- 1Department of Neurological and Rehabilitation Sciences, IRCCS San Raffaele Pisana, Rome, Italy
- 2Department of Human Sciences and Promotion of the Quality of Life, San Raffaele University, Rome, Italy
Background: The limitation to the use of ElectroMyoGraphy (sEMG) in rehabilitation services is in contrast with its potential diagnostic capacity for rational planning and monitoring of the rehabilitation treatments, especially the overground Robot-Assisted Gait Training (o-RAGT).
Objective: To assess the barriers to the implementation of a sEMG-based assessment protocol in a clinical context for evaluating the effects of o-RAGT in subacute stroke patients.
Methods: An observational study was conducted in a rehabilitation hospital. The primary outcome was the success rate of the implementation of the sEMG-based assessment. The number of dropouts and the motivations have been registered. A detailed report on difficulties in implementing the sEMG protocol has been edited for each patient. The educational level and the working status of the staff have been registered. Each member of staff completed a brief survey indicating their level of knowledge of sEMG, using a five-point Likert scale.
Results: The sEMG protocol was carried out by a multidisciplinary team composed of Physical Therapists (PTs) and Biomedical Engineers (BEs). Indeed, the educational level and the expertise of the members of staff influenced the fulfillment of the implementation of the study. The PTs involved in the study did not receive any formal education on sEMG during their course of study. The low success rate (22.7%) of the protocol was caused by several factors which could be grouped in: patient-related barriers; cultural barriers; technical barriers; and administrative barriers.
Conclusions: Since a series of barriers limited the use of sEMG in the clinical rehabilitative environment, concrete actions are needed for disseminating sEMG in rehabilitation services. The sEMG assessment should be included in health systems regulations and specific education should be part of the rehabilitation professionals' curriculum.
Clinical Trial Registration: www.ClinicalTrials.gov, identifier: NCT03395717.
Introduction
In neurorehabilitation services, new devices have been widely used for both assessment and rehabilitation. The technology-based assessment includes instrumentation for medical imaging, for measuring electrophysiological signals (Electroencephalography, EEG; surface ElectroMyoGraphy, sEMG) and biomechanics (motion capture, inertial sensors). The technology for rehabilitation includes a wide range of devices such as: electrical stimulators, mechanical vibrators, robotics, virtual reality-based systems, etc. While the diffusion of technology for rehabilitation has been simplified by the great clinical interest in maximizing the outcomes of the rehabilitative treatment, the technology for assessing electrophysiological signals (EEG and sEMG) has been usually confined to research studies. As a consequence, the clinical acceptance of measuring tools, like sEMG, is low and such devices are not included in the usual clinical practice (1). On the other hand, devices like robots for rehabilitation have been rapidly accepted and integrated into rehabilitation services since they have been considered by clinicians as devices which could help to maximize patient recovery (2). Recent cutting-edge robots include the Wearable Powered Exoskeletons (WPEs) which allow a person to walk on hard and flat surfaces by moving the lower limbs with a pre-programmed physiological gait pattern (3–6). The success of WPEs in neurorehabilitation services is mainly due to their capacity to allow overground ambulation even in subjects who are not able to maintain the upright position (7–10), inducing a coordinated, multisensory motor control stimulation. Since these mechanisms are crucial for the restoration of motor control, the o-RAGT could be considered as a rehabilitation treatment which generates a more complex, controlled multisensory stimulation of the patient and which may modify the plasticity of neural connections through the experience of movement (11). While the literature on overground Robot–Assisted Gait Training (o-RAGT) with a WPE is rapidly growing up (12–18), studies which included technology-based assessment, like the sEMG, are restricted (19–23).
The limitation to the use of sEMG in rehabilitation services is in contrast with its potential diagnostic capacity for rational planning and monitoring of the rehabilitation treatments (24–27). A number of barriers limiting the clinical diffusion of sEMG have been recently highlighted by Feldner et al. (28). Cultural, technical and administrative barriers seem to be the principal limitation to sEMG in the clinical practice. The cultural barriers impact on the clinical acceptance of sEMG among Physical Therapists (PTs) and clinicians. In fact, low acceptance is mainly due to the insufficient knowledge to interpret the sEMG outcomes and thus to recognize the clinical relevance of sEMG. Technical barriers are often caused by the limited user confidence of some equipment which may make the acquisition and processing phases problematic. Administrative barriers include device costs and the time required to perform acquisition and processing, which are characteristics common to most biomedical technologies. The cultural and technical barriers mainly depend on the educational background of the clinical staff. Indeed, wide differences between countries exist, since the training programs of PTs and clinicians are very different, especially in approaching new technologies (29, 30). Moreover, to our best knowledge, studies on the barriers to sEMG in clinical practice lack. For these reasons, this paper focuses on introducing the sEMG in a clinical context where technology-based rehabilitation is promoted.
