that ranged from 15 to 24.5 and MCI studies from 26.75 to 28.3, we noticed that the effects of tDCS benefits on cognition were significantly better for patients with mild to moderate cognitive decline.
When comparing the effectiveness of tDCS, in single and multisession interventions, positive changes occurred in both behavioral and neural systems. In this systematic review, we aimed to reveal robust interventions by identifying similar elements across studies. One main concern when designing interventions in NIBS is the treatment duration in multisession trials. There is similarity in terms of the number of sessions across the selected studies: four to ten sessions, staggered over 1–2 weeks. These short interventions can provide valuable data that allow tDCS to be proposed as a potential option in CR. However, the benefit is rather short-term with a medium effect size of 0.39. This also contrasts with other long intervention frameworks for clinical use in which more time is needed to evaluate whether the changes have a real benefit in reversible conditions such as MCI (Portet et al., 2006) or have an impact in long-term neurodegenerative processes such as dementia. For example, an alternative was proposed by Bystad et al. (2017) that adopted an 8-month protocol of daily tDCS use in a person with AD to stabilize cognitive decline. The long-term outcome probably requires prolonged periods of intervention.
Although six studies reported side effects (Ferrucci et al., 2008; Khedr et al., 2014; Suemoto et al., 2014; Bystad et al., 2017; Ladenbauer et al., 2017; Murugaraja et al., 2017), all participants tolerated the therapies well and the sensations experienced were mild. This suggests that the parameters employed are sufficiently safe (up to 30 min, 2 mA). Another concern is that the range of the parameters for intensity and duration stimulation and the size of the electrodes were highly diverse, making it difficult to draw conclusions in order to select a specific protocol for future research.
Another view is that when selecting a region of interest for stimulation, most of the studies targeted the temporal regions (Ferrucci et al., 2008; Boggio et al., 2012; Bystad et al., 2016a,b, 2017), for the role this area plays in certain memory processes (Brown et al., 1987; Kaye et al., 1997) as well as language (Nguyen et al., 2018). Another common region of interest is the DLPFC because of its importance in high-order cognitive mechanisms (Tremblay et al., 2014). Language-oriented work has targeted the inferior frontal gyrus and DLPFC as well, successfully achieving better performance in semantic word retrieval (Meinzer et al., 2015) and comprehension of language (Costa et al., 2017). In the same way, studies that applied tDCS combined with CT operated a CT related with a cognitive domain associated with the brain area targeted by tDCS. Although this approach is reasonable and consistent, the studies failed to assess if other cognitive domains associated with other brain regions were affected. Due to the lack of focality of tDCS and the variability of the current flow direction, there is a possibility that other neural networks, not directly targeted by tDCS, could have been affected (Woods et al., 2016).
Three studies used an extracephalic cathodal montage (Ferrucci et al., 2008; Boggio et al., 2012; Cotelli et al., 2014) but the majority of the studies selected a cephalic montage by placing the cathode on the supraorbital region (Fp2) (Boggio et al., 2009; Khedr et al., 2014; Suemoto et al., 2014; Meinzer et al., 2015; Penolazzi et al., 2015; André et al., 2016; Bystad et al., 2016a,b, 2017; Costa et al., 2017; Murugaraja et al., 2017).
Overall, these studies have selected predominantly global cognition and memory domain as experimental evaluators. Despite the fact that these constructs are similar in nature, there is great variability in terms of assessment and CT chosen. All the studies but two (Suemoto et al., 2014; Bystad et al., 2016a) report positive effects of the application of tDCS. Against this trend, among the other articles, we must emphasize that only six studies translated these improvements into standardized cognitive assessments (Ferrucci et al., 2008; Khedr et al., 2014; André et al., 2016; Bystad et al., 2016b; Yun et al., 2016; Ladenbauer et al., 2017) while other studies reporting improvements in non-standardized CT to prove the effects of tDCS. Yet it must be acknowledged that certain cognitive functions are mediated by networks of various brain sites and might be difficult to be influenced by targeting only a subset of their brain regions (Reinhart et al., 2017), besides the short length of the intervention might have contributed to these changes being insufficient to translate into standardized test results.