The aim of this work is to assess the barriers faced during the implementation of a sEMG protocol in a clinical study on o-RAGT in subacute stroke patients. The analysis of difficulties encountered during the protocol will allow us to discuss strategies and options which could help to reduce them.
Materials and Methods
An observational study was conducted in a rehabilitation hospital (IRCCS San Raffaele Pisana of Rome) in order to investigate the barriers in implementing a sEMG-based assessment protocol in subacute stroke patients who underwent o-RAGT.
Barriers Assessment
The primary outcome was the success rate of the implementation of the sEMG-based assessment, expressed as the percentage of successfully assessed patients relative to the total number of recruited ones. The number of dropouts and the motivations were registered. A detailed report on difficulties in implementing the sEMG protocol was edited for each patient.
The educational level and the working status of the staff involved in each phase of the study (patient recruitment; clinical assessment; sEMG acquisition; o-RAGT; sEMG analysis) were registered.
Each member of staff completed a brief survey indicating their level of knowledge of sEMG, using a five-point Likert scale: very poor (no knowledge about sEMG); poor (basic knowledge about muscle electrophysiology); fair (good knowledge of muscle electrophysiology and basic knowledge of detection/interpretation techniques); good (good knowledge of detection/interpretation techniques and ability to recognize artifacts, interference); excellent (good ability to detect, collect, process and interpret the signals).
The Clinical Study
A pilot clinical trial was carried out on a group of subacute stroke subjects who underwent o-RAGT with a WPE. This study was a subgroup analysis from a large multicenter clinical trial assessing the effects of o-RAGT (15, 16). The inclusion and exclusion criteria, the rehabilitation protocol, and the clinical assessment procedure were provided in the previous papers of the authors (15, 16). Ethical approval of the treatment and of the evaluation protocol was granted by the Ethics Committee of IRCCS San Raffaele Pisana of Rome (date: 18/11/2015; code number: 09/15). The study protocol was registered on ClinicalTrials.gov by the unique identifier number: NCT03395717, and all subjects gave informed written consent in accordance with the Declaration of Helsinki.
The sEMG Procedure
The subjects were screened at the beginning (T1) and at the end (T2: three weeks after T1) of the o-RAGT with both clinical measures and sEMG-based assessment. The sEMG-based assessment was carried out for assessing electromyographic activity during the following walking trials: at T1 and T2 during ecological overground gait at a self-selected speed along a 10-m walkway with assistance (e.g., crutches with or without antebrachial support, walker, tripod stick, ankle support orthoses, etc.); and during the first session of o-RAGT (o-RAGT1). Two gait tasks for each walking trial were collected. The total duration of the experimental session was about 2 h. An eight-channel wireless sEMG device (FREEEMG 1000—BTS Bioengineering, Milan, Italy) was used to acquire (sampled at 1 kHz, filtered at 8–500 Hz) the activity of the agonist/antagonist muscles of the distal and proximal compartment of lower limbs. The skin was abraded and cleaned with alcohol, then electrodes were placed on Tibialis Anterior (TA), Gastrocnemius Medialis (GM), Rectus Femoris (RF), and Biceps Femoris caput longus (BF) muscles of each leg according to the SENIAM guidelines (31). In order to establish the gait phases, kinematics data were measured using two electrogoniometers for knee joint measurements (BTS Bioengineering, Milan, Italy) and an inertial measurement unit (G-Sensor—BTS Bioengineering, Milan, Italy), placed on the spinous process of the fifth lumbar vertebra. The SMART Analyzer software (BTS Bioengineering, Milan, Italy) was employed for the synchronization of kinematic and sEMG signals for data pre-processing and exporting. Specifically, data from the inertial sensor were analyzed in comparison with electrogoniometers with the SMART Analyzer software, and the heel strike and toe-off gait cycle events were identified for all walking trials. The gait cycle was considered as the interval of time between heel-strikes of the same foot (i.e., the right foot). These temporal events were used for all subsequent sEMG analyses. The sEMG and temporal events were exported for further custom analysis in MATLAB (MATLAB R2019a, The MathWorks Inc., Natick, MA, USA).
The sEMG data were high-pass filtered (20 Hz, 6th-order Butterworth filter, bidirectional) and full-wave rectified. For standardization, the sEMG data were normalized to 100% of a gait cycle based on the temporal events (heel strikes and toe-offs) extracted from the kinematic data. The sEMG signals of each gait cycle were processed as follows: (1) the sEMG envelope was obtained using a moving average filter (window duration equal to 120 ms) and normalized at the maximum sEMG amplitude level; (2) the activation threshold identifying onset and offset status of muscle activity was detected as the 20% of minimum-maximum amplitude level distance (32), when kept for at least 50 ms. Subsequently, the following sEMG outcomes were extracted, considering five gait cycles: (i) the Bilateral Symmetry (BS) coefficient (33); (ii) the Co-Contraction (CC) coefficient (34); (iii) and the Root Mean Square (RMS) value (35). Details on the calculation of BS and CC coefficients are described in the Appendix.
Descriptive statistics were computed in order to appropriately explain the clinical and demographic characteristics of the sample. Data were represented for each recruited stroke subject. The sEMG outcomes were averaged from the two gait tasks for each walking trial and were used for subsequent analyses. The one-way ANalysis Of VAriance (ANOVA) was applied between T1 and T2 in order to test the treatment effect of o-RAGT on sEMG outcomes.
Results
The study was carried out by a multidisciplinary team composed of two Medical Doctors (MDs), four Physical Therapists (PTs), and two Biomedical Engineers (BEs). The MDs were Physical Medicine & Rehabilitation specialists. The sEMG acquisition and analysis were conducted by PTs and BEs. Table 1 depicts the characteristics of the members of staff who were involved in the study. PT1 and PT2 are the PTs who worked in the clinical department and administered the o-RAGT: they hold an M.Sc. in physical therapy and the patent to use the WPE in rehabilitation. The patient recruitment and clinical assessment were conducted by PT3 and PT4, both working in the research department and having an M.Sc. and Ph.D. educational level, respectively. The sEMG acquisition phase was executed by members of the research department only (two PTs and two BEs). BE1 and BE2 were in charge of the sEMG analysis and have an M.Sc. and Ph.D. educational level, respectively. The self-administered evaluation of the sEMG knowledge shows that the PTs were unfamiliar with sEMG assessment, while BEs considered themselves as experts.
Indeed, the educational level and the expertise of the members of staff influenced the accomplishment of the implementation of the study. The PTs involved in the study did not receive any formal education on sEMG during their course of study. Two of them (PT3 and PT4) were part of the research staff and studied the sEMG recording procedures (electrodes' positioning, basic use of the device for the sEMG acquisition) as a self-taught. However, no PTs had enough knowledge to recognize artifacts and interferences or process the signals. The BEs, on the other hand, were responsible for the entire sEMG procedure from signal acquisition to processing. The limitation of sufficient knowledge in sEMG among PTs was one of the reasons for the limited diffusion of electromyography in the rehabilitation hospital, and specifically in the clinical study on o-RAGT.
A total of 22 subacute stroke patients were recruited in the clinical study. Two patients dropped out due to medical issues not related to the training or to the assessment. The remaining 20 participants completed the o-RAGT without reporting any adverse event. Fourteen patients agreed to participate in the sEMG-based assessment procedure. However, six of them could not complete the 10-m-long ecological overground gait at T1 and therefore were excluded from the study. Therefore, we recorded sEMG during ecological overground gait at T1 and T2, and at o-RAGT1 of a sample composed of 8 patients (the demographic and clinical characteristics of each subject are depicted in Table 2). Data acquired during the o-RAGT1 were partially altered and it was not possible to reliably study the muscle activity. Thus, the sEMG outcomes from 3 patients (PAT03, PAT11, PAT14) were not available. Specifically, the experimental setup for data acquisition during o-RAGT1 was partially influenced by the presence of the WPE: the electrode application procedure did not always comply with the SENIAM guidelines (31), because of the cumbersome WPE braces and straps. Moreover, during movement, the placement of the electrodes was moderately affected by the relative movement between the subject and the WPE. In conclusion, the number of successfully assessed patients was 5 out of the 22 initially recruited ones, and the success rate of the implementation of the sEMG-based assessment was equal to 22.7%. Figure 1 shows the flowchart of the experimental procedure.
sEMG Outcomes
The visual assessment of sEMG patterns during walking reveals a vast heterogeneity of the data. The mean sEMG data (Figure 2) shows volitional muscle activations during gait at both T1 and T2 during ecological overground gait. Although such muscular activity is visible on both the affected and unaffected limbs, the level of activations are characterized by variations in amplitude and timing and do not consistently correlate with the activation timing of healthy gait (36).