It is hypothesized that targeting a neural circuit with tDCS paired with a CT may produce stronger therapeutic effects than stimulating the same brain area without cognitive stimuli (Birba et al., 2017; Cruz Gonzalez et al., 2018). The evidence on whether using tDCS alone or in combination with other CT yields identical results and seems to be inconclusive in both PwD or PwMCI. Recently, a single-subject design study using cognitive stimulation practice across sessions in combination with simultaneous anodal tDCS showed significantly stronger effects on planning ability, processing speed, and attention of cognitive stimulation practice than both sham tDCS and the application of cognitive stimulation practice alone in PwMCI (Cruz Gonzalez et al., 2018). This finding prompts the plausible speculation that tDCS, combined with cognitive training, might have synergic effects. A recent review of CR or cognitive training interventions with control conditions for PwD shows that RCTs on the effect of cognitive training on PwD are limited and there is no indication of any significant benefits from cognitive training (Bahar-Fuchs et al., 2013). Following this line of thought, future studies would carry more weight if they considered combining both interventions in comparison with control groups receiving tDCS or cognitive training alone, and would report not just benefits in the trained CT but also generalization to the trained cognitive domains and daily functioning.
Only five studies reported the use of brain imaging as an outcome demonstrating the neuromodulatory effects of tDCS (Khedr et al., 2014; Meinzer et al., 2015; Bystad et al., 2016b; Yun et al., 2016; Ladenbauer et al., 2017). In the absence of imaging techniques, we can only speculate on the results of behavioral tests without examining the underlying neural mechanism of tDCS in MCI or dementia.
This is the first meta-analysis to explore the short- and long-term effects of tDCS in the memory domain, targeting the DLPFC in PwD. We have found evidence that tDCS has a significant immediate effect but that it is not significantly sustained with the passage of time. We suggest that future research address the need to evaluate the long-lasting effects of tDCS on the cognitive domain, implementing both behavioral and imaging follow-up evaluations.
This study has several limitations. For instance, although the pooled outcomes for meta-analysis were all memory-based, the selected studies used different tests. In addition, only four studies could be included, this might contribute to making the meta-analyses somewhat underpowered, thus the findings should be interpreted with cautions. Another striking example is the AD stage, which varied among the studies. Moreover, we have not included the most recent work published since November 2017 (Cruz Gonzalez et al., 2018), because of the time eligibility criteria. This systematic review included all tDCS trials carried out in dementia and MCI populations, and subsequently reported a few papers that did not use a comparison group (sham tDCS), which weakens the conclusions somewhat.
Conclusion
Our meta-analysis suggests that there is modest evidence supporting tDCS on the DLPFC ameliorates memory in PwD, however, the benefits are not long-term. Our review shows that tDCS alone seems to have a positive effect on cognition particularly for memory and language in PwD, with mild to moderate cognitive decline, and MCI. Whether tDCS might produce better outcomes on PwMCI and PwD in coupling with another CT than when administered alone remains unclear.
Although all these findings are promising, the administration of tDCS might not yet be a valid option for clinical intervention for dementia or MCI. Some of the results come from non-RCT studies, and the heterogeneity of the clinical trials does not allow one to define a clear protocol with optimal parameters. Furthermore, the interventions were too short to determine the real effects on cognitive functions and none of the studies assessed the impact of treatments on everyday cognition in daily functioning, which is an essential domain to be considered due to the functional consequences of dementia. We recommend that future studies include prolonged periods of intervention, neuroimaging techniques, and consider more robust, standardized methodology of tDCS in order to establish whether tDCS can serve as an evidence-based clinical intervention for PwMCI and PwD.
Author Contributions
PC and KF designed the study, collected the data, conducted the statistical analysis, and wrote the manuscript. RC supervised the statistical analysis. K-HT and LL provided advice writing the manuscript. TB supervised the design and provided guidance.
Conflict of Interest Statement
The authors declare that the research was conducted in the absence of any commercial or financial relationships that could be construed as a potential conflict of interest.
Footnotes
1. ^1https://www.cebm.net/2009/06/oxford-centre-evidence-based-medicine-levels-evidence-march-2009 (Accessed March 29, 2018).
References
American Psychiatric Association (2000). Diagnostic and Statistical Manual of Mental Disorders, 4th Edn. Washington, DC: American Psychiatric Association.
André, S., Heinrich, S., Kayser, F., Menzler, K., Kesselring, J., Khader, P. H., et al. (2016). At-home tDCS of the left dorsolateral prefrontal cortex improves visual short-term memory in mild vascular dementia. J. Neurol. Sci. 369, 185–190. doi: 10.1016/j.jns.2016.07.065
Bahar-Fuchs, A., Clare, L., and Woods, B. (2013). Cognitive training and cognitive rehabilitation for mild to moderate Alzheimer's disease and vascular dementia. Cochrane Database Syst. Rev. 6:CD003260. doi: 10.1002/14651858.CD003260.pub2
Ball, K., Berch, D. B., Helmers, K. F., Jobe, J. B., Leveck, M. D., Marsiske, M., et al. (2002). Effects of cognitive training interventions with older adults. JAMA 288, 2271–2281. doi: 10.1001/jama.288.18.2271
Birba, A., Ibáñez, A., Sedeño, L., Ferrari, J., García, A. M., and Zimerman, M. (2017). Non-invasive brain stimulation: a new strategy in mild cognitive impairment? Front. Aging Neurosci. 9:16. doi: 10.3389/fnagi.2017.00016
Boggio, P. S., Ferrucci, R., Mameli, F., Martins, D., Martins, O., Vergari, M., et al. (2012). Prolonged visual memory enhancement after direct current stimulation in Alzheimer's disease. Brain Stimul. 5, 223–230. doi: 10.1016/j.brs.2011.06.006
Boggio, P. S., Khoury, L. P., Martins, D. C. S., Martins, O. E. M. S., de Macedo, E. C., and Fregni, F. (2009). Temporal cortex direct current stimulation enhances performance on a visual recognition memory task in Alzheimer disease. J. Neurol. Neurosurg. Psychiatry 80, 444–447. doi: 10.1136/jnnp.2007.141853
Brown, M. W., Wilson, F. A. W., and Riches, I. P. (1987). Neuronal evidence that inferomedial temporal cortex is more important than hippocampus in certain processes underlying recognition memory. Brain Res. 409, 158–162. doi: 10.1016/0006-8993(87)90753-0
Bystad, M., Grønli, O., Rasmussen, I. D., Gundersen, N., Nordvang, L., Wang-Iversen, H., et al. (2016a). Transcranial direct current stimulation as a memory enhancer in patients with Alzheimer's disease: a randomized, placebo-controlled trial. Alzheimer Res. Ther. 8:13. doi: 10.1186/s13195-016-0180-3
Bystad, M., Rasmussen, I. D., Abeler, K., and Aslaksen, P. M. (2016b). Accelerated transcranial direct current stimulation in Alzheimer's disease: a case study. Brain Stimul. 9, 634–635. doi: 10.1016/j.brs.2016.04.018
Bystad, M., Rasmussen, I. D., Grønli, O., and Aslaksen, P. M. (2017). Can 8 months of daily tDCS application slow the cognitive decline in Alzheimer's disease? A case study. Neurocase 23, 146–148. doi: 10.1080/13554794.2017.1325911
Cohen, J. (1992). Statistical power analysis. Curr. Dir. Psychol. Sci. 1, 98–101. doi: 10.1111/1467-8721.ep10768783
Costa, V., Brighina, F., Piccoli, T., Realmuto, S., and Fierro, B. (2017). Anodal transcranial direct current stimulation over the right hemisphere improves auditory comprehension in a case of dementia. NeuroRehabilitation 41, 567–575. doi: 10.3233/NRE-162062
Cotelli, M., Manenti, R., Brambilla, M., Petesi, M., Rosini, S., Ferrari, C., et al. (2014). Anodal tDCS during face-name associations memory training in Alzheimer's patients. Front. Aging Neurosci. 6:38. doi: 10.3389/fnagi.2014.00038
Cruz Gonzalez, P., Fong, K. N. K., and Brown, T. (2018). The effects of transcranial direct current stimulation on the cognitive functions in older adults with mild cognitive impairment: a pilot study. Behav. Neurol. 2018, 1–14. doi: 10.1155/2018/5971385
Ferrucci, R., Mameli, F., Guidi, I., Mrakic-Sposta, S., Vergari, M., Marceglia, S., et al. (2008). Transcranial direct current stimulation improves recognition memory in Alzheimer disease. Neurology 71, 493–498. doi: 10.1212/01.wnl.0000317060.43722.a3
Freitas, C., Mondragón-Llorca, H., and Pascual-Leone, A. (2011). Noninvasive brain stimulation in Alzheimer's disease: systematic review and perspectives for the future. Exp. Gerontol. 46, 611–627. doi: 10.1016/J.EXGER.2011.04.001
Higgins, J. P. T., and Green, S. (2008). Cochrane Handbook for Systematic Reviews of Interventions. Chichester: Wiley-Blackwell.