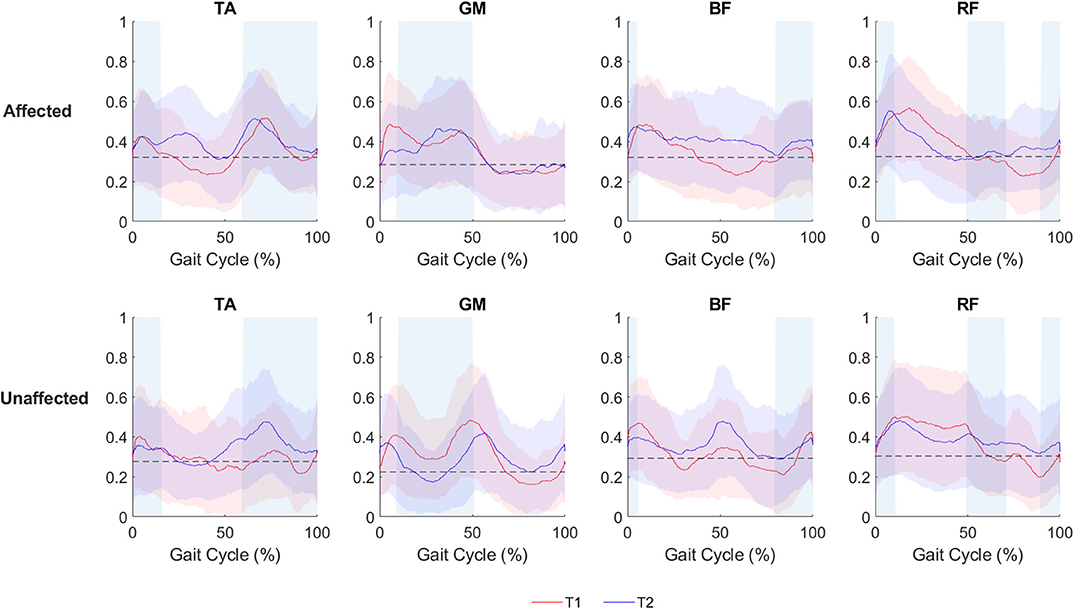
Figure 2. sEMG activation of the affected and the unaffected limb for all patients (N = 8), depicted as mean and standard deviation plot, during ecological overground gait. The red line (mean) and the red band (standard deviation) represent the sEMG envelopes (normalized with respect to the maximum sEMG amplitude level of each side) before o-RAGT (T1). The blue line (mean) and the blue band (standard deviation) represent the sEMG envelopes at the end of o-RAGT (T2). For each subject, five gait cycles have been considered. Shaded rectangular areas indicate when a muscle is active based on normative healthy adult gait, Perry and Burnfield (36).
A traditional amplitude analysis was conducted on the sEMG data, and the following sEMG outcomes were calculated: (i) the BS coefficient; (ii) the CC coefficient; (iii) and the RMS value (35). The results of the one-way ANOVA between T1 and T2 did not reveal any significant difference. The BS coefficient registered a relevant improvement between T1 and o-RAGT1 in a subset of patients (PAT02 at TA and GM; PAT06 at TA, GM, BF, RF; PAT09 at TA, GM, BF; PAT11 at TA; and PAT18 at TA and BF). The effects of the 15 sessions of o-RAGT improved the BS in TA (PAT02, PAT06, PAT09, PAT11, PAT20) and moderately in RF (PAT06, PAT20). The proximal muscles registered a decrease in CC between T1 and o-RAGT1 (PAT02, PAT06, PAT18, PAT20) and between T1 and T2 (PAT02, PAT11, PAT14, PAT18), while the distal muscles did not. In the future, an advanced analysis of sEMG (by means of a time-varying multi-muscle coactivation function) could help to investigate the o-RAGT effects, in terms of simultaneous coactivation of a group of muscles during gait (37–39). The RMS revealed differences between the same muscles of different limbs, although the one-way ANOVA did not reveal statistical significance. Data showed a mean increase of muscle activity at T2 of TA (affected and unaffected sides), GM (affected side), BF (affected and unaffected sides), and RF (unaffected sides), although the standard deviations were high. The o-RAGT1 registered an increase in the RMS of TA, GM, BF, and RF of the affected side.
Discussion
This paper focuses primarily on the difficulties encountered in the investigation of the o-RAGT technique by mean of sEMG, and secondarily on the results obtained in the o-RAGT study. This experience evidences a number of barriers limiting the implementation of the study on the effects of o-RAGT in terms of muscle activation. The low success rate (22.7%) of the protocol has been caused by several factors which can be grouped in: patient-related barriers; cultural barriers; technical barriers; and administrative barriers.
Patient-related barriers are mainly caused by low patient compliance. Except for two drop-outs caused by medical issues [no adverse events were evidenced during the o-RAGT as in (15, 16, 22)], from the 22 recruited subjects, 12 patients were excluded from the study for these reasons: (1) six patients did not agree to participate in the sEMG assessment due to tiredness; (2) six patients found the gait motor task at baseline too challenging and could not complete the sEMG assessment at T1. Both motivations depended on the subacute and complex phase of the disease. The acceptance of the sEMG assessment from patients could be improved introducing new strategies for increasing the perceived usefulness of sEMG (e.g., dedicating time to give the patient accurate and detailed information about the benefits of sEMG). Indeed, the typical impairment of stroke patients in the subacute phase should be considered in the definition of future sEMG-based protocols, and thus the analysis of basic motor tasks should be preferred.
Cultural barriers depend on the insufficient knowledge of sEMG among PTs. The role of PTs in the study was mainly as assistants of BEs during the sEMG acquisition phase. Specifically, PT3 and PT4 placed the electrodes and helped the patients to conduct the requested motor tasks. This situation is representative of the condition of PTs with respect to sEMG in Italy. In fact, the Italian academic curriculum in Physical Therapy does not include any courses on electromyography, and thus the professional figure of PT is usually focused on conventional therapy. Indeed, considering the dramatic introduction of new technologies in rehabilitation services, the role of PT should be drastically changed, and this change should start from the university curricula (40). While the bachelor's degree in PT could remain more oriented to the classical clinical role of PT, the master's degree in PT should include more technical courses. Specifically, academic courses on sEMG, EEG, gait analysis, and statistics should be added to the Italian Universities. Considering the sEMG, the PTs should be able to carry out the sEMG recording procedure (electrodes' positioning, sEMG acquisition) autonomously, using a commercial device. Moreover, the PTs should have enough knowledge to distinguish the “quality” of the acquired signals and to use commercial and user-friendly software for the basic sEMG analysis. Of course, it does not mean that the figure of BEs is not necessary for the rehabilitation hospital, but their role should be more oriented to the use of innovative prototypal sEMG devices and to the development of novel advanced signal processing techniques.
Technical barriers have been encountered during o-RAGT1. The sEMG signal was affected by the change of electrode location (41, 42), due to the presence of the WPE: the space between the limbs and the WPE did not allow to place the electrodes appropriately and the electrodes were partially moved by the exoskeleton during the movement of the patient. Thus, the quality of the sEMG signals of 4 patients during the o-RAGT1 was altered and the sEMG outcomes were unreliable. This barrier could be overcome in future studies by using high-density sEMG (43, 44) or making structural changes to the WPE.
Administrative barriers related to management and time-related issues had a negative impact on the dissemination of sEMG assessment in our clinical environment: a limited amount of time was available for the sEMG acquisition, because of the intensive schedule of rehabilitative treatments. These time-related barriers have been highlighted also by Feldner et al. (28) and by Swank et al. (21). A solution for increasing the diffusion of electromyography could be the inclusion of sEMG acquisition in the routine clinical practice for patient assessment (recognized by regional regulations for public health), thus dedicating a timeslot of the planned schedule to this procedure. Indeed, in this case, the clinical PTs should have an appropriate educational background on sEMG. Our results evidence that a multidisciplinary team is required to conduct the study because of the heterogeneity in technical skills: while the BEs, which were involved in both the sEMG acquisition and analysis, had a solid knowledge of sEMG, mainly learned during their course of study in higher education (M.Sc. and Ph.D.), the PTs did not receive any specific training on the topic during their course of study. In this context, a higher diffusion of a sEMG in an intensive rehabilitation hospital could be facilitated by the introduction of a specific education of PTs on sEMG. In our experience, while the background of clinical PTs on WPE is influenced by the need to have a patent to use the device in rehabilitation, the knowledge of sEMG is rarely supported by the Italian PT university curricula and by the need to use this technology in daily clinical practice. A solution to overcome the educational barriers could be the constitution of appropriate training of PTs on both the theory and the technical aspects of sEMG. An alternative solution could be the introduction of a new figure (i.e., the Clinical Technologist) as a healthcare professional who has the expertise to translate medical technology use into improved patient-specific procedures (45). However, in our opinion, the competence of rehabilitation professionals in the use of new technologies (i.e., sEMG) should be increased with the diffusion of specific training courses [like the one described by De la Fuente et al. (46)].
Conclusion
In conclusion, this paper offers an insight into the barriers limiting the use of sEMG during o-RAGT in subacute stroke patients. Certainly, since a series of barriers limited the application of sEMG in the clinical rehabilitative environment, concrete actions are needed for disseminating sEMG in rehabilitation services. The sEMG assessment should be included in health systems regulations and specific education should be part of the rehabilitation professionals' curriculum.
Data Availability Statement
The raw data supporting the conclusions of this article will be made available by the authors, without undue reservation.
Ethics Statement
The studies involving human participants were reviewed and approved by IRCCS San Raffaele Pisana, Via della Pisana, 235, 00163 Rome, Italy. The patients/participants provided their written informed consent to participate in this study.