Hsu, W.-Y., Ku, Y., Zanto, T. P., and Gazzaley, A. (2015). Effects of noninvasive brain stimulation on cognitive function in healthy aging and Alzheimer's disease: a systematic review and meta-analysis. Neurobiol. Aging 36, 2348–2359. doi: 10.1016/j.neurobiolaging.2015.04.016
Kadosh, R., Levy, N., O'Shea, J., Shea, N., and Savulescu, J. (2012). The neuroethics of non-invasive brain stimulation. Curr. Biol. 22, R108–R111. doi: 10.1016/j.cub.2012.01.013
Kaye, J. A., Swihart, T., Howieson, D., Dame, A., Moore, M. M., Karnos, T., et al. (1997). Volume loss of the hippocampus and temporal lobe in healthy elderly persons destined to develop dementia. Neurology 48, 1297–1304. doi: 10.1212/WNL.48.5.1297
Khedr, E. M., Gamal, N. F. El., El-Fetoh, N. A., Khalifa, H., Ahmed, E. M., Ali, A. M., et al. (2014). A double-blind randomized clinical trial on the efficacy of cortical direct current stimulation for the treatment of Alzheimer's disease. Front. Aging Neurosci. 6:275. doi: 10.3389/fnagi.2014.00275
Kuo, M.-F., and Nitsche, M. A. (2012). Effects of transcranial electrical stimulation on cognition. Clin. EEG Neurosci. 43, 192–199. doi: 10.1177/1550059412444975
Ladenbauer, J., Ladenbauer, J., Külzow, N., de Boor, R., Avramova, E., Grittner, U., et al. (2017). Promoting sleep oscillations and their functional coupling by transcranial stimulation enhances memory consolidation in mild cognitive impairment. J. Neurosci. 37, 7111–7124. doi: 10.1523/JNEUROSCI.0260-17.2017
Langa, K. M., and Levine, D. A. (2014). The diagnosis and management of mild cognitive impairment. JAMA 312, 2551–2561. doi: 10.1001/jama.2014.13806
Liberati, A., Altman, D. G., Tetzlaff, J., Mulrow, C., Gøtzsche, P. C., Ioannidis, J. P. A., et al. (2009). The PRISMA statement for reporting systematic reviews and meta-analyses of studies that evaluate health care interventions: explanation and elaboration. PLoS Med. 6:e1000100. doi: 10.1371/journal.pmed.1000100
McKhann, G., Drachman, D., Folstein, M., Katzman, R., Price, D., and Stadlan, E. M. (1984). Clinical diagnosis of Alzheimer's disease: report of the NINCDS-ADRDA work group under the auspices of department of health and human services task force on Alzheimer's disease. Neurology 34, 939–944.
Meinzer, M., Lindenberg, R., Phan, M. T., Ulm, L., Volk, C., and Flöel, A. (2015). Transcranial direct current stimulation in mild cognitive impairment: behavioral effects and neural mechanisms. Alzheimer Dement. 11, 1032–1040. doi: 10.1016/j.jalz.2014.07.159
Miniussi, C., Cappa, S. F., Cohen, L. G., Floel, A., Fregni, F., Nitsche, M. A., et al. (2008). Efficacy of repetitive transcranial magnetic stimulation/transcranial direct current stimulation in cognitive neurorehabilitation. Brain Stimul. 1, 326–336. doi: 10.1016/j.brs.2008.07.002
Miniussi, C., Harris, J. A., and Ruzzoli, M. (2013). Modelling non-invasive brain stimulation in cognitive neuroscience. Neurosci. Biobehav. Rev. 37, 1702–1712. doi: 10.1016/J.NEUBIOREV.2013.06.014
Murugaraja, V., Shivakumar, V., Sivakumar, P. T., Sinha, P., and Venkatasubramanian, G. (2017). Clinical utility and tolerability of transcranial direct current stimulation in mild cognitive impairment. Asian J. Psychiatr. 30, 135–140. doi: 10.1016/j.ajp.2017.09.001
Nguyen, J.-P., Suarez, A., Le Saout, E., Meignier, M., Nizard, J., and Lefaucheur, J.-P. (2018). Combining cognitive training and multi-site rTMS to improve cognitive functions in Alzheimer's disease. Brain Stimul. 11, 651–652. doi: 10.1016/j.brs.2018.02.013
Nitsche, M. A., Cohen, L. G., Wassermann, E. M., Priori, A., Lang, N., Antal, A., et al. (2008). Transcranial direct current stimulation: state of the art 2008. Brain Stimul. 1, 206–223. doi: 10.1016/j.brs.2008.06.004
Nitsche, M. A., Liebetanz, D., Antal, A., Lang, N., Tergau, F., and Paulus, W. (2003). Chapter 27 Modulation of cortical excitability by weak direct current stimulation – technical, safety and functional aspects. Suppl. Clin. Neurophysiol. 56, 255–276. doi: 10.1016/S1567-424X(09)70230-2
Penolazzi, B., Bergamaschi, S., Pastore, M., Villani, D., Sartori, G., and Mondini, S. (2015). Transcranial direct current stimulation and cognitive training in the rehabilitation of Alzheimer disease: A case study. Neuropsychol. Rehabil. 25, 799–817. doi: 10.1080/09602011.2014.977301
Petersen, R. C., Caracciolo, B., Brayne, C., Gauthier, S., Jelic, V., and Fratiglioni, L. (2014). Mild cognitive impairment: a concept in evolution. J. Intern. Med. 275, 214–228. doi: 10.1111/joim.12190
Petersen, R. C., Smith, G. E., Waring, S. C., Ivnik, R. J., Tangalos, E. G., and Kokmen, E. (1999). Mild cognitive impairment: clinical characterization and outcome. Arch. Neurol. 56, 303–308.