Author Contributions
MG, FI, and MF have made substantial contributions to conception and design. SP, DGal, and DGab participated in the enrollment phase and carried out the o-RAGT treatment. SP, MG, and PR carried out the clinical and sEMG assessments. MO and PR designed the algorithm for sEMG data analysis. LP, PR, MO, and AG participated in the study design and coordination, and statistical analysis. SP and FI participated in the manuscript revisions. MF and FI gave the final approval of the version. All authors contributed to the article and approved the submitted version.
Funding
This project was partially funded by The Ministry of Health (Ricerca corrente).
Conflict of Interest
The authors declare that the research was conducted in the absence of any commercial or financial relationships that could be construed as a potential conflict of interest.
Supplementary Material
The Supplementary Material for this article can be found online at: https://www.frontiersin.org/articles/10.3389/fneur.2020.564067/full#supplementary-material
References
1. Hogrel J-Y. Clinical applications of surface electromyography in neuromuscular disorders. Clin. Neurophysiol. (2005) 35:59–71. doi: 10.1016/j.neucli.2005.03.001
2. Mehrholz J, Thomas S, Werner C, Kugler J, Pohl M, Elsner B. Electromechanical-assisted training for walking after stroke. Cochrane Database Syst. Rev. (2017) 10:CD006185. doi: 10.1002/14651858.CD006185.pub4
3. Yan T, Cempini, M, Oddo CM, Vitiello N. Review of assistive strategies in powered lower-limb orthoses and exoskeletons. Rob. Auton. Syst. (2015) 64:120–36. doi: 10.1016/j.robot.2014.09.032
4. Barbeau, H. Locomotor training in neurorehabilitation: emerging rehabilitation concepts. Neurorehabil. Neural Repair. (2003) 17:3–11. doi: 10.1177/0888439002250442
5. Morone G, Paolucci S, Cherubini A, De Angelis D, Venturiero V, Coiro P, et al. Robot-assisted gait training for stroke patients: current state of the art and perspectives of robotics. Neuropsychiatr. Dis. Treat. (2017) 13:1303–11. doi: 10.2147/NDT.S114102
6. Masiero S, Poli P, Rosati G, Zanotto D, Iosa M, Paolucci S, et al. The value of robotic systems in stroke rehabilitation. Expert Rev. Med. Devices. (2014) 11:187–98. doi: 10.1586/17434440.2014.882766
7. Chen G, Chan CK, Guo Z, Yu H. A review of lower extremity assistive robotic exoskeletons in rehabilitation therapy. Crit. Rev. Biomed. Eng. (2013) 41:343–63. doi: 10.1615/CritRevBiomedEng.2014010453
8. Louie DR, Eng JJ. Powered robotic exoskeletons in post-stroke rehabilitation of gait: a scoping review. J. Neuroengineering Rehabil. (2016) 13:53. doi: 10.1186/s12984-016-0162-5
9. Molteni F, Gasperini G, Cannaviello G, Guanziroli E. Exoskeleton and end-effector robots for upper and lower limbs rehabilitation: narrative review. PM&R. (2018) 10:S174–88. doi: 10.1016/j.pmrj.2018.06.005
10. Tedla JS, Dixit S, Gular K, Abohashrh M. Robotic-assisted gait training effect on function and gait speed in subacute and chronic stroke population: a systematic review and meta-analysis of randomized controlled trials. Eur. Neurol. (2019) 81:1–9. doi: 10.1159/000500747
11. Peters S, Handy TC, Lakhani B, Boyd LA, Garland SJ. Motor and visuospatial attention and motor planning after stroke: considerations for the rehabilitation of standing balance and gait. Phys. Ther. (2015) 95:1423–32. doi: 10.2522/ptj.20140492
12. Buesing C, Fisch G, O'Donnell M, Shahidi I, Thomas L, Mummidisetty CK, et al. Effects of a wearable exoskeleton stride management assist system (SMA®) on spatiotemporal gait characteristics in individuals after stroke: a randomized controlled trial. J. NeuroEngineering Rehabil. (2015) 20:12–69. doi: 10.1186/s12984-015-0062-0
13. Li L, Ding L, Chen N, Mao Y, Huang D, Li L. Improved walking ability with wearable robot-assisted training in patients suffering chronic stroke. Biomed. Mater. Eng. (2015) 26:S329–40. doi: 10.3233/BME-151320
14. Gasperini G, Gaffuri M, Guanziroli E, Goffredo M, Pournajaf S, Galafate D, et al. Recovery of gait function with a wearable powered exoskeleton in sub-acute stroke patients using SEMG for fine tuning: preliminary results. Ann. Phys. Rehabil. Med. (2018) 61:e93. doi: 10.1016/j.rehab.2018.05.198
15. Goffredo M, Guanziroli E, Pournajaf S, Gaffuri M, Gasperini G, Filoni S, et al. Overground wearable powered exoskeleton for gait training in subacute stroke subjects: clinical and gait assessments. Eur. J. Phys. Rehabil. Med. (2019) 55:710–21. doi: 10.23736/S1973-9087.19.05574-6
16. Goffredo M, Iacovelli C, Russo E, Pournajaf S, Di Blasi C, Galafate D, et al. Stroke gait rehabilitation: a comparison of end-effector, overground exoskeleton, and conventional gait training. Appl. Sci. (2019) 9:2627. doi: 10.3390/app9132627
17. Pournajaf S, Goffredo M, Agosti M, Massucci M, Ferro S, Franceschini M. Community ambulation of stroke survivors at 6 months follow-up: an observational study on sociodemographic and sub-acute clinical indicators. Eur. J. Phys. Rehabil. Med. (2019) 55:433–41. doi: 10.23736/S1973-9087.18.05489-8
18. Lee HJ, Lee SH, Seo K, Lee M, Chang WH, Choi BO, et al. Training for walking efficiency with a wearable hip-assist robot in patients with stroke: a pilot randomized controlled trial. Stroke. (2019) 50:3545–52. doi: 10.1161/STROKEAHA.119.025950
19. Sylos-Labini F, La Scaleia V, d'Avella A, Pisotta I, Tamburella F, Scivoletto G, et al. EMG patterns during assisted walking in the exoskeleton. Front. Hum. Neurosci. (2014) 8:423. doi: 10.3389/fnhum.2014.00423
20. Androwis GJ, Pilkar R, Ramanujam A, Nolan KJ. Electromyography assessment during gait in a robotic exoskeleton for acute stroke. Front. Neurol. (2018) 9:630. doi: 10.3389/fneur.2018.00630
21. Swank C, Sikka S, Driver S, Bennett M, Callender L. Feasibility of integrating robotic exoskeleton gait training in inpatient rehabilitation. Disabil. Rehabil. (2020) 15:409–17. doi: 10.1080/17483107.2019.1587014
22. Calabrò RS, Naro A, Russo M, Bramanti P, Carioti L, Balletta T, et al. Shaping neuroplasticity by using powered exoskeletons in patients with stroke: a randomized clinical trial. J. NeuroEngineering Rehabil. (2018) 15:35. doi: 10.1186/s12984-018-0377-8
23. Tan CK, Kadone H, Watanabe H, Marushima A, Yamazaki M, Sankai Y, et al. Lateral symmetry of synergies in lower limb muscles of acute post-stroke patients after robotic intervention. Front. Neurosci. (2018) 12:276. doi: 10.3389/fnins.2018.00276
24. Sutherland DH. The evolution of clinical gait analysis part l: kinesiological EMG. Gait Posture. (2001) 14:61–70. doi: 10.1016/S0966-6362(01)00100-X
25. Zwarts MJ, Stegeman DF. Multichannel surface EMG: basic aspects and clinical utility. Muscle Nerve. (2003) 28:1–17. doi: 10.1002/mus.10358
26. World Health Organization. Neurological Disorders: Public Health Challenges. Geneva: World Health Organization (2006).
27. Frigo C, Crenna P. Multichannel SEMG in clinical gait analysis: a review and state-of-the-art. Clin. Biomech. (2009) 24:236–45. doi: 10.1016/j.clinbiomech.2008.07.012
28. Feldner HA, Howell D, Kelly VE, McCoy SW, Steele KM. “Look, your muscles are firing!”: a qualitative study of clinician perspectives on the use of surface electromyography in neurorehabilitation. Arch. Phys. Med. Rehabil. (2019) 100:663–75. doi: 10.1016/j.apmr.2018.09.120
29. Swinkels RAHM, Van Peppen RPPS, Wittink H, Custers JWH, Beurskens AJHM. Current use and barriers and facilitators for implementation of standardised measures in physical therapy in the Netherlands. BMC Musculoskelet Disord. (2011) 12:106. doi: 10.1186/1471-2474-12-106
30. Braun T, Rieckmann A, Weber F, Grüneberg C. Current use of measurement instruments by physiotherapists working in Germany: a cross-sectional online survey. BMC Health Serv. Res. (2018) 18:1–16. doi: 10.1186/s12913-018-3563-2
31. Hermens HJ, Freriks B, Merletti R, Stegeman D, Blok J, Rau G, et al. European recommendations for surface electromyography. Roessingh Res. Dev. (1999) 8:13–54.