Portet, F., Ousset, P., Visser, P., and Frisoni, G. (2006). Mild cognitive impairment (MCI) in medical practice: a critical review of the concept and new diagnostic procedure. Report of the MCI Working Group of the European Consortium on Alzheimer's disease. J. Neurol. Neurosurg. Psychiatr. 77, 714–718. doi: 10.1136/jnnp.2005.085332
Prehn, K., and Flöel, A. (2015). Potentials and limits to enhance cognitive functions in healthy and pathological aging by tDCS. Front. Cell. Neurosci. 9:355. doi: 10.3389/fncel.2015.00355
Reinhart, R. M. G., Cosman, J. D., Fukuda, K., and Woodman, G. F. (2017). Using transcranial direct-current stimulation (tDCS) to understand cognitive processing. Atten. Percept. Psychophys. 79, 3–23. doi: 10.3758/s13414-016-1224-2
Sohlberg, M. M., and Mateer, C. A. (1989). Training use of compensatory memory books: a three stage behavioral approach. J. Clin. Exp. Neuropsychol. 11, 871–891. doi: 10.1080/01688638908400941
Suemoto, C. K., Apolinario, D., Nakamura-Palacios, E. M., Lopes, L., Leite, R. E. P., Sales, M. C., et al. (2014). Effects of a non-focal plasticity protocol on apathy in moderate Alzheimer's disease: a randomized, double-blind, sham-controlled trial. Brain Stimul. 7, 308–313. doi: 10.1016/j.brs.2013.10.003
Tatti, E., Rossi, S., Innocenti, I., Rossi, A., and Santarnecchi, E. (2016). Non-invasive brain stimulation of the aging brain: State of the art and future perspectives. Ageing Res. Rev. 29, 66–89. doi: 10.1016/J.ARR.2016.05.006
Tremblay, S., Lepage, J.-F., Latulipe-Loiselle, A., Fregni, F., Pascual-Leone, A., and Théoret, H. (2014). The uncertain outcome of prefrontal tDCS. Brain Stimul. 7, 773–83. doi: 10.1016/j.brs.2014.10.003
Woods, A. J., Antal, A., Bikson, M., Boggio, P. S., Brunoni, A. R., Celnik, P., et al. (2016). A technical guide to tDCS, and related non-invasive brain stimulation tools. Clin. Neurophysiol. 127, 1031–1048. doi: 10.1016/j.clinph.2015.11.012
Keywords: tDCS (transcranial direct-current stimulation), neuromodulation, MCI (mild cognitive impairment), dementia, cognitive rehabilitation, cognitive training, systematic review, meta-analysis
Citation: Cruz Gonzalez P, Fong KNK, Chung RCK, Ting K-H, Law LLF and Brown T (2018) Can Transcranial Direct-Current Stimulation Alone or Combined With Cognitive Training Be Used as a Clinical Intervention to Improve Cognitive Functioning in Persons With Mild Cognitive Impairment and Dementia? A Systematic Review and Meta-Analysis. Front. Hum. Neurosci. 12:416. doi: 10.3389/fnhum.2018.00416
Received: 17 May 2018; Accepted: 26 September 2018;
Published: 16 October 2018.
Edited by:
Keiichiro Nishida, Kansai Medical University, JapanReviewed by:
Wei-Peng Teo, Nanyang Technological University, SingaporeMasafumi Yoshimura, Kansai Medical University, Japan
Copyright © 2018 Cruz Gonzalez, Fong, Chung, Ting, Law and Brown. This is an open-access article distributed under the terms of the Creative Commons Attribution License (CC BY). The use, distribution or reproduction in other forums is permitted, provided the original author(s) and the copyright owner(s) are credited and that the original publication in this journal is cited, in accordance with accepted academic practice. No use, distribution or reproduction is permitted which does not comply with these terms.
*Correspondence: Kenneth N. K. Fong, cnNua2ZvbmdAcG9seXUuZWR1Lmhr