32. Paoloni M, Mangone M, Scettri P, Procaccianti R, Cometa A, Santilli V. Segmental muscle vibration improves walking in chronic stroke patients with foot drop: a randomized controlled trial. Neurorehabil. Neural Repair. (2010) 24:254–62. doi: 10.1177/1545968309349940
33. Wren TA, Do KP, Rethlefsen SA, Healy B. Cross-correlation as a method for comparing dynamic electromyography signals during gait. J. Biomech. (2006) 39:2714–18. doi: 10.1016/j.jbiomech.2005.09.006
34. Di Nardo F, Mengarelli A, Burattini L, Maranesi E, Agostini V, et al. Normative EMG patterns of ankle muscle co-contractions in school-age children during gait. Gait Posture. (2016) 46:161–66. doi: 10.1016/j.gaitpost.2016.03.002
35. Bailey CA, Corona F, Pilloni G, Porta M, Fastame MC, Hitchcott PK, et al. Sex-dependent and sex-independent muscle activation patterns in adult gait as a function of age. Exp. Gerontol. (2018) 110:1–8. doi: 10.1016/j.exger.2018.05.005
36. Perry J, Burnfield JM. Gait Analysis: Normal and Pathological Function. 2nd ed. Thorofare, NJ: Slack Inc. (2010).
37. Fiori L, Ranavolo A, Varrecchia T, Tatarelli A, Conte C, Draicchio F, et al. Impairment of global lower limb muscle coactivation during walking in cerebellar ataxias. Cerebellum. (2020) 19:583–96. doi: 10.1007/s12311-020-01142-6
38. Tatarelli A, Serrao M, Varrecchia T, Fiori L, Draicchio F, Silvetti A, et al. A. global muscle coactivation of the sound limb in gait of people with transfemoral and transtibial amputation. Sensors. (2020) 20:2543. doi: 10.3390/s20092543
39. Varrecchia T, Rinaldi M, Serrao M, Draicchio F, Conte C, Conforto S, et al. Global lower limb muscle coactivation during walking at different speeds: relationship between spatio-temporal, kinematic, kinetic, and energetic parameters. J. Electromyogr. kinesiol. (2018) 43:148–57. doi: 10.1016/j.jelekin.2018.09.012
40. Campanini I, Disselhorst-Klug C, Rymer WZ, Merletti R. Surface EMG in clinical assessment and neurorehabilitation: barriers limiting its use. Front. Neurol. (2020) 11:934. doi: 10.3389/fneur.2020.00934
41. Campanini I, Merlo A, Degola P, Merletti R, Vezzosi G, Farina D. Effect of electrode location on EMG signal envelope in leg muscles during gait. J. Electromyogr. Kinesiol. (2007) 17:515–26. doi: 10.1016/j.jelekin.2006.06.001
42. Mesin L, Merletti R, Rainoldi A. Surface EMG: the issue of electrode location. J. Electromyogr. Kinesiol. (2009) 19:719–26. doi: 10.1016/j.jelekin.2008.07.006
43. Besomi M, Hodges PW, Van Dieën J, Carson RG, Clancy EA, Disselhorst-Klug C, et al. Consensus for experimental design in electromyography (CEDE) project: electrode selection matrix. J. Electromyogr. Kinesiol. (2019) 48:128–44. doi: 10.1016/j.jelekin.2019.07.008
44. Merletti R, Muceli S. Tutorial. Surface EMG detection in space and time: best practices. J. Electromyogr. Kinesiol. (2019) 49:102363. doi: 10.1016/j.jelekin.2019.102363
45. Groenier M, Pieters JM, Miedema HA. Technical medicine: designing medical technological solutions for improved health care. Med. Sci. Educator. (2017) 27:621–31. doi: 10.1007/s40670-017-0443-z
Keywords: surface electromyography, overground robot-assisted gait rehabilitation, stroke, clinical applications, sEMG barriers
Citation: Goffredo M, Infarinato F, Pournajaf S, Romano P, Ottaviani M, Pellicciari L, Galafate D, Gabbani D, Gison A and Franceschini M (2020) Barriers to sEMG Assessment During Overground Robot-Assisted Gait Training in Subacute Stroke Patients. Front. Neurol. 11:564067. doi: 10.3389/fneur.2020.564067
Received: 20 May 2020; Accepted: 15 September 2020;
Published: 19 October 2020.
Edited by:
Roberto Merletti, Politecnico di Torino, ItalyReviewed by:
Carlotte Kiekens, Montecatone Rehabilitation Institute, ItalyAlberto Ranavolo, National Institute for Insurance Against Accidents at Work (INAIL), Italy
Copyright © 2020 Goffredo, Infarinato, Pournajaf, Romano, Ottaviani, Pellicciari, Galafate, Gabbani, Gison and Franceschini. This is an open-access article distributed under the terms of the Creative Commons Attribution License (CC BY). The use, distribution or reproduction in other forums is permitted, provided the original author(s) and the copyright owner(s) are credited and that the original publication in this journal is cited, in accordance with accepted academic practice. No use, distribution or reproduction is permitted which does not comply with these terms.
*Correspondence: Michela Goffredo, michela.goffredo@sanraffaele.